NCBI Bookshelf. A service of the National Library of Medicine, National Institutes of Health.
Varki A, Cummings RD, Esko JD, et al., editors. Essentials of Glycobiology [Internet]. 3rd edition. Cold Spring Harbor (NY): Cold Spring Harbor Laboratory Press; 2015-2017. doi: 10.1101/glycobiology.3e.036
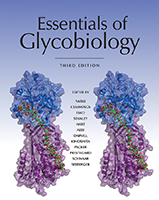
Essentials of Glycobiology [Internet]. 3rd edition.
Show detailsGalectins are among the most widely expressed class of lectins in all organisms. They typically bind β-galactose-containing glycoconjugates and share primary structural homology in their carbohydrate-recognition domains (CRDs). Galectins have many biological functions, including roles in development, regulation of immune cell activities, and microbial recognition as part of the innate immune system. This chapter describes the diversity of the galectin family and presents an overview of what is known about their biosynthesis, secretion, and biological roles.
HISTORICAL BACKGROUND ON DISCOVERY OF GALECTINS
Following the discovery of the Ashwell–Morell asialoglycoprotein receptor in the liver, many investigators sought other such receptors by affinity chromatography with immobilized asialoglycoproteins. In 1975, a novel lectin of ∼15 kDa was isolated from electric organs of the electric eel, and named “electrolectin.” This noncovalently linked homodimer displayed hemagglutinating activity toward trypsinized rabbit erythrocytes that was inhibitable by β-galactosides; inclusion of β-mercaptoethanol in the isolation buffers was required to maintain the binding activity. Soon thereafter, in 1976, similar β-galactoside-binding lectins were isolated from chicken muscle and from extracts of calf heart and lung (∼15 kDa; now designated as galectin-1). These proteins were initially referred to as S-type lectins to denote their sulfhydryl dependency, presence of free cysteine residues, and solubility, along with shared primary sequence. Interestingly, and unlike most galectins, electrolectin does not contain free Cys residues, but a key Trp in the binding site of its CRD can be oxidized, causing loss of activity. In the early 1980s, a 35 kDa protein termed CBP35 that also bound to β-galactosides was identified in mouse fibroblasts. The same protein was studied by other groups under the names IgE-binding protein, L-29, and L-31 and is currently known as galectin-3.
The nomenclature for galectins was systematized in 1994 and the first galectin type found (∼15 kDa, discussed above) was renamed galectin-1. All other members of this family were numbered consecutively by order of discovery. Multiple distinct galectins are usually expressed in a single species (up to 15 in the mouse). A list of the vertebrate galectins is shown in Figure 36.1A.
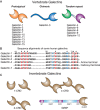
FIGURE 36.1.
Different types of galectins in vertebrates and invertebrates and their organization and sequences. (A) Fifteen types of vertebrate galectins are known. (B) Examples of sequence alignments of some representative human galectins are shown. The amino acid (more...)
THE GALECTIN FAMILY
In 1982, a shared amino acid sequence motif was identified in the CRD of all galectins. The canonical CRD of galectins has ∼130 amino acids, although only a small number of residues within the CRD directly contact glycan ligands. A comparison of more than 100 galectin sequences from many different sources reveals that eight residues, which have been shown to be involved in glycan binding by X-ray crystallographic analyses, are mostly invariant. In addition, another dozen residues appear to be highly conserved. Part of the conserved galectin sequence motif is shown in Figure 36.1b, along with a comparison of several human galectins.
Based on their CRD number and organization, galectins have been classified into three major groups, termed prototypical galectins (galectin-1, -2, -7, -10, -11, -13, -14, and -15) that contain a single CRD that may associate as homodimers, chimera-type galectins (galectin-3), which are characterized by having a single CRD and an amino-terminal polypeptide “tail” rich in proline, glycine, and tyrosine residues, through which it can form oligmers, and tandem-repeat galectins (galectin-4, -8, -9, and -12, which comprise proteins that carry two CRDs connected by a peptide linker that can range from five to >50 amino acids in length. Galectin transcripts may be differentially spliced to generate multiple isoforms. Invertebrates have galectins with one, two, or four tandemly arrayed CRDs.
Some galectins appear to be species specific, such as galectin-5 (prototype) and galectin-6 (tandem repeat) that are found in rodents but not humans, and galectin-11 (ovagal11; prototype) that has only been reported in sheep, and galectin-15 has only been found in sheep and goat. The amino-terminal domain of galectin-12 has significant homologies with other galectins, whereas the carboxy-terminal domain displays greater divergence from other galectins.
Several proteins with sequence homology with galectins, which do not bind typical β-galactosides, have been described as galectin-related proteins (GRPs). For example, galectin-10, also known as the Charcot–Leyden crystal protein, is highly abundant and is present in eosinophil granules and preferentially binds to β-mannosides. Several GRPs, including GRIFIN (galectin-related interfiber protein), PP-13, and PPL-13 that lack glycan-binding activity, are related to galectin-10. It is noteworthy that the GRIFIN homolog in the zebrafish (Danio rerio DrGRIFIN) is a functional β-galactoside-binding protein and, like its mammalian counterpart, is also highly expressed in the eye lens.
TAXONOMIC DISTRIBUTION AND EVOLUTION OF GALECTINS
Hallmarks of the galectin family are the extensive taxonomic distribution of its members, together with the evolutionary conservation of their primary structures, gene organization, and structure of the CRD. The early emergence and structural conservation of the galectin family in eukaryotic evolution is revealed in the fungus Coprinopsis cinerea in the sponge Geodia cydonium, and in a protein that displays the galectin fold in the protozoan parasite Toxoplasma gondii. Galectin-like sequences have also been identified in plants (Arabidopsis thaliana) and some viruses that infect pigs and fish, such as the porcine adenovirus and lymphocystis disease virus. However, galectin-like proteins or sequence motifs in the latter should be interpreted with caution as it could result from horizontal gene transfer.
The evolution of the galectin family has been inferred from the rigorous analysis of primary structures, gene organization, and taxonomic distribution of family members identified in a variety of organisms, including fungi, invertebrates, and vertebrates. Among the arthropods and nematodes, galectins are abundantly present in Drosophila melanogaster (six candidate genes) and Caenorhabditis elegans (26 candidate genes), respectively. At least 14 of these candidate galectin genes in C. elegans produce transcripts encoding proteins. Generally, the primary structures and domain organizations of galectins from invertebrates do not fit the canonical features of vertebrate galectins described above (Figure 36.1C).
Galectins are ubiquitous in vertebrates. In fish, galectins have been identified and characterized in numerous species. The zebrafish (D. rerio) genome has ortholog genes for all three galectin types (proto-, chimera-, and tandem-repeat) identified in mammals. Among amphibians, several galectin subtypes have been found in various tissues, mucus, and eggs from salamanders (the axolotl Ambystoma mexicanum), toads (Bufo arenarum), and frogs (Rana catesbeiana and Xenopus laevis).
The evolution of galectins along the vertebrate taxa leading to the mammals has been rationalized as the duplication of an early single-CRD galectin gene that would have led to a bi-CRD galectin gene, in which the amino- and carboxy-terminal CRDs subsequently diverged into two different subtypes, defined by an exon–intron structure (F4-CRD and F3-CRD) (F refers to the β-sandwich strand structure discussed below). All vertebrate single-CRD galectins belong to either the F3- (e.g., galectin-1, -2, -3, -5) or F4- (e.g., galectin-7, -10, -13, -14) subtype, whereas the mammalian tandem-repeat galectins such as galectin-4, -6, -8, -9, and -12 contain both F4 and F3 subtypes. However, how the multiple CRD galectins from invertebrates relate to the vertebrate tandem-repeat galectins remains to be fully understood, but a preliminary phylogenetic analysis of the oyster galectin CvGal1 revealed that the individual CRDs cluster with the mammalian single-CRD galectins rather than with the tandem-repeat galectins, suggesting that the CvGal1 gene is the product of two consecutive gene duplications of a single-CRD galectin gene ancestral to the early invertebrate taxa that was conserved along the chordate lineages. The striking structural and evolutionary conservation of the CRD architecture and arrangements supports the key roles of galectins in essential biological functions related to β-galactoside recognition.
STRUCTURE OF GALECTINS
The crystal structures of several galectins in complexes with glycan ligands are known, including galectins-1, -2, and -7, the carboxy-terminal domain of galectin-3, and individual domains of galectin-4, -8, and -9. In all cases, the galectin CRD is comprised of five- and six-stranded antiparallel β sheets arranged in a β-sandwich or jelly-roll configuration that lacks an α-helix (Figure 36.2A). In the dimeric proteins, such as galectin-1, -2, and -7, the subunits are related by a twofold rotational axis perpendicular to the plane of the β sheets. The glycan-binding sites in the CRD are located at opposite ends of the dimer, although the orientation of the subunits in the galectin-7 dimer is different from the other canonical galectin dimers. The compactly arranged structure of the CRD partly explains the protease resistance of the galectin CRD and the high degree of conservation and requirement for the 130 amino acids in the CRD.
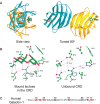
FIGURE 36.2.
(A) Ribbon diagram of the crystal structure of human galectin-1 complexed with lactose. The homodimer is shown with each monomer colored differently and orthogonal views are presented. The subunit interface is based on interactions between the carboxy- (more...)
The galectin-1 CRD displays highly specific interactions with Gal and GlcNAc residues in typical glycans. Interactions with glycans are generally through hydrogen bonding, electrostatic interactions, and van der Waals interactions through ring stacking with Gal and the highly conserved Trp residue (Figures 36.2B,C). In general, the open-ended structure of the glycan-binding site is predicted to allow access to extended Gal-containing glycans, such as the poly-N-acetyllactosamines and blood group–related structures (Chapter 14).
The binding preference of invertebrate galectins for blood group glycans can be illustrated by a unique structural feature of the binding site of the C. elegans and oyster galectins. They show a significantly shorter loop 4 that accommodates the 2´-fucosyl moiety that is a common feature of both the A and B blood groups (Figures 36.3A,B). The α(1-3)-linked GalNAc or Gal moieties of the A and B blood group tetrasaccharides, respectively, are recognized by the -NH at position 5 of the conserved Trp, and a hydrophobic pocket at the external side of the β3-strand (amino acids 30–38) recognizes the methyl of the -NAc group in α(1-3)GalNAc of the A1/2 antigens.
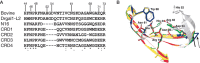
FIGURE 36.3.
Structural aspects of galectins from mammals and invertebrates. (A) Sequence alignment of the carbohydrate-recognition domain (CRD) regions from bovine galectin-1, zebrafish Drgal1-L2, C. elegans N16, and the CRDs 1–4 of CvGal1. (B) Homology modeling (more...)
There are several known and predicted subsites on galectins near the carbohydrate-binding site that could serve to enhance affinity for more extended glycans. Some of these subsites have been identified by cocrystal structural analysis with glycans and by glycan-binding studies. The crystal structure of bovine galectin-1 was derived in a complex with a biantennary N-glycan containing two terminal β-Gal residues. The N-glycan is bridged between two galectin-1 dimers, thus effectively creating a crystal latticework. This type of crystal latticework may be unique among vertebrate galectins and may be critical for their signaling and adhesive functions (Figures 36.4 and 36.5).
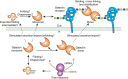
FIGURE 36.4.
Possible biosynthetic routes for galectins in animal cells, using galectin-1 as an example. The mRNA for the protein is translated on free polysomes in the cytoplasm and the newly synthesized protein there is capable of binding glycan ligands or interacting (more...)
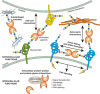
FIGURE 36.5.
Functional interactions of galectins with cell-surface glycoconjugates and to extracellular glycoconjugates leads to cell adhesion and cell signaling. Interactions of galectins with intracellular ligands may contribute to regulating intracellular pathways. (more...)
GLYCAN LIGANDS FOR GALECTINS
The interactions of galectins with glycans are complex and several factors contribute to high-affinity binding, including their natural multivalency and oligomeric state, as well as the multivalency of their natural ligands. Although most galectins bind weakly to simple β-galactosides, such as disaccharides or trisaccharides (Kd in the high micromolar to low millimolar range), they typically bind much stronger to natural glycoconjugate ligands (apparent Kd in the submicromolar range). Studies utilizing large libraries of glycans reveal that each galectin CRD recognizes different glycan ligands and shows highest affinity binding to different structures. For example, galectin-3 binds tightly to glycans with repeating [-3Galβ1-4GlcNAcβ-]n or poly-N-acetyllactosamine sequences containing 3-4 repeating units, regardless of the presence of terminal β-Gal residue, and binding is further enhanced if the penultimate β-Gal residues are substituted with Galα1-3, GalNAcα1-3, or Fucα1-2 residues. In contrast, human galectin-1 binds well only to glycans with terminal β-Gal residues, and does not bind blood group antigens. Galectin-8 has two CRDs within its single polypeptide and the two CRDs bind different glycans. For example, the amino-terminal CRD of human galectin-8 binds α2-3-sialylated glycans with high affinity (Kd ∼ 50 nm), whereas the carboxy-terminal CRD binds to the blood group A determinant on a LacNAc core, and does not bind sialylated glycans.
Cocrystallization of galectins with simple β-Gal-containing disaccharides has revealed that many galectins have H-bonds to the C4 and C6 hydroxyl of Gal and the C3 hydroxyl of GlcNAc (Figure 36.3). The binding sites of galectins may be viewed as containing several subsites: one for Gal, another for GlcNAc, and other subsites that may be filled by other sugars and the aglycone moiety, e.g., protein or lipid. Thus, other sugar residues linked to the disaccharide Gal-GlcNAc can enhance or decrease binding affinity, depending on the galectin. Some galectins from fungi, invertebrates, and mammals (such as galectin-4 and -8) preferentially bind selected ABO(H) blood group glycans as discussed above.
Galectins bind selectively to only some cell-surface and extracellular matrix ligands at low lectin concentrations but at higher concentrations they more often show broad binding to many different glycoproteins. However, the precise physiological ligands for each galectin and the physiological concentrations of galectins at sites of interactions are not well understood.
Galectins can also bind to exogenous ligands, such as exposed glycans on the surface of viruses, bacteria, fungi, and parasites, displaying a remarkable diversity of recognition. For example, galectin-1 can bind to complex type N-glycans on the HIV-1 gp120 envelope glycoprotein and to Trichomonas vaginalis lipophosphoglycan, whereas galectin-3 recognizes both terminal and internal N-acetyllactosamine units in lipopolysaccharides from meningococcus (Neisseria meningitidis), LacdiNAc from Schistosoma mansoni, and oligomannans from Candida albicans. The lipophosphoglycan from Leishmania major is recognized by both galectin-3 and -9, whereas galectin-4 recognizes Escherichia coli strains that display blood group B glycans.
BIOSYNTHESIS AND EXPORT OF GALECTINS
All members of the galectin family lack a classical signal sequence or membrane-anchoring domains, and are synthesized on free polysomes in the cytoplasm and accumulate there before secretion. Galectins are unusual among all types of animal lectins in that they can be found in the nucleus, cytosol, outer plasma membrane, and extracellular matrix. One rather common modification of galectins in animal cells is blockage of the amino terminus, although galectin-3 has been shown to also have two serine residues in its unique amino-terminal region that can be phosphorylated. Newly synthesized galectins isolated directly from the cytosol of cells are functional in binding β-galactosides.
Interestingly, there is compelling evidence for galectins having non-glycan-binding partners in the cytosol. The complexity of galectin biosynthesis, secretion, and oligomerization is illustrated schematically for galectin-1 in Figure 36.4. Curiously, the export of galectins from cells does not involve direct movement through the secretory pathway. It appears the secretion and availability of glycan ligands in the cells generating the galectin may also regulate export, activity, and stability of galectins.
BIOLOGICAL ROLES OF GALECTINS
Members of the galectin family display a striking functional diversification that ranges from key roles in early development, tissue regeneration, and cancer, to immune homeostasis and recognition/effector functions against potential pathogens. By binding to endogenous glycans, galectins can contribute to cell–cell and cell–matrix interactions, and galectin signaling at the cell surface can also modulate cellular functions. In addition, intracellular galectins may, as in the case of galectins-3, regulate cellular activities and may contribute to some fundamental processes such as pre-mRNA splicing (Figure 36.5). The galectin-mediated recognition of glycans on the surface of potential pathogens and parasites discovered in the past few years has revealed their role(s) as pattern recognition receptors and effector factors in innate immune functions. Much information about the biological functions of galectins has been revealed largely through using galectin-deficient mouse models. Such studies, which have been performed primarily in mice deficient in galectin-1, -3, -7, and -9, have revealed roles of galectins in a wide range of physiological pathways (Chapter 41).
Roles of Galectins in Development and Tissue Regeneration
Studies in murine and zebrafish models revealed that during early embryonic stages, galectin-1 and -3 are expressed in the notochord and play subtle, albeit key roles in notochord development, somitogenesis, and development of muscle tissue and the central nervous system. Lack of galectin-3 in knockout mice is associated with several subtle and apparently unrelated phenotypic changes, whereas lack of galectin-1 in mice is associated with a different set of phenotypic changes, including decreased sensitivity to noxious thermal stimuli, altered primary afferent neural anatomy, and aberrant topography of olfactory axons. In addition, expression of galectin-3 at the onset of chondrification suggests its role in bone development. Recent studies have unraveled the critical role of galectin-1 in angiogenesis, as the basis for resistance of certain tumors to otherwise effective anti-VEGF (vascular endothelial growth factor)-targeted therapy. It should also be noted that some galectins are involved in regeneration of injured tissue. For example, on photoinduced retinal damage in the zebrafish, galectin-1 is one of the first proteins secreted by stem cells in the Müller glia, and is responsible for rod regeneration. Galectin-7 is important in the maintenance of epidermal homeostasis and skin repair.
Roles of Galectins in Apoptosis and Induction of Cell-Surface Phosphatidylserine Exposure
Several galectins induce apoptosis in some types of blood cells. For galectin-1, this activity has been studied most in human T cells, where apoptotic pathways may involve cell-surface glycoproteins, including CD7, CD29, and CD43. In contrast, for galectin-3 induction of apoptosis in T cells involves CD71 and CD45. In some cells, apoptotic signaling may function through down-regulation of Bcl-2 and activation of caspases. The interactions of galectin-9 with Tim-3 on Th1 cells may induce their apoptosis. In addition, some galectins, such as galectin-1, -2, and -4, also can induce exposure of cell-surface phosphatidylserine (PS) independently of apoptotic events. In addition, overexpression of intracellular galectin-3 shows antiapoptotic activity, whereas overexpression of galectin-7 and -12 may promote apoptosis in cells. Some potential intracellular binding partners of galectins, especially for galectin-3, include several proteins involved in regulating apoptosis, such as Bcl-2, Fas receptor (CD95), synexin (a Ca++- and phospholipid-binding protein), and Alix/Alg-2.
Roles of Galectins in Cancer
Many tumors overexpress various galectins and their heightened expression usually correlates with clinical aggressiveness of the tumor as well as the progression to a metastatic phenotype. The immunosuppressive and apoptotic effects of galectin-1 can contribute to tumor survival, as revealed by knockdown studies, in which decreased galectin-1 expression is associated with decreased tumor survival, owing to increased survival of IFN-γ-producing Th1 cells and heightened T-cell-mediated tumor rejection. Recent studies using galectin-1 knockout cell lines and mice have shown that expression of galectin-1 in tumor cell endothelium is essential for tumor angiogenesis, based on the binding of galectin-1 to complex N-glycans on VEGF receptor 2 (VEGFR2), and activation of VEGF-like signaling. Thus, galectins play important roles in tumor progression and metastasis through indirect effects in regulating tumor immune responses and direct effects in tumor angiogenesis. Although silencing of galectin-3 expression by methylation of its promoter is associated with early stages of prostate cancer, overexpression of galectin-3 correlates well with neoplastic transformation and tumor progression toward metastasis.
Roles of Galectins as Regulators of Immune Responses, Inflammation, and Adipogenesis
Galectins are important as regulators of immune and inflammatory responses. Galectins are expressed by a number of different immune and inflammatory cells. In addition, galectins can promote pro- or antiinflammatory responses, depending on the inflammatory stimulus, microenvironment, and the target cell. Immune cell responses to galectins also depend on the specific glycosylation of surface glycoproteins to generate galectin ligands. However, many of these functions were shown by using recombinant galectins added to cultured cells. Whether these reflect the functions of endogenous galectins is not well understood.
Galectin-3 is described to form lattices with cell-surface glycoproteins, for example, with T-cell receptor (TCR). Expression of such N-glycans is partly regulated by branching of N-glycans through β6 N-acetylglucosaminyltransferase V (MGAT-5). Mgat5 null T cells show enhanced clustering of TCRs suggesting that branched N-glycans interacting with galectin-3 may normally restrict TCR clustering and serve as a hindrance to the development of T-cell responses. Interestingly, subcutaneous injections of recombinant human galectin-1 and -9 can reduce the severity of several autoimmune diseases in various animal models, but the underlying mechanisms for these in vivo immunosuppressive activities are unclear.
Studies of mice deficient in a certain galectin indicate that galectins have roles in immune responses and inflammation. Studies of isolated cells from knockout mice have provided some insights. Endogenous galectin-3 plays a role in phagocytosis by macrophages and mediator release as well as cytokine production by mast cells. These have been linked to this galectin functioning intracellularly, in a fashion that is independent of glycan binding.
Current studies suggest a complex set of functions for galectin-3 in regulating many aspects of inflammatory responses and its role as a proinflammatory mediator. Although clearly complex, there have been some exciting developments linking galectins to inflammatory diseases in humans. Elevated serum galectin-3 levels are associated with a worse prognosis in patients with heart failure, and the plasma level of galectin-3 is a promising biomarker for acute coronary syndrome. Studies of galectin-3-knockout mice suggest a role for galectin-3 in cardiomyopathy and cardiac fibrosis. In other mouse disease models, endogenous galectin-3 contributes to fibrosis in various organs and tissues.
Recent studies have revealed critical and opposing roles of galectin-3 and -12 in adipogenesis and their association with obesity, inflammation, and type-2 diabetes. Galectin-12 is expressed in adipocytes and associated with lipid droplets, and functions as a negative regulator of lipolysis and insulin sensitivity. Galectin-12 knockout mice show increased lipolysis and reduced adiposity. In contrast, galectin-3 knockout mice display age-dependent increased adiposity, dysregulated glucose metabolism, and systemic inflammation. Furthermore, these mice show accelerated diabetes-associated kidney damage and diet-induced atherogenesis, with proinflammatory changes in visceral adipose tissue and pancreas.
Roles of Galectins as Recognition and Effector Factors on Infection
Galectins can also recognize exogenous glycans and related moieties on the surfaces of microbial pathogens and parasites, and function not only as pattern recognition receptors but also as effector factors, by directly inhibiting adhesion and/or entry into the host cell, or by directly killing or promoting phagocytosis of the potential pathogen. For example, galectin MjGal from the kuruma shrimp can recognize bacterial pathogens and promote their phagocytosis. Galectin-1 directly binds to Dengue virus and inhibits viral adhesion and internalization into host cells. Similarly, the zebrafish galectins Drgal1-L2 and Drgal3-L1 interact directly with the glycosylated envelope of the infectious hematopoietic necrosis virus and with the glycans on the epithelial cell surface, significantly reducing viral adhesion. In mammals, the tandem-repeat galectin-4 and -8 can recognize and kill E. coli strains that display B-blood group oligosaccharides (BGB+E. coli). Mutation of key residues in either CRD revealed that the C-CRD mediates recognition the BGB+E. coli and promotes activity toward bacteria. Taken together, the results of these studies indicate that galectins can function not only in immune recognition but also as effector factors in innate immune responses against pathogens.
In contrast, some pathogens and parasites can “subvert” the roles of host galectins in immune defense, to attach to or gain entry into host cells. For example, galectin-1 promotes infection by HIV-1 by facilitating viral attachment to CD4 receptors, and increasing infection efficiency. Leishmania species, which are transmitted by sandflies, attach to the insect midgut epithelium via the sandfly galectin PpGalec that binds to Gal(β1-3) side chains on the Leishmania lipophosphoglycan (LPG), to prevent their excretion along with the digested bloodmeal, and allow them to differentiate into free-swimming infective metacyclics. Similarly, the protozoan parasite Perkinsus marinus, a facultative intracellular parasite of the eastern oyster Crassostrea virginica is recognized via the galectins CvGal1 and CvGal2, that are expressed by phagocytic hemocytes. The parasite is phagocytosed by the oyster hemocytes and proliferates, eventually causing systemic infection and death of the oyster host. A recent study identified galectin-1 as the receptor for the protozoan parasite T. vaginalis, the causative agent of the most prevalent nonviral sexually transmitted human infection in both women and men. Like Leishmania spp, T. vaginalis displays a surface LPG rich in Gal and GlcNAc, which is recognized in a glycan-dependent manner by galectin-1 expressed by the epithelial cells in the cervical linings (as well as placenta, prostate, endometrial, and decidual tissue), colonized by the parasite. Related types of studies also show the roles of galectin-1 and -3 in influenza/pneumococcal pneumonia infections of bronchoalveolar space, and that galectins might be involved in virus and bacterial binding. The expression of galectins in animal tissues is tightly regulated, but can be modulated by a variety of stimuli, including infectious challenge, and this may be especially important in innate immune responses.
FURTHER READING
- Drickamer K. 1988. Two distinct classes of carbohydrate-recognition domains in animal lectins. J Biol Chem 263: 9557–9560. [PubMed: 3290208]
- Barondes SH, Castronovo V, Cooper DN, Cummings RD, Drickamer K, Feizi T, Gitt MA, Hirabayashi J, Hughes C, Kasai K, et al. 1994. Galectins: A family of animal β-galactoside-binding lectins. Cell 76: 597–598. [PubMed: 8124704]
- Houzelstein D, Gonçalves IR, Fadden AJ, Sidhu SS, Cooper DNW, Drickamer K, Leffler H, Poirier F. 2004. Phylogenetic analysis of the vertebrate galectin family. Mol Biol Evol 21: 1177–1187. [PubMed: 14963092]
- Liu FT, Rabinovich GA. 2005. Galectins as modulators of tumour progression. Nat Rev Cancer 5: 29–41. [PubMed: 15630413]
- van Die I, Cummings RD. 2006. Glycans modulate immune responses in helminth infections and allergy. Chem Immunol Allergy 90: 91–112. [PubMed: 16210905]
- Elola MT, Wolfenstein-Todel C, Troncoso MF, Vasta GR, Rabinovich GA. 2007. Galectins: Matricellular glycan-binding proteins linking cell adhesion, migration, and survival. Cell Mol Life Sci 64: 1679–1700. [PubMed: 17497244]
- Mercier S, St-Pierre C, Pelletier I, Ouellet M, Tremblay MJ, Sato S. 2008. Galectin-1 promotes HIV-1 infectivity in macrophages through stabilization of viral adsorption. Virology 371: 121–129. [PubMed: 18028978]
- Stowell SR, Cummings RD. 2008. Interactions of galectins with leukocytes. In Animal lectins: A functional view (ed. Vasta GR, Ahmed H.). CRC Press, Boca Raton, FL.
- Vasta GR. 2009. Roles of galectins in infection. Nat Rev Microbiol 7: 424–438. [PMC free article: PMC3759161] [PubMed: 19444247]
- Dam TK, Brewer CF. 2010. Maintenance of cell surface glycan density by lectin–glycan interactions: A homeostatic and innate immune regulatory mechanism. Glycobiology 20: 1061–1064. [PubMed: 20548106]
- Stowell SR, Arthur CM, Dias-Baruffi M, Rodrigues LC, Gourdine JP, Heimburg-Molinaro J, Ju T, Molinaro RJ, Rivera-Marrero C, Xia B, et al. 2010. Innate immune lectins kill bacteria expressing blood group antigen. Nat Med 16: 295–301. [PMC free article: PMC2853181] [PubMed: 20154696]
- DiLella S, Sundblad V, Cerliani JP, Guardia CM, Estrin DA, Vasta GR, Rabinovich GA. 2011. When galectins recognize glycans: From biochemistry to physiology and back again. Biochemistry 50: 7842–7857. [PMC free article: PMC3429939] [PubMed: 21848324]
- Liu FT, Yang RY, Hsu DK. 2012. Galectins in acute and chronic inflammation. Ann NY Acad Sci 1253: 80–91. [PubMed: 22329844]
- Rabinovich GA, van Kooyk Y, Cobb BA. 2012. Glycobiology of immune responses. Ann NY Acad Sci 1253: 1–15. [PMC free article: PMC3884643] [PubMed: 22524422]
- Thurston TL, Wandel MP, vonMuhlinen N, Foeglein A, Randow F. 2012. Galectin 8 targets damaged vesicles for autophagy to defend cells against bacterial invasion. Nature 482: 414–418. [PMC free article: PMC3343631] [PubMed: 22246324]
- Vasta GR, Ahmed H, Nita-Lazar M, Banerjee A, Pasek M, Shridhar S, Guha P, Fernández-Robledo JA. 2012. Galectins as self/non-self recognition receptors in innate and adaptive immunity: An unresolved paradox. Front Immunol 3: 1–14. [PMC free article: PMC3396283] [PubMed: 22811679]
- Yang RY, Havel PJ, Liu FT. 2012. Galectin-12: A protein associated with lipid droplets that regulates lipid metabolism and energy balance. Adipocyte 1: 96–100. [PMC free article: PMC3609087] [PubMed: 23700518]
- Chen HY, Weng IC, Hong MH, Liu FT. 2014. Galectins as bacterial sensors in the host innate response. Curr Opin Microbiol 17: 75–81. [PubMed: 24581696]
- Croci DO, Cerliani JP, Dalotto-Moreno T, Méndez-Huergo SP, Mascanfroni ID, Dergan-Dylon S, Toscano MA, Caramelo JJ, García-Vallejo JJ, Ouyang J, et al. 2014. Glycosylation-dependent lectin–receptor interactions preserve angiogenesis in anti-VEGF refractory tumors. Cell 156: 744–758. [PubMed: 24529377]
- Toledo KA, Fermino ML, AndradeCdel C, Riul TB, Alves RT, Muller VD, Russo RR, Stowell SR, Cummings RD, Aquino VH, et al. 2014. Galectin-1 exerts inhibitory effects during DENV-1 infection. PLoS One 9: e112474. [PMC free article: PMC4231055] [PubMed: 25392933]
- Vladoiu MC, Labrie M, St-Pierre Y. 2014. Intracellular galectins in cancer cells: Potential new targets for therapy. Int J Oncol 44: 1001–1014. [PubMed: 24452506]
- Arthur CM, Baruffi MD, Cummings RD, Stowell SR. 2015. Evolving mechanistic insights into galectin functions. Methods Mol Biol 1207: 1–35. [PMC free article: PMC5755386] [PubMed: 25253130]
- Blidner AG, Méndez-Huergo SP, Cagnoni AJ, Rabinovich GA. 2015. Re-wiring regulatory cell networks in immunity by galectin-glycan interactions. FEBS Lett 589: 3407–3418. [PubMed: 26352298]
- Than NG, Romero R, Balogh A, Karpati E, Mastrolia SA, Staretz-Chacham O, Hahn S, Erez O, Papp Z, Kim CJ. 2015. Galectins: Double-edged swords in the cross-roads of pregnancy complications and female reproductive tract inflammation and neoplasia. J Pathol Transl Med 49: 181–208. [PMC free article: PMC4440931] [PubMed: 26018511]
- Rabinovich GA, Conejo-Garcia JR. 2017. Shaping the immune landscape in cancer by galectin-driven regulatory pathways. J Mol Biol 428: 3266–3281. [PubMed: 27038510]
- Salvagno GL, Pavan C. 2017. Prognostic biomarkers in acute coronary syndrome. Ann Transl Med 4: 258. [PMC free article: PMC4958728] [PubMed: 27500159]
- Review Galectins.[Essentials of Glycobiology. 2022]Review Galectins.Cummings RD, Liu FT, Rabinovich GA, Stowell SR, Vasta GR. Essentials of Glycobiology. 2022
- Review Galectins.[Essentials of Glycobiology. 2009]Review Galectins.Cummings RD, Liu FT. Essentials of Glycobiology. 2009
- Review Role of Galectins in Multiple Myeloma.[Int J Mol Sci. 2017]Review Role of Galectins in Multiple Myeloma.Storti P, Marchica V, Giuliani N. Int J Mol Sci. 2017 Dec 17; 18(12). Epub 2017 Dec 17.
- Review Oligosaccharide specificity of galectins: a search by frontal affinity chromatography.[Biochim Biophys Acta. 2002]Review Oligosaccharide specificity of galectins: a search by frontal affinity chromatography.Hirabayashi J, Hashidate T, Arata Y, Nishi N, Nakamura T, Hirashima M, Urashima T, Oka T, Futai M, Muller WE, et al. Biochim Biophys Acta. 2002 Sep 19; 1572(2-3):232-54.
- Cloning, expression, and purification of galectins for in vitro studies.[Methods Mol Biol. 2015]Cloning, expression, and purification of galectins for in vitro studies.Poland PA, Kinlough CL, Hughey RP. Methods Mol Biol. 2015; 1207:37-49.
- Galectins - Essentials of GlycobiologyGalectins - Essentials of Glycobiology
Your browsing activity is empty.
Activity recording is turned off.
See more...