NCBI Bookshelf. A service of the National Library of Medicine, National Institutes of Health.
National Academies of Sciences, Engineering, and Medicine; Division on Earth and Life Studies; Board on Agriculture and Natural Resources; Committee on Nutrient Requirements of Dairy Cattle. Nutrient Requirements of Dairy Cattle: Eighth Revised Edition. Washington (DC): National Academies Press (US); 2021 Aug 30.
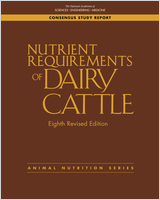
Nutrient Requirements of Dairy Cattle: Eighth Revised Edition.
Show detailsINTRODUCTION
From birth until weaning to solid feed, calves undergo tremendous physiologic and metabolic changes (Toullec and Guilloteau, 1989; Meale et al., 2017). During the preruminant stage, digestion and metabolism are similar to those of nonruminant animals, and dietary requirements must be met with high-quality liquid diets containing sources of carbohydrates, proteins, and fats that are digested efficiently. The most critical period is the first 2 to 3 weeks of life when the calf's digestive system is immature but developing rapidly with regard to digestive secretions and enzymatic activity (Toullec and Guilloteau, 1989; Davis and Drackley, 1998).
Except for calves raised for veal production, calves should be encouraged to consume solid feed at an early age to stimulate development of a functional rumen. Development of the ruminal epithelial tissue that is responsible for absorption of volatile fatty acids (VFAs) is stimulated by the VFAs, particularly butyrate, produced by ruminal microbes (Sander et al., 1959). A starter concentrate high in readily fermentable carbohydrates supports development of the microbiota and its fermentation necessary for proper ruminal tissue growth (Brownlee, 1956; Flatt et al., 1958; Williams and Frost, 1992; Greenwood et al., 1997). The functioning rumen epithelium can absorb and metabolize the VFAs, which aids in raising ruminal pH to levels suitable for fiber fermentation.
The rumen and its microbial population are immature at this stage (Anderson et al., 1987a,b, and ruminal cellulose digestibility is limited (Williams and Frost, 1992). Consequently, forages other than fresh grass that is high in sugars (Ohtani et al., 1976) are not effective in developing a functional rumen and may limit metabolizable energy (ME) intake (MEI) in young calves (Stobo et al., 1966). Calves have limited ability to use forages until well after weaning (Quigley, 1996a; Davis and Drackley, 1998). Nevertheless, adequate particle size of starter feed—whether pelleted, ground, or texturized—is important to prevent abnormal development and keratinization of ruminal papillae and to prevent impaction of fine particles between papillae (McGavin and Morrill, 1976; Greenwood et al., 1997; Beharka et al., 1998).
With respect to the nutrient requirements of the calf, three phases for development of digestive function are recognized (Davis and Clark, 1981):
- Liquid-feeding phase. Essentially all of the nutrient requirements are met by milk or milk replacer (MR). The functional reticular (esophageal) groove shunts liquid feeds directly to the omasum to avoid microbial breakdown in the reticulorumen (Orskov, 1972).
- Transition phase. Liquid feed and starter contribute to meeting the nutrient requirements. Starter enables development of the reticulorumen.
- Ruminant phase. The calf derives its nutrients from solid feeds, primarily through microbial fermentation in the reticulorumen. Ruminal fermentation and microbial protein synthesis are not yet mature during the early stages of this phase.
Similar to the previous edition (NRC, 2001), this chapter discusses the nutrient requirements of calves in each of these phases, but in this edition, the committee made the following changes: (1) empty body weight (EBW) was used for all calculations; (2) an equation to estimate starter intake was added; (3) energy requirements were updated and include different maintenance requirements for different classes of animals, breeds, and environmental conditions, and estimating composition of body gain was included and efficiency of ME use for gain was updated; (4) calculation of feed ME values was revised; (5) a new metabolizable protein system (MP) was used; and (6) mineral requirement system was changed and some vitamin recommendations were revised. For purposes of estimating nutrient requirements, it is assumed that cattle less than 18 percent of mature body weight (MatBW; 125 kg for Holsteins with mature weight of 700 kg) are calves and those that are >18 percent are heifers. Growth requirements for the latter are discussed in Chapter 11.
BODY WEIGHT CONVERSIONS
All calculations in this edition were made on an EBW basis unless otherwise noted. Factors for converting body weight (BW) into EBW for various calves and diets are in Table 10-1 and range from 0.85 to 0.96 (Diaz et al., 2001; Tikofsky et al., 2001; Blome et al., 2003; Meyer, 2005; Bartlett et al., 2006; Hill et al., 2008a; Mills et al., 2010; Stamey Lanier et al., 2021). Mathematically, if EBW as a proportion of BW is unchanged, then EBW gain (EBG) should be the same proportion to BW gain as the ratio of EBW/BW. However, because the ratio of EBW to BW decreases as the rumen develops, the ratio of EBG to average daily gain (ADG) will be less than the ratio of EBW to BW. Factors for converting ADG into EBG are in Table 10-1 (Meyer, 2005; Hill et al., 2008a; Stamey Lanier, 2021). For heavy veal calves (>125 kg), EBW was calculated as 0.93 BW based on data from Gerrits et al. (1996). The decrease in proportion of BW as EBW likely occurs because of the rapid change in solid feed intake and expansion of rumen and gut size. At any point during this changeover to solid feed, the change in EBW is less than the change in BW. Consequently, during weaning transition to solid food only, EBW/BW will be somewhere between 0.93 and 0.85, and as a result, EBG will be in the range of 0.5 to 0.6 ADG. The time course and implications in these changes in body composition during the weaning transition deserve further research.
DRY MATTER INTAKE
Historically, most dairy calves have not been fed milk or MR for ad libitum intake. Maximal dry matter intake (DMI) from milk or MR is about 2.25 percent of BW, with calves achieving DMI of 2 percent of BW during the first week of life (Jasper and Weary, 2002). Near ad libitum intake of MR dry matter (DM) increased to approximately 2.5 percent of BW for calves >65 kg (Diaz et al., 2001). For veal calves, voluntary intake of MR DM declines to less than 2 percent of BW by 120 kg BW (Gerrits et al., 1996).
For most herd replacements, calves are offered a fixed and limited quantity of milk or MR DM daily with ad libitum access to starter and, perhaps, limited amounts of forage. Total DMI during this period will increase as starter intake increases. In an analysis of 219 treatment means from 64 published studies (see later section on model evaluation), mean DMI for calves (<8 weeks old, BW = 54.5 ± 8.8 kg, mean ± SD) was 1.93 ± 0.33 percent of BW, with a range of 1.17 to 3.06 percent of BW. During the weaning process, DMI increases rapidly. From the same data summary (79 treatment means from 27 studies), intake of DM from solid feeds for weaned calves (>8 weeks old, BW = 95.6 ± 19.0 kg, mean ± SD) averaged 3.06 ± 0.31 percent of BW, with a range of 2.16 to 4.45 percent of BW.
Many factors affect starter intake in young calves, the most important of which are milk or MR DMI and age (Kertz et al., 1979). Other factors include initial BW, water availability, starter quality and physical form, and quality of MR (summarized by Davis and Drackley, 1998). Starter intake is highly variable, with coefficients of variation among published studies of 90 percent and 19 to 38 percent among similar studies conducted at the same location (Kertz et al., 1979). Much of this variability may be from individual calf differences in how quickly rumen pH is stabilized as solid feed begins to be consumed (Williams and Frost, 1992).
The committee developed models to predict starter intake for use with this edition. BW, daily MEI from the liquid diet (MEiLD), ADG, and time relative to first offer of starter (FPstarter) were used as independent variables. For calves in temperate climates, a model was developed using individual animal data (n = 26,952 observations from 1,356 calves) from 28 studies carried out in 4 U.S. states and the Netherlands (Georgia, n = 168; Illinois, n = 1,925; Minnesota, n = 6,052; Ohio, n = 16,457; and the Netherlands, n = 2,350). An external data set (n = 8,891 individual observations, nine studies) was developed to evaluate the models using data from four U.S. states (Iowa, n = 6,332; New Hampshire, n = 1,519; New York, n = 892; Virginia, n = 148). Intake of milk or MR ranged from 0.11 to 1.99 kg/d and starter intake from 0.00 to 2.85 kg/d. For calves in subtropical environments, equations to predict starter intake were developed using individual animal data (n = 3,491 observations from 853 calves) from 15 studies carried out in the United States and Brazil (Florida, n = 1,127; Georgia, n = 179; Brazil, n = 2,185). An independent data set (n = 479 individual observations, five studies) was used to evaluate the models using data from the United States and Brazil (Georgia, n = 96; Brazil, n = 383). Thus, 25 percent of studies (5 of 20 studies) were used for model evaluation. Intake of milk or MR DM ranged from 0.21 to 2.07 kg/d and starter intake from 0.00 to 1.93 kg/d.
For each environmental condition, two model-fitting approaches were used. First, a set of linear mixed models was developed using an automated model selection approach (“MuMIn” and “lme4” packages) and parallel computation in R (version 4.0.1). The linear mixed models included the random effect of study. Interactions and squared terms were tested. Then, the linear mixed models with the lowest Akaike information criterion (AIC) values were selected and evaluated. Second, for nonlinear models (exponential and logistic), the initial parameters were fitted using the “nls” function (nonlinear least squares) and plots from the “easynls” package. Then, the initial parameters were used to fit nonlinear mixed-effects regression using the “nlme” package in R. The random effect of study was added to the A coefficient. Nonlinear models with or without the random effect of study were evaluated, and adjustments with different fixed effects were tested. Finally, the best models from the external validation were selected based on small AIC values from derivation, high concordance correlation coefficient (CCC), minimal slope and mean biases, and low root mean squared prediction error (RMSE). The best models from the external evaluation were reevaluated using a repeated K-fold cross-validation (n = 500, k = 10) using both development and external databases to have more information about model performance.
The equation selected for calves in temperate conditions is as follows:
where BW is in kg, MEiLD is in Mcal/d, and FPstarter is in weeks. In the model, age is used as a proxy for week when starter is first offered, assuming starter was offered during first week of life. This model had an RMSE of 262 g/d, with a CCC of 0.71. The average R2 using the development database in repeated K-fold cross-validation was 0.66 ± 0.01 and from the evaluation database was 0.67 ± 0.02.
The equation for calves in semitropical conditions is as follows:
This model had an RMSE of 222 g/d and a CCC of 0.78. When users enter an environmental temperature >35°C, Equation 10-2 is used. Otherwise Equation 10-1 is used.
ENERGY REQUIREMENTS OF CALVES
Energy Units and Classes of Calves
The current revision expresses energy requirements and dietary energy supply for calves in terms of ME, although derivation of these requirements is based in part on determination of net energy (NE) values. Data on energy requirements were derived for classes of calves fed only milk or MR, calves fed milk or MR plus starter feed (without or with forage), and weaned calves to 125 kg of BW fed starter or grower diets and limited forage. Requirements for veal calves fed only milk or MR also were evaluated.
Description of the Database
The previous edition (NRC, 2001) adopted the summary equation of Toullec (1989), which was derived from a number of studies with veal calves, for prediction of ME requirements of all classes of calves. Because this equation was derived from older studies with heavier calves of greater relative maturity and with the goal of fattening, the previous committee recognized the need for data on the composition of live weight gain for dairy calves of current genetics. Since that publication, a number of studies have provided data on body composition and composition of EBG of Holstein (Diaz et al., 2001; Tikofsky et al., 2001; Blome et al., 2003; Brown et al., 2005b; Meyer, 2005; Bartlett et al., 2006; Hill et al., 2008a; Mills et al., 2010; Stamey Lanier et al., 2021), Jersey (Bascom et al., 2007), and Holstein × Gyr crossbred (Silva et al., 2017) calves. The present committee derived equations for energy and protein requirements from a subset of these body composition studies (Diaz et al., 2001; Tikofsky et al., 2001; Meyer, 2005; Bartlett et al., 2006; Bascom et al., 2007; Mills et al., 2010; Stamey Lanier et al., 2021).
Individual data were available for 255 calves from seven comparative slaughter studies with appropriate baseline groups to measure changes in body composition as calves grew. Six of the studies used Holstein calves (Diaz et al., 2001; Tikofsky et al., 2001; Meyer, 2005; Bartlett et al., 2006; Mills et al., 2010; Stamey Lanier et al., 2021) and one used Jersey calves (Bascom et al., 2007). In two of the studies, calves were fed both MR and solid feeds (Meyer, 2005; Stamey Lanier et al., 2021), while calves in the remaining studies were fed only milk or MR. Data were combined into a common database for analysis.
Maintenance Energy Requirements
Conceptually, maintenance is a simple idea, but its determination is fraught with difficulties, including whether to define it as the point of zero weight change or zero change in body energy. Methodological considerations also complicate measurement of maintenance, whether by calorimetric or comparative slaughter procedures. In previous NRC systems, net energy for maintenance (NEm) was defined as heat production (HP) at zero MEI, by extrapolation of the regression of HP on MEI to the y-intercept, plus an activity allowance. Labussiere et al. (2009, 2011) proposed a method to calculate maintenance that considered increases in apparent maintenance HP with increasing MEI prior to determination of fasting heat production. Others (ARC, 1980; Moe, 1981) have argued against the use of measured fasting HP as a baseline for maintenance. Regardless of methodology, reliable estimates of maintenance are necessary for defining energy use by growing calves.
The second component of maintenance has been an allowance for activity, typically 10 percent of NEm. Thus, the NEm value adopted by NRC (2001) was 0.086 Mcal/kg BW0.75, which was similar to calorimetric data from the USDA Beltsville station, where activity was embedded in the total estimate of NEm. Others have argued that the activity allowance is too high for growing cattle, which would inflate estimates of maintenance (Ainslie et al., 1992; Van Amburgh et al., 1998). Labussiere et al. (2008b, 2009, 2011) measured the energy cost of standing activity in veal calves by calorimetry and found that it accounted for about 3.5 to 8 percent of MEI, supporting the idea that a maintenance activity allowance of 10 percent is too large. The NEm value determined by extrapolation of regression equations to zero MEI in comparative slaughter experiments would include the components of basal metabolism and activity and thus may be more reliable.
Maintenance energy requirements historically have been expressed relative to BW0.75, but this relationship has not been evaluated experimentally in dairy calves until recently (Labussière et al., 2008b). Those researchers found that expressing BW raised to a power of 0.85 best fit the data and minimized variation for fasting heat production measured by calorimetry in Holstein veal calves over a range of BW from 73 to 221 kg. Furthermore, use of the coefficient 0.75 resulted in significantly different estimates compared with the exponents 0.80, 0.85, or 0.90. However, use of 0.85 as the exponent to calculate metabolic BW in the current database increased variation in estimates of energy relationships, and thus the exponent 0.75 continues to be used in this revision.
Heat production was calculated as the difference between MEI and retained energy (RE), where RE was calculated from the increase in body energy content between baseline calves slaughtered at the start of the feeding period and calves at the end of the feeding period (Lofgreen and Garrett, 1968). The NEm was calculated as the intercept (a) of the equation of exponential regression (NEm = a × eb × MEI) when MEI = 0, as described by Ferrell and Jenkins (1998a,b). In this equation, NEm = heat production in units of Mcal/EBW0.75 per day, MEI = metabolizable energy intake (Mcal/EBW0.75 per day), and a and b are equation parameters. Regressions explored data from all calves, Holstein calves fed milk or MR only, Holstein calves fed milk or MR plus solid feed, and Jersey calves fed MR or milk only. Study was included as a random effect in a nonlinear mixed model using R software. Treatments that were known to be protein deficient in Bartlett et al. (2006) were removed as were data for two extreme outliers, leaving 235 observations for the analysis. From multiple regression analyses, the committee concluded that separate values for maintenance could not be justified among those groups. Therefore, for small or large breed calves fed milk or MR without or with starter, the final nonlinear equation was as follows (see Figure 10-1a):

FIGURE 10-1
Relationship between daily HP and MEI (both as Mcal/kg BW0.75) and plots of observed − predicted versus predicted and residuals.
Analysis of regressions and residuals indicated no mean or slope bias (see Figure 10-1b). The NEm derived from the intercept (0.077 Mcal/EBW0.75) is considerably lower than the value established by previous NRC committees (0.086 Mcal/BW0.75; NRC 1989, 2001) but is in the range of estimates by others (ARC, 1980; NRC, 1978; Silva et al., 2017) and the value used by the beef NASEM (2016).
By the iterative method, the ME requirement for maintenance (MEm, Mcal/EBW0.75 per day) was determined as the point where MEI and heat production are equal (i.e., the point at which there is no energy retention in the body) (Lofgreen and Garrett, 1968). MEm was determined to be 0.107 Mcal/kg EBW0.75, or 0.101 Mcal/kg BW0.75, which is similar to the value used by NRC (2001) as well as others (ARC, 1980; Labussiere et al., 2007). The efficiency of use of ME for maintenance (km) was calculated as the ratio between the NE and MEm. The resulting value (0.719) also is lower than the value (0.86) used previously by NRC (2001) but is similar to the efficiency values of 0.726 and 0.706 from INRA (2019) and Silva et al. (2017), respectively.
Based on the studies in the database with calves fed both starter and liquid feed (Meyer, 2005; Stamey Lanier et al., 2021), their maintenance energy requirements were about 2 percent higher than calves fed milk only, but these were not statistically different. Greater requirements as calves consume solid feeds might be predicted, as the size of metabolically active organs, such as the gastrointestinal tract and liver, increases along with rumen development.
The NEm for weaned heifers up to 125 kg BW was set to 0.097 Mcal/kg EBW0.75 (0.0825 Mcal/kg BW0.75), based on estimates from Stamey Lanier (2021) and Meyer (2005). This value is intermediate between the preweaned and growing heifer values (see Chapter 11). The corresponding MEm values are 0.138 Mcal ME/kg EBW0.75 or 0.117 Mcal ME/kg BW0.75. These values are higher than NRC (2001) but lower than those in other systems (ARC, 1980; INRA, 2019). The National Academies Beef Model (NASEM, 2016) set maintenance NEm for growing dairy breed calves to 0.095 Mcal/kg BW0.75, or 0.112 Mcal/kg EBW0.75. Assuming an efficiency of ME use for maintenance of about 0.70 implicit in the beef guidelines (NASEM, 2016), these equate to 0.137 Mcal ME/kg BW0.75 and 0.160 Mcal ME/kg EBW0.75. Because of the paucity of body composition studies with weaned calves in this weight range, additional data are needed to more accurately model requirements for maintenance.
Based on the very limited data available for estimation of energy requirements for milk-fed Jersey calves (Bascom et al., 2007), the determined coefficient for NEm was about 6.6 percent greater and the coefficient for MEm about 15.1 percent greater than that for Holstein calves fed milk (not statistically significant). The comparative slaughter data from Bascom et al. (2007) are directionally consistent with observations by others that maintenance for Jersey calves may be up to 20 percent greater than Holsteins (Ballou and DePeters, 2008; Ballou, 2012; Van Amburgh et al., 2019), even when corrected by use of metabolic BW. The discrepancy may be attributable to the ratios of surface area to body mass. Brody (1945) found that surface area in Holstein cattle from 41 to 617 kg BW was described by the equation 0.14 × BW0.57. Consequently, surface area to mass ratios are greater in calves that are smaller than the average Holstein calf, so rate of heat loss would increase more than predicted by BW0.75 alone. In turn, the metabolic rate and hence maintenance HP would be greater in smaller Jersey calves. However, in the absence of sufficient data, the committee left the values the same as for large-breed calves. Additional research is needed to better define the requirements of small-breed calves.
TABLE 10-1Ratios of EBW to BW and Energy Requirements for Maintenancea
Item | Milk or Milk Replacer Only | Milk or Milk Replacer Plus Solid Feed | Weaned, Solid Feeds Only | |||
---|---|---|---|---|---|---|
Holstein/Large Breed | Jersey/Small Breed | Holstein/Large Breed | Jersey/Small Breed | Holstein/Large Breed | Jersey/Small Breed | |
EBW/BW | 0.94 | 0.94 | 0.93 | 0.93 | 0.85 | 0.85 |
EBG/ADG | 0.94 | 0.94 | 0.91 | 0.91 | 0.85 | 0.85 |
NEm, kcal/kg EBW0.75 | 76.9 | 76.9 | 76.9 | 76.9 | 97.0 | 97.0 |
NEm, kcal/kg BW0.75 | 72.3 | 72.3 | 72.3 | 72.3 | 82.5 | 82.5 |
MEm, kcal/kg EBW0.75 | 107.0 | 107.0 | 113.8 | 113.8 | Varb | Varb |
MEm, kcal/kg BW0.75 | 100.0 | 100.0 | 105.8 | 105.8 | Varb | Varb |
Efficiency of ME use for NEm, km | 0.72 | 0.72 | 0.69 | 0.69 | Varb | Varb |
- a
Expressed as a function of metabolic EBW or live BW and efficiency of ME use for NEm (km).
- b
Determined as efficiency of solid feed use (as determined from ME density by Equation 10-4).
The efficiency of use of ME from solid feeds is calculated from the ratio of NEm to ME using the equation from Galyean et al. (2016), as follows:

where ME is expressed in terms of Mcal/kg DM. Over the range of ME values encountered for starters (i.e., 2.5 to 3.5 Mcal/kg DM), efficiencies would vary only from 0.60 to 0.64. The overall dietary efficiency of ME use is calculated as the weighted mean of ME provided by liquid and solid feeds as in the previous edition (NRC, 2001).
Effects of Environment on Maintenance Requirements
Calves are born with limited body energy reserves and only modest insulation afforded by hair coat and body fat. Without feed, a newborn calf probably has enough body energy stores in the form of fat and glycogen to last no more than about 1 day in temperatures below its lower critical temperature (Alexander et al., 1975; Okamoto et al., 1986; Rowan, 1992).
Energy standards are based on the premise that the animal is in a thermoneutral environment. In such an environment, the animal is not required to elicit specific heat-conserving or heat-dissipating mechanisms to maintain core body temperature (NRC, 1981). The thermoneutral zone shifts depending on many factors, the more important being age, amount of feed intake, amount of subcutaneous fat, and length and thickness of hair coat. The thermoneutral zone in very young calves ranges from 15°C to 25°C (NRC, 2001). Thus, when the environmental temperature drops below 15°C, which is referred to as the lower critical temperature, the calf must expend energy to maintain body temperature. In practical terms, the maintenance energy requirement is increased. For older calves and calves at greater feed intakes, cold tolerance is greater and the lower critical temperature may be as low as –5°C to –10°C (Webster et al., 1978). Data in Table 10-2 illustrate the effects of a decrease in environmental temperature above the upper critical temperature or below the lower critical temperature on energy requirements for maintenance. For cold stress, the values were calculated from research data of Schrama et al. (1992a). In the example given in Table 10-2, if the lower critical temperature is 15°C and the effective ambient temperature is 0°C, the maintenance energy requirement for calves <3 weeks old is increased by 38 percent. Scibilia et al. (1987) reported that maintenance ME requirement was increased by 32 percent for calves housed at –4°C compared with calves housed at 10°C. Table 10-2 suggests that calves at this temperature (–5°C) would have a 30 percent greater maintenance requirement. These estimates agree at least qualitatively with other reports (Soberon and Van Amburgh, 2012). Effects of cold stress in increasing maintenance requirements have been incorporated into the model provided with this publication as 2.01 kcal/kg0.75 per day for each degree decrease in environmental temperature (°C) below the lower critical temperature (Schrama et al., 1992a).
TABLE 10-2Effect of Environmental Temperature on Energy Requirement of Young Calvesa
Environmental Temperature | Increase in Maintenance Energy Requirement (kcal of NEm/d) | Maintenance Energy Requirement (kcal of ME/d) | Increase in ME Required for Maintenance (%) | ||||
---|---|---|---|---|---|---|---|
°F | °C | Birth to 3 Weeks of Ageb | >3 Weeks of Agec | Birth to 3 Weeks of Ageb | >3 Weeks of Agec | Birth to 3 Weeks of Ageb | >3 Weeks of Agec |
113 | 45 | 698 | 698 | 2,557 | 2,675 | 38 | 40 |
104 | 40 | 524 | 524 | 2,383 | 2,501 | 28 | 30 |
95 | 35 | 349 | 349 | 2,208 | 2,326 | 19 | 20 |
86 | 30 | 175 | 175 | 2,034 | 2,152 | 9 | 10 |
77 | 25 | 0 | 0 | 1,859 | 1,977 | 0 | 0 |
68 | 20 | 0 | 0 | 1,859 | 1,977 | 0 | 0 |
59 | 15 | 175 | 0 | 2,034 | 1,977 | 9 | 0 |
50 | 10 | 349 | 0 | 2,208 | 1,977 | 19 | 0 |
41 | 5 | 524 | 175 | 2,383 | 2,152 | 28 | 9 |
32 | 0 | 698 | 349 | 2,557 | 2,326 | 38 | 18 |
23 | –5 | 873 | 524 | 2,732 | 2,501 | 47 | 26 |
14 | –10 | 1,048 | 698 | 2,907 | 2,675 | 56 | 35 |
5 | –15 | 1,222 | 873 | 3,081 | 2,850 | 66 | 44 |
–4 | –20 | 1,397 | 1,048 | 3,256 | 3,025 | 75 | 53 |
–13 | –25 | 1,572 | 1,222 | 3,431 | 3,199 | 85 | 62 |
–22 | –30 | 1,746 | 1,397 | 3,605 | 3,374 | 94 | 71 |
- a
Calculated for a calf with EBW of 45 kg. Extra heat production = 2.01 kcal/kg0.75 per day for each degree decrease in environmental temperature (°C) below lower critical temperature (Schrama et al., 1992a) or for each degree increase above upper critical temperature. Heat production is in terms of net energy (NE), but metabolizable energy (ME) is assumed to be used with 100 percent efficiency for HP.
- b
Maintenance energy requirement 107 kcal/kg0.75 EBW per day. Calves from birth to 3 weeks of age have lower critical temperature in the range of 15°C to 25°C. Data above were calculated on the basis of a lower critical temperature of 15°C and an upper critical temperature of 25°C.
- c
Maintenance energy requirement 113.8 kcal/kg0.75 EBW per day. Data for calves older than 3 weeks of age were calculated on the basis of a lower critical temperature of 5°C and an upper critical temperature of 25°C.
Calves, especially very young calves, must be fed extra energy during cold weather to satisfy the increase in maintenance energy requirements. That can be accomplished by increasing the amount of liquid diet being fed, by adding an additional feeding daily, by adding additional milk solids to the liquid diet (Schingoethe et al., 1986), by incorporating additional fat into the liquid diet (Scibilia et al., 1987; Jaster et al., 1990), or by addition of fat to calf starter (Johnson et al., 1956; Araujo et al., 2014; Hill et al., 2015; Ghasemi et al., 2017; Berends et al., 2018; Doolatabad et al., 2020; Ghorbani et al., 2020). Additional fat in MR or starter decreased starter intake in one study (Kuehn et al., 1994), which negated at least a portion of the increased energy density from fat supplementation. However, in more recent studies in which milk or MR was fed in larger amounts, fat in starter did not decrease starter intake so that energy intake was increased (Araujo et al., 2014; Hill et al., 2015; Berends et al., 2018; Doolatabad et al., 2020; Ghorbani et al., 2020). If additional solids are fed, the DM concentration of MR should not exceed 20 percent to avoid problems with excessive mineral intake (Jenny et al., 1978; Ternouth et al., 1985) that can lead to osmotic imbalances in the gut, and supplemental water should always be provided. The availability of free water is critically important to starter intake (Kertz et al., 1984); provision of warm water two to three times daily during cold weather may help to stimulate starter feed intake, which would help to counteract cold stress.
Heat stress also increases maintenance energy requirements for panting and heat dissipation. Unlike cold stress, however, heat stress decreases DMI in cattle (West, 2003). Little research has been conducted to quantify the increased requirements resulting from heat stress in calves (Roland et al., 2016). Spain and Spiers (1996) found that calves began to pant at 26°C, which is similar to older cattle (Spain and Spiers, 1996). The committee adopted this temperature (26°C) as the upper critical temperature for calves. At environmental temperatures above this, increasing amounts of ME are used to cool the calf, thereby increasing maintenance ME requirements. In the absence of data, the model assumes that the increase of maintenance requirement per degree of temperature above the upper critical temperature occurs in the same proportion as the response to cold temperatures. Empirically, linearizing the qualitative recommendations for heat stress in older heifers in the previous version (NRC, 2001) approximates the approach adopted. Conditions such as proper heat abatement and nighttime cooling would reduce the effect of heat stress on maintenance requirement. Calves will not eat more to meet the greater maintenance requirement for heat dissipation; rather, the calf will decrease voluntary intake, particularly for starter, in response to heat stress (Roland et al., 2016). The result is that growth may decrease during periods of heat stress. In addition, heat stress is detrimental to the immune system, thereby increasing potential for morbidity, which further increases maintenance requirements due to activity of the immune system and decreases growth (Bagath et al., 2019).
Energy Requirements for Growth
Retained Energy and Empty Body Weight Gain
The current system for calf growth is based on ME-allowable growth and MP-allowable growth. Establishment of the energy requirements for growth relies on accurate estimation of the amount of RE per unit of growing tissue, as determined by relative amounts of fat and protein deposited. The amount of protein deposited per unit of BW gain generally is quite invariable if MP is sufficient, whereas tissue fat deposition is variable depending on total MEI or limitation of growth by MP supply (Van Amburgh et al., 2019).
The database of individual calves from comparative slaughter studies described above was used to derive equations to predict RE from EBW and EBG. Three equation forms were evaluated using R software to relate RE to EBW and EBG. Each equation included the random effect of study. The first form was that used by NRC (2001): RE (Mcal/d) = a × (EBGb, kg/d) × (EBWc, kg), where a, b, and c are equation parameters. The second form was the same but EBW0.75 was used rather than a model-derived exponent. Finally, the committee used the previous NRC equation form but without the a coefficient.
The first form had an unacceptably high (>964) variance inflation function as a result of the substantial correlations between the a and c parameters, which made this model highly unstable to changes in inputs, and it was removed from further consideration. Of the other two forms, the form without the a coefficient had a lower RMSE and less mean bias and slope bias and was selected to estimate RE:
Mean bias (0.004) and slope bias (–0.012) were not significantly different from zero (RMSE = 0.186, CCC = 0.966). Validation of the model against literature values is presented in a later section.
Use of Metabolizable Energy for Retained Energy
To predict RE and growth from a quantity of ME available to the animal, the efficiency of ME use for RE must be known. The reported efficiency of ME use for RE varies from approximately 0.40 to more than 0.70, largely due to the age of the calf and whether it is accreting protein rapidly with minimal fat deposition or is actively fattening, as well as the dietary fat content relative to total ME and protein. Protein deposition is energetically expensive because of the adenosine triphosphate (ATP) costs for peptide bond formation but also because of concurrent protein turnover. In contrast, fat deposition is energetically much more efficient. van den Borne et al. (2007) demonstrated that body fat deposition does not originate from dietary carbohydrate in milk-fed calves but rather from dietary fat. This finding in preruminant calves means that they are similar to adult cattle since ruminants use little glucose carbon to form the carbon chains of fatty acids (FAs). Instead, glucose arising from lactose digestion is largely used to fuel protein synthesis (Roy et al., 1970; Donnelly, 1983; Tikofsky et al., 2001). The committee concluded that insufficient data were available to model the effects of the interrelationships of dietary fat content, total MEI, and dietary MP intake on the efficiency of ME use for RE because the resulting partitioning of RE into fat or lean tissue growth was not always predictable.
Because HP was modeled with a curvilinear relationship to MEI, by default, the relationship between RE and ME must also be curvilinear. However, the difference in RE/ME across the range of MEI encountered in practice is small, and this relationship is usually approximated as linear. Therefore, the use of ME for RE was calculated by regressing RE on MEI using Proc Mixed in SAS (v 9.4): RE = a + b × MEI, where RE and MEI are in Mcal/EBW0.75 per day, and a and b are equation parameters. Study was included as a random effect. The efficiency of use of the ME for weight gain (kg) was assumed to be the slope coefficient (b) of the regression of the RE as a function of MEI, according to Ferrell and Jenkins (1998b). The resulting efficiency for use of milk or MR ME was 0.46, which is much lower than the NRC (2001) value (0.69) and on the low end of previous literature estimates, most from heavier calves in veal-type settings. The equation from Toullec (1989) used by NRC (2001) was derived from veal calves at heavier BW where fat deposition would be greater, in contrast to the young and rapidly growing, leaner calves making up the current database. Labussiere et al. (2007) calculated an average efficiency of 0.64 from 12 previous studies that measured RE by comparative slaughter or calorimetry. In an extensive analysis of growth data, INRA (2019) determined that the efficiency of use of ME for RE was 0.55. Van Amburgh et al. (2019) adopted the value of 0.55 in an analysis of growth data. The current committee adopted the value of 0.55 for kg, which is also the average of the current database value and the summary of previous literature estimates (Labussiere et al., 2007).
The efficiency of ME use from starter is less than for milk components. Multiple regression analysis indicated that although a common intercept could be used for calves fed milk only or milk plus starter, the interaction of diet type and MEI was significant, indicating that efficiency of ME use for RE was different between milk only and milk plus starter. The committee adopted the modified equations from Galyean et al. (2016) to calculate RE/ME as the ratio of NE (i.e., RE) to dietary ME. The NEg was calculated as follows:
where ME is in Mcal/kg DM. Over the range of starter ME concentrations encountered in practice (i.e., 2.5 to 3.5 Mcal/kg), RE/ME varies from 0.378 to 0.441. These efficiencies also are lower than the 0.69 used by NRC (2001) but are consistent with calves depositing a greater proportion of RE as protein at this growth stage (INRA, 2019). Silva et al. (2017) reported results of a comparative slaughter study with Holstein or Holstein × Gyr crossbred calves in Brazil that were fed whole milk without or with starter. They found that the apparent RE/ME was 0.574 for calves fed whole milk and 0.516 for calves fed whole milk plus starter. Silva et al. (2017) calculated that the kg for starter alone would be 0.393, which is in the range of efficiencies predicted by the present model.
Equations to predict proportions of fat and protein in EBW gain were derived from the six Holstein studies in the data set according to NASEM (2016) methodology with study as a random effect:
At the mean RE for the data set (1.456 Mcal/d), predicted proportions of fat and protein in EBG are 0.132 and 0.181.
EFFECTS OF SOURCES OF ENERGY
Energy requirements are calculated as ME regardless of whether the source of ME is fat, carbohydrate, or protein. Nevertheless, source of energy may alter partitioning of nutrients, growth, and health.
Fats and Fatty Acids
Compared to adult dairy cows, surprisingly little published research has addressed digestibility of different fatty acid (FA) sources in MR, despite fat supplying 20 to >40 percent of total energy in MR. Typically, milk fat has been replaced with tallow, lard, and coconut oil, but restrictions on animal fat usage in animal feeds in many countries have shifted use to various blends of palm oil, rapeseed oil, and other hydrogenated vegetable oils. Huuskonen et al. (2005) replaced lard in MR with blends of 75 percent palm, 20 percent coconut, and 5 percent rapeseed oils or 75 percent palm and 25 percent coconut oils. Fat digestibility and calf gains were similar among all three diets. Overall, the paucity of data on intestinal digestibility of different FAs in young calves precludes efforts to model it. Given the importance of FAs to the ME of calf diets, continued research into FA digestibility and effects of dietary FA profile is warranted.
More studies have determined production and immune responses to altered dietary FA profiles in MR or starter. Bowen Yoho et al. (2013) compared pasteurized Jersey milk and MR containing fat that was 100:0, 80:20, or 60:40 lard/coconut oil fed to Jersey calves, and the MR with 20 percent coconut oil increased measures of stature but ADG did not differ. MR fortified with increased concentrations of butyrate (C4:0), medium-chain FAs (C8:0, C10:0, C12:0, C14:0), and essential polyunsaturated FAs (PUFAs; C18:3) increased ADG and improved gain/feed in calves less than 2 months of age (Hill et al., 2007a, 2009a). Subsequent studies confirmed these effects and showed that the FA blend enhanced some aspects of immune response when added to MR (Hill et al., 2011c; Esselburn et al., 2013). Given the complexity of the blend that was added, the specific FA or acids responsible for the enhanced growth and immune responses could not be determined. Ballou et al. (2008) and Ballou and DePeters (2008) fed Jersey calves MR in which 5 or 10 percent of the lipid was replaced with fish oil. Fish oil attenuated the acute phase response and modified several other indicators of immune function in a linear response to dose but did not affect growth or health of calves. Karcher et al. (2014) fed MR with 17 percent fat from lard, 15 percent lard plus 2 percent flax oil, or 15 percent lard plus 2 percent fish oil. The flax oil–supplemented MR resulted in greater ADG and feed efficiency, whereas fish oil had no effect. The flax oil diet also modulated some aspects of immune function in a beneficial direction.
Fat is often included in starters, usually in amounts less than 5 percent of DM. Several studies evaluated addition of fats to calf starters (discussed in Ghasemi et al., 2017) with variable results. Hill et al. (2007c) found that the same mixture of butyrate, medium-chain FAs, and C18:3 as used in MR (Hill et al., 2007a) improved growth and efficiency when added to the starter. Hill et al. (2009a) fed starter with calcium (Ca) salts of either flax oil (rich in C18:3) or fish oil (rich in long-chain n-3 FAs), and the Ca salts of flax oil, but not fish oil, increased ADG and feed efficiency in a dose-dependent manner in preweaned calves; the Ca salts of flax oil increased ADG and feed efficiency in postweaned calves.
Dairy cattle have tissue requirements for dietary essential (those that cannot be synthesized de novo) PUFAs, which are linoleic (C18:2) and linoleic (C18:3) acids. Despite extensive biohydrogenation in the rumen, enough of these FAs escape from the rumen so that dairy cows are not overtly deficient (Palmquist, 2009). Nevertheless, there is interest in optimizing supply of essential PUFAs and the ratio of the omega-6 (C18:2 and its elongation–desaturation products) to omega-3 (C18:3 and its elongation–desaturation products). While no requirements for C18:2 and C18:3 have been established for young calves, comparing MR with typical milk fat can be a starting point for adequacy. Standard milk fat contains 1 to 3 percent C18:2 and 0.5 to 2 percent C18:3 (Jensen et al., 1991), yielding typical C18:2 to C18:3 ratios of 4:1 to 6:1. Assuming a milk fat content of 3.8 percent and contents in fat of 2 percent C18:2 and 0.6 percent C18:3, a calf consuming 1 kg of milk solids daily takes in 5.1 g/d of C18:2 and 1.5 g/d of 18:3. MR containing 20 percent fat based on lard (~8.5 percent C18:2, 1.1 percent C18:3) or tallow (~4.5 percent C18:2, 0.8 percent C18:3) would supply similar amounts of these PUFAs. Based on the results summarized above, supplementation of sources of C18:3 to MR may have merit by narrowing the ratio of C18:2 to C18:3 as well as ensuring adequate daily intakes of these FAs.
Carbohydrates
The young calf lacks the digestive enzymes necessary to digest starch but has a high capacity to digest lactose. Although the capacity for lactose digestion has long been the subject of controversy (e.g., Roy, 1969), Gilbert et al. (2015) demonstrated that more than 97 percent of lactose disappears by the end of the ileum. Some of this could be attributable to fermentation (Tanan, 2005), but under most circumstances, enzymatic capacity does not limit lactose utilization in calves (Gilbert et al., 2015).
A substantial body of research has examined the ability of starch to replace some lactose in calf MR. Gilbert et al. (2015) substituted gelatinized starch, maltodextrins, branched maltodextrins, or maltose for lactose in increasing amounts. Apparent ileal disappearance was 61.6 percent and total tract disappearance over 99 percent. However, fermentation accounted for an amount equivalent to 89 percent of starch intake, with half of that fermentation occurring before the terminal ileum regardless of the starch product. Maltase activity may be limiting in vivo starch digestion (Gilbert et al., 2015). Thus, while small amounts of starch can replace lactose, much of its disappearance will be attributable to fermentation rather than enzymatic digestion (Tanan, 2005).
Other alternatives to lactose have been explored in young calves, including glucose, galactose, fructose, glycerol, and dextrins. Gilbert et al. (2016) replaced one-third of the lactose content of MR fed to male Holstein veal calves averaging 114 kg BW with glucose, fructose, or glycerol. The control MR contained 46 percent lactose. Energy and nitrogen (N) retention did not differ among treatments, although greater fecal losses were measured for fructose, and fructose was oxidized more slowly than glucose or glycerol.
Carbohydrates Versus Fats as Energy Source
The goal of early nutrition and growth may be different depending on the class of calf under consideration. For herd replacements, lean growth of frame (bone and muscle) is the primary concern, whereas for veal calves, early fattening is key. For male calves destined for feedlots, frame growth also is important in the calf stage. High rates of lean growth depend on nonlimiting quantities of protein as a source of essential amino acids (AAs) and sufficient available energy to drive protein synthesis. Gain of BW is affected most markedly by deposition of protein, which brings with it water in a 4:1 ratio. Roy (1980) described the relationship between BW gain and protein and fat deposition in equation form as follows:
In turn, body protein deposition responds in a linear fashion to increasing dietary protein over the range of practical feed intakes in calves (Gerrits et al., 1996; Bartlett et al., 2006). Gerrits et al. (1996) detected a plateau in body protein deposition with increasing dietary crude protein (CP) only in heavy veal calves (160–240 kg BW) at a digestible CP intake of 498 g/d when body protein deposition reached 244 g/d.
The optimal fuel to drive high rates of protein deposition has been the subject of debate. Tikofsky et al. (2001) found that increasing dietary fat intake in isonitrogenous and isocaloric diets increased fat deposition but did not change EBW gain or EB protein gain. Similar results were obtained by Roy et al. (1970) when fat was increased from 20 percent to 30 percent of the diet. Body fat does not originate from dietary carbohydrate in milk-fed calves (van den Borne et al., 2007), so if dietary protein is not limiting, extra energy from lactose will fuel more body protein deposition. In contrast, extra energy as fat may increase body fat deposition. Measurement of BW gain does not necessarily reflect all aspects of energy utilization. If the calf partitions dietary energy preferentially to body fat storage, an increase in RE might not appear as a corresponding increase in EBW gain. Overall, it is clear that carbohydrate oxidation rather than FA oxidation drives the majority of protein synthesis in young calves.
PROTEIN REQUIREMENTS
Similar to the previous edition, the model is driven on the basis of ME-allowable gain, with protein requirements calculated for maintenance and the predicted growth rate. In this edition, the committee adopted use of MP instead of apparently digestible protein (ADP) used in the previous edition (NRC, 2001).
Maintenance
Maintenance uses of MP constitute those losses that do not contribute to structural growth. Calculation of maintenance requirements was modified slightly from the previous version. An allowance for scurf (hair, skin, secretions) was added, calculated as follows:
(Equation 10-10)
where BW is in kg (Swanson, 1977). The calculation of endogenous urinary CP loss (EUCP) was adjusted slightly from the previous edition because of incongruities associated with use of BW0.75 according to Swanson (1977):
(Equation 10-11)
Calculation of metabolic fecal CP (MFP) remained the same as the previous edition:
(Equation 10-12)
where LFDMI is liquid feed DMI, and SFDMI is solid feed DMI.
Total maintenance net protein (NP) is the sum of EUCP, MFP, and scurf CP. To convert NP to MP, an assumed efficiency of 0.68 was adopted for scurf and MFP and 1.0 for EUCP, consistent with calculations for other classes of cattle (see Chapter 6).
(Equation 10-13)
Growth
NP for growth (NPg, g/d) as in the previous edition was calculated as the CP retained in the EBG but now is calculated as a function of the rate of gain and energy content of the gain as in the beef report (NASEM, 2016). The equation derived from the database of 255 individual calves from seven studies (Diaz et al., 2001; Tikofsky et al., 2001; Meyer, 2005; Bartlett et al., 2006; Bascom et al., 2007; Mills et al., 2010; Stamey Lanier et al., 2021) described earlier is the following:
(Equation 10-14)
In NRC (2001), the amount of N in gain (G) was constant at 30 g N/kg BW gain, which was roughly the average of a range of values (Blaxter and Wood, 1951; Roy, 1970; Donnelly and Hutton, 1976b; NRC, 1978; Davis and Drackley, 1998). However, this value is not constant but should be calculated based on the rate and composition of BW gain.
Efficiency of converting MPg to NPg decreases with age of calves (Labussiere et al., 2007). Rather than use a fixed correction, the committee adopted an empirical equation that decreased the efficiency of use from 0.70 at birth (6 percent of MatBW) to 0.55 at 200 kg BW (28 percent of MatBW):
(Equation 10-15)
This approach is based on literature estimates (summarized by Labussiere et al., 2007) showing that in young calves, the efficiency of MP or ADP use for BW gain was approximately 0.70, and data from heavy veal calves (Gerrits et al., 1996; Labussiere et al., 2008a) showed the efficiency was lower. Basing the adjustment on a proportion of MatBW allows use for both large-breed and small-breed calves. The equation is not intended to be used for animals greater than 200 kg and is not applied to ruminant heifers >125 kg BW (see Chapter 11).
In NRC (2001), efficiency of protein use was based on the biological value (BV) concept as used in the original calculations of ADP by Mitchell (1943). The BV of milk proteins, equated to the efficiency of N use for growth above maintenance, was assigned a value of 0.80 (Donnelly and Hutton, 1976a). The same factor was assumed to apply for efficiency of use of dietary protein for maintenance functions. This value was determined at limiting protein intakes and assumes that the diet being fed is properly balanced for all essential nutrients and that energy intake is sufficient to support protein synthesis. Protein intake must not be in excess of that required for the targeted gain allowed by energy intake. The BV decreased as protein intake was increased in the studies of Donnelly and Hutton (1976a). A value of 0.77 was used by NRC (1978). Studies by Terosky et al. (1997) found that apparent BV for MR containing 21 percent CP from skim milk protein, whey protein concentrate, or mixtures of the two ranged from 0.692 to 0.765. Estimates of true biological value (corrected for endogenous N loss and metabolic fecal N [MFN]) from that study are in excess of 0.80.
However, BV is meant to describe the relative protein quality of different sources when the protein is fed at limiting concentrations (Blaxter and Mitchell, 1948). Use of the efficiency values in the present edition more accurately reflects the utilization of AAs for growth at production intakes where energy and protein are designed to be approximately in balance.
Metabolizable Protein
Conversion of CP to MP uses a factor of 0.95 for milk or milk-derived ingredients, 0.75 for dietary proteins digested postruminally in the young calf fed both milk and starter, and a value of 0.70 for conversion of CP to MP for calves with a functioning rumen. For calves fed a combination of both milk or MR and starter, the conversion of CP to MP is an average of the efficiencies for the liquid diet (0.95) and for liquid plus starter (0.75) weighted by the amounts of protein provided from each source. The conversion of CP to MP at 0.95 for milk proteins agrees with literature data and other requirement systems (ARC, 1980) but is slightly higher than the value for conversion of dietary CP to absorbable AAs (0.91) used by NRC (1978). The value of 0.95 represents true digestibility of milk proteins in young calves. Because digestion of even high-quality milk proteins is immature during the first 2 to 3 weeks of age (Arieli et al., 1995; Terosky et al., 1997), the value of milk proteins may be overestimated during the early liquid-feeding period and may be underestimated for older calves. The committee concluded that information was insufficient to model age-related CP digestibility in the young calf. The value of 0.75 for calves fed milk or MR plus starter is retained from NRC (2001).
Requirements for MP and CP have been established on the basis of diets containing milk proteins with high digestibility and high BV; calves might not use alternative, nonmilk proteins in MR at these high efficiencies. When using nonmilk protein sources, the AA profile should be considered, and the AA most likely to be limiting (lysine [Lys], methionine [Met], threonine [Thr]; Williams and Hewitt, 1979) should be supplemented to the levels found in milk proteins (Hill et al., 2008c). In addition, vegetable proteins may increase endogenous CP flows in the intestine that would decrease apparent fecal or intestinal digestibilities of CP (Lallès, 1993). Montagne et al. (2001) measured endogenous flows of CP at the ileum and found that nonmilk proteins increased ileal CP flow with resulting decreases in apparent digestibility relative to a skim milk–based diet. Apparent digestibilities of CP at the ileum were 0.85 with soy protein concentrate, 0.73 with soy protein isolate, and 0.81 with potato concentrate compared with 0.91 with skim milk powder. After adjustment for the increased endogenous losses, real digestibilities (i.e., after correction for both specific and nonspecific endogenous losses) of the proteins were 0.96, 0.95, 0.94, and 0.99, respectively. Such endogenous losses necessitate recalculation of the endogenous N loss to 4 to 7 g N per kilogram of DMI from MR to provide a more correct estimate of true protein digestibility. Greater MFN loss places an additional maintenance cost on energy as well, although the value for this additional energetic cost is not easily estimated. In the model, users have the option to specify that an MR contains vegetable proteins, which changes the calculation of MFP (but not energy) to 34.4 g/kg of DMI.
Rumen Microbial Protein
Developing rumen function has profound effects on the supply of nutrients to the calf. Resident bacteria ferment ingested starter and forage and produce VFAs and microbial crude protein (MCP), which becomes a source of AAs for the calf. Increasing fermentation occurs with increasing intake of fermentable carbohydrate, so that the flow of microbial N becomes a greater proportion of the total N reaching the intestine (Leibholz, 1975, 1978; Quigley et al., 1985; Lallès and Poncet, 1990; Obitsu et al., 1995). Quigley and Schwab (1988) reported a high correlation (r = 0.92) between calf starter intake and percentage of N as microbial N in abomasal contents of calves from 2 to 11 weeks of age, suggesting that the key driver to changing the nature of abomasal N was intake of dry feed.
A meta-analysis was conducted using studies that reported the ratio of microbial N to total N in abomasal or duodenal content of calves from 2 to 20 weeks of age (see Table 10-3). A total of 66 observations were used in the analysis using the techniques outlined by St-Pierre (2001). A subsequent broken-line regression analysis was conducted using methods by Robbins et al. (2006) to determine the DMI at which no further increase in microbial contribution as a proportion of total N occurred. Microbial N as a proportion of total N increased with increasing DMI to 1.3 kg/d; thereafter, microbial N contribution was constant, as defined by the broken-line regression (see Figure 10-2). Thus, once starter intake reaches 1.3 kg/d, the proportion of total CP reaching the intestine for digestion that is of microbial origin will be maximized.

FIGURE 10-2
Broken-line regression (A) and residuals (B) of contribution of microbial N to total N to dry feed DMI in calves from 0 to 20 weeks of age. Data derived from meta-analysis of four published studies. The breakpoint occurred at 1.32 kg/d (SE = 0.118), resulting (more...)
TABLE 10-3Studies Comprising the Data Used to Relate DMI to Microbial N Flow
Reference | N | Forage (%) | Age (Weeks) | DMI (kg/d) | MNa (%) |
---|---|---|---|---|---|
Lallès and Poncet, 1990 | 6 | 20 | 9–20 | 0.8–2.4 | 60–68 |
Leibholz, 1975 | 8 | 15 | 6–13 | 0.6–2.5 | 32–74 |
Leibholz, 1978 | 5 | 15 | 6–10 | 0.8–1.8 | 28–70 |
Obitsu et al., 1995 | 8 | 30–40 | 10–15 | 1.5–2.4 | 48–64 |
Quigley et al., 1985 | 39 | 0/ad libitumb | 2–11 | 0.1–3.1 | 6–83 |
- a
Microbial N flow as percentage of total N flow at the abomasum or duodenum.
- b
Half of calves had ad libitum access to long grass hay.
Based on these data, conversion efficiency of CP to MP for ruminating calves consuming starter is set at 0.70 (NRC, 1978). Insufficient data were available to allow calculations of the amounts of rumen-degradable protein or rumen-undegradable protein (RUP) supplied with any degree of confidence. However, assuming that N flow to the abomasum approximates N intake, that microbial CP is 80 percent true protein that is 80 percent digestible (see Chapter 6), and that undegraded feed proteins are 0.80 digestible (NRC, 1989) leads to a conversion of CP to MP of about 0.71. The efficiency used in this edition (0.70) is slightly lower than the value of 0.75 from ARC (1980) used in the last version of this publication (NRC, 2001). Use of the lower value provides more consistent predictions of literature values. Conversions of CP to MP for calves fed starter and milk or starter and MR are assumed to be additive based on the relative amounts of CP supplied by starter and milk (or MR).
CALCULATION OF METABOLIZABLE ENERGY VALUES OF FEEDS
Milk, MR, and ingredients used in MR use different coefficients for digestibility and a different method for calculating ME than solid feeds. When users select “calf” as the target animal, ingredients listed under “calf feeds” must be used for the liquid feeds in the diet. Starter feeds and ingredients used to make starter feeds follow the protocols set out for feeds for other classes of cattle as described in Chapters 3 and 19. Composition data are shown for the most common ingredients used in manufacture of MR in Table 10-4.
The ME values of liquid feeds are calculated similarly to the previous edition with modifications. First, the gross energy (GE) of the feed is calculated by multiplying the percentage composition on a DM basis by the respective heats of combustion, according to the following formula:
(Equation 10-16)
where values are on a DM basis. FA concentration is better than crude fat (CF) for nutritional characterization (see Chapter 4) of feeds, and it is used in Equation 10-16; however, many feed labels such as those on MR are based on CF. CF from ingredients commonly used in MRs can be converted to FA by multiplying CF by 0.945 (Paul and Southgate, 1978). Feeds can contain other organic compounds such as partially hydrolyzed starch, dextrins, glucose, or glycerol that may be incorporated in small amounts (usually less than 10 percent of DM in aggregate) into MR. This fraction is assumed to have the same heat of combustion as lactose (4 Mcal/kg). Values for whole milk are determined similarly after converting the composition to a DM basis.
TABLE 10-4Composition of Some Common Ingredients Used in the Manufacture of MRs
Feed or Ingredient | DM (%) | DE (Mcal/kg DM) | ME (Mcal/kg DM) | Ash (% of DM) | CP (% of DM) | CF (% of DM) | Lactose (% of DM) |
---|---|---|---|---|---|---|---|
Whole milk | 12.5 | 5.59 | 5.37 | 6.3 | 25.4 | 30.8 | 39.2 |
Skim milk, fresh | 10 | 4.19 | 4.02 | 6.9 | 35.5 | 0.3 | 56 |
Skim milk, powder | 94 | 4.25 | 4.08 | 6.9 | 37.4 | 1.0 | 54 |
Whey, dried | 93 | 3.80 | 3.65 | 8.1 | 13.5 | 1.0 | 76 |
Whey protein concentrate | 93 | 4.35 | 4.17 | 6.0 | 37.1 | 2.2 | 54 |
Whey, fresh | 7 | 3.78 | 3.62 | 8.7 | 14.2 | 0.7 | 76 |
Whey, delactosed | 93 | 3.54 | 3.40 | 16.5 | 23.0 | 1.5 | 55 |
Whey permeate | 98 | 3.55 | 3.41 | 9.0 | 3.7 | 0 | 87 |
Casein | 91 | 5.29 | 5.08 | 4.0 | 92.7 | 0.7 | — |
Caseinate, sodium | 96 | 2.5 | 85 | 0.5 | — | ||
Soy protein concentrate | 96 | 7.0 | 67 | 0.3 | — | ||
Soy protein isolate | 95 | 4.5 | 86 | 0.5 | — | ||
Soy flour | 96 | 6.3 | 53 | 0.2 | — | ||
Modified wheat protein | 96 | 3.0 | 82 | 2.0 | — | ||
Porcine plasma protein | 97 | 67 | 0.5 | — | |||
Bovine plasma protein | 97 | 68 | 0.5 | — |
Ash content normally is not listed on feed tags but generally will be 6 to 12 percent of total MR DM. Because ash has no energy, it affects the ME value and should always be determined analytically. Users are cautioned that feed tag values for MR components are given on an “as fed” or air-dry basis, which for MR is usually 95 to 97 percent DM. Failure to account for this residual moisture will introduce error into the calculation of ME.
The ME values for MR then are derived by multiplying the gross energy content by 0.91, which is the product of the average digestibility (0.95) and metabolizability of the digestible energy (DE) (0.96) for MR (Gerrits et al., 1996; Diaz et al., 2001; Blome et al., 2003; Labussiere et al., 2007, 2008a). For whole milk, the GE is multiplied by 0.93 because of the slightly higher digestibility for milk (0.97; NRC, 2001).
The DE values for solid feeds are calculated as in Chapter 3 with the exception that the digestibility coefficient for fat is assumed to be 0.81 rather than 0.74 as for older cattle. The coefficient of 0.81 for fat digestibility represents the average of studies that measured digestibilities for CF in weaned calves (see Table 10-5). The DE was calculated without discounting for intake or starch concentration (i.e., intake was set at 3.5 percent of BW and dietary starch was assumed to be 25 percent in equations). The efficiency of converting DE to ME by young calves fed MR and various starters (Pattanaik et al., 2003) varied from 0.91 to 0.95; therefore, the ME of dry feeds was set at DE × 0.93. To derive accurate estimates, starter should be analyzed as described in Chapter 3.
TABLE 10-5Summary of Studies in Which CF Digestibility Was Measured in Weaned Calves (Seven Studies, 37 Treatment Means)a
Mean | Range | SD | |
---|---|---|---|
BW, kg | 98.7 | 63–135 | 24.6 |
Age, days | 76 | 51–112 | 24.8 |
DMI, kg/d | 2.5 | 1.3–4.3 | 0.76 |
Dietary fat, % of DM | 4.0 | 2.2–5.1 | 0.69 |
Fat digestibility | 0.81 | 0.70–0.91 | 0.05 |
- a
Sources of data: Chapman et al. (2016); Dennis et al. (2018); Hill et al. (2010, 2016b, 2016c); Hu et al. (2019); Stewart and Schingoethe (1984).
For calves to achieve the calculated ME values, the rumen must be sufficiently developed to support near-mature rumen fermentation, both in terms of microbiota and rumen epithelial (papillae) development. In calves in which the development is not complete, digestibilities will be lower, particularly for neutral detergent fiber (NDF) (Terre et al., 2007; Hill et al., 2010; Chapman et al., 2016). This situation may be a problem when calves are fed large amounts of milk or MR early in life, which limits the intake of calf starter. If such calves are weaned too early, rumen development may be incomplete so that the ME obtained by the calf is less than estimated. While rumen development depends on roughly 3 weeks of starter consumption, Quigley et al. (2019b) found that the cumulative intake of nonstructural carbohydrates was most highly related to digestion and achievement of predicted ME values. Because this variable will be difficult to determine on farm, the committee has incorporated a somewhat arbitrary discount of 10 percent of calculated ME of starter for preweaned calves consuming ≥1.5 percent of BW as milk or MR solids. The option to use this adjustment can be turned on or off by the user within the computer software.
VALIDATION OF MODEL WITH EXPERIMENTAL DATA
To evaluate the ability of the model to predict values from the literature, the committee assembled a database of 416 treatment means from 94 published studies that provided enough information to estimate MEIs, BW, and BW gains (see Table 10-6). Studies included some that were conducted before the previous NRC (2001) document as well as those conducted since and represented a range of milk or MR intakes, starter intakes, and ADG.
Studies included calves fed milk or MR only (21 studies), calves fed milk or MR plus starter without or with forage (64 studies), and weaned calves (23 studies). The ME densities of liquid and solid feeds were calculated according to the methods described in this chapter, including discounted ME for starter when early milk intakes were greater than 1.5 percent of BW. Mean BW was calculated as the average of BW at the beginning of the growth period and BW at the end of the period, and ADG was calculated for that period. Maintenance ME was calculated, including any requirements for thermoregulation, which was subtracted from total MEI to yield ME for gain. The MEg was multiplied by the efficiency of ME use for gain, resulting in NE (i.e., RE). Rearrangement of Equation 10-6 as shown in Equation 10-17 allowed calculation of both ME-allowable and MP-allowable EBW gain and then ADG:
(Equation 10-17)
The more limiting of ME-allowable or MP-allowable ADG was compared with the actual ADG reported in the studies. The regression of observed study ADG on model predicted ADG (pADG), with study as a random effect, resulted in the following equation:
(Equation 10-18)
This model resulted in a mean predicted value of 0.679 kg/d compared with an observed mean of 0.689 kg/d, with an RMSE of 0.110 kg/d (15.9 percent of the mean). The plots of observed versus predicted values and residuals are shown in Figure 10-3. The model RMSEP was 0.110. The CCC was 0.95. The model, therefore, was robust in predicting calf growth.

FIGURE 10-3
Observed minus predicted values for ADG from 397 literature treatment means, with residuals plotted. The ADGs were predicted with Equation 10-18, and the studies used (see Table 10-6) represented a wide range of years published, amounts of milk or MR (more...)
TABLE 10-6Studies Used to Validate Prediction Models
A comparison of ADG predicted by Equation 10-18 with ADG predicted by the equation from the previous edition (NRC, 2001) was made for a randomly chosen subset of 111 means from the studies listed in Table 10-6. Predictions of observed ADG by the current model and by the model from NRC (2001) are shown in Figure 10-4. For this subset of studies, the regression of observed versus predicted values using Equation 10-18 was 0.98x + 0.01, with an RMSE of 0.12, an AIC of –171.0, and a CCC of 0.93. For the NRC (2001) prediction, the equation was 1.01x – 0.09, with an RMSE of 0.16, an AIC of –120, and a CCC of 0.85. The NRC (2001) model showed significant mean bias (21.6 percent of MSE). Therefore, the model fit and predictions in the current edition are an improvement over the previous model (NRC, 2001), particularly for calves at low ADG and for those receiving both milk or MR and starter.

FIGURE 10-4
Comparison of actual mean ADG from 111 treatment means from the literature with values predicted by the current model or the previous (NRC, 2001) model.
ENERGY AND PROTEIN REQUIREMENTS FOR CALVES
Examples of requirements for ME and MP for various classes of calves are in Tables 10-7 through 10-11. The DMI listed in the tables has been computed as the amount of DM necessary to meet the ME requirement. Consequently, these should not be construed as predictions of voluntary feed intake, which was discussed in a previous section.
TABLE 10-7Daily Energy and Protein Requirements of Young Replacement Calves Fed Only Milk or MR
BW (kg) | Breeda | ADG (g/d) | DMIb (kg/d) | ME (Mcal/d) | NEm (Mcal/d) | MP (g/d) | CP c (g/d) | CP (% of DMI) |
---|---|---|---|---|---|---|---|---|
25 | SB | 200 | 0.36 | 1.69 | 0.82 | 83 | 87 | 24.2 |
400 | 0.49 | 2.33 | 0.82 | 132 | 139 | 28.2 | ||
30 | SB | 200 | 0.40 | 1.88 | 0.94 | 86 | 91 | 22.7 |
400 | 0.54 | 2.54 | 0.94 | 136 | 143 | 26.6 | ||
600 | 0.69 | 3.23 | 0.94 | 186 | 196 | 28.5 | ||
35 | SB | 200 | 0.44 | 2.06 | 1.06 | 89 | 94 | 21.4 |
400 | 0.58 | 2.74 | 1.06 | 140 | 147 | 25.2 | ||
600 | 0.73 | 3.45 | 1.06 | 190 | 200 | 27.2 | ||
800 | 0.89 | 4.19 | 1.06 | 240 | 253 | 28.3 | ||
40 | LB | 200 | 0.48 | 2.23 | 1.17 | 91 | 96 | 19.8 |
400 | 0.64 | 2.93 | 1.17 | 142 | 149 | 23.4 | ||
600 | 0.80 | 3.66 | 1.17 | 192 | 202 | 25.4 | ||
800 | 0.96 | 4.42 | 1.17 | 242 | 254 | 26.5 | ||
45 | LB | 200 | 0.52 | 2.40 | 1.28 | 94 | 99 | 19.0 |
400 | 0.68 | 3.11 | 1.28 | 145 | 152 | 22.5 | ||
600 | 0.84 | 3.86 | 1.28 | 195 | 205 | 24.5 | ||
800 | 1.01 | 4.64 | 1.28 | 245 | 258 | 25.6 | ||
50 | LB | 200 | 0.56 | 2.56 | 1.38 | 97 | 102 | 18.3 |
400 | 0.71 | 3.29 | 1.38 | 148 | 155 | 21.8 | ||
600 | 0.88 | 4.05 | 1.38 | 198 | 209 | 23.7 | ||
800 | 1.05 | 4.85 | 1.38 | 249 | 262 | 24.9 | ||
1,000 | 1.23 | 5.66 | 1.38 | 299 | 315 | 25.6 |
- a
SB = small breed (based on Jersey, MatBW = 530 kg) and LB = large breed (based on Holstein, MatBW = 700 kg).
- b
DMI necessary to meet requirement for ME when fed milk replacer containing 4.7 Mcal ME/kg of DM (SB calves) or 4.6 ME/kg of DM (LB calves).
- c
Assumes all milk protein with MP/CP of 0.95.
Young Replacement Calves Fed Milk or Milk Replacer Only
The energy requirements of young large-breed and small-breed calves fed only milk or MR and weighing 25 to 50 kg are given in Table 10-7. Users who desire requirements for higher rates of gain should refer to Table 10-11. The energy content of BW gain predicted by Equation 10-6 is 1.62 Mcal/kg for a 40-kg calf gaining 0.20 kg/d and 2.11 Mcal/kg for a 75-kg calf gaining 0.80 kg/d. Values predicted by this equation are similar to those predicted by NRC (2001) for the smaller calf (1.56 Mcal/kg) but are 18 percent lower for the larger calf gaining more rapidly (2.57 Mcal/kg BW gain). The ME requirements for the 40-kg calf gaining 0.20 kg/d (2.20 Mcal/d) and the 75-kg calf gaining 0.80 kg/d (5.66 Mcal/d) predicted by the current equations compare with 2.04 Mcal/d and 5.52 Mcal/d, respectively, predicted by NRC (2001). The current edition predicts lower ADG for a given intake than the previous edition. In the database of literature studies used to validate the current models, there were 103 treatment means from 21 studies. The regression (St-Pierre, 2001) of observed values on predicted values (kg/d) for ADG was as follows: Observed ADG = 0.070 + 0.903 × Predicted ADG.
Users should be aware that ME requirements for maintenance may be underestimated for calves during the first week of life because of the high and variable basal metabolic rate observed during this time (Roy et al., 1957; Gonzalez-Jimenez and Blaxter, 1962; Vermorel et al., 1983; Okamoto et al., 1986; Schrama et al., 1992b; Ortigues et al., 1994; Arieli et al., 1995). Furthermore, because the digestive tract is immature and developing rapidly, the digestibility of diets may be lower during this time (Schrama et al., 1992b; Arieli et al., 1995; LianG et al., 2016), thereby overestimating dietary energy supply. The net result of these effects is that ADG of calves during the first week of life may be considerably less than the predicted energy-allowable gains shown in Table 10-7.
Young Calves Fed Milk and Starter Feed or Milk Replacer and Starter Feed
Under good management, calves should be consuming appreciable nutrients from starter feed by the second week of life. To encourage early consumption of calf starter, calves should be given free access to water and a nutritious, highly palatable starter from the first week of life until they are weaned. Consumption of starter is critical to development of an active, functioning rumen. Fermentation products, principally butyrate, from fermentation of solid feeds in the developing rumen are responsible for development of functional ruminal epithelial tissue (Sander et al., 1959).
TABLE 10-8Daily Energy and Protein Requirements of Small-Breed Calves Fed Milk or MR and Starter at Two Different Ratiosa
BW (kg) | ADG (g/d) | Diet | DMIb (kg/d) | ME (Mcal/d) | NEm (Mcal/d) | MP (g/d) | CP c (g/d) | CP (% of DMI) |
---|---|---|---|---|---|---|---|---|
30 | 200 | 80:20d | 0.43 | 1.96 | 0.93 | 86 | 94 | 21.8 |
200 | 40:60e | 0.53 | 2.02 | 0.93 | 91 | 109 | 20.6 | |
400 | 80:20 | 0.58 | 2.62 | 0.93 | 135 | 148 | 25.6 | |
400 | 40:60 | 0.72 | 2.75 | 0.93 | 142 | 170 | 23.6 | |
600 | 80:20 | 0.73 | 3.32 | 0.93 | 184 | 202 | 27.6 | |
600 | 40:60 | 0.93 | 3.52 | 0.93 | 192 | 232 | 25.0 | |
40 | 200 | 80:20 | 0.51 | 2.33 | 1.16 | 92 | 101 | 19.7 |
200 | 40:60 | 0.63 | 2.39 | 1.16 | 98 | 118 | 18.7 | |
400 | 80:20 | 0.67 | 3.03 | 1.16 | 142 | 156 | 23.4 | |
400 | 40:60 | 0.83 | 3.16 | 1.16 | 150 | 180 | 21.7 | |
600 | 80:20 | 0.83 | 3.77 | 1.16 | 192 | 211 | 25.4 | |
600 | 40:60 | 1.05 | 3.98 | 1.16 | 202 | 243 | 23.2 | |
800 | 80:20 | 1.00 | 4.54 | 1.16 | 241 | 265 | 26.5 | |
800 | 40:60 | 1.27 | 4.82 | 1.16 | 253 | 305 | 24.0 | |
50 | 400 | 80:20 | 0.75 | 3.41 | 1.37 | 149 | 163 | 21.8 |
400 | 40:60 | 0.93 | 3.54 | 1.37 | 157 | 189 | 20.3 | |
600 | 80:20 | 0.92 | 4.18 | 1.37 | 199 | 219 | 23.8 | |
600 | 40:60 | 1.16 | 4.40 | 1.37 | 210 | 253 | 21.9 | |
800 | 80:20 | 1.10 | 4.98 | 1.37 | 250 | 274 | 25.0 | |
800 | 40:60 | 1.39 | 5.28 | 1.37 | 263 | 317 | 22.8 | |
1,000 | 80:20 | 1.28 | 5.80 | 1.37 | 300 | 330 | 25.8 | |
1,000 | 40:60 | 1.63 | 6.19 | 1.37 | 316 | 380 | 23.4 | |
60 | 400 | 80:20 | 0.83 | 3.75 | 1.57 | 155 | 170 | 20.6 |
400 | 40:60 | 1.03 | 3.90 | 1.57 | 164 | 198 | 19.3 | |
600 | 80:20 | 1.00 | 4.56 | 1.57 | 206 | 227 | 22.6 | |
600 | 40:60 | 1.26 | 4.79 | 1.57 | 218 | 263 | 20.9 | |
800 | 80:20 | 1.19 | 5.39 | 1.57 | 258 | 283 | 23.8 | |
800 | 40:60 | 1.50 | 5.70 | 1.57 | 272 | 328 | 21.8 | |
1,000 | 80:20 | 1.38 | 6.25 | 1.57 | 309 | 340 | 24.7 | |
1,000 | 40:60 | 1.75 | 6.64 | 1.57 | 326 | 392 | 22.4 |
- a
Expressed as proportion of DM from MR to proportion of DM from starter.
- b
Total DMI with mean ME density needed to meet ME requirements.
- c
Total dietary CP needed, assuming all-milk protein MR.
- d
Assumes MR contains 4.9 Mcal ME/kg DM and starter contains 3.1 Mcal ME/kg DM.
- e
Assumes MR contains 4.7 Mcal ME/kg DM and starter contains 3.2 Mcal ME/kg DM.
Efficiencies of utilization of ME for maintenance and gain will be somewhat lower for starter feeds than for milk or MR (NRC, 1978). In the current edition, the committee has returned to the use of the equations of Garrett (1980), as updated by Galyean et al. (2016), to derive the efficiencies of utilization of ME from starter for maintenance (km) and gain (kg). The efficiency of use of ME from the total diet is the average of individual efficiencies for milk or MR and starter, weighted according to their contribution to the total ME in the diet (see Tables 10-8 and 10-9). The computer model included with this edition calculates these values for varied proportions of DMI from milk and starter or MR and starter.
The ME requirement (Mcal/d) of a 50-kg large-breed calf gaining 0.60 kg/d when fed only milk or MR (see Table 10-7) is 3.89 compared to 4.18 and 4.40 for the same calf obtaining 80 and 40 percent of her DM from MR (see Table 10-8). The ME requirements for calves consuming both starter and MR are higher than those in the 2001 edition (NRC, 2001), but the relationship between the current model predictions and literature data is robust. From the literature database, with 219 treatment means from 64 studies, the equation was as follows: Observed ADG = 0.112 + 0.773 × Predicted ADG. In most cases, ME, and not MP, limited growth.
Calves from Weaning to Body Weight of 125 kg
Since the publication of NRC (2001), a few studies have provided information about body composition of weaned calves (Brown et al., 2005b; Meyer, 2005; Stamey Lanier et al., 2021), and many more have provided data on intake and growth rates. Requirements have been derived using the same methodology as described for younger calves (see Table 10-10). Comparison of literature data for ADG (79 treatment means from 23 published studies) with model predictions yielded the following relationship: Observed ADG = 0.366 + 0.662 × Predicted ADG. The large intercept of the equation indicates that the model underpredicts growth at low growth rates and overpredicts growth at high growth rates. In the absence of more comparative slaughter studies with calves of this weight range, however, the committee was not able to derive an equation with less slope bias.
TABLE 10-9Daily Energy and Protein Requirements of Large-Breed Calves Fed Milk or MR and Starter at Two Different Ratiosa
BW (kg) | ADG (g/d) | Diet | DMIb (kg/d) | ME (Mcal/d) | NEm (Mcal/d) | MP (g/d) | CPc (g/d) | CP (% of DMI) |
---|---|---|---|---|---|---|---|---|
50 | 400 | 80:20d | 0.79 | 3.40 | 1.37 | 147 | 162 | 20.6 |
400 | 40:60e | 0.93 | 3.54 | 1.37 | 155 | 187 | 20.0 | |
600 | 80:20 | 0.97 | 4.18 | 1.37 | 197 | 217 | 22.4 | |
600 | 40:60 | 1.16 | 4.40 | 1.37 | 207 | 250 | 21.6 | |
800 | 80:20 | 1.15 | 4.98 | 1.37 | 247 | 271 | 23.6 | |
800 | 40:60 | 1.39 | 5.28 | 1.37 | 259 | 312 | 22.5 | |
60 | 400 | 80:20 | 0.87 | 3.75 | 1.57 | 153 | 168 | 19.4 |
400 | 40:60 | 1.03 | 3.90 | 1.57 | 162 | 195 | 19.0 | |
600 | 80:20 | 1.05 | 4.55 | 1.57 | 204 | 224 | 21.2 | |
600 | 40:60 | 1.26 | 4.79 | 1.57 | 214 | 258 | 20.5 | |
800 | 80:20 | 1.25 | 5.38 | 1.57 | 254 | 279 | 22.4 | |
800 | 40:60 | 1.50 | 5.70 | 1.57 | 267 | 322 | 21.4 | |
1,000 | 80:20 | 1.44 | 6.24 | 1.57 | 305 | 335 | 23.2 | |
1,000 | 40:60 | 1.75 | 6.64 | 1.57 | 320 | 386 | 22.0 | |
70 | 400 | 80:20 | 0.94 | 4.08 | 1.76 | 159 | 174 | 18.4 |
400 | 40:60 | 1.11 | 4.24 | 1.76 | 168 | 202 | 18.1 | |
600 | 80:20 | 1.14 | 4.91 | 1.76 | 210 | 231 | 20.3 | |
600 | 40:60 | 1.35 | 5.15 | 1.76 | 221 | 267 | 19.7 | |
800 | 80:20 | 1.33 | 5.77 | 1.76 | 261 | 287 | 21.5 | |
800 | 40:60 | 1.60 | 6.10 | 1.76 | 275 | 332 | 20.6 | |
1,000 | 80:20 | 1.54 | 6.65 | 1.76 | 312 | 343 | 22.3 | |
1,000 | 40:60 | 1.86 | 7.07 | 1.76 | 328 | 396 | 21.3 | |
80 | 600 | 80:20 | 1.21 | 5.25 | 1.90 | 216 | 237 | 19.5 |
600 | 40:60 | 1.45 | 5.50 | 1.90 | 228 | 275 | 19.0 | |
800 | 80:20 | 1.42 | 6.13 | 1.90 | 268 | 294 | 20.7 | |
800 | 40:60 | 1.70 | 6.47 | 1.90 | 282 | 340 | 20.0 | |
1,000 | 80:20 | 1.63 | 7.03 | 1.90 | 320 | 351 | 21.6 | |
1,000 | 40:60 | 1.96 | 7.47 | 1.90 | 337 | 406 | 20.6 |
- a
Expressed as proportion of DM from MR to proportion of DM from starter.
- b
Total DMI with mean ME density needed to meet ME requirements.
- c
Total dietary CP needed, assuming all-milk protein MR.
- d
Assumes MR contains 4.6 Mcal ME/kg DM and starter contains 3.2 Mcal ME/kg DM.
- e
Assumes MR contains 4.7 Mcal ME/kg DM and starter contains 3.2 Mcal ME/kg DM.
Veal Calves
The calculations used to derive the ME requirements for veal calves (see Table 10-11) are the same as those for milk-fed replacement calves (see Table 10-7) with the exception that EBW/ADG gain is set at 0.91, as described in an earlier section. Predicted requirements agree closely with experimental data for veal calf growth, even at BW greater than those in the database. Veal calves are fed at rates approaching ad libitum intake, so rates of gain will be higher than those of limit-fed replacement calves. The ME, MP, and DM requirements given here agree closely with data in the literature (Gerrits et al., 1996; van den Borne et al., 2006; Labussiere et al., 2011). Current systems of veal production in many areas provide small amounts of solid feed in addition to high intakes of milk (Suárez et al., 2006; Labussiere et al., 2009). Requirements for these calves can be estimated by using the principles established for calves fed both milk and solid feeds.
MINERAL AND VITAMIN REQUIREMENTS OF CALVES
Minerals
Detailed information on the roles of minerals is given in Chapter 7. Mineral absorption and factors affecting mineral absorption are also discussed in Chapter 7; however, that discussion emphasizes absorption by functioning ruminants. Clear differences in mineral absorption between preruminant calves and older cattle have been shown for many minerals likely because of both diet and physiology. In the previous edition (NRC, 2001), mineral recommendations were presented on a dietary concentration basis, and those recommendations were largely unchanged from NRC (1989).
TABLE 10-10Daily Energy and Protein Requirements of Weaned Large- or Small-Breed Calves Fed Only Solid Feeds
BW (kg) | ADG (g/d) | DMIa (kg/d) | ME (Mcal/d) | NEm (Mcal/d) | MP (g/d) | CP (g/d) | CP (% of DMI) |
---|---|---|---|---|---|---|---|
55 | 400 | 1.465 | 4.394 | 1.734 | 174.3 | 249.0 | 17.0 |
600 | 1.768 | 5.305 | 1.734 | 227.9 | 325.6 | 18.4 | |
800 | 2.083 | 6.248 | 1.734 | 281.6 | 402.3 | 19.3 | |
65 | 400 | 1.607 | 4.820 | 1.966 | 183.1 | 261.6 | 16.3 |
600 | 1.921 | 5.763 | 1.966 | 237.7 | 339.6 | 17.7 | |
800 | 2.246 | 6.739 | 1.966 | 292.5 | 417.9 | 18.6 | |
75 | 400 | 1.742 | 5.226 | 2.188 | 191.5 | 273.6 | 15.7 |
600 | 2.066 | 6.197 | 2.188 | 247.2 | 353.1 | 17.1 | |
800 | 2.401 | 7.202 | 2.188 | 303.0 | 432.9 | 18.0 | |
85 | 600 | 2.204 | 6.612 | 2.404 | 251.2 | 358.8 | 16.3 |
800 | 2.548 | 7.643 | 2.404 | 306.5 | 437.9 | 17.2 | |
1,000 | 2.900 | 8.700 | 2.404 | 362.0 | 517.1 | 17.8 | |
95 | 600 | 2.337 | 7.010 | 2.613 | 259.4 | 370.5 | 15.9 |
800 | 2.688 | 8.065 | 2.613 | 315.5 | 450.7 | 16.8 | |
1,000 | 3.049 | 9.146 | 2.613 | 371.7 | 531.0 | 17.4 | |
105 | 600 | 2.465 | 7.394 | 2.817 | 267.3 | 381.9 | 15.5 |
800 | 2.824 | 8.471 | 2.817 | 324.2 | 463.1 | 16.4 | |
1,000 | 3.192 | 9.575 | 2.817 | 381.2 | 544.6 | 17.1 | |
1,200 | 3.567 | 10.701 | 2.817 | 438.4 | 626.3 | 17.6 | |
115 | 600 | 2.589 | 7.766 | 3.015 | 275.1 | 393.0 | 15.2 |
800 | 2.954 | 8.863 | 3.015 | 332.8 | 475.4 | 16.1 | |
1,000 | 3.329 | 9.988 | 3.015 | 390.6 | 558.0 | 16.8 | |
1,200 | 3.712 | 11.136 | 3.015 | 448.6 | 640.8 | 17.3 | |
125 | 600 | 2.709 | 8.128 | 3.210 | 282.7 | 403.9 | 14.9 |
800 | 3.081 | 9.244 | 3.210 | 341.2 | 487.4 | 15.8 | |
1,000 | 3.463 | 10.388 | 3.210 | 399.8 | 571.1 | 16.5 | |
1,200 | 3.852 | 11.556 | 3.210 | 458.6 | 655.1 | 17.0 | |
1,400 | 4.248 | 12.743 | 3.210 | 517.4 | 739.2 | 17.4 |
- a
Assumes starter contains 3.0 Mcal ME/kg DM.
The committee took a more quantitative approach to establishing minerals recommendations than in the past; however, these recommendations should be considered as Adequate Intakes (AIs) rather than requirements. AIs for calcium (Ca), phosphorus (P), and magnesium (Mg) in g/d of total diet were calculated using equations from Castro et al. (2019). Their equations were derived from data from multiple studies with preweaned calves (Holstein and Holstein × Gyr cross) that used the comparative slaughter technique. Calves in four of those studies were fed milk and starter, and in one study, calves were fed only MR. Breed generally did not affect results. In the equations below, the value in the denominator is the experimentally derived retention coefficient that converts retained mineral to dietary mineral. Those coefficients likely will be influenced by source of mineral, but the committee assumed that those coefficients will be reasonably accurate for mixed diets of liquid and solid feed for preweaned calves. Absorption coefficients (ACs) are not used for macrominerals for young calves.
Dietary AIs are calculated as follows:
(Equation 10-19)
(Equation 10-20)
(Equation 10-21)
(Equation 10-22)
(Equation 10-23)
For these equations, empty BW (EBW) and daily empty body gain (EBG) equal 0.94 × BW and 0.91 × ADG in kilograms. K is potassium and Na is sodium.
Essentially no information is available on the chloride (Cl–) requirement of young calves; therefore, the committee decided to use the ratio of Cl– to Na requirement calculated for lactating cows (i.e., 0.8) and multiply that by the Na requirement as calculated using Equation 10-23.
TABLE 10-11Daily Energy and Protein Requirements of Large-Breed Veal Calves Fed Only Milk or MR
BW (kg) | ADG (g/d) | DMIa (kg/d) | ME (Mcal/d) | NEm (Mcal/d) | MP (g/d) | CPb (g/d) | CP (% of DMI) |
---|---|---|---|---|---|---|---|
40 | 300 | 0.55 | 2.54 | 1.17 | 114 | 120 | 21.8 |
600 | 0.78 | 3.59 | 1.17 | 187 | 197 | 25.2 | |
50 | 300 | 0.63 | 2.88 | 1.38 | 120 | 126 | 20.1 |
600 | 0.86 | 3.98 | 1.38 | 193 | 204 | 23.6 | |
900 | 1.12 | 5.13 | 1.38 | 267 | 281 | 25.2 | |
75 | 300 | 0.79 | 3.65 | 1.87 | 132 | 139 | 17.6 |
600 | 1.05 | 4.84 | 1.87 | 208 | 219 | 20.8 | |
900 | 1.32 | 6.10 | 1.87 | 284 | 299 | 22.6 | |
1,200 | 1.61 | 7.40 | 1.87 | 360 | 379 | 23.6 | |
100 | 600 | 1.22 | 5.60 | 2.32 | 222 | 234 | 19.2 |
900 | 1.51 | 6.93 | 2.32 | 300 | 316 | 21.0 | |
1,200 | 1.81 | 8.31 | 2.32 | 379 | 399 | 22.0 | |
1,500 | 2.11 | 9.73 | 2.32 | 457 | 480 | 22.8 | |
125 | 600 | 1.37 | 6.30 | 2.75 | 235 | 247 | 18.1 |
900 | 1.67 | 7.70 | 2.75 | 316 | 333 | 19.9 | |
1,200 | 1.99 | 9.14 | 2.75 | 397 | 418 | 21.0 | |
1,500 | 2.31 | 10.62 | 2.75 | 478 | 503 | 21.8 | |
150 | 600 | 1.51 | 6.95 | 3.15 | 248 | 261 | 17.2 |
900 | 1.83 | 8.40 | 3.15 | 331 | 349 | 19.1 | |
1,200 | 2.15 | 9.90 | 3.15 | 415 | 437 | 20.3 | |
1,500 | 2.49 | 11.40 | 3.15 | 498 | 525 | 21.1 | |
175 | 600 | 1.65 | 7.57 | 3.54 | 260 | 274 | 16.6 |
900 | 1.97 | 9.07 | 3.54 | 349 | 365 | 18.5 | |
1,200 | 2.31 | 10.62 | 3.54 | 433 | 456 | 19.8 | |
1,500 | 2.65 | 12.20 | 3.54 | 519 | 547 | 20.6 | |
200 | 600 | 1.77 | 8.17 | 3.91 | 273 | 287 | 16.2 |
900 | 2.11 | 9.70 | 3.91 | 362 | 381 | 18.1 | |
1,200 | 2.45 | 11.29 | 3.91 | 452 | 475 | 19.4 | |
1,500 | 2.81 | 12.92 | 3.91 | 541 | 569 | 20.3 | |
225 | 600 | 1.90 | 8.73 | 4.27 | 286 | 301 | 15.8 |
900 | 2.24 | 10.31 | 4.27 | 378 | 398 | 17.8 | |
1,200 | 2.59 | 11.94 | 4.27 | 471 | 496 | 19.1 | |
1,500 | 2.96 | 13.61 | 4.27 | 563 | 593 | 20.0 |
- a
DMI necessary to meet requirement for ME when fed MR containing 4.6 ME/kg of DM.
- b
Assumes MP/CP of 0.95.
For the trace minerals, copper (Cu), iron (Fe), manganese (Mn), and zinc (Zn), equations developed for older cattle were applied to calves, but different ACs were used. No AI is given for cobalt (Co) in MR because young calves lack a functioning rumen and cannot convert Co into vitamin B12. The AIs for trace minerals are calculated as follows:
(Equation 10-24)
(Equation 10-25)
(Equation 10-26)
(Equation 10-27)
For Cu, 2.5 mg/kg ADG was used for young calves, rather than 2.0 used for growing heifers, which reflects greater concentrations of Cu in organs than in muscle (see Chapter 7). For Zn, 24 mg/kg ADG was used for young calves, which increased to 30 mg/kg ADG for growing heifers, reflecting the greater concentration of Zn in muscle than in organs (Watson et al., 2018). The denominators in the above equations are calf-specific AC (see Table 10-12). Inadequate data are available to generate feed-specific AC for calves; therefore, all diets fed to young calves have the same AC. The calf ACs were derived from experiments conducted on young calves, and when data on absorption of minerals by preruminant calves could not be found, nonruminant data (e.g., human, swine, rodents) were used (see Chapter 7). When multiple ACs were available, the committee used the lower value to reduce the risk of deficiencies.
In the model, when users select calf as animal type and the diet does not include any liquid feed (i.e., a weaned calf), requirement equations used for older animals (see Chapter 7) are used for all minerals along with the ACs in Table 10-12 under the weaned calf column. A single AC for each mineral is used for the total diet; individual feed ACs are not used if calf is selected.
TABLE 10-12ACs for Minerals Used for Young Calves
Mineral | Milk Replacer and Startera | Weaned Dietb |
---|---|---|
Ca | NAc | 0.60 |
P | NA | 0.75 |
Mg | NA | 0.26 |
K | NA | 1.0 |
Na | NA | 1.0 |
Cu | 0.5 | 0.10 |
Fe | 0.25 | 0.10 |
Mn | 0.01 | 0.005 |
Zn | 0.20 | 0.20 |
- a
ACs in this column are appropriate for preweaned calves (i.e., nonmature rumen).
- b
ACs in this column are used for postweaned calves. The ACs for macrominerals and most trace minerals are typical for diets fed to adult cattle. The AC for Cu is derived from newly weaned lambs (Suttle, 1975) and will continue to decrease to the value used for older cattle (0.05) as dietary forage increases.
- c
For preweaned calves, retention coefficients (Castro et al., 2019) rather than AC are used for macrominerals.
TABLE 10-13Recommended Concentrations of Minerals in MR and Starter (DM Basis) to Provide AIs for Calves Between 35 and 125 kg of BW and Growing Between 0.5 and 1.2 kg/d
Mineral | Milk Replacer | Starter | Grower |
---|---|---|---|
Ca, % | 0.80 | 0.75 | 0.65 |
P, % | 0.60 | 0.37 | 0.33 |
Mg, % | 0.15 | 0.15 | 0.16 |
K, % | 1.10 | 0.60 | 0.60 |
Na, % | 0.40 | 0.22 | 0.20 |
Cl, % | 0.32 | 0.17 | 0.15 |
Co, mg/kg | NA | 0.2 | 0.2 |
Cu, mg/kg | 5 | 12 | 12 |
I, mg/kg | 0.8 | 0.8 | 0.5 |
Fe, mg/kg | 85 | 60 | 55 |
Mn, mg/kg | 60 | 40 | 60 |
Se, mg/kg | 0.3 | 0.3 | 0.3 |
Zn, mg/kg | 65 | 55 | 50 |
To derive recommended concentrations of minerals in MR, daily dietary requirements were calculated for different-sized calves (35 to 85 kg) with different rates of gain (0.5 to 1.0 kg/d), and appropriate DMI values based on different amounts of MR and starter were assigned so that total dietary concentrations could be calculated. This was done for 20 different situations. The concentrations of mineral needed to meet AI were averaged across the different situations to obtain recommended dietary concentrations of minerals. Because calves consume liquid feed as their sole diet for at least several days, recommended concentrations in MR (see Table 10-13) were the same concentrations as recommended for total diet. This approach resulted in macromineral concentrations that were quite similar to that of milk on a DM basis (see Table 10-14).
TABLE 10-14Concentrations of Minerals and Fat-Soluble Vitamins in Whole Milk (per Liter)a
Mineral | Range | Meanb |
---|---|---|
Ca, g | 0.93–1.47 | 1.0 |
P, g | 0.8–1.0 | 0.9 |
Mg, g | 0.10–0.13 | 0.11 |
K, g | 1.27–1.71 | 1.49 |
Na, g | 0.33–0.48 | 0.41 |
Cl−, g | 0.85–1.09 | 0.97 |
S, g | 0.18–0.31 | 0.25 |
Cu, mg | 0.03–0.06c | 0.04 |
I, mg | 0.1–0.4d | 0.2 |
Fe, mg | 0.1–0.4 | 0.2 |
Mn, mg | 0.012–0.05 | 0.03 |
Se, mg | 0.018–0.04e | 0.02 |
Zn, mg | 3–6 | 4.0 |
Vitaminsf | Range | Meanb |
Vitamin A, IU | 300–1,300 | 1,000 |
Vitamin D, IU | 14–40 | 27 |
Vitamin E, IU | 0.7–1.8 | 1.2 |
- a
Sources of data can be found in Chapter 7.
- b
Mean values are for Holstein cows; however, breed can affect concentrations of some minerals. Limited data are available for other breeds (Cerbulis and Farrell, 1976; Carroll et al., 2006; Stocco et al., 2019).
- c
Concentrations can be greater if cows are fed very high amounts of Cu.
- d
Concentrations in milk have a positive linear relationship to iodine (I) intake. These ranges reflect feeding at approximate I requirements.
- e
Lower concentrations reflect milk from cows fed inorganic selenium (Se) at approximate requirements. The higher concentrations reflect milk from cows fed Se yeast at approximate requirements.
- f
Concentrations of vitamins A, D, and E have a positive relationship to intake of the vitamin by the cow and with the fat concentration of the milk.
NOTE: S = sulfur.
Starter was assumed to be the primary nutrient source for calves immediately postweaning. Recommended concentrations of minerals in starter were determined by calculating mineral requirements using the equations above for calves weighing 110 and 60 kg (representing Holsteins and Jerseys) gaining between 0.5 and 1.2 kg/d with appropriate intakes (only fed dry feeds). Nutrient concentrations to meet the requirements were calculated and averaged. However, Equations 10-25 to 10-28 were developed with data from young calves with limited rumen function, but after weaning, calves are becoming functional ruminants. Therefore, requirements and dietary concentrations were also calculated using mineral equations and AC developed for functioning ruminants (see Chapter 7), except for Cu. The recommended Cu concentrations for starter calculated using calf and functioning ruminant equations differed markedly (sometimes by more than a factor of 2). These animals are transitioning into functioning ruminants, and no data are available on mineral absorption by this type of animal; therefore, the recommended Cu concentrations in starter and grower (see Table 10-14) reflect Cu absorption measured in newly weaned lambs (Suttle, 1975). The recommended dietary concentrations of other minerals in the grower were calculated using the same method as used for starter except the committee used calves that weighed between 80 and 125 kg to calculate AIs.
For iodine (I), the AI for nonruminating calves was set based on the AI established for human infants (0.8 mg I/kg DMI; see Chapter 7), and no AC is used. The AI for supplemental selenium (Se) is set at 0.3 mg Se/kg DMI, which is the same as that used for older cattle and NRC (2001). No AC is used. Based on all available data, Se supplementation at that rate should prevent white muscle disease. In most cases, whole milk will not provide adequate Se and will need to be supplemented.
Compared to recommended concentrations in the previous edition (NRC, 2001), recommended Ca concentrations are lower for MR but similar for starter and grower. Recommended concentrations of P are about 15 percent lower for MR, starter and grower. Recommended K concentration in MR is about 70 percent higher but similar for starter and grower, and recommended Na concentrations are similar to the previous edition. Recommended concentrations of Cu are about half the previous value, and Fe is about 15 percent lower for MR but similar for starter and grower. Recommended concentration of Mn is higher for MR but similar to the previous edition for starter and grower. Zn concentrations are about 40 percent greater than those in the previous edition.
In the computer model, when growing heifer is selected, the ACs change to those described in Chapter 7. Therefore, the growing calf submodel should be used until calves are greater than 125 kg of BW.
Vitamins
Detailed information on the roles of vitamins is given in Chapter 8. No new information is available on the responses by calves to supplemental water-soluble vitamins; therefore, the previous recommendations were retained (see Table 10-15). The B-vitamins (including vitamin B12) and choline should be added to MR, but once calves are weaned and consuming dry feed, the basal diet and ruminal synthesis appear adequate to meet the needs for water-soluble vitamins by the growing calf. New data are also not available regarding vitamin K supplementation to calves. Based on limited data (Nestor and Conrad, 1990), supplemental vitamin K is not needed by young calves if not fed moldy sweet clover.
Vitamin A
Because of limited placental transfer, calves are born with very low stores of retinol and β-carotene and are dependent on an adequate and timely intake of colostrum that contains adequate concentrations of retinol. A calf fed 3 L of first milking colostrum could ingest more than 30,000 IU of retinol (ca. 0.9 mg of retinol), which will elevate hepatic concentrations of retinol into the acceptable range (ca. >20 mg/kg of liver wet weight). However, vitamin A nutrition of the dam during the prepartum period and time after birth when the calf is fed (Zanker et al., 2000; Puvogel et al., 2008) affect vitamin A status of the very young calf, which likely will affect the calf's response to vitamin A supplementation during the first several weeks of life. Holstein calves that received adequate retinol via colostrum and were fed about 5,400 IU of vitamin A/d maintained hepatic concentration of retinol at approximately 20 mg/kg wet weight during the first 4 weeks of life (Swanson et al., 2000). However, hepatic retinol decreased when calves were fed 3,800 IU of vitamin A/d (indicative of inadequate consumption). Liver concentrations increased over time to about 40 mg/kg wet weight when calves consumed 10,600 IU/d and to about 100 mg/kg wet weight when fed 26,600 IU/d (indicative of excess consumption). Based on this study, Holstein calves fed MR need to consume about 5,400 IU/d of vitamin A. That is contingent on calves receiving adequate colostral retinol to elevate hepatic retinol to about 20 mg/kg wet weight. The AI of vitamin A for older cattle was set at 110 IU/kg BW; if that value was applied to young Holstein calves, the AI would be approximately 6,600 IU/d. Because of the uncertainty associated with colostral retinol, the AI of vitamin A was set at 110 IU/kg BW for young calves, which is approximately equivalent to 11,000 IU/kg of MR solids when fed at 0.6 kg/d. At high rates of MR intake, that concentration may lead to excessive intake of vitamin A. Higher rates of MR intake lead to greater growth rates, but increasing growth rates (0 to 1.2 kg/d) in young calves did not affect serum concentrations of retinol, although all calves were fed excess vitamin A (Nonnecke et al., 2010). Vitamin A intakes of approximately 17,000 to 39,000 IU/d reduce plasma α-tocopherol concentrations substantially in young calves (Franklin et al., 1998; Ametaj et al., 2000). This suggests that at high rates of MR intake (e.g., >1 kg of solid/d), the concentration of vitamin A in the MR should be less than 9,900 IU/kg of MR solids. No available data show any benefit of feeding more than the current recommended AI (i.e., ca. 7,000 IU/d) of vitamin A, whereas data are available showing negative effects of higher intakes of vitamin A on vitamin E status.
Vitamin D
Although vitamin D is receiving renewed research for adult cows, new data are still very limited for young calves. Vitamin D status (as measured by plasma concentrations of 25-OH vitamin D) was depressed when young calves were infected with bovine diarrhea virus (Nonnecke et al., 2014). Young calves fed 5,000 IU/d of vitamin D3 tended to have fewer health issues than calves fed no supplemental vitamin D (Krueger et al., 2016). Calves fed no supplemental vitamin D had plasma 25-OH vitamin D concentrations <20 ng/mL by 14 days of age, and intake of supplemental vitamin D by calves is linearly related to plasma or serum concentrations of 25-OH vitamin D. To obtain a serum concentration of 30 ng/mL, calves need to consume approximately 2,100 IU of vitamin D3/d (Nelson et al., 2016). Concentrations of serum 25-OH vitamin D <30 ng/mL are associated with increased health problems in humans (Norman, 2008). The optimal serum concentration of 25-OH vitamin D for dairy calves is not known. The committee set the AI of vitamin D3 at 32 IU/kg BW (i.e., 2,100 IU/d for a 65-kg calf) based on maintaining serum 25-OH vitamin D at 30 ng/mL, which is close to the recommended AI for older cattle. At an intake of 0.6 kg/d, MR would need to contain 3,500 IU of vitamin D3/kg solids to meet the AI of vitamin D (see Table 10-15). This represents a substantial increase from NRC (2001).
Vitamin E
The recommended intake of vitamin E was increased to 50 IU/kg of MR solids (approximately 30 IU/d) for young calves in 2001 (NRC, 2001). Although one study (Reddy et al., 1987) reported improved growth rates when calves were fed 125 or 250 IU of vitamin E/d compared to those fed no supplemental vitamin E, the NRC (2001) committee concluded that inadequate data were available to increase the recommendation further. The ADG in Reddy et al. (1987) was low (<150 g/d), and growth rate appears to affect vitamin E status of young calves. Serum α-tocopherol concentrations were lower in calves gaining 1.2 kg/d compared to calves gaining 0.55 kg/d, even though vitamin E intakes (approximately 300 IU/d) were similar (Nonnecke et al., 2010). Supplemental vitamin E (500 IU/d) increased growth in calves fed adequate energy and protein to grow at 0.5 kg/d but did not affect growth in calves fed to grow at 0.25 kg/d (Krueger et al., 2014). Conversely, Sehested et al. (2004) reported no difference in growth rate (approximately 0.8 kg/d) between young Holstein calves fed 0 or 500 IU of supplemental vitamin E. In nonruminants (and preruminant calves), the vitamin E requirement is a function of intake of PUFA, and based on typical intakes of PUFA by young calves, vitamin E intake needs to be about 30 IU/d just to prevent oxidative stress caused by PUFA. Infections can significantly deplete plasma stores of vitamin E (Nonnecke et al., 2014), and many studies have shown enhanced immune function when vitamin E is supplemented at rates greater than 30 IU/d to young calves (Reddy et al., 1986; Eicher-Pruiett et al., 1992; Eicher et al., 1994; Samanta et al., 2006; Pekmezci and Cakiroglu, 2009). These data in total strongly suggest that intake of vitamin E should be greater than 30 IU/d. In lieu of perfect data and based on the definition of AI, the committee chose the lowest supplementation rate that has been shown to be beneficial (i.e., 125 IU/d or about 2 IU/kg BW; see Table 10-15) as the AI for vitamin E. The committee acknowledges that this may not be adequate for rapidly growing calves.
TABLE 10-15Recommended AIs for Fat-Soluble Vitaminsa
IU/kg BW | IU/kg DM | |||
---|---|---|---|---|
Milk Replacerb | Starter c | Grower d | ||
Vitamin A | 110 | 11,000 | 3,700 | 3,700 |
Vitamin D | 32 | 3200 | 1,100 | 1,100 |
Vitamin E | 2.0 | 200 | 67 | 67 |
- a
Water-soluble vitamins are needed in MR. Recommended concentrations (per kilogram of DM) are 6.5 mg of DM for thiamin, riboflavin, and pyridoxine; 13 mg pantothenic acid; 10 mg niacin; 0.1 mg biotin; 0.5 mg folic acid; 0.07 B12; and 1,000 mg choline (NRC, 2001). Microbial synthesis of vitamin K within the intestines appears adequate, and supplemental vitamin K is usually not needed (Nestor and Conrad, 1990).
- b
These values assume a 60-kg calf that is consuming 0.6 kg of MR solids. Concentrations should be reduced if calves are fed substantially greater amounts of MR (e.g., >1 kg/d of solids).
- c
These values assume an 80-kg calf consuming 2.4 kg of starter DM.
- d
These values assume a 110-kg calf consuming 3.3 kg of grower DM.
PRACTICAL FEEDING CONSIDERATIONS
Rates of Milk or Milk Replacer Feeding
The optimal amount of milk or MR to provide remains controversial. The primary point of contention is that feeding larger amounts of liquid delays increases in starter intake, which, in turn, stimulate development of ruminal fermentation and the absorptive epithelium. A large body of research evidence since NRC (2001) highlights the greater early growth obtained by feeding milk at higher rates (12 to 20 percent of BW) than the “traditional” 8 to 10 percent of BW (Drackley, 2008; Khan et al., 2011a). Like other mammalian neonates, calves given free access to milk will drink large amounts in preference to dry feed. Calves with ad libitum access to milk will consume about 20 percent of their BW daily, which for 50- to 60-kg Holstein calves would be 10 to 12 L of whole milk daily (Jasper and Weary, 2002; Khan et al., 2007; Sweeney et al., 2010). Assuming 12.5 percent solids, this amount equates to 1.3 to 1.6 kg/d of milk solids or about 2.5 percent of BW. Likewise, Holstein calves with ad libitum access to MR will consume 1.2 to 1.4 kg of DM daily (Schaff et al., 2016; Frieten et al., 2017; Korst et al., 2017; Curtis et al., 2018). Because calves respond to greater amounts of milk or MR with greater growth (Khouri and Pickering, 1968; Hodgson, 1971; Huber et al., 1984; Yunta et al., 2015; Rosenberger et al., 2017), defining a requirement for a level of feeding is not possible except to establish the relationship between rates of growth and the amounts of nutrients needed to fuel that growth. Recommendations about how much milk or MR calves should be fed must be made with the understanding that calves willingly drink much more milk or MR than the limited amounts offered in standard practice for decades.
It is useful to establish a standardized framework for feeding rates for the discussion that follows. Consumption of <400 g of milk or MR solids daily for large-breed calves (300 g/d for small-breed calves) will be referred to as “severely restricted” because this amount may not cover maintenance requirements, especially if an immune challenge is present or in adverse environmental conditions. Feeding rates of 400 to 600 g/d for large-breed calves (300 to 450 g/d for small breeds) will be referred to as “low” rates of milk or MR feeding. Rates between 600 and 900 g/d (451 to 700 g/d for small breeds) are referred to as “moderate” feeding rates, and anything >900 g/d (700 g/d) and less than ad libitum is referred to as “high” rates of feeding. The median (50th percentile) milk or MR intake for preweaned Holstein heifers in the United States was 5.5 L/d (Urie et al., 2018), which at 12.5 percent solids and density of 1.03 g/mL would equate to about 708 g/d of milk solids, or a “moderate” rate of feeding. Put another way, about 50 percent of dairy producers fed Holstein calves only 4 to 5 L of milk or MR daily (515 to 644 g/d of milk solids; USDA, 2016).
The rationale for severely restricted or low rates of feeding for neonatal calves includes seeking to stimulate early intake of starter, which is less expensive per unit of feed (although not necessarily less expensive per unit of BW gain); to encourage early weaning; and to decrease the incidence of digestive disorders such as diarrhea (Khan et al., 2011a). During the first 2 weeks of life, the limited amount of milk provides calves with only enough nutrients in excess of maintenance to grow 0.2 to 0.3 kg/d under thermoneutral and non-immune-challenged conditions. With low rates of milk intake, calves will rapidly increase their intake of starter beginning at around 2 weeks of age (Williams and Frost, 1992). Greater intakes of the fermentable carbohydrates in starter stimulate microbial growth and ruminal fermentation, resulting in a sharp increase in growth rate (Kertz et al., 1979).
Development of early starter intake is inversely proportional to the amount of liquid feed offered (Hodgson, 1971; Jasper and Weary, 2002; Stamey et al., 2012), which should not be surprising since calves have a maximum total DMI or energy intake like other animals. Rumen development takes about 3 weeks of intake of a typical starter (Williams and Frost, 1992), although recent studies have shown that it is the cumulative consumption of nonfiber carbohydrates (starch and sugars) rather than just total starter intake that is more highly related to rumen development (Quigley et al., 2019a). When weaning occurs before the calf has consumed sufficient fermentable carbohydrates, the rumen may not be able to efficiently convert feeds to metabolizable nutrients, and growth rates suffer. Nutrient digestibility, particularly NDF, was decreased after weaning for calves fed larger amounts of MR before weaning (Terre et al., 2007; Hill et al., 2010), which likely was due to inadequate development of the rumen function. No studies have reported effects of different milk feeding levels on digestibility, where the time of measurement relative to achieving a certain starter intake was controlled; thus, at the same age, calves fed less milk will by default have consumed more starter and have more well-developed rumen function than calves fed larger volumes of liquid feed.
Under good management, limited liquid feeding programs have been successful. However, when calves are challenged by infectious or environmental stressors, severely restricted or low nutrient intakes may limit effective immune responses or not allow sufficient heat production for thermoregulation (Godden et al., 2005; Olivett et al., 2012; Ballou et al., 2015). Considerable evidence indicates that feeding rates that do not achieve maintenance are inadequate to support optimal health and function of the immune system, especially under adverse environmental conditions (Williams et al., 1981; Griebel et al., 1987; Pollock et al., 1993, 1994; Godden et al., 2005; Ollivett et al., 2012; Ballou et al., 2013, 2015). The advantages to calf health of greater amounts of liquid feed likely relate to providing more nutrients to support an immune response (Nonnecke et al., 2003; Foote et al., 2005a,b, 2007; Ballou, 2012; Obeidat et al., 2013). Some aspects of the immune system in isolated immune cells appear to be downregulated by high rates of milk feeding (Nonnecke et al., 2003; Foote et al., 2005a,b, 2007), although the significance of these changes in vivo has not been delineated.
Greater preweaning growth rates from feeding more milk or increased starter intake are associated with greater milk yields in first lactation (see studies summarized in Bach et al., 2012; Soberon et al., 2012; Gelsinger et al., 2016). Heifers that grow more rapidly in early life are not heavier at first parturition but may calve earlier (Van Amburgh et al., 2019). The mechanism(s) responsible for such an effect of early growth remain unclear, although several lines of evidence have emerged from studies where early growth was stimulated by greater amounts of MR. Greater rates of MR feeding increased mammary parenchymal mass and parenchymal DNA and RNA without fat deposition (Brown et al., 2005a). Greater MR intake was associated with greater mass and increased proliferation of mammary epithelial cells in heifers killed at 100 kg BW (Meyer et al., 2006a,b. An enhanced plane of nutrition (1.1 kg versus 0.44 kg of MR) resulted in greater mammary parenchymal tissue growth in response to estrogen stimulation (Geiger et al., 2016), although starter intake was restricted in the low-feeding group. Finally, changes in the mammary gland transcriptome when calves are fed different rates of MR were consistent with greater mammary development in heifers fed greater amounts of MR (Piantoni et al., 2008, 2010, 2012; Vailati-Riboni et al., 2018). Studies on the mechanisms of the effect of greater early ADG achieved by greater starter intake are not available but could be reasoned to occur by similar mechanisms. More research is needed in this area.
Since publication of NRC (2001) guidelines, a large body of behavioral studies has been published. These studies established that calves fed low amounts of milk demonstrate behavioral signs of hunger, including increased vocalization and decreased resting time compared with calves fed at least 8 L/d (Thomas et al., 2001; de Paula Vieira et al., 2008). In a titration study in which calves were fed 6, 8, 10, or 12 L/d of whole milk, calves actually consumed 5.7, 7.2, 8.3, and 9.4 kg/d (Rosenberger et al., 2017). Calves made 11.1, 3.6, 1.7, and 0.4 unrewarded visits to the automated feeder, indicating that calves offered less than 8 L/d displayed clear signs of hunger. The amount of solids consumed by calves consuming 7.2 kg/d was 890 g/d.
Hill and colleagues (Hill et al., 2006b, 2007d,e, 2009b,c) established that feeding Holstein calves 0.68 kg/d of MR solids containing 26 percent CP and 17 percent fat increases ADG relative to lower feeding rates but does not significantly decrease starter intake. A limitation of this body of work is that the calves were males transported to the research facility within 1 to 2 days of birth, and replication by other research groups has been limited. Based on the preponderance of evidence, the committee recommends that the minimum amount of milk or MR solids to be fed under thermoneutral conditions should be 1.5 percent of birth BW, similar to the body of work by Hill and colleagues. The welfare argument for feeding calves more than this (8 L/d or ca. 1,000 g/d of milk solids) to avoid hunger is compelling. The committee encourages adoption of programs that provide greater rates of liquid feeding than this minimum of 1.5 percent of BW as milk solids, based on considerations for calf well-being and enhanced nutrient supply for early growth.
Limited evidence suggests that increased feeding rates can start almost immediately after birth. Knauer et al. (2017) compared a gradual increase of milk offered (from 5–6 L/d to 6–8 L/d over a 7- to 14-day period) with a fixed amount of 6 to 8 L/d from day 1 of life. Offering a fixed milk allowance from day 1 improved calf growth during the first 3 weeks compared with the gradual increase in milk allowance, with no detrimental effect on calf health.
Typical MR contains 10 to 20 percent less energy than comparable volumes of whole milk because of lower fat content (i.e., 15 to 20 percent in MRs compared with 25 to 30 percent in milk). A 45-kg calf fed 0.51 kg of MR solids (9 percent of BW at 12.5 percent solids) that contains ME at 4.7 Mcal/kg of DM would consume enough energy for maintenance and a body weight gain of 0.19 kg/d under thermoneutral conditions. According to the model presented in this edition, feeding the same volume of whole milk would support a gain of 0.29 kg/d. If the same calf is housed at –5°C, 0.51 g/d of MR powder is insufficient even for maintenance and weight loss would ensue.
Starter
Concentrates are more effective than forages in stimulating development of the rumen (Hibbs et al., 1956; Warner et al., 1956). The VFAs produced from carbohydrate fermentation are at least partly responsible for development of ruminal papillae and the corresponding epithelium, with limited studies suggesting that butyrate is the most effective, followed by propionate, and acetate least effective (Flatt et al., 1958; Sander et al., 1959; Tamate et al., 1962). The actual cellular mechanisms responsible for stimulation of rumen epithelial development by fermentable substrates remain unresolved (Baldwin and Connor, 2017). Small amounts of chopped or ground forage can help prevent acidosis and parakeratosis if the concentrate particles are too small (Brownlee, 1956). Forage also stimulates muscle growth and rumen volume (Flatt et al., 1958). Calves raised in pasture systems likely rely on sugars and fructosans in fresh grass as the initial fermentative substrates.
Calves raised as herd replacements should be encouraged to eat starter from the first week of life. Starter should be kept clean and fresh and physically separated from water to avoid cross-contamination. Because starter provides the fermentable substrate for the developing ruminal microbiota, the most important factors are palatability and content of fermentable carbohydrates. Starter formulations have ranged between <20 percent and >40 percent starch, supplied mainly from cereal grains. Corn generally has promoted the greatest intake and resulted in the greatest ADG, with oats, barley, wheat, rice, and sorghum grains also used (Khan et al., 2016). Oats were an acceptable substitute for corn, but molasses and soy hulls resulted in decreased ADG (Hill et al., 2008d). Compared with oats or barley, corn and wheat resulted in greater DMI, higher rumen pH, increased papillae length, and heavier rumens (Khan et al., 2008). Under most situations, starter formulations will contain between 22 percent and 38 percent starch (see Table 10-16). Studies have examined the effectiveness of grain-processing methods, including steam-flaking, grinding, cracking, and rolling, but with variable effects on calf performance (Abdelgadir et al., 1996b; Lesmeister and Henrichs, 2004).
TABLE 10-16Example Nutrient Specifications for Typical Starter Varying in CP and Starch Content
Variables | Units | 16% CP, Low Starch | 18% CP, Low Starch | 18% CP, High Starch | 18% CP, High Starch | 22% CP, Moderate Starch | 22% CP, High Starch |
---|---|---|---|---|---|---|---|
DM | % As fed | 87.7 | 87.5 | 88.7 | 86.3 | 87.8 | 89.0 |
Starch | % DM | 15.1 | 20.7 | 36.9 | 39.0 | 25.5 | 32.9 |
CP | % DM | 18.8 | 20.0 | 18.7 | 20.2 | 24.7 | 25.0 |
ADF | % DM | 10.1 | 14.2 | 7.9 | 7.6 | 9.4 | 7.0 |
NDF | % DM | 24.8 | 29.5 | 18.9 | 15.9 | 16.3 | 13.7 |
NDFD48 | % NDF | 49.1 | 60.0 | 53.1 | 55.4 | 65.3 | 59.6 |
Lignin | % DM | 2.91 | 2.19 | 2.09 | 1.83 | 1.61 | 1.61 |
Ash | % DM | 8.0 | 9.1 | 8.3 | 7.9 | 7.0 | 8.8 |
Starch | % DM | 15.1 | 20.7 | 36.9 | 39.0 | 25.5 | 32.9 |
Water-soluble carbohydrates | % DM | 4.2 | 3.5 | 8.2 | 7.2 | 12.0 | 9.2 |
Crude fat | % DM | 6.9 | 5.1 | 3.9 | 3.6 | 3.3 | 3.2 |
DE, base | Mcal/kg | 2.67 | 3.21 | 3.22 | 3.19 | 3.62 | 3.38 |
ME | Mcal/kg | 2.48 | 2.99 | 2.99 | 2.97 | 3.37 | 3.14 |
The concept of palatability can be extended to address “appetence” or the actual preference to consume a given ingredient or mixed feed. While molasses is generally considered to be a palatable feed ingredient that may promote intake, excessive molasses (12 percent of DM) decreased starter intake compared with a formula containing 5 percent molasses (Lesmeister and Heinrichs, 2005). Miller-Cushon et al. (2014a,b) conducted extensive pairwise preference tests of energy and high-protein ingredients. They found that wheat meal was the highest-ranked feed type for preference to be consumed, followed by sorghum meal. Barley meal and corn meal were equally ranked, falling below wheat meal and sorghum meal but above all other feed types. Corn gluten feed and rice meal were ranked lowest. According to this method of comparison, soybean meal was the highest-ranked high-protein ingredient, followed by dried distillers grains. Corn gluten meal was the lowest ranked, followed by rapeseed meal. The preference for ingredient mixtures followed the rankings of individual ingredients (Miller-Cushon et al., 2014a,b. Montoro et al. (2012) determined that calves consumed similar total DM but different ratios of ingredients when they were offered separately compared with when they were provided as a mixed starter feed. Providing chopped grass hay promoted greater feed intake and digestibilities compared with providing the same amount of the hay in ground form (Montoro and Bach, 2012).
Digestion of NDF is negligible in very young calves. During the first week of life, before initiation of starter intake, ruminal pH ranges between 6.0 and 6.3 but then rapidly falls as starter intake increases (Anderson et al., 1987a). Although high-starch starter formulas (>32 percent) provide the most digestible energy, the resulting rumen pH is often very low (<5.5; Anderson et al., 1987a; Williams and Frost, 1992), in ranges that would be considered detrimental for mature ruminants. The low rumen pH results from the rapid fermentation of starch, the slower VFA absorption rates by the immature epithelium, and the low rate of saliva production in preruminants (Williams and Frost, 1992). Starter formulas with less starch or more slowly fermented starch and more digestible fiber can help to prevent the drastic drop in ruminal pH and help maintain pH greater than 6.0, allowing fibrolytic bacteria to become established and functional and help maintain rumen health. Good sources of readily fermentable fiber include beet pulp, brewers grains, soy hulls, and citrus pulp (Suárez et al., 2006; Porter et al., 2007; Hill et al., 2016a; Oltramari et al., 2018). Small amounts (<15 percent) of forage fiber, such as alfalfa meal, ground grass, or chopped grass hays, can be included to help buffer the rumen and to provide abrasive effects to help keep the keratin layer of epithelium thin so that absorption is maximized (Greenwood et al., 1997).
Starter DE and ME are calculated as described earlier using NDFD48, which as a concept has not been applied previously to calves. The NDFD48 values may not be accurate for calves, but they serve to provide a relative measure of the fermentation characteristics of various NDF sources. The committee chose a convenience sample of 17 studies that measured NDF digestibility in calves (Spanski et al., 1997; Khuntia and Chaudary, 2002; Terré et al., 2006; Porter et al., 2007; Castells et al., 2012, 2015; Ghassemi Nejad et al., 2012; Montoro et al., 2013; Chapman et al., 2016, 2017; Hill et al., 2016a,b Ghasemi et al., 2017; Dennis et al., 2018; Mojahedi et al., 2018; Quigley et al., 2018; Hu et al., 2019). Digestibility was determined when calves were consuming both milk or MR and starter before weaning (n = 23 treatment means), immediately after weaning (n = 39 treatment means), or in calves at least 3 weeks postweaning (n = 26 treatment means). Digestibility of NDF averaged 32.0 percent (range, 4.8 to 69.3 percent; coefficient of variation (CV) = 81.2 percent) in preweaned calves, 42.6 percent (range, 4.6 to 71.2 percent; CV = 34.2 percent) in recently weaned calves, and 57.6 percent (range, 42.3 to 70.7 percent; CV = 15.4 percent) in weaned calves. Thus, NDF digestibility increased with age and time after weaning, but the variability of results demonstrates that many factors must affect digestibility in addition to the nature of the NDF source. These may include starter intake, milk or MR intake, environment, and individual animal variability. Quigley et al. (2018) demonstrated that cumulative intake of NDF was a major predictor of NDF digestibility, with digestibility increasing sharply until approximately 2 kg of cumulative NDF intake and then beginning to plateau with additional intake. As described earlier, users can select to discount the ME value of starter for cases where NDF digestibility is expected to be depressed, such as when calves have been fed high amounts of milk or MR.
While a common recommendation is that NDF content of the starter should be >13 percent of DM, the physical form of the starter and its relationships with bedding material and forage provision are also important (Khan et al., 2016). Terre et al. (2013) reported that calves bedded on sawdust and fed a pelleted starter with 18 percent NDF had greater ADG than a pelleted starter with 27 percent NDF, regardless of whether calves were offered chopped forage. The physical characteristics of the starter mix are important to prevent anatomic or physiological abnormalities in the developing rumen. A minimum particle size is necessary to prevent parakeratosis of the rumen epithelium and impaction of fine particles among papillae (McGavin and Morrill, 1976; Greenwood et al., 1997). Studies suggest (Warner, 1991; Hill et al., 2008c; Porter et al., 2007) that at least 80 percent of the particles in a complete starter should be greater than 1,190 µm, and the starter should have a weighted mean particle size of approximately 2,000 µm or greater to prevent parakeratosis and bloat. The guidelines hold true regardless of the physical presentation of the starter (ground, mash, pellet, multiparticle, or texturized) since pellets would dissociate once hydrated in the rumen, depending on pellet hardness. Particle size determined by wet sieving techniques probably represents the true particle size availability in the rumen after consumption by the calf.
The optimal physical form is widely debated in the field, but research studies show few repeatable effects when confounding factors are controlled (Bateman et al., 2009). Porter et al. (2007) reported greater ADG, starter intake, and earlier rumination in calves fed a coarse mash (meal) starter compared with the same ingredients in pelleted form after being ground to fine particle size. Franklin et al. (2003) reported less starter intake and decreased ADG in calves fed a pelleted starter compared with those fed a textured starter, with calves fed the textured starter having greater intakes and ADG than either pelleted or meal forms; unfortunately, the ingredient composition was not identical among starter physical forms. Other studies also reported lower intakes and ADG when starters were fed as finely ground meals rather than as pelleted meals or coarse particles as a mash or textured (Lassiter et al., 1955; Gardner, 1967; Kertz et al., 1979), but experimental details were limited. Beharka et al. (1998) fed a diet of 25 percent alfalfa and 75 percent concentrate, either as coarse particles or finely ground. Ruminal pH was greater and papillae in the dorsal area of the rumen were longer for calves fed the coarse diet. Coverdale et al. (2004) restricted starter intake and reported that ADG was greater for calves fed a textured starter compared with a finely ground starter, but no difference was detected when calves had ad libitum access to the starters. Bach et al. (2007) fed diets of the same composition either as a pellet or a coarse mash and starter intake was lower for the pelleted diet, although this resulted in increased gain to feed ratio. Kertz (2007) questioned this study because no mention of the impact of bedding consumption was provided for calves fed the pelleted diet, which could confound the conclusions about effects of physical form and particle size. In a follow-up study, growth and intake were not different between pelleted and textured starters of the same ingredient composition when fed with forage to ensure a favorable rumen environment in calves bedded on sawdust (Terre et al., 2015). Hill et al. (2012) concluded that high-starch, low-fiber textured starters provided the greatest DMI and ADG for weaned calves between 2 and 4 months of age.
This body of research has tended to encourage the conclusion that calves should not be fed finely ground starters or pelleted starters without some forage when calves are not bedded with straw. However, Castells et al. (2015) reported high intakes of ground starter when provided with free access to chopped hay in calves bedded on wood shavings. Pazoki et al. (2017) found that calves bedded on sand and fed a fine meal with the addition of 10 percent chopped alfalfa outperformed calves fed the same starter as a pellet or as a textured mixture but without forage. Bateman et al. (2009) found that calves fed a pelleted starter with half of the DM provided as a fine meal had decreased intakes and ADG compared with calves fed only the pelleted form. Thus, the inconsistency of the physical form (e.g., pellets with abundant fines or coarse and fine particles together) may be what inhibits intake and performance, as long as sufficient particle size is provided by forage or bedding.
A variety of protein sources are used in starters, including soybean meal, canola meal, cottonseed meal, sunflower seed meal, linseed meal, corn gluten meal, and distillers dried grains (Khan et al., 2016). Soybean meal is the most widely used among any of the common proteins (Drackley, 2008). Attempts to increase MP by supplementing protein sources high in RUP generally have been ineffective in increasing ADG of calves (Drackley, 2008). In contrast, increasing soybean meal increased both MP supply and calf growth around weaning (Stamey et al., 2012).
The required protein content of the starter often has been taken out of context from the calf's growth rate and the amount of milk being fed before weaning. A CP content of 18 percent (DM basis) may be adequate for systems where 0.45 to 0.55 kg/d of milk or MR DM is fed (Akayezu et al., 1994; NRC, 2001), but when feeding 0.9 kg/d or more of milk or MR DM, starter CP content of 22 to 25 percent of DM may result in increased growth (Stamey et al., 2012; Stamey Lanier et al., 2021). Starter CP content should be consistent with requirements calculated elsewhere in this chapter. For example, small-breed calves growing rapidly may need to be fed a starter containing >22 percent CP depending on the protein content and amount of the MR fed (see Table 10-8).
Supplemental fat has been added to starter in an attempt to increase energy density and improve calf growth. Supplementing specific functional FAs was discussed in an earlier section. Fat addition to starter has generally shown few or inconsistent effects, either before or after weaning (Khan et al., 2016). Johnson et al. (1956) fed up to 10 percent tallow in calf starter and reported that DMI and ADG were not affected. Miller (1962) compared starters with 10 percent added fat from tallow, lard, butter, and hydrogenated cottonseed oil. Intake of starter and ADG did not differ among treatments. In contrast, Miller et al. (1959) showed that feeding 10 percent brown grease or hydrogenated cottonseed oil significantly decreased starter intake. Araujo et al. (2014) fed full-fat soybeans to supply 11 percent fat in the starter DM and observed no effects on DMI, in contrast to an earlier study (Kuehn et al., 1994) that reported negative effects of starter containing 7 percent added fat from soybeans. Hill et al. (2015) determined that supplementation of starters with 2 percent tallow or soybean oil decreased ADG. Berends et al. (2015) fed an extruded pellet that contained hydrogenated palm FAs and increased starter fat content to 7 percent. They reported increased starter intake and ADG with the higher-fat starter. Ghasemi et al. (2017) supplemented 3 percent fat from tallow, soybean oil, palm fat, or a mixture of palm, soybean oil, and fish oil. Inclusion of soybean oil increased calf performance, but palm fat and tallow did not. Doolatabad et al. (2020) fed a starter containing 7.5 percent fat from full-fat soybeans and prilled palm fat and noted no improvements in calf growth. Ghorbani et al. (2020) provided 2 percent fat from soybean oil or extruded or heated soybeans. Growth rate of calves was not affected. Overall, the variability in responses among studies indicates that more research is needed on factors affecting responses to fat.
In summary, starter composition and physical form are areas that still need more careful research attention, particularly for calves at higher milk feeding rates. Researchers must control and report factors such as whether the calves were housed on straw or other organic bedding, the particle size distribution of the starter or of the ingredients before pelleting, whether calves received any forage, and the particle size distribution of that forage.
Forage Provision
For many years, feeding forage prior to weaning was not recommended, and only very limited amounts were recommended after weaning. This recommendation was based on studies showing that ad libitum forage availability decreased concentrate consumption, which was key to rumen development (Tamate et al., 1962). In calves fed a limited amount of concentrate, greater forage intakes decreased EBW gain (Stobo et al., 1966). Forage is not well used by the young calf because of the limited rumen functionality to allow for forage fermentation (Anderson et al., 1987a; Khan et al., 2011b). However, recent research has challenged these assertions and indicates that the blanket recommendation to not feed forage is too simplistic.
When assessing the value of forage, several confounding factors must be considered, including particle size and physical form of the starter, type and amount of forage, whether the forage is offered separately or as a part of a total mixed ration (TMR), and whether the calf is bedded on straw or other organic bedding (Kertz, 2007). A meta-analysis (Imani et al., 2017) that did not consider bedding type found that improvement in overall starter intake was greater for calves offered alfalfa hay compared with those offered other types of forages (ryegrass hay, oat hay, barley straw, triticale silage, or corn silage). This analysis also found that ADG was greater for calves fed >10 percent of DM as forage compared with those fed ≤10 percent of DM as forage. However, the authors stated that the advantages in BW gain at the higher amount of forage provision could be due to increased gut fill. Increases of ADG were less for calves fed forages with textured starter compared with those fed forage with ground starters (Imani et al., 2017).
Calves bedded on straw and fed a textured starter with an adequate particle size had decreased starter intake and ADG when fed grass hay or cottonseed hulls (Hill et al., 2008b). In calves bedded on sawdust and fed large amounts of milk (8 kg/d) and a textured starter of undescribed particle size, providing ad libitum access to grass hay increased rumen pH and empty rumen weight without significant effects on EBW or stature measurements (Khan et al., 2011b). In contrast, for calves bedded on sawdust and fed a pelleted starter, ad libitum access to different forages (except alfalfa hay) increased starter intake and ADG (Castells et al., 2012). In that study, alfalfa hay was consumed in the largest amount (14 percent of total DMI) and reduced starter intake. Oat hay was consumed at 8 percent of total DMI but stimulated starter intake. The other forages, including barley straw, ryegrass straw, triticale silage, and corn silage, were consumed only in limited amounts (4 to 5 percent of DM) but also stimulated starter intake. For calves bedded on sawdust and fed pelleted starters with either 18 or 27 percent NDF, providing access to chopped oat hay did not affect intake or ADG before weaning but increased both postweaning (Terré et al., 2013). Terré et al. (2015a) demonstrated the interactions between starter physical form and access to chopped oat hay in calves bedded on sawdust.
One reason for not recommending feeding forage was that the low digestibility would increase gut fill, which would be measured as ADG (Stobo et al., 1966). Calves consuming large volumes of milk and offered long grass hay for ad libitum intake showed an increase in gut contents (Khan et al., 2011b). In contrast, calves with ad libitum access to chopped oat hay consumed 4 percent of their total DMI as forage and did not have any change in gut contents, compared with calves in the same study that had ad libitum access to alfalfa hay and consumed 14 percent of their total DMI as forage (Castells et al., 2013). In calves in whom chopped alfalfa hay was provided in the starter mix, no difference in gut contents was observed (Pazoki et al., 2017). Overall, increases in gut fill are more likely when feeding large amounts of forage (about >15 percent of total intake) or when alfalfa is fed rather than grasses.
In summary, current evidence indicates that calves fed textured starters of adequate particle size and bedded on chopped straw likely will obtain little benefit from forage provision, and ADG may be decreased. However, calves fed pelleted starters and not bedded with straw (or bedded with long straw) should be fed some chopped forage to maintain rumen environment and promote starter intake. Alfalfa hay should be limited to no more than 10 percent of total DMI. Other chopped forages should either be provided in small amounts for ad libitum intake or included in the starter or TMR at no more than 5 percent of total DM.
Weaning Management
Recommended weaning time is often based on a set amount of starter consumption on a consistent basis (e.g., large-breed calves can be weaned when consuming at least 0.9 kg of DM from a good-quality starter daily for 3 consecutive days) (Drackley, 2008). These recommendations assume that intake of starter will increase rapidly once milk feeding is reduced or eliminated (Stamey et al., 2012) so that slumps in growth rate are minimal and short-lived. However, the nutritional adaptation that must take place for the calf to wean successfully with minimal challenge to health and well-being is substantial. Table 10-17 shows EBW gains predicted by the equations in this chapter for calves before and after weaning for different amounts of starter intake. The drastic differences between preweaned and weaned calves arise from the differences in calculation of maintenance. These differences would likely be less in practice because the adjustments in gut size will be gradual. Nevertheless, the calculations make clear that for a period of a few days, weaned calves will struggle to gain BW at anywhere near the rate before weaning. Consequently, health and well-being will be put at risk (Williams et al., 1981; Griebel et al., 1987; Pollock et al., 1993, 1994). Based on these reasons, the committee recommends that small-breed calves be consuming at least 1.25 kg/d (assumed 90 percent DM) of starter and large-breed calves at least 1.5 kg/d before complete weaning. These higher thresholds of starter intake for weaning should help ease the weaning transition for calves fed larger amounts of milk and growing faster before weaning.
TABLE 10-17Predicted EBW Gains for Calves (Small-Breed and Large-Breed Calves Are Similar) of Equal EBW Before and After Weaninga
Preweaned Calf | Weaned Calf | |||||||
---|---|---|---|---|---|---|---|---|
BW, kg | EBW, kg | Milk Replacer DMI, kg/d | Starter DMI,b kg/d | EBW Gain, kg/d | BW, kg | EBW, kg | Starter DMI,b kg/d | EBW Gain, kg/d |
60 | 55.8 | 0.25 | 0.75 | 0.20 | 65.6 | 55.8 | 0.75 | –0.16 |
60 | 55.8 | 0.25 | 1.25 | 0.52 | 65.6 | 55.8 | 1.25 | 0.14 |
60 | 55.8 | 0.25 | 1.75 | 0.83 | 65.6 | 55.8 | 1.75 | 0.45 |
60 | 55.8 | 0.25 | 2.25 | 1.14 | 65.6 | 55.8 | 2.25 | 0.76 |
80 | 74.4 | 0.25 | 1.0 | 0.24 | 87 | 74.4 | 1.0 | –0.15 |
80 | 74.4 | 0.25 | 1.5 | 0.54 | 87 | 74.4 | 1.5 | 0.14 |
80 | 74.4 | 0.25 | 2.0 | 0.84 | 87 | 74.4 | 2.0 | 0.51 |
80 | 74.4 | 0.25 | 2.5 | 1.13 | 87 | 74.4 | 2.5 | 0.724 |
- a
Note that because of gut fill, EBW makes up a smaller proportion of BW after weaning than before weaning.
- b
Starter ME assumed to be 3.1 Mcal/kg.
With restricted milk or MR feeding under good management, successful weaning has been reported as early as 4 weeks of age (Hodgson, 1965; Kertz et al., 1979, 1984). Early weaning (24 days) suppressed some aspects of the innate immune system relative to weaning at 45 days (Hulbert et al., 2011). More aggressive milk-feeding programs will delay development of starter intake and weaning age (Hodgson, 1971; Huber et al., 1984), and weaning at an older age will help ease the transition (Hodgson, 1965; de Pasillé et al., 2011; Eckert et al., 2015; de Pasillé and Rushen, 2016). Gradual weaning over a period of 4 to 10 days is recommended rather than abrupt weaning (Sweeney et al., 2010).
Feeding Frequency
Williams et al. (1986) compared feeding frequencies of one, two, four, or six times daily for calves fed either 0.55 or 0.86 kg of DM daily of MR containing skim milk. Heat production, energy retention, respiratory quotient, and ADG were similar among feeding frequencies. Research conducted during the 1960s and 1970s (Appleman and Owen, 1975; Otterby and Linn, 1981) demonstrated that once-daily feeding resulted in mainly similar performance relative to twice or more daily feedings. More recent studies have confirmed those findings (Kehoe et al., 2007). A concern with once-daily feeding is that observation of calves may be less frequent and early signs of disease might be missed if management is not optimal (Davis and Drackley, 1998).
For calves fed larger amounts of liquid feed, increasing feeding frequency may improve efficiency of nutrient use. Strzetelski et al. (2001) tested feeding frequencies of one, two, and three times daily at either limited feeding or feeding to appetite of an MR containing dried skim milk, whey, buttermilk, and processed soy proteins. Calves fed limited MR two and three times daily and calves fed to appetite three times daily had greater weight gains and lower starter consumption than calves fed once daily. More frequent feedings may prevent abomasal ulcers (Ahmed et al., 2002). Efficiency of both protein and energy use was improved by increasing feeding frequency of an MR containing only whey proteins from two to four times daily, particularly when fed at 2.5 times maintenance compared with 1.5 times maintenance (van den Borne et al., 2006). In contrast, Kmicikewycz et al. (2013) found no benefit to increasing to four feedings daily compared with two feedings daily.
In summary, in calves fed low or moderate amounts of liquid feed, feeding more than twice daily had no repeatable effect on ADG or health. At higher feeding rates (≥2.5 times maintenance), increasing the number of feedings daily may improve efficiency of nutrient use, especially for calves fed nonclotting MR. More frequent feedings also may help abomasal health. Calves may benefit from an extra feeding (i.e., greater total daily intake) when housed outside during cold weather (Schingoethe et al., 1986).
Group Housing and Automated Calf Feeders
For many years, individual housing in hutches or stalls has been the gold standard in the dairy industry (Callan and Garry, 2002), but interest in group housing and automated feeders has increased in recent years (USDA, 2016). Resistance to group housing arises from concerns about calf health and veterinary recommendations to prevent calf-to-calf contact (Callan and Garry, 2002). Early studies found that calves housed individually had lower morbidity and mortality rates (Waltner-Toews et al., 1986a,b. However, later larger-scale, observational studies found no differences in health between individually housed calves and calves housed in small groups of six to eight (Losinger and Heinrichs, 1997; Svensson et al., 2006). Individual housing is criticized for restricting physical movement and social interaction among calves and faces increasing public opposition (Rushen et al., 2008).
Group housing offers several potential advantages for calf growth and welfare (Costa et al., 2016). Housing calves in groups allows social interaction and more normal behaviors than individual housing (Chua et al., 2002). Group housing can facilitate transition to solid feed, leading to better postweaning weight gains (de Paula Vieira et al., 2010; Costa et al., 2015; Miller-Cushon and DeVries, 2016). Group housing also decreases labor for feeding and management (Nordlund, 2008). Concern remains, however, that group housing can result in more disease spread among calves, particularly if ventilation is poor (Lago et al., 2006; Nordlund, 2008). Larger group size (>8 calves per pen) may increase risk for mortality and respiratory disease (Losinger and Heinrichs, 1997; Svensson and Liberg, 2006; Svensson et al., 2006). All-in, all-out systems have lower risk for mortality and morbidity than continuous-flow systems (Pedersen et al., 2009).
Automated feeders are becoming widely used because of inherent advantages in labor allocation and the ability to feed more milk or MR to calves in several meals per day. While direct comparisons of the systems are limited, the autofeeder system has resulted in similar performance and was cost-effective (Kung et al., 1997; Kack and Ziemerink, 2010). The computer control systems can simplify daily changes in milk feeding amounts, leading to peak milk consumption and weaning. In addition, the computer collects information about feeding behavior, which can be used to alert the producer to changes than may signal onset of disease (Svensson and Jensen, 2007; Knauer et al., 2017). The systems can reduce mortality to below-national averages (Jorgensen et al., 2017b), but as with any technology, management affects success. This includes fundamental management practices such as care of the newborn calf, excellent colostrum management, and limiting exposure to pathogens, particularly those in the liquid diet (Jorgensen et al., 2017b). Other key issues relate to the facility, because many autofeeders are installed in retrofitted existing structures. Adequate ventilation is critical to success and must be addressed when retrofitting (Nordlund, 2008; Jorgensen et al., 2017c). Air should not be shared with older cattle, which is associated with increased incidence of diarrhea and respiratory disease (Medrano-Galarza et al., 2018b). Bedding must be kept clean and dry, as wet bedding packs were associated with increased incidence of respiratory disease (Medrano-Galarza et al., 2018b), and the areas around the feeders should be cleaned daily (Jorgensen et al., 2017c). Of particular importance is proper cleaning and sanitation of the feeder, including the mixing chamber, milk lines, and nipples (Jorgensen et al., 2017a,b,c; Medrano-Galarza et al., 2018b).
Milk, pasteurized waste milk, or MR can be fed successfully through autofeeders. The feeding program and feeding management are critical. Early introduction (within 24 hours after birth) to the feeder compared with the more common practice of introducing calves at 5 to 14 days after birth results in both better outcomes for the calf and less total labor per calf (Medrano-Galarza et al., 2018a). Feeding larger volumes of milk per calf (>6 L/d) decreases feeder occupancy and thereby decreases competition in the pen (Jensen, 2006; Borderas et al., 2009). Shortening the time to peak milk intake by the calf has positive effects on growth and health (Jorgensen et al., 2017a; Medrano-Galarza et al., 2018a). Consumption of solid feeds from computer-controlled feeders can be used (de Passillé and Rushen, 2016) to monitor dry feed intake and adjust milk allowance downward for easier weaning (de Passillé and Rushen, 2012).
OTHER ASPECTS OF CALF NUTRITION
Fetal Nutrition
The developing fetal calf requires a balanced supply of nutrients from the mother via the placenta throughout gestation, but quantitatively fetal nutrient demands become significant only during the last trimester. More than 60 percent of total fetal weight gain occurs during the last 60 days of gestation (Eley et al., 1978) and is linear during that period through 270 days of pregnancy (Bell et al., 1995). Most of the carbon and N for fetal growth and energy supply comes from glucose, AAs, and lactate; the latter arises from glycolytic metabolism in the placenta (Reynolds et al., 1986). Glucose supplies approximately half of the energy needs of the conceptus, with 30 to 40 percent of respiratory fuel provided by AAs (Bell, 1995).
Although severe undernutrition can impair normal fetal development (NRC, 1968), the developing fetus is afforded a high priority for maternal nutrients. Moderate underfeeding or overfeeding of either protein or energy during the dry period (last 2 months of gestation) did not result in significant changes in calf birth weight (Nocek et al., 1983; Grum et al., 1996; Dewhurst et al., 2002; Dann et al., 2006; Douglas et al., 2006; Silva-Del Rio et al., 2010; Janovick and Drackley, 2010; Litherland et al., 2012; Mann et al., 2015). Likewise, neither viability nor health of newborn calves were affected by moderate maternal under- or overfeeding during the dry period (Davis and Drackley, 1998; Quigley and Drewry, 1998). Prolonged restriction of protein or energy during gestation decreased thermogenic abilities of beef calves at birth (Carstens et al., 1987; Ridder et al., 1991). Micke et al. (2010) found that overfeeding both energy and protein during the middle trimester of pregnancy in beef cattle resulted in greater calf birth weight than overfeeding energy with protein limited to 63 to 75 percent of requirement.
Maternal deficiencies of P, Mn, Co, Cu, Zn, or Se can result in deficiencies in the fetus and newborn calf (NRC, 1968). The fetus can concentrate some of these minerals, particularly Cu (Hidiroglou and Knipfel, 1981) and Se (Van Saun et al., 1989a), providing some protection against marginal deficiencies in the mother. Se supplementation of pregnant cows increased Se reserves in the newborn calves (Abdelrahman and Kincaid, 1995). Placental transfer of vitamin E to the developing fetus is low, although the fetal calf appears to have some ability to concentrate vitamin E from the dam (Van Saun et al., 1989b). The calf is born with a low vitamin E status and is highly dependent on intake of colostrum and then milk or MR to obtain needed vitamin E during early postnatal life. This is also true for retinol (Nonnecke et al., 1999) and vitamin D (Nonnecke et al., 2009). Overall, if diets for pregnant cows are balanced to meet recommendations for pregnancy and maternal growth (see Chapters 7 and 8), as well as for optimal transition success (see Chapter 12), nutrient supply should be adequate for normal growth and development of the fetal calf (Davis and Drackley, 1998; Quigley and Drewry, 1998).
Supplemental fats fed to the dam during the dry period may affect the developing fetal calf. Garcia et al. (2014a,b) fed either mostly saturated free FAs or Ca salts of unsaturated FAs enriched in C18:2 n-6 during the last 4 weeks of gestation. FA composition of colostrum and calf plasma reflected the composition of the fat supplement. Elongation and transfer of n-3 FAs by the placenta were decreased, but elongation and transfer of n-6 FA were increased. Differences in calf plasma FA profiles persisted through at least 60 days of age (Garcia et al., 2014b). Birth weight was increased by fat supplements for calves from parous dams but not from nulliparous dams. Apparent efficiency of immunoglobulin G (IgG) absorption from colostrum was greater for calves from dams fed fat, especially those fed saturated FAs (Garcia et al., 2014a). Calves from dams fed saturated FAs before parturition tended to have higher ADG; health or immune measures were not affected (Garcia et al., 2014b). Whether a mixture of FAs can be identified that will benefit newborn calves when fed to the cow in late gestation remains to be determined.
Feeding rumen-protected Met to cows during the last month of gestation increased Met concentration in maternal plasma by 29 percent and increased calf birth weight, perhaps related to increased expression of placental genes encoding transporters for neutral AAs and glucose, as well as increasing mTOR protein abundance (Batistel et al., 2017). Rumen-protected Met fed during the last 28 days of gestation also increased calf birth weight and ADG through 9 weeks of age (Alharthi et al., 2018). Methylation and demethylation of cytosine moieties in DNA modulate expression of many genes; some of these changes may alter the phenotype of the offspring. No measures of DNA methylation were made in these studies, but results are consistent with possible epigenetic effects of methionine (Chavatte-Palmer et al., 2018).
Colostrum
Calves are born with a naive immune system and essentially devoid of circulating immunoglobulins (Ig) due to the synepitheliochorial placenta of ruminants. Ig from colostrum (defined as the first lacteal secretion produced by the cow after calving) consumed prior to cessation of macromolecular transport, at approximately 24 hours after birth, are absorbed into intestinal cells via nonspecific pinocytosis and delivered intact to the circulation. Colostrum contains large, but variable, amounts of Ig, particularly IgG, which are transported from the maternal circulation into the colostrum during the final 3 weeks of gestation. Intake of adequate, high-quality (>50 g IgG per liter), and sanitary colostrum by the newborn calf is one of the most, if not the most, important factors related to reduced calf morbidity and mortality (Nocek et al., 1984; Wells et al., 1996; Godden et al., 2019).
Serum IgG concentration is an indicator of consumption and quality of colostrum. Serum IgG concentrations ≥10 g/L, when measured at 24 to 48 hours of age, are associated with lower risks of morbidity and mortality (Wells et al., 1996; Windeyer et al., 2014; Godden et al., 2019). To achieve a serum IgG concentration of 10 g/L, calves should be fed 150 to 200 g IgG from colostrum or colostrum replacement products in the first 24 hours. Concentration of IgG in the serum of a calf may be estimated using the following formula (Quigley and Drewry, 1998): Serum IgG (g/L) = IgG intake (grams) × AEA / PV, where AEA is apparent efficiency of IgG absorption (%), and PV = plasma volume (liters). The AEA is calculated as AEA (%) = serum IgG (g/L) × PV (liters) / IgG intake (grams).
The AEA of ingested IgG is affected by many factors related to the calf, stress at calving, environmental factors, and characteristics of the IgG source (Quigley and Drewry, 1998; Godden, 2008; Godden et al., 2019). The most important factor affecting AEA is age of the calf. The AEA declines with advancing age, maturation of the gastrointestinal tract, and turnover of intestinal cells capable of pinocytosis of macromolecules, so that by 22 to 24 hours after birth, the calf is no longer able to absorb IgG into the bloodstream (Bush and Staley, 1980; Kruse, 1983). This phenomenon is known as “gut closure.” Penhale et al. (1973) concluded that absorption declines gradually and progressively to closure, which occurs independently for each class of Ig (16, 22, and 27 hours for IgM, IgA, and IgG). Stott et al. (1979) estimated time of closure by Joinpoint regression with data from 210 calves. Closure was estimated near 24 hours of age with a normal distribution and standard deviation of approximately 4 hours, and the authors found no significant differences in closure time for IgG, IgM, and IgA. Estimated time for closure was delayed as feeding time was delayed; nevertheless, closure occurred spontaneously with age at a progressively increased rate after 12 hours postpartum.
Typical AEA ranges from <10 percent to approximately 40 percent. Ig not measured in the circulation may move into extravascular pools (MacDougall and Mulligan, 1969) or migrate into the intestine (Besser et al., 1998), thereby limiting AEA to approximately 50 percent (McEwan et al., 1970). Plasma volume is related to body size and is typically estimated using BW and averages about 8.9 percent of BW for Holstein and Jersey newborn calves (Quigley et al., 1998; Cabral et al., 2015).
Concentration of IgG in colostrum is highly variable (Morrill et al., 2012), and this variation contributes significantly to variation in serum IgG concentration and failure of calves to achieve satisfactory serum IgG concentration. In a survey of calf management practices on U.S. dairy farms, Urie et al. (2018a) reported serum IgG in 2,498 calves fed varying sources and amounts of colostrum or colostrum replacers, and most calves (72.7 percent) had serum IgG >15 g/L but 14.3 percent had serum IgG between 10 and 14 g/L, and 13 percent of calves had serum IgG <10 g/L, which is considered failure of passive transfer of immunity. Management factors associated with greater serum IgG concentrations were feeding a greater mass of IgG from colostrum and feeding colostrum at an early age (Shivley et al., 2018).
Time after parturition has a significant effect on IgG concentrations in colostrum. Concentrations of IgG were 27 percent lower in colostrum collected 10 hours postparturition compared with colostrum collected 2 hours postpartum (Moore et al., 2005). The IgG concentration in second-milking (12 hours later) colostrum from Jersey cows was 44 percent lower than that in first-milking colostrum (Silva-del-Río et al., 2017). Greater yields of colostrum are associated with lower concentrations of IgG (Pritchett et al., 1991; Silva-del-Río et al., 2017). Lesser factors affecting Ig concentrations are parity (increased concentrations with increasing parity; Muller and Ellinger, 1981; Kehoe et al., 2011) and perhaps breed (Muller and Ellinger, 1981; Morrill et al., 2012). Nutrition of the late-gestation cow does not appear to have much effect on Ig concentrations in colostrum (Quigley and Drewry, 1998; Dunn et al., 2017) but research data are limited. Responses to dietary protein fed to the dam on absorption of colostrum Ig by the calf are inconsistent (Quigley and Drewry, 1998). Some studies reported that low dietary protein fed to the late-gestation dam reduced Ig absorption by the calf, but other studies found no effect. Late-gestation heifers that were exposed to heat stress produced colostrum with lower concentrations of IgG (Nardone et al., 1997), whereas heat-stressed late-gestation multiparous cows produced colostrum with about 10 percent greater concentration (not statistically different) of IgG (Tao et al., 2012). Accumulating evidence suggests that maternal heat stress in late gestation reduces the immunocompetence of the neonate partly because of reduced absorption of colostral IgG (Tao and Dahl, 2013). Cabral et al. (2016) found that colostral IgG concentration could be predicted from previous lactation Dairy Herd Improvement Association data and weather data.
Amount of colostrum to feed in the first feeding and first 24 hours of life necessarily depends on concentration of IgG in the colostrum. A reasonable goal is to feed 150 g IgG in the first feeding and 200 g in the first 24 hours (Godden et al., 2019). Approximately 25 percent of first-milking colostrum contains too little IgG to provide >150 g IgG in a reasonable volume (e.g., ≤4 L in the first feeding; Morrill et al., 2012; Godden et al., 2019). To achieve >150 g IgG, colostrum containing greater than 50 g IgG per liter must be fed. A BRIX refractometer may be used to estimate the IgG concentration of colostrum and determine the optimal amount of colostrum to be fed (Bielmann et al., 2010; Quigley et al., 2013). The BRIX refractometer is inexpensive, and the method is reasonably accurate. The refractometer measures the refractive index (nD) of a solution and calculates BRIX percentage, based on the statistical relationship between nD and sugar in the solution. Table 10-18 has equations relating the BRIX reading to IgG; equations differ for colostrum from Holstein and Jersey cows (Morrill et al., 2015). The breakpoint is the BRIX reading at which colostrum contains at least 50 g IgG per liter. Holstein calves should be fed 3 L of colostrum with at least 22 percent BRIX within 1 hour of birth and 2 to 3 L fed at 10 to 12 hours of age. For Jersey calves, 2 L of colostrum with at least 18 percent BRIX should be fed within 1 hour after birth and again at 10 to 12 hours of age. Another approach is to administer 3.8 to 4.0 L of colostrum in the first feeding within 1 hour of age via an esophageal feeder with an optional second feeding of 2 L at 10 to 12 hours of age. Feeding a larger volume of colostrum in the first feeding generally increases absorption of IgG, as the calf is more efficient in absorbing IgG early in life. However, even with this approach, low BRIX colostrum should not be used. Conneely et al. (2014) compared effects on calf serum IgG concentrations when first-milking colostrum was fed within 2 hours of birth at 7.0, 8.5, or 10.0 percent of calf birth BW. Average first-milking colostrum intake for the three treatments was 2.6 L (range, 1.7 to 3.4), 3.2 L (2.0 to 4.2), and 3.8 L (2.4 to 4.9), respectively. Calf serum IgG concentrations (measured from 24 hours to 26 days of life) did not differ between calves fed colostrum at 7.0 or 10 percent of birth weight. However, calves fed colostrum at 8.5 percent of birth weight had significantly greater (approximately 15 to 25 percent) IgG concentrations than the other two groups at all sampling points. This study supports the 3-L feeding rate; however, the authors suggested that feeding 8.5 percent of birth weight rather than a fixed 3 L may enhance Ig absorption by reducing distension of abomasum in small calves and enhancing absomasal emptying.
Transition milk (milk produced during days 2 and 3 after calving) should be fed during days 2 and 3 if possible. Transition milk is higher in solids, protein, fat, and immunoglobulins compared to normal milk (Godden et al., 2019). Additional nutrients and other components of transition milk may promote intestinal development (Hammon and Blum, 1997; Rauprich et al., 2000; Blättler et al., 2001) and provide local intestinal immunity to reduce the risk of infection (Berge et al., 2009; Chamorro et al., 2017).
TABLE 10-18Regression Equations to Estimate IgG in Colostrum Using BRIX Readingsa
Author | N | Breed | Intercept | Slope | R 2 | BPb |
---|---|---|---|---|---|---|
Chigerwe et al., 2008 | 171 | Holstein | –24.7 | 3.96 | 0.41 | 22 |
Bielman et al., 2010 (optical) | 273 | Holstein | –188.262 | 10.730 | 0.51 | 22 |
Bielman et al., 2010 (digital) | 273 | Holstein | –207.434 | 11.561 | 0.53 | 22 |
Morrill et al., 2012 c | 823 | Mixd | –40.509 | 5.2358 | 0.53 | 18 |
Quigley et al., 2013 | 183 | Holstein | –61.896 | 5.666 | 0.75 | 21 |
Bartier et al., 2015 | 460 | Not reported | –29.257 | 3.8393 | 0.43 | 23 |
Morrill et al., 2015 | 58 | Jersey | –49.292 | 6.0052 | 0.63 | 18 |
Løkke et al., 2016 | 126 | Mixd | –58.00 | 4.82 | 0.66 | 22 |
Silva-del-Río et al., 2017 | 202 | Jerseye | –53.3 | 5.5 | 0.58 | 21 |
- a
Equation: Colostrum IgG (g/L) = Intercept + slope × BRIX (%).
- b
Breakpoint = Recommended BRIX concentration (%) providing a minimum of 50 g/L IgG.
- c
BRIX values were calculated from refractive index, which was measured in the study.
- d
Samples from Holstein, Jersey, unclassified and pooled samples.
- e
First- and second-milking colostrum from multiparous Jersey cows only.
The importance of the non-IgG compounds in colostrum is now receiving research attention. Concentration of fat in colostrum decreases as time after parturition increases, and the composition of the lipid fraction (e.g., higher concentrations of cholesterol and very long-chain FAs in colostrum compared to transition milk) also changes (Contarini et al., 2014). Changes in the lipid fraction may be important to calf health and development. Concentrations of several potentially bioactive compounds (e.g., cytokines and lactoferrin) are very high in first-milking colostrum but decrease rapidly after parturition, often by 70 to 80 percent within three milkings after parturition (Sobczuk-Szul et al., 2013). Colostrum contains elevated concentrations of some hormones (e.g., insulin, growth hormone), growth factors such as IGF-1, enzymes, nucleotides, and oligosaccharides (reviewed by McGrath et al., 2016; Ontsouka et al., 2016). High-quality colostrum is a good source of many vitamins (Foley and Otterby, 1978; Godden et al., 2019).
Nutritional manipulations have been investigated as ways to improve colostrum IgG content or absorption. Addition of supranutritional amounts of Se (3 mg per calf) as sodium selenite to colostrum increased IgG absorption in young calves (Kamada et al., 2007; Hall et al., 2014). Feeding dry cows Se-yeast (105 mg/d) tended to increase absorption of IgG by their calves (Hall et al., 2014). Supplementing prepartum cows with 48 g/d of nicotinic acid increased IgG concentrations in colostrum but did not affect absorption of IgG by calves (Aragona et al., 2016, 2019).
Factors affecting yield of first-milking colostrum have not been investigated extensively. Cows fed diets that provided approximately 95, 120, or 130 percent of their energy requirement (based on NRC, 2001) during the last 2 weeks of gestation produced statistically similar yields of first-milking colostrum (5.9, 7.0, and 7.3 kg; Mann et al., 2016). A prospective study on a Jersey farm in Texas determined that month of calving had the greatest effect on colostrum yield (lowest in winter, highest in summer); however, length of previous lactation and length of dry period (longer periods associated with reduced yield) also affected yield (Gavin et al., 2018). Primiparous cows had greater yield of colostrum than multiparous cows but only between late autumn and early spring. Kruse (1970) reported that multiparous cows produced more colostrum than primiparous animals, but variation in yield was extremely large. Colostrum yield may respond to genetic selection (Cabral et al., 2016; Gavin et al., 2018).
Bacterial contamination of colostrum, especially when stored and handled incorrectly, can be substantial (Houser et al., 2008; Morrill et al., 2012; Cummins et al., 2017), and high bacterial counts in colostrum can be detrimental to the calf (Godden et al., 2012; Cummins et al., 2017). Pasteurization of colostrum reduces bacterial counts without affecting Ig concentrations (Godden et al., 2006; Elizondo-Salazar and Heinrichs, 2009). Feeding heat-treated colostrum increases serum concentrations of IgG (Johnson et al., 2007; Elizondo-Salazar and Heinrichs, 2009) and reduces calf morbidity (Godden et al., 2012) compared to feeding raw colostrum.
Colostrum replacer products are widely available and are generally powders that contain a spray-dried source of bovine immunoglobulins, including whey, colostrum, or bovine plasma (Cabral et al., 2013). Some products are composed exclusively of spray-dried bovine colostrum, whereas others contain ingredients such as dry fat, vitamins and minerals, and emulsifiers. The mass of IgG per dose varies widely among products. Results generally suggest that AEA is similar to or less than maternal colostrum. Methods of manufacturing are important to quality of the product and influence the ability of the calf to absorb and use ingested IgG (Chelack et al., 1993; Campbell et al., 2007).
Performance of calves fed a colostrum replacer was improved compared to calves fed poor-quality colostrum (Aly et al., 2013), suggesting that a viable option for colostrum replacer management is to monitor colostrum quality and replace low IgG or contaminated colostrum with a colostrum product that provides 150 to 200 g IgG. Other studies suggest that colostrum replacement products administered as a substitute for maternal colostrum result in lower levels of calf IgG but can confer adequate transfer of immunity provided that an Ig mass of 150 to 200 g is delivered in a timely manner (Lago et al., 2018; Desjardins-Morrissette et al., 2018; Shivley, 2018). Contribution of nutrients such as fat and non-Ig proteins may influence early life energy metabolism; therefore, compositions providing greater amounts of protein and fat in addition to IgG are more effective.
Water and Electrolytes
The importance of clean drinking water, available from the first few days after birth, cannot be overstated. Approximately 75 to 80 percent of the weight of the animal is water. It functions as a solvent for nutrients, thermoregulator, and osmoregulator (Davis and Drackley, 1998). Calves, due to the greater risk of digestive disorders, experience greater problems with water balance than older animals. Water should be offered to calves beginning in the first week of life. Warm water (16°C to 18°C) stimulates water intake compared to offering cold (6°C to 8°C) water (Huuskonen et al., 2011). A lack of water can impair starter intake and BW gain by >30 percent (Kertz et al., 1984). Water intake and dry feed intake are highly correlated (Kertz et al., 1984; Quigley et al., 2006; Hepola et al., 2008; Eckert et al., 2015); thus, offering water early in life will promote dry feed intake and promote early rumen development. Thickett et al. (1981) calculated that for each liter of water consumed during the first 5 weeks of life, calves consumed an additional 82 g of calf starter and increased BW gain by 56 g/d. Similarly, recent data suggest that offering water from birth resulted in greater starter intake, BW gain, and nutrient digestibility compared to offering water beginning at 17 days of age (Wickramasinghe et al., 2019). Frequent replacement of water will encourage consumption. Postweaning ratio of water consumed per kilogram of dry (ca. 90 percent DM) feed intake was approximately 4:1 (Quigley et al., 2006; Hepola et al., 2008).
Calves <1 week of age that were transported to a research facility consumed an average of about 1.5 L/d of free water within the first week of age and from 1.5 to 3 L/d from weeks 1 to 3 of life (Morrison et al., 2019). Other reports suggest that young calves will consume less than 2 L/d for the first 3 weeks and then increase water intake in a fashion correlated with starter intake and weaning (Thickett et al., 1981; Kertz et al., 1984; Quigley et al., 2006).
Calves fed large volumes of milk preweaning will consume less free water compared to calves fed less milk or MR (Hepola et al., 2008; de Passillé et al., 2011). However, large volumes of high-protein MR increased consumption of water (Guindon et al., 2015; Stamey et al., 2021). Quigley et al. (2006) reported that calves fed limited MR tended to consume more water (4 L/d) than calves that were fed up to 0.9 kg of MR powder per day and weaned at 42 days (3.6 L/d).
Calves that develop diarrhea may lose 1 to 10 percent of BW. Mortality is common when dehydration exceeds about 12 percent (Davis and Drackley, 1998). Replacement of both water and electrolytes is essential in the treatment of diarrhea, and scouring calves require additional water to replace that lost in feces. Additional feedings of reconstituted electrolytes are needed to provide liquid in addition to electrolytes. Mixing electrolytes with milk or MR and feeding according to normal milk feeding program do not provide additional water and do not replace water lost due to diarrhea (Smith and Berchtold, 2014). Addition of electrolytes containing Na and glucose to milk or MR may dramatically increase osmolality of the resulting mixture, decreasing the rate of abomasal emptying and increasing the risk of abomasal bloat (Burgstaller et al., 2017). Smith (2009) suggested that osmolality should be less than 700 to 750 mOsm/L of the final solution.
Smith (2009) identified four requirements for oral rehydration solutions for calves: (1) provide sufficient Na to normalize extracellular fluid volume, (2) provide agents (glucose, citrate, acetate, propionate, or glycine) to facilitate absorption of Na and water from the intestine, (3) provide an alkalinizing or buffering agent (Na salts of acetate, propionate, or bicarbonate) to correct metabolic acidosis, and (4) provide energy, because most calves with diarrhea are in negative energy balance. Milk feeding should be continued during treatment with oral rehydration solution (Garthwaite et al., 1994; Smith, 2009). Electrolytes containing sodium bicarbonate (NaHCO3) may inhibit clot formation if preweaned calves are fed liquid diets containing casein (e.g., whole milk or MR containing skim milk); however, products containing other alkalinizing agents (Na salts of acetate, propionate) do not interfere with clot formation.
Disease
According to a survey of dairy farms in the United States (Urie et al., 2018b), morbidity of preweaned dairy heifer calves was 34 percent (859 calves of 2,545 surveyed). Over half of all cases involved digestive signs (usually diarrhea) and another 31 percent was due to respiratory disease. Thus, it is important to consider effects of diseases common to preweaned calves on nutrient requirements and supply of young calves.
Malnutrition increases susceptibility to infection and severity of infections once they occur (França et al., 2009). The relationship between undernutrition and infection was highlighted by Calder and Jackson (2000). Undernutrition compromises barrier and immune functions, thereby allowing pathogens access to the body and decreasing the ability of the host to eliminate pathogens once they enter the body. Subsequently, infections may alter nutritional status by inducing anorexia, reducing nutrient absorption, increasing nutrient requirements, and increasing losses of endogenous nutrients (Calder and Jackson, 2000). Nutrition may play an important role in the outcome of an infection, potentially by affecting pathogen virulence and resource availability (Pike et al., 2019). Furthermore, a decline in nutrient availability caused by, for example, anorexia may separately reduce the immune system's capacity to suppress the infection (Pike et al., 2019).
Effects of disease on nutrient requirements have been researched for many years (e.g., Scrimshaw, 1977); however, effects of disease on nutrient requirements and supply in young calves are not well documented. Data suggest that diarrhea increases rate of passage of nutrients; increases fecal excretion of water, solids, and nutrients; and reduces nutrient digestibility. Diarrhea, however, is only a clinical sign of alimentary tract dysfunction. An important mechanism by which the intestinal tract of a young calf reacts to pathogenic bacteria or viruses or indigestible dietary nutrients is hypersecretion and reduced absorption, resulting in loss of fluids, electrolytes, and nutrients, and the net effect is diarrhea (Radostitis, 1975).
Fecal excretion in a group of scouring calves was 20 to 40 times the total fecal mass excreted by healthy calves (Blaxter and Wood, 1953). Apparent digestibility of DM fell dramatically, in some cases to approximately 40 percent. Digestion of fat was markedly impaired and fecal excretion of FAs increased more than 20-fold. Excretion of purine N was indicative of extensive microbial fermentation, which reduced fecal pH (6.0 in diarrheic calves compared to 6.8 in normal calves). Consequently, fecal solids excretion increased, as did excretion of fecal water. Increased rate of passage was at least partially responsible, as digesta passage time through the gastrointestinal tract declined from 48 hours in normal calves to 6 hours in scouring calves. Furthermore, excretion of Ca, Mg, and P increased in scouring calves in proportion to excretion of fecal soaps. Excretion of Na and K was much greater and was correlated with excretion of water.
Diarrheic calves had increased fecal excretion of fat, lactate, and acetate compared to healthy calves (Youanes and Herdt, 1987). Due to impaired nutrient digestibility, more than 50 percent of diarrheic calves were in negative energy balance even though intake of ME was above normal maintenance ME requirement. Doll et al. (2004) reported that apparent digestibility of milk lipids declined linearly (r = 0.8) with increasing severity of diarrhea in milk-fed calves, and in cases of watery diarrhea (fecal excretion >50 g/kg BW), apparent digestibility of fat was <50 percent. On the other hand, apparent digestibility of crude fiber increased in diarrheic calves experimentally infected with Eimeria bovis (Daugschies et al., 1998), which causes coccidiosis primarily in older calves shortly after weaning. Increase in digestibility was attributed to slower rate of ruminal nutrient passage in infected calves.
Morrison et al. (2019) reported effects of diarrhea on intake of MR, free water, electrolytes, and starter and BW gain for 21 days after arrival at the research farm. Calves that developed diarrhea refused MR and consumed less total solids (15.9 versus 16.6 kg) and total water (104 versus 108 L) from MR over the 21-day period compared to healthy calves. Total starter DMI during the first 21 days was decreased about 40 percent in diarrheic calves. As a result, cumulative total DMI from all sources was less when calves had diarrhea. Healthy calves were heavier and had greater hip height and heart girth at 21 days compared to diarrheic calves.
Improving the ability to formulate diets that maximize production and maintain competence of immune function and disease resistance would increase both welfare and profitability (Kogut and Klasing, 2009). Further research is needed in young calves to refine the understanding of the interactions among nutrient requirements, nutrient supply, immune function, and resistance to disease to improve the ability to meet requirements for both optimal growth and disease resistance.
Milk Replacers
According to a recent review of feeding practices in the United States (Urie et al., 2018a), 39 percent of dairy operations used MR alone and another 38.5 percent used MR in combination with milk. Numerous changes have occurred in the formulation of MR in the dairy industry since publication of NRC (2001) gudelines (Kertz and Loften, 2013), primarily related to use of various protein sources in MR formulas. The ability of these protein sources to supply an adequate amount and profile of AAs for growth of preruminant calves depends on the AA profile of the protein, the quality of the manufacturing process, and the ability of the calf to digest the protein. High temperatures during drying can damage proteins and lessen their biologic value (Wilson and Wheelock, 1972). Heat damage can be determined by various methods, including measurement of furosine in the ingredients or MR (Guerra-Hernandez et al., 2002). Furthermore, antinutritional factors present in some protein sources such as soy or pea proteins can decrease AA net absorption and efficiency of AA use (Huisman, 1989; Lallès, 1993).
Proteins in MR are mainly dairy in origin and are mostly from whey proteins and dried skim milk. Skim milk can be replaced by whey protein without change in digestibility or animal performance (Terosky et al., 1997; Lammers et al., 1998; Huuskonen et al., 2017). Although casein-containing ingredients (e.g., skim milk) will generally form a clot in the abomasum after consumption, this does not appear to be an advantage to clot formation in terms of digestibility or animal performance (Davis and Drackley, 1998; Longenbach and Heinrichs, 1998).
Research on and use of nonmilk proteins in MR have advanced significantly in the past 20 years. Vegetable proteins such as hydrolyzed wheat gluten, soy protein concentrate, and pea protein are processed to improve solubility and digestibility. Although older work suggests that incompletely processed ingredients such as soy flour may cause allergic reactions in the intestine of calves (Lallès et al., 1996; Dréau and Lalles, 1999), more recent data using improved protein production methods indicate that vegetable proteins can provide a portion of the MR formula with animal performance similar to all-milk formulas. For example, performance of calves fed MR in which hydrolyzed wheat protein replaced approximately 50 percent of milk proteins was similar to calves fed all-milk proteins (Terui et al., 1996; Ortigues-Marty et al., 2003; Castro et al., 2016c) although nutrient digestibility was slightly lower (Branco-Pardal et al., 1995). Conversely, Hill et al. (2008d) reported lower growth when calves were fed MR containing hydrolyzed wheat gluten compared to milk protein. More highly processed pea protein isolate had digestibility similar to that of milk protein, whereas pea protein concentrate (still containing starch and oligosaccharides) was poorly used (Bhatty and Christison, 1980).
Other alternative protein sources include spray-dried animal plasma (Morrill et al., 1995; Quigley and Bernard, 1996; Quigley and Drew, 2000; Quigley et al., 2002; Quigley and Wolfe, 2003; Raeth et al., 2016; Vasquez et al., 2017; Morrison et al., 2017) and whole egg or egg yolk (Quigley et al., 2002; Touchette et al., 2003; Santoro et al., 2004; Dennis et al., 2017). Significant variability among reports suggests that quality and processing of raw materials (e.g., heating, spray-drying) may influence digestibility and animal performance.
An important consideration in MR formulation is to meet AA requirements of the calf, especially when nonmilk proteins are incorporated into the MR (Morrison et al., 2017; Vasquez et al., 2017). Hill et al. (2008e) reported that optimal concentrations of Lys and Met in MR containing 26 percent CP were 2.34 and 0.72 percent of DM, respectively. Others (Wang et al., 2012; Margerison et al., 2013) have generally supported this finding, although Castro et al. (2016c) concluded that addition of Met was unnecessary when MR (DM basis) contained >28 percent CP and 0.57 percent Met. Low rates of BW gain due to stressors or ill health may limit expression of improved nutrition associated with AA formulation (da Silva et al., 2018). Addition of arginine (Arg) or histidine (His) to MR containing 27 percent CP, 2.43 percent Lys, 0.76 percent Met, 0.68 percent Arg, and 0.48 percent His (DM basis) had no effect on calf performance when MR were fed at 680 g of solids daily (Hill et al., 2011b).
Fat sources in MR are typically tallow, choice white grease, lard, or palm oil components. Smaller amounts (typically <15 percent of total lipid) of coconut oil are often included to enhance digestibility and for the antimicrobial effects of the medium-chain FAs (López-Colum et al., 2019). The degree of homogenization is critical for high fat digestibility (Raven, 1970). Emulsifiers, such as lecithin and monoglycerides, often are added to enhance mixing characteristics and fat digestibility. In general, vegetable oils and fat sources that contain large amounts of free FAs are poorly digested by calves (Jenkins et al., 1985). The primary carbohydrate in MR is lactose from dried whey. Other carbohydrates such as maltodextrin or starch are poorly digested, particularly in young calves, and are not recommended for calves less than 4 weeks of age.
Increasing the protein to energy ratio is beneficial when increased amounts of MR are offered (Bartlett et al., 2006; Bascom et al., 2007). Such changes in the composition of MR have achieved greater growth rates and energy retention, along with lower fat and greater lean tissue deposition (Diaz et al., 2001; Blome et al., 2003; Bartlett et al., 2006). For growth rates of 0.2 to 0.4 kg/d, protein/energy ratios (percent CP/ME Mcal/kg) generally should be in the range of 4.2 to 4.9, for growth of 0.4 to 0.7 kg/d in the range of 5 to 5.7, and for growth >0.7 kg/d in the range of 5.8 to 6.2.
Management of MR is critical to ensuring adequate growth rates and health of calves. MRs should be mixed in water at temperatures recommended by the manufacturer. Consistency of the amount of liquid fed daily to calves improves intake and BW gain (Hill et al., 2008b).
Solids concentration of whole milk (Holstein) is about 12.5 percent, and most MRs are formulated to be reconstituted to 12.5 to 15 percent solids. Increasing solids is a strategy to increase nutrient intake when feed management limits volume that can be fed—for example, when feeding from nipple bottles. Increasing solids concentration of MR to 20 percent DM did not influence nutrient digestibility (Ternouth et al., 1985a,b Azevedo et al., 2016), although free water intake increased (Ternouth et al., 1985a). Jenny et al. (1982) reported that feces became more fluid with increasing solids concentration. Abomasal bloat seems to become more prevalent with MR concentrations over approximately 15 percent (Burgstaller et al., 2017). Availability and management of free water are important if attempting to feed solids concentrations >17 percent.
Whole Milk
Whole milk, whether saleable or unsaleable, remains the most commonly used liquid feed for calves prior to weaning on dairy farms (Urie et al., 2018a). Nonsaleable milk, also called waste milk, is not suitable for commercial sale and may be produced by cows immediately after calving, cows with active mammary infections, or those that have been treated with antibiotics.
Composition
Although bulk saleable milk has a reasonably consistent nutrient concentration, waste milk is by nature more variable (Jorgensen et al., 2006; Moore et al., 2009). Solids and nutrient content of waste milk can vary from that of whole milk, depending on the contribution of colostrum and transition milk (which increases solids, protein, and fat), water (contamination from washing procedures), and milk from sick or treated cows. Moore et al. (2009) reported that waste milk from one calf ranch in California averaged 11.2 percent solids (range, 5.1 to 13.4 percent), which was significantly different from the average solids in saleable milk.
Variation in nutrient content of milk may negatively influence calf performance. For example, Hill et al. (2008b) fed two MRs at either a variable daily rate (0.525 to 0.788 kg DM/d) or a fixed daily rate (0.653 kg DM/d) to provide an average of 0.653 kg DM/d in four treatments. The MRs were either 27 percent CP and 31 percent fat (to simulate Holstein milk) or 27 percent CP and 17 percent fat. Calves fed a fixed daily rate of MR solids grew faster, ate more calf starter, and were more efficient compared to calves that were fed the variable amount of milk solids daily. Solids content of milk can be standardized with addition of powdered MR or specialized extender products designed for that purpose. Adjusting solids concentration up to 20 percent DM can be done without negative effect on calf performance (Azevedo et al., 2016), if free water is always available. However, increasing solids concentration of milk increases osmolality and may influence abomasal outflow rates if osmolality exceeds approximately 600 mOsm/kg (Burgstaller et al., 2017). In addition, milk contains some imbalances in vitamins (vitamins D and E) and minerals (Fe, Mn, Zn, Cu, I, Se) compared to nutrient requirements, and supplementing whole milk with vitamins and minerals and increasing the protein to energy ratio may improve growth and feed efficiency (Glosson et al., 2015).
Microbial Contamination and Pasteurization
Waste milk may be contaminated due to mastitis and other organisms that may be passed from cow to calf. Waste milk has been implicated in the transmission of numerous disease-causing organisms, including Cryptosporidium parvum, Mycoplasma bovis, and Mycobacterium avium subsp. paratuberculosis (Kesler, 1981; Selim and Cullor, 1997; Walz et al., 1997; Butler et al., 2000; Stabel, 2001). Pasteurization reduces bacterial counts in milk (Butler et al., 2000; Stabel, 2001; Stabel et al., 2004; Elizondo-Salazar et al., 2010) and improves health and growth of calves (Jamaluddin et al., 1996; Selim and Cullor, 1997; Armengol and Fraile, 2016). Regrowth of bacteria following pasteurization is possible if the waste milk is not cooled quickly and is stored for extended periods (Elizondo-Salazar et al., 2010); therefore, milk should be fed within an hour of pasteurization. Routine evaluation of pasteurization efficacy is also necessary by monitoring total plate counts and coliform counts. Jorgensen et al. (2006) reported that pasteurization was ineffective in up to 13 percent of operations evaluated in the study. Ruzante et al. (2008) reported survival of M. avium subsp. paratuberculosis in pasteurized waste milk from commercial dairy farms.
Antibiotic Residues
A significant risk of using waste milk is the transmission of antibiotic residues that may promote development of antibiotic resistance in bacteria in the milk and the animal. Even low levels of antibiotics fed to calves influence the composition of intestinal microflora (Yousif et al., 2018), the presence of antimicrobial-resistant organisms (Langford et al., 2003; Randall et al., 2014; Maynou et al., 2017; Pereira et al., 2014, 2018; Tempini et al., 2018), and calf nutrient metabolism (Pereira et al., 2018; Li et al., 2019). Maynou et al. (2017) reported changes in composition of both fecal and nasal microbiota, pointing to systemic effects on animal metabolism or direct contact of nasal microbiota to other animals or waste milk. Development of antibiotic-resistant bacteria from the use of waste milk is a significant risk to animal and human health. If possible, only pasteurized waste milk from cows not treated with antimicrobials should be fed (Aust et al., 2013; EFSA Panel on Biological Hazards, 2017), and waste milk from treated cows should be discarded in an environmentally safe manner by incorporation into manure (Payer and Holmes, 1994).
Feed Additives
A wide variety of additives may be included in the diets of young calves. Generally, these additives can be categorized by function: stabilize or modify gastrointestinal function, support gut or systemic immune function, interfere with growth of potential pathogens, or increase digestibility. Some products may fit into multiple categories, depending on dosage and application.
The effectiveness of a feed additive to improve growth, intake, health, or efficiency necessarily depends on the mode of action of the additive and whether an effective dose was administered at the appropriate time. Additives that may influence calf health by reducing exposure to ingested pathogens (e.g., yeast products, plasma, and essential oils [EOs]) may not be effective in reducing diarrhea on farms where pathogen exposure is already low. Maintenance of viability of living organisms (yeasts and bacteria) and functional proteins (immunoglobulins, lactoferrin) intended to alter digestive function is also an important consideration in feed manufacturing, preparation, and storage. Practices such as pelleting calf starters at high temperatures or extended storage of products at temperatures >40°C may degrade effectiveness and economic benefit of additives.
The committee organized feed additives for calves by primary function in the animal. However, the committee recognizes that many additives have multiple functional roles—for example, yeast culture has been shown to modify the rumen environment (Xiao et al., 2016; Ma et al., 2020a,b) but also may influence intestinal immunity (Ma et al., 2020b) and severity of diarrhea (Alugongo et al., 2017), although not all studies have reported significant changes in gut permeability or plasma metabolites in response to yeast culture addition (Pisoni and Relling, 2020). Therefore, the groupings should be considered as a means to facilitate presentation and are not indicative of sole functions of the respective additives.
Selected additives reported for use in calves are intended to support or maintain rumen function (ionophores, rumen buffers, live yeast), are used as antimicrobials (antibiotics, lactoferrin, EOs, immunoglobulins), modulate gut immunity or microflora (oligosaccharides, yeast culture, direct-fed microbials), and stimulate organ growth (butyrate).
Rumen Function
Ionophores include monensin, lasalocid, and decoquinate and are added to feeds to modify rumen fermentation and control coccidiosis. Dietary lasalocid and decoquinate are effective in control of coccidiosis (Hoblet et al., 1989; Heinrichs et al., 1990; Heinrichs and Bush, 1991; Eicher-Pruiett et al., 1992; Quigley et al., 1997a). Supplementation in calf starter requires adequate feed intake to achieve effective dosages, but infection with coccidia often occurs before starter intake is sufficient (Quigley et al., 1997a). To counter this, amprolium is often added to milk or MR to control Eimeria sp. (Ghanem et al., 2008), although amprolium can induce thiamin deficiency unless thiamin is supplemented in milk (Dodd et al., 1996). Concentrations of ionophores effective against coccidia may be insufficient to influence rumen VFA profiles (Klotz and Heitmann, 2006).
Addition of NaHCO3 to calf starters helps maintain rumen pH and normal rumen function. Addition of 3 percent bicarbonate in calf starters to young calves increased rumen pH (Quigley et al., 1992), although no performance changes were reported. Others have added 1 to 3 percent of DM as NaHCO3 with no response (Foroozandeh and Shakeri, 2017) or improved growth and starter intake (Curnick et al., 1983). Addition of 2 percent NaHCO3 was shown to increase K excretion by the calf (Tucker et al., 1991).
Live yeast added to calf starter (Saccharomyces cerevisiae) or milk (S. cerevisiae, spp. boulardii) for 42 days increased or tended to increase intake and growth while reducing days with diarrhea (Galvão et al., 2005). Fomenky et al. (2017) reported that S. cerevisiae spp. boulardii altered intestinal lactobacilli populations and colonic morphology. Improved performance may also be associated with stabilization of rumen fermentation during the rumen development period (Terré et al., 2015a,b). However, other studies reported no significant performance benefit to addition of yeast products (Pinos-Rodriguez et al., 2008; Huuskonen and Pesonen, 2015). Typical inclusion rate for live yeast products is approximately 1.5 × 106 cfu/g of calf starter.
Antimicrobials
Antibiotics have been used as feed additives for young calves since the 1950s (Kertz et al., 2017) and have consistently resulted in improved health and performance of calves (Kiser, 1976; Tomkins and Jaster, 1991; Quigley et al., 1997b; Quigley and Drew, 2000; Heinrichs et al., 2003; Berge et al., 2005; Kehoe and Carlson, 2015). However, because of concerns regarding development and transmission of antibiotic resistance in gut microflora of calves fed antibiotics (e.g., Berge et al., 2006), the addition of antimicrobials such as neomycin, oxytetracycline, and chlortetracycline to diets of young calves as a growth promoter is no longer permitted in the United States, without veterinary prescription (Mzyk et al., 2017).
Supplemental lactoferrin can improve intake (Joslin et al., 2002), decrease fecal scores, and reduce the number of days that calves experience diarrhea (Roblee et al., 2003; Prenner et al., 2007), although it has not always been effective (English et al., 2007). It was effective in reducing the risk of death in calves with diarrhea (Habing et al., 2017) when fed at the onset of clinical signs of diarrhea for 3 days; however, a field trial did not report improved health in calves with diarrhea that were treated with lactoferrin (Pempek et al., 2019). Adding lactoferrin up to 1 g/d to colostrum replacer reduced apparent efficiency of IgG absorption in newborn calves (Shea et al., 2009). Its mode of action may be via anti-inflammatory functions (Kushibiki et al., 2008).
EOs, including garlic, cinnamaldehyde, eugenol, capsicum, and anethole, among others, are considered antimicrobial and have been evaluated as a means of manipulating rumen fermentation. At this writing, data on the use of EOs in calf diets are sparse. Blends of EOs added to calf starter improved DMI, BW gain, and feed efficiency (Kazemi-Bonchenari et al., 2018; Salazar et al., 2019). The addition of EOs to starter appears to influence rumen fermentation patterns (Santos et al., 2015; Saeedi et al., 2017), which may be valuable in promoting rumen development. Froehlich et al. (2017) reported that calves fed a blend of EOs (carvacrol, caryophyllene, cymene, cineole, terpinene, and thymol) at 2.5 g/d had greater BW gain than calves fed EOs at 5.0 or 7.5 g/d, as well as greater BW gain and increased body length and withers heights to 56 days compared with another group of calves fed a yeast cell wall product at 4 g/d. Types of EOs used and dosage in each product affect the likelihood of a positive response, but the mechanism of action remains unknown where positive responses have been observed.
Ig, including colostrum, colostrum replacers, and animal plasma, have been fed to calves in MR. Orally administered Ig are immunologically active in the gut and may provide local protection against potential pathogens. Reduced days with diarrhea occurred when calves were fed MR containing 4 to 5 percent plasma (Quigley and Drew, 2000; Quigley et al., 2002; Quigley and Wolfe, 2003) or bovine colostrum (Berge et al., 2009; Chamorro et al., 2017). Another source of Ig is IgY produced from eggs collected from chickens that have been hyperimmunized against one or more pathogens. Typical inclusion rates are approximately 5 to 10 g/d of egg yolk (Yokoyama et al., 1988; Ikemori et al., 1997; Kuroki et al., 1997).
Modifiers of Gut Immunity or Microflora
Oligosaccharides have been used to manipulate bacterial flora of the intestinal tract of animals, potentially reducing the incidence of disease. These carbohydrates reduce adhesion of certain bacterial species to the intestinal epithelium, most notably Escherichia coli (K99+) and Salmonella sp. Oligosaccharides may also increase the growth of beneficial intestinal bacteria, including lactobacilli and bifidobacteria. Oligosaccharides containing mannose and fructose have been fed to calves to improve intestinal health and to reduce the incidence of disease (Morrison et al., 2010; Grand et al., 2013; Heinrichs et al., 2013; Kara et al., 2015), although not all studies have reported significant benefit to the addition of oligosaccharides to MR (Terré et al., 2007; Hill et al., 2008c; Heinrichs et al., 2009; Quezada-Mendoza et al., 2011; da Silva et al., 2012). Heinrichs et al. (2003) reported that the addition of mannose oligosaccharides to MR was equally effective as neomycin plus oxytetracycline in controlling the incidence and severity of diarrhea.
Galactosyl-lactose, a trisaccharide (galactose plus lactose) that is produced by enzymatic treatment of whey using β-galactosidase derived from Aspergillus oryzae, was equally effective as antibiotics in reducing diarrhea when added at 1 percent of DM to MR (Quigley et al., 1997b). MR formulated to have about 3 percent galactooligosaccharides (GOSs) produced notable changes in intestinal microflora but did not benefit calf growth or health, perhaps because lactose content was decreased by the GOS production process (Castro et al., 2016a,b.
Yeast culture, when added to high-grain diets, reduces ruminal lactate concentrations (Quigley et al., 1992) and alters rumen bacterial populations and butyrate concentrations (Xiao et al., 2016) in young calves. Improved growth (Leismaster et al., 2004) and health (Magalhães et al., 2008; Fokkink et al., 2009; Alugongo et al., 2017) were reported when yeast culture was included in the diet, although not all studies have reported improved calf performance (Fokkink et al., 2009). Yeast culture in MR and calf starter also reduced effects of challenge with Salmonella enterica serotype Typhimurium (Brewer et al., 2014; Harris et al., 2017). Depending on the specific formulation, typical inclusion rates for yeast culture are 3.5 to 14 g/d. Hydrolyzed yeast also can improve immune response in young calves when added to MR (Kim et al., 2011). Conversely, preparations primarily of nonviable yeast did not significantly affect calf performance (Saldana et al., 2019).
Direct-fed microbial (DFM) products may be added to calf MR to influence gut microflora and protect against potential pathogenic infection. Some studies have reported positive effects on calf health (Abe et al., 1995; Timmerman et al., 2005; Morrison et al., 2010) or performance (Cruywagen et al., 1996); however, others (Geiger et al., 2014) found no effect of these additives. Inclusion rates of DFM products vary depending on the types of bacteria, yeast, and fungi included in the product.
Butyrate
Adding sodium or calcium butyrate has sometimes (Guilloteau et al., 2009, 2010; Roh et al., 2018) but not always improved calf health, growth, and efficiency when included in milk (Araujo et al., 2015; Frieten et al., 2017), calf starters (McCurdy et al., 2019), or both (Górka et al., 2011a,b, 2014, 2018). Typical inclusion rate for sodium butyrate is 0.3 percent of DM in MR or calf starter. Sodium butyrate, when fed in combination with other FAs, has increased intake, BW gain, digestibility of nutrients, and improved overall immune response (Hill et al., 2007b,c, 2011a,c; Quigley et al., 2019), improved gut morphology (Koch et al., 2019), and altered microbial profiles in the hindgut (O'Hara et al., 2018). Guilloteau et al. (2009) concluded that 0.3 percent of DM as butyrate enhanced production of digestive enzymes and increased absorptive capability in the upper small intestine. Kato et al. (2011) opined that improved performance and digestibility by butyrate was due to improved insulin sensitivity and a better digestive functional development.
REFERENCES
- Abdelgadir IEO, Morrill JL, Higgins JJ. Effect of roasted soybeans and corn on performance and ruminal and blood metabolites of dairy calves. J. Dairy Sci. 1996a;79:465–474. [PubMed: 8708109]
- Abdelgadir IEO, Morrill JL, Higgins JJ. Ruminal availabilities of protein and starch: Effects on growth and ruminal and plasma metabolites of dairy calves. J. Dairy Sci. 1996b;79:283–290. [PubMed: 8708089]
- Abdelrahman MM, Kincaid RL. Effect of selenium supplementation of cows on maternal transfer of selenium to fetal and newborn calves. J. Dairy Sci. 1995;78:625–630. [PubMed: 7782518]
- Abe F, Ishibashi N, Shimamura S. Effect of administration of bifidobacteria and lactic acid bacteria to newborn calves and piglets. J. Dairy Sci. 1995;78:2838–2846. [PubMed: 8675766]
- Ahmed AF, Constable PD, Misk NA. Effect of feeding frequency and route of administration on abomasal luminal pH in dairy calves fed milk replacer. J. Dairy Sci. 2002;85:1502–1508. [PubMed: 12146482]
- Ainslie SJ, Fox DG, Perry TC. Management systems for Holstein steers that utilize alfalfa silage and improve carcass value. J. Anim. Sci. 1992;70:2643–2651. [PubMed: 1399877]
- Akayezu JM, Linn JG, Otterby DE, Hansen WP, Johnson DG. Evaluation of calf starters containing different amounts of crude protein for growth of Holstein calves. J. Dairy Sci. 1994;77:1882–1889. [PubMed: 7929949]
- Alexander G, Bennett JW, Gammell RT. Brown adipose tissue in the newborn calf (Bos taurus). J. Physiol. 1975;244:223–234. [PMC free article: PMC1330754] [PubMed: 1123748]
- Alharthi AS, Batistel F, Abdelmegeid MK, Lascano G, Parys C, Helmbrecht A, Trevisi E, Loor JJ. Maternal supply of methionine during late-pregnancy enhances rate of Holstein calf development in utero and postnatal growth to a greater extent than colostrum source. J. Anim. Sci. Biotechnol. 2018;9:83. [PMC free article: PMC6251175] [PubMed: 30498570]
- Alugongo GM, Xiao JX, Chung YH, Dong SZ, Li SL, Yoon I, Wu ZH, Cao ZJ. Effects of Saccharomyces cerevisiae fermentation products on dairy calves: Performance and health. J. Dairy Sci. 2017;100:1189–1199. [PubMed: 28012624]
- Aly SS, Pithua P, Champagne JD, Haines DM. A randomized controlled trial on preweaning morbidity, growth and mortality in Holstein heifers fed a lacteal-derived colostrum replacer or pooled maternal colostrum. BMC Vet. Res. 2013;9:168. [PMC free article: PMC3765984] [PubMed: 23965249]
- Amado L, Berends H, Leal LN, Wilms J, Van Laar H, Gerrits WJJ, Martín-Tereso J. Effect of energy source in calf milk replacer on performance, digestibility, and gut permeability in rearing calves. J. Dairy Sci. 2019;102:3994–4001. [PubMed: 30852011]
- Ametaj BN, Nonnecke BJ, Franklin ST, Horst RL, Bidlack WR, Stuart RL, Beitz DC. Dietary vitamin A modulates the concentrations of RRR-alpha-tocopherol in plasma lipoproteins from calves fed milk replacer. J. Nutr. 2000;130(3):629–636. [PubMed: 10702596]
- Anderson KL, Nagaraja TG, Morrill JL. Ruminal metabolic development in calves weaned conventionally or early. J. Dairy Sci. 1987a;70:1000–1005. [PubMed: 3597925]
- Anderson KL, Nagaraja TG, Morrill JL, Avery TB, Galitzer SJ, Boyer JE. Ruminal microbial development in conventionally or early weaned calves. J. Anim. Sci. 1987b;64:1215–1226. [PubMed: 3571026]
- Appleman RD, Owen FG. Breeding, housing, and feeding management. J. Dairy Sci. 1975;58:447–464.
- Aragona KM, Chapman CE, Pereira ABD, Isenberg BJ, Standish RB, Maugeri CJ, Cabral RG, Erickson PS. Prepartum supplementation of nicotinic acid: Effects on health of the dam, colostrum quality, and acquisition of immunity in the calf. J. Dairy Sci. 2016;99:3529–3538. [PubMed: 26898282]
- Aragona KM, Rice EM, Engstrom M, Erickson PS. Supplementation of nicotinic acid to prepartum Holstein cows increases colostral immunoglobulin G, excretion of urinary purine derivatives, and feed efficiency in calves. J. Dairy Sci. 2019;103:2287–2302. [PubMed: 31882224]
- Araujo G, Terré M, Bach A. Interaction between milk allowance and fat content of the starter feed on performance of Holstein calves. J. Dairy Sci. 2014;97:6511–6518. [PubMed: 25129500]
- Araujo G, Terré M, Mereu A, Ipharraguerre IR, Bach A. Effects of supplementing a milk replacer with sodium butyrate or tributyrin on performance and metabolism of Holstein calves. Anim. Prod. Sci. 2015;56:1834–1841.
- ARC (Agricultural Research Council). The Nutrient Requirements of Ruminant Livestock. Farnham Royal, England: Commonwealth Agricultural Bureaux; 1980.
- Arieli A, Schrama JW, Van Der Hel W, Verstegen MWA. Development of metabolic partitioning of energy in young calves. J. Dairy Sci. 1995;78:1154–1162. [PubMed: 7622726]
- Armengol R, Fraile L. Colostrum and milk pasteurization improve health status and decrease mortality in neonatal calves receiving appropriate colostrum ingestion. J. Dairy Sci. 2016;99:4718–4725. [PubMed: 26995131]
- Aust V, Knappstein K, Kunz H-J, Kaspar H, Wallmann J, Kaske M. Feeding untreated and pasteurized waste milk and bulk milk to calves: Effects on calf performance, health status and antibiotic resistance of faecal bacteria. J. Anim. Physiol. Anim. Nutr. 2013;97:1091–1103. [PubMed: 23205592]
- Azevedo RA, Machado FS, Campos MM, Lopes DRG, Costa SF, Mantovani HC, Lopes FCF, Marcondes MI, Pereira LGR, Tomich TR, Coelho SG. The effects of increasing amounts of milk replacer powder added to whole milk on passage rate, nutrient digestibility, ruminal development, and body composition in dairy calves. J. Dairy Sci. 2016;99:8746–8758. [PubMed: 27592428]
- Bach A. Optimizing performance of the offspring: Nourishing and managing the dam and postnatal calf for optimal lactation, reproduction, and immunity. J. Anim. Sci. 2012;90:1835–1845. [PubMed: 21926322]
- Bach A, Gimenez A, Juaristi JL, Ahedo J. Effects of physical form of a starter for dairy replacement calves on feed intake and performance. J. Dairy Sci. 2007;90:3028–3033. [PubMed: 17517745]
- Bach A, Terré M, Pinto A. Performance and health responses of dairy calves offered different milk replacer allowances. J. Dairy Sci. 2013;96:7790–7797. [PubMed: 24119797]
- Bagath M, Krishnan G, Devaraj C, Rashamol VP, Pragna P, Lees AM, Sejian V. The impact of heat stress on the immune system in dairy cattle: A review. Res. Vet. Sci. 2019;126:94–102. [PubMed: 31445399]
- Ballou MA. Immune responses of Holstein and Jersey calves during the preweaning and immediate postweaned periods when fed varying planes of milk replacer. J. Dairy Sci. 2012;95:7319–7330. [PubMed: 23063147]
- Ballou MA, DePeters EJ. Supplementing milk replacer with fatty acids from fish oil on immunocompetence and health of Jersey calves. J. Dairy Sci. 2008;91:3488–3500. [PubMed: 18765608]
- Ballou MA, Cruz GD, Pittroff W, Keisler DH, DePeters EJ. Modifying the acute phase response of Jersey calves by supplementing milk replacer with omega-3 fatty acids from fish oil. J. Dairy Sci. 2008;91:3478–3487. [PubMed: 18765607]
- Ballou MA, Cobb CJ, Earleywine TJ, Obeidat BS. Interaction of breed and plane of milk replacer nutrition on the performance of pre- and postweaned dairy calves. Prof. Anim. Sci. 2013;29:116–123.
- Ballou MA, Hanson DL, Cobb CJ, Obeidat BS, Sellers MD, Pepper-Yowell AR, Carroll JA, Earleywine TJ, Lawhon SD. Plane of nutrition influences the performance, innate leukocyte responses, and resistance to an oral Salmonella enterica serotype Typhimurium challenge in Jersey calves. J. Dairy Sci. 2015;98:1972–1982. [PubMed: 25597967]
- Bartier AL, Windeyer MC, Doepel L. Evaluation of on-farm tools for colostrum quality measurement. J. Dairy Sci. 2015;98:1878–1884. [PubMed: 25582586]
- Bartlett KS, McKeith FK, VandeHaar MJ, Dahl GE, Drackley JK. Growth and body composition of dairy calves fed milk replacers containing different amounts of protein at two feeding rates. J. Anim. Sci. 2006;84:1454–1467. [PubMed: 16699102]
- Bascom SA, James RE, McGilliard ML, Van Amburgh ME. Influence of dietary fat and protein on body composition of Jersey bull calves. J. Dairy Sci. 2007;90:5600–5609. [PMC free article: PMC7094342] [PubMed: 18024752]
- Bateman HG II, Hill TM, Aldrich JM, Schlotterbeck RL. Effects of corn processing, particle size, and diet form on performance of calves in bedded pens. J. Dairy Sci. 2009;92:782–789. [PubMed: 19164692]
- Batista CG, Coelho SG, Rabelo E, Lana AMQ, Carvalho AU, Reis RB, Saturnino HM. Performance and health of calves fed milk without antimicrobials residue or milk from mastitis treated cows with or without probiotic. Arq. Bras. Med. Vet. Zootec. 2008;60:185–191.
- Batistel F, Alharthi AS, Wang L, Parys C, Pan YX, C CardosoF, Loor JJ. Placentome nutrient transporters and mammalian target of rapamycin signaling proteins are altered by the methionine supply during late gestation in dairy cows and are associated with newborn birth weight. J. Nutr. 2017;147:1640–1647. [PubMed: 28768834]
- Beharka AA, Nagaraja TG, Morrill JL, Kennedy GA, Klemm RD. Effects of form of the diet on anatomical, microbial, and fermentative development of the rumen of neonatal calves. J. Dairy Sci. 1998;81:1946–1955. [PubMed: 9710764]
- Bell AW. Regulation of organic nutrient metabolism during transition from late pregnancy to early lactation. J. Anim. Sci. 1995;73:2804–2819. [PubMed: 8582872]
- Bell AS, Slepitis R, Ehrhardt RA. Growth and accretion of energy and protein in the gravid uterus during late pregnancy in Holstein cows. J. Dairy Sci. 1995;78:1954–1961. [PubMed: 8550905]
- Berends H, Vidal M, Terré M, Leal LN, Martin-Tereso J, Bach A. Effects of fat inclusion in starter feeds for dairy calves by mixing increasing levels of a high-fat extruded pellet with a conventional highly fermentable pellet. J. Dairy Sci. 2018;101:10962–10972. [PubMed: 30316590]
- Berge AC, Lindeque P, Moore DA, Sischo WM. A clinical trial evaluating prophylactic and therapeutic antibiotic use on health and performance of preweaned calves. J. Dairy Sci. 2005;88:2166–2177. [PubMed: 15905446]
- Berge AC, Moore DA, Sischo WM. Field trial evaluating the influence of prophylactic and therapeutic antimicrobial administration on antimicrobial resistance of fecal Escherichia coli in dairy calves. Appl. Environ. Microbiol. 2006;72:3872–3878. [PMC free article: PMC1489621] [PubMed: 16751491]
- Berge AC, Besser TE, Moore DA, Sischo WM. Evaluation of the effects of oral colostrum supplementation during the first fourteen days on the health and performance of preweaned calves. J. Dairy Sci. 2009;92:286–295. [PMC free article: PMC7125711] [PubMed: 19109287]
- Besser TE, McGuire TC, Gay CC, Pritchett LC. Transfer of functional immunoglobulin G (IgG) antibody into the gastrointestinal tract accounts for IgG clearance in calves. J. Virol. 1998;62:2234–2237. [PMC free article: PMC253360] [PubMed: 3373569]
- Bhatty RS, Christison GI. Digestibility of pea proteins by pre-ruminant calves. Can. J. Anim. Sci. 1980;60:925–930.
- Bielmann V, Gillan J, Perkins NR, Skidmore AL, Godden S, Leslie KE. An evaluation of Brix refractometry instruments for measurement of colostrum quality in dairy cattle. J. Dairy Sci. 2010;93:3713–3721. [PubMed: 20655440]
- Blättler U, Hammon HM, Morel C, Philipona C, Rauprich A, Romé V, Le Huërou-Luron I, Guilloteau P, Blum JW. Feeding colostrum, its composition and feeding duration variably modify proliferation and morphology of the intestine and digestive enzyme activities of neonatal calves. J. Nutr. 2001;131:1256–1263. [PubMed: 11285335]
- Blaxter KL, Mitchell HH. The factorization of the protein requirements of ruminants and of the protein value of feeds, with particular reference to the significance of the metabolic fecal nitrogen. J. Anim. Sci. 1948;7:351–372.
- Blaxter KL, Wood WA. The nutrition of the young Ayrshire calf: 4. Some factors affecting the biological value of protein determined by nitrogen balance methods. Br. J. Nutr. 1951;5:55–67. [PubMed: 14886521]
- Blaxter KL, Wood WA. Some observations on the biochemical and physiological events associated with diarrhea in calves. Vet. Rec. 1953;65:889–892.
- Blome RM, Drackley JK, McKeith FK, Hutjens MF, McCoy GC. Growth, nutrient utilization, and body composition of dairy calves fed milk replacers containing different amounts of protein. J. Anim. Sci. 2003;81:1641–1655. [PMC free article: PMC7110375] [PubMed: 12817512]
- Borderas TF, de Passillé AM, Rushen J. Feeding behavior of calves fed small or large amounts of milk. J. Dairy Sci. 2009;92:2843–2852. [PubMed: 19448018]
- Bowen Yoho WS, Swank VA, Eastridge ML, O'Diam KM, Daniels KM. Jersey calf performance in response to high-protein, high-fat liquid feeds with varied fatty acid profiles: Intake and performance. J. Dairy Sci. 2013;96:2494–2506. [PubMed: 23462163]
- Branco-Pardal P, Lallès JP, Formal M, Guilloteau P, Toullec R. Digestion of wheat gluten and potato protein by the preruminant calf: Digestibility, amino acid composition and immunoreactive proteins in ileal digesta. Reprod. Nutr. Dev. 1995;35:639–654. [PubMed: 8534358]
- Brewer MT, Anderson KL, Yoon I, Scott MF, Carlson SA. Amelioration of salmonellosis in pre-weaned dairy calves fed Saccharomyces cerevisiae fermentation products in feed and milk replacer. Vet. Microbiol. 2014;172:248–255. [PubMed: 24954478]
- Brody S. Bioenergetics and growth with Special Reference to the Energetic Efficiency Complex in Domestic Animals. New York: Reinhold; 1945.
- Brown EG, VandeHaar MJ, Daniels KM, Liesman JS, Chapin LT, Forrest JW, Akers RM, Pearson RE, Weber Nielsen MS. Effect of increasing energy and protein intake in heifer calves on mammary development. J. Dairy Sci. 2005a;88:595–603. [PubMed: 15653526]
- Brown EG, VandeHaar MJ, Daniels KM, Liesman JS, Chapin LT, Keisler DH, Weber Nielsen MS. Effect of increasing energy and protein intake on body growth and carcass composition of heifer calves. J. Dairy Sci. 2005b;88:585–594. [PubMed: 15653525]
- Brownlee A. The development of rumen papillae in cattle fed on different diets. Br. Vet. J. 1956;112:369–375.
- Burgstaller J, Wittek T, Smith GW. Invited review: Abomasal emptying in calves and its potential influence on gastrointestinal disease. J. Dairy Sci. 2017;100:17–35. [PubMed: 27865497]
- Bush LJ, Staley TE. Absorption of colostral immunoglobulins in newborn calves. J. Dairy Sci. 1980;63:672–680. [PubMed: 6991559]
- Butler JA, Sickles SA, Johanns CP, Rosenbusch RF. Pasteurization of discard mycoplasma milk used to feed calves: Thermal effects on various mycoplasma. J. Dairy Sci. 2000;83:2285–2288. [PubMed: 11049070]
- Byrne CJ, Fair S, English AM, Johnston D, Lonergan P, Kenny DA. Effect of milk replacer and concentrate on growth rate, feeding behaviour and systemic metabolite concentrations of preweaned bull calves of two dairy breeds. Animal. 2017;11:1531–1538. [PubMed: 28215216]
- Cabral RG, Chapman CE, Erickson PS. Colostrum supplements and replacers for dairy calves. Prof. Anim. Sci. 2013;29:449–456.
- Cabral RG, Chapman CE, Kent EJ, Erickson PS. Estimating plasma volume in neonatal Holstein calves fed one or two feedings of a lacteal-based colostrum replacer using Evans blue dye and hematocrit values at various time points. Can. J. Anim. Sci. 2015;95:293–298.
- G CabralR, Chapman CE, Aragona KM, Clark E, Lunak M, Erickson PW. Predicting colostrum quality from performance in the previous lactation and environmental changes. J. Dairy Sci. 2016;99:4048–4055. [PubMed: 26971147]
- Calder PC, Jackson AA. Undernutrition, infection and immune function. Nutr. Res. Rev. 2000;13(1):3–29. [PubMed: 19087431]
- Callan RJ, Garry FB. Biosecurity and bovine respiratory disease. Vet. Clin. North Am. Food Anim. Pract. 2002;18:57–77. [PMC free article: PMC7126375] [PubMed: 12064169]
- Campbell JM, Russell LE, Crenshaw JD, Weaver EM, Godden S, Quigley JD, Coverdale J, Tyler H. Impact of irradiation and immunoglobulin G concentration on absorption of protein and immunoglobulin G in calves fed colostrum replacer. J. Dairy Sci. 2007;90:5726–5731. [PubMed: 18024765]
- Carroll SM, DePeters EJ, Taylor SJ, Rosenberg M, Perez-Monti H, Capps VA. Milk composition of Holstein, Jersey, and Brown Swiss cows in response to increasing levels of dietary fat. Anim. Feed Sci. Technol. 2006;131(3):451–473.
- Carstens GE, Johnson DE, Holland MD, Odde KG. Effects of prepartum protein nutrition and birth weight on basal metabolism in bovine neonates. J. Anim. Sci. 1987;65:745–751. [PubMed: 3667438]
- Castells L, Bach A, Araujo G, Montoro C, Terré M. Effect of different forage sources on performance and feeding behavior of Holstein calves. J. Dairy Sci. 2012;95:286–293. [PubMed: 22192208]
- Castells L, Bach A, Aris A, Terré M. Effects of forage provision to young calves on rumen fermentation and development of the gastrointestinal tract. J. Dairy Sci. 2013;96:5226–5236. [PubMed: 23706491]
- Castells L, Bach A, Terré M. Short- and long-term effects of forage supplementation of calves during the preweaning period on performance, reproduction, and milk yield at first lactation. J. Dairy Sci. 2015;98:4748–4753. [PubMed: 25958292]
- Castro JJ, Gomez A, White BA, Mangian HJ, Loften JR, Drackley JK. Changes in the intestinal bacterial community, short-chain fatty acid profile, and intestinal development of pre-weaned Holstein calves: 1. Effects of prebiotic supplementation depend on site and age. J. Dairy Sci. 2016a;99:9682–9702. [PubMed: 27720150]
- Castro JJ, Gomez A, White B, Loften JR, Drackley JK. Changes in the intestinal bacterial community, short-chain fatty acid profile, and intestinal development of pre-weaned Holstein calves: 2. Effects of gastrointestinal site and age. J. Dairy Sci. 2016b;99:9703–9715. [PubMed: 27720148]
- Castro JJ, Hwang GH, Saito A, Vermeire DA, Drackley JK. Assessment of the effect of methionine supplementation and inclusion of hydrolyzed wheat protein in milk protein-based milk replacers on the performance of intensively fed Holstein calves. J. Dairy Sci. 2016c;99:6324–6333. [PubMed: 27179863]
- Castro MMD, Silva AL, Costa e Silva LF, Rotta PP, Engle TE, Marcondes MI. Determination of macromineral requirements for preweaned dairy calves in tropical conditions. J. Dairy Sci. 2019;102(4):2973–2984. [PubMed: 30738689]
- Cerbulis J, Farrell HM Jr. Composition of the milks of dairy cattle: II. Ash, calcium, magnesium, and phosphorus. J. Dairy Sci. 1976;59(4):589–593. [PubMed: 1262573]
- Chagas JCC, Ferreira MA, Faciola AP, Machado FS, Campos MM, Entjes MR, Donzele JL, Marcondes MI. Effects of methionine plus cysteine inclusion on performance and body composition of liquid-fed crossbred calves fed a commercial milk replacer and no starter feed. J. Dairy Sci. 2018;101:6055–6065. [PubMed: 29605314]
- Chamorro MF, Cernicchiaro N, Haines DM. Evaluation of the effects of colostrum replacer supplementation of the milk replacer ration on the occurrence of disease, antibiotic therapy, and performance of pre-weaned dairy calves. J. Dairy Sci. 2017;100:1378–1387. [PubMed: 27939546]
- Chapman CE, Erickson PS, Quigley JD, Hill TM, G. Bateman IIH, Suarez-Mena FX, Schlotterbeck RL. Effect of milk replacer program on calf performance and digestion of nutrients with age of the dairy calf. J. Dairy Sci. 2016;99:2740–2747. [PubMed: 26805983]
- Chapman CE, Hill TM, Elder DR, Erickson PS. Nitrogen utilization, preweaning nutrient digestibility, and growth effects of Holstein dairy calves fed 2 amounts of a moderately high protein or conventional milk replacer. J. Dairy Sci. 2017;100:279–292. [PMC free article: PMC7094470] [PubMed: 27865505]
- Chavatte-Palmer P, Velazquez MA, Jammes H, Duranthon V. Epigenetics, developmental programming and nutrition in herbivores. Animal. 2018;12(Suppl. 2):s363–s371. [PubMed: 30139395]
- Chelack BJ, Morley PS, Haines DM. Evaluation of methods for dehydration of bovine colostrum for total replacement of normal colostrum in calves. Can. Vet. J. 1993;34:407–412. [PMC free article: PMC1686489] [PubMed: 17424250]
- Chester-Jones H, Ziegler DM, Meiske JC. Feeding whole or rolled corn with pelleted supplement to Holstein steers from weaning to 190 kilograms. J. Dairy Sci. 1991;74:1765–1771.
- Chigerwe M, Tyler JW, Middleton JR, Spain JN, Dill JS, Steevens BJ. Comparison of four methods to assess colostral IgG concentration in dairy cows. J. Am. Vet. Med. Assoc. 2008;233:761–766. [PubMed: 18764714]
- Chua B, Coenen E, van Delen DJ, Weary DM. Effects of pair versus individual housing on the behavior and performance of dairy calves. J. Dairy Sci. 2002;85:360–364. [PubMed: 11913695]
- Conneely M, Berry DP, Murphy JP, Lorenz I, Doherty ML, Kennedy E. Effect of feeding colostrum at different volumes and subsequent number of transition milk feeds on the serum immunoglobulin G concentration and health status of dairy calves. J. Dairy Sci. 2014;97:6991–7000. [PubMed: 25200772]
- Contarini G, Povolo M, Pelizzola V, Monti L, Bruni A, Passolungo L, Abeni F, Degano L. Bovine colostrum: Changes in lipid constituents in the first 5 days after parturition. J. Dairy Sci. 2014;97:5065–5072. [PubMed: 24931528]
- Costa JHC, Meagher RK, von Keyserlingk MAG, Weary DM. Early pair housing increases solid feed intake and weight gains in dairy calves. J. Dairy Sci. 2015;98:6381–6386. [PubMed: 26188578]
- Costa JHC, von Keyserlingk MAG, Weary DM. Invited review: Effects of group housing of dairy calves on behavior, cognition, performance, and health. J. Dairy Sci. 2016;99:2453–2467. [PubMed: 26874423]
- Coverdale JA, Tyler HD, D. Quigley IIIJ, Brumm JA. Effect of various levels of forage and form of diet on rumen development and growth in calves. J. Dairy Sci. 2004;87:2554–2562. [PubMed: 15328279]
- Cowles KE, White RA, Whitehouse NL, Erickson PS. Growth characteristics of calves fed an intensified milk replacer regimen with additional lactoferrin. J. Dairy Sci. 2006;89:4835–4845. [PubMed: 17106114]
- Cruywagen CW, Jordaan I, Venter L. Effect of Lactobacillus acidophilus supplementation of milk replacer on preweaning performance of calves. J. Dairy Sci. 1996;79:483–486. [PubMed: 8708111]
- Cummins C, Berry DP, Murphy JP, Lorenz I, Kennedy E. The effect of colostrum storage conditions on dairy heifer calf serum immunoglobulin G concentration and preweaning health and growth rate. J. Dairy Sci. 2017;100:525–535. [PubMed: 27837982]
- Curnick KE, Muller LD, Rogers JA, Snyder TJ, Sweeney TF. Addition of sodium bicarbonate to calf starter rations varying in protein percent. J. Dairy Sci. 1983;66:2149–2160. [PubMed: 6315800]
- Curtis G, McGregor Argo C, Jones D, Grove-White D. The impact of early life nutrition and housing on growth and reproduction in dairy cattle. PLoS ONE. 2018;13:e0191687. [PMC free article: PMC5812595] [PubMed: 29444092]
- da Silva JT, Machado Bittar CM, Ferreira LS. Evaluation of mannan-oligosaccharides offered in milk replacers or calf starters and their effect on performance and rumen development of dairy calves. R. Bras. Zootec. 2012;41:746–752.
- da Silva JT, Manzoni T, Rocha NB, Santos G, Miqueo E, Slanzon GS, Machado Bittar CM. Evaluation of milk replacer supplemented with lysine and methionine in combination with glutamate and glutamine in dairy calves. J. Appl. Anim. Res. 2018;46:960–966.
- Dann HM, Litherland NB, Underwood JP, Bionaz M, D'Angelo A, McFadden JW, Drackley JK. Diets during far-off and close-up dry periods affect periparturient metabolism and lactation in multiparous cows. J. Dairy Sci. 2006;89:3563–3577. [PubMed: 16899692]
- Daugschies A, Bürger H-J, Akimaru M. Apparent digestibility of nutrients and nitrogen balance during experimental infection of calves with Eimeria bovis. Vet. Parasitol. 1998;77:93–102. [PubMed: 9746279]
- Davis CL, Clark JH. Ruminant digestion and metabolism. Dev. Ind. Microbiol. 1981;22:247–259.
- Davis CL, Drackley JK. The Development, Nutrition, and Management of the Young Calf. Ames: Iowa State University Press; 1998.
- Davis Rincker LE, VandeHaar MJ, Wolf CA, Liesman JS, Chapin LT, Weber Nielsen MS. Effect of intensified feeding of heifer calves on growth, pubertal age, calving age, milk yield, and economics. J. Dairy Sci. 2011;94:3554–3567. [PubMed: 21700043]
- de Passillé AM, Rushen J. Adjusting the weaning age of calves fed by automated feeders according to individual intakes of solid feed. J. Dairy Sci. 2012;95:5292–5298. [PubMed: 22916934]
- de Passillé AM, Rushen J. Using automated feeders to wean calves fed large amounts of milk according to their ability to eat solid feed. J. Dairy Sci. 2016;99:3578–3583. [PubMed: 26898277]
- de Passillé AM, Borderas TF, Rushen J. Weaning age of calves fed a high milk allowance by automated feeders: Effects on feed, water, and energy intake, behavioral signs of hunger, and weight gains. J. Dairy Sci. 2011;94:1401–1408. [PubMed: 21338805]
- de Paula Vieira A, Guesdon V, de Passillé AM, von Keyserlingk MAG, Weary DM. Behavioural indicators of hunger in dairy calves. Appl. Anim. Behav. Sci. 2008;109:180–189.
- de Paula Vieira A, von Keyserlingk MAG, Weary DM. Effects of pair versus single housing on performance and behavior of dairy calves before and after weaning from milk. J. Dairy Sci. 2010;93(7):3079–3085. [PubMed: 20630226]
- Dennis TS, Suarez-Mena FX, Hill TM, Quigley JD, Schlotterbeck RL. Effects of egg yolk inclusion, milk replacer feeding rate, and low-starch (pelleted) or high-starch (texturized) starter on Holstein calf performance through 4 months of age. J. Dairy Sci. 2017;100:8995–9006. [PubMed: 28888600]
- Dennis TS, Suarez-Mena FX, Hill TM, Quigley JD, Schlotterbeck RL, Hulbert L. Effect of milk replacer feeding rate, age at weaning, and method of reducing milk replacer to weaning on digestion, performance, rumination, and activity in dairy calves to 4 months of age. J. Dairy Sci. 2018;101:268–278. [PubMed: 29103727]
- M Desjardins-Morrissette, van Niekerk JK, Haines D, Sugino T, Oba M, Steele MA. The effect of tube versus bottle feeding colostrum on immunoglobulin G absorption, abomasal emptying, and plasma hormone concentrations in newborn calves. J. Dairy Sci. 2018;101:4168–4179. [PubMed: 29454696]
- Dewhurst RJ, Moorby JM, Dhanoa MS, Fishert WJ. Effects of level of concentrate feeding during the second gestation of Holstein-Friesian dairy cows: 1. Feed intake and milk production. J. Dairy Sci. 2002;85:169–177. [PubMed: 11860108]
- Diaz MC, Van Amburgh ME, Smith JM, Kelsey JM, Hutten EL. Composition of growth of Holstein calves fed milk replacer from birth to 105-kilogram body weight. J. Dairy Sci. 2001;84:830–842. [PubMed: 11352160]
- Dodd PR, Thomas GJ, McCloskey A, Crane DI, Smith ID. The neurochemical pathology of thiamine deficiency: GABAA and glutamateNMDA receptor binding sites in a goat model. Metab. Brain Dis. 1996;11:39–54. [PubMed: 8815389]
- Doll K, Riepl H, Dirksen G. Pathophysiologische Bedeutung von Steatorrhoe sowie von Hydroxystearinsäuren im Kot von Durchfallkälbern [Pathophysiological significance of steatorrhea as well as of hydroxystearic acids in the feces of calves with diarrhea. Dtsch. Tierarztl. Wochenschr. 2004;111:22–28. [PubMed: 14983751]
- Donnelly PE. Effects of dietary carbohydrate:fat ratio on growth and body composition of milk-fed calves. N. Z. J. Agric. Res. 1983;26:71–77.
- Donnelly PE, Hutton JB. Effects of dietary protein and energy on the growth of Friesian bull calves. I. Food intake, growth, and protein requirements. N. Z. J. Agric. Res. 1976a;19:289–297.
- Donnelly PE, Hutton JB. Effects of dietary protein and energy on the growth of Friesian bull calves. II. Effects of level of feed intake and dietary protein content on body composition. N. Z. J. Agric. Res. 1976b;19:409–414.
- Doolatabad SS, Sari M, Ghorbani GR. Effect of partial replacement of dietary starch with fiber and fat on performance, feeding behavior, ruminal fermentation and some blood metabolites of Holstein calves. Anim. Feed Sci. Technol. 2020;270:114691.
- Douglas GN, Overton TR, G. Bateman IIH, Dann HM, Drackley JK. Prepartal plane of nutrition, regardless of dietary energy source, affects periparturient metabolism and dry matter intake in Holstein cows. J. Dairy Sci. 2006;89:2141–2157. [PubMed: 16702281]
- Drackley JK. Calf nutrition from birth to breeding. Vet. Clin. North Am. Food Anim. Pract. 2008;24:55–86. [PubMed: 18299032]
- Drackley JK, Blome RM, Bartlett KS, Bailey KL. Supplementation of 1% l-glutamine to milk replacer does not overcome the growth depression in calves caused by soy protein concentrate. J. Dairy Sci. 2006;89:1688–1693. [PubMed: 16606739]
- Dréau D, Lallès JP. Contribution to the study of gut hypersensitivity reactions to soybean proteins in preruminant calves and early-weaned piglets. Livestock Prod. Sci. 1999;60:209–218.
- Dunn A, Ashfield A, Earley B, Welsh M, Gordon A, McGee M, Morrison SJ. Effect of concentrate supplementation during the dry period on colostrum quality and effect of colostrum feeding regimen on passive transfer of immunity, calf health, and performance. J. Dairy Sci. 2017;100:357–370. [PubMed: 27865490]
- Eckert E, Brown HE, Leslie KE, DeVries TJ, Steele MA. Weaning age affects growth, feed intake, gastrointestinal development, and behavior in Holstein calves fed an elevated plane of nutrition during the preweaning stage. J. Dairy Sci. 2015;98:6315–6326. [PubMed: 26142851]
- EFSA Panel on Biological Hazards. Antimicrobial resistance (AMR) due to feeding of calves with milk containing residues of antibiotics. EFSA J. 2017;15:101. [PMC free article: PMC7372110] [PubMed: 32704309]
- Eicher SD, Morrill JL, Blecha F, Chitko-McKown CG, Anderson NV, Higgins JJ. Leukocyte functions of young dairy calves fed milk replacers supplemented with vitamins A and E. J. Dairy Sci. 1994;77:1399–1407. [PubMed: 8046079]
- Eicher-Pruiett SD, Morrill JL, Blecha F, Higgins JJ, Anderson NV, Reddy PG. Neutrophil and lymphocyte response to supplementation with vitamins C and E in young calves. J. Dairy Sci. 1992;75:1635–1642. [PubMed: 1500563]
- Eley RM, Thatcher WW, Bazer FW, Wilcox CJ, Becker RB, Head HH, Adkinson RW. Development of the conceptus in the bovine. J. Dairy Sci. 1978;61:467–473. [PubMed: 659690]
- Elizondo-Salazar JA, Heinrichs AJ. Feeding heat-treated colostrum or unheated colostrum with two different bacterial concentrations to neonatal dairy calves. J. Dairy Sci. 2009;92:4565–4571. [PubMed: 19700719]
- Elizondo-Salazar JA, Jones CM, Heinrichs AJ. Evaluation of calf milk pasteurization systems on 6 Pennsylvania dairy farms. J. Dairy Sci. 2010;93:5509–5513. [PubMed: 20965367]
- English EA, Hopkins BA, Stroud JS, Davidson S, Smith G, Brownie C, Whitlow LW. Lactoferrin supplementation to Holstein calves during the preweaning and postweaning phases. J. Dairy Sci. 2007;90:5276–5281. [PubMed: 17954768]
- Esselburn KM, O'Diam KM, Hill TM, G. Bateman IIH, Aldrich JM, Schlotterbeck RL, Daniels KM. Intake of specific fatty acids and fat alters growth, health, and titers following vaccination in dairy calves. J. Dairy Sci. 2013;96:5826–5835. [PubMed: 23810586]
- Ferrell CL, Jenkins TG. Body composition and energy utilization by steers of diverse genotypes fed a high-concentrate diet during the finishing period: I. Angus, Belgian Blue, Hereford, and Piedmontese sires. J. Anim. Sci. 1998a;76:637–646. [PubMed: 9498375]
- Ferrell CL, Jenkins TG. Body composition and energy utilization by steers of diverse genotypes fed a high-concentrate diet during the finishing period: II. Angus, Boran, Brahman, Hereford, and Tuli sires. J. Anim. Sci. 1998b;76:647–657. [PubMed: 9498376]
- Flatt WG, Warner RG, Loosli JK. Influence of purified materials on the development of the ruminant stomach. J. Dairy Sci. 1958;41:1593–1600.
- Fokkink WB, Hill TM, Aldrich JM, Bateman II HG, Schlotterbeck RL. Case Study: Effect of yeast culture, fatty acids, whey, and a peptide source on dairy calf performance. Prof. Anim. Sci. 2009;25:794–800.
- Foley JA, Otterby DE. Availability, storage, treatment, composition, and feeding value of surplus colostrum: A review. J. Dairy Sci. 1978;61:1033–1060.
- Fomenky BE, Chiquette J, Bissonnette N, Talbot G, Chouinard PY, Ibeagha-Awemu EM. Impact of Saccharomyces cerevisiae boulardii CNCMI-1079 and Lactobacillus acidophilus BT1386 on total lactobacilli population in the gastrointestinal tract and colon histomorphology of Holstein dairy calves. Anim. Feed Sci. Technol. 2017;234:151–161.
- Foote MR, Nonnecke BJ, Fowler MA, Miller BL, Beitz DC, Waters WR. Effects of age and nutrition on expression of CD25, CD44, and L-selectin (CD62L) on T-cells from neonatal calves. J. Dairy Sci. 2005a;88:2718–2729. [PubMed: 16027185]
- Foote MR, Nonnecke BJ, Waters WR, Palmer MV, Beitz DC, Fowler MA, Miller BL, Johnson TE, Perry HB. Effects of intensified nutrition on antigen-specific, cell-mediated immune responses of milk replacer-fed calves. Int. J. Vitam. Nutr. Res. 2005b;75:357–368. [PubMed: 16477768]
- Foote MR, Nonnecke BJ, Beitz DC, Waters WR. High growth rate fails to enhance adaptive immune responses of neonatal calves and is associated with reduced lymphocyte viability. J. Dairy Sci. 2007;90:404–417. [PubMed: 17183108]
- Foroozandeh AD, Shakeri P. Effects of alfalfa hay and sodium bicarbonate in the starter diet on performance of Holstein dairy calves. Anim. Sci. J. 2017;30:247–256.
- França TGD, Ishikawa LLW, Zorzella-Pezavento SFG, Chiuso-Minicucci F, da Cunha MLRS, Sartori A. Impact of malnutrition on immunity and infection. J. Venom. Anim. Toxins Incl. Trop. Dis. 2009;15:374–390.
- Franklin ST, Sorenson CE, Hammell DC. Influence of vitamin A supplementation in milk on growth, health, concentrations of vitamins in plasma, and immune parameters of calves. J. Dairy Sci. 1998;81(10):2623–2632. [PubMed: 9812268]
- Franklin ST, Amaral-Philips DM, Jackson JA, Campbell AA. Health and performance of Holstein calves that suckled or were hand-fed colostrum and were fed one of three physical forms of starter. J. Dairy Sci. 2003;86:2145–2153. [PMC free article: PMC7095194] [PubMed: 12836951]
- Franzoni APS. Master's thesis, Universidade Federal De Minas Gerais, Escola De Veterinária. 2012. Effects of corn processing on rumen development, performance, and digestibility in vitro of corn grain.
- Frieten D, Gerbert C, Koch C, Dusel G, Eder K, Kanitz E, Weitzel JM, Hammon HM. Ad libitum milk replacer feeding, but not butyrate supplementation, affects growth performance as well as metabolic and endocrine traits in Holstein calves. J. Dairy Sci. 2017;100:6648–6661. [PubMed: 28601458]
- Froehlich KA, Abdelsalam KW, Chase C, Koppien-Fox J, Casper DP. Evaluation of essential oils and prebiotics for newborn dairy calves. J. Anim. Sci. 2017;95:3772–3782. [PubMed: 28805907]
- Galvão KN, Santos JEP, Coscioni A, Villaseñor M, Sisco WM, Berge ACB. Effect of feeding live yeast products to calves with failure of passive transfer on performance and patterns of antibiotic aresistance in fecal Escherichia coli. Reprod. Nutr. Dev. 2005;45:427–440. [PubMed: 16045891]
- Galyean ML, Cole NA, Tedeschi LO, Branine ME. Board-invited review: Efficiency of converting digestible energy to metabolizable energy and reevaluation of the California Net Energy System maintenance requirements and equations for predicting dietary net energy values for beef cattle. J. Anim. Sci. 2016;94:1329–1341. [PubMed: 27135993]
- Garcia M, Greco LF, Favoreto MG, Marsola RS, Martins LT, Bisinotto RS, Wang D, Shin JH, Lock AL, Block E, Thatcher WW, Santos JEP, Staples CR. Effect of supplementing fat to pregnant nonlactating cows on colostral fatty acid profile and passive immunity of the newborn calf. J. Dairy Sci. 2014a;97:392–405. [PubMed: 24239079]
- Garcia M, Greco LF, Favoreto MG, Marsola RS, Wang D, Shin JH, Block E, Thatcher WW, Santos JEP, Staples CR. Effect of supplementing essential fatty acids to pregnant nonlactating Holstein cows and their preweaned calves on calf performance, immune response, and health. J. Dairy Sci. 2014b;97:5045–5064. [PubMed: 24931531]
- Gardner RW. Acceptability and nutritional response comparisons between calf starters. J. Dairy Sci. 1967;50:729–734.
- Garrett WN. Energy utilization by growing cattle as determined in 72 comparative slaughter experiments. In: Mount LE, editor. Energy Metabolism. London, UK: Butterworths; 1980. pp. 3–7. (EAAP Publ. No 26).
- Garthwaite BD, Drackley JK, McCoy GC, Jaster EH. Whole milk and oral rehydration solution for calves with diarrhea of spontaneous origin. J. Dairy Sci. 1994;77:835–843. [PubMed: 8169291]
- Gavin K, Neibergs H, Hoffman A, Kiser JN, Cornmesser MA, Haredasht SA, Martínez-López B, Wenz JR, Moore DA. Low colostrum yield in Jersey cattle and potential risk factors. J. Dairy Sci. 2018;101:6388–6398. [PubMed: 29605315]
- Geiger AJ, Ward SH, Williams CC, Rude BJ, Cabrera CJ, Kalestch KN, Voelz BE. Short communication: Effects of increasing protein and energy in the milk replacer with or without direct-fed microbial supplementation on growth and performance of preweaned Holstein calves. J. Dairy Sci. 2014;97:7212–7219. [PubMed: 25200791]
- Geiger AJ, Parsons CLM, James RE, Akers RM. Growth, intake, and health of Holstein heifer calves fed an enhanced preweaning diet with or without postweaning exogenous estrogen. J. Dairy Sci. 2016;99:3995–4004. [PubMed: 26898286]
- Gelsinger SL, Heinrichs AJ, Jones CM. A meta-analysis of the effects of preweaned calf nutrition and growth on first-lactation performance. J. Dairy Sci. 2016;99:6206–6214. [PubMed: 27209128]
- Gerrits WJJ, Tolman GH, Schrama JW, Tamminga S, Bosch MW, Verstegen MWA. Effect of protein and protein-free energy intake on protein and fat deposition rates in preruminant calves of 80 to 240 kg live weight. J. Anim. Sci. 1996;74:2129–2139. [PubMed: 8880415]
- Ghanem MM, Radwaan ME, Moustafa AM, Ebeid MH. Comparative therapeutic effect of toltrazuril, sulphadimidine and amprolium on Eimeria bovis and Eimeria zuernii given at different times following infection in buffalo calves (Bubalus bubalis). Prev. Vet. Med. 2008;84:161–170. [PubMed: 18262668]
- Ghasemi E, Azad-Shahraki M, Khorvash M. Effect of different fat supplements on performance of dairy calves during cold season. J. Dairy Sci. 2017;100:5319–5328. [PubMed: 28501409]
- Ghassemi Nejad J, Torbatinejad N, Naserian AA, Kumar S, Kim JD, Song YH, Ra CS, Sung KI. Effects of processing of starter diets on performance, nutrient digestibility, rumen biochemical parameters and body measurements of Brown Swiss dairy calves. Asian Aust. J. Anim. Sci. 2012;25:980–987. [PMC free article: PMC4092968] [PubMed: 25049653]
- Ghorbani H, Kazemi-Bonchenari M, HosseinYazdi M, Mahjoubi E. Effects of various fat delivery methods in starter diet on growth performance, nutrients digestibility and blood metabolites of Holstein dairy calves. Anim. Feed Sci. Technol. 2020;262:114429.
- Gilbert MS, Pantophlet AJ, Berends H, Pluschke AM, van den Borne JJGC, Hendriks WH, Schols HA, Gerrits WJJ. Fermentation in the small intestine contributes substantially to intestinal starch disappearance in calves. J. Nutr. 2015;145:1147–1155. [PubMed: 25878206]
- Gilbert MS, Pantophlet AJ, van den Borne JJGC, Hendriks WH, Schols HA, Gerrits WJJ. Effects of replacing lactose from milk replacer by glucose, fructose, or glycerol on energy partitioning in veal calves. J. Dairy Sci. 2016;99:1121–1132. [PubMed: 26627854]
- Glosson KM, Hopkins BA, Washburn SP, Davidson S, Smith G, Earleywine T, Ma C. Effect of supplementing pasteurized milk balancer products to heat-treated whole milk on the growth and health of dairy calves. J. Dairy Sci. 2015;98:1127–1135. [PubMed: 25483198]
- Godden S. Colostrum management for dairy calves. Vet. Clin. North. Am. Food Anim. Pract. 2008;24:19–39. [PMC free article: PMC7127126] [PubMed: 18299030]
- Godden SM, Fetrow JP, Feirtag JM, Green LR, Wells SJ. Economic analysis of feeding pasteurized nonsaleable milk versus conventional milk replacer to dairy calves. J. Am. Vet. Med. Assoc. 2005;226:1547–1554. [PubMed: 15882008]
- Godden S, McMartin S, Feirtag J, Stabel J, Bey R, Goyal S, Metzger L, Fetrow J, Wells S, Chester-Jones H. Heat-treatment of bovine colostrum. II: Effects of heating duration on pathogen viability and immunoglobulin g. J. Dairy Sci. 2006;89:3476–3483. [PubMed: 16899682]
- Godden SM, Smolenski DJ, Donahue M, Oakes JM, Bey R, Wells S, Sreevatsan S, Stabel J, Fetrow J. Heat-treated colostrum and reduced morbidity in preweaned dairy calves: Results of a randomized trial and examination of mechanisms of effectiveness. J. Dairy Sci. 2012;95:4029–4040. [PubMed: 22720957]
- Godden SM, Lombard JE, Woolums AR. Colostrum management for dairy calves. Vet. Clin. Food Anim. 2019;35:535–556. [PMC free article: PMC7125574] [PubMed: 31590901]
- Gonzalez-Jimenez E, Blaxter KL. The metabolism and thermal regulation of calves in the first month of life. Br. J. Nutr. 1962;16:199–212. [PubMed: 13900231]
- Górka P, Kowalski ZM, Pietrzak P, Kotunia A, Jagusiak W, Holst JJ, Guilloteau R, Zabielski R. Effect of method of delivery of sodium butyrate on rumen development in newborn calves. J. Dairy Sci. 2011a;94:5578–5588. [PubMed: 22032381]
- Górka P, Kowalski ZM, Pietrzak P, Kotunia A, Jagusiak W, Zabielski R. Is rumen development in newborn calves affected by different liquid feeds and small intestine development? J. Dairy Sci. 2011b;94:3002–3013. [PubMed: 21605770]
- Górka P, Pietrzak P, Kotunia A, Zabielski R, Kowalski ZM. Effect of method of delivery of sodium butyrate on maturation of the small intestine. J. Dairy Sci. 2014;97:1026–1035. [PubMed: 24342681]
- Górka P, Kowalski ZM, Zabielski R, Guilloteau P. Invited review: Use of butyrate to promote gastrointestinal tract development in calves. J. Dairy Sci. 2018;101:4785–4800. [PubMed: 29525310]
- Grand E, Respondek F, Martineau C, Detilleux J, Bertrand G. Effects of short-chain fructooligosaccharides on growth performance of preruminant veal calves. J. Dairy Sci. 2013;96:1094–1101. [PubMed: 23200477]
- Greenwood RH, Morrill JL, Titgemeyer EC, Kennedy GA. A new method of measuring diet abrasion and its effect on the development of the forestomach. J. Dairy Sci. 1997;80:2534–2541. [PubMed: 9361226]
- Griebel PJ, Schoonderwoerd M, Babiuk LA. Ontogeny of the immune response: Effect of protein energy malnutrition in neonatal calves. Can. J. Vet. Res. 1987;51:428–435. [PMC free article: PMC1255359] [PubMed: 3134116]
- Grum DE, Drackley JK, Younker RS, LaCount DW, Veenhuizen JJ. Nutrition during the dry period and hepatic lipid metabolism of periparturient dairy cows. J. Dairy Sci. 1996;79:1850–1864. [PubMed: 8923256]
- Guerra-Hernández E, Leon C, Corzo N, García-Villanova B, Romera JM. Chemical changes in powdered infant formulas during storage. Int. J. Dairy Technol. 2002;55:171–176.
- Guilloteau P, Zabielski R, David JC, Blum JW, Morisset JA, Biernat M, Woliński J, Laubitz D, Hamon Y. Sodium-butyrate as a growth promoter in milk replacer formula for young calves. J. Dairy Sci. 2009;92:1038–1049. [PubMed: 19233797]
- Guilloteau P, Savary G, Jaguelin-Peyrault Y, Rome V, Le Normand L, Zabielski R. Dietary sodium butyrate supple-mentation increases digestibility and pancreatic secretion in young milk-fed calves. J. Dairy Sci. 2010;93:5842–5850. [PubMed: 21094757]
- Guindon NE, Antaya NT, Cabral RG, Whitehouse NL, Earleywine TJ, Erickson PS. Effects of human visitation on calf growth and performance of calves fed different milk replacer feeding levels. J. Dairy Sci. 2015;98:8952–8961. [PMC free article: PMC7094226] [PubMed: 26476943]
- Habing G, Harris K, Schuenemann GM, Piñeiro JM, Lakritz J, Alcaraz Clavijo X. Lactoferrin reduces mortality in preweaned calves with diarrhea. J. Dairy Sci. 2017;100:3940–3948. [PubMed: 28318585]
- Hall JA, Bobe G, Vorachek WR, Estill CT, Mosher WD, Pirelli GJ, Gamroth M. Effect of supranutritional maternal and colostral selenium supplementation on passive absorption of immunoglobulin G in selenium-replete dairy calves. J. Dairy Sci. 2014;97:4379–4391. [PubMed: 24767888]
- Hammon H, Blum JW. Prolonged colostrum feeding enhances xylose absorption in neonatal calves. J. Anim. Sci. 1997;75:2915–2919. [PubMed: 9374304]
- Harris TL, Liang Y, Sharon KP, Sellers MD, Yoon I, Scott MF, Carroll JA, Ballou MA. Influence of Saccharomyces cerevisiae fermentation products, SmartCare in milk replacer and Original XPC in calf starter, on the performance and health of preweaned Holstein calves challenged with Salmonella enterica serotype Typhimurium. J. Dairy Sci. 2017;100:7154–7164. [PubMed: 28734601]
- Heinrichs AJ, Bush GJ. Evaluation of decoquinate or lasalocid against coccidiosis from natural exposure in neonatal dairy calves. J. Dairy Sci. 1991;74:3223–3227. [PubMed: 1779071]
- Heinrichs AJ, Swartz LA, Drake TR, Travis PA. Influence of decoquinate fed to neonatal dairy calves on early and conventional weaning systems. J. Dairy Sci. 1990;73:1851–1856. [PubMed: 2229594]
- Heinrichs AJ, Jones CM, Heinrichs BS. Effects of mannan oligosaccharide or antibiotics in neonatal diets on health and growth of dairy calves. J. Dairy Sci. 2003;86:4064–4069. [PubMed: 14740845]
- Heinrichs AJ, Jones CM, Elizondo-Salazar JA, Terrill SJ. Effects of a prebiotic supplement on health of neonatal dairy calves. Livestock Sci. 2009;125:149–154.
- Heinrichs AJ, Heinrichs BS, Jones CM. Fecal and saliva IgA secretion when feeding a concentrated mannan oligosaccharide to neonatal dairy calves. Prof. Anim. Sci. 2013;29:457–462.
- Hepola HP, Hänninen LT, Raussi SM, Pursiainen PA, Aarnikoivu A-M, Saloniemi HS. Effects of providing water from a bucket or a nipple on the performance and behavior of calves fed ad libitum volumes of acidified milk replacer. J. Dairy Sci. 2008;91:1486–1496. [PubMed: 18349242]
- Hibbs JW, Conrad HR, Pounden WD, Frank N. A high roughage system for raising calves based on early development of rumen function: VI. Influence of hay to grain ratio on calf performance, rumen development, and certain blood changes. J. Dairy Sci. 1956;39:171–179.
- Hidiroglou M, Knipfel JE. Maternal-fetal relationships of copper, manganese, and sulfur in ruminants: A review. J. Dairy Sci. 1981;64:1637–1647. [PubMed: 7028809]
- Hill SR, Knowlton KF, Daniels KM, James RE, Pearson RE, Capuco AV, Akers RM. Effect of milk replacer composition on growth, body composition, and nutrient excretion in preweaned Holstein heifers. J. Dairy Sci. 2008a;91:3145–3155. [PubMed: 18650291]
- Hill TM, Aldrich JM, Schlotterbeck RL, G. Bateman IIH. Effects of feeding calves different rates and protein concentrations of twenty percent fat milk replacers on growth during the neonatal period. Prof. Anim. Sci. 2006a;22:252–260.
- Hill TM, Aldrich JM, Schlotterbeck RL, G. Bateman IIH. Effects of feeding rate and concentrations of protein and fat of milk replacers fed to neonatal calves. Prof. Anim. Sci. 2006b;22:374–381.
- Hill TM, Aldrich JM, Schlotterbeck RL, G. Bateman IIH. Effects of changing the fat and fatty acid composition of milk replacers fed to neonatal calves. Prof. Anim. Sci. 2007a;23:135–143.
- Hill TM, Aldrich JM, Schlotterbeck RL, G. Bateman IIH. Amino acids, fatty acids, and fat sources for calf milk replacers. Prof. Anim. Sci. 2007b;23:401–408.
- Hill TM, Aldrich JM, Schlotterbeck RL, Bateman HG II. Effects of changing the fatty acid composition of calf starters. Prof. Anim. Sci. 2007c;23:665–671.
- Hill TM, G. Bateman IIH, Aldrich JM, Schlotterbeck RL. Effects of the feeding rate of high protein calf milk replacers. Prof. Anim. Sci. 2007d;23:649–655.
- Hill TM, G. Bateman IIH, Aldrich JM, Schlotterbeck RL. Effects of feeding rate of milk replacers and bedding material for calves in a cold, naturally ventilated nursery. Prof. Anim. Sci. 2007e;23:656–664.
- Hill TM, G. Bateman IIH, Aldrich JM, Schlotterbeck RL. Effect of consistency of nutrient intake from milk and milk replacer on dairy calf performance. Prof. Anim. Sci. 2008b;24:85–92.
- Hill TM, G. Bateman IIH, Aldrich JM, Schlotterbeck RL. Oligosaccharides for dairy calves. Prof. Anim. Sci. 2008c;24:460–464.
- Hill TM, G. Bateman IIH, Aldrich JM, Schlotterbeck RL. Effects of using wheat gluten and rice protein concentrate in dairy calf milk replacers. Prof. Anim. Sci. 2008d;24:465–472.
- Hill TM, G. Bateman IIH, Aldrich JM, Schlotterbeck RL, Tanan KG. Optimal concentrations of lysine, methionine, and threonine in milk replacers for calves less than five weeks of age. J. Dairy Sci. 2008e;91:2433–2442. [PubMed: 18487666]
- Hill TM, G. Bateman IIH, Aldrich JM, Schlotterbeck RL. Effects of changing the essential and functional fatty acid intake of dairy calves. J. Dairy Sci. 2009a;92:670–676. [PubMed: 19164679]
- Hill TM, G. Bateman IIH, Aldrich JM, Schlotterbeck RL. Optimizing nutrient ratios in milk replacers for calves less than five weeks of age. J. Dairy Sci. 2009b;92:3281–3291. [PubMed: 19528605]
- Hill TM, G. Bateman IIH, Aldrich JM, Schlotterbeck RL. Effects of fat concentration of a high-protein milk replacer on calf performance. J. Dairy Sci. 2009c;92:5147–5153. [PubMed: 19762833]
- Hill TM, G. Bateman IIH, Aldrich JM, Schlotterbeck RL. Effect of milk replacer program on digestion of nutrients in dairy calves. J. Dairy Sci. 2010;93:1105–1115. [PubMed: 20172232]
- Hill TM, G. Bateman IIH, Aldrich JM, Schlotterbeck RL. Effect of various fatty acids on dairy calf performance. Prof. Anim. Sci. 2011a;27:167–175.
- Hill TM, G. Bateman IIH, Aldrich JM, Schlotterbeck RL. Case study: Effects of adding arginine and histidine to dairy calf milk replacers. Prof. Anim. Sci. 2011b;27:565–570.
- Hill TM, VandeHaar MJ, Sordillo LM, Catherman DR, G. Bateman IIH, Schlotterbeck RL. Fatty acid intake alters growth and immunity in milk-fed calves. J. Dairy Sci. 2011c;94:3936–3948. [PubMed: 21787930]
- Hill TM, G. Bateman IIH, Aldrich JM, Schlotterbeck RL. High-starch, coarse-grain, low-fiber diets maximize growth of weaned dairy calves less than 4 months of age. Prof. Anim. Sci. 2012;28:325–331.
- Hill TM, G. Bateman IIH, Aldrich JM, Quigley JD, Schlotterbeck RL. Evaluation of ad libitum acidified milk replacer programs for dairy calves. J. Dairy Sci. 2013;96:3153–3162. [PubMed: 23466327]
- Hill TM, G. Bateman IIH, Aldrich JM, Quigley JD, Schlotterbeck RL. Inclusion of tallow and soybean oil to calf starters fed to dairy calves from birth to four months of age on calf performance and digestion. J. Dairy Sci. 2015;98:4882–4888. [PubMed: 25912868]
- Hill TM, Quigley JD, G. Bateman IIH, Aldrich JM, Schlotterbeck RL. Source of carbohydrate and metabolizable lysine and methionine in the diet of recently weaned dairy calves on digestion and growth. J. Dairy Sci. 2016a;99(4):2788–2796. [PubMed: 26851852]
- Hill TM, Quigley JD, G. Bateman IIH, Suarez-Mena FX, Dennis TS, Schlotterbeck RL. Effect of milk replacer program on calf performance and digestion of nutrients in dairy calves to 4 months of age. J. Dairy Sci. 2016b;99(10):8103–8110. [PubMed: 27497902]
- Hill TM, Quigley JD, Suarez-Mena FX, G. Bateman IIH, Schlotterbeck RL. Effect of milk replacer feeding rate and functional fatty acids on dairy calf performance and digestion of nutrients. J. Dairy Sci. 2016c;99:6352–6361. [PubMed: 27289150]
- Hoblet KH, Charles TP, Howard RP. Evaluation of lasalocid and decoquinate against coccidiosis resulting from natural exposure in weaned dairy calves. Am. J. Vet. Res. 1989;50:1060–1064. [PubMed: 2774324]
- Hodgson J. The effect of weaning treatment on the development of solid food intake in calves. Anim. Prod. 1965;7:7–17.
- Hodgson J. The development of solid food intake in calves: 5. The relationship between liquid and solid food intake. Anim. Prod. 1971;13:593–597.
- Houser BA, Donaldson SC, Kehoe SI, Heinrichs AJ, Jayarao BM. A survey of bacteriological quality and the occurrence of salmonella in raw bovine colostrum. Foodborne Pathogens Dis. 2008;5:853–858. [PubMed: 18991543]
- Hu W, Hill TM, Dennis TS, Suarez-Mena FX, Quigley JD, Schlotterbeck RL. Intake, nutrient digestibility, and growth performance of Holstein dairy calves consuming a milk replacer at moderate or high feeding rates. J. Dairy Sci. 2019;102:7917–7926. [PubMed: 31255275]
- Huber JT, Silva AG, Campos OF, Mathieu CM. Influence of feeding different amounts of milk on performance, health, and absorption capability of baby calves. J. Dairy Sci. 1984;67:2957–2963. [PubMed: 6530491]
- Huisman J. Antinutritional factors (ANFs) in the nutrition of monogastric farm animals. In: van Weerden EJ, Huisman J, editors. Nutrition and Digestive Physiology in Monogastric Farm Animals. Wageningen, The Netherlands: Pudoc; 1989. pp. 17–35.
- Hulbert LE, Cobb CJ, Carroll JA, Ballou MA. The effects of early weaning on innate immune responses of Holstein calves. J. Dairy Sci. 2011;94:2545–2556. [PubMed: 21524546]
- Huuskonen A. Effects of skim milk and whey-based milk replacers on feed intake and growth of dairy calves. J. Appl. Anim. Res. 2017;45:480–484.
- Huuskonen A, Pesonen M. Does yeast (Saccharomyces cerevisiae) supplementation in calf starter modify feed intake and liveweight gain of dairy bull calves? J. Anim. Feed Sci. 2015;24:295–301.
- Huuskonen A, Khalili H, Kiljala J, Joki-Tokola E, Nousiainen J. Effects of vegetable fats versus lard in milk replacers on feed intake, digestibility, and growth in Finnish Ayrshire bull calves. J. Dairy Sci. 2005;88:3575–3581. [PubMed: 16162531]
- Huuskonen A, Tuomisto L, Kauppinen R. Effect of drinking water temperature on water intake and performance of dairy calves. J. Dairy Sci. 2011;94(5):2475–2480. [PubMed: 21524539]
- Ikemori Y, Ohta M, Umeda K, Icatlo FC Jr, Kuroki M, Yokoyama H, Kodama Y. Passive protection of neonatal calves against bovine coronavirus-induced diarrhea by administration of egg yolk or colostrum antibody powder. Vet. Microbiol. 1997;58:105–111. [PMC free article: PMC7117124] [PubMed: 9453122]
- Imani M, Mirzaei M, Baghbanzadeh-Nobari B, Ghaffari MH. Effects of forage provision to dairy calves on growth performance and rumen fermentation: A meta-analysis and meta-regression. J. Dairy Sci. 2017;100:1136–1150. [PubMed: 27939538]
- INRA. INRA Feeding System for Ruminants. 2nd ed. Wageningen, The Netherlands: Wageningen Academic Publishers; 2019.
- Jamaluddin AA, Carpenter TE, Hird DW, Thurmond MC. Economics of feeding pasteurized colostrum and pasteurized waste milk to dairy calves. J. Am. Vet. Med. Assoc. 1996;209:751–756. [PubMed: 8756874]
- Janovick NA, Drackley JK. Prepartum dietary management of energy intake affects postpartum intake and lactation performance by primiparous and multiparous Holstein cows. J. Dairy Sci. 2010;93:3086–3102. [PubMed: 20630227]
- Jasper J, Weary DM. Effects of ad libitum milk intake on dairy calves. J. Dairy Sci. 2002;85:3054–3058. [PubMed: 12487471]
- Jaster EH, McCoy GC, Fernando RL. Dietary fat in milk or milk replacers for young calves raised in hutches during winter. J. Dairy Sci. 1990;73:1843–1850.
- Jaster EH, McCoy GC, Spanski N, Tomkins T. Effect of extra energy as fat or milk replacer solids in diets of young dairy calves on growth during cold weather. J. Dairy Sci. 1992;75:2524–2531. [PubMed: 1452856]
- Jenkins KJ, Emmons DB. Fortification of calf milk replacers with amino acids in free form or plastein-bound. Can. J. Anim. Sci. 1983;63:893–903.
- Jenkins KJ, Kramer JKG, Sauer FD, Emmons DB. Influence of triglycerides and free fatty acids in milk replacers on calf performance, blood plasma, and adipose lipids. J. Dairy Sci. 1985;68:669–680. [PubMed: 3989087]
- Jenny BF, Mills SE, Johnston WE, O'Dell GD. Effect of fluid intake and dry matter concentration on scours and water intake in calves fed once daily. J. Dairy Sci. 1978;61:765–770.
- Jenny BF, Van Dijk HJ, Grimes LW. Performance of calves fed milk replacer once daily at various fluid intakes and dry matter concentrations. J. Dairy Sci. 1982;65:2345–2350. [PubMed: 7161432]
- Jensen MB. Computer-controlled milk feeding of group-housed calves: The effect of milk allowance and weaning type. J. Dairy Sci. 2006;89:201–206. [PubMed: 16357283]
- Jensen RG, Ferris AM, Lammi-Keefe CJ. The composition of milk fat. J. Dairy Sci. 1991;74:3228–3243. [PubMed: 1779072]
- Johnson, D Jr, Dolge KL, Rousseau JE Jr, Teichman R, Eaton HD, Beall G, Moore LA. Effect of the addition of inedible tallow to a calf starter fed to Holstein calves. J. Dairy Sci. 1956;39:1268–1279.
- Johnson JL, Godden SM, Molitor T, Ames T, Hagman D. Effects of feeding heat-treated colostrum on passive transfer of immune and nutritional parameters in neonatal dairy calves. J. Dairy Sci. 2007;90:5189–5198. [PubMed: 17954759]
- Johnson PTC, Elliott RC. Dietary energy intake and utilization by young Friesland calves: 2. Digestibility and metabolizable energy contents of whole milk and spray-dried skimmilk powder, and energy retentions of calves given these foods. Rhod. J. Agric. Res. 1972a;10:125–133.
- Johnson PTC, Elliott RC. Dietary energy intake and utilization by young Friesland calves: 3. The utilization by calves of energy in whole milk. Rhod. J. Agric. Res. 1972b;10:135–142.
- Jorgensen MA, Hoffman PC, Nytes AJ. Case study: A field survey of on-farm milk pasteurization efficacy. Prof. Anim. Sci. 2006;22:472–476.
- Jorgensen MW, Adams-Progar A, de Passillé AM, Rushen J, Godden SM, Chester-Jones H, Endres MI. Factors associated with dairy calf health in automated feeding systems in the Upper Midwest United States. J. Dairy Sci. 2017a;100:5675–5686. [PubMed: 28456403]
- Jorgensen MW, Adams-Progar A, de Passillé AM, Rushen J, Salfer JA, Endres MI. Mortality and health treatment rates of dairy calves in automated milk feeding systems in the Upper Midwest of the United States. J. Dairy Sci. 2017b;100:9186–9193. [PubMed: 28918142]
- Jorgensen MW, Janni K, Adams-Progar A, Chester-Jones H, Salfer JA, Endres MI. Housing and management characteristics of calf automated feeding systems in the Upper Midwest of the United States. J. Dairy Sci. 2017c;100:9881–9891. [PubMed: 28987578]
- Joslin RS, Erickson PS, Santoro HM, Whitehouse NL, Schwab CG, Rejman JJ. Lactoferrin supplementation to dairy calves. J. Dairy Sci. 2002;85:1237–1242. [PubMed: 12086060]
- Kamada H, Nonaka I, Ueda Y, Murai M. Selenium addition to colostrum increases immunoglobulin G absorption by newborn calves. J. Dairy Sci. 2007;90:5665–5670. [PubMed: 18024758]
- Kara C, Cihan H, Temizel M, Catik S, Meral Y, Orman A, Yibar A, Gencoglu H. Effects of supplemental mannanoligosaccharides on growth performance, faecal characteristics and health in dairy calves. Asian Aust. J. Anim. Sci. 2015;28:1599–1605. [PMC free article: PMC4647100] [PubMed: 26580284]
- Karcher EL, Hill TM, G. Bateman IIH, Schlotterbeck RL, Vito N, Sordillo LM, VandeHaar MJ. Comparison of supplementation of n-3 fatty acids from fish and flax oil on cytokine gene expression and growth of milk-fed Holstein calves. J. Dairy Sci. 2014;97:2329–2337. [PubMed: 24485693]
- Kato S, Sato K, Chida H, Roh S, Ohwada S, Sato S, Guilloteau P, Katoh K. Effects of Na-butyrate supplementation in milk formula on plasma concentrations of GH and insulin, and on rumen papilla development in calves. J. Endocrinol. 2011;211:241–248. [PubMed: 21911440]
- Kazemi-Bonchenari M, Falahati R, Poorhamdollah M, Heidari SR, Pezeshki A. Essential oils improved weight gain, growth and feed efficiency of young dairy calves fed 18 or 20% crude protein starter diets. J. Anim. Physiol. Anim. Nutr. 2018;102(3):652–661. [PubMed: 29341260]
- Kehoe SI, Carlson DB. Influence of nonmedicated additives as alternatives to antibiotics on calf growth and health. Prof. Anim. Sci. 2015;31:516–522.
- Kehoe SI, Dechow CD, Heinrichs AJ. Effects of weaning age and milk feeding frequency on dairy calf growth, health and rumen parameters. Livestock Sci. 2007;110:267–272.
- Kehoe SI, Heinrichs AJ, Moody ML, Jones CM, Long MR. Comparison of immunoglobulin G concentrations in primiparous and multiparous bovine colostrum. Prof. Anim. Sci. 2011;27:176–180.
- Kertz AF. Letter to the editor: Pelleted calf starter with straw access can confound results: A comment on Bach et al (2007). J. Dairy Sci. 2007;90:4924. [PubMed: 17954730]
- Kertz AF, Loften JR. A historical perspective of specific milk-replacer feeding programs in the United States and effects on eventual performance of Holstein dairy calves. Prof. Anim. Sci. 2013;29:321–332.
- Kertz AF, Prewitt LR, Everett JP Jr. An early weaning calf program: Summarization and review. J. Dairy Sci. 1979;62:1835–1843.
- Kertz AF, Reutzel LF, Mahoney JH. Ad libitum water intake by neonatal calves and its relationship to calf starter intake, weight gain, fecal score, and season. J. Dairy Sci. 1984;76:2964–2969. [PubMed: 6530492]
- Kertz AF, Hill TM, D. Quigley IIIJ, Heinrichs AJ, Linn JG, Drackley JK. A 100-year review: Calf nutrition and management. J. Dairy Sci. 2017;100:10151–10172. [PubMed: 29153160]
- Kesler EM. Feeding mastitic milk to calves: Review. J. Dairy Sci. 1981;64:719–723. [PubMed: 7264027]
- Khan MA, Lee HJ, Lee WS, Kim HS, Kim SB, Ki KS, Ha JK, Lee HG, Choi YJ. Pre- and postweaning performance of Holstein female calves fed milk through step-down and conventional methods. J. Dairy Sci. 2007;90:876–885. [PubMed: 17235164]
- Khan MA, Lee HJ, Lee WS, Kim HS, Kim SB, Park SB, Baek KS, Ha JK, Choi YJ. Starch source evaluation in calf starter: II. Ruminal parameters, rumen development, nutrient digestibilities, and nitrogen utilization in Holstein calves. J. Dairy Sci. 2008;91:1140–1149. [PubMed: 18292270]
- Khan MA, Weary DM, von Keyserlingk MAG. Invited review: Effects of milk ration on solid feed intake, weaning, and performance in dairy heifers. J. Dairy Sci. 2011a;94:1071–1081. [PubMed: 21338773]
- Khan MA, Weary DM, von Keyserlingk MAG. Hay intake improves performance and rumen development of calves fed higher quantities of milk. J. Dairy Sci. 2011b;94:3547–3553. [PubMed: 21700042]
- Khan MA, Bach A, Weary DM, von Keyserlingk MAG. Invited review: Transitioning from milk to solid feed in dairy heifers. J. Dairy Sci. 2016;99:885–902. [PubMed: 26709160]
- Khouri RH, Pickering FS. Nutrition of the milk-fed calf: I. Performance of calves fed on different levels of whole milk relative to body weight. N. Z. J. Agric. Res. 1968;11:227–236.
- Khuntia A, Chaudhary LC. Performance of male crossbred calves as influenced by substitution of grain by wheat bran and the addition of lactic acid bacteria to diet. Asian Aust. J. Anim. Sci. 2002;15:188–194.
- Kiezebrink DJ, Edwards AM, Wright TC, Cant JP, Osborne VR. Effect of enhanced whole-milk feeding in calves on subsequent first-lactation performance. J. Dairy Sci. 2015;98:349–356. [PubMed: 25468697]
- Kim MH, Seo JK, Yun CH, Kang SJ, Ko JY, Ha JK. Effects of hydrolyzed yeast supplementation in calf starter on immune responses to vaccine challenge in neonatal calves. Animal. 2011;5:953–960. [PubMed: 22440035]
- Kiser JS. A perspective on the use of antibiotics in animal feeds. J. Anim. Sci. 1976;42:1058–1072. [PubMed: 770412]
- Klotz JL, Heitmann RN. Effects of weaning and ionophore supplementation on selected blood metabolites and growth in dairy calves. J. Dairy Sci. 2006;89:3587–3598. [PubMed: 16899694]
- Kmicikewycz AD, da Silva DN, Linn JG, Litherland NB. Effects of milk replacer program fed 2 or 4 times daily on nutrient intake and calf growth. J. Dairy Sci. 2013;96:1125–1134. [PubMed: 23219113]
- Knauer WA, Godden SM, Dietrich A, James RE. The association between daily average feeding behaviors and morbidity in automatically fed group-housed preweaned dairy calves. J. Dairy Sci. 2017;100:5642–5652. [PubMed: 28478006]
- C Koch, Gerbert C, Frieten D, Dusel G, Eder K, Zitnan R, Hammon HM. Effects of ad libitum milk replacer feeding and butyrate supplementation on the epithelial growth and development of the gastrointestinal tract in Holstein calves. J. Dairy Sci. 2019;102:8513–8526. [PubMed: 31255268]
- Kogut MH, Klasing K. An immunologist's perspective on nutrition, immunity, and infectious diseases: Introduction and overview. J. App. Poultry Res. 2009;18:103–110.
- Korst M, Koch C, Kesser J, Müller U, Romberg F-J, Rehage J, Eder K, Sauerwein H. Different milk feeding intensities during the first 4 weeks of rearing in dairy calves: Part 1: Effects on performance and production from birth over the first lactation. J. Dairy Sci. 2017;100:3096–3108. [PubMed: 28131579]
- Krueger LA, Beitz DC, Onda K, Osman M, O'Neil MR, Lei S, Wattoo FH, Stuart RL, Tyler HD, Nonnecke B. Effects of d-α-tocopherol and dietary energy on growth and health of preruminant dairy calves. J. Dairy Sci. 2014;97(6):3715–3727. [PubMed: 24704235]
- Krueger LA, Reinhardt TA, Beitz DC, Stuart RL, Stabel JR. Effects of fractionated colostrum replacer and vitamins A, D, and E on haptoglobin and clinical health in neonatal Holstein calves challenged with Mycobacterium avium ssp. paratuberculosis. J. Dairy Sci. 2016;99(4):2884–2895. [PMC free article: PMC7094477] [PubMed: 26805975]
- Kruse PE. The importance of colostral immunoglobulins and their absorption from the intestine of the newborn animals. Ann. Rech. Vet. 1983;14:349–353. [PubMed: 6677175]
- Kruse V. Yield of colostrum and immunoglobulin in cattle at the first milking after parturition. Anim. Prod. 1970;12:619–626.
- Kuehn CS, Otterby DE, Linn JG, Olson WG, Chester-Jones H, Marx GD, Barmore JA. The effect of dietary energy concentration on calf performance. J. Dairy Sci. 1994;77:2621–2629. [PMC free article: PMC7130903] [PubMed: 7814732]
- Kung, L Jr, Demarco S, Siebenson LN, Joyner E, Haenlein GFW, Morris RM. An evaluation of two management systems for rearing calves fed milk replacer. J. Dairy Sci. 1997;80(10):2529–2533. [PubMed: 9361225]
- Kuroki M, Ohta M, Ikemori Y, Icatlo FC Jr, Kobayashi C, Yokoyama H, Kodama Y. Field evaluation of chicken egg yolk immunoglobulins specific for bovine rotavirus in neonatal calves. Arch. Virol. 1997;142:843–851. [PubMed: 9170509]
- Kushibiki S, Shingu H, Kawasaki R, Komatsu T, Itoh F, Watanabe A, Touno E, Oshibe A, Katoh K, Obara Y, Hodate K. Effect of bovine lactoferrin feeding on lipopolysaccharide-induced metabolic and hormonal disturbances in preruminant calves. Anim. Sci. J. 2008;79:375–381.
- Labussiere E, Bertrand G, Noblet J. The protein and energy needs of veal: determination and factors of variation. INRA Prod. Anim. 2007;20:355–368.
- Labussiere E, Dubois S, van Milgen J, Bertrand G, Noblet J. Effects of dietary crude protein on protein and fat deposition in milk-fed veal calves. J. Dairy Sci. 2008a;91:4741–4754. [PubMed: 19038950]
- Labussiere E, Dubois S, van Milgen J, Bertrand G, Noblet J. Fasting heat production and energy cost of standing activity in veal calves. Br. J. Nutr. 2008b;100:1315–1324. [PubMed: 18445304]
- Labussiere E, Dubois S, van Milgen J, Bertrand G, Noblet J. Effect of solid feed on energy and protein utilization in milk-fed veal calves. J. Anim. Sci. 2009;87:1106–1119. [PubMed: 18997064]
- Labussiere E, van Milgen J, de Lange CFM, Noblet J. Maintenance energy requirements of growing pigs and calves are influenced by feeding level. J. Nutr. 2011;141:1855–1861. [PubMed: 21865565]
- Lago A, McGuirk SM, Bennett TB, Cook NB, Nordlund KV. Calf respiratory disease and pen microenvironments in naturally ventilated calf barns in winter. J. Dairy Sci. 2006;89:4014–4025. [PubMed: 16960078]
- Lago AI, Socha M, Geiger A, Cook D, Silva-del-Rio N, Blanc C, Quesnell R, Leonardi C. Efficacy of colostrum replacer versus maternal colostrum on immunological status, health and growth of preweaned dairy calves. J. Dairy Sci. 2018;101:1344–1354. [PubMed: 29153521]
- Lallès JP. Nutritional and antinutritional aspects of soyabean and field pea proteins used in veal calf production: A review. Livestock Prod. Sci. 1993;34:181–202.
- Lallès JP, Poncet C. Changes in ruminal and intestinal digestion during and after weaning in dairy calves fed concentrate diets containing pea or soya bean meal: 1. Digestion of organic matter and nitrogen. Livestock Prod. Sci. 1990;24:129–142.
- Lallès JP, Dréau D, Salmon H, Toullec R. Identification of soyabean allergens and immune mechanisms of dietary sensitivities in preruminant calves. Res. Vet. Sci. 1996;60:111–116. [PubMed: 8685530]
- Lammers BP, Heinrichs AJ, Aydin A. The effect of whey protein concentrate or dried skim milk in milk replacer on calf performance and blood metabolites. J. Dairy Sci. 1998;81:1940–1945. [PubMed: 9710763]
- Langford FM, Weary DM, Fisher L. Antibiotic resistance in gut bacteria from dairy calves: A dose response to the level of antibiotics fed in milk. J. Dairy Sci. 2003;86:3963–3966. [PubMed: 14740833]
- Lassiter CA, Denton TW, Brown LD, Rust JW. The nutritional merits of pelleting calf starters. J. Dairy Sci. 1955;38:1242–1245.
- Leão JM, Lima JAM, Lana ÂMQ, Saturnino HM, Reis RB, Barbosa FA, de Azevedo RA, Sá Fortes RV, Coelho SG. Performance of crossbred heifers in different step-down waste milk-feeding strategies. Trop. Anim. health Prod. 2016;48:1607–1612. [PubMed: 27557701]
- Lee HJ, Khan MA, Lee WS, Yang SH, Kim SB, Ki KS, Kim HS, Ha JK, Choi YJ. Influence of equalizing the gross composition of milk replacer to that of whole milk on the performance of Holstein calves. J. Anim. Sci. 2009;87:1129–1137. [PubMed: 18997077]
- Leibholz J. The development of ruminant digestion in the calf: I. The digestion of barley and soya-bean meal. Aust. J. Agric. Res. 1975;26:1081–1091.
- Leibholz J. The development of ruminant digestion in the calf: II. The digestion of barley and urea. Aust. J. Agric. Res. 1978;29:1293–1299.
- Lesmeister KE, Heinrichs AJ. Effects of corn processing on growth characteristics, rumen development, and rumen parameters in neonatal dairy calves. J. Dairy Sci. 2004;87:3439–3450. [PubMed: 15377622]
- Lesmeister KE, Heinrichs AJ. Effects of adding extra molasses to a texturized calf starter on rumen development, growth characteristics, and blood parameters in neonatal dairy calves. J. Dairy Sci. 2005;88:411–418. [PubMed: 15591407]
- Li JH, Yousif MH, Li ZQ, Wu ZH, Li SL, Yang HJ, Wang YJ, Cao ZJ. Effects of antibiotic residues in milk on growth, ruminal fermentation, and microbial community of preweaning dairy calves. J. Dairy Sci. 2019;102:2298–2307. [PubMed: 30692007]
- Liang Y, Carroll JA, Ballou MA. The digestive system of 1-week-old Jersey calves is well suited to digest, absorb, and incorporate protein and energy into tissue growth even when calves are fed a high plane of milk replacer. J. Dairy Sci. 2016;99:1929–1937. [PubMed: 26723121]
- Litherland NB, Weich WD, P HansenW, Linn JG. Effects of feeding wheat straw or orchgardgrass at ad libitum or restricted intake during the dry period on postpartum performance and lipid metabolism. J. Dairy Sci. 2012;95:7236–7247. [PubMed: 23040018]
- Lofgreen G, Garrett W. A system for expressing net energy requirements and feed values for growing and finishing beef cattle. J. Anim. Sci. 1968;27:793–806.
- Løkke MM, Engelbrecht R, Wiking L. Covariance structures of fat and protein influence the estimation of IgG in bovine colostrum. J. Dairy Res. 2016;83:58–66. [PubMed: 26869112]
- Longenbach JI, Heinrichs AJ. A review of the importance and physiological role of curd formation in the abomasum of young calves. Anim. Feed Sci. Technol. 1998;73:85–97.
- López-Colum P, Castillejos L, Rodríguez-Sorrento A, Puyalto M, Mallo JJ, Martín-Orúe SM. Efficacy of medium-chain fatty acid salts distilled from coconut oil against two enteric pathogen challenges in weanling piglets. J. Anim. Sci. Biotechnol. 2019;10:89. [PMC free article: PMC6842466] [PubMed: 31728192]
- Losinger WC, Heinrichs AJ. Management practices associated with high mortality among preweaned dairy heifers. J. Dairy Res. 1997;64:1–11. [PubMed: 9120071]
- Ma J, Shah AM, Shao Y, Wang Z, Zou H, Hu R, Peng Q, Kang K, Wanapat M. Effects of yeast cell wall on the growth performance, ruminal fermentation, and microbial community of weaned calves. Livestock Sci. 2020a;239:104170.
- Ma J, Shah AM, Shao Y, Wang Z, Zou H, Kang K. Dietary supplementation of yeast cell wall improves the gastrointestinal development of weaned calves. Anim. Nutr. 2020b;6:507–512. [PMC free article: PMC7750790] [PubMed: 33364467]
- MacDougall DF, Mulligan W. The distribution and metabolism of fast IgG immunoglobulin in the neonatal calf. J. Physiol. 1969;201:77P–78P. [PMC free article: PMC1351548] [PubMed: 5780575]
- MacPherson JAR, Berends H, Leal LN, Cant JP, Martín-Tereso J, Steele MA. Effect of plane of milk replacer intake and age on glucose and insulin kinetics and abomasal emptying in female Holstein Friesian dairy calves fed twice daily. J. Dairy Sci. 2016;99:8007–8017. [PubMed: 27522426]
- Magalhães VJA, Susca F, Lima FS, Branco AF, Yoon I, Santos JEP. Effect of feeding yeast culture on performance, health, and immunocompetence of dairy calves. J. Dairy Sci. 2008;91:1497–1509. [PubMed: 18349243]
- Mann S, Yepes FA, Overton TR, Wakshlag JJ, Lock AL, Ryan CM, Nydam DV. Dry period plane of energy: Effects on feed intake, energy balance, milk production, and composition in transition dairy cows. J. Dairy Sci. 2015;98:3366–3382. [PubMed: 25771059]
- Mann S, Leal Yepes FA, Overton TR, Lock AL, Lamb SV, Wakshlag JJ, Nydam DV. Effect of dry period dietary energy level in dairy cattle on volume, concentrations of immunoglobulin G, insulin, and fatty acid composition of colostrum. J. Dairy Sci. 2016;99:1515–1526. [PubMed: 26709187]
- Margerison JK, Robarts ADJ, Reynolds GW. The effect of increasing the nutrient and amino acid concentration of milk diets on dairy heifer individual feed intake, growth, development, and lactation performance. J. Dairy Sci. 2013;96:6539–6549. [PubMed: 23958020]
- Marshall SP, Smith KL. Effect of different milks and level of intake upon growth of young dairy calves. J. Dairy Sci. 1970;53:1622–1626.
- Maynou G, Bach A, Terré M. Feeding of waste milk to Holstein calves affects antimicrobial resistance of Escherichia coli and Pasteurella multocida isolated from fecal and nasal swabs. J. Dairy Sci. 2017;100:2682–2694. [PubMed: 28215892]
- McCurdy DE, Wilkins KR, Hiltz RL, Moreland S, Klanderman K, Laarman AH. Effects of supplemental butyrate and weaning on rumen fermentation in Holstein calves. J. Dairy Sci. 2019;102:8874–8882. [PubMed: 31351719]
- McEwan AD, Fisher EW, Selman IE. An estimate of the efficiency of the absorption of immune globulins from colostrum by newborn calves. Res. Vet. Sci. 1970;11:239–243. [PubMed: 5498552]
- McGavin MD, Morrill JL. Scanning electron microscopy of ruminal papillae in calves fed various amounts and forms of roughage. Am. J. Vet. Res. 1976;37:497–508. [PubMed: 1275333]
- McGrath BA, Fox PF, McSweeney PLH, Kelly AL. Composition and properties of bovine colostrum: A review. Dairy Sci. Technol. 2016;96:133–158.
- Meale SJ, Leal LN, Martín-Tereso J, Steele MA. Delayed weaning of Holstein bull calves fed an elevated plane of nutrition impacts feed intake, growth and potential markers of gastrointestinal development. Anim. Feed Sci. Technol. 2015;209:268–273.
- Meale SJ, Chaucheyras-Durand F, Berends H, Guan LL, Steele MA. From pre- to postweaning: Transformation of the young calf's gastrointestinal tract. J. Dairy Sci. 2017;100:5984–5995. [PubMed: 28527800]
- Medrano-Galarza C, LeBlanc SJ, DeVries TJ, Jones-Bitton A, Rushen J, de Passillé AM, Endres MI, Haley DB. Effect of age of introduction to an automated milk feeder on calf learning and performance and labor requirements. J. Dairy Sci. 2018a;101:9371–9384. [PubMed: 30055924]
- Medrano-Galarza C, LeBlanc SJ, Jones-Bitton A, DeVries TJ, Rushen J, de Passillé AM, Endres MI, Haley DB. Associations between management practices and within-pen prevalence of calf diarrhea and respiratory disease on dairy farms using automated milk feeders. J. Dairy Sci. 2018b;101:2293–2308. [PubMed: 29290433]
- Meyer MJ. Department of Animal Science, Cornell University; Ithaca, NY: 2005. Developmental, nutritional, and hormonal regulation of mammary growth, steroid receptor gene expression and chemical composition of retained tissues in prepubertal bovine PhD.
- Meyer MJ, Capuco AV, Ross DA, Lintault LM, Van Amburgh ME. Developmental and nutritional regulation of the prepubertal heifer mammary gland: I. Parenchyma and fat pad mass and composition. J. Dairy Sci. 2006a;89:4289–4297. [PubMed: 17033016]
- Meyer MJ, Capuco AV, Ross DA, Lintault LM, Van Amburgh ME. Developmental and nutritional regulation of the prepubertal bovine mammary gland: II. Epithelial cell proliferation, parenchymal accretion rate, and allometric growth. J. Dairy Sci. 2006b;89:4298–4304. [PubMed: 17033017]
- Micke GC, Sullivan TM, Gatford KL, Owens JA, Perry VE. Nutrient intake in the bovine during early and mid-gestation causes sex-specific changes in progeny plasma IGF-I, liveweight, height and carcass traits. Anim. Reprod. Sci. 2010;121:208–217. [PubMed: 20591585]
- Miller WJ. Comparison of lard, tallow, butter, and hydrogenated cottonseed oil in starters and of pelleted vs. nonpelleted coastal bermudagrass hay for calves. J. Dairy Sci. 1962;45:759–764.
- Miller WJ, Carmon JL, Dalton HL. Influence of high levels of plant and animal fats in calf starters on growth, feed consumption, and palatability. J. Dairy Sci. 1959;42:153–158.
- Miller-Cushon EK, DeVries TJ. Effect of social housing on the development of feeding behavior and social feeding preferences of dairy calves. J. Dairy Sci. 2016;99:1406–1417. [PubMed: 26686722]
- Miller-Cushon EK, Montoro C, Ipharraguerre IR, Bach A. Dietary preference in dairy calves for feed ingredients high in energy and protein. J. Dairy Sci. 2014a;97:1634–1644. [PubMed: 24418273]
- Miller-Cushon EK, Terré M, DeVries TJ, Bach A. The effect of palatability of protein source on dietary selection in dairy calves. J. Dairy Sci. 2014b;97:4444–4454. [PubMed: 24767889]
- Mills JK, Ross DA, Van Amburgh ME. The effects of feeding medium-chain triglycerides on the growth, insulin responsiveness, and body composition of Holstein calves from birth to 85 kg of body weight. J. Dairy Sci. 2010;93:4262–4273. [PubMed: 20723699]
- Mitchell HH. Biological methods of measuring the protein values of feeds. J. Anim. Sci. 1943;2:263–277.
- Moallem U, Werner D, Lehrer H, Zachut M, Livshitz L, Yakoby S, Shamay A. Long-term effects of ad libitum whole milk prior to weaning and prepubertal protein supplementation on skeletal growth rate and first-lactation milk production. J. Dairy Sci. 2010;93:2639–2650. [PubMed: 20494173]
- Moe PW. Energy metabolism of dairy cattle. J. Dairy Sci. 1981;64:1120–1139. [PubMed: 7024342]
- Mojahedi S, Khorvash M, Ghorbani GR, Ghasemi E, Mirzaei M, Hashemzadeh-Cigari F. Performance, nutritional behavior, and metabolic responses of calves supplemented with forage depend on starch fermentability. J. Dairy Sci. 2018;101:7061–7072. [PubMed: 29778475]
- Montagne L, Toullec R, Lallès JP. Intestinal digestion of dietary and endogenous proteins along the small intestine of calves fed soybean or potato. J. Anim. Sci. 2001;79:2719–2730. [PubMed: 11721853]
- Montoro C, Bach A. Voluntary selection of starter feed ingredients offered separately to nursing calves. Livestock Sci. 2012;149:62–69.
- Montoro C, Miller-Cushon EK, DeVries TJ, Bach A. Effect of physical form of forage on performance, feeding behavior, and digestibility of Holstein calves. J. Dairy Sci. 2013;96:1117–1124. [PubMed: 23219116]
- Moore DA, Taylor J, Hartman ML, Sischo WM. Quality assessments of waste milk at a calf ranch. J. Dairy Sci. 2009;92:3503–3509.
- Moore M, Tyler JW, Chigerwe M, Dawes ME, Middleton JR. Effect of delayed colostrum collection on colostral IgG concentration in dairy cows. J. Am. Vet. Med. Assoc. 2005;226:1375–1377. [PubMed: 15844432]
- Morrill JL, Morrill JM, Feyerherm AM, Laster JF. Plasma proteins and a probiotic as ingredients in milk replacer. J. Dairy Sci. 1995;78:902–907. [PubMed: 7790582]
- Morrill KM, Conrad E, Lago A, Campbell J, Quigley J, Tyler H. Nationwide evaluation of quality and composition of colostrum on dairy farms in the United States. J. Dairy Sci. 2012;95:3997–4005. [PubMed: 22720954]
- Morrill KM, Robertson KE, Spring MM, Robinson AL, Tyler HD. Validating a refractometer to evaluate immunoglobulin G concentration in Jersey colostrum and the effect of multiple freeze–thaw cycles on evaluating colostrum quality. J. Dairy Sci. 2015;98:595–601. [PubMed: 25465569]
- Morrison SJ, Wicks HCF, Fallon RJ, Twigge J, Dawson LER, Wylie ARG, Carson AF. Effects of feeding level and protein content of milk replacer on the performance of dairy herd replacements. Animal. 2009;3:1570–1579. [PubMed: 22444990]
- Morrison SJ, Dawson S, Carson AF. The effects of mannan oligosaccharide and Streptococcus faecium addition to milk replacer on calf health and performance. Livestock Sci. 2010;131:292–296.
- Morrison SY, Campbell JM, Drackley JK. Amino acid supplementation of calf milk replacers containing plasma protein. J. Dairy Sci. J. Dairy Sci. 2017;100:4637–4649. [PubMed: 28342613]
- Morrison SY, LaPierre PA, Brost KN, Drackley JK. Intake and growth in transported Holstein calves classified as diarrheic or healthy within the first 21 days after arrival in a retrospective observational study. J. Dairy Sci. 2019;102:10997–11008. [PMC free article: PMC7094609] [PubMed: 31521358]
- Muller LD, Ellinger DK. Colostral immunoglobulin concentrations among breeds of dairy cattle. J. Dairy Sci. 1981;64:1727–1730. [PubMed: 7298971]
- Mzyk DA, Gehring R, Tell LA, Vickroy TW, Riviere JE, Ragan G, Baynes RE, Smith GW. Considerations for extra label drug use in calves. J. Am. Vet. Med. Assoc. 2017;250:1275–1282. [PubMed: 28509644]
- Nardone A, Lacetera N, Bernabucci U, Ronchi B. Composition of colostrum from dairy heifers exposed to high air temperatures during late pregnancy and the early postpartum period. J. Dairy Sci. 1997;80:838–844. [PubMed: 9178123]
- NASEM (National Academies of Sciences, Engineering, and Medicine). Nutrient Requirements of Beef Cattle. 8th rev. Washington, DC: The National Academies Press; 2016.
- Neathery MW, Crowe CT, Hartnell GF, Veenhuizen JJ, Reagan JO, Blackmon DM. Effects of sometribove on performance, carcass composition, and chemical blood characteristics of dairy calves. J. Dairy Sci. 1991;74:3933–3939. [PubMed: 1757630]
- Nelson CD, Lippolis JD, Reinhardt TA, Sacco RE, Powell JL, Drewnoski ME, O'Neil M, Beitz DC, Weiss WP. Vitamin D status of dairy cattle: Outcomes of current practices in the dairy industry. J. Dairy Sci. 2016;99(12):10150–10160. [PubMed: 27743666]
- Nestor KE Jr, Conrad HR. Metabolism of vitamin K and influence on prothrombin time in milk-fed preruminant calves. J. Dairy Sci. 1990;73(11):3291–3296. [PubMed: 2273156]
- Nocek JE, English JE, Braund DG. Effects of various forage feeding programs during the dry period on body condition and subsequent lactation health, production, and reproduction. J. Dairy Sci. 1983;66:1108–1118. [PubMed: 6875059]
- Nocek JE, Braund DG, Warner RG. Influence of neonatal colostrum administration, immunoglobulin, and continued feeding of colostrum on calf gain, health, and serum protein. J. Dairy Sci. 1984;67:319–333. [PubMed: 6715627]
- Nonnecke BJ, Horst RL, Waters WR, Dubeski P, Harp JA. Modulation of fat-soluble vitamin concentrations and blood mononuclear leukocyte populations in milk replacer-fed calves by dietary vitamin A and 13-carotene. J. Dairy Sci. 1999;82:2632–2641. [PubMed: 10629811]
- Nonnecke BJ, Foote MR, Smith JM, Pesch BA, Van Amburgh ME. Composition and functional capacity of blood mononuclear leukocyte populations from neonatal calves on standard and intensified milk replacer diets. J. Dairy Sci. 2003;86:3592–3604. [PubMed: 14672190]
- Nonnecke BJ, Reinhardt TA, Waters WR. Short communication: The preruminant calf as a model for characterizing the effects of vitamin D status in the neonate. J. Dairy Sci. 2009;92:5692–5696. [PubMed: 19841228]
- Nonnecke BJ, Foote MR, Miller BL, Beitz DC, Horst RL. Short communication: Fat-soluble vitamin and mineral status of milk replacer-fed dairy calves: Effect of growth rate during the preruminant period. J. Dairy Sci. 2010;93(6):2684–2690. [PubMed: 20494177]
- Nonnecke BJ, McGill JL, Ridpath JF, Sacco RE, Lippolis JD, Reinhardt TA. Acute phase response elicited by experimental bovine diarrhea virus (BVDV) infection is associated with decreased vitamin D and E status of vitamin-replete preruminant calves. J. Dairy Sci. 2014;97(9):5566–5579. [PubMed: 25022687]
- Nordlund KV. Practical considerations for ventilating calf barns in winter. Vet. Clin. Food. Anim. 2008;24:41–54. [PubMed: 18299031]
- Norman AW. From vitamin D to hormone D: Fundamentals of the vitamin D endocrine system essential for good health. Am. J. Clin. Nutr. 2008;88(2):491S–499S. [PubMed: 18689389]
- NRC (National Research Council). Prenatal and Postnatal Mortality in Cattle. Publication 1685. Washington, DC: National Academy Press; 1968.
- NRC. Nutrient Requirements of Dairy Cattle. 5th rev. Washington, DC: National Academy Press; 1978.
- NRC. Nutritional Energetics of Domestic Animals. Washington, DC: National Academy Press; 1981.
- NRC. Nutrient Requirements of Dairy Cattle. 6th rev. Washington, DC: National Academy Press; 1989.
- NRC. Nutrient Requirements of Dairy Cattle. 7th rev. Washington, DC: National Academy Press; 2001.
- Obeidat BS, Cobb CJ, Sellers MD, Pepper-Yowell AR, Earleywine TJ, Ballou MA. Plate of nutrition during the preweaning period but not the grower phase influences neutrophil activity of Holstein calves. J. Dairy Sci. 2013;96:7155–7166. [PubMed: 24035017]
- Obitsu T, Morooka T, Okubo M, Asahida Y. Ruminal and postruminal digestion and nitrogen balance in early weaned calves fed soybean meal and heated soybean meal. Asian Aust. J. Anim. Sci. 1995;8:75–82.
- O'Hara E, Kelly A, McCabe MS, Kenny DA, Guan LL, Waters SM. Effect of a butyrate-fortified milk replacer on gastrointestinal microbiota and products of fermentation in artificially reared dairy calves at weaning. Sci. Rep. 2018;8:14901. [PMC free article: PMC6175921] [PubMed: 30297834]
- Ohtani S, Asahida Y, Hirose Y. The effect of grazing on the development of ruminal mucosa and papillae in the young calf. Nihon Chikusan gakkaiho. 1976;47:218–223.
- Okamoto M, Robinson JB, Christopherson RJ, Young BA. Summit metabolism of newborn calves with and without colostrum feeding. Can. J. Anim. Sci. 1986;66:937–944.
- Ollivett TL, Nydam DV, Linden TC, Bowman D, Van Amburgh ME. Effect of nutritional plane on health and performance in dairy calves after experimental infection with Cryptosporidium parvum. J. Am. Vet. Med. Assoc. 2012;241:1514–1520. [PubMed: 23176246]
- Oltramari CE, Nápoles GGO, De Paula MR, Silva JT, Gallo MPC, Soares MC, Bittar CMM. Performance and metabolism of dairy calves fed starter feed containing citrus pulp as a replacement for corn. Anim. Prod. Sci. 2018;58:561–567. [PMC free article: PMC4932592] [PubMed: 26954149]
- Ontsouka EC, Albrecht C, Bruckmaier RM. Invited review: Growth-promoting effects of colostrum in calves based on interaction with intestinal cell surface receptors and receptor-like transporters. J. Dairy Sci. 2016;99:4111–4123. [PubMed: 26874414]
- Orskov ER. Reflex closure of the oesophageal groove and its potential application in ruminant nutrition. S. Afr. J. Anim. Sci. 1972;2:169–176.
- Ortigues I, Martin C, Vermorel M, Anglaret Y. Energy cost of standing and circadian changes in energy expenditure in the preruminant calf. J. Anim. Sci. 1994;72:2131–2140. [PubMed: 7982844]
- Ortigues-Marty I, Hocquette JF, Bertrand G, Martineau C, Vermorel M, Toullec R. The incorporation of solubilized wheat proteins in milk replacers for veal calves: Effects on growth performance and muscle oxidative capacity. Reprod. Nutr. Dev. 2003;43:57–76. [PubMed: 12785450]
- Osorio JS, Wallace RL, Tomlinson DJ, Earleywine TJ, Socha MT, Drackley JK. Effects of source of trace minerals and plane of nutrition on growth and health of transported neonatal dairy calves. J. Dairy Sci. 2012;95:5831–5844. [PubMed: 22884337]
- Otterby DE, Linn JG. Advances in nutrition and management of calves and heifers. J. Dairy Sci. 1981;64:1365–1377.
- Palmquist DL. Omega-3 fatty acids in metabolism, health, and nutrition and for modified animal product foods. Prof. Anim. Sci. 2009;25:207–249.
- Pattanaik AK, Sastry VRB, Katiyar RC, Lal M. Influence of grain processing and dietary protein degradability on nitrogen metabolism, energy balance and methane production in young calves. Asian Aust. J. Anim. Sci. 2003;16:1443–1450.
- Paul AA, Southgate DA. McCance andWiddowson's The Composition of Foods. 4th ed. London, UK: Her Majesty's Stationary Office; 1978.
- Payer DC, Holmes BJ. Fact sheet A3610. University of Wisconsin-Extension, Madison; 1994. Managing waste milk.
- Pazoki A, Ghorbani GR, Kargar S, Sadeghi-Sefidmazgi A, Drackley JK, Ghaffari MH. Growth performance, nutrient digestibility, ruminal fermentation, and rumen development of calves during transition from liquid to solid feed: Effects of physical form of starter feed and forage provision. Anim. Feed Sci. Technol. 2017;234:173–185.
- Pedersen RE, Sørensen JT, Skjøth F, Hindhede J, Rousing Nielsen T. How milk-fed dairy calves perform in stable versus dynamic groups. Livestock Sci. 2009;121:215–218.
- Pekmezci D, Cakiroglu D. Investigation of immunmodulatory effects of levamisole and vitamin E on immunity and some blood parameters in newborn Jersey calves. Vet. Res. Commun. 2009;33(7):711–721. [PubMed: 19387857]
- Pempek JA, Watkins LR, Bruner CE, Habing GG. A multisite, randomized field trial to evaluate the influence of lactoferrin on the morbidity and mortality of dairy calves with diarrhea. J. Dairy Sci. 2019;102:9259–9267. [PMC free article: PMC7094274] [PubMed: 31400894]
- Penhale WJ, Logan EF, Selman IE, Fisher E, McEwan AD. Observations on the absorption of colostral immunoglobulins by the neonatal calf and their significance in colibacillosis. Ann. Rech. Vet. 1973;4:223.
- Pereira RV, Siler JD, Bicalho RC, Warnick LD. Multiresidue screening of milk withheld for sale at dairy farms in central New York State. J. Dairy Sci. 2014;97:1513–1519. [PMC free article: PMC4349524] [PubMed: 24440252]
- Pereira RV, Carroll LM, Lima S, Foditsch C, Siler JD, Bicalho RC, Warnick LD. Impacts of feeding preweaned calves milk containing drug residues on the functional profile of the fecal microbiota. Sci. Rep. 2018;8:554. [PMC free article: PMC5764986] [PubMed: 29323259]
- Piantoni P, Bionaz M, Graugnard DE, Daniels KM, Akers RM, Loor JJ. Gene expression ratio stability evaluation in prepubertal bovine mammary tissue from calves fed different milk replacers reveals novel internal controls for quantitative polymerase chain reaction. J. Nutr. 2008;138:1158–1164. [PubMed: 18492850]
- Piantoni P, Bionaz M, Graugnard DE, Daniels KM, Everts RE, Rodriguez-Zas SL, Lewin HA, Hurley WL, Akers M, Loor JJ. Functional and gene network analyses of transcriptional signatures characterizing pre-weaned bovine mammary parenchyma or fat pad uncovered novel inter-tissue signaling networks during development. BMC genomics. 2010;11:331. [PMC free article: PMC2890563] [PubMed: 20504330]
- Piantoni P, Daniels KM, Everts RE, Rodriguez-Zas SL, Lewin HA, Hurley WL, Akers RM, Loor JJ. Level of nutrient intake affects mammary gland gene expression profiles in preweaned Holstein heifers. J. Dairy Sci. 2012;95:2550–2561. [PubMed: 22541482]
- Pike VL, Lythgoe KA, King KC. On the diverse and opposing effects of nutrition on pathogen virulence. Proc. R. Soc. B. 2019;286:20191220. [PMC free article: PMC6650706] [PubMed: 31288706]
- Pinos-Rodríguez JM, Robinson PH, Ortega ME, Berry SL, Mendoza G, Bárcena R. Performance and rumen fermentation of dairy calves supplemented with Saccharomyces cerevisiae 1077 or Saccharomyces boulardii 1079. Anim. Feed Sci. Technol. 2008;140:223–232.
- Pisoni L, Relling AE. The effects of supplementing yeast fermentation products on gut permeability, hormone concentration, and growth in newborn dairy calves. Transl. Anim. Sci. 2020;4:809–821. [PMC free article: PMC7001108] [PubMed: 32705006]
- Pollock JM, Rowan TG, Dixon JB, Carter SD, Spiller D, Warenius H. Alteration of cellular immune responses by nutrition and weaning in calves. Res. Vet. Sci. 1993;55:298–306. [PubMed: 8284492]
- Pollock JM, Rowan TG, Dixon JB, Carter SD. Level of nutrition and age at weaning: effects on humoral immunity in young calves. Br. J. Nutr. 1994;71:239–248. [PubMed: 8142335]
- Porter JC, Warner RG, Kertz AF. Effect of fiber level and physical form of starter on growth and development of dairy calves fed no forage. Prof. Anim. Sci. 2007;23:395–400.
- Prenner ML, Prgomet C, Sauerwein H, Pfaffl MW, Broz J, Schwarz FJ. Effects of lactoferrin feeding on growth, feed intake and health of calves. Arch. Anim. Nutr. 2007;61:20–30. [PubMed: 17361945]
- Pritchett LC, Gay CC, Besser TE, Hancock DD. Management and production factors influencing immunoglobulin G1 concentration in colostrum from Holstein cows. J. Dairy Sci. 1991;74:2336–2341. [PubMed: 1894821]
- Puvogel G, Baumrucker C, Blum JW. Plasma vitamin A status in calves fed colostrum from cows that were fed vitamin A during late pregnancy. J. Anim. Physiol. Anim. Nutr. 2008;92(5):614–620. [PubMed: 19012606]
- Quezada-Mendoza VC, Heinrichs AJ, Jones CM. The effects of a prebiotic supplement (Prebio Support) on fecal and salivary IgA in neonatal dairy calves. Livestock Sci. 2011;142:222–228. [PMC free article: PMC7102690] [PubMed: 32288870]
- Quigley JD III. Calves, heifers, and Dairy Profitability: Facilities, Nutrition, and health. Ithaca, NY: Northeast Regional Agricultural Engineering Service; 1996a. Feeding prior to weaning; pp. 245–255.
- Quigley JD III. Influence of weaning method on growth, intake, and selected blood metabolites in Jersey calves. J. Dairy Sci. 1996b;79:2255–2260. [PubMed: 9029364]
- Quigley JD III. Effects of spray-dried whole egg and biotin in calf milk replacer. J. Dairy Sci. 2002;85:198–203. [PubMed: 11860112]
- Quigley JD III, Bernard JK. Milk replacers with or without animal plasma for dairy calves. J. Dairy Sci. 1996;79:1881–1884. [PubMed: 8923259]
- Quigley JD III, Drew MD. Effects of oral antibiotics or bovine plasma on survival, health and growth in dairy calves challenged with Escherichia coli. Food Agric. Immunol. 2000;12:311–318.
- Quigley JD III, Drewry JJ. Nutrient and immunity transfer from cow to calf pre- and postcalving. J. Dairy Sci. 1998;81:2779–2790. [PubMed: 9812284]
- Quigley JD III, Schwab CG. Comparison of D-alanine and diaminopimelic acid as bacterial markers in young calves. J. Anim. Sci. 1988;66:758–763. [PubMed: 3378931]
- Quigley JD III, Wolfe TM. Effects of spray-dried animal plasma in calf milk replacer on health and growth of dairy calves. J. Dairy Sci. 2003;86:586–592. [PubMed: 12647965]
- Quigley JD III, Schwab CG, Hylton WE. Development of rumen function in calves: nature of protein reaching the abomasum. J. Dairy Sci. 1985;68:694–702. [PubMed: 3989088]
- Quigley JD III, Wallis LB, Dowlen HH, Heitmann RN. Sodium bicarbonate and yeast culture effects on ruminal fermentation, growth, and intake in dairy calves. J. Dairy Sci. 1992;75:3531–3538. [PubMed: 1335462]
- Quigley JD III, Bernard JK, Tyberendt TL, Martin KR. Intake, growth, and selected blood parameters in calves fed calf starter via bucket or bottle. J. Dairy Sci. 1994;77:354–357. [PubMed: 8120205]
- Quigley JD III, Martin KR, Bemis DA, Potgieter LND, Reinemeyer CR, Rohrbach BW, Dowlen HH, Lamar KC. Effects of housing and colostrum feeding on serum immunoglobulins, growth, and fecal score of Jersey calves. J. Dairy Sci. 1995;78:893–901. [PMC free article: PMC7131206] [PubMed: 7790581]
- Quigley JD III, Drewry JJ, Murray LM, Ivey SJ. Body weight gain, feed efficiency, and fecal scores of dairy calves in response to galactosyl-lactose or antibiotics in milk replacers. J. Dairy Sci. 1997a;80:1751–1754. [PubMed: 9276816]
- Quigley JD III, Drewry JJ, Murray LM, Ivey SJ. Effects of lasalocid in milk replacer or calf starter on health and performance of calves challenged with Eimeria species. J. Dairy Sci. 1997b;80:2972–2976. [PubMed: 9406090]
- Quigley JD III, Drewry JJ, Martin KR. Estimation of plasma volume in Holstein and Jersey calves. J. Dairy Sci. 1998;81:1308–1312. [PubMed: 9621233]
- Quigley JD III, Kost CJ, Wolfe TA. Effects of spray-dried animal plasma in milk replacers or additives containing serum and oligosaccharides on growth and health of calves. J. Dairy Sci. 2002;85:413–421. [PubMed: 11913702]
- Quigley JD III, Wolfe TA, Elsasser TH. Effects of additional milk replacer feeding on calf health, growth, and selected blood metabolites in calves. J. Dairy Sci. 2006;89:207–216. [PMC free article: PMC7164769] [PubMed: 16357284]
- Quigley JD III, Lago A, Chapman C, Erickson P, Polo J. Evaluation of the Brix refractometer to estimate immunoglobulin G concentration in bovine colostrum. J. Dairy Sci. 2013;96:1148–1155. [PubMed: 23200468]
- Quigley JD III, Hill TM, Dennis TS, Suarez-Mena FX, Schlotterbeck RL. Effects of feeding milk replacer at 2 rates with pelleted, low-starch or texturized, high-starch starters on calf performance and digestion. J. Dairy Sci. 2018;101:5937–5948. [PubMed: 29655558]
- Quigley JD III, Hill TM, Hulbert LE, Dennis TS, Suarez-Mena XF, Bortoluzzi EM. Effects of fatty acids and calf starter form on intake, growth, digestion, and selected blood metabolites in male calves from 0 to 4 months of age. J. Dairy Sci. 2019a;102:8074–8091. [PubMed: 31326170]
- Quigley JD III, Hu W, Knapp JR, Dennis TS, Suarez-Mena FX, Hill TM. Estimates of calf starter energy affected by consumption of nutrients: 2. Effect of changing digestion on energy content in calf starters. J. Dairy Sci. 2019b;102:2242–2253. [PubMed: 30692011]
- Radostits OM. Treatment and control of neonatal diarrhea in calves. J. Dairy Sci. 1975;58:464–470. [PMC free article: PMC7130761] [PubMed: 163850]
- Raeth M, Chester-Jones H, Ziegler D, Ziegler B, Schimek D, Cook DL, Golombeski G, Grove AV. Pre- and postweaning performance and health of dairy calves fed milk replacers with differing protein sources. Prof. Anim. Sci. 2016;32:833–841.
- Raeth-Knight M, Chester-Jones H, Hayes S, Linn J, Larson R, Ziegler D, Ziegler B, Broadwater N. Impact of conventional or intensive milk replacer programs on Holstein heifer performance through six months of age and during first lactation. J. Dairy Sci. 2009;92:799–809. [PubMed: 19164694]
- Randall L, Heinrich K, Horton R, Brunton L, Sharman M, Bailey-Horne V, Sharma M, McLaren I, Coldham N, Teale C, Jones J. Detection of antibiotic residues and association of cefquinome residues with the occurrence of extended-spectrum β-Lactamase (ESBL)–producing bacteria in waste milk samples from dairy farms in England and Wales in 2011. Res. Vet. Sci. 2014;96:15–24. [PubMed: 24314891]
- Rauprich ABE, Hammon HM, W J. Blum. Effects of feeding colostrum and a formula with nutrient contents as colostrum on metabolic and endocrine traits in neonatal calves. Biol. Neonate. 2000;78:53–64. [PubMed: 10878423]
- Raven AM. Fat in milk replacers for calves. J. Sci. Food Agric. 1970;21:352–359.
- Reddy PG, Morrill JL, Minocha HC, Morrill MB, Dayton AD, Frey RA. Effect of supplemental vitamin E on the immune system of calves. J. Dairy Sci. 1986;69:164–171. [PubMed: 3009573]
- Reddy PG, Morrill JL, Frey RA. Vitamin E requirements of dairy calves. J. Dairy Sci. 1987;70(1):123–129. [PubMed: 3571614]
- Reynolds LP, Ferrell CL, Robertson DA, Ford SP. Metabolism of the gravid uterus, foetus and utero-placenta at several stages of gestation in cows. J. Agric. Sci. 1986;106:437–444.
- Richard AL, Muller LD, Heinrichs AJ. Ad libitum or twice daily feeding of acidified milk replacer to calves housed individually in warm and cold environments. J. Dairy Sci. 1988;71:2193–2202.
- Ridder TA, Young JW, Anderson KA, Lodman DW, Odde KG, Johnson DE. Effects of prepartum energy nutrition and body condition on birthweight and basal metabolism in bovine neonates. J. Anim. Sci. 1991;69(Suppl. 1):450. abstract.
- Robbins KR, Saxton AM, Southern LL. Estimation of nutrient requirements using broken-line regression analysis. J. Anim. Sci. 2006;84(E. Suppl.):E155–E165. [PubMed: 16582088]
- Roblee ED, Erickson PS, Whitehouse NL, McLaughlin AM, Schwab CG, Rejman JJ, Rompala RE. Supplemental lactoferrin improves health and growth of Holstein calves during the preweaning phase. J. Dairy Sci. 2003;86:1458–1464. [PMC free article: PMC7095195] [PubMed: 12741570]
- Roh S, Kimura N, Sakamoto K, Nishihara K, Suzuki K, Katoh K. Effects of butyrate supplementation in antibiotic-free milk replacer and starter on growth performance in suckling calves. Anim. Sci. J. 2018;89:1486–1491. [PubMed: 30117232]
- Roland L, Drillich M, Klein-Jöbstl D, Iwersen M. Invited review: Influence of climatic conditions on the development, performance, and health of calves. J. Dairy Sci. 2016;99:2438–2452. [PubMed: 26874416]
- Rosenberger K, Costa JHC, Neave HW, von Keyserlingk MAG, Weary DM. The effect of milk allowance on behavior and weight gains in dairy calves. J. Dairy Sci. 2017;100:504–512. [PubMed: 27865513]
- Rowan TG. Thermoregulation in neonatal ruminants. In: Varley MA, Williams PEV, Lawrence TLJ, editors. Neonatal Survival and growth. Edinburgh, UK: British Society of Animal Production; 1992. pp. 13–24.
- Roy JHB. Diarrhoea of nutritional origin. Proc. Nutr. Soc. 1969;28:160–170. [PubMed: 5786591]
- Roy JHB. Protein in milk replacers for calves. J. Sci. Food Agric. 1970;21:346–351.
- Roy JHB. The Calf. 4th ed. Boston: Butterworths; 1980.
- Roy JHB, Huffman CF, Reineke EP. The basal metabolism of the newborn calf. Br. J. Nutr. 1957;11:373–381. [PubMed: 13489146]
- Rushen J, de Passillé AM, von Keyserlingk MAG, Weary DM. Housing for growing animals. In: Phillips C, editor. The Welfare of Cattle. Dordrecht, The Netherlands: Springer; 2008. pp. 181–210.
- Ruzante JM, Gardner IA, Cullor JS, Smith WL, Kirk JH, Adaska JM. Isolation of Mycobacterium avium subsp. paratuberculosis from waste milk delivered to California calf ranches. Foodborne Pathog. Dis. 2008;5:681–686. [PubMed: 18681799]
- Saeedi S, Dayani O, Tahmasbi R, Khezri A. Effect of supplementation of calf starter with fennel powder on performance, weaning age and fermentation characteristics in Holstein dairy calves. J. Anim. Physiol. Anim. Nutr. 2017;101(1):81–87. [PubMed: 28063208]
- Salazar LFL, Nero LA, Campos-Galvão MEM, Cortinhas CS, Acedo TS, Tamassia LFM, Busato KC, Morais VC, Rotta PP, Silva AL, Marcondes MI. Effect of selected feed additives to improve growth and health of dairy calves. PLoS ONE. 2019;14(5):e0216066. [PMC free article: PMC6499422] [PubMed: 31050665]
- Saldana DJ, Jones CM, Gehman AM, Heinrichs AJ. Effects of once- versus twice-a-day feeding of pasteurized milk supplemented with yeast-derived feed additives on growth and health in female dairy calves. J. Dairy Sci. 2019;102:3654–3660. [PubMed: 30799103]
- Samanta AK, Dass RS, Rawat M, Mishra SC, Mehra UR. Effect of dietary vitamin E supplementation on serum a-tocopherol and immune status of crossbred calves. Asian Aust. J. Anim. Sci. 2006;19(4):500–506.
- Sander EG, Warner RG, Harrison HN, Loosli JK. The stimulatory effect of sodium butyrate and sodium propionate on the development of rumen mucosa in the young calf. J. Dairy Sci. 1959;42:1600–1605.
- Santoro HM, Erickson PS, Whitehouse NL, McLaughlin AM, Schwab CG, D. Quigley IIIJ. Evaluation of a colostrum supplement, with or without trypsin inhibitor, and an egg protein milk replacer for dairy calves. J. Dairy Sci. 2004;87:1739–1746. [PubMed: 15453487]
- Santos FH, De Paula MR, Lezier D, Silva JT, Santos G, Bittar CM. Essential oils for dairy calves: Effects on performance, scours, rumen fermentation and intestinal fauna. Animal. 2015;9:958–965. [PubMed: 25690024]
- Schäff CT, Gruse J, Maciej J, Mielenz M, Wirthgen E, Hoeflich A, Schmicke M, Pfuhl R, Jawor P, Stefaniak T, Hammon HM. Effects of feeding milk replacer ad libitum or in restricted amounts for the first five weeks of life on the growth, metabolic adaptation, and immune status of newborn calves. PLoS ONE. 2016;11:e0168974. [PMC free article: PMC5201283] [PubMed: 28036351]
- Schingoethe DJ, Casper DP, Drackley JK, Ludens FC. Increased solids intake and feeding frequency for calves in hutches during cold weather. J. Dairy Sci. 1986;69:1063–1069. [PubMed: 3722528]
- Schrama JW, Arieli A, Heetkamp MJW, Verstegen MWA. Responses of young calves to low ambient temperatures at two levels of feeding. Anim. Prod. 1992a;53:397–405.
- Schrama JW, van der Hel W, Arieli A, Verstegen MWA. Alteration of energy metabolism of calves fed below maintenance during 6 to 14 days of age. J. Anim. Sci. 1992b;70:2527–2532. [PubMed: 1506314]
- Scibilia LS, Muller LD, Kensinger RS, Sweeney TF, Shellenberger PR. Effect of environmental temperature and dietary fat on growth and physiological responses of newborn calves. J. Dairy Sci. 1987;70:1426–1433. [PubMed: 3624595]
- Scrimshaw NS. Effect of infection on nutrient requirements. Am. J. Clin. Nutr. 1977;30:1526–1544. [PubMed: 331935]
- Sehested J, Jørgensen C, Mortensen SB, Jensen SK, Vestergaard M, Koch P, Jungersen G, Eriksen L. Effect of oral α-tocopherol and zinc on plasma status, IGF-I levels, weight gain and immune response in young calves. J. Anim. Feed Sci. 2004;13(Suppl. 1):609–612.
- Selim SA, Cullor JS. Number of viable bacteria and presumptive antibiotic residues in milk fed to calves on commercial dairies. J. Am. Vet. Med. Assoc. 1997;211:1029–1035. [PubMed: 9343549]
- Shea C, Whitehouse NL, Erickson PS. Effects of colostrum replacer supplemented with lactoferrin on the blood plasma. J. Anim. Sci. 2009;87:2047–2054. [PubMed: 19286825]
- Shivley CB, Lombard JE, Urie NJ, Haines DM, Sargent R, Kopral CA, Earleywine TJ, Olson JD, Garry FB. Preweaned heifer management on US dairy operations: Part II. Factors associated with colostrum quality and passive transfer status of dairy heifer calves. J. Dairy Sci. 2018;101:9185–9198. [PubMed: 29908806]
- Silva AL, Marcondes MI, Detmann E, Campos MM, Machado FS, Valadares Filho SC, Castro MMD, Dijkstra J. Determination of energy and protein requirements for crossbred Holstein × Gyr preweaned dairy calves. J. Dairy Sci. 2017;100:1170–1178. [PubMed: 27939536]
- Silva-del-Rio N, Fricke PM, Grummer RR. Effects of twin pregnancy and dry period strategy on milk production, energy balance, and metabolic profile in dairy cows. J. Anim. Sci. 2010;88:1048–1060. [PubMed: 19854992]
- Silva-del-Río N, Rolle D, García-Muñoz A, Rodríguez-Jiménez S, Valldecabres A, Lago A, Pandey P. Colostrum immunoglobulin G concentration of multiparous Jersey cows at first and second milking is associated with parity, colostrum yield, and time of first milking, and can be estimated with Brix refractometry. J. Dairy Sci. 2017;100:5774–5781. [PubMed: 28478013]
- Smith GW. Treatment of calf diarrhea: Oral fluid therapy. Vet. Clin. Food Anim. 2009;25:55–72. [PubMed: 19174283]
- Smith GW, Berchtold J. Fluid therapy in calves. Vet. Clin. North Am. Food Anim. Pract. 2014;30:409–427. [PubMed: 24980729]
- Sobczuk-Szul M, Wielgosz-Groth Z, Wronski M, Rzemieniewski A. Changes in bioactive protein concentrations in the bovine colostrum of jersey and polish Holstein-Friesian cows. Turk. J. Vet. Anim. Sci. 2013;37:43–49.
- Soberon F, Raffrenato E, Everett RW, Van Amburgh ME. Preweaning milk replacer intake and effects on long-term productivity of dairy calves. J. Dairy Sci. 2012;95:783–793. [PubMed: 22281343]
- Spain JN, Spiers DE. Effects of supplemental shade on thermoregulatory response of calves to heat challenge in a hutch environment. J. Dairy Sci. 1996;79:639446. [PubMed: 8744229]
- Spanski NA, Drackley JK, Davis CL, Jaster EH. Utilization of supplemental triglycerides or free fatty acids by calves from 4 to 10 weeks of age. J. Dairy Sci. 1997;80:573–585. [PubMed: 9098809]
- Stabel JR. On-farm batch pasteurization destroys Mycobacterium paratuberculosis in waste milk. J. Dairy Sci. 2001;84:524–527. [PubMed: 11233038]
- Stabel JR, Hurd S, Calvente L, Rosenbusch RF. Destruction of Mycobacterium paratuberculosis, Salmonella spp., and Mycoplasma spp. in raw milk by a commercial on-farm high-temperature, short-time pasteurizer. J. Dairy Sci. 2004;87:2177–2183. [PubMed: 15328232]
- Stamey JA, Janovick NA, Kertz AF, Drackley JK. Influence of starter protein content on growth of dairy calves in an enhanced early nutrition program. J. Dairy Sci. 2012;95:3327–3336. [PubMed: 22612966]
- Stamey Lanier J, McKeith FK, Janovick NA, Molano RA, Van Amburgh ME, Drackley JK. Influence of starter crude protein content on growth and body composition of dairy calves in an enhanced early nutrition program. J. Dairy Sci. 2021;104:3082–3097. [PubMed: 33358790]
- Stewart GD, Schingoethe DJ. Evaluation of high starch and high fat rations for dairy calves. J. Dairy Sci. 1984;67:598–605.
- Stobo IJF, Roy JHB, Gaston HJ. Rumen development in the calf: I. The effect of diets containing different proportions of concentrates to hay on rumen development. Br. J. Nutr. 1966;20:171–188. [PubMed: 5938699]
- Stobo IJF, Roy JHB, Gaston HJ. The protein requirement of the ruminant calf: I. The effect of protein content of the concentrate mixture on the performance of calves weaned at an early age. Anim. Sci. 1967;9:7–21.
- Stocco G, Summer A, Malacarne M, Cecchinato A, Bittante G. Detailed macro- and micromineral profile of milk: Effects of herd productivity, parity, and stage of lactation of cows of 6 dairy and dual-purpose breeds. J. Dairy Sci. 2019;102(11):9727–9739. [PubMed: 31477292]
- Stott GH, Marx DB, Menefee BE, Nightengale GT. Colostral immunoglobulin transfer in calves: I. Period of absorption. J. Dairy Sci. 1979;62:1632–1638. [PubMed: 536479]
- St-Pierre NR. Invited review: Integrating quantitative findings from multiple studies using mixed model methodology. J. Dairy Sci. 2001;84:741–755. [PubMed: 11352149]
- Strzetelski J, Niwinska B, Kowalezyk J, Jurkiewiez A. Effect of milk replacer feeding frequency and level of concentrate intake and rearing performance of calves. J. Anim. Feed Sci. 2001;10:413–420.
- Suárez BJ, Van Reenen CG, Beldman G, van Delen J, Dijkstra J, Gerrits WJJ. Effects of supplementing concentrates differing in carbohydrate composition in veal calf diets: I. Animal performance and rumen fermentation characteristics. J. Dairy Sci. 2006;89:4365–4375. [PubMed: 17033024]
- Suttle NF. Changes in the availability of dietary copper to young lambs associated with age and weaning. J. Agri. Sci. 1975;84:255–261.
- Svensson C, Jensen MB. Short communication: Identification of diseased calves by use of data from automatic milk feeders. J. Dairy Sci. 2007;90:994–997. [PubMed: 17235177]
- Svensson C, Liberg P. The effect of group size on health and growth rate of Swedish dairy calves housed in pens with automatic milk-feeders. Prev. Vet. Med. 2006;73:43–53. [PubMed: 16191449]
- Svensson C, Linder A, Olsson SO. Mortality in Swedish dairy calves and replacement heifers. J. Dairy Sci. 2006;89:4769–4777. [PubMed: 17106108]
- Swanson EW. Factors for computing requirements of protein for maintenance of cattle. J. Dairy Sci. 1977;60:1583–1593.
- Swanson KS, Merchen NR, Erdman JW Jr, Drackley JK, Orias F, Morin DE, Haddad MF. Influence of dietary vitamin A content on serum and liver vitamin A concentrations and health in preruminant Holstein calves fed milk replacer. J Dairy Sci. 2000;83:2027–2036. [PubMed: 11003235]
- Sweeney BC, Rushen J, Weary DM, de Passillé AM. Duration of weaning, starter intake, and weight gain of dairy calves fed large amounts of milk. J. Dairy Sci. 2010;93:148–152. [PubMed: 20059913]
- Tamate H, McGilliard AD, Jacobson NL, Getty R. Effect of various dietaries on the anatomical development of the stomach in the calf. J. Dairy Sci. 1962;45:408–420.
- Tanan KG. Nutrient sources for liquid feeding of calves. In: Garnsworthy PC, editor. Calf and heifer Rearing. Nottingham, UK: Nottingham University Press; 2005. pp. 83–112.
- Tao S, Dahl GE. Invited review: Heat stress effects during late gestation on dry cows and their calves. J. Dairy Sci. 2013;96:4079–4093. [PubMed: 23664343]
- Tao S, Monteiro APA, Thompson IM, Hayen MJ, Dahl GE. Effect of late-gestation maternal heat stress on growth and immune function of dairy calves. J. Dairy Sci. 2012;95:7128–7136. [PubMed: 23021751]
- Tempini PN, Aly SS, Karle BM, Pereira RV. Multidrug residues and antimicrobial resistance patterns in waste milk from dairy farms in Central California. J. Dairy Sci. 2018;101:8110–8122. [PubMed: 30126599]
- Ternouth JH, Stobo IJF, Roy JHB, Beattie AW. The effect of milk substitute concentration upon the intake, digestion and growth of calves. Anim. Sci. 1985a;41:151–159.
- Ternouth JH, Stobo IJF, Roy JHB, Beattie AW. The live-weight gain and dry-matter and water intakes of calves offered milk substitute diets at high concentrations. Anim. Sci. 1985b;41:161–166.
- Terosky TL, Heinrichs AJ, Wilson LL. A comparison of milk protein sources in diets of calves up to eight weeks of age. J. Dairy Sci. 1997;80:2977–2983. [PubMed: 9406091]
- Terré M, Devant M, Bach A. Performance and nitrogen metabolism of calves fed conventionally or following an enhanced-growth feeding program during the preweaning period. Livestock Sci. 2006;105:109–119.
- Terré M, Calvo MA, Adelantado C, Kocher A, Bach A. Effects of mannan oligosaccharides on performance and microorganism fecal counts of calves following an enhanced-growth feeding program. Anim. Feed Sci. Technol. 2007;137:115–125.
- Terré M, Tejero C, Bach A. Long-term effects on heifer performance of an enhanced-growth feeding programme applied during the preweaning period. J. Dairy Res. 2009;76:331–339. [PubMed: 19445830]
- Terré M, Pedrals E, Dalmau A, Bach A. What do preweaned and weaned calves need in the diet: A high fiber content or a forage source? J. Dairy Sci. 2013;96:5217–5225. [PubMed: 23706487]
- Terré M, Castells L, Khan MA, Bach A. Interaction between the physical form of the starter feed and straw provision on growth performance of Holstein calves. J. Dairy Sci. 2015a;98:1101–1109. [PubMed: 25497821]
- Terré M, Maynou G, Bach A, Gauthier M. Effect of Saccharomyces cerevisiae CNCM I-1077 supplementation on performance and rumen microbiota of dairy calves. Prof. Anim. Sci. 2015b;31:153–158.
- Terui H, Morrill JL, Higgins JJ. Evaluation of wheat gluten in milk replacers and calf starters. J. Dairy Sci. 1996;79:1261–1266. [PubMed: 8872721]
- Thickett WS, Cuthbert NH, Brigstocke TDA, Linedman MA. The management of calves on an early-weaning system: The relationship of voluntary water intake to dry feed intake and live-weight gain to 5 weeks. Anim. Sci. 1981;33:25–30.
- Thomas TJ, Weary DM, Appleby MC. Newborn and 5-week-old calves vocalize in response to milk deprivation. Appl. Anim. Behav. Sci. 2001;74:165–173.
- Tikofsky JN, Van Amburgh ME, Ross DA. Effect of varying carbohydrate and fat content of milk replacer on body composition of Holstein bull calves. J. Anim. Sci. 2001;79:2260–2267. [PubMed: 11583412]
- Timmerman HM, Mulder L, Everts H, van Espen DC, van der Wal E, Klaassen G, Rouwers SM, Hartemink R, Rombouts FM, Beynen AC. Health and growth of veal calves fed milk replacers with or without probiotics. J. Dairy Sci. 2005;88:2154–2165. [PubMed: 15905445]
- Tomkins T, Jaster EH. Preruminant calf nutrition. Vet. Clin. North Am. Food Anim. Pract. 1991;7:557–576. [PubMed: 1893285]
- Touchette KJ, O'Brien ML, Coalson JA. Liquid egg as an alternative protein source in calf milk replacers. J. Dairy Sci. 2003;86:2622–2628. [PubMed: 12939086]
- Toullec R. Veal calves. In: Jarrige R, editor. Ruminant Nutrition—Recommended Allowances and Feed Tables. Paris, France: INRA; 1989. pp. 109–119.
- Toullec R, Guilloteau P. Research into the digestive physiology of the milk-fed calf. In: van Weerden EJ, Huisman J, editors. Nutrition and Digestive Physiology in Monogastric Farm Animals. Wageningen, The Netherlands: Pudoc; 1989. pp. 37–55.
- Tucker WB, Jackson JA, Hopkins DM, Hogue JF. Influence of dietary sodium bicarbonate on the potassium metabolism of growing dairy calves. J. Dairy Sci. 1991;74:2296–2302. [PubMed: 1654349]
- Urie NJ, Lombard JE, Shivley CB, Kopral CA, Adams AE, Earleywine TJ, Olson JD, Garry FB. Preweaned heifer management on US dairy operations: Part I. Descriptive characteristics of preweaned heifer raising practices. J. Dairy Sci. 2018a;101:9168–9184. [PMC free article: PMC7094552] [PubMed: 29908815]
- Urie NJ, Lombard JE, Shivley CB, Kopral CA, Adams AE, Earleywine TJ, Olson JD, Garry FB. Preweaned heifer management on US dairy operations: Part V. Factors associated with morbidity and mortality in preweaned dairy heifer calves. J. Dairy Sci. 2018b;101:9229–9244. [PMC free article: PMC7094390] [PubMed: 29935825]
- USDA (U.S. Department of Agriculture). Dairy Cattle Management Practices in the United States, 2014. Fort Collins, CO: USDA, Animal and Plant Health Inspection Service. Veterinary Services. National Animal Health Monitoring System (USDA-APHIS-VS-CEAH-NAHMS); 2016.
- Vailati-Riboni M, Bucktrout RE, Zhan S, Geiger A, McCann JC, Akers RM, Loor JJ. Higher plane of nutrition pre-weaning enhances Holstein calf mammary gland development through alterations in the parenchyma and fat pad transcriptome. BMC genomics. 2018;19:900. [PMC free article: PMC6290502] [PubMed: 30537932]
- Van Amburgh ME, Galton DM, Bauman DE, Everett RW, Fox DG, Chase LE, Erb HN. Effects of three prepubertal body growth rates on performance of Holstein heifers during first lactation. J. Dairy Sci. 1998;81:527–538. [PubMed: 9532507]
- Van Amburgh ME, Soberon F, Meyer MJ, Molano RA. Symposium review: Integration of postweaning nutrient requirements and supply with composition of growth and mammary development in modern dairy heifers. J. Dairy Sci. 2019;102:3692–3705. [PubMed: 30660424]
- van den Borne JJG C, Verstegen MWA, Alferink SJJ, Giebels RMM, Gerrits WJJ. Effects of feeding frequency and feeding level on nutrient utilization in heavy preruminant calves. J. Dairy Sci. 2006;89:3578–3586. [PubMed: 16899693]
- van den Borne JJG C, Lobley GF, Verstegen MW, Muijlaert JM, Alferink SJJ, Gerrits WJ. Body fat deposition does not originate from carbohydrates in milk-fed calves. J. Nutr. 2007;137:2234–2241. [PubMed: 17885004]
- Van Saun RJ, Herdt TH, Stowe HD. Maternal and fetal selenium concentrations and their interrelationships in dairy cattle. J. Nutr. 1989a;119:1128–1137. [PubMed: 2778538]
- Van Saun RJ, Herdt TH, Stowe HD. Maternal and fetal vitamin E concentrations and selenium–vitamin E interrelationships in dairy cattle. J. Nutr. 1989b;119:1156–1164. [PubMed: 2778541]
- Vasquez KM, Morrison SY, Campbell JM, Drackley JK. Plasma protein and supplemental isoleucine in milk replacers for dairy calves. J. Dairy Sci. 2017;100:293–304. [PubMed: 27865507]
- Vermorel M, Dardillat C, Vernat J, Saido, Demigne C. Energy metabolism and thermoregulation in the newborn calf. Ann. Rech. Vet. 1983;14:382–389. [PubMed: 6677179]
- Waltner-Toews D, Martin S, Meek A. Dairy calf management, morbidity and mortality in Ontario Holstein herds: II. Age and seasonal patterns. Prev. Vet. Med. 1986a;4:125–135.
- Waltner-Toews D, Martin S, Meek A. Dairy calf management, morbidity and mortality in Ontario Holstein herds: III. Association of management with morbidity. Prev. Vet. Med. 1986b;4:137–158.
- Walz PH, Mullaney TP, Render JA, Walker RD, Mosser T, Baker JC. Otitis media in preweaned Holstein dairy calves in Michigan due to Mycoplasma bovis. J. Vet. Diagn. Invest. 1997;9:250–254. [PubMed: 9249163]
- Wang J, Diao Q, Tu Y, Zhang N, Xu X. The limiting sequence and proper ratio of lysine, methionine and threonine for calves fed milk replacers containing soy protein. Asian Aust. J. Anim. Sci. 2012;25:224–233. [PMC free article: PMC4093134] [PubMed: 25049555]
- Warner RG. Proceedings Cornell Nutrition Conference for Feed Manufacturers. Ithaca, NY: Cornell, University; 1991. Nutritional factors affecting the development of a functional ruminant—A historical perspective; pp. 1–12.
- Warner RG, Flatt WP, Loosli JK. Dietary factors influencing the development of the animal's stomach. J. Agric. Food Chem. 1956;4:788–792.
- Watson AK, Hales KE, Hersom MJ, Horn GW, Wagner JJ, Krehbiel CR, McCurdy MP, Erickson GE. Mineral retention of growing and finishing beef cattle across different production systems. Prof. Anim. Sci. 2018;34(3):250–260.
- Webster AJF, Gordon JG, McGregor R. The cold tolerance of beef and dairy type calves in the first weeks of life. Anim. Prod. 1978;26:85–92.
- Wells SJ, Dargatz DA, Ott SL. Factors associated with mortality to 21 days of life in dairy heifers in the United States. Prev. Vet. Med. 1996;29:9–19.
- West JW. Effects of heat-stress on production in dairy cattle. J. Dairy Sci. 2003;86:2131–2144. [PubMed: 12836950]
- Wickramasinghe HKJ P, Kramer AJ, Appuhamy JA.DRN. Drinking water intake of newborn dairy calves and its effects on feed intake, growth performance, health status, and nutrient digestibility. J. Dairy Sci. 2019;102:377–387. [PubMed: 30415859]
- Williams AP, Hewitt D. The amino acid requirements of the preruminant calf. Br. J. Nutr. 1979;41:311–319. [PubMed: 427084]
- Williams PEV, Frost AJ. Feeding the young ruminant. In: Varley M, Williams PEV, Lawrence TLJ, editors. Neonatal Survival and growth. Edinburgh, UK: British Society of Animal Production; 1992. pp. 109–118.
- Williams PEV, Day D, Raven AM, McLean JA. The effect of climatic housing and level of nutrition on the performance of calves. Anim. Prod. 1981;32:133–141.
- Williams PEV, Fallon RJ, Brockway JM, Innes GM, Brewer AC. The effect of frequency of feeding milk replacer to pre-ruminant calves on respiratory quotient and the efficiency of food utilization. Anim. Prod. 1986;43:367–375.
- Wilson GA, Wheelock JV. Factors affecting the action of rennin in heated milk. J. Dairy Res. 1972;39:413–419.
- Windeyer MC, Leslie KE, Godden SM, Hodgins DC, Lissemore KD, LeBlanc SJ. Factors associated with morbidity, mortality, and growth of dairy heifer calves up to 3 months of age. Prev. Vet. Med. 2014;113:231–240. [PubMed: 24269039]
- Xiao JX, Alugongo GM, Chung R, Dong SZ, Li SL, Yoon I, Wu ZH, Cao ZJ. Effects of Saccharomyces cerevisiae fermentation products on dairy calves: Ruminal fermentation, gastrointestinal morphology, and microbial community. J. Dairy Sci. 2016;99:5401–5412. [PubMed: 27157569]
- Yokoyama H, Peralta RC, Umeda K, Hashi T, Icatlo FC Jr, Kuroki M, Ikemori Y, Kodama Y. Prevention of fatal salmonellosis in neonatal calves, using orally administered chicken egg yolk Salmonella specific antibodies. Am. J. Vet. Res. 1988;59:416–420. [PubMed: 9563623]
- Youanes YD, Herdt TH. Changes in small intestinal morphology and flora associated with decreased energy digestibility in calves with naturally occurring diarrhea. Am. J. Vet. Res. 1987;48:719–725. [PubMed: 3592372]
- Yousif MH, Li JH, Li ZQ, Alugongo GM, Ji SK, Li YX, Wang YJ, Li SL, Cao ZJ. Low concentration of antibiotics modulates gut microbiota at different levels in pre-weaning dairy calves. Microorganisms. 2018;6:118. [PMC free article: PMC6313529] [PubMed: 30486334]
- Yunta C, Terré M, Bach A. Short- and medium-term changes in performance and metabolism of dairy calves offered different amounts of milk replacers. Livestock Sci. 2015;181:249–255.
- Zanker I, Hammon H, Blum J. Beta-carotene, retinol and alpha-tocopherol status in calves fed the first colostrum at 0–2, 6–7, 12–13 or 24–25 hours after birth. Int. J. Vit. Nutr. Res. 2000;70(6):305–310. [PubMed: 11214356]
- INTRODUCTION
- BODY WEIGHT CONVERSIONS
- DRY MATTER INTAKE
- ENERGY REQUIREMENTS OF CALVES
- EFFECTS OF SOURCES OF ENERGY
- PROTEIN REQUIREMENTS
- CALCULATION OF METABOLIZABLE ENERGY VALUES OF FEEDS
- VALIDATION OF MODEL WITH EXPERIMENTAL DATA
- ENERGY AND PROTEIN REQUIREMENTS FOR CALVES
- MINERAL AND VITAMIN REQUIREMENTS OF CALVES
- PRACTICAL FEEDING CONSIDERATIONS
- OTHER ASPECTS OF CALF NUTRITION
- REFERENCES
- Nutrient Requirements of the Young Calf - Nutrient Requirements of Dairy CattleNutrient Requirements of the Young Calf - Nutrient Requirements of Dairy Cattle
Your browsing activity is empty.
Activity recording is turned off.
See more...