By agreement with the publisher, this book is accessible by the search feature, but cannot be browsed.
NCBI Bookshelf. A service of the National Library of Medicine, National Institutes of Health.
Siegel GJ, Agranoff BW, Albers RW, et al., editors. Basic Neurochemistry: Molecular, Cellular and Medical Aspects. 6th edition. Philadelphia: Lippincott-Raven; 1999.
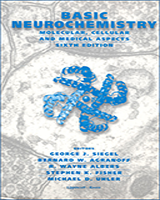
Basic Neurochemistry: Molecular, Cellular and Medical Aspects. 6th edition.
Show detailsThe mammalian olfactory system possesses enormous discriminatory power
It is claimed that humans can perceive many thousands of different odorous molecules, termed odorants, and that they can discriminate at least two to three thousand of these. It is also well known that even slight alterations in the structure of an odorant can lead to profound changes in perceived odor quality. One commonly cited example is carvone, whose l- and d-stereoisomers are perceived as spearmint and caraway, respectively. However, more subtle molecular alterations can also generate striking changes in perception.
The fine discriminatory power of the mammalian olfactory system is likely to derive from information-processing events that occur at several distinct anatomical sites: the olfactory epithelium of the nasal cavity, where odors are first sensed by olfactory sensory neurons; the olfactory bulb, where information received from the sensory neurons is presumably processed; and the cortex, where information received from the olfactory bulb is thought to be further refined to allow for the discrimination of thousands of different odors [1].
The initial events in olfaction occur in a specialized olfactory neurepithelium
In mammals, this structure lines the posterior nasal cavity (Fig. 48-1). The olfactory epithelium contains three predominant cell types: the olfactory sensory neuron; the supporting, or sustentacular, cell; and the basal, or stem, cell. Olfactory neurons are the only mammalian neurons that are known to turn over throughout life. They are continuously replaced from the basal layer of stem cells. Two morphologically distinguishable types of dividing basal cell have been identified in mammals, the horizontal basal cell and the globose basal cell. At present, it is not clear what lineage relationship these two cell types bear to each other, but both have been proposed as stem cell precursors to the olfactory neuron.

Figure 48-1
A schematic diagram of the olfactory epithelium. The initial events in odor perception occur in the olfactory epithelium of the nasal cavity. Odorants interact with specific odorant receptors on the lumenal cilia of olfactory sensory neurons. The signals (more...)
The olfactory neuron is a bipolar cell that extends a single dendrite to the epithelial surface (Fig. 48-1). From the dendritic terminus, numerous fine cilia extend into the layer of mucus that lines the nasal lumen. The cilia are the loci of the molecular transduction machinery, as has been shown by physiological [2] and biochemical [3] experiments. The details of signal generation and propagation will be discussed in a later section.
Each olfactory neuron projects an unbranched axon to the olfactory bulb, where it forms synapses within specialized regions of neuropil, called glomeruli, with periglomerular interneurons and the two major output neurons of the bulb, the mitral and tufted cells. The bulbar neurons, in turn, project to the olfactory cortex.
Odor discrimination is likely to depend upon the ability of different olfactory sensory neurons to recognize different odorants
However, electrophysiological studies of olfactory sensory neurons exposed to different odorants indicate that most neurons recognize multiple odorants or sets of odorants [4,5]. It is thought that the patterns of synapses formed in the olfactory bulb by olfactory neurons that recognize different odorants might constitute elementary odor codes. It is not clear how many different types of odorant receptor would be required in such a coding scheme nor is it obvious how narrowly or broadly tuned they might be or how their expression might be organized to achieve a high level of sensory discrimination.
Odor discrimination could involve a very large number of different odorant receptors, each highly specific for one or a small set of odorants
At the other extreme, there could be a relatively small number of different odorant receptors, each of low specificity and capable of interacting with a wide assortment of different odorous ligands. Individual olfactory neurons could each express only a single odorant-receptor type or multiple different odorant receptors.
The recent identification and cloning of genes encoding odorant receptors [6] has made it possible to begin to address these questions. By assuming that odorant receptors would be encoded by a multigene family and that the odorant receptors would belong to a large superfamily of receptors that transduce signals via interaction with heterotrimeric G proteins, Buck and Axel [6] were able to identify a novel multigene family in rat which appears to code for hundreds of diverse odorant receptors. Northern blot analyses and cDNA library screens demonstrated that the members of this family are expressed in the olfactory epithelium but not in a variety of other neural or non-neural tissues and that within the olfactory epithelium they are expressed exclusively by olfactory neurons. In a series of Southern blotting and genomic library screening experiments, they further showed that the odorant receptor multigene family contains nearly 1,000 separate genes, making this one of the largest, if not the largest, multigene family in the genome.
The amino acid sequences encoded by ten cDNAs sequenced in these initial experiments are shown in Figure 48-2 [6]. As expected, the odorant receptors belong to the G protein-coupled receptor superfamily. Like other G protein-coupled receptors, the odorant receptors all display seven hydrophobic domains, which are likely to serve as membrane-spanning regions, giving rise to a molecule that transverses the membrane seven times (Fig. 48-3). The odorant receptors also contain several limited sequence motifs which are commonly seen in members of the G protein-coupled superfamily, such as the NP pair in transmembrane domain 7 (TM7). However, the odorant receptors share extensive sequence motifs with one another that are not seen in other G protein-coupled receptors (Fig. 48-2) (see Chap. 20).

Figure 48-2
The protein sequences encoded by ten odorant receptor cDNA clones. Shaded residues are those conserved in 60% or more of the proteins. The presence of seven hydrophobic domains (I–VII), as well as short conserved motifs shared with other members (more...)

Figure 48-3
Sequence divergence in the odorant receptor family. In this schematic, the protein encoded by one rat cDNA clone (l15) is shown traversing the plasma membrane seven times. The N-terminus is located extracellularly and the C-terminus, intracellularly. (more...)
There are two striking features of the odorant receptors that may be relevant to the role of these molecules in odor discrimination [6]. Although the odorant receptors, as a group, display a number of conserved sequence motifs, they also display an impressive degree of diversity in the nonconserved regions. Detailed studies involving in vitro mutagenesis and domain swapping have been performed with the β-adrenergic receptor and several other members of the G protein-coupled superfamily in order to ascertain what regions of these molecules are involved in ligand binding [7]. These studies indicate that many G protein-coupled receptors bind to ligand via a ligand-binding pocket, which is formed in the plane of the membrane by a combination of the transmembrane domains. The importance of the transmembrane domains in ligand binding is further stressed in alignments of members of small families of G protein-coupled receptors that bind to the same ligand, such as the adrenergic receptor family or the muscarinic acetylcholine receptor family. These comparisons reveal that sequence conservation among receptors that bind the same ligand is highest in the transmembrane domains. In contrast, the odorant receptor family exhibits extensive sequence divergence in several transmembrane domains (TM3, TM4 and TM5) (Figs. 48-2 and 48-3). This diversity in potential ligand-binding regions within the odorant receptor family is consistent with an ability of this family to interact with a large number of structurally diverse odorous ligands.
A second interesting feature of the odorant-receptor family that may be important in odor discrimination is the presence of subfamilies [6]. As already mentioned, TM5, which could be involved in ligand binding, is highly divergent in members of the odorant receptor family. However, as shown in Figure 48-4, members of the odorant receptor-family appear to fall into subfamilies whose members can be almost identical in this divergent region. It is tempting to propose that the members of divergent subfamilies recognize different structural classes of odorants. The highly related members of a subfamily might either recognize the same odorants or detect subtle differences between structurally related odorants.

Figure 48-4
Evidence for subfamilies within the odorant receptor family. The deduced protein sequences of 18 different cDNA clones in and around transmembrane domain V are shown. A: Residues shared by 60% or more of the proteins at a given position are shaded. Transmembrane (more...)
Interestingly, Southern blotting experiments indicate that subfamilies are a characteristic feature of the odorant receptor multigene family [6,8,9]. When different members of the multigene family are hybridized to Southern blots of restriction enzyme-digested genomic DNA, distinct patterns of hybridizing species are seen, each of which is likely to represent a different member of the subfamily. The individual subfamilies appear to range in size from one to 20 members but on average have about seven to ten members.
The remarkable size and diversity of the odorant receptor multigene family in mammals suggests that odor discrimination relies heavily on the initial event in olfactory perception, the interaction of odorant receptor with odorant. In this respect, olfaction contrasts sharply with color vision, where signals derived from only three peripheral receptor types, the three color opsins, are processed to allow the perception of a multitude of different hues. The extremely large number of odorant receptor genes further suggests that individual receptors could be fairly specific, each one recognizing only one or a small set of structurally similar odorants.
The information generated by hundreds of different receptor types must be organized to achieve a high level of olfactory discrimination
Most sensory systems localize environmental information in space and possess neural topographical maps of that spatial information. The olfactory system does not perceptually localize environmental information in external space. However, it could use spatial determinants within the nervous system to encode information. If so, topographical maps or spatial codes for odors might be evident within the olfactory epithelium or olfactory bulb. For example, olfactory neurons that express a particular odorant receptor gene, and therefore recognize the same odorants, might be clustered in one region of the olfactory epithelium or might all form synapses at a discrete site in the olfactory bulb. On the other hand, neurons that express the same odorant receptor gene could be broadly distributed in the olfactory epithelium or form synapses at many different bulbar sites, encoding information by a nontopographical strategy.
Zonal organization in the olfactory epithelium. To address these questions, Ressler et al. [8] and Vassar et al. [9] analyzed the patterns of expression of different odorant receptor genes within the olfactory epithelium of the mouse. They found that different odorant receptors are expressed in distinct topographical patterns within the nasal cavity. It appears that the olfactory epithelium is divided into a limited series of expression zones. These zones exhibit bilateral symmetry in the two nasal cavities and are virtually identical in different individuals. Within each zone, many different members of the odorant receptor gene family are expressed. However, each individual gene may be expressed only within a single zone. Although the zonal assignment of each gene appears to be strictly regulated, within each zone neurons that express a particular receptor gene are broadly distributed. It thus appears that when an olfactory neuron or its progenitor chooses which odorant-receptor gene(s) to express, it is restricted to a single zonal gene set but may choose a receptor gene or set of genes to express from among the zonal set via a stochastic mechanism.
Organization in the epithelium to bulb projection. The odorant receptor expression zones in the olfactory epithelium exhibit a pronounced dorsal-ventral and medial-lateral organization [8]. Interestingly, the axonal projection from the olfactory epithelium to the olfactory bulb is also organized along the dorsal-ventral and medial-lateral axes [10]. Comparisons of the locations of the expression zones with the topography of projections between the olfactory epithelium and olfactory bulb indicate that the organization of odorant receptor gene expression in the epithelium is preserved in the axonal projection to the olfactory bulb [8]. Indeed, the map is even more specific than this. For reasons that remain obscure, mRNA for receptors can be found at low levels in the synaptic terminals of olfactory axons. With in situ hybridization using receptor probes it has been possible to visualize specific glomeruli that are “hot spots” for each receptor gene type. That is, each of the 1,900 glomeruli in a rat is dominated by inputs from cells expressing the same receptor. In an even more remarkable set of experiments on transgenic mice [11], the marker gene lacz was placed under the control of a specific, odor receptor promoter such that all of the cells expressing that receptor would be a dark blue color. Several thousand cells, scattered throughout a single expression zone in the epithelium, could be seen sending their axons to two glomeruli in each bulb. Thus, it appears that all of the cells expressing a particular receptor are somehow targeted to one or two among approximately 1,900 glomeruli in the mouse olfactory bulb.
These results suggest that an initial organization of olfactory sensory information occurs in the olfactory epithelium and that this organization is maintained in the patterns of signals transmitted to the olfactory bulb [12]. This conclusion is consistent with the results of recent electrophysiological studies which showed that responses in mitral cells to odors applied to the epithelium are narrowly tuned and that sensitivity to particular types of odor, determined by either functional group or carbon chain length, is spatially organized in the bulb [13]. Thus, the organization of receptors into broad zones in the epithelium is further refined in the bulb, where receptors find common targets.
The reputed sensitivity of the olfactory system is likely to derive from the capacity of the olfactory transduction apparatus to effectively amplify and rapidly terminate signals
The olfactory system is thought to respond to extremely low concentrations of odorants and to have a fast recovery of perceptual sensitivity. In recent years, significant advances have been made in the understanding of the signal-transduction events that take place when an olfactory sensory neuron is exposed to an odorant. Before discussing the biochemical events involved in the signal-transduction process, it is instructive to consider the sensitivity of the olfactory system at the level of perception versus the individual neuron.
Although olfactory perception is believed to be extremely sensitive, the degree of sensitivity is controversial. Some reports, especially in insects, have suggested that odors involved in sexual attraction could be detected by male animals at the level of the single molecule, that is, concentrations as low as 10−14 M. Human olfactory thresholds of 10−11 M have been measured by psychophysical methods. In such studies, however, the actual concentration of odorant at the odorant receptor cannot be known definitively. Due to factors such as odor molecule interactions, diffusion, adsorption into mucus, and anatomical structures which may trap and concentrate odor molecules, these estimates could be as much as two to four orders of magnitude too low.
Physiological recordings from single receptor neurons suggest somewhat lower sensitivities, with K1/2 values between 1 and 50 μM [14]. These values could allow for broad specificity and rapid termination of odorant responses. For example, since Kd = koff/kon and the on rate, approximately 108 M−1·sec−1, is ultimately limited by diffusional encounters between ligand and receptor, the Kd is limited by the range of acceptable off rates. A Kd of 10−6 would result in molecular encounters lasting a few hundred milliseconds, whereas a Kd of 10−11 would result in dwell times of more than 5 min.
It can be shown from the law of mass action that with significant amplification mechanisms, such that occupation of only a few receptors would be sufficient to elicit a response, thresholds as much as five or six orders of magnitude lower than the Kd can be attained. Thus, Kd values of 10−6 could, in theory, produce threshold responses at odor concentrations as low as 10−11, permitting the olfactory system to maintain the broad specificity seen in physiological recordings [5] and the high threshold sensitivity measured in psychophysical experiments. Several amplification mechanisms that could serve this purpose are described below.
Odorant recognition initiates a second-messenger system leading to the depolarization of the neuron and the generation of action potentials
Recordings of the electrical activity of single olfactory receptor neurons show that exposure to odorants causes the membrane to depolarize, leading to the generation of action potentials [2]. Underlying the initial depolarization is the influx of cations through a conductance in the specialized cilia extending from the distal end of the cell into the mucous lining of the nasal cavity (Fig. 48-5). The biochemical elements coupling odorant receptor binding to the opening of a cation channel are now understood in some detail [15]. A consensus view is presented in Figure 48-6.

Figure 48-5
Schematic drawing of an olfactory neuron showing the single bipolar morphology, with a single thick dendrite ending in a knob-like swelling and an unbranched axon projecting from the proximal soma centrally to the olfactory bulb. The cell is highly compartmentalized (more...)

Figure 48-6
A model for the operation of the cAMP-based second-messenger system in olfactory neurons. The individual steps are detailed in the text. Note that there are several feedback loops that modulate the response. These include inhibition of the channel by (more...)
The second-messenger cascade of olfactory transduction is a classic cyclic nucleotide-based system with one or two interesting modifications. A G protein that is likely to couple odorant receptors to other intracellular elements of the cascade, Golf, was identified by Reed [15] and shown to be an isoform of Gs specific to olfactory neurons. It has been shown that high concentrations of Golf are present in olfactory cilia. Since Golf can interact with G protein-coupled receptors other than odorant receptors, it has been proposed that the existence of a separate gene for Golf may, in some manner, permit the specific and high level of expression of this protein observed in olfactory neurons (Chaps. 20, 23).
An adenylyl cyclase has been identified in olfactory neurons which also appears to be specific to these cells and is biochemically distinct from other known isoforms of this enzyme. Also cloned and characterized by Reed, it has been termed adenylyl cyclase type III [15]. An important characteristic of this isozyme is that, when expressed in a mammalian cell line, its basal activity is extremely low, while in its stimulated state, it has a catalytic rate higher than other known cyclases. These properties could confer a high signal-to-noise ratio on the system, being quiescent in the absence of stimulus but able to rapidly generate large amounts of cAMP upon odor exposure.
Consistent with this idea are the kinetics of cAMP accumulation, which have been followed on a sub-second time scale by Breer [3] using a rapid quench technique. When odorants were added to an olfactory cilia preparation, cAMP increased linearly with odorant concentration up to a level fourfold greater than baseline. The increase peaked within 50 msec of exposure to the odor stimuli and returned to baseline levels within about 300 msec, suggesting that odorant stimulates the pulsatile production of cAMP.
In 1987, Nakamura and Gold [16] recorded ion currents from a ciliary conductance that was activated directly by cAMP, without an intervening phosphorylation step. This was the final link between the biochemical cascade and the electrical signal. The channel was subsequently cloned and characterized in several species [15]. The channel is selective for cations; is curiously sensitive to both cAMP, with a Kd of 20 μM, and cGMP with a Kd of 5 μM; and requires at least three molecules of cyclic nucleotide to bind for activation. It shares 65% similarity with the cGMP-activated channel found in photoreceptors, and, most interestingly, it bears strong homology with voltage-sensitive channels, such as K+ and Ca2+ channels.
The activation of tens to hundreds of these channels and the subsequent influx of cations, including both Na+ and Ca2+, lead to depolarization of the cell membrane. Olfactory neurons have one additional level of amplication that is rather unusual. A Ca2+-activated Cl− channel is also present on the cilia and is opened during the odor response by the Ca2+ ions flowing into the cilia through the cAMP-gated channel [17]. Curiously, olfactory neurons maintain a very high intracellular Cl− concentration, perhaps as high as 125 mM, so that the driving force for Cl− ions is outward and, therefore, further depolarizes the cell. This additional current may be important for preserving ionic driving forces across a membrane in a compartment that is not highly regulated, that is, the mucus. Changing ion concetrations in the mucus could affect the driving forces on ions, so the olfactory neuron supplies its own driving force by maintaining a high intracellular concentration of Cl−.
Some of the important physiological characteristics of the odor response can be seen in recordings of currents through the cAMP-sensitive channels in response to pulses of odor stimuli (Fig. 48-7) [14]. There is a long, concentration-dependent latency between the binding of odor molecules and the activation of the current, lasting 150 to 450 msec. The peak current amplitude is a sigmoidal function of the log odor concentration that can be fit by the Hill equation with a Hill coefficient of between 2 and 4. The cooperativity in the response appears to emanate from the requirement of the cAMP channel for cooperative activation by cAMP and from activation of the Cl− current by Ca2+ ions [17]. There is no indication that odorant receptors bind odorant ligands cooperatively.

Figure 48-7
Responses of single neurons to varying concentrations of odorant. A: A family of responses in a single olfactory neuron to the odor cineole at concentrations varying from 1 to 100 μM. Downward deflections represent inward ionic currents whose (more...)
This pathway provides several amplification steps between odorant binding and signal generation. Due to the electrically compact structure of the cell, it is possible for the activation of only a few tens of channels to drive the membrane to threshold for action potential generation. Thus, it is theoretically possible that the limit of olfactory detection is the single molecule, although this has not yet been conclusively demonstrated.
Negative feedback processes mediate adaptation in the odor-induced response
Upon application of a sustained odor stimulus to an olfactory neuron, the current response is transient, falling back to baseline within 4 to 5 sec [2]. The termination of a response in the continued presence of agonist is characteristic of many signaling systems and is variously known as adaptation or desensitization, depending on the putative site of the off mechanism. Typically, a negative feedback process is at work such that accumulation of a product of the agonist response serves to turn off an upstream link in the signal-generating cascade. In the olfactory neuron, two feedback messengers have been identified, and, as might be expected, they are Ca2+ and cAMP.
The ion channel activated by cAMP is permeable to cations, including Ca2+ [18]. Thus, increased channel activation results in influx of Ca2+ and a transient rise in the intracellular concentration of Ca2+. Intracellular Ca2+ concentrations of 1 to 3 μM have been found to lead to a decrease in the open probability of the ion channel, even in the presence of high concentrations of cAMP [19]. The mechanism could involve a direct effect of Ca2+ on the channel or be mediated via an intermediate Ca2+-binding protein. In either case, this is an attractive mechanism for mediating a rapid but short-lasting form of adaptation since it is dependent on the influx of Ca2+ during the response to an odor. It has been shown that virtually all short-term adaptation could indeed be accounted for in this way. Comparing adaptation to odors with that induced by the photolytic release of cAMP in the cell, it was found that adaptation to both was identical. That is, the rise in cAMP and the consequent opening of cAMP-gated channels alone was enough to cause the cell to adapt to further stimulation, suggesting that this last step in the transduction cascade was the primary site of adaptation.
A second pathway, utilizing a phosphorylation step, resembles desensitization mechanisms described for the β-adrenergic receptor and rhodopsin [3,7,20]. cAMP, in addition to opening the ion channel, appears to activate a form of cAMP-dependent protein kinase (PKA), which inactivates some earlier step in the transduction process. Addition of a peptide fragment of the Walsh inhibitor protein (WIPTIDE), a PKA antagonist, prolongs the odorant-induced production of cAMP [3]. Studies of desensitization of the β-adrenergic receptor and rhodopsin have revealed two specialized but closely related kinases, β-adrenergic receptor kinase (βARK) (Chap. 24) and rhodopsin kinase (Chap. 47), respectively, that phosphorylate the activated receptor. Interestingly, a recently characterized isoform of βARK has been shown to be highly enriched in olfactory sensory neurons [20]. Odorant-induced phosphorylation of ciliary proteins by both PKA and protein kinase C (PKC) has been demonstrated, but the phosphorylated proteins have not been conclusively identified. Thus, it is possible that the cAMP generated in response to odor stimulation activates negative-feedback pathways that act to truncate an ongoing response.
Alternative second-messenger pathways may be at work in olfactory transduction
The role of cAMP in olfactory transduction is well established. Are there alternative pathways, such as those involving phospholipids and Ca2+? Breer and his colleagues [3] have assayed for inositol 1,4,5,-trisphosphate (IP3) production in the rat using odorants that failed to produce cAMP in biochemical assays. They found rapid and significant increases in IP3, and that a given odorant led to the production of either cAMP or IP3, never both. More recently, they have found evidence for two pathways for adaptation, one utilizing PKA and the other PKC, and have shown that the responses to odors using one or the other pathway are completely additive. Utilizing cultured mammalian olfactory neurons, Ronnett and her colleagues have found evidence that many odorants can stimulate both IP3 and cAMP production but that the ratio of IP3 to cAMP varies according to odorant.
In contrast to these data, a mouse in which the cAMP-gated channel was knocked out was determined to be completely anosmic, that is, incapable of smelling, indicating that there is only one second-messenger pathway and it utilizes cAMP [21]. This is also consistent with most physiological data, which show that exogenous application of IP3 to intact olfactory neurons does not affect the odor-transduction system. It may still be determined that IP3 may have a modulatory role, but it appears unlikely to be a signal-transduction pathway in vertebrate olfaction. It should be noted, however, that among invertebrates there is good evidence that IP3, as well as cAMP, play important roles in olfactory signal transduction [22].
The vomeronasal organ is an accessory chemosensing system devoted to detecting conspecific chemical cues, also known as pheromones
These cues are important in rearing, territorial, courtship and, in particular, sexual behaviors. The vomeronasal organ (VNO) is separate from the main epithelium in mammals, comprising a thin epithelial tissue within a bony capsule in the lateral wall of the nasal cavity. At least two populations of chemosensory neurons share this tissue, as determined by the differential expression of the G proteins Gi or Go. Aside from this single fact, little is known of the transduction pathway in the VNO neurons, but it appears to be neither cyclic nucleotides nor phospholipids.
A family of G protein-coupled receptors was cloned in the VNO and apparently represents the pheromone receptors [23]. These receptors are in the seven-transmembrane domain family of receptors but are only weakly similar to the odor receptors of the main epithelium. There appear to be at least 50 to 100 different receptors specific to the VNO, and it remains a possibility that this is not the only family of pheromone receptors since all of these receptors are expressed in only one of the two classes of VNO neuron.
- The mammalian olfactory system possesses enormous discriminatory power
- The initial events in olfaction occur in a specialized olfactory neurepithelium
- Odor discrimination is likely to depend upon the ability of different olfactory sensory neurons to recognize different odorants
- Odor discrimination could involve a very large number of different odorant receptors, each highly specific for one or a small set of odorants
- The information generated by hundreds of different receptor types must be organized to achieve a high level of olfactory discrimination
- The reputed sensitivity of the olfactory system is likely to derive from the capacity of the olfactory transduction apparatus to effectively amplify and rapidly terminate signals
- Odorant recognition initiates a second-messenger system leading to the depolarization of the neuron and the generation of action potentials
- Negative feedback processes mediate adaptation in the odor-induced response
- Alternative second-messenger pathways may be at work in olfactory transduction
- The vomeronasal organ is an accessory chemosensing system devoted to detecting conspecific chemical cues, also known as pheromones
- Olfaction - Basic NeurochemistryOlfaction - Basic Neurochemistry
- Gart phosphoribosylglycinamide formyltransferase, phosphoribosylglycinamide synt...Gart phosphoribosylglycinamide formyltransferase, phosphoribosylglycinamide synthetase, phosphoribosylaminoimidazole synthetase [Rattus norvegicus]Gene ID:288259Gene
Your browsing activity is empty.
Activity recording is turned off.
See more...