The content of this book is licensed under a Creative Commons Attribution-NonCommercial-NoDerivs 4.0 Unported license. To view the terms and conditions of this license, visit https://creativecommons.org/licenses/by-nc-nd/4.0/
NCBI Bookshelf. A service of the National Library of Medicine, National Institutes of Health.
Varki A, Cummings RD, Esko JD, et al., editors. Essentials of Glycobiology [Internet]. 4th edition. Cold Spring Harbor (NY): Cold Spring Harbor Laboratory Press; 2022. doi: 10.1101/glycobiology.4e.47
Altered glycosylation is a universal feature of cancer cells, and certain glycans are well-known markers of tumor progression. This chapter discusses glycan biosynthetic pathways that are altered in cancer cells; correlations between altered glycosylation, diagnosis, and clinical prognosis; the genetic bases of some of these changes; and the functional role of glycans in cancer biology and pathogenesis.
HISTORICAL BACKGROUND
The earliest evidence of altered glycosylation as a hallmark of cancer was that some plant lectins show enhanced binding and agglutination of tumor cells. Next, it was found that transformation of cultured cells is frequently accompanied by a general increase in the size of glycopeptides of cell-surface glycoproteins. With the advent of monoclonal antibody technology, many “tumor-specific” antibodies directed against glycan epitopes were found. In many instances, these epitopes represented “oncofetal antigens”—that is, glycan epitopes expressed on tumor cells and embryonic tissues. Like normal cells during embryogenesis, tumor cells also undergo rapid growth and invade tissues. Correlations between certain types of altered glycosylation and the prognosis of tumor-bearing animals or patients increased interest in glycan changes. In vitro cellular assays and in vivo animal studies have now supported the conclusion that glycan changes are critical to several aspects of tumor cell behavior.
GLYCOSYLATION CHANGES IN CANCER ARE NONRANDOM
Glycan changes in malignant cells take a variety of forms: loss of expression or excessive expression of certain glycans, increased expression of incomplete or truncated glycans, and, less commonly, the appearance of novel glycans. However, this is not simply the random consequence of disordered biosynthesis in tumor cells. It is striking that a very limited subset of changes are correlated with malignant transformation and tumor progression, highlighting a potential functional role in tumor biology. Given that cancer is a “microevolutionary” process in which only the fittest cells survive, and that tumors are under immune surveillance pressure, it is likely that these specific glycan changes are selected for during tumor progression.
ALTERED BRANCHING AND FUCOSYLATION OF N-GLYCANS
Classic reports of increased size of tumor cell glycopeptides have now been partly explained by an increase in β1-6 branching of N-glycans (Figure 47.1), resulting from enhanced expression of N-acetylglucosaminyltransferase V (GnT-V, MGAT5) (Chapter 9). Increased transcription of the MGAT5 gene is induced by various oncogenic transcription factors, as well as viral- and chemical-induced carcinogenesis. Cells with up-regulated MGAT5 show an increased frequency of metastasis in mice, and spontaneous revertants lacking MGAT5 lose the metastatic phenotype. Overexpression of MGAT5 in cultured cells causes a transformed phenotype, whereas Mgat5-deficient mice show a striking reduction in the growth and metastasis of mammary tumors induced by a viral oncogene. Increased expression of MGAT4 to form a β1-4 branched tetra-antennary N-glycan also enhances tumor progression. Possible mechanisms by which increased N-glycan branching and extension enhances progression include lattice formation via galectin binding to poly-N-acetyllactosamines (LacNAc) leading to prolonged growth factor signaling, and the generation of sialyl-Lewis x (SLex) tetrasaccharides recognized by selectins because of increased outer-chain sialylation and fucosylation (Figure 47.1). Increased expression of FUT8 that transfers the core Fuc to N-glycans (Figure 47.1) is also observed in solid tumors and promotes tumor progression in lung cancer and melanoma. The expression of N-acetylglucosaminyltransferase III (GnT-III, MGAT3) that catalyzes the transfer of β1-4GlcNAc to Man on N-glycans to form the bisecting GlcNAc (Figure 47.1) is up-regulated in rat hepatomas and mouse mammary tumors. However, in this case, tumor progression is suppressed by the presence of N-glycans with the bisecting GlcNAc. Mice lacking MGAT3 show increased mammary tumors and lung metastases, whereas high MGAT3 expression correlates with better relapse-free survival in human breast cancer. However, liver tumors are reduced in mice lacking MGAT3, and MGAT3 activity endows ovarian cancer cells with cancer stem cell features. Thus, the effects of MGAT3 on cancer progression depend on tissue context.
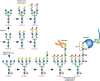
FIGURE 47.1.
N-Glycans increase in size on neoplastic transformation of cells, in part because of increased MGAT4 and MGAT5 activity, which catalyzes GlcNAc branching of N-glycans. This may lead to increased numbers of LacNAc units, which can also be sialylated and (more...)
ALTERED MUCIN EXPRESSION AND TRUNCATED O-GLYCANS
Mucins are large glycoproteins rich in O-GalNAc glycans on Ser or Thr in tandem repeat regions (Chapter 10). In normal polarized epithelium, mucins are expressed in the apical membrane, facing the lumen of a hollow organ, and soluble mucins are secreted exclusively into the lumen. Loss of adhesion junctions and topology in malignant epithelial cells destroys polarization, allowing mucins to enter the extracellular space and the blood. Loss of polarization and overexpression of mucins displaying aberrant glycosylation has made these glycoproteins a major source of biomarkers with clinical applications. The rod-like structure and negative charge of mucins are thought to repel intercellular interactions and sterically inhibit adhesion molecules such as cadherins and integrins from carrying out their functions. Thus, mucins may act as “antiadhesins” to promote displacement of a cell from the primary tumor during the initiation of metastasis. Tumor mucins bearing selectin ligands facilitate several aspects of cancer progression (see below). Mucins might also interfere with immune cell recognition and block or mask presentation of antigenic peptides by major histocompatibility complex (MHC) molecules.
A hallmark feature of carcinomas is the aberrant synthesis of incomplete O-glycans, present in mucins and other glycoproteins, typified by the Tn and T antigens and their sialylated glycoforms, sialyl-Tn (STn) and sialyl-T (ST) (Figure 47.2). Secreted glycoproteins expressing STn often appear in the bloodstream of patients with cancer. Because these glycans occur infrequently in normal tissues, they serve as prognostic biomarkers and therapeutic targets. Truncated O-glycans evoke immune responses in the patient, and accordingly, vaccines against these glycans have been developed. As an alternative, incomplete O-glycans can serve as targets for a variety of therapeutic antibodies and chimeric antigen receptor (CAR) T-cell therapies. The appearance of excessive Tn and STn on tumor cells correlates in some instances with silencing of the COSMC gene (C1GALT1C1). The β1-3 galactosyltransferase (C1GALT1) that synthesizes Galβ1-3GalNAcα1-Ser/Thr requires the chaperone C1GALT1C1 for activity. The gene for C1GALT1C1 is on the X chromosome, thus a single mutation may be sufficient to eliminate expression. In other instances, STn accumulation results from overexpression of the sialyltransferase ST6GALNAC1 (Figure 47.2). The expression of Tn and STn can enhance tumorigenic and invasive properties, and promote immunosuppression.
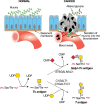
FIGURE 47.2.
Loss of normal topology and polarization of epithelial cells in cancer results in secretion of mucins with truncated O-GalNAc glycans, such as sialyl-Tn (STn) and Tn, into the bloodstream. Tumor cells invading tissues and the bloodstream also present (more...)
ALTERED SIALIC ACID EXPRESSION
Sialylation is generally increased in tumor cells, both on N-glycans and O-glycans. N-glycan sialylation serves as a regulatory mechanism for multiple receptor tyrosine kinases, illustrated by activation of MET and RON receptors by α2-3Sia. Increased α2-6Sia on N-glycans, due to ST6GAL1 up-regulation in cancer cells, enhances integrin-mediated cell motility and protects cells against apoptosis induced by galectins, death receptor ligands, and chemotherapeutic drugs. Additionally, some receptor glycoproteins and their downstream signaling pathways are dysregulated by addition of SLex and sialyl-Lewis a (SLea) structures on either N- or O-glycans. Other functions for tumor cell Sias include binding to factor H to limit complement activation and modulation of tumor cell attachment to matrix, facilitating invasion and metastasis. As well, tumor cell Sias play a prominent role in suppressing antitumor immunity through engagement of inhibitory Siglecs (Chapter 35), which are Sia-recognizing receptors expressed primarily on immune cells. There can also be changes in Sia modifications in tumor cells. Sia 9-O-acetylation can be increased (e.g., 9-O-acetylated GD3 [Figure 47.3] in melanoma cells) or decreased (e.g., on the O-glycans of colon carcinomas). Some tumor cells express small amounts of de-N-acetyl (deNAc) gangliosides (Figure 47.3). O-Acetylation of gangliosides appears to protect tumor cells from apoptosis, and de-N-acetylgangliosides may activate epidermal growth factor receptor (EGFR).
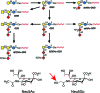
FIGURE 47.3.
Gangliosides expressed in human neuroectodermal tumors. Heavy arrows indicate up-regulated reactions; dashed arrow indicates a potential reaction. O-Acetylation of Sia can occur at the 7- or 9-position. An O-acetyl group at position 7 will migrate to (more...)
Many years ago, cancer patients were reported to express “Hanganutziu–Deicher” antibodies, which were later shown to recognize gangliosides carrying the nonhuman sialic acid N-glycolylneuraminic acid (Neu5Gc), which was also found in human tumors. This unusual phenomenon has now been explained by the metabolic incorporation of diet-derived Neu5Gc into human glycans (Chapter 15). This Sia differs from the common human Sia, Neu5Ac, by addition of a single oxygen atom (Figure 47.3). Humans show variable levels of circulating antibodies against Neu5Gc-containing epitopes. The resulting weak immune response (termed “xenosialitis”) can promote tumor growth by enhancing chronic inflammation and angiogenesis. Given this selective advantage, it is not surprising that tumors are better at accumulating Neu5Gc in the face of an increased immune response. Because the primary dietary source of Neu5Gc is red meat, this may help explain the increased cancer risk associated with its consumption.
INCREASED SELECTIN LIGAND EXPRESSION
SLex and SLea epitopes (Chapter 14) were first identified as tumor antigens on glycosphingolipids. Expression of these antigens by epithelial carcinomas correlates with metastatic potential in mice and with tumor progression, metastatic spread, and poor prognosis in humans. SLex and SLea epitopes on glycoprotein ligands are key recognition determinants of the selectins (Chapter 34). Indeed, selectin ligands are expressed on carcinoma cells, and mucin-like tumor antigens carrying SLex and SLea are found in the blood of carcinoma patients (Figure 47.4). Transgenic overexpression of E-selectin in mouse liver causes carcinoma cells that would normally metastasize to the lung to be redirected toward colonization of the liver, supporting the concept that SLe/selectin interactions are important mediators of metastasis. Furthermore, metastasis is attenuated in mice lacking P-selectin or L-selectin or by administering heparin, which blocks binding by these selectins. Selectin interactions also help explain the classic observation that cancer cells entering the bloodstream form thromboemboli with platelets and leukocytes, which facilitate arrest in the vasculature, assist extravasation through the endothelium, and help in evasion of the immune system. Similar interactions involving soluble cancer mucins may contribute to hypercoagulability (Trousseau's syndrome), a condition responsive to heparin treatment. Because of the prominent role of selectins in cancer progression, these receptors are major therapeutic targets (see below). Certain sialyl-Lewis-related structures may also influence carcinoma progression by interacting with Siglecs, which generally have immunosuppressive functions. For example, disialyl-Lea and sialyl-6-sulfo-Lex structures may protect against early carcinogenic events by binding to Siglec-7 on macrophages. This interaction suppresses macrophage production of the pro-oncogenic inflammatory mediator, Cox2, thereby exerting an anti-oncogenic function. However, during cancer development, many other sialylated glycan ligands for Siglecs are increased, and these bind to inhibitory Siglecs on various immune cell populations to induce immunosuppression and facilitate tumor progression.
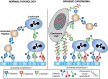
FIGURE 47.4.
In normal physiology platelets, leukocytes, and endothelial cells interact via selectins and selectin ligands. In invasive carcinoma, interactions that promote invasion occur between tumor cells expressing selectin ligands and selectins on endothelial (more...)
ALTERED EXPRESSION OF BLOOD GROUPS
Loss of AB blood group expression in the context of carcinoma (accompanied by exposure of underlying H and Ley epitopes) (Chapter 14) is associated with poor prognosis. The Sda (or Cad) antigen, a blood group glycan abundantly expressed in the colon, is lost in colon carcinoma. Sulfation of the C-3 position of terminal Gal residues is also reduced in cancers. Sialyl-6-sulfo-Lewis x and disialyl-Lewis a, expressed on colonic epithelial cells, are reduced in colon cancer cells. These changes may reflect the enhanced production of SLex and SLea in cancers. DNA methylation and histone deacetylation, epigenetic mechanisms for suppression of gene transcription commonly observed in cancers, are proposed to underlie these glycan alterations. There are also rare instances in which a tumor may present a “forbidden” blood group structure (i.e., expression of a B blood group antigen in an A-positive patient) (Chapter 14). Regardless of the underlying mechanism, tumor regression has been noted in a few such cases, presumably mediated by the naturally occurring endogenous antibodies directed against the foreign structure.
ALTERED EXPRESSION OF GLYCOSPHINGOLIPIDS
Many “tumor-specific” monoclonal antibodies raised against cancer cells recognize the glycan portion of glycosphingolipids. Some glycolipids are highly enriched in specific cancers (e.g., Gb3/CD77 in Burkitt's lymphoma and GM3, GD2, and GD3 in melanomas) (Figure 47.3). Several types of tumors (particularly melanoma and neuroblastoma) are characterized by the synthesis of very high levels of gangliosides (Chapter 11). Some of these (e.g., GD2) are not normally found at high levels in extraneural tissues and are therefore targets for both passive immunotherapy (monoclonal antibody infusion) and active immunotherapy (immunization with purified glycolipids). In some cases, gangliosides are also major carriers of modified Sias (Figure 47.3). Cell culture studies suggest that some gangliosides promote tumor cell growth and invasion. As a principal constituent of lipid raft membrane microdomains, gangliosides modulate cell signaling by numerous receptors. Additionally, gangliosides shed by some tumors appear to have immunosuppressive effects.
LOSS OF GPI-ANCHOR EXPRESSION
A complete loss of glycosylphosphatidylinositol (GPI)-anchored proteins is seen in some cases of malignant and premalignant states involving the hematopoietic system. This results from acquired mutations in hematopoietic stem cells in the PIGA gene (required for an early step in GPI-anchor biosynthesis [Chapter 12]). Conversely, some GPI-anchored proteins, such as members of the carcinoembryonic antigen family, like CEACAM5 (CEA), are overexpressed in cancers of the gastrointestinal tract, lung, breast, and female reproductive system, among others. Members of the CEACAM family have been implicated in tumor biology via interference with the integrin signaling pathway.
CHANGES IN HYALURONAN
Many classes of malignant tumors express high levels of hyaluronan, a large negatively charged polysaccharide composed of the repeating disaccharide unit [GlcAβ1-3GlcNAcβ1-4]n (Chapter 16). In carcinomas, hyaluronan is localized near the tumor cell surface, and is also enriched in tumor-associated stroma. In normal tissues, hyaluronan serves at least three functions, which may also contribute to tumor progression. First, it increases tissue hydration, facilitating cell movement through tissues. Second, it is intrinsic to the assembly of extracellular matrices through specific interactions with other macromolecules, and thus it participates in tumor cell–matrix interactions that facilitate or inhibit tumor cell survival and invasion. Finally, hyaluronan interacts with several types of cell-surface receptors, especially CD44, which mediate or modify cell signaling pathways. These interactions, notably with alternatively spliced isoforms of CD44 that are elevated in most cancer cells, are often crucial to tumor malignancy and are a current target for novel therapies.
In normal adult tissues, hyaluronan appears to be relatively inert with respect to cell signaling and behavior. However, during embryonic development, tissue regeneration, and in various pathologies, hyaluronan-CD44 signaling becomes activated, possibly because of limited cleavage of hyaluronan by hyaluronidases. The consequences of this signaling are dramatic because they promote cell proliferation, survival, epithelial–mesenchymal transition (EMT), and invasion, which are key elements of the malignant phenotype (Figure 47.5). Hyaluronan–CD44 interactions are required for the constitutive activation of some oncogenes, especially receptor tyrosine kinases such as ERBB2, which is amplified or mutated in numerous carcinomas. Accordingly, hyaluronan–CD44 interaction promotes downstream intracellular pathways that are also hallmarks of cancer, such as the phosphatidylinositol 3-kinase (PI3K)/AKT and mitogen-activated protein kinase pathways. Antagonists of hyaluronan–CD44 interaction cause inactivation of these pathways in malignant cells in culture and inhibit tumor growth and metastasis in animal models. Hyaluronan–CD44 interaction also stimulates multidrug resistance, and antagonists sensitize resistant cancer cells to chemotherapeutic drugs. In addition, interactions of hyaluronan with its receptors are important for the activities of several types of metabolic and multidrug transporters. These widespread effects may be due to stabilization of signaling platforms in the plasma membrane (e.g., lipid rafts) that are dependent on multivalent interactions of hyaluronan with CD44 or, in some cases, with another hyaluronan receptor (e.g., LYVE-1 or RHAMM/CD168) (Figure 47.5).
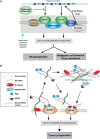
FIGURE 47.5.
Glycosaminoglycans (GAGs) in cancer. (A) Hyaluronan interacts multivalently with CD44, which interacts with several types of signaling molecules in lipid rafts in the plasma membrane, thus promoting cell survival, proliferation, invasion, and epithelial–mesenchymal (more...)
CHANGES IN SULFATED GLYCOSAMINOGLYCANS
Proteoglycans are comprised of core proteins decorated with negatively charged, sulfated glycosaminoglycan (GAG) side chains—namely, chondroitin sulfate (CS), dermatan sulfate (DS), keratan sulfate (KS), and heparan sulfate (HS) (Chapter 17). The content and distribution of many proteoglycans are altered during tumorigenesis, and the structurally and functionally diverse GAGs, particularly HS chains, have been implicated in tumor pathogenesis. HS chains are covalently linked to the core protein of proteoglycans (e.g., syndecans, glypicans, and perlecan). Other proteoglycans such as decorin, biglycan, versican, and lumican, carrying CS, DS, or KS chains, are also present in most tissues, including many tumor types.
Major functions of HS proteoglycans relevant to tumor formation are to promote cell–cell and cell–matrix interactions important in tissue assembly and to bind a wide array of bioactive factors such as fibroblast growth factor-2 (FGF2), vascular endothelial growth factor (VEGF), hepatocyte growth factor, WNT, and numerous other cytokines and chemokines. Thus, HS proteoglycans can serve to build either inhibitory barriers or permissive pathways for cell invasion and can either sequester factors away from their receptors or present them efficaciously to those receptors (Figure 47.5). Syndecan-1 illustrates the multiple important roles of HS, especially in promoting metastasis and angiogenesis. Early work suggested that syndecan-1 helps maintain the normal differentiated state of epithelia and that low levels of tumor-associated syndecan-1 correlate with malignancy. The biological activity of syndecan-1 (and other HS proteoglycans) is influenced by enzymatic processing by proteases and heparanase, an enzyme that cleaves the HS chains at specific sites. High levels of proteolytically released HS-rich ectodomain fragments of syndecan are present in sera of patients with myeloma as well as some types of carcinoma and predict poor prognosis. Syndecan-1 ectodomains also accumulate in the tumor microenvironment, in which they play an important role in activation of both chemokine and growth factor signaling, and consequently tumor cell behavior. Especially significant is the finding that ectodomains promote metastasis to bone tissue, which is characteristic of myeloma and certain carcinomas (e.g., of breast or prostate) in human patients.
HS side chains of several HS proteoglycans are cleaved to fragments containing 10 to 20 sugar moieties by heparanase and endo-β-glucuronidase. Heparanase is elevated in numerous types of cancer, and increased heparanase activity can lead to induction of angiogenesis and metastasis, processes associated with a poor prognosis. HS cleavage promotes blood vessel remodeling required for angiogenesis and release of HS-bound angiogenic factors, growth factors, and chemokines. Furthermore, heparanase products are more bioactive compared with intact HS proteoglycan. Heparanase trimming of syndecan-1 HS chains and up-regulation of matrix metalloproteinase-9 (MMP-9) in myeloma cells results in enhanced shedding of syndecan-1, activating signaling pathways that promote cell migration and angiogenesis. Notably, in addition to their roles in regulating tumor angiogenesis and metastasis, heparanase and syndecans cooperate in regulating extracellular vesicle secretion by tumor cells. The therapeutic targeting of heparanase has potential to not only block tumor growth, but also interfere with the tumor microenvironment.
Along with HS cleavage, modifications in the sulfation pattern of HS, and other GAG chains, are important contributors to tumor biology. GAG sulfation patterns often determine their biological function and serve as specific recognition motifs for a variety of growth factors, cytokines, and chemokines. Many enzymes involved in GAG sulfation are altered in malignant cells. Changes in expression of CS 4-O-sulfotransferases are noted in both ovarian and breast cancers, whereas cancerous lung tissues display elevated 6-O-sulfated CS, when compared to nonmalignant tissue. Additionally, increased expression of 6-O-sulfotransferases in ovarian and colorectal cancers, and 3-O-sulfotransferases in breast and pancreatic cancers, have been reported.
CHANGES IN CYTOPLASMIC AND NUCLEAR O-GlcNAc
O-GlcNAc levels, regulated by the O-GlcNAc transferase OGT and the hexosaminidase OGA, are altered in many cancers. O-GlcNAcylation occurs outside of the secretory pathway and is prevalent on numerous cytosolic, nuclear, and mitochondrial proteins, as well as the intracellular domains of some transmembrane surface proteins. O-GlcNAc cycling serves as a nutrient sensor to regulate signaling, transcription, mitochondrial activity, and cytoskeletal functions. There is often cross-talk between O-GlcNAcylation and phosphorylation, not only at the same or proximal sites on polypeptides, but also through the regulation of enzymes that control cycles of these modifications. O-GlcNAcylation can influence cancer cell metabolism because of alterations in the stability or activity of transcription factors and kinases. O-GlcNAcylation affects phosphofructokinase 1 activity and redirects glucose flux through the pentose phosphate pathway. This metabolic switch can confer a growth advantage on cancer cells. Additionally, O-GlcNAcylation regulates glycolysis in cancer cells via the hypoxia-inducible factor-1α (HIF-1α) transcription factor. Many oncogene and tumor suppressor gene products, including c-Myc, cyclin D1, NF-κB/p65, PFK1, SNAIL, Rb, and p53, are O-GlcNAcylated.
MECHANISMS OF ALTERED GLYCAN EXPRESSION
The Cancer Genome Atlas (TCGA) has identified many alterations in expression of glycosylation genes that correlate with cancers. The mechanistic basis is known in some cases. For instance, the transcription of MGAT5 (Figure 47.1) is induced by v-src, H-ras, and v-fps and the transcription factor ets-1. ST6GAL1 is likewise up-regulated by oncogenic ras isoforms, as well as through gene amplification. The expression of FUT7, which generates SLex in leukocytes, is induced by the transcriptional activator protein Tax of HTLV-1, which causes leukemia. The consequent increase in SLex expression may underlie the strong tissue infiltrative capacity of adult T-cell leukemia cells, likely mediated by selectins. Under the hypoxic conditions found in advanced tumors, hypoxia-resistant cancer cells survive by up-regulating HIF-1α. HIF-1α induces transcription of several glycosylation genes, leading to altered tumor glycans, including enhanced SLex and SLea expression. Tumor hypoxia may also affect the incorporation of diet-derived nonhuman Neu5Gc in human tumor cells by up-regulating transcription of the lysosomal sialin Sia transporter. Down-regulation of glycosylation genes by DNA methylation or histone deacetylation/trimethylation may silence glycosylation genes that appear to suppress tumor progression in some types of cancers, like MGAT3 or ST6GAL1. Posttranscriptional regulation of glycosylation pathways through microRNAs is also involved in tumor progression.
GLYCAN CHANGES IN CANCER STEM CELLS AND DURING EMT
Cancer stem cells, or tumor-initiating cells, constitute a small subpopulation of cancer cells that has tumor-initiating capability. Several glycans that are specific markers for embryonic stem cells (stage-specific embryonic antigen-3 [SSEA-3], SSEA-3 with fucose [Globo H], and SSEA-4) are also expressed by cancer stem cells. SSEA-1, an embryonic stem cell marker in mice, is found in cancer stem cells in human gliomas. Thus the expression of these glycans appears to be associated with the “stemness” of cells. Other cancer stem cell markers include the glycoproteins, CD133 (prominin-1), CD24, and CD44, all of which are regulated by their glycosylation status. Among these receptors, the activation of CD44 by its ligand, hyaluronan, is particularly important for maintaining cancer stem cell features.
Cancer stem cells are often investigated in the context of EMT. Cells that have undergone EMT are highly similar to cancer stem cells. EMT is a critical event in tumor progression that prepares cancer cells for metastasis. It is governed by several well-defined transcription factors such as SNAIL and ZEB. EMT induces the differential expression of a subset of glycosyltransferases. Cancer cells with a mesenchymal phenotype up-regulate ST6GAL1 and MGAT5, while down-regulating MGAT3. The corresponding changes in N-glycan branching and sialylation affect the stability and/or activity of many target molecules central to the process of EMT, such as cadherins and integrins. Other EMT-associated glycan modifications include the GD1 ganglioside, and decreased expression of Gg4 and GM2 glycolipids, along with increases in SLex and SLea. EMT is typified by alterations in cell adhesion and invasiveness; however, marked changes in cellular metabolism also occur, many of which are directed by O-GlcNAcylation. The addition of O-GlcNAc to E-cadherin, Snail, and other EMT-related proteins modulates protein stability or trafficking, leading ultimately to dysregulated expression of metabolic genes.
CLINICAL SIGNIFICANCE
Several glycan antigens are used for detecting and monitoring the growth status of tumors. A classical serological assay for CA19-9, which detects SLea, is used to monitor tumor burden, clinical response to therapy, and disease relapse in patients with gastric, colorectal, and pancreatic cancers. Other serological biomarkers include carcinoembryonic antigen (CEA), a highly glycosylated glycoprotein used in the early detection of recurrent or metastatic colorectal cancer, and CA125, which recognizes MUC16 and is used in monitoring ovarian cancer. In addition, the core-fucosylated α-fetoprotein (AFP-L3) can be a sensitive and specific circulating biomarker for the early diagnosis of hepatocellular carcinoma (HCC).
Truncated O-GalNAc glycans Tn, STn, and T (Figure 47.2) are good markers for the detection of cancer in tumor sections. Furthermore, the detection of specific glycoproteins bearing these truncated O-glycans, such as cancer-specific MUC16 glycoforms, enhances the specificity of diagnosis and monitoring of cancer. In addition, specific glycoproteins modified with truncated glycans, including Tn and STn, have been used as targets to generate glycopeptide-specific antibodies that have shown potential utility in clinical trials. Other important biomarkers include SLex-related glycans, which are used in lung and breast cancer to monitor residual disease after surgery. In recent years, there has been a major interest in glycomic profiling of serum and other body fluid glycoproteins, in addition to glycoproteins in extracellular vesicles. Classic findings of glycan-specific antibodies associated with cancer, such as the antibodies against the nonhuman Sia, Neu5Gc, are also being reinvestigated. Another potential advance lies in improving the specificity of known cancer biomarkers by defining glycoprotein glycoforms uniquely expressed by cancer cells (e.g., prostate-specific antigen).
In tandem with their roles as biomarkers, glycans are important targets for cancer therapeutics. A monoclonal antibody against GD2 has been U.S. Federal Drug Administration (FDA)-approved for the treatment of pediatric neuroblastoma, and other GD2-targeting antibodies, as well as CAR-T cells, are in clinical trials for the treatment of neuroblastoma, glioma, melanoma, and other cancers. Vaccines against GD2 are also being explored. The Tn-bearing form of MUC1 is another promising target, with both therapeutic antibodies and CAR-T cells against Tn-MUC1 under active investigation. Additionally, several selectin inhibitors are showing efficacy in clinical trials, including E-selectin-targeting glycomimetic agents used in the treatment of multiple myeloma and acute myelogenous leukemia. Heparin has long been known to have potent antitumor effects, in part because of its ability to block P- and L-selectin binding to tumor and/or host ligands (Chapter 34). However, heparin therapy for cancer treatment can be limited by its anticoagulant properties, and thus low-molecular-weight heparins or heparinoids that lack such activity may be needed. Low-molecular-weight heparins may also inhibit tumor progression by blocking heparanase activity or by interfering with constitutive HS activities. Other heparanase inhibitors such as the sulfated phosphomannopentaose PI-88, which inhibits angiogenesis and metastasis, may work by blocking selectins or competitively inhibiting HS binding and function. Low-molecular-weight oligosaccharides of hyaluronan could also be useful therapeutically, because they inhibit the pro-oncogenic influences of constitutive polymeric hyaluronan, especially drug resistance and signaling events induced by hyaluronan–CD44 interaction. Disaccharides that can enter the cell and act as decoys to divert glycosylation pathways are also showing promise (Chapter 55).
Recently, there has been growing interest in targeting glycan-dependent molecular interactions to enhance immune checkpoint inhibition. N-glycosylation is important for stabilization of the PD-L1 checkpoint molecule, as well as recognition by PD-1. Thus, therapeutic interference with PD-L1 glycosylation could provide a mechanism for hindering the activation of PD-1 on immune cells. The sialoglycan–Siglec interaction represents another critical immune checkpoint, and function-blocking antibodies against select Siglecs have entered clinical trials. Additionally, methods are being developed to block or ablate tumor cell Sia ligands for Siglecs via administration of sialic acid mimetics or the targeted delivery of sialidases to tumor cell surfaces. Alternatively, Siglecs expressed by hematopoietic cancer cells (e.g., Siglec 2) can serve as recognition molecules for CAR-T cell immunotherapy or delivery of cytotoxic drugs. In general, the leveraging of glycans and glycan-binding proteins to modulate antitumor immunity holds much potential, given the well-known roles of these molecules in regulating the overall immune response.
ACKNOWLEDGMENTS
The authors appreciate contributions from Bryan Toole to the previous version and helpful comments and suggestions from Breeanna Urbanowicz, Melanie Simpson, and Koichi Furukawa to this update.
FURTHER READING
- Feizi T. 1985. Demonstration by monoclonal antibodies that carbohydrate structures of glycoproteins and glycolipids are onco-developmental antigens. Nature 314: 53–57. doi:10.1038/314053a0 [PubMed: 2579340] [CrossRef]
- Borsig L, Stevenson JL, Varki A. 2007. Heparin in cancer: role of selectin interactions. In Cancer-associated thrombosis (ed. Khorana AA, Francis CW, editors. ), pp. 95–111. Taylor-Francis, Boca Raton, FL.
- Kannagi R, Sakuma K, Miyazaki K, Lim KT, Yusa A, Yin J, Izawa M. 2010. Altered expression of glycan genes in cancers induced by epigenetic silencing and tumor hypoxia: clues in the ongoing search for new tumor markers. Cancer Sci 101: 586–593. doi:10.1111/j.1349-7006.2009.01455.x [PubMed: 20085584] [CrossRef]
- Schultz MJ, Swindall AF, Bellis SL. 2012. Regulation of the metastatic cell phenotype by sialylated glycans. Cancer Metastasis Rev 31: 501–518. doi:10.1007/s10555-012-9359-7 [PMC free article: PMC4079276] [PubMed: 22699311] [CrossRef]
- Miwa HE, Koba WR, Fine EJ, Giricz O, Kenny PA, Stanley P. 2013. Bisected, complex N-glycans and galectins in mouse mammary tumor progression and human breast cancer. Glycobiology 23: 1477–1490. doi:10.1093/glycob/cwt075 [PMC free article: PMC3816629] [PubMed: 24037315] [CrossRef]
- Knelson EH, Nee JC, Blobe GC. 2014. Heparan sulfate signaling in cancer. Trends Biochem Sci 39: 277–288. doi:10.1016/j.tibs.2014.03.001 [PMC free article: PMC4065786] [PubMed: 24755488] [CrossRef]
- Ma Z, Vosseller K. 2014. Cancer metabolism and elevated O-GlcNAc in oncogenic signaling. J Biol Chem 289: 34457–34465. doi:10.1074/jbc.r114.577718 [PMC free article: PMC4263853] [PubMed: 25336642] [CrossRef]
- Nabi IR, Shankar J, Dennis JW. 2015. The galectin lattice at a glance. J Cell Sci 128: 2213–2219. doi:10.1242/jcs.151159 [PubMed: 26092931] [CrossRef]
- Pinho SS, Reis CA. 2015. Glycosylation in cancer: mechanisms and clinical implications. Nat Rev Cancer 15: 540–555. doi:10.1038/nrc3982 [PubMed: 26289314] [CrossRef]
- Taniguchi N, Kizuka Y. 2015. Glycans and cancer: role of N-glycans in cancer biomarker, progression and metastasis, and therapeutics. Adv Cancer Res 126: 11–51. doi:10.1016/bs.acr.2014.11.001 [PubMed: 25727145] [CrossRef]
- Alisson-Silva F, Kawanishi K, Varki A. 2017. Human risk of diseases associated with red meat intake: analysis of current theories and proposed role for metabolic incorporation of a non-human sialic acid. Mol Aspects Med 51: 16–30. doi:10.1016/j.mam.2016.07.002 [PMC free article: PMC5035214] [PubMed: 27421909] [CrossRef]
- Groux-Degroote S, Guérardel Y, Delannoy P. 2017. Gangliosides: structures, biosynthesis, analysis, and roles in cancer. ChemBioChem 18: 1146–1154. doi:10.1002/cbic.201600705 [PubMed: 28295942] [CrossRef]
- Pearce OM, Laübli H. 2017. Sialic acids in cancer biology and immunity. Glycobiology 26: 111–128. doi:10.1093/glycob/cwv097 [PubMed: 26518624] [CrossRef]
- Steentoft C, Migliorini D, King TR, Mandel U, June CH, Posey AD Jr. 2018. Glycan-directed CAR-T cells. Glycobiology 28: 656–669. doi:10.1093/glycob/cwy008 [PubMed: 29370379] [CrossRef]
- Furukawa K, Ohmi Y, Ohkawa Y, Bhuiyan RH, Zhang P, Tajima O, Hashimoto N, Hamamura K, Furukawa K. 2019. New era of research on cancer-associated glycosphingolipids. Cancer Sci 110: 1544–1551. doi:10.1111/cas.14005 [PMC free article: PMC6501054] [PubMed: 30895683] [CrossRef]
- Mereiter S, Balmaña M, Campos D, Gomes J, Reis CA. 2019. Glycosylation in the era of cancer-targeted therapy: where are we heading? Cancer Cell 36: 6–16. doi:10.1016/j.ccell.2019.06.006 [PubMed: 31287993] [CrossRef]
- HISTORICAL BACKGROUND
- GLYCOSYLATION CHANGES IN CANCER ARE NONRANDOM
- ALTERED BRANCHING AND FUCOSYLATION OF N-GLYCANS
- ALTERED MUCIN EXPRESSION AND TRUNCATED O-GLYCANS
- ALTERED SIALIC ACID EXPRESSION
- INCREASED SELECTIN LIGAND EXPRESSION
- ALTERED EXPRESSION OF BLOOD GROUPS
- ALTERED EXPRESSION OF GLYCOSPHINGOLIPIDS
- LOSS OF GPI-ANCHOR EXPRESSION
- CHANGES IN HYALURONAN
- CHANGES IN SULFATED GLYCOSAMINOGLYCANS
- CHANGES IN CYTOPLASMIC AND NUCLEAR O-GlcNAc
- MECHANISMS OF ALTERED GLYCAN EXPRESSION
- GLYCAN CHANGES IN CANCER STEM CELLS AND DURING EMT
- CLINICAL SIGNIFICANCE
- ACKNOWLEDGMENTS
- FURTHER READING
- Review Glycosylation Changes in Cancer.[Essentials of Glycobiology. 2015]Review Glycosylation Changes in Cancer.Varki A, Kannagi R, Toole B, Stanley P. Essentials of Glycobiology. 2015
- Review Glycosylation Changes in Cancer.[Essentials of Glycobiology. 2009]Review Glycosylation Changes in Cancer.Varki A, Kannagi R, Toole BP. Essentials of Glycobiology. 2009
- Review Altered glycosylation in cancer: A promising target for biomarkers and therapeutics.[Biochim Biophys Acta Rev Cance...]Review Altered glycosylation in cancer: A promising target for biomarkers and therapeutics.Thomas D, Rathinavel AK, Radhakrishnan P. Biochim Biophys Acta Rev Cancer. 2021 Jan; 1875(1):188464. Epub 2020 Nov 4.
- Review Glycosylation markers in cancer.[Adv Clin Chem. 2019]Review Glycosylation markers in cancer.Silsirivanit A. Adv Clin Chem. 2019; 89:189-213. Epub 2019 Jan 17.
- Review Protein glycosylation in cancers and its potential therapeutic applications in neuroblastoma.[J Hematol Oncol. 2016]Review Protein glycosylation in cancers and its potential therapeutic applications in neuroblastoma.Ho WL, Hsu WM, Huang MC, Kadomatsu K, Nakagawara A. J Hematol Oncol. 2016 Sep 29; 9(1):100. Epub 2016 Sep 29.
- Glycosylation Changes in Cancer - Essentials of GlycobiologyGlycosylation Changes in Cancer - Essentials of Glycobiology
Your browsing activity is empty.
Activity recording is turned off.
See more...