By agreement with the publisher, this book is accessible by the search feature, but cannot be browsed.
NCBI Bookshelf. A service of the National Library of Medicine, National Institutes of Health.
Siegel GJ, Agranoff BW, Albers RW, et al., editors. Basic Neurochemistry: Molecular, Cellular and Medical Aspects. 6th edition. Philadelphia: Lippincott-Raven; 1999.
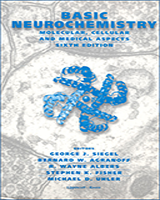
Basic Neurochemistry: Molecular, Cellular and Medical Aspects. 6th edition.
Show detailsBenzodiazepines have revolutionized the treatment of anxiety disorders
The introduction of benzodiazepines has also set the stage for greatly increasing our understanding of the biochemistry of anxiety. Anxiolytic drugs can be considered to be among the earliest effective pharmaceutical agents devised since one of the most prominent effects of ethanol is its tendency to obliterate anxiety, which is a major reason for its continuing popularity. Opiate alkaloids and belladonna derivatives also have long been known to have potent anxiolytic activities, as do barbiturates. Propanediol carbamates, such as meprobamate, were used extensively in the past for the relief of anxiety; their discovery and use was an important step conceptually. The use of each of these drugs is limited by several side effects, most notably their serious toxicity at high doses and, with the exception of belladonna, their high liability for addiction.
The benzodiazepines are highly effective for the relief of anxiety [3–5,18–21]. They have a lower potential for addiction than many other drugs that were used earlier and are less likely to cause death or serious, lasting harm when taken in overdoses. There are now several dozen benzodiazepine drugs in clinical use worldwide, although use has become less popular because of side effects, including dependence. The various compounds appear to differ primarily in their pharmacokinetics, that is, the speed with which they are taken up and eliminated by the body, rather than in differences in their clinical effects.
The clinical effects of the benzodiazepines are fourfold: anxiolytic, sedative or sleep-inducing, anticonvulsant and muscle relaxant. It was thought at first that the benzodiazepines might have a different mechanism of action for each of these effects. For example, it was noted that tolerance to sedation, but not anxiolytic action, quickly develops in patients taking benzodiazepines. While the mechanisms of these differences are not fully understood, all clinical effects of benzodiazepines appear to be mediated centrally.
Receptors for the benzodiazepines relate to their behavioral effects
In 1977, two groups independently discovered high-affinity binding sites for diazepam in the brain. The affinities of a variety of benzodiazepines for these sites correlated well with their clinical potency. Correlations with potency in animal models of anxiety were also sought. The most common animal model for anxiety involves pairing a reward for which the animal must perform some behavior, such as lever pressing, with an aversive stimulus, such as a mild electric shock. A conflict is thus produced. Agents that appear to reduce this conflict and to increase the rate of responses punished with the shock, termed punished-responding agents, generally act as anxiolytics in humans.
Benzodiazepines that were most potent in releasing punished-responding behavior had the highest affinity for benzodiazepine-binding sites. These binding sites were found in highest density in areas of the brain that developed later in evolution, for example, in cerebral cortex, and are thought to be concerned with the production of emotional responses such as anxiety. The interaction of the benzodiazepines with their receptors is quite specific, and benzodiazepines have not demonstrated high affinity for any other neurotransmitter receptors in competitive binding assays. These studies were taken as evidence that it was the binding to these sites that mediated the action of the benzodiazepines [37].
Central nervous system benzodiazepine-binding sites are associated with the GABAA receptor
Much effort has gone into determining the nature of benzodiazepine-binding sites. For some time, it was suspected that benzodiazepine action might be closely associated with GABAergic mechanisms; electrophysiological studies demonstrated that diazepam facilitated GABAergic synaptic transmission. Based on responsiveness to agonist stimulation, GABA receptors were divided into two major classes, GABAA, which is bicuculline-sensitive, and GABAB, which is baclofen-sensitive.
It is now known that the benzodiazepines interact with the GABAA subtype of GABA receptor, which is widely distributed in the CNS, primarily postsynaptically, and which mediates changes in neuronal membrane potential by opening Cl− channels. The GABAA receptor, the benzodiazepine-binding site and the Cl− ionophore are part of a single large macromolecular complex that is believed to be a critical key to the action of the drugs. The receptor has been cloned and consists of several distinct subunits. GABA and the benzodiazepines each allosterically modulate the binding of the other to this macromolecular complex: the benzodiazepines act by binding to the α subunit and GABA by binding to the β subunit (see Chap. 16).
In vitro, Cl− increases benzodiazepine receptor affinity, and it is necessary for the reciprocal regulation of GABA and benzodiazepine binding. Benzodiazepines facilitate GABAergic transmission primarily by increasing the frequency of Cl− channel opening in response to occupancy of the GABAA receptor by GABA. There is also a distinct benzodiazepine-binding site in the periphery that is not associated with GABA receptors.
Barbiturates also mediate at least some of their important actions via binding to some portion of this complex closely associated with the Cl− ionophore (see Chap. 16). Like benzodiazepines, they facilitate GABA-dependent Cl− flux in brain slices and cultured neurons. In addition, barbiturates enhance benzodiazepine and GABA agonist binding to their respective sites in in vitro binding assays; however, the exact mechanism appears to be somewhat different from that of the benzodiazepines. First, barbiturates are not competitive inhibitors of ligands for either GABAA- or benzodiazepine-binding sites. Second, unlike benzodiazepines, barbiturates decrease the frequency of opening of GABA-activated Cl− channels but still potentiate GABA responses by increasing the mean open time of the ionophore.
GABA agonists have anxiolytic effects
The GABA agonist muscimol has anxiolytic activity in animal models, and picrotoxin, a drug that potentially inhibits GABA-promoted Cl−flux, has the opposite effect. The experimental and clinical utility of GABAergic drugs is restricted, however, by their toxicity and in some cases by their limited ability to cross the blood—brain barrier. There is some evidence that ethanol and the propanediol carbamates also produce some of their anxiolytic effects by acting at the GABA—benzodiazepine receptor—Cl− ionophore complex.
Several classes of compounds act as benzodiazepine antagonists
Compounds that act as benzodiazepine antagonists have also been called inverse agonists because they have behavioral actions opposite to those produced by benzodiazepines; that is, they increase sleeplessness and in higher doses cause seizures. The first type of benzodiazepine antagonists described were esters of β-carbolines. These compounds were first studied as possible ligands for the benzodiazepine-binding site. Several candidate endogenous ligands for the receptor, including peptides, have been suggested. The existence and physiological function of these endogenous ligands have yet to be established in humans, but research continues. Inverse agonist drugs have proven medically useful in the reversal of benzodiazepine-induced anesthesia, such as following endoscopic procedures.
Multiple benzodiazepine receptors are involved in differential pharmacological actions
Considerable progress in understanding the structure of the receptors is facilitating the understanding of benzodiazepine actions. Molecular biological investigations of the subunits of the benzodiazepine receptor have revealed that there is substantial heterogeneity. At least five classes of subunits have been identified, which can be coexpressed in varying combinations to create a GABAA receptor (see Chap. 16). The initial classification of benzodiazepine receptors into the BZ-1 form, found throughout the brain with highest concentrations in the cerebellum, and the BZ-2 form, found primarily in the cortex and hippocampus, has now been shown to be determined by different receptor subunit combinations.
Experiments with recombinant receptors have allowed analysis of the various forms with the demonstration of differential affinities of the subunit forms for various ligands. Behavioral and physiological effects of pharmacological agents can be differentiated. The anatomical pattern of gene expression in rat brain has been determined for six forms of the α subunit using in situ hybridization, with dramatic differences in localization observed. Information regarding these different receptor subtypes is being used by chemists to design more specific pharmacological agents, some of which are based upon partial agonist actions.
Neurosteroids may modulate anxiety symptoms
Several steroid hormones are thought of as neurosteroids because they can be synthesized within the CNS by neurons and glial cells (Chap. 49). Neurosteroids seem to modulate neurotransmission both by acting directly on the neuronal membrane and by affecting gene transcription. There is good evidence that, among other actions, neurosteroids modulate DA release from striatal neurons and modulate function of the nicotinic acetylcholine, GABAA and NMDA receptors [38]. Some neurosteroids have excitatory and others inhibitory effects on neuronal activity and behavior. One of the best studied effects of a neurosteroid is the potent facilitation of GABA action at GABAA receptors by 3α,5α-pregnan-3α-ol-20-one (THPROG). This steroid has anesthetic, hypnotic and anxiolytic effects. Many of the neurosteroid hormones are produced both in the brain and in the periphery, by the gonads and adrenal glands, from which they pass easily into the brain. Thus, the action of these steroid hormones may be tied to fluctuations in peripheral hormone and hormone precursor concentrations which occur during pregnancy, across the menstrual cycle and during stress (see Chap. 49). The therapeutic potential of synthetic steroids that may be anxiolytic without sedative effects is under active investigation.
Mechanisms involving catecholamine function may be important in anxiety
An important hypothesis of anxiety mechanisms has centered about brain NE systems, particularly those in the locus ceruleus. The locus ceruleus is the nucleus for 80% of brain noradrenergic neurons. This nucleus projects to multiple brain areas, including the limbic system, hypothalamus and cortex. Activation of the locus ceruleus and the peripheral autonomic nervous system are major components of the normal stress response. Stress increases the turnover of NE in the brain, and this can be inhibited by anti-anxiety agents. The locus ceruleus receives a wide range of inputs and, thus, can integrate information from a variety of sources. There are particularly strong connections between the locus ceruleus and the amygdala, a well-known site of integration of danger stimuli. In addition, multiple neuroregulatory systems, including GABA, opioid peptides, CRH and serotonin, to mention a few, interact at the level of the locus ceruleus.
Evidence linking the locus ceruleus to anxiety is based upon pharmacological as well as physiological studies. Drugs that increase activity in the locus ceruleus increase anxiety, while those that decrease this activity decrease anxiety. In freely moving animals, fear-inducing stimuli produce a rapid increase in the firing of neurons in the locus ceruleus. Many of the somatic signs of anxiety, such as tachycardia, increased blood pressure and shortness of breath, are mediated by the autonomic nervous system. Noradrenergic mechanisms have been related to both panic attacks and post-traumatic stress disorder [39]. Some of the somatic symptoms associated with phobias and panic, for example, can be reversed in humans by behavioral training [40].
Further evidence for the importance of catecholamine systems in the generation of anxiety comes from challenge studies in humans using adrenergic receptor agonists and antagonists. In addition, as described above, antidepressant agents, which are also effective treatment for panic and other anxiety disorders, downregulate β-adrenergic receptors.
Serotonin has been linked to anxiety processes
Aside from the GABA-benzodiazepine receptor complex, no neurotransmitter system has received as much attention in relation to anxiety disorders as has serotonin (5-HT) [41]. It has long been known that depletion of 5-HT with PCPA or lesioning of the dorsal raphe nucleus, the site of most CNS 5-HT neuronal bodies, with 5,7-dihydroxytryptamine produces an anxiolytic-like state in rodents (Chap. 13). It is also known that in some cases serotonergic agonists increase anxiety; however, some 5-HT antagonists tend to change punished-responding behavior in animals or reduce anxiety in humans, whereas others have the opposite effect. Confidence in a serotonergic role in anxiety has also been tempered by the fact that the lesioning procedures produce a number of alterations in neurotransmitter systems other than those utilizing 5-HT. It has long been suspected that many serotonergic drugs are nonspecific, and this has been borne out by the demonstration of multiple distinct CNS 5-HT receptor subtypes. Brain serotonin receptors have been divided into a wide range of subtypes based on their pharmacological specificities, anatomical distribution and function (see Chap. 13). Activation of the 5-HT2 and 5-HT3 postsynaptic receptors seems to produce anxiogenic effects, while activation of 5-HT1a postsynaptic receptors seems to have a more anxiolytic effect. In humans, the 5-HT2 agonist m-chlorophenylpiperazine (mCPP) has been shown to generate anxiety in control subjects and in patients with a variety of anxiety disorders.
The compound buspirone has been introduced as the first anxiolytic agent whose clinical effects are probably mediated primarily by effects on the 5-HT system. Buspirone and related compounds may both alter the treatment of anxiety and offer new tools for its study, just as the benzodiazepines did a generation ago. In contrast to the benzodiazepines, buspirone has a delayed onset of action in that it must be administered for up to several weeks before a significant reduction of anxiety is observed. This delay raises interesting questions as to the mode of action and cascade of effects beyond impacting on the 5-HTla receptor. The drug has almost no sedative, anticonvulsant or muscle-relaxant activity and no significant addiction liability. These last considerations make this type of drug an advance in the treatment of anxiety in many patients. Buspirone is useful in the treatment of generalized anxiety disorder but not in the treatment of panic.
Buspirone has no direct effect on the GABAA—benzodiazepine receptor system and only a weak effect on DA receptors. Several additional 5-HT1A-active compounds also have demonstrated anxiolytic activity in animals. The 5-HT1A receptor is linked via Gi proteins to either inhibition of adenylyl cyclase or the opening of potassium channels (Chap. 20). It is widely distributed as a postsynaptic receptor in the forebrain and serves as a somatodendritic autoreceptor on raphe neurons. Drugs believed to act as partial agonists at 5-HT1a autoreceptors may decrease the firing of the serotonergic raphe neurons.
Important hypotheses of panic disorder and obsessive-compulsive disorder come from findings that serotonin-reuptake inhibitors have proven useful. The finding that a number of drugs that are useful in panic disorder are not useful in generalized anxiety disorder, and vice versa, suggest that the fundamental mechanisms of these processes are different.
Neuropeptides function in anxiety processes
Many neuropeptides and neuropeptide receptor agonists and antagonists have been shown to have anxiogenic or anxiolytic effects when administered centrally to animals. Opiates have long been used for that purpose. CRH, vasopressin, oxytocin, somatostatin, neuropeptide Y, CCK and other peptides have been studied (Chap. 18) [42]. Behavioral effects of these peptides also have been studied using molecular biological techniques, including central administration of antisense sequences that block translation of peptides or peptide receptor proteins, overexpression of peptides in intact animals and generation of knockout mice lacking particular peptides or peptide receptors [43]. Changes in anxiety behaviors have been demonstrated using these techniques with several neuropeptides.
Only a fraction of the neuropeptides that may act as critical neuroregulators have been identified. Some neuropeptides may be coreleased with other neuroregulators and have important transmitter and regulatory roles (see Chap. 18). Changes in CSF concentration of several neuropeptides, particularly CRH and somatostatin, have been noted in patients with anxiety disorders.
An important question is whether there may be new approaches to the understanding and treatment of anxiety in humans using neuropeptide agonists or antagonists. Currently, this approach is limited since peptides cannot be given orally and have limited penetration of the blood—brain barrier. However, nonpeptide agonist and antagonist compounds have the potential to overcome such limitations. Several nonpeptide compounds are currently under active development and should prove useful in further study and possible treatment of anxiety disorders.
Obsessive-compulsive disorder has neurobiological concomitants
The pharmacological therapy of obsessive-compulsive disorder centers on specific serotonin-reuptake inhibitors, which were originally developed as antidepressant agents [18,20]. Most antidepressant drugs are not active in obsessive-compulsive disorder, but those that are show a striking specificity and are of benefit in patients with obsessive-compulsive disorder but not depression. Compared to use of these agents in depression, the effectiveness in obsessional states occurs at higher doses, requires longer treatment and usually results in a lessening of symptoms rather than a total remission. There is frequently a relapse following discontinuation of the drugs.
A particularly useful animal model of obsessive-compulsive behavior may have been found in a naturally occurring disorder in dogs, canine acral lick, involving excessive licking of paws or flanks. The canine problem can produce ulcers and infections. The investigators who found the model have demonstrated in an experimentally controlled manner that serotonin-reuptake blockers are a specific treatment. This model should permit further pharmacological and neurochemical investigation [44]. The finding in humans of a genotype linking low catechol-O-methyltransferase (COMT) to obsessive-compulsive disorder may open another avenue of study [45].
DA antagonists are useful adjunctive treatment agents in a subgroup of patients with obsessive-compulsive disorder. Response to treatment may help to define biological subtypes of patients with obsessive-compulsive disorder.
Another new line of research suggests that childhood-onset cases of obsessive-compulsive disorder may be triggered by an autoimmune reaction to streptococcal infection, in a manner similar to the etiology of Sydenham's chorea, a variant of rheumatic fever. Sydenham's chorea is often accompanied by obsessive-compulsive symptoms. Both patients with childhood-onset obsessive-compulsive disorder and patients with Sydenham's chorea have increased expression of the B-cell marker D8/17, which was previously thought to be associated only with rheumatic fever [46].
Panic disorder has specific biochemical characteristics
Patients with panic disorder have enhanced sensitivity to several challenge agents compared to healthy controls and patients with other anxiety disorders. These sensitivities may provide clues to the pathophysiology of panic disorder. Panic disorder patients are particularly sensitive to CO2 inhalation, caffeine, other xanthines and the α2-adrenergic receptor antagonist yohimbine. A suffocation alarm hypothesis [47] has been proposed to explain the enhanced sensitivity to CO2, but the neurobiological substrates of this sensitivity remain to be determined.
Brain mapping and imaging are being applied to the study of anxiety disorders
Brain mapping and functional imaging methods can add a new dimension to the understanding of anxiety disorders by identifying brain areas involved in the generation of anxiety disorders. Intense interest is now focused on the use of various forms of brain imaging for the study of anxiety disorders, with particular attention to obsessive-compulsive disorder. Most baseline and symptom provocation studies of obsessive-compulsive disorder patients using PET, single photon emission computed tomography (SPECT) and functional MRI show relative activation in the caudate, thalamus, amygdala and paralimbic areas, including the anterior cingulate and orbitofrontal cortex. Anatomical and functional evidence suggests that these areas are part of a circuit or loop which is hyperactive in obsessive-compulsive disorder [48]. Evidence that behavior can influence neurochemistry comes from studies showing normalization of the functional imaging abnormalities when obsessive-compulsive disorder patients are treated with either behavior therapy or with pharmacotherapy [49]. Although caudate and lenticular activation seems to be specific to obsessive-compulsive disorder, activation of paralimbic areas and the amygdala is also seen in post-traumatic stress disorder, panic disorder and simple phobia. Limbic and paralimbic areas are also activated in healthy control subjects by internally generated emotions and autonomic stimulation [50]. There are many differences among studies, and the technologies and methodologies are undergoing rapid change.
One can anticipate, in the near future, new systems of diagnosis for severe mental disorders based on enhanced understanding that will be gleaned from the combination of several technologies, such as more sophisticated clinical assessments, new neurochemical imaging techniques and the use of genetic markers. Improved and more specific pharmacotherapies will be developed as a result of the enormous advances in our understanding of the molecular biology and genetics of neuroregulatory mechanisms, including the neurotransmitter receptors and transporters as well as associated second messenger systems [51].
- Benzodiazepines have revolutionized the treatment of anxiety disorders
- Receptors for the benzodiazepines relate to their behavioral effects
- Central nervous system benzodiazepine-binding sites are associated with the GABAA receptor
- GABA agonists have anxiolytic effects
- Several classes of compounds act as benzodiazepine antagonists
- Multiple benzodiazepine receptors are involved in differential pharmacological actions
- Neurosteroids may modulate anxiety symptoms
- Mechanisms involving catecholamine function may be important in anxiety
- Serotonin has been linked to anxiety processes
- Neuropeptides function in anxiety processes
- Obsessive-compulsive disorder has neurobiological concomitants
- Panic disorder has specific biochemical characteristics
- Brain mapping and imaging are being applied to the study of anxiety disorders
- Biochemical Aspects of Anxiety - Basic NeurochemistryBiochemical Aspects of Anxiety - Basic Neurochemistry
Your browsing activity is empty.
Activity recording is turned off.
See more...