NCBI Bookshelf. A service of the National Library of Medicine, National Institutes of Health.
National Research Council (US) Panel for the Workshop on the Biodemography of Fertility and Family Behavior; Wachter KW, Bulatao RA, editors. Offspring: Human Fertility Behavior in Biodemographic Perspective. Washington (DC): National Academies Press (US); 2003.
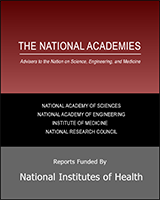
Offspring: Human Fertility Behavior in Biodemographic Perspective.
Show detailsDespite their essential participation in the reproductive process, the role of males in fertility variation has tended to be downplayed by demographers, who are primarily interested in fertility differentials between groups, though there is increasing interest in the role of men (see, for instance, Bledsoe et al., 2000). A focus on females makes sense for all of the standard reasons in terms of reproductive accounting because of maternal certainty and the fact that females have more constrained reproductive spans and fertility. However, the benefits of this accounting approach begin to decline as we move further afield to consider factors less directly related to pregnancy, such as behavior. Here there is more room for other perspectives with which to orient our thinking.
From an evolutionary point of view, male humans, as the sex with greater variation in reproductive success, can be expected to be subject to more intense selection (Bateman, 1948). Recently, genetic evidence supports the idea that male reproductive genes undergo more rapid evolution than females (Wyckoff et al., 2000), though it is unclear if this represents greater mutation rates in the male germ line because of more replication events (Vogel and Ratherberg, 1975) or the impact of other processes such as methylation of genes (Huttley et al., 2000) or incorporation of transposable elements (Erlandsson et al., 2000).
The impact of selection on male reproductive biology is evident in morphological differences in male genitalia across species (see Eberhard, 1985, for graphic examples), as well as functional differences in sperm production. Across primate species the ratio of testes size to body size (Harcourt et al., 1981) and even the morphology of sperm themselves (Anderson and Dixson, 2002) vary between monogamous and polygynous mating systems as a result of selection for sperm competition. For instance, seasonally breeding rhesus macaques have 60 percent larger testes than do the closely related and similarly sized nonseasonally breeding pigtailed macaques, a result of the greater mating competition during seasonal breeding. In addition, rhesus males have 60 percent larger abdominal fatfolds than pigtail males (Muhlenbein et al., 2002), suggesting that substantial energy reserves are needed to offset the energetic costs of male-male competition, among male rhesus.
Such findings are powerful evidence for the impact of selection on sperm production and delivery across species. However, the relatively small testicular to body size ratio exhibited by humans suggests that sperm competition in human males has played only a small role in human evolution (Dixson, 1998; Smith, 1984). Furthermore, the low percentage of normal sperm in human ejaculates is comparable to that of gorillas and very different from that of chimpanzees (Small, 1988), suggesting that the human male reproductive strategy has more in common with preinsemination mate guarding among gorillas than the postinsemination sperm competition of the chimpanzee. Thus, the fact that the size of male ejaculates has been shown to vary with time since exposure to their partners is less evidence for sperm competition in humans (Baker and Bellis, 1989) per se than it is for the importance of mate guarding.
However, in the case of humans, the role of paternal investment in offspring means that selection on males includes not only behavior leading to sex, and aspects of the male reproductive tract associated with insemination, but also behaviors related to pair bonding and the provisioning of offspring. Anecdotal evidence that testosterone increases in males exposed to mates after a prolonged absence (Bribiescas, 2001) suggests a role for testosterone in sexual behavior and pair bonding (see Young, this volume). Recent reports of lower testosterone levels in fathers of young children than in nonfathers (Berg and Wynne-Edwards, 2001; Storey et al., 2000) suggest that changes in testosterone may play a role in shifting behavior from mating to parenting.
With that brief orientation toward the critical elements of male reproductive strategies among humans, I turn to the central focus of this paper, the role of reproductive maturation in the development of male sexual behavior, pair bonding, and parenting.
Here I argue that this process can be considered to have two major dimensions: 1) sexual behavior that is related to reproductive maturation and somatic growth and that is organized by testosterone as part of the reproductive axis, and 2) social behavior that is related to early childhood experience and to the adrenal axis, which produces cortisol and dehydroepiandrosterone and its sulfate DHEA/S. Briefly put, maturation of the reproductive axis is crucial to sexual behavior, whereas development of the adrenal axis is crucial to social behavior and attachment and hence the expression of sexual behavior, as fertility and family formation. It is only by understanding the separate roles of these two important domains of human behavior and their interaction during maturation that we can get a differentiated account of the development of male reproductive behavior.
Of course, any such model represents a vast simplification of all that goes into male reproductive maturation. At the same time, it is intended to be a human model in the essential sense of the word, a model that integrates factors we encounter as part of our everyday existence, without necessarily experiencing them fully or in isolation. As such it should be applicable to variation across societies, including variation in social structures, and biological differences in the timing of adolescent maturation, as well as cultural practices that may have independent effects on behavior.
Finally, much of what will be discussed here is necessarily speculative. Thus, I conclude with recommendations for research that might elaborate and test how these hormones mediate the interaction of biological, psychological, and social levels of fertility behavior in human males.
THE SCOPE OF MALE REPRODUCTIVE MATURATION
Most biological analyses of male reproductive maturation focus exclusively on adolescence because of the obvious connection between pubertal maturation and the onset of sexual behavior. However, the onset of reproductive capacity and sexual behavior may be considered a necessary, but not sufficient, condition for reproduction among human males for whom pair bonding and paternal investment play an additional role (Lancaster and Lancaster, 1987). Thus, changes in familial relationships (Bee, 1997), cognition (Nelson, 1996), and the subjective sense of self (Herdt and McClintock, 2000) that begin prepubertally may be considered as part of the reproductive transition.
In addition, reproduction among males is generally associated with young adulthood and family formation, which do not take place until the early 20s. For instance, in the United States only 3 percent of births are fathered by men under the age of 20, while traditional Kikuyu men marry and become fathers around the age of 25 (Bogin, 2001). Thus, the establishment of productive skills and the development of social position can also be considered part of the process of male reproductive maturation (see Kaplan and Lancaster, this volume).
Furthermore, juvenile, adolescent, and young adult stages appear to be linked as a coherent biological process by increases in the production of DHEA/S by the adrenal gland (because of the close relationship of the two hormones, I will refer to them here collectively as DHEA/S unless discussing results that are specifically based on one of the two hormones). Based on Western populations, increases in the production of DHEA/S by the adrenal gland, a process referred to as adrenarche, begin around age 8 and continue until the mid-20s (Worthman, 1999), thus bracketing the progression of pubertal development from roughly ages 10 to 18 (see Figure 9-1). Furthermore, the increase of DHEA/S in males appears to extend some 5 years beyond that for females (Worthman, 1999) suggesting important sex differences in the onset of reproduction.
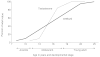
FIGURE 9-1
Temporal relationship of adrenarche and puberty in human males. NOTE: This graph indicates the relative role of changes in DHEA/S and testosterone at different stages of the development of males from age 8 to 25. Both the young adult and juvenile stages (more...)
DHEA/S is the most common hormone in the human body. Yet despite its well-known importance as a precursor for estrogen production during fetal development (Parker 1999) and its rise during maturation (Orentreich et al., 1992), a functional understanding of DHEA/S's role in postnatal development remains hazy. DHEA/S is a neurosteroid, meaning that it is produced in the brain as well as the adrenal gland (Baulieu, 1998), though a specific DHEA/S receptor is lacking (Robel and Baulieu et al., 1995). However, DHEA/S is known to occupy receptors for the neurotransmitter gamma aminobutyric acid type (GABA-A) acting as an antagonist (Majewska, 1995) and presumably influencing neural mechanisms in this way.
In addition, DHEA/S is converted to estrogen and testosterone in peripheral tissues (Labrie et al., 1995), meaning that it may also have effects through testosterone and estrogen receptors, not only within the brain but also on immune function and bone and muscle growth. For instance, males with 21-hydroxylase deficiency, a condition that leads to the overproduction of DHEA/S, have reduced fertility, as a result of increased estrogen concentrations suppressing gonadotropin stimulation of the testes (Cabrera et al., 2001; Stikkelbroeck et al., 2001). However, such individuals are also likely to have testicular tumors, clouding the implication of these findings for normal maturation.
DHEA/S is known to have a variety of physiological effects during maturation, including promoting muscle and bone growth (Argquitt et al., 1991; Zemel and Katz, 1986) and disease resistance (Kurtis et al., 2001), as well as playing a role in mood (Goodyer et al., 1996). In addition premature adrenarche has been shown to have effects on both cognition and psychosocial development (Dorn et al. 1999; Nass et al. 1990). Though it is unclear how far findings on cognition can be generalized, Goodyer et al. found that DHEA/S hyposecretion was related to major depression in a sample of 8- to 16-year olds, suggesting that DHEA/S does have important effects on mood during development in normal children.
BIOLOGY OF REPRODUCTIVE MATURATION IN HUMAN MALES
To understand the role of reproductive maturation in the development of reproductive behavior in human males, its important to separate various strands, including reproductive maturation, pubertal development, and somatic growth. Reproductive maturation involves maturation of the hypothalamic-pituitary testicular axis. This starts with gonadarche or the beginning of testicular growth and the onset of spermatogenesis and testosterone production by the testes, which plays some role in libido. Pubertal maturation, or the development of secondary sexual characteristics as a consequence of increasing testosterone levels, represents the outward appearance of reproductive maturation and plays a role in changing social status.
Both reproductive maturation and pubertal maturation are necessary conditions for adult maturation common to mammalian species. Somatic growth, on the other hand, is not essential to the beginning of reproduction; in fact, in many species reproductive maturation begins when somatic growth has reached its nadir. In contrast, for humans somatic growth during adolescence includes a reacceleration of growth in both weight and height that has been slowing down ever since infancy, suggesting that the pubertal growth spurt may be a specifically human adaptive feature (Bogin, 1999).
However, more recent work suggests that other primate species exhibit a similar growth spurt (Leigh, 1998) in weight, especially among sexually dimorphic species. Chimp males, for instance, show important gains in weight during reproductive maturation (Leigh and Shea, 1996). Thus, the distinctive element of the adolescent growth spurt in humans is not increased growth per se but, more specifically, the dramatic increases in stature. This is easily attributable to our bipedality, which makes height a more salient dimension of size.
Unfortunately, definitive fossil evidence for the origins of the adolescent growth spurt in height during human evolution is not available. Current speculations focus on Homo ergaster when increases in size and loss of sexual dimorphism suggest important changes in the timing of maturation (Hawkes, 2002). However, interpretation of the almost complete skeleton of the Nariokotome boy at 1.8MYA does not provide conclusive evidence for the existence of a growth spurt during this time period. Smith (1993) argues that the relationship between dental and postcranial developmental timing demonstrated by the specimen is at variance with that seen in modern humans, while Clegg and Aiello (1999) argue that it is, in fact, within the range of variation found in modern humans. Thus, the temporal origin of the human growth spurt remains an open issue.
In addition to the pubertal growth spurt, the existence of adrenarche as part of human maturation deserves attention as a distinctive clue to the human life course. Again, adrenarche is not uniquely a human attribute but rather one we share with other great apes (Collins et al., 1981; Smail et al., 1982) that may take on a distinctively human pattern. Importantly, adrenarche follows the completion of brain growth around the age of 7 and precedes the onset of puberty (Bogin, 1999), suggesting that it plays some role between the energetic demands of brain growth and those of puberty.
While adrenarche was once thought to be directly related to the onset of puberty, there is no evidence for an obligate role of adrenarche in the onset of pubertal maturation, and adrenarche and gonadarche are considered functionally separate processes. Instead it appears that adrenarche may be more directly related to processes of brain maturation. DHEA/S has an impact on Gamma-aminobutyric acid (GABA(a)) neurons (Majewska, 1995) that act to inhibit dopaminergic neurons in the prefrontal cortex that regulate judgment and the control of behavioral impulses (Niehoff, 1999). In addition, the impact of DHEA/S on GABA(a) neurons in the hypothalamus may act to inhibit GnRH production and retard the onset of puberty (Genazzani et al., 2000). Thus, while adrenarche and gonadarche are fundamentally separate processes, they are not unrelated, a point that comes home when we consider the role of energetics in reproductive maturation.
ENERGETICS AND MALE REPRODUCTIVE MATURATION
For males, increases in stature and muscle mass come well after the onset of testicular growth and the onset of spermatogenesis (Tanner, 1978). This is in clear contrast to human females for whom menarche, the first clear sign of reproductive function, occurs after peak height velocity. At the broadest level, this difference in the relative timing of reproductive maturation and somatic growth between the sexes can be explained in terms of energetics. Reproduction is energetically costly in females and so reproductive function matures only after the energetic demands of growth are nearly finished. In contrast, the low relatively energetic cost of sperm production in males means that its early onset does not detract from further growth in stature and muscle mass (Ellison, 2001).
This energetic perspective can be applied to understanding the process of reproductive maturation in males as well. As mentioned earlier, previous attempts to link adrenarche and pubertal onset have focused on the potential role of adrenarche in the onset of pubertal maturation. Increases in adrenal androgens were thought to desensitize the hypothamalus to negative feedback from gonadal steroids thus allowing gonadal production to rise and initiating puberty (Grumbach et al., 1974; see Figure 9-2A). However, it is now clear that adrenarche is not necessary for pubertal maturation (Counts et al., 1987), and the idea of adrenarche as a specific event that causes the onset of pubertal maturation has been largely discarded (Parker, 1999).
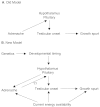
FIGURE 9-2
Causal relationship of adrenarche and pubertal maturation. NOTE: The old model suggests that adrenal androgens produced by adrenarche are necessary for desensitizing the hypothalamus to the feedback effects of gonadal androgens thus allowing testicular (more...)
Nonetheless, adrenarche and gonadarche may be related by a third factor on which both depend—energetic status, as suggested by the model presented in Figure 9-2B. In this model the timing of adrenarche and gonadarche is the product of two separate processes: maturation of the hypothalamus and pituitary and maturation of the adrenal gland and the testes. Hypothalamic and pituitary maturation appears to be a largely internal process reflecting more general developmental processes extending back to the womb. More importantly, maturation of the hypothalamus and pituitary appears to precede maturation of the adrenal gland and testes, suggesting that variation in the timing of adrenarche and gonadarche is largely dependent on development of adrenal gland and gonadals.
In fact, the onset of adrenal and gonadal androgen production has been tied to variations in energetic availability, specifically fat stores. Recent evidence indicates that the timing of adrenarche is associated with maximum increases in body mass index (Remer, 2000; Remer and Manz, 1999), while the timing of pubertal onset—that is, testicular growth—is directly related to fat stores (Vizmanos and Marti-Henneberg, 2000). Thus, greater food availability would lead to earlier onset of adrenarche as well as an earlier onset of puberty and a correlation of testosterone and DHEA/S levels without any direct relationship between the two processes.
Once testosterone production has begun, pubertal development and somatic growth are related to both increasing testicular production of testosterone and energetic availability through the effects of blood glucose, insulin, and activity, as shown in Figure 9-2B. Continued increases in DHEA/S contribute to the development of somatic growth as well, though their role appears to be negligible compared to that of testosterone. However, in populations where energetic constraints lead to lowered testosterone levels, the contribution of DHEA/S to the progression of pubertal maturation may increase.
EVOLUTIONARY EXPLANATIONS OF HUMAN REPRODUCTIVE MATURATION
The model of energetics and pubertal timing outlined above suggests that, in the case of humans, energetic constraints on the simultaneous development of the brain and reproductive maturation acted as the primary selective force in the development of the maturation process beginning with adrenarche and marked by continuing increases in DHEA/S. It is difficult to be sure when changes in human life history evolved. In the scenario suggested here, changes in life history patterns associated increased size, slowed childhood growth, and changes in the relative metabolic costs of the gut versus the brain would have started with the origins of Homo ergaster (Aiello and Wells, 2002). Initially, the increased metabolic demands of the brain may have been met by an increase in caloric intake, involving dietary shift, changes in relative size of the gut, and slower childhood growth.
With further increases in brain size subsequent to Homo ergaster full development of a larger brain would have required additional energy and time. In order to allow for continued brain development without engendering the energetic costs of reproductive maturation, increases in DHEA/S production would have promoted development of the prefrontal cortex, while suppressing GnRH production and the onset of pubertal maturation. At younger ages, reproductive suppression may have been complete, creating a juvenile phase in which the brain could develop without the competing demands of reproductive maturation. However, continued increases in DHEA/S would allow for further brain maturation even as reproductive maturation and sexual behavior commenced, thus allowing the integration of sexual impulses and their behavioral expression.
BEHAVIORAL IMPLICATIONS
Bogin (1994) has argued that the adolescent growth spurt in human males reflects the results of male-male competition. He suggests that, once males exhibit the somatic cues of maturity, they enter into a competitive world of adult males that inevitably carries costs, including the risk of injury. Thus it would be beneficial to delay development of full secondary sexual characteristics, including growth, until one is as competitive as possible so that the benefits of engaging in male-male competition are more likely to outweigh the costs. Bercovitch and Ziegler (2002) make a similar argument for primates.
Among orangutans the presence of adult males does directly suppress the development of secondary sexual characteristics in developing males without suppressing reproductive function (Maggioncalda et al., 2002), suggesting that the presence of older males is a critical social signal of reproductive opportunities. There is no evidence for such reproductive suppression in humans and the role of male-male competition during adolescence seems secondary to the role of energetic factors. However, the fact that the presence/absence of the father during early childhood has been linked to hormonal profiles (Flinn et al., 1996) suggests that male parental care may play a role as a social cue about later reproductive opportunities (see section on Cultural Variation).
Nonetheless, male-male competition would make adolescence a stressful time. In addition to a potential role in suppressing the onset of reproductive maturation, increased DHEA/S production during reproductive maturation may have been selected for because of its protective role in the stress response. Under conditions of stress such as those associated with major life transitions (Dorn and Chrousos, 1997), elevated cortisol is associated with anxiety (Frankenhaeuser, 1980), which may in turn interfere with social interaction and learning. As Fredrickson (2000) has put it, activation of the hypothalamic-pituitary-adrenol (HPA) axis may be associated with execution of narrow behavioral programs mediated by the limbic system. In contrast, DHEA/S has been associated with improved mood among HIV-positive men (Cruess et al., 1999) and improved mood and cognition in aging men (van Niekerk et al., 2001). Furthermore Boudarene and Legos (2002) report that low anxiety levels are associated with increased levels of DHEA/S during cognitive stress tests.
Importantly, DHEA/S is a cortisol antagonist, meaning that it acts to block the effects of cortisol on neurons in the brain (Kimonides et al., 1999; Yoo et al., 1996). Given these actions of cortisol and DHEA on the hippocampus for learning and memory (McEwen, 1999), increased levels of DHEA would alleviate the effects of cortisol in narrowing concentration (Stansbury and Gunnar, 1994) and lead to increased inputs to the hippocampus from the cortex allowing for greater possibility of flexible behavior and increased learning.
In the case of adolescent males for whom increased testosterone levels are associated with increases in sexual behavior (Halpern et al., 1998), learning may be very much focused on interactions with the opposite sex as well as competitive interactions with other males (Weisfield, 1999). Increasing levels of testosterone can be thought of as making such interactions increasingly salient and promoting impulses toward sex and aggression. Cortisol and DHEA/S may be thought of as having contrasting effects on the execution of these impulses. Increased levels of DHEA/S would promote their expression and relate them to a wider arrange of social inputs through its effects on the prefrontal cortex. Cortisol, on the other hand, would interfere with the broader associations of such behavior by blocking the formation of new memories by the hippocampus (see Figure 9-3 for a diagrammatic representation).
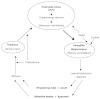
FIGURE 9-3
Schematic representation of the impact of gonadal and adrenal hormones on reproductive strategies. NOTE: This diagram, loosely based on Ledoux (1998) and Zuckerman (1995) suggests that testosterone, DHEA/S, and cortisol all play different roles in brain (more...)
In more human terms, Weisfield (1999) has argued that adolescence represents a time in which social emotions become associated with sexual behavior and the social relationships that go with them. In particular he argues that pride and shame have important roles as males start to judge achievement in terms of their ability to attract mates. If, as Damassio (1999) argues, emotions involve the cognitive appraisal by the cortex of emotional impulses generated by the limbic system, testosterone would potentiate the original impulses involved in attracting and competing for mates. DHEA/S would act to expand these impulses by promoting neural pathways that incorporate the positive judgment of social factors that lead to pride while cortisol would tend to lead to the negative awareness of social factors contained in shame. Such a process might also underlie the creativity that Miller (2000) argues is the basis of courtship in human males.
So far, I have argued, on largely theoretical grounds, that the existence of adrenarche in humans gives us special reason to expand our understanding of the processes involved in human reproductive maturation beyond simply the development of sexual behavior. The next section follows through on this argument by considering empirical evidence for gonadal and adrenal hormones in the sexual behavior of adolescent boys. We start with findings on the role of pubertal maturation and testosterone in the onset of sexual behavior and then move to evidence that both cortisol and DHEA/S may have a role in aspects of social behavior important for the full expression of sexual behavior.
HORMONES AND ADOLESCENT SEXUAL BEHAVIOR
Udry has developed a biosocial model of the determinants of sexual behavior among adolescent boys (Udry et al., 1985; Udry and Billy, 1987). In its original formulation the model argued that increasing levels of testosterone during puberty will result in increasing levels of endogenous sexual motivation in boys. To the extent that sexual motivation is not socially controlled, it will result in the onset of sexual behavior in boys. And compared to girls, it appears that there are relatively few social controls on boys.
PUBERTAL MATURATION
The role of testosterone in pubertal maturation remains hard to pin down. Halpern (1997) was able to demonstrate a direct relationship between salivary testosterone and sexual behavior in adolescent boys, though an earlier analysis found that secondary sexual characteristics, not serum testosterone, was the more important predictor (Halpern et al., 1993). Differentiating behavior and physical effects of testosterone is difficult because testosterone levels rise quite quickly in individual boys (Nielsen et al., 1986). Thus, changes in motivation associated with testosterone stimulation of the brain may happen in the absence of social opportunities for sexual behavior.
Fortunately, the distinction between direct and indirect effects of testosterone may not be crucial to understanding the role of pubertal maturation in the onset of sexual behavior. To the extent that increases in testosterone are important to development of the nervous system and secondary sexual characteristics, their effects will be synchronized, and a general measure of pubertal timing may be sufficient to represent the effects of testosterone.
RISK TAKING
A model of adolescent sexual behavior based entirely on the biological effects of pubertal maturation and the role of testosterone is, however, clearly insufficient to capture the process of sexual initiation. Sexual behavior includes an important element of social behavior, including risk taking. This is most apparent in early adolescence when sexual behavior is not a normative behavior and is associated with socially proscribed risk-taking behaviors such as smoking, drinking, and stealing (Mott and Haurin, 1988; Rosenthal et al., 1999; Tapert et al., 2001; Tubman et al., 1996; Wagner, 2001). Even later in adolescence sexual behavior generally involves initiation on the part of the male, which may be considered a form of risk taking.
Though testosterone may play a role in sensation-seeking and impulsivity (Virkkunen et al., 1994), risk taking is more closely related to adrenal hormones, particularly cortisol. Cortisol has been shown to be inversely related to novelty seeking in Vietnam veterans with posttraumatic stress disorder (Wang et al., 1997) and sensation seeking in college-age men (Rosenblitt et al., 2001). In addition, increased cortisol reactivity has been associated with social inhibition and anxiety among adolescents (Granger et al., 1994) and internalizing symptoms (Klimes-Dougan et al., 2001). In contrast, boys with conduct disorder exhibit low cortisol on average (McBurnett et al., 2000).
There is less evidence demonstrating how the role of cortisol in behavioral inhibition (or lack of it) may be related to sexual behavior. However, Halpern et al. (2002) have shown that cortisol reactivity is inversely related to the number of sex partners and frequency of condom use in a sample of 18- to 25-year old males. In addition, cortisol reactivity was also related to substance use, suggesting that individual differences in the HPA axis may underlie the relationship between various aspects of risk taking in adolescent boys.
BIOLOGICAL MODEL: INTEGRATING PUBERTAL MATURATION AND RISK TAKING
Given the evidence outlined above, pubertal maturation and risk taking can be conceived of as two separate dimensions of adolescent sexual behavior, associated with gonadal and adrenal steroids, respectively. Pubertal maturation is associated with increases in testosterone that are related to the development of secondary sexual characteristics and the onset of sexual motivation. As such it is related to sexual opportunities.
Risk taking, on the other hand, is associated with socially problematic behavior. As such it may be related to taking advantage of sexual opportunities when such behavior is not normative. Risk taking is related to cortisol, and low cortisol is related to acting on impulse. However, in contrast to testosterone, cortisol levels do not change dramatically at puberty (Walker et al., 2001) and represent a stable individual characteristic preceding puberty.
In the model proposed here, the two dimensions of pubertal maturation and risk taking come together to produce sexual behavior with cortisol modifying the expression of developing sexual impulses produced by increasing testosterone levels, as shown in Figure 9-4.
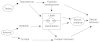
FIGURE 9-4
Conceptual model of biological dimension of adolescent sexual behavior. NOTE: This figure proposes a two-dimensional model of biological influences on the onset of sexual behavior among adolescent males. Increasing levels of testosterone are known to (more...)
IMPLICATIONS FOR ADULT FERTILITY BEHAVIOR
Timing of reproductive maturation by itself seems unlikely to play a major role in fertility behavior in males. In many societies reproduction does not start until the mid-20s long after reproductive maturation is complete and late maturers have had a chance to catch up with early maturers in terms of the impact of testosterone and its behavioral and somatic effects. For instance, early maturers start the pubertal growth spurt earlier and go through it with greater intensity; they are not taller than late maturers at the end of puberty (Bourguignon, 1988; Vizmanos et al., 2001). Thus, although height has been related to male fertility (Pawlowski et al., 2000) as well as sperm count (Manning et al., 1998), variation in height is not established at puberty.
Instead, pubertal timing in males is likely a proxy for individual variation in the activity of the hypothalamic-pituitary-testicular axis established early in development and persisting into adulthood (Bribiescas, 2001). Variations in testosterone levels as well as production rates in Western populations are quite heritable (Handelsman, 1997; Meikle et al., 1997), suggesting that individual differences in testosterone and its effects on behavior go back at least to prenatal development. For instance, testosterone has been related to aggression in both preschool boys (Sanchez-Martin et al., 2000) and prepubertal adolescent males (Gerra et al., 1998). Scerbo and Kolko (1994) report that testosterone was related to aggression in a sample of disruptive prepubertal children, although Constantino et al. (1993) did not find elevated testosterone and aggression in 18 highly aggressive prepubertal boys compared to normal controls.
Such a developmental model may help explain recent findings of genetic influences on the timing of first intercourse among adolescent boys (Miller et al., 1999; Rodgers et al., 1999). Rodgers et al.'s findings fail to account for the effects of pubertal timing, which presumably has genetic elements in boys, as it does in girls (Campbell and Udry, 1995). On the other hand, Miller et al.'s findings that variation in genes for dopamine receptor type helps account for variation in age at first sexual intercourse in a sample of non-Hispanic European American men and women, even controlling for a variety of psychosocial factors, is compatible with individual variation in risk taking, given evidence for dopamine in impulsivity among adolescents with attention-deficit disorder with hyperactivity (Rosa Neto et al., 2002).
However, Rodger et al.'s genetic findings hold for European American males, but not females and not African American males, suggesting an important interaction of genetic factors with the social context in which behavior is expressed. We will come back to this point in our discussion of cultural variation in the development of male fertility behavior.
The fact that both testosterone (Handelsman, 1997; Meikle et al., 1997) and cortisol (Meikle et al., 1988) levels are heritable suggests that individual variation in these two hormones may play a role in the sexual behavior of adult males, just as they do in adolescents. In the case of testosterone, levels decline gradually with age, suggesting that they may underlie age-related declines in sexual behavior. At the same time, cortisol levels change little with age, suggesting a relatively fixed effect of individual variation. However, sexual behavior in adults depends much more heavily on the development of long-term relationships, in which the role of libido and risk taking in sexual behavior may be very different and may lead to changes in the observable effects of testosterone and cortisol.
In fact, individual variation in testosterone does appear to be related to the maintenance of sexual relationships and marriage. For instance, Bogaert and Fischer (1995) reported a small positive effect of testosterone on the number of sex partners among young adult men, even controlling for psychological variables such as disinhibition. Furthermore, several studies have documented lower testosterone levels in married men compared to unmarried men (Booth and Dabbs, 1993; Booth and Osgood, 1993; Gray et al., 2002). Finally, testosterone increases around the time of divorce and then declines, suggesting that it may respond to increased competition associated with marital dissolution (Mazur and Michalek, 1998).
At a more mechanistic level, testosterone has demonstrable effects on sexual awareness and arousal in adult males (Alexander and Sherwin, 1991; Anderson et al., 1992). At the same time, recent experimental work using exogenous testosterone provides little evidence to sustain an important effect of testosterone levels on the frequency of sexual behavior among adult men (Bhasin et al., 2001; Buena et al., 1993). Anderson et al. reconcile this difference by pointing to the possibly constraining influence of sex partners. Unfortunately, similar evidence for the role of cortisol in male sexual or social behavior is lacking.
CULTURAL VARIATION
Draper and Harpending (1982) have suggested that variation in social structure has an important impact on the sexual and parenting behaviors of human males. They argue that so-called father-absent societies would produce males who would tend to put their energy into mating effort over paternal investment in offspring, while males in father-present societies put their energy into parental investment over mating effort.
Given that males in father-absent societies grow up to be absent fathers, Draper and Harpending's argument might seem almost tautological. However, the important question is whether there is a biological mechanism translating variation in early childhood experience into predictable behavioral predispositions underlying mating in adulthood. Belsky et al. (1991) offered one such mechanism in the case of girls; internalizing behavior associated with early stress would lead to increased eating, hasten age at reproductive maturation, and lead to a greater number of sexual partners. The data do not bear out this mechanism (Belsky et al., 1991; Campbell and Udry, 1995).
However, another potential mechanism relating early experience to adult behavior in boys is the impact of father absence on the development of the adrenal axis (Flinn et al., 1996). In humans, several studies have shown that cortisol reactivity in infancy is related to attachment (Gunnar et al., 1996; Hertsgaard et al., 1995). Furthermore, there are sex differences in cortisol reactivity from infancy (Davis and Emory, 1995), and the effects of childhood trauma appear to increase cortisol responsiveness in women (Heim et al., 2002) while leading to decreased cortisol levels among males (Dawes et al., 1999; Moss et al., 1999).
The lack of a father in the household may result in disruption of maternal care and hence poorer attachment and subsequent changes in adrenal reactivity. In the case of boys, early childhood stress associated with poor maternal care and physical abuse is more likely to lead to low cortisol levels in some samples of boys (Dawes et al., 1999; Moss et al., 1999), which in turn is related to the acting out of problem behavior.
Flinn et al. (1996) also found that boys from father-absent homes exhibited lower cortisol levels in a sample from Bwa Mawego, a village on the Island of Dominica, suggesting the cross-cultural generalizability of such effects. In fact, because fetal undernutrition results in elevated cortisol levels (Clark et al., 1996; Levitt et al., 2000; Phillips et al., 1998, 2000; Reynolds et al., 2001), the effects of father absence in food-limited populations may act through both prenatal development and early childhood attachment, thus multiplying its impact on cortisol reactivity.
In addition to cortisol, DHEA/S may be involved in the externalizing behavior of adolescent boys. For instance, Dmitrieva et al. (2001) reported higher levels of DHEA/S among a group of 10- to 18-year old boys with conduct disorder compared to normal controls. In addition, Van Goozen et al (1998) report that prepubertal boys with conduct disorder have higher levels of DHEA/S but not testosterone compared to normal controls. I speculate that among boys who experience poor attachment and early physical abuse, prepubertal increases in DHEA/S, a weak androgen, combined with a lack of inhibition associated with low cortisol levels, may be sufficient to lead to sexual behavior in social settings without strong social controls on adolescent males.
SUMMARY
This chapter began by arguing that an evolutionary perspective directs us to consider the fertility behavior of men because selective forces on males, as the sex with less direct energetic parental investment, may be particularly strong. Yet at the same time human males clearly do invest in offspring postnatally, adding an additional level of complexity to the way in which selection has shaped the reproductive biology and behavior of human males from that of our primate ancestors.
My description of reproductive maturation in humans as stretching from adrenarche to young adulthood suggests a distinct human version of male reproductive maturation. Changes in behavior needed for successful reproduction span this entire period, beginning with changes in cognition and social relationships during the prepubertal period, continuing with reproductive maturation during adolescence and the onset of sexual behavior, and ending in the onset of reproduction and parenting during the young adult phase.
This extended definition of reproductive maturation in human males highlights the role of both the testicular and adrenal axis in male reproductive behavior. Sufficient evidence exists to argue that pubertal maturation in males is part of individual variation in the reproductive axis that persists into adulthood and underlies individual continuity in sexual behavior. In the same way, cortisol is a marker of the adrenal axis, which underlies individual continuity in social behavior. However, the role of adrenal hormones in the onset of sexual behavior is only beginning to receive attention. While the association of cortisol and risk taking suggests a role for cortisol in the expression of sexual behavior, at this point only the slimmest of data substantiate the link.
There is even less empirical evidence demonstrating that DHEA/S is related to variation in adolescent sexual behavior, yet DHEA/S potentially is involved in a variety of mechanisms that may affect sexual behavior. On the one hand, conversion of DHEA/S to testosterone means that it may augment the essential role of gonadally produced testosterone in the maturation of the reproductive system, including promoting libido and somatic growth, both of which have been related to adolescent sexual behavior. In addition, DHEA/S and testosterone have been shown to have similar effects on gene expression in immune cells (Maurer et al., 2001). On the other hand, conversion of DHEA/S to estrogen means that it may play a suppressive effect in reproductive maturation but promote aspects of cognitive function related to the forebrain (Keenan et al., 2001).
In addition to its potential role as an androgen or estrogen, DHEA/S may play a role in adolescent behavior through more direct mechanisms. These include its role in stimulating GABA(A) neurons, with effects on precortical control of behavior. In addition, DHEA/S's antiglucocorticoid effects suggest a potentially important role for DHEA/S in memory development and the ability to produce novel behavior patterns in response to familiar stimuli, under conditions of arousal. The very fact that DHEA/S levels rise during maturation in humans represents an important evolutionary feature of the human life cycle that may shed light on the role of brain development and attachment in humans as well as fertility behavior more generally.
FUTURE RESEARCH DIRECTIONS
To more fully understand the implications of adrenarche for the development of boys, I suggest three important lines of investigation. The first of these is a better understanding of the interaction of DHEA/S and cortisol with testosterone in the disruption of normal emotional development in boys. The second is the study of cross-population differences in age-related patterns in adrenal and gonadal steroids and the insight they may provide into maturational timing. The third is a more detailed investigation of the role of adrenal and gonadal steroids on behavioral development in chimpanzees and gorillas as a background for understanding the truly human aspects of male reproductive maturation.
There is already sufficient evidence for the effects of cortisol and DHEA on major depressive disorder in adolescence to warrant further investigation (Goodyer et al., 2001). What the perspective offered here adds is a functional understanding that for adolescent boys mood dysregulation reflects not only the shifting patterns of psychosocial adjustment during this period but also the role of testosterone in producing the underlying motivations for attachment. DHEA/S, cortisol, and testosterone are only some of the many factors involved in this process, but they can help provide insight into the specific aspects of brain functioning that fail to develop normally in these conditions.
Researchers will also want to investigate the impact of variations in maturational timing across populations on the development of emotions and behavior. One very important issue in understanding the interaction of gonadal and adrenal steroids on adolescent maturation among boys is the role of DHEA/S in mediating the effects of testosterone on sexual behavior. If because of its relationship to brain growth, the timing of adrenarche is less variable than that of gonadarche (Worthman, 1993), individuals in early-maturing populations such as our own may be exposed to the maturational effects of DHEA/S for a much shorter time before being exposed to the sexually motivating effects of testosterone. Faster rates of maturation among adolescent boys may simply provide less time for the effects of DHEA/S on the brain and hence less time to integrate attachment and emotional development with sexual behavior.
The final line of investigation involves comparison with our most closely related species, great apes, in order to more fully understand the relationship of adrenarche and gonadarche in reproductive maturation specifically in humans, given their large brains. While adrenarche has been demonstrated in both chimpanzees and gorillas (Smail et al., 1982; Collins et al., 1981), and DHEA/S has not demonstrated a relationship to reproductive behavior (Nadler et al., 1987), we have very little information on the timing and tempo of increases in DHEA/S and its relationship to the development of reproductive behavior. It is only by comparing such patterns from great apes with those from humans that we will be able to understand the contribution of adrenal steroids to a uniquely human reproductive maturation.
REFERENCES
- Aiello LC, Wells JCK. Energetics and the evolution of the genus Homo. Annual Review of Anthropology. 2002;31:323–338.
- Alexander GM, Sherwin BB. The association between testosterone, sexual arousal and selective attention for erotic stimuli in men. Hormones and Behavior. 1991;25(3):367–381. [PubMed: 1937428]
- Anderson MJ, Dixon AF. Sperm competition: Motility and the midpiece in primates. Nature. 2002;416(6880):496. [PubMed: 11932733]
- Anderson RA, Bancroft J, Wu FC. The effects of exogenous testosterone on sexuality and mood of normal men. Journal of Clinical Endocrinology and Metabolism. 1992;75(6):1503–1507. [PubMed: 1464655]
- Argquitt AB, Stoecker BJ, Hermann JS, Winterfeldt EA. Dehydroepiandrosterone sulfate, cholesterol, hemoglobin, and anthropometric measures related to growth in male adolescents. Journal of the American Dietetic Association. 1991;91(5):575–579. [PubMed: 1826915]
- Baker RR, Bellis MA. Number of sperm in human ejaculates varies in accordance with sperm competition theory. Animal Behavior. 1989;37:867–869.
- Bateman AJ. Intrasexual selection in Drosophila. Heredity. 1948;2:349–368. [PubMed: 18103134]
- Baulieu EE. Neurosteroids: A novel function of the brain. Psychoneuroendocrinology. 1998;23(8):963–987. [PubMed: 9924747]
- Bee H. The Developing Child. 8th ed. New York: Addison-Wesley; 1997.
- Belsky J, Steinberg L, Draper P. Childhood experience, interpersonal development and reproductive strategy: An evolutionary theory of socialization. Child Development. 1991;62(4):647–670. [PubMed: 1935336]
- Bercovitch FB, Ziegler TE. Current topics in primate socioendocrinology. Annual Review of Anthropology. 2002;31:1–23.
- Berg SJ, Wynne-Edwards KE. Changes in testosterone, cortisol, and estradiol levels in men becoming fathers. Mayo Clinic Proceedings. 2001;76:582–592. [PubMed: 11393496]
- Bledsoe C, Lerner S, Guyer JI. Fertility and the Male Life Cycle in the Era of Fertility Decline. New York: Oxford University Press; 2000.
- Bhasin S, Woodhouse L, Casaburi R, Singh AB, Bhasin D, Berman N, Chen X, Yarasheski KE, Magliano L, Dzekov C, Dzekov J, Broos R, Phillips J, Sinha-Hikim I, Shen R, Storer TW. Testosterone dose-response relationships in healthy young men. American Journal of Physiological Endocrinology and Metabolism. 2001;281(6):E1172–E1181. [PubMed: 11701431]
- Bogaert AF, Fischer WA. Predictors of university men's number of sexual partners. Journal of Sex Research. 1995;32:119–130.
- Bogin B. Adolescence in evolutionary perspective. Acta Paediatrica. 1994;406(Suppl.):29–35. [PubMed: 7734808]
- Bogin B. Patterns of Human Growth. 2nd ed. Cambridge University Press; 1999.
- Bogin B. The Growth of Humanity. New York: Wiley-Liss; 2001.
- Booth A, Dabbs J. Testosterone and men's marriages. Social Forces. 1993;72(2):463–477.
- Booth A, Osgood DW. The influence of testosterone in deviance in adulthood: Assessing and explaining the relationship. Criminology. 1993;31:93–117.
- Boudarene M, Legos JJ. Study of the stress response: Role of anxiety, cortisol and DHEA/S. Encephale. 2002;28(2):139–146. [PubMed: 11972140]
- Bourguignon JP. Variations in duration of pubertal growth: A mechanism compensating for differences in the timing of puberty and minimizing their effects on final height. Belgian Study Group for Paediatric Endocrinology. Acta Paediatrica Scandavia. 1988;347(Suppl.):16–24. [PubMed: 3254030]
- Bribiescas RG. Reproductive ecology and the life history of the human male. American Journal of Physical Anthropology. 2001;33(Suppl.):148–176. [PubMed: 11786994]
- Buena F, Swerdloff RS, Steiner BS, Lutchmansigh P, Peterson MA, Pandian MR, Galmarini M, Bhasin S. Sexual function does not change when serum testosterone levels are pharmacologically varied within the normal male range. Fertility and Sterility. 1993;59:1118–1123. [PubMed: 8486184]
- Campbell BC, Udry JR. Stress and age at menarche of mothers and daughters. Journal of Biosocial Science. 1995;27(2):127–134. [PubMed: 7738076]
- Cabrera MS, Vogiatzi MG, New MI. Long term outcome in adult males with classic congenital adrenal hyperplasia. Journal of Clinical Endocrinology and Metabolism. 2001;86(7):3070–3078. [PubMed: 11443169]
- Clark PM, Hindmarsh PC, Shiell AW, Law CM, Honour JW, Barker DJ. Size at birth and adrenocortical function in childhood. Clinical Endocrinology. 1996;45(6):721–726. [PubMed: 9039338]
- Clegg M, Aiello LC. A comparison of the nariokotome Homo Erectus with juveniles from a modern population. American Journal of Physical Anthropology. 1999;110(1):81–93. [PubMed: 10490470]
- Collins DC, Nadler RD, Preedy JRK. Adrenarche in the great apes (Abstract). American journal of Primatology. 1981;1:344.
- Constantino JN, Grosz D, Saenger P, Chandler DW, Nandi R, Earls FJ. Testosterone and aggression in children. Journal of the American Academy of Child and Adolescent Psychiatry. 1993;32(6):1217–1222. [PubMed: 8282667]
- Cruess DG, Antoni MH, Kumar M, Ironson G, McCabe P, Fernandez JB, Fletcher M, Schneiderman N. Cognitive-behavioral stress management buffer decreases in dehydroepiandrosterone sulfate (DHEA-S) and increases in the cortisol/DHEA-S ratio and reduces mood disturbance and perceived stress among HIV-seropositive men. Psychoneuroendocrinology. 1999;24(5):537–549. [PubMed: 10378240]
- Counts DR, Pescovitz OH, Barnes KM, Hench KD, Chrousos GP, Sherins RJ, Comite F, Loriaux DL, Cutler GB Jr. Dissociation of adrenarche and gonadarche in precocious puberty and in isolated hypogonadotropic hypogonadism. Journal of Clinical Endocrinology and Metabolism. 1987;64(6):1174–1178. [PubMed: 3571422]
- Damassio AR. The Feeling of What Happens. New York: Harcourt Brace; 1999.
- Davis M, Emory E. Sex differences in neonatal stress reactivity. Child Development. 1995;66(1):14–27. [PubMed: 7497822]
- Dawes MA, Dorn LD, Moss HB, Yao JK, Kirisci L, Ammerman RT, Tarter RE. Hormonal and behavioral homeostasis in boys at risk for substance abuse. Drug and Alcohol Dependency. 1999;55(1-2):165–176. [PubMed: 10402161]
- Dixson AF. Primate Sexuality: Comparative Studies of the Prosimians, Monkeys, Apes, and Human Beings. New York: Oxford University Press; 1998.
- Dmitrieva TN, Oades RD, Hauffa BP, Eggers C. Dehydroepiandrosterone sulphate and corticotropin levels are high in young male patients with conduct disorder: Comparisons for growth factors, thyroid and gonadal hormones. Neuropsychobiology. 2001;43:134–140. [PubMed: 11287791]
- Dorn LD, Chrousos GP. The neurobiology of stress: Understanding regulation of affect during female biological transitions. Seminars in Reproductive Endocrinology. 1997;15:19–35. [PubMed: 9065975]
- Dorn LD, Hitt SF, Rotenstein D. Biopsychological and cognitive differences in children with premature vs. on-time adrenarche. Archives of Pediatric and Adolescent Medicine. 1999;153(2):137–146. [PubMed: 9988243]
- Draper P, Harpending HC. Father absence and reproductive strategy: An evolutionary perspective. Journal of Anthropological Research. 1982;38:255–273.
- Eberhard WG. Sexual Selection and Animal Genitalia. Cambridge, MA: Harvard University Press; 1985.
- Ellison PT. On Fertile Ground: A Natural History of Human Reproduction. Cambridge, MA: Harvard University Press; 2001.
- Erlandsson R, Wilson JF, Paabo S. Sex chromosomal transposable element accumulation and male-driven substitutional evolution in humans. Molecular Biology and Evolution. 2000;17:804–812. [PubMed: 10779541]
- Flinn MV, Guinlan RJ, Decker SA, Turner MT, England BG. Male-female differences in effects of parental absence on glucocorticoid stress response. Human Nature. 1996;7(2):125–162. [PubMed: 24203317]
- Frankenhaeuser M. Psychobiological aspects of life stress. In: Levin S, Ursin H, editors. Coping and Health. New York: Plenum Press; 1980. pp. 203–223.
- Fredrickson BL. Cultivating positive emotions to optimize health and well-being. Prevention and Treatment. 2000;3(March)
- Genazzani AR, Bernardi F, Montetone P, Luisi S, Laurie M. Neuropeptides, neurotransmitters, neurosteroids and the onset of puberty. Annals of the New York Academy of Sciences. 2000;900:1–9. [PubMed: 10818386]
- Gerra G, Zaimovic A, Giucastro G, Folli F, Maestri D, Tessoni A, Avznzini P, Caccavari R, Bernasconi S, Brambilla F. Neurotransmitter-hormonal responses to psychological stress in peripubertal subjects: Relationship to aggressive behavior. Life Sciences. 1998;62:617–625. [PubMed: 9472721]
- Goodyer IM, Herbert J, Altham PME, Pearson J, Secher SM, Shiers HM. Adrenal secretion during major depression in 8-to 16-year olds. I. Altered diurnal rhythms in salivary cortisol and dehydroepiandrosterone (DHEA) at presentation. Psychological Medicine. 1996;26:245–256. [PubMed: 8685281]
- Goodyer IM, Park RJ, Netherton CM, Herbert J. Possible role of cortisol and dehydroepiandrosterone in human development and psychopathology. British Journal of Psychiatry. 2001;179:243–249. [PubMed: 11532802]
- Granger DA, Weisz JR, Kauneckis D. Neuroendocrine reactivity, internalizing behavior problems, and control-related cognitions in clinic-referred children and adolescents. Journal of Abnormal Psychology. 1994;103:267–276. [PubMed: 8040496]
- Gray PB, Kahlenbery SM, Barrett ES, Lipson SF, Ellison PT. Marriage and fatherhood are associated with lower testosterone in males. Evolution and Human Behavior. 2002;23:193–201.
- Grumbach MM, Roth JC, Kaplan SL, Kelch RP. Hypothalamic-pituitary regulation of puberty in man: Evidence and concepts derived from clinical research. In: Grumbach MM, Grave GD, Fayer FE, editors. Control of the Onset of Puberty. New York: Wiley; 1974. pp. 115–166.
- Gunnar MR, Brodersen L, Nachmias M, Buss K, Rigatuso J. Stress reactivity and attachment security. Developmental Psychobiology. 1996;29(3):191–204. [PubMed: 8666128]
- Halpern CT, Campbell BC, Agnew CR, Thompson V, Udry JR. Associations between stress reactivity and sexual and non-sexual risk taking in young adult human males. Hormones and Behavior. 2002;42:387–398. [PubMed: 12488106]
- Halpern CT, Udry JR, Campbell B, Suchindran C. Testosterone and pubertal development as predictors of sexual activity: A panel analysis of adoloescent males. Psychosomatic Medicine. 1993;55:436–447. [PubMed: 8265746]
- Halpern CT, Udry JR, Suchindran C. Monthly measures of salivary testosterone predict sexual activity in adolescent males. Archives of Sexual Behavior. 1998;27(5):445–465. [PubMed: 9795726]
- Handelsman DJ. Estimating familial and genetic contributions to variability in human testicular function: A pilot twin study. International Journal of Andrology. 1997;20:215–221. [PubMed: 9401824]
- Harcourt AH, Harvey PH, Larson SG, Short RV. Testis weight, body weight and breeding system in primates. Nature. 1981;293(5827):55–57. [PubMed: 7266658]
- Hawkes K. Grandmothers and the evolution of human longevity. Paper presented at the Human Biology Association Meeting; Buffalo, NY. April 2002.
- Heim C, Newport DJ, Wagner D, Wilcox MM, Miller AH, Nemeroff CB. The role of early adverse experience and adulthood stress in the prediction of neuroendocrine stress reactivity in women: A multiple regression analysis. Depression and Anxiety. 2002;15(3):117–125. [PubMed: 12001180]
- Herdt G, McClintock M. The magical age of 10. Archives of Sexual Behavior. 2000;29(6):587–606. [PubMed: 11100264]
- Hertsgaard L, Gunnar M, Erickson MF, Nachmias M. Adrenocortical responses to the strange situation in infants with disorganized/disoriented attachment relationships. Child Development. 1995;66(4):1100–1106. [PubMed: 7671652]
- Huttley GA, Jakobsen LB, Wilson SR, Easteal S. How important in DNA replication for mutagenesis? Molecular Biology and Evolution. 2000;17(6):929–937. [PubMed: 10833199]
- Keenan PA, Ezzat WH, Ginsburg K, Moore GJ. Prefrontal cortex as the site of estrogen's effect on cognition. Psychoneuroendocrinology. 2001;26(6):577–590. [PubMed: 11403979]
- Kimonides VG, Spillantini MG, Sofroniew MW, Fawcett JW, Herbert J. Dehydroepiandrosterone (DHEA) antagonizes the neurotoxic effects of corticosterone and translocation of stress-activated protein kinase 3 in hippocampal primary cultures. Neuroscience. 1999;89(2):429–436. [PubMed: 10077325]
- Klimes-Dougan B, Hastings PD, Granger DA, Usher BA, Zahn-Waxler C. Adrenocortical activity in at-risk and normally developing adolescents: Individual differences in salivary cortisol basal levels, diurnal variation, and responses to social challenges. Developmental Psychopathology. 2001;13:695–719. [PubMed: 11523855]
- Kurtis JD, Mtalib R, Onyango FK, Duffy PE. Human resistance to Plasmodium falciparum increases during puberty and is predicted by dehydroepiandrosterone sulfate levels. Infection and Immunity. 2001;69(1):123–128. [PMC free article: PMC97863] [PubMed: 11119497]
- Labrie F, Belanger A, Simard J, Luu-The Van, Labrie C. DHEA and peripheral androgen and estrogen formation: Intracinology. Annals of the New York Academy of Sciences. 1995;774:16–28. [PubMed: 8597456]
- Lancaster J, Lancaster CS. The watershed: Change in parental investment and family-formation strategies in the course of human evolution. In: Lancaster JB, Altman J, Rossi AS, Sherrod LR, editors. ParentingAacross the Lifespan: Biosocial Dimension. New York: Aldine de Gruyter; 1987. pp. 187–206.
- Ledoux JE. The Emotional Brain: The Mysterious Underpinnings of Emotional Life. London: Weidenfeld and Nicolson; 1998.
- Leigh SR. Evolution of human growth spurts. American Journal of Physical Anthropology. 1998;101(1):455–474. [PubMed: 9016361]
- Leigh SR, Shea BT. Ontogeny of body size variation in African apes. American Journal of Physical Anthropology. 1996;99(1):48–65. [PubMed: 8928723]
- Levitt NS, Lambert EV, Woods D, Hales CN, Andrew R, Seckl JR. Impaired glucose tolerance and elevated blood pressure in low birth weight, nonobese, young South African adults: Early programming of the cortisol axis. Journal of Clinical Endocrinology and Metabolism. 2000;85(12):4611–4618. [PubMed: 11134116]
- Maggioncalda AN, Czekala NM, Sapolsky RM. Male orangutan subadulthood: A new twist on the relationship between chronic stress and developmental arrest. American Journal of Physical Anthropology. 2002;118(1):25–32. [PubMed: 11953942]
- Majewska MD. Neuronal actions of dehydroepiandrosterone. Possible role in brain development, aging, memory and affect. Annals of the New York Academy of Sciences. 1995;774:111–120. [PubMed: 8597451]
- Manning JT, Scutt D, Wilson J, Lewis-Jones DI. The ratio of 2nd to 4th digit length: A predictor of sperm numbers and concentrations of testosterone, luteinizing hormone and oestrogen. Human Reproduction. 1998;13(11):3000–3004. [PubMed: 9853845]
- Maurer M, Trajanoski Z, Frey G, Hiroi N, Galon J, Willenberg HS, Gold PW, Chrousos GR, Scherbaum WA, Bornstein SR. Differential gene expression profile of glucocorticoids, testosterone, and dehydroepiandrosterone in human cells. Hormone and Metabolic Research. 2001;33:691–695. [PubMed: 11753752]
- Mazur A, Michalek J. Marriage, divorce and male testosterone. Social Forces. 1998;77:315–330.
- McBurnett K, Lahey BB, Rathouz PJ, Loeber R. Low salivary cortisol and persistent aggression in boys referred for disruptive behavior. Archives of General Psychiatry. 2000;57(1):38–43. [PubMed: 10632231]
- McEwen BS. Stress and the aging hippocampus. Frontiers in Neuroendocrinology. 1999;20:49–70. [PubMed: 9882536]
- Meikle AW, Stringham JD, Woodward MG, Bishop DT. Heritability of plasma cortisol levels. Metabolism. 1988;37(6):514–517. [PubMed: 2967419]
- Meikle AW, Stephenson RA, Lewis CM, Wiebke GA, Middleton RG. Age, genetic and nongenetic factors influencing variation in serum sex steroids and zonal volume of the prostate and benign prostate hyperplasia in twins. Prostate. 1997;33:105–111. [PubMed: 9316651]
- Miller G. The Mating Mind: How Sexual Choice Shaped the Evolution of Human Nature. New York: Anchor Books; 2000.
- Miller WB, Pasta DJ, MacMurray J, Chiu C, Wu H, Comings DE. Dopamine receptor genes are associated with age at first sexual intercourse. Journal of Biosocial Science. 1999;31(1):43–54. [PubMed: 10081236]
- Moss HB, Vanyukov M, Yao JK, Kirillova GP. Salivary cortisol responses in prepubertal boys: The effects of parental substance abuse and association with drug use during adolescence. Biological Psychiatry. 1999;45(10):1293–1299. [PubMed: 10349035]
- Mott FL, Haurin RJ. Linkages between sexual activity and alcohol and drug use among American adolescents. Family Planning Perspectives. 1988;20(3):128–136. [PubMed: 3417003]
- Muhlenbein MP, Campbell BC, Murchison MA, Phillipi KM. Morphological and hormonal parameters in two species of Macaques: Impact of seasonal breeding. American Journal of Physical Anthropology. 2002;117:218–227. [PubMed: 11842401]
- Nadler RD, Wallis J, Roth-Meyer C, Cooper RW, Baulieu EE. Hormones and behavior of prepubertal and peripubertal chimpanzees. Hormones and Behavior. 1987;21(1):118–131. [PubMed: 2951315]
- Nass R, Baker S, Sadler AE, Sidtis JJ. The effects of precocious adrenarche on cognition and hemispheric specialization. Brain and Cognition. 1990;14(1):59–69. [PubMed: 2223045]
- Nelson K. Memory development from 4 to 7 years. In: Sameroff AJ, Haith MM, editors. The Five to Seven Year Shift. Chicago: University of Chicago Press; 1996. pp. 141–160.
- Niehoff D. The Biology of Violence: How Understanding the Brain, Behavior and Environment Can Break the Vicious Circle of Aggression. New York: The Free Press; 1999.
- Nielsen CT, Skakkebaek NE, Darling JA, Hunter WM, Richarson DW, Jorgensen M, Keiding N. Longitudinal study of testosterone and luteinizing hormone (LH) in relation to spermarche, pubic hair, height and sitting height in normal boys. Acta Endocrinologica Supplement (Copenhagen). 1986;279:98–106. [PubMed: 3465183]
- Orentreich N, Brind JL, Vogelman JH, Andres R, Baldwin H. Long-term longitudinal measures of plasma dehydroepiandrosterone sulfate in normal men. Journal of Clinical Endocrinology and Metabolism. 1992;75(4):1002–1014. [PubMed: 1400863]
- Parker CR Jr. Dehydroepiandrosterone and dehydroepiandrosterone sulfate production in the human adrenal during development and aging. Steroids. 1999;64(9):640–647. [PubMed: 10503722]
- Pawlowski B, Dunbar RI, Lipowicz A. Tall men have more reproductive success. Nature. 2000;403(6766):156. [PubMed: 10646589]
- Phillips DIW, Barker DJP, Fall CHD, Seckl JR, Whorwood CB, Wood PJ, Walker BR. Elevated plasma cortisol concentrations: A link between low birthweight and the insulin resistance syndrome? Journal of Clinical Endocrinology and Metabolism. 1998;83:757–760. [PubMed: 9506721]
- Phillips DI, Walker RB, Reynolds RM, Flanagan DE, Wood PJ, Osmond C, Barker DJ, Whorwood CB. Low birth weight predicts elevated plasma cortisol concentrations in adults from 3 populations. Hypertension. 2000;35:1301–1306. [PubMed: 10856281]
- Reynolds RM, Walker BR, Syddall HE, Andrew R, Wood PJ, Whorwood CB, Phillips DI. Altered control of cortisol secretion in adult men with low birth weight and cardiovascular risk factors. Journal of Clinical Endocrinology and Metabolism. 2001;86(1):245–250. [PubMed: 11232008]
- Remer T. Adrenarche and nutritional status. Journal of Pediatric Endocrinology and Metabolism. 2000;13(Suppl. 5):1253–1255. [PubMed: 11117664]
- Remer T, Manz F. Role of nutritional status in the regulation of adrenarche. Journal of Clinical Endocrinology and Metabolism. 1999;84(11):3936–3944. [PubMed: 10566631]
- Robel P, Baulieu EE. Dehydroepiandrosterone (DHEA) is a neuroactive steroid. Annals of the New York Academy of Sciences. 1995;774:82–110. [PubMed: 8597486]
- Rodgers JL, Rowe DC, Buster M. Nature, nurture and first sexual intercourse in the USA: Fitting behavioral genetic models to NLSY kinship data. Journal of Biosocial Science. 1999;31(1):29–41. [PubMed: 10081235]
- Rosenblitt JC, Soler H, Johnson SE, Quadango DM. Sensation-seeking and hormones in men and women: Exploring the link. Hormones and Behavior. 2001;40:396–402. [PubMed: 11673912]
- Rosenthal DA, Smith AMA, deVisser R. Personal and social factors influencing age at first sexual intercourse. Archives of Sexual Behavior. 1999;28:319–333. [PubMed: 10553493]
- Rosa Neto P, Lou H, Cumming P, Pryds O, Gjedde A. Methylphenidate-evoked potentiation of extracellular dopamine in the brain of adolescents with premature birth: Correlation with attentional deficit. Annals of the New York Academy of Sciences. 2002;965:434–439. [PubMed: 12105118]
- Sanchez-Martin JR, Fano E, Ahedo L, Cardas J, Brain PF, Azpiroz A. Relating testosterone levels and free play social behavior in male-female preschool children. Psychoneuroendocrinology. 2000;25(8):773–783. [PubMed: 10996473]
- Scerbo AS, Kolko DJ. Salivary testosterone and cortisol in disruptive children: Relationship to aggressive, hyperactive and internalizing behaviors. Journal of the American Academy of Child and Adolescent Psychiatry. 1994;33(8):1174–1184. [PubMed: 7982868]
- Smail PJ, Faiman C, Hobson WC, Fuller GB, Winter JD. Further studies on adrenarche in non-human primates. Endocrinology. 1982;111:844–848. [PubMed: 6213402]
- Small MF. Female primate behavior and conception: Are there enough sperm to spare? Current Anthropology. 1988;29(1):81–100.
- Smith BH. Physiological age of KMN-WT 1500 and its significance for growth and development of early Homo. In: Walker AC, Leakey RF, editors. The Nariokotome Homo Erectus Skeleton. Cambridge MA: Belknap, Harvard University Press; 1993. pp. 195–220.
- Smith RL. Human sperm competition. In: Smith RL, editor. Sperm Competition and the Evolution of Animal Matings Systems. New York: Academic Press; 1984. pp. 601–659.
- Stansbury K, Gunnar MR. Adrenocortical activity and emotion regulation. Fox NA, editor. The Development of Emotion Regulation: Biological and Behavioral Considerations. 1994;59(240):108–134. Monographs of the Society for Research in Child Development. [PubMed: 7984156]
- Stikkelbroeck NM, Otten BJ, Pasic A, Jager GJ, Sweep CG, Noordam K, Hermus AR. High prevalence of testicular adrenal rest tumors, impaired spermatogenesis, and Leydig cell failure in adolescent and adult males with congenital adrenal hyperplasia. Journal of Clinical Endocrinology and Metabolism. 2001;86(12):5721–5728. [PubMed: 11739428]
- Storey AE, Walsh CJ, Quinton RL, Wynne-Edwards KE. Hormonal correlates of paternal responsiveness in new and expectant fathers. Evolution and Human Behavior. 2000;21(2):79–95. [PubMed: 10785345]
- Tanner JM. Fetus in Man: Physical Growth from Conception to Maturity. Cambridge MA: Harvard University Press; 1978.
- Tapert SF, Aarons GA, Sedlar GR, Brown SA. Adolescent substance use and sexual risk-taking. Journal of Adolescent Health. 2001;28(3):181–189. [PubMed: 11226840]
- Tubman JG, Windle M, Windle RC. Cumulative sexual intercourse patterns among middle adolescents: Problem behavior precursors and concurrent health risk behaviors. Journal of Adolescent Health. 1996;18(3):182–191. [PubMed: 8777194]
- Udry JR, Billy JOG. Initiation of coitus in early adolescence. American Sociological Review. 1987;52(6):841–855.
- Udry JR, Billy JOG, Morris NM, Groff T, Raj M. Serum androgenic hormones motivate sexual behavior in adolescent boys. Fertility and Sterility. 1985;43:90–94. [PubMed: 4038388]
- Van Goozen SH, Matthys W, Cohen-Kettenis PT, Thijssen JH, van Engeland H. Adrenal androgens and aggression in conduct disorder prepubertal boys and normal controls. Biological Psychiatry. 1998;43(2):156–158. [PubMed: 9474448]
- Van Niekerk JK, Huppert FA, Herbert J. Salivary cortisol and DHEA: Association with measures of cognition and well-being in normal older men, and effects of three months of DHEA supplementation. Psychoneuroendocrinology. 2001;26(6):591–612. [PubMed: 11403980]
- Virkkunen M, Kallio E, Rawlings R, Tokola R, Poland RE, Guidotti A, Nemeroff C, Bissette G, Kalogeras K, Karonen SL, Limmoila M. Personality profiles and state aggressiveness in Finnish alcoholic, violent offenders, fire setters, and healthy volunteers. Archives of General Psychiatry. 1994;51:28–33. [PubMed: 7506516]
- Vizmanos B, Marti-Henneberg C. Puberty begins with a characteristic body fat mass in each sex. European Journal of Clinical Nutrition. 2000;54:203–208. [PubMed: 10713741]
- Vizmanos B, Marti-Henneberg C, Cliville R, Moreno A, Fernandez-Ballart J. Age of pubertal onset affects the intensity and duration of pubertal growth peak but not final height. American Journal of Human Biology. 2001;13(3):409–416. [PubMed: 11460907]
- Vogel F, Ratherberg R. Spontaneous mutations in humans. In: Harris H, Hirschhord K, editors. Advances in Human Genetics. New York: Plenum Press; 1975. pp. 5–223.
- Wagner MK. Behavioral characteristics related to substance abuse and risk-taking, sensation-seeking, anxiety sensitivity, and self-reinforcement. Addictive Behavior. 2001;26(1):115–120. [PubMed: 11196285]
- Walker EF, Walder DJ, Reynolds F. Developmental changes in cortisol secretion in normal and at-risk youth. Developmental Psychopathology. 2001;13(3):721–732. [PubMed: 11523856]
- Wang S, Mason J, Charney D, Yehuda R, Riney S, Southwick S. Relationships between hormonal profile and novelty seeking in combat-related posttraumatic stress disorder. Biological Psychiatry. 1997;41(2):145–151. [PubMed: 9018384]
- Weisfeld G. Evolutionary Principles of Human Adolescence. New York: Basic Books; 1999.
- Worthman CM. Biocultural interactions in human development. In: Pereira ME, Fairbanks LA, editors. Juvenile Primates: Life History, Development and Behavior. New York: Oxford University Press; 1993. pp. 339–358.
- Worthman CM. The epidemiology of human development. In: Worthman CM, Panter-Brick C, editors. Hormones, Health and Behavior. Cambridge: Cambridge University Press; 1999. pp. 47–104.
- Wyckoff GJ, Wang W, Wu CI. Rapid evolution of male reproductive genes in the descent of man. Nature. 2000;403(6767):304–309. [PubMed: 10659848]
- Yoo A, Harris J, Dubrovsky B. Dose-response study of dehydroepiandrosterone sulfate on dentate gyrus long-term potentiation. Experimental Neurology. 1996;137(1):151–156. [PubMed: 8566207]
- Zemel BS, Katz SH. The contribution of adrenal and gonadal steroids to the growth in the height of adolescent males. American Journal of Physical Anthropology. 1986;71(4):459–466. [PubMed: 2949623]
- Zuckerman M. Good and bad humors: Biochemical bases of personality and its disorders. Psychological Science. 1995;6:325–332.
- THE SCOPE OF MALE REPRODUCTIVE MATURATION
- BIOLOGY OF REPRODUCTIVE MATURATION IN HUMAN MALES
- ENERGETICS AND MALE REPRODUCTIVE MATURATION
- EVOLUTIONARY EXPLANATIONS OF HUMAN REPRODUCTIVE MATURATION
- BEHAVIORAL IMPLICATIONS
- HORMONES AND ADOLESCENT SEXUAL BEHAVIOR
- PUBERTAL MATURATION
- RISK TAKING
- BIOLOGICAL MODEL: INTEGRATING PUBERTAL MATURATION AND RISK TAKING
- IMPLICATIONS FOR ADULT FERTILITY BEHAVIOR
- CULTURAL VARIATION
- SUMMARY
- FUTURE RESEARCH DIRECTIONS
- REFERENCES
- Pubertal Maturation, Adrenarche, and the Onset of Reproduction in Human Males - ...Pubertal Maturation, Adrenarche, and the Onset of Reproduction in Human Males - Offspring
Your browsing activity is empty.
Activity recording is turned off.
See more...