By agreement with the publisher, this book is accessible by the search feature, but cannot be browsed.
NCBI Bookshelf. A service of the National Library of Medicine, National Institutes of Health.
Siegel GJ, Agranoff BW, Albers RW, et al., editors. Basic Neurochemistry: Molecular, Cellular and Medical Aspects. 6th edition. Philadelphia: Lippincott-Raven; 1999.
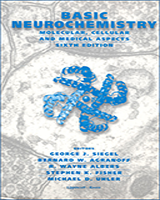
Basic Neurochemistry: Molecular, Cellular and Medical Aspects. 6th edition.
Show detailsThe peripheral and central nervous systems have many anatomical and molecular features in common
Somatic motor and sensory neurons that give rise to peripheral nervous system (PNS) axons maintain large fractions of their total protoplasmic bulk within the central nervous system (CNS). Extracellular matrix components involved in the guidance of CNS development are employed for the same purpose in the PNS. PNS microglia-like cells, as microglia in the CNS, are bone marrow-derived and have a similar repertoire of responses to activation. Both oligodendroglia and Schwann cells speed axonal action potential propagation by assembling and maintaining myelin. Capillary endothelial cells linked by tight junctions serve to restrict entry of polar molecules into the PNS, as into the CNS.
One substantial difference between the PNS and CNS is that PNS regeneration is robust, a difference attributable in part to the contrasting inhibitory effect of oligodendroglia and the stimulatory effect of Schwann cells on axonal elongation. We provide here a short description of common patterns of PNS degeneration and regeneration.
Despite the resemblances between the PNS and CNS, there are many PNS-specific diseases. Some of the reasons for PNS disease selectivity are listed in Table 36-1. We briefly discuss a few of these PNS-selective diseases (Table 36-2) and some disorders affecting the CNS as well as the PNS (Table 36-3). The chapter concludes with a short consideration of the enteric nervous system (ENS), a clinically important but relatively neglected region of the nervous system.
Table 36-1
Mechanisms of PNS-Specific Disease Pathogenesis.
Table 36-2
Examples of Diseases Affecting Exclusively or Principally the PNS.
Table 36-3
Examples of Diseases Affecting Both PNS and CNS.
Cellular and molecular properties of the peripheral nervous system are important in disease susceptibility and regenerative capacity
Whereas in the CNS, oligodendroglia invest large axons in myelin and astroglia regulate ion and metabolite concentrations in the neuropil, these functions are the combined responsibility of Schwann cells in the PNS. There are two major Schwann cell phenotypes: myelinating Schwann cells, which ensheath single axons greater than 1 μm in diameter for hundreds of microns and synthesize myelin-specific molecules, such as P0 [1], and nonmyelinating Schwann cells, which invest multiple small axons and do not synthesize myelin (see Chaps. 1 and 4). The choice between myelin-forming and nonmyelinating Schwann cell specializations is determined by the caliber of the axons with which the Schwann cells are in contact [2]. Reciprocally, Schwann cells regulate the cytoskeletal organization and axonal caliber of the axons they invest [3].
Myelin, a paracrystalline array of lipid-rich glial or Schwann cell plasma membranes, supports saltatory, rapid conduction of nerve impulses by providing a high-resistance, low-capacitance sheath around large axons. Unlike oligodendroglia, Schwann cells form myelin around no more than one axon. Both PNS and CNS myelin contain various isoforms of P1 myelin basic protein [4]; multiple isoforms of myelin-associated glycoprotein (MAG), which is involved in glia—axon adhesion [5]; abundant cholesterol and phospholipids; and the galactosphingolipids galactocerebroside and sulfatide. While proteolipid protein (PLP) is the most abundant protein in CNS myelin [6] and is made by Schwann cells as well [7], it is only a minor PNS myelin constituent. Myelin proteins present in PNS myelin but not in CNS myelin of most higher vertebrates include P0 and P2 (see Chap. 4).
PNS sensory and motor axons may exceed 1 m in length. Since PNS axons lack ribosomes, the supply of essential structural proteins and enzymes to distal regions derived from the neuronal perikaryon must be transported through the axons for great distances (see Chap. 28). The proteins that polymerize to form neurofilaments and microtubules as well as many enzymes move centrifugally at 1 to 10 mm/day. Organelles such as neurotransmitter vesicles and mitochondria are transported through the axoplasm at velocities of greater than 100 mm/day by a process involving interactions between kinesin and microtubules [8]. Conversely, proteins internalized by the nerve terminal are translocated toward the neuronal perikaryon at approximately 100 mm/day, employing a mechanism in which dynein and microtubules play roles [9]. PNS neurons with long axons are particularly vulnerable to disorders that compromise either perikaryal synthesis of axonal proteins or the local axonal machinery responsible for axoplasmic transport. Long axons are also more likely to sustain localized damage owing to trauma, ischemia or other noxious influences.
Wallerian degeneration. When Schwann cell—axon contact is lost, as occurs in the distal stump of nerves undergoing Wallerian degeneration, mononuclear cells from the bloodstream enter the trauma zone and assist resident Schwann cells and microglia-like cells in catabolizing fragmented myelin. These cells secrete cytokines (for example, interleukin-1 and platelet-derived growth factor), which stimulate Schwann cell proliferation. These cycling Schwann cells downregulate expression of myelin components and form tubular cellular aggregates (“bands of Büngner”), which facilitate axonal regeneration. In the nerves of young animals undergoing Wallerian degeneration, some of these excess Schwann cells generated during Wallerian degeneration undergo apoptosis [10]. Myelin-forming and nonmyelinating Schwann cell phenotypes are restored as axonal regeneration through the distal stump occurs.
Segmental demyelination. Schwann cells injured by immune-mediated mechanisms or subjected to trauma may lose axonal contact, proliferate and participate in the catabolism of myelin fragments. If several successive internodes along an axon are demyelinated, nerve action potential conduction through the demyelinated segment is blocked, resulting in clinically detectable neurological dysfunction. Since thousands of Schwann cells are required to myelinate long PNS axons, the likelihood is high that a pathological process that randomly compromises the capacity of Schwann cells to maintain myelin will interfere with saltatory conduction along such a fiber. Thin, regenerating myelin sheaths permit nerve action potential propagation but at markedly diminished velocity. Excess Schwann cells generated during the phase of Schwann-cell proliferation that are not successful in re-establishing contact with an axon are pruned from the nerve, perhaps by apoptosis. Repeated cycles of demyelination and remyelination, which may occur in various inherited and acquired demyelinative neuropathies, lead to the deposition of layers of redundant Schwann cells, which encircle demyelinated or partially remyelinated axons, forming “onion bulbs” [11]. Under these circumstances, full regeneration of normal PNS anatomy may become impossible.
Clinical features of polyneuropathies reflect vulnerability of the longest axons to degeneration and segmental demyelination
Typically, patients manifest symmetrical distal sensory disturbances, usually first in the feet. Distal muscles are weakened more than proximal muscles, and distal tendon reflexes are lost prior to proximal tendon reflexes. This pattern of clinical deficit is referred to as symmetrical distal polyneuropathy. In some instances, symmetrical distal polyneuropathy causes selective impairment of transmission of motor function, such as acute motor axonal neuropathy [12], or of sensory and autonomic function, such as familial amyloid neuropathy [13]. Signs of autonomic neuropathy include defective regulation of blood pressure, heart rate, pupillary responses and bowel motility. Other clinical patterns of PNS disease include mononeuropathy, which is the dysfunction of a single peripheral nerve, and mononeuropathy multiplex, which involves several to many, but not all, peripheral nerves. Mononeuropathy multiplex is caused most commonly by diseases affecting PNS blood flow, including periarteritis nodosa and diabetes mellitus [14].
Extracellular matrix. Flattened, basal lamina-lined perineurial cells [15] delineate nerve fascicles, providing a barrier to movement of ionic compounds and macromolecules between nonneural tissues and the endoneurium (see also Chap. 32). Fibroblasts constitute roughly 10% of total cells within the endoneurium and are far more uncommon in the CNS. The collagens are a family of extracellular structural proteins characterized by a triple α-helical configuration and a high content of hydroxyproline, hydroxylysine and glycine. Collagens comprise 30% or more of total PNS protein and are present in the endoneurium both in the form of interstitial collagen fibrils and as a component of the basal lamina surrounding the processes of Schwann cells and perineurial cells, in addition to lining the outer aspect of endoneurial capillaries. The bulk of interstitial collagen fibrils are synthesized by fibroblasts, although Schwann cells and perineurial mesothelial cells secrete the more heavily glycosylated basal lamina forms of collagen. Fibroblasts augment production of interstitial collagen both in a traumatized nerve segment and distal to it. This increases the tensile strength of the damaged nerve and provides the collagenous framework required for axonal ensheathment by Schwann cells. The extracellular accumulation of collagen in the injured PNS [16] contrasts sharply with the astrogliosis and increased intracellular glial fibrillary acidic protein (GFAP) elicited by trauma in the CNS. As regeneration proceeds, axonal growth cones penetrate the scar and extend into the tubular Schwann cell aggregates.
Compression injury. Movements of the limbs subject nerves to repeated stretch. Although collagen fibrils afford tensile strength, there is some waviness in the course of axons that permits a degree of nerve elongation without axonal ripping. However, stretch that is too great causes axonal disruption. Nerves in exposed regions, for example, the median nerve at the wrist, the ulnar nerve at the elbow and the common peroneal nerve at the knee, are vulnerable to compression that can cause either demyelination or axonal transection if it is sufficiently severe. Such compression also can interrupt blood flow, causing nerve infarction. In addition to these mechanical injuries, superficial nerves experience considerable thermal fluctuations that can kill cells directly or predispose nerves to damage by precipitation of cold-insoluble proteins or other mechanisms (see discussion of leprosy, for example, below).
Blood—nerve barrier. Both the CNS and PNS demonstrate local regions of incompetence of their respective blood—tissue barrier systems, and in both tissues these regions have specific physiological functions. In the CNS, for example, the function of medullary chemosensory nuclei requires free passage of polar molecules from the blood. In the PNS, trophic proteins secreted by target organs gain access to axon terminals that are not invested in a perineurial sheath. Lectins and other proteins useful as anatomical tracers also gain access to the PNS at nerve terminals, and some viruses are able to penetrate at this site [9]. Another region of relative incompetence of the blood—nerve barrier is at the level of the dorsal root ganglia. Neurotoxic molecules may gain entry to the PNS at this point, producing degeneration of sensory neurons [17,18].
- The peripheral and central nervous systems have many anatomical and molecular features in common
- Cellular and molecular properties of the peripheral nervous system are important in disease susceptibility and regenerative capacity
- Clinical features of polyneuropathies reflect vulnerability of the longest axons to degeneration and segmental demyelination
- Regeneration in the Central and Peripheral Nervous Systems - Basic Neurochemistr...Regeneration in the Central and Peripheral Nervous Systems - Basic Neurochemistry
- LOC4329556 [Oryza sativa Japonica Group]LOC4329556 [Oryza sativa Japonica Group]Gene ID:4329556Gene
Your browsing activity is empty.
Activity recording is turned off.
See more...