NCBI Bookshelf. A service of the National Library of Medicine, National Institutes of Health.
Substance Abuse and Mental Health Services Administration (US); Office of the Surgeon General (US). Facing Addiction in America: The Surgeon General's Report on Alcohol, Drugs, and Health [Internet]. Washington (DC): US Department of Health and Human Services; 2016 Nov.
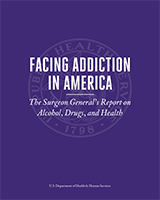
Facing Addiction in America: The Surgeon General's Report on Alcohol, Drugs, and Health [Internet].
Show detailsChapter 2 Preview
A substantial body of research has accumulated over several decades and transformed our understanding of substance use and its effects on the brain. This knowledge has opened the door to new ways of thinking about prevention and treatment of substance use disorders.
This chapter describes the neurobiological framework underlying substance use and why some people transition from using or misusing alcohol or drugs to a substance use disorder—including its most severe form, addiction. The chapter explains how these substances produce changes in brain structure and function that promote and sustain addiction and contribute to relapse. The chapter also addresses similarities and differences in how the various classes of addictive substances affect the brain and behavior and provides a brief overview of key factors that influence risk for substance use disorders.
An Evolving Understanding of Substance Use Disorders
Scientific breakthroughs have revolutionized the understanding of substance use disorders. For example, severe substance use disorders, commonly called addictions, were once viewed largely as a moral failing or character flaw, but are now understood to be chronic illnesses characterized by clinically significant impairments in health, social function, and voluntary control over substance use.3 Although the mechanisms may be different, addiction has many features in common with disorders such as diabetes, asthma, and hypertension. All of these disorders are chronic, subject to relapse, and influenced by genetic, developmental, behavioral, social, and environmental factors. In all of these disorders, affected individuals may have difficulty in complying with the prescribed treatment.4
This evolving understanding of substance use disorders as medical conditions has had important implications for prevention and treatment. Research demonstrating that addiction is driven by changes in the brain has helped to reduce the negative attitudes associated with substance use disorders and provided support for integrating treatment for substance use disorders into mainstream health care. Moreover, research on the basic neurobiology of addiction has already resulted in several effective medications for the treatment of alcohol, opioid, and nicotine use disorders, and clinical trials are ongoing to test other potential new treatments.5
KEY FINDINGS*
- Well-supported scientific evidence shows that addiction to alcohol or drugs is a chronic brain disease that has potential for recurrence and recovery.
- Well-supported evidence suggests that the addiction process involves a three-stage cycle: binge/intoxication, withdrawal/negative affect, and preoccupation/anticipation. This cycle becomes more severe as a person continues substance use and as it produces dramatic changes in brain function that reduce a person's ability to control his or her substance use.
- Well-supported scientific evidence shows that disruptions in three areas of the brain are particularly important in the onset, development, and maintenance of substance use disorders: the basal ganglia, the extended amygdala, and the prefrontal cortex. These disruptions: (1) enable substance-associated cues to trigger substance seeking (i.e., they increase incentive salience); (2) reduce sensitivity of brain systems involved in the experience of pleasure or reward, and heighten activation of brain stress systems; and (3) reduce functioning of brain executive control systems, which are involved in the ability to make decisions and regulate one's actions, emotions, and impulses.
- Supported scientific evidence shows that these changes in the brain persist long after substance use stops. It is not yet known how much these changes may be reversed or how long that process may take.
- Well-supported scientific evidence shows that adolescence is a critical “at-risk period” for substance use and addiction. All addictive drugs, including alcohol and marijuana, have especially harmful effects on the adolescent brain, which is still undergoing significant development.
- *
Well-supported: when evidence is derived from multiple rigorous human and nonhuman studies; Supported: when evidence is derived from rigorous but fewer human and nonhuman studies.
All addictive substances have powerful effects on the brain. These effects account for the euphoric or intensely pleasurable feelings that people experience during their initial use of alcohol or other substances, and these feelings motivate people to use those substances again and again, despite the risks for significant harms.
FOR MORE ON THIS TOPIC
See the section on “Factors that Increase Risk for Substance Use, Misuse, and Addiction” later in this chapter.
As individuals continue to misuse alcohol or other substances, progressive changes, called neuroadaptations, occur in the structure and function of the brain. These neuroadaptations compromise brain function and also drive the transition from controlled, occasional substance use to chronic misuse, which can be difficult to control. Moreover, these brain changes endure long after an individual stops using substances. They may produce continued, periodic craving for the substance that can lead to relapse: More than 60 percent of people treated for a substance use disorder experience relapse within the first year after they are discharged from treatment,4,6 and a person can remain at increased risk of relapse for many years.7,8
However, addiction is not an inevitable consequence of substance use. Whether an individual ever uses alcohol or another substance, and whether that initial use progresses to a substance use disorder of any severity, depends on a number of factors. These include: a person's genetic makeup and other individual biological factors; the age when use begins; psychological factors related to a person's unique history and personality; and environmental factors, such as the availability of drugs, family and peer dynamics, financial resources, cultural norms, exposure to stress, and access to social support.9 Some of these factors increase risk for substance use, misuse, and use disorders, whereas other factors provide buffers against those risks. Nonetheless, specific combinations of factors can drive the emergence and continuation of substance misuse and the progression to a disorder or an addiction.
FOR MORE ON THIS TOPIC
See Chapter 3 - Prevention Programs and Policies.
Conducting Research on the Neurobiology of Substance Use, Misuse, and Addiction
Until recently, much of our knowledge about the neurobiology of substance use, misuse, and addiction came from the study of laboratory animals. Although no animal model fully reflects the human experience, animal studies let researchers investigate addiction under highly controlled conditions that may not be possible or ethical to replicate in humans. These types of studies have greatly helped to answer questions about how particular genes, developmental processes, and environmental factors, such as stressors, affect substance-taking behavior.
KEY TERMS
Neurobiology. The study of the anatomy, function, and diseases of the brain and nervous system.
Neurobiology studies in animals have historically focused on what happens in the brain right after taking an addictive substance (this is called the acute impact), but research has shifted to the study of how ongoing, long-term (or chronic) substance use changes the brain. One of the main goals of this research is to understand at the most basic level the mechanisms through which substance use alters brain structure and function and drives the transition from occasional use to misuse, addiction, and relapse.10
A growing body of substance use research conducted with humans is complementing the work in animals. For example, human studies have benefited greatly from the use of brain-imaging technologies, such as magnetic resonance imaging (MRI) and positron emission tomography (PET) scans. These technologies allow researchers to “see” inside the living human brain so that they can investigate and characterize the biochemical, functional, and structural changes in the brain that result from alcohol and drug use. The technologies also allow them to understand how differences in brain structure and function may contribute to substance use, misuse, and addiction.
Animal and human studies build on and inform each other, and in combination provide a more complete picture of the neurobiology of addiction. The rest of this chapter weaves together the most compelling data from both types of studies to describe a neurobiological framework for addiction.
A Basic Primer on the Human Brain
To understand how addictive substances affect the brain, it is important to first understand the basic biology of healthy brain function. The brain is an amazingly complex organ that is constantly at work. Within the brain, a mix of chemical and electrical processes controls the body's most basic functions, like breathing and digestion. These processes also control how people react to the multitudes of sounds, smells, and other sensory stimuli around them, and they organize and direct individuals' highest thinking and emotive powers so that they can interact with other people, carry out daily activities, and make complex decisions.
The brain is made of an estimated 86 billion nerve cells—called neurons—as well as other cell types. Each neuron has a cell body, an axon, and dendrites (Figure 2.1). The cell body and its nucleus control the neuron's activities. The axon extends out from the cell body and transmits messages to other neurons. Dendrites branch out from the cell body and receive messages from the axons of other neurons.
Neurons communicate with one another through chemical messengers called neurotransmitters. The neurotransmitters cross a tiny gap, or synapse, between neurons and attach to receptors on the receiving neuron. Some neurotransmitters are inhibitory—they make it less likely that the receiving neuron will carry out some action. Others are excitatory, meaning that they stimulate neuronal function, priming it to send signals to other neurons.
Neurons are organized in clusters that perform specific functions (described as networks or circuits). For example, some networks are involved with thinking, learning, emotions, and memory. Other networks communicate with muscles, stimulating them into action. Still others receive and interpret stimuli from the sensory organs, such as the eyes and ears, or the skin. The addiction cycle disrupts the normal functions of some of these neuronal networks.

Figure 2.1
A Neuron and its Parts.
The Primary Brain Regions Involved in Substance Use Disorders
The brain has many regions that are interconnected with one another, forming dynamic networks that are responsible for specific functions, such as attention, self-regulation, perception, language, reward, emotion, and movement, along with many other functions. This chapter focuses on three regions that are the key components of networks that are intimately involved in the development and persistence of substance use disorders: the basal ganglia, the extended amygdala, and the prefrontal cortex (Figure 2.2). The basal ganglia control the rewarding, or pleasurable, effects of substance use and are also responsible for the formation of habitual substance taking. The extended amygdala is involved in stress and the feelings of unease, anxiety, and irritability that typically accompany substance withdrawal. The prefrontal cortex is involved in executive function (i.e., the ability to organize thoughts and activities, prioritize tasks, manage time, and make decisions), including exerting control over substance taking.

Figure 2.2
Areas of the Human Brain that Are Especially Important in Addiction.
These brain areas and their associated networks are not solely involved in substance use disorders. Indeed, these systems are broadly integrated and serve many critical roles in helping humans and other animals survive. For example, when people engage in certain activities, such as consuming food or having sex, chemicals within the basal ganglia produce feelings of pleasure. This reward motivates individuals to continue to engage in these activities, thereby ensuring the survival of the species. Likewise, in the face of danger, activation of the brain's stress systems within the extended amygdala drives “fight or flight” responses. These responses, too, are critical for survival. As described in more detail below, these and other survival systems are “hijacked” by addictive substances.
The Basal Ganglia
The basal ganglia are a group of structures located deep within the brain that play an important role in keeping body movements smooth and coordinated. They are also involved in learning routine behaviors and forming habits. Two sub-regions of the basal ganglia are particularly important in substance use disorders:
- The nucleus accumbens, which is involved in motivation and the experience of reward, and
- The dorsal striatum, which is involved in forming habits and other routine behaviors.11
The Extended Amygdala
The extended amygdala and its sub-regions, located beneath the basal ganglia, regulate the brain's reactions to stress-including behavioral responses like “fight or flight” and negative emotions like unease, anxiety, and irritability. This region also interacts with the hypothalamus, an area of the brain that controls activity of multiple hormone-producing glands, such as the pituitary gland at the base of the brain and the adrenal glands at the top of each kidney. These glands, in turn, control reactions to stress and regulate many other bodily processes.12
The Prefrontal Cortex
The prefrontal cortex is located at the very front of the brain, over the eyes, and is responsible for complex cognitive processes described as “executive function.” Executive function is the ability to organize thoughts and activities, prioritize tasks, manage time, make decisions, and regulate one's actions, emotions, and impulses.13
The Addiction Cycle
Addiction can be described as a repeating cycle with three stages. Each stage is particularly associated with one of the brain regions described above—basal ganglia, extended amygdala, and prefrontal cortex (Figure 2.3).10 This three-stage model draws on decades of human and animal research and provides a useful way to understand the symptoms of addiction, how it can be prevented and treated, and how people can recover from it.14 The three stages of addiction are:

Figure 2.3
The Three Stages of the Addiction Cycle and the Brain Regions Associated with Them.
- Binge/Intoxication, the stage at which an individual consumes an intoxicating substance and experiences its rewarding or pleasurable effects;
- Withdrawal/Negative Affect, the stage at which an individual experiences a negative emotional state in the absence of the substance; and
- Preoccupation/Anticipation, the stage at which one seeks substances again after a period of abstinence.
The three stages are linked to and feed on each other, but they also involve different brain regions, circuits (or networks), and neurotransmitters and result in specific kinds of changes in the brain. A person may go through this three-stage cycle over the course of weeks or months or progress through it several times in a day. There may be variation in how people progress through the cycle and the intensity with which they experience each of the stages. Nonetheless, the addiction cycle tends to intensify over time, leading to greater physical and psychological harm.10
The following sections describe each of the stages in more detail. But first, it is necessary to explain four behaviors that are central to the addiction cycle: impulsivity, positive reinforcement, negative reinforcement, and compulsivity.
For many people, initial substance use involves an element of impulsivity, or acting without foresight or regard for the consequences. For example, an adolescent may impulsively take a first drink, smoke a cigarette, begin experimenting with marijuana, or succumb to peer pressure to try a party drug. If the experience is pleasurable, this feeling positively reinforces the substance use, making the person more likely to take the substance again.
Another person may take a substance to relieve negative feelings such as stress, anxiety, or depression. In this case, the temporary relief the substance brings from the negative feelings negatively reinforces substance use, increasing the likelihood that the person will use again. Importantly, positive and negative reinforcement need not be driven solely by the effects of the drugs. Many other environmental and social stimuli can reinforce a behavior. For example, the approval of peers positively reinforces substance use for some people. Likewise, if drinking or using drugs with others provides relief from social isolation, substance use behavior could be negatively reinforced.
KEY TERMS
Impulsivity. An inability to resist urges, deficits in delaying gratification, and unreflective decision-making. It is a tendency to act without foresight or regard for consequences and to prioritize immediate rewards over long-term goals.1
Positive reinforcement. The process by which presentation of a stimulus such as a drug increases the probability of a response like drug taking.
Negative reinforcement. The process by which removal of a stimulus such as negative feelings or emotions increases the probability of a response like drug taking.
Compulsivity. Repetitive behaviors in the face of adverse consequences, and repetitive behaviors that are inappropriate to a particular situation. People suffering from compulsions often recognize that the behaviors are harmful, but they nonetheless feel emotionally compelled to perform them. Doing so reduces tension, stress, or anxiety.1
The positively reinforcing effects of substances tend to diminish with repeated use. This is called tolerance and may lead to use of the substance in greater amounts and/or more frequently in an attempt to experience the initial level of reinforcement. Eventually, in the absence of the substance, a person may experience negative emotions such as stress, anxiety, or depression, or feel physically ill. This is called withdrawal, which often leads the person to use the substance again to relieve the withdrawal symptoms.
As use becomes an ingrained behavior, impulsivity shifts to compulsivity, and the primary drivers of repeated substance use shift from positive reinforcement (feeling pleasure) to negative reinforcement (feeling relief), as the person seeks to stop the negative feelings and physical illness that accompany withdrawal.15 Eventually, the person begins taking the substance not to get “high,” but rather to escape the “low” feelings to which, ironically, chronic drug use has contributed. Compulsive substance seeking is a key characteristic of addiction, as is the loss of control over use. Compulsivity helps to explain why many people with addiction experience relapses after attempting to abstain from or reduce use.
The following sections provide more detail about each of the three stages—binge/intoxication, withdrawal/negative affect, and preoccupation/anticipation—and the neurobiological processes underlying them.
Binge/Intoxication Stage: Basal Ganglia
The binge/intoxication stage of the addiction cycle is the stage at which an individual consumes the substance of choice. This stage heavily involves the basal ganglia (Figure 2.4) and its two key brain sub-regions, the nucleus accumbens and the dorsal striatum. In this stage, substances affect the brain in several ways.

Figure 2.4
The Binge/Intoxication Stage and the Basal Ganglia.
Addictive Substances “Hijack” Brain Reward Systems
All addictive substances produce feelings of pleasure. These “rewarding effects” positively reinforce their use and increase the likelihood of repeated use. The rewarding effects of substances involve activity in the nucleus accumbens, including activation of the brain's dopamine and opioid signaling system. Many studies have shown that neurons that release dopamine are activated, either directly or indirectly, by all addictive substances, but particularly by stimulants such as cocaine, amphetamines, and nicotine (Figure 2.5).16 In addition, the brain's opioid system, which includes naturally occurring opioid molecules (i.e., endorphins, enkephalins, and dynorphins) and three types of opioid receptors (i.e., mu, delta, and kappa), plays a key role in mediating the rewarding effects of other addictive substances, including opioids and alcohol. Activation of the opioid system by these substances stimulates the nucleus accumbens directly or indirectly through the dopamine system. Brain imaging studies in humans show activation of dopamine and opioid neurotransmitters during alcohol and other substance use (including nicotine).10,17 Other studies show that antagonists, or inhibitors, of dopamine and opioid receptors can block drug and alcohol seeking in both animals and humans.14,18,19

Figure 2.5
Actions of Addictive Substances on the Brain. Notes: Figure 2.5 is a simplified schematic of converging acute rewarding actions of addictive substances on the nucleus accumbens (NAc). Dopamine neurons that originate in the ventral tegmental area (VTA) (more...)
KEY TERMS
Antagonist. A chemical substance that binds to and blocks the activation of certain receptors on cells, preventing a biological response. Naloxone is an example of an opioid receptor antagonist.
Cannabinoids such as delta-9-tetrahydrocannabinol (THC), the primary psychoactive component of marijuana, target the brain's internal or endogenous cannabinoid system. This system also contributes to reward by affecting the function of dopamine neurons and the release of dopamine in the nucleus accumbens.
Stimuli Associated with Addictive Substances Can Trigger Substance Use
Activation of the brain's reward system by alcohol and drugs not only generates the pleasurable feelings associated with those substances, it also ultimately triggers changes in the way a person responds to stimuli associated with the use of those substances. A person learns to associate the stimuli present while using a substance—including people, places, drug paraphernalia, and even internal states, such as mood—with the substance's rewarding effects. Over time, these stimuli can activate the dopamine system on their own and trigger powerful urges to take the substance. These “wanting” urges are called incentive salience and they can persist even after the rewarding effects of the substance have diminished. As a result, exposure to people, places, or things previously associated with substance use can serve as “triggers” or cues that promote substance seeking and taking, even in people who are in recovery.
Figure 2.6 shows the major neurotransmitter systems involved in the binge/intoxication stage of addiction. In this stage, the neurons in the basal ganglia contribute to the rewarding effects of addictive substances and to incentive salience through the release of dopamine and the brain's natural opioids.

Figure 2.6
Major Neurotransmitter Systems Implicated in the Neuroadaptations Associated with the Binge/Intoxication Stage of Addiction. Notes: Blue represents the basal ganglia involved in the Binge/Intoxication stage. Red represents the extended amygdala involved (more...)
Early studies in animals demonstrated how incentive salience works. For example, after researchers repeatedly gave an animal a stimulant drug (e.g., cocaine) along with a previously neutral stimulus, such as a light or a sound, they found that the neutral stimulus by itself caused the animal to engage in drug-seeking behavior, and it also resulted in dopamine release that had previously occurred only in response to the drug.20 Even more compelling results were seen when scientists recorded the electrical activity of dopamine-transmitting neurons in animals that had been exposed multiple times to a neutral (non-drug) stimulus followed by a drug. At first, the neurons responded only when they were exposed to the drug. However, over time, the neurons stopped firing in response to the drug and instead fired when they were exposed to the neutral stimulus associated with it. This means that the animals associated the stimulus with the substance and, in anticipation of getting the substance, their brains began releasing dopamine, resulting in a strong motivation to seek the drug.21,22 Imaging studies in humans have shown similar results. For example, dopamine is released in the brains of people addicted to cocaine when they are exposed to cues they have come to associate with cocaine.23,24 This effect occurs even though cocaine itself causes less dopamine to be released in these individuals compared to those who are not addicted to cocaine (an effect also seen with other substances).25
Together, these studies indicate that stimuli associated with addictive drugs can, by themselves, produce drug-like effects on the brain and trigger drug use. These findings help to explain why individuals with substance use disorders who are trying to maintain abstinence are at increased risk of relapse if they continue to have contact with the people they previously used drugs with or the places where they used drugs.
Substances Stimulate Areas of the Brain Involved in Habit Formation
A second sub-region of the basal ganglia, the dorsal striatum, is involved in another critical component of the binge/intoxication stage: habit formation. The release of dopamine (along with activation of brain opioid systems) and release of glutamate (an excitatory neurotransmitter) can eventually trigger changes in the dorsal striatum.2,26 These changes strengthen substance-seeking and substance-taking habits as addiction progresses, ultimately contributing to compulsive use.
In Summary: The Binge/Intoxication Stage and the Basal Ganglia
The “reward circuitry” of the basal ganglia (i.e., the nucleus accumbens), along with dopamine and naturally occurring opioids, play a key role in the rewarding effects of alcohol and other substances and the ability of stimuli, or cues, associated with that substance use to trigger craving, substance seeking, and use.
As alcohol or substance use progresses, repeated activation of the “habit circuitry” of the basal ganglia (i.e., the dorsal striatum) contributes to the compulsive substance seeking and taking that are associated with addiction.
The involvement of these reward and habit neurocircuits helps explain the intense desire for the substance (craving) and the compulsive substance seeking that occurs when actively or previously addicted individuals are exposed to alcohol and/or drug cues in their surroundings.
Withdrawal/Negative Affect Stage: Extended Amygdala
The withdrawal/negative affect stage of addiction follows the binge/intoxication stage, and, in turn, sets up future rounds of binge/intoxication. During this stage, a person who has been using alcohol or drugs experiences withdrawal symptoms, which include negative emotions and, sometimes, symptoms of physical illness, when they stop taking the substance. Symptoms of withdrawal may occur with all addictive substances, including marijuana, though they vary in intensity and duration depending on both the type of substance and the severity of use. The negative feelings associated with withdrawal are thought to come from two sources: diminished activation in the reward circuitry of the basal ganglia14 and activation of the brain's stress systems in the extended amygdala (Figure 2.7).

Figure 2.7
The Withdrawal/Negative Affect Stage and the Extended Amygdala.
When used over the long-term, all substances of abuse cause dysfunction in the brain's dopamine reward system.27 For example, brain imaging studies in humans with addiction have consistently shown long-lasting decreases in a particular type of dopamine receptor, the D2 receptor, compared with non-addicted individuals (Figure 2.8).25,28 Decreases in the activity of the dopamine system have been observed during withdrawal from stimulants, opioids, nicotine, and alcohol. Other studies also show that when an addicted person is given a stimulant, it causes a smaller release of dopamine than when the same dose is given to a person who is not addicted.

Figure 2.8
Time-Related Decrease in Dopamine Released in the Brain of a Cocaine User. Notes: These fMRI images compare the brain of an individual with a history of cocaine use disorder (middle and right) to the brain of an individual without a history of cocaine (more...)
These findings suggest that people addicted to substances experience an overall reduction in the sensitivity of the brain's reward system (especially the brain circuits involving dopamine), both to addictive substances and also to natural reinforcers, such as food and sex. This is because natural reinforcers also depend upon the same reward system and circuits. This impairment explains why those who develop a substance use disorder often do not derive the same level of satisfaction or pleasure from once-pleasurable activities. This general loss of reward sensitivity may also account for the compulsive escalation of substance use as addicted individuals attempt to regain the pleasurable feelings the reward system once provided.15
At the same time, a second process occurs during the withdrawal stage: activation of stress neurotransmitters in the extended amygdala. These stress neurotransmitters include corticotropin-releasing factor (CRF), norepinephrine, and dynorphin (Figure 2.9).30

Figure 2.9
Major Neurotransmitter Systems Implicated in the Neuroadaptations Associated with the Withdrawal/Negative Affect Stage of Addiction. Notes: Not shown is the neurotransmitter norepinephrine which is also activated in the extended amygdala during withdrawal. (more...)
Studies suggest that these neurotransmitters play a key role in the negative feelings associated with withdrawal and in stress-triggered substance use. In animal and human studies, when researchers use special chemicals called antagonists to block activation of the stress neurotransmitter systems, it has the effect of reducing substance intake in response to withdrawal and stress. For example, blocking the activation of stress receptors in the brain reduced alcohol consumption in both alcohol-dependent rats and humans with an alcohol use disorder.31 Thus, it may be that an additional motivation for drug and alcohol seeking among individuals with substance use disorders is to suppress overactive brain stress systems that produce negative emotions or feelings. Recent research also suggests that neuroadaptations in the endogenous cannabinoid system within the extended amygdala contribute to increased stress reactivity and negative emotional states in addiction.32
The desire to remove the negative feelings that accompany withdrawal can be a strong motivator of continued substance use. As noted previously, this motivation is strengthened through negative reinforcement, because taking the substance relieves the negative feelings associated with withdrawal, at least temporarily. Of course, this process is a vicious cycle: Taking drugs or alcohol to lessen the symptoms of withdrawal that occur during a period of abstinence actually causes those symptoms to be even worse the next time a person stops taking the substance, making it even harder to maintain abstinence.
In Summary: The Withdrawal/Negative Affect Stage and the Extended Amygdala
This stage of addiction involves a decrease in the function of the brain reward systems and an activation of stress neurotransmitters, such as CRF and dynorphin, in the extended amygdala. Together, these phenomena provide a powerful neurochemical basis for the negative emotional state associated with withdrawal. The drive to alleviate these negative feelings negatively reinforces alcohol or drug use and drives compulsive substance taking.
Preoccupation/Anticipation Stage: Prefrontal Cortex
The preoccupation/anticipation stage of the addiction cycle is the stage in which a person may begin to seek substances again after a period of abstinence. In people with severe substance use disorders, that period of abstinence may be quite short (hours). In this stage, an addicted person becomes preoccupied with using substances again. This is commonly called “craving.” Craving has been difficult to measure in human studies and often does not directly link with relapse.
This stage of addiction involves the brain's prefrontal cortex (Figure 2.10) the region that controls executive function: the ability to organize thoughts and activities, prioritize tasks, manage time, make decisions, and regulate one's own actions, emotions, and impulses. Executive function is essential for a person to make appropriate choices about whether or not to use a substance and to override often strong urges to use, especially when the person experiences triggers, such as stimuli associated with that substance (e.g., being at a party where alcohol is served or where people are smoking) or stressful experiences.

Figure 2.10
The Preoccupation/Anticipation Stage and the Prefrontal Cortex.
To help explain how the prefrontal cortex is involved in addiction, some scientists divide the functions of this brain region into a “Go system” and an opposing “Stop system.”33 The Go system helps people make decisions, particularly those that require significant attention and those involved with planning. People also engage the Go system when they begin behaviors that help them achieve goals. Indeed, research shows that when substance-seeking behavior is triggered by substance-associated environmental cues (incentive salience), activity in the Go circuits of the prefrontal cortex increases dramatically. This increased activity stimulates the nucleus accumbens to release glutamate, the main excitatory neurotransmitter in the brain. This release, in turn, promotes incentive salience, which creates a powerful urge to use the substance in the presence of drug-associated cues.
The Go system also engages habit-response systems in the dorsal striatum, and it contributes to the impulsivity associated with substance seeking. Habitual responding can occur automatically and subconsciously, meaning a person may not even be aware that they are engaging in such behaviors. The neurons in the Go circuits of the prefrontal cortex stimulate the habit systems of the dorsal striatum through connections that use glutamate (Figure 2.11).

Figure 2.11
Major Neurotransmitter Systems Implicated in the Neuroadaptations Associated with the Preoccupation/Anticipation Stage of Addiction. Abbreviations: PFC - prefrontal cortex, DS - dorsal striatum, NAc - nucleus accumbens, BNST - bed nucleus of the stria (more...)
The Stop system inhibits the activity of the Go system. Especially relevant to its role in addiction, this system controls the dorsal striatum and the nucleus accumbens, the areas of the basal ganglia that are involved in the binge/intoxication stage of addiction. Specifically, the Stop system controls habit responses driven by the dorsal striatum, and scientists think that it plays a role in reducing the ability of substance-associated stimuli to trigger relapse—in other words, it inhibits incentive salience.34
The Stop system also controls the brain's stress and emotional systems, and plays an important role in relapse triggered by stressful life events or circumstances. Stress-induced relapse is driven by activation of neurotransmitters such as CRF, dynorphin, and norepinephrine in the extended amygdala. As described above, these neurotransmitters are activated during prolonged abstinence during the withdrawal/negative affect stage of addiction. More recent work in animals also implicates disruptions in the brain's cannabinoid system, which also regulates the stress systems in the extended amygdala, in relapse. Studies show that lower activity in the Stop component of the prefrontal cortex is associated with increased activity of stress circuitry involving the extended amygdala, and this increased activity drives substance-taking behavior and relapse.37
Brain imaging studies in people with addiction show disruptions in the function of both the Go and Stop circuits.35-37 For example, people with alcohol, cocaine, or opioid use disorders show impairments in executive function, including disruption of decision-making and behavioral inhibition. These executive function deficits parallel changes in the prefrontal cortex and suggest decreased activity in the Stop system and greater reactivity of the Go system in response to substance-related stimuli.
Indeed, a smaller volume of the prefrontal cortex in abstinent, previously addicted individuals predicts a shorter time to relapse.38 Studies also show that diminished prefrontal cortex control over the extended amygdala is particularly prominent in humans with post-traumatic stress disorder (PTSD), a condition that is frequently accompanied by drug and alcohol use disorders.39 These findings bolster support for the role of the prefrontal cortex-extended amygdala circuit in stress-induced relapse, and suggest that strengthening prefrontal cortex circuits could aid substance use disorder treatment.
In Summary: The Preoccupation/Anticipation Stage and the Prefrontal Cortex
This stage of the addiction cycle is characterized by a disruption of executive function caused by a compromised prefrontal cortex. The activity of the neurotransmitter glutamate is increased, which drives substance use habits associated with craving, and disrupts how dopamine influences the frontal cortex.2 The over-activation of the Go system in the prefrontal cortex promotes habit-like substance seeking, and the under-activation of the Stop system of the prefrontal cortex promotes impulsive and compulsive substance seeking.
To recap, addiction involves a three-stage cycle—binge/intoxication, withdrawal/negative affect, and preoccupation/anticipation—that worsens over time and involves dramatic changes in the brain reward, stress, and executive function systems. Progression through this cycle involves three major regions of the brain: the basal ganglia, the extended amygdala, and the prefrontal cortex, as well as multiple neurotransmitter systems (Figure 2.12). The power of addictive substances to produce positive feelings and relieve negative feelings fuels the development of compulsive use of substances. The combination of increased incentive salience (binge/intoxication stage), decreased reward sensitivity and increased stress sensitivity (withdrawal/negative affect stage), and compromised executive function (preoccupation/anticipation stage) provides an often overwhelming drive for substance seeking that can be unrelenting.

Figure 2.12
The Primary Brain Regions and Neurotransmitter Systems Involved in Each of the Three Stages of the Addiction Cycle.
Different Classes of Substances Affect the Brain and Behavior in Different Ways
Although the three stages of addiction generally apply to all addictive substances, different substances affect the brain and behavior in different ways during each stage of the addiction cycle. Differences in the pharmacokinetics of various substances determine the duration of their effects on the body and partly account for the differences in their patterns of use. For example, nicotine has a short half-life, which means smokers need to smoke often to maintain the effect. In contrast, THC, the primary psychoactive compound in marijuana, has a much longer half-life. As a result, marijuana smokers do not typically smoke as frequently as tobacco smokers.40 Typical patterns of use are described below for the major classes of addictive substances. However, people often use these substances in combination.41 Additional research is needed to understand how using more than one substance affects the brain and the development and progression of addiction, as well as how use of one substance affects the use of others.
KEY TERMS
Pharmacokinetics. What the body does to a drug after it has been taken, including how rapidly the drug is absorbed, broken down, and processed by the body.
Opioids
Opioids attach to opioid receptors in the brain, which leads to a release of dopamine in the nucleus accumbens, causing euphoria (the high), drowsiness, and slowed breathing, as well as reduced pain signaling (which is why they are frequently prescribed as pain relievers). Opioid addiction typically involves a pattern of: (1) intense intoxication, (2) the development of tolerance, (3) escalation in use, and (4) withdrawal signs that include profound negative emotions and physical symptoms, such as bodily discomfort, pain, sweating, and intestinal distress and, in the most severe cases, seizures. As use progresses, the opioid must be taken to avoid the severe negative effects that occur during withdrawal. With repeated exposure to opioids, stimuli associated with the pleasant effects of the substances (e.g., places, persons, moods, and paraphernalia) and with the negative mental and physical effects of withdrawal can trigger intense craving or preoccupation with use.
Alcohol
When alcohol is consumed it interacts with several neurotransmitter systems in the brain, including the inhibitory neurotransmitter GABA, glutamate, and others that produce euphoria as well as the sedating, motor impairing, and anxiety-reducing effects of alcohol intoxication. Alcohol addiction often involves a similar pattern as opioid addiction, often characterized by periods of binge or heavy drinking followed by withdrawal. As with opioids, addiction to alcohol is characterized by intense craving that is often driven by negative emotional states, positive emotional states, and stimuli that have been associated with drinking, as well as a severe emotional and physical withdrawal syndrome. Many people with severe alcohol use disorder engage in patterns of binge drinking followed by withdrawal for extended periods of time. Extreme patterns of use may evolve into an opioid-like use pattern in which alcohol must be available at all times to avoid the negative consequences of withdrawal.
KEY TERMS
Binge drinking. For men, drinking 5 or more standard alcoholic drinks, and for women, 4 or more standard alcoholic drinks on the same occasion on at least 1 day in the past 30 days.
Stimulants
Stimulants increase the amount of dopamine in the reward circuit (causing the euphoric high) either by directly stimulating the release of dopamine or by temporarily inhibiting the removal of dopamine from synapses, the gaps between neurons. These drugs also boost dopamine levels in brain regions responsible for attention and focus on tasks (which is why stimulants like methylphenidate [Ritalin®] or dextroamphetamine [Adderall®] are often prescribed for people with attention deficit hyperactivity disorder). Stimulants also cause the release of norepinephrine, a neurotransmitter that affects autonomic functions like heart rate, causing a user to feel energized.
Addiction to stimulants, such as cocaine and amphetamines (including methamphetamine), typically follows a pattern that emphasizes the binge/intoxication stage. A person will take the stimulant repeatedly during a concentrated period of time lasting for hours or days (these episodes are called binges). The binge is often followed by a crash, characterized by negative emotions, fatigue, and inactivity. Intense craving then follows, which is driven by environmental cues associated with the availability of the substance, as well as by a person's internal state, such as their emotions or mood.
Marijuana (Cannabis)
Like other drugs, marijuana (also called cannabis) leads to increased dopamine in the basal ganglia, producing the pleasurable high. It also interacts with a wide variety of other systems and circuits in the brain that contain receptors for the body's natural cannabinoid neurotransmitters. Effects can be different from user to user, but often include distortions in motor coordination and time perception. Cannabis addiction follows a pattern similar to opioids. This pattern involves a significant binge/intoxication stage characterized by episodes of using the substance to the point of intoxication. Over time, individuals begin to use the substance throughout the day and show chronic intoxication during waking hours. Withdrawal is characterized by negative emotions, irritability, and sleep disturbances.40 Although the craving associated with cannabis42 has been less studied than for other substances, it is most likely linked to both environmental and internal states, similar to those of other addictive substances.43,44
Synthetic Drugs
Different classes of chemically synthesized (hence the term synthetic) drugs have been developed, each used in different ways and having different effects in the brain. Synthetic cathinones, more commonly known as “bath salts,” target the release of dopamine in a similar manner as the stimulant drugs described above. To a lesser extent, they also activate the serotonin neurotransmitter system, which can affect perception. Synthetic cannabinoids, sometimes referred to as “K2”, “Spice”, or “herbal incense,” somewhat mimic the effects of marijuana but are often much more powerful. Drugs such as MDMA (ecstasy) and lysergic acid diethylamide (LSD) also act on the serotonin neurotransmitter system to produce changes in perception. Fentanyl is a synthetic opioid medication that is used for severe pain management and is considerably more potent than heroin. Prescription fentanyl, as well as illicitly manufactured fentanyl and related synthetic opioids, are often mixed with heroin but are also increasingly used alone or sold on the street as counterfeit pills made to look like prescription opioids or sedatives.
Factors that Increase Risk for Substance Use, Misuse, and Addiction
Not all people use substances, and even among those who use them, not all are equally likely to become addicted. Many factors influence the development of substance use disorders, including developmental, environmental, social, and genetic factors, as well as co-occurring mental disorders. Other factors protect people from developing a substance use disorder or addiction. The relative influence of these risk and protective factors varies across individuals and the lifespan. The following sections discuss some of these factors.
Early Life Experiences
The experiences a person has early in childhood and in adolescence can set the stage for future substance use and, sometimes, escalation to a substance use disorder or addiction. Early life stressors can include physical, emotional, and sexual abuse; neglect; household instability (such as parental substance use and conflict, mental illness, or incarceration of household members);45 and poverty.46 Research suggests that the stress caused by these risk factors may act on the same stress circuits in the brain as addictive substances, which may explain why they increase addiction risk.47
FOR MORE ON THIS TOPIC
See Chapter 1 - Introduction and Overview and Chapter 3 - Prevention Programs and Policies.
Adolescence is a critical period in the vulnerability to substance use and use disorders, because a hallmark of this developmental period is risk taking and experimentation, which for some young people includes trying alcohol, marijuana, or other drugs. In addition, the brain undergoes significant changes during this life stage, making it particularly vulnerable to substance exposure.48 Importantly, the frontal cortex—a region in the front part of the brain that includes the prefrontal cortex—does not fully develop until the early to mid-20s, and research shows that heavy drinking and drug use during adolescence affects development of this critical area of the brain.49
About three quarters (74 percent) of 18- to 30-year-olds admitted to treatment programs began using substances at the age of 17 or younger.50 Individuals who start using substances during adolescence often experience more chronic and intensive use, and they are at greater risk of developing a substance use disorder compared with those who begin use at an older age. In other words, the earlier the exposure, the greater the risk.51
Not all adolescents who experiment with alcohol, cigarettes, or other substances go on to develop a substance use disorder, but research suggests that those who do progress to more harmful use may have pre-existing differences in their brains. For example, a brain imaging study of adolescents revealed that the volume of the frontal cortex was smaller in youth who transitioned from no or minimal drinking to heavy drinking over the course of adolescence than it was in youth who did not drink during adolescence.49 Additional research can shed light on how these differences contribute to the progression from use to a disorder, as well as how changes caused by substance use affect brain function and behavior and whether they can be reversed.
Genetic and Molecular Factors
Genetic factors are thought to account for 40 to 70 percent of individual differences in risk for addiction.52,53 Although multiple genes are likely involved in the development of addiction, only a few specific gene variants have been identified that either predispose to or protect against addiction. Some of these variants have been associated with the metabolism of alcohol and nicotine, while others involve receptors and other proteins associated with key neurotransmitters and molecules involved in all parts of the addiction cycle.54 Genes involved in strengthening the connections between neurons and in forming drug memories have also been associated with addiction risk.55,56 Like other chronic health conditions, substance use disorders are influenced by the complex interplay between a person's genes and environment. Additional research on the mechanisms underlying gene by environment interactions is expected to provide insight into how substance use disorders develop and how they can be prevented and treated.
Use of Multiple Substances and Co-occurring Mental Health Conditions
Many individuals with a substance use disorder also have a mental disorder,57,58 and some have multiple substance use disorders. For example, according to the 2015 National Survey on Drug Use and Health (NSDUH), of the 20.8 million people aged 12 or older who had a substance use disorder during the past year, about 2.7 million (13 percent) had both an alcohol use and an illicit drug use disorder, and 41.2 percent also had a mental illness.59 Particularly striking is the 3- to 4-fold higher rate of tobacco smoking among patients with schizophrenia and the high prevalence of co-existing alcohol use disorder in those meeting criteria for PTSD. It is estimated that 30-60 percent of patients seeking treatment for alcohol use disorder meet criteria for PTSD,60,61 and approximately one third of individuals who have experienced PTSD have also experienced alcohol dependence at some point in their lives.60
The reasons why substance use disorders and mental disorders often occur together are not clear, and establishing the relationships between these conditions is difficult. Still, three possible explanations deserve attention. One reason for the overlap may be that having a mental disorder increases vulnerability to substance use disorders because certain substances may, at least temporarily, be able to reduce mental disorder symptoms and thus are particularly negatively reinforcing in these individuals. Second, substance use disorders may increase vulnerability for mental disorders,62-64 meaning that the use of certain substances might trigger a mental disorder that otherwise would have not occurred. For example, research suggests that alcohol use increases risk for PTSD by altering the brain's ability to recover from traumatic experiences.65,66 Similarly, the use of marijuana, particularly marijuana with a high THC content, might contribute to schizophrenia in those who have specific genetic vulnerabilities.67 Third, it is also possible that both substance use disorders and mental disorders are caused by shared, overlapping factors, such as particular genes, neurobiological deficits, and exposure to traumatic or stressful life experiences. As these possibilities are not mutually exclusive, the relationship between substance use disorders and mental disorders may result from a combination of these processes.
Regardless of which one might influence the development of the other, mental and substance use disorders have overlapping symptoms, making diagnosis and treatment planning particularly difficult. For example, people who use methamphetamine for a long time may experience paranoia, hallucinations, and delusions that may be mistaken for symptoms of schizophrenia. And, the psychological symptoms that accompany withdrawal, such as depression and anxiety, may be mistaken as simply part of withdrawal instead of an underlying mood disorder that requires independent treatment in its own right. Given the prevalence of co-occurring substance use and mental disorders, it is critical to continue to advance research on the genetic, neurobiological, and environmental factors that contribute to co-occurring disorders and to develop interventions to prevent and treat them.
Biological Factors Contributing to Population-based Differences in Substance Misuse and Substance Use Disorders
Differences Based on Sex
Some groups of people are also more vulnerable to substance misuse and substance use disorders. For example, men tend to drink more than women and they are at higher risk for alcohol use disorder, although the gender differences in alcohol use are declining.68 Men are also more likely to have other substance use disorders.69 However, clinical reports suggest that women who use cocaine, opioids, or alcohol progress from initial use to a disorder at a faster rate than do men (called “telescoping”).70-72 Compared with men, women also exhibit greater symptoms of withdrawal from some drugs, such as nicotine. They also report worse negative affects during withdrawal and have higher levels of the stress hormone cortisol.73
Sex differences in reaction to addictive substances are not particular to humans. Female rats, in general, learn to self-administer drugs and alcohol more rapidly, escalate their drug taking more quickly, show greater symptoms of withdrawal, and are more likely to resume drug seeking in response to drugs, drug-related cues, or stressors. The one exception is that female rats show less withdrawal symptoms related to alcohol use.74 Researchers are investigating the neurobiological bases for these differences.
Differences Based on Race and Ethnicity
Research on the neurobiological factors contributing to differential rates of substance use and substance use disorders in particular racial and ethnic groups is much more limited. A study using functional magnetic resonance imaging (fMRI) found that African American smokers showed greater activation of the prefrontal cortex upon exposure to smoking-related cues than did White smokers, an effect that may partly contribute to the lower smoking-cessation success rates observed among African Americans.75
Alcohol research with racial and ethnic groups has shown that approximately 36 percent of East Asians carry a gene variant that alters the rate at which members of that racial group metabolize alcohol, causing a buildup of acetaldehyde, a toxic byproduct of alcohol metabolism that produces symptoms such as flushing, nausea, and rapid heartbeat. Although these effects may protect some individuals of East Asian descent from alcohol use disorder, those who drink despite the effects are at increased risk for esophageal76 and head and neck cancers.77 Another study found that even low levels of alcohol consumption by Japanese Americans may result in adverse effects on the brain, a finding that may be related to the differences in alcohol metabolism described above.78 Additional research will help to clarify the interactions between race, ethnicity, and the neuroadaptations that underlie substance misuse and addiction. This work may inform the development of more precise preventive and treatment interventions.
Recommendations for Research
Decades of research demonstrate that chronic substance misuse leads to profound disruptions of brain circuits involved in the experience of pleasure or reward, habit formation, stress, and decision-making. This work has paved the way for the development of a variety of therapies that effectively help people reduce or abstain from alcohol and drug misuse and regain control over their lives. In spite of this progress, our understanding of how substance use affects the brain and behavior is far from complete. Four research areas are specifically emphasized in the text below.
Effects of Substance Use on Brain Circuits and Functions
Continued research is necessary to more thoroughly explain how substance use affects the brain at the molecular, cellular, and circuit levels. Such research has the potential to identify common neurobiological mechanisms underlying substance use disorders, as well as other related mental disorders. This research is expected to reveal new neurobiological targets, leading to new medications and non-pharmacological treatments—such as transcranial magnetic stimulation or vaccines—for the treatment of substance use disorders. A better understanding of the neurobiological mechanisms underlying substance use disorders could also help to inform behavioral interventions. Therefore, basic research that further elucidates the neurobiological framework of substance use disorders and co-occurring mental disorders, as well as research leading to the development of new medications and other therapeutics to treat the underlying neurobiological mechanisms of substance use disorders should be accelerated.
As with other diseases, individuals vary in the development and progression of substance use disorders. Not only are some people more likely to use and misuse substances than are others and to progress from initial use to addiction differently, individuals also differ in their vulnerability to relapse and in how they respond to treatments. For example, some people with substance use disorders are particularly vulnerable to stress-induced relapse, but others may be more likely to resume substance use after being exposed to drug-related cues. Developing a thorough understanding of how neurobiological differences account for variation among individuals and groups will guide the development of more effective, personalized prevention and treatment interventions. Additionally, determining how neurobiological factors contribute to differences in substance misuse and addiction between women and men and among racial and ethnic groups is critical.
Continued advances in neuroscience research will further enhance our understanding of substance use disorders and accelerate the development of new interventions. Data gathered through the National Institutes of Health's Adolescent Brain Cognitive Development study, the largest long-term study of cognitive and brain development in children across the United States, is expected to yield unprecedented information about how substance use affects adolescent brain development. The Human Connectome Project and the Brain Research through Advancing Innovative Neurotechnologies (BRAIN) initiative are poised to spur an explosion of knowledge about the structure and function of brain circuits and how the brain affects behavior. Technologies that can alter the activity of dysfunctional circuits are being explored as possible treatments. Moreover, continued advances in genomics, along with President Obama's Precision Medicine Initiative, a national effort to better understand how individual variability in genes, environment, and lifestyle contribute to disease, are expected to bring us closer to developing individually-tailored preventive and treatment interventions for substance-related conditions.
Neurobiological Effects of Recovery
Little is known about the factors that facilitate or inhibit long-term recovery from substance use disorders or how the brain changes over the course of recovery. Developing a better understanding of the recovery process, and the neurobiological mechanisms that enable people to maintain changes in their substance use behavior and promote resilience to relapse, will inform the development of additional effective treatment and recovery support interventions. Therefore, an investigation of the neurobiological processes that underlie recovery and contribute to improvements in social, educational, and professional functioning is necessary.
Adolescence, Brain Change, and Vulnerability to Substance Use Disorders
Although young people are particularly vulnerable to the adverse effects of substance use, not all adolescents who experiment with alcohol or drugs go on to develop a substance use disorder. Prospective, longitudinal studies are needed to investigate whether pre-existing neurobiological factors contribute to adolescent substance use and the development of substance use disorders, how adolescent substance use affects brain structure and function, and whether the changes in brain structure and function that accompany chronic substance use can recover over time. Studies that follow groups of adolescents over time to learn about the developing human brain should be conducted. These studies should investigate how pre-existing neurobiological factors contribute to substance use, misuse, and addiction, and how adolescent substance use affects brain function and behavior.
KEY TERMS
Longitudinal study. A type of study in which data on a particular group of people are gathered repeatedly over a period of years or even decades.
Neurobiological Effects of Polysubstance Use and Emerging Drug Products
Patterns of alcohol and drug use change over time. New drugs or drug combinations, delivery systems, and routes of administration emerge, and with them new questions for public health. For example, concern is growing that increasing use of marijuana extracts with extremely high amounts of THC could lead to higher rates of addiction among marijuana users. Concerns also are emerging about how new products about which little is known, such as synthetic cannabinoids and synthetic cathinones, affect the brain. Additional research is needed to better understand how such products - as well as emerging addictive substances - affect brain function and behavior, and contribute to addiction.
References
- 1.
- Berlin GS, Hollander E. Compulsivity, impulsivity, and the DSM-5 process. CNS Spectrums. 2014;19(1):62–68. [PubMed: 24229702]
- 2.
- Kalivas PW. The glutamate homeostasis hypothesis of addiction. Nature Reviews Neuroscience. 2009;10(8):561–572. [PubMed: 19571793]
- 3.
- American Psychiatric Association. Diagnostic and statistical manual of mental disorders (DSM-5). 5th ed. Arlington, VA: American Psychiatric Publishing; 2013.
- 4.
- McLellan AT, Lewis DC, O'Brien CP, Kleber HD. Drug dependence, a chronic medical illness: Implications for treatment, insurance, and outcomes evaluation. JAMA. 2000;284(13):1689–1695. [PubMed: 11015800]
- 5.
- Volkow ND, Koob GF, McLellan AT. Neurobiologic advances from the brain disease model of addiction. New England Journal of Medicine. 2016;374(4):363–371. [PMC free article: PMC6135257] [PubMed: 26816013]
- 6.
- Hubbard RL, Craddock SG, Anderson J. Overview of 5-year followup outcomes in the drug abuse treatment outcome studies (DATOS). Journal of Substance Abuse Treatment. 2003;25(3):125–134. [PubMed: 14670518]
- 7.
- Hser Y-I, Hoffman V, Grella CE, Anglin MD. A 33-year follow-up of narcotics addicts. Archives of General Psychiatry. 2001;58(5):503–508. [PubMed: 11343531]
- 8.
- Vaillant GE. The natural history of alcoholism revisited. Cambridge, MA: Harvard University Press; 1995.
- 9.
- Stone AL, Becker LG, Huber AM, Catalano RF. Review of risk and protective factors of substance use and problem use in emerging adulthood. Addictive Behaviors. 2012;37(7):747–775. [PubMed: 22445418]
- 10.
- Koob GF, Le Moal M. Drug abuse: Hedonic homeostatic dysregulation. Science. 1997;278(5335):52–58. [PubMed: 9311926]
- 11.
- Kalivas PW, Volkow ND. The neural basis of addiction: A pathology of motivation and choice. The American Journal of Psychiatry. 2005;162(8):1403–1413. [PubMed: 16055761]
- 12.
- Davis M, Walker DL, Miles L, Grillon C. Phasic vs sustained fear in rats and humans: Role of the extended amygdala in fear vs anxiety. Neuropsychopharmacology. 2010;35(1):105–135. [PMC free article: PMC2795099] [PubMed: 19693004]
- 13.
- Ball G, Stokes PR, Rhodes RA, Bose SK, Rezek I, Wink A-M, Turkheimer FE. Executive functions and prefrontal cortex: A matter of persistence? Frontiers in Systems Neuroscience. 2011;5(3):1–13. [PMC free article: PMC3031025] [PubMed: 21286223]
- 14.
- Koob GF, Volkow ND. Neurocircuitry of addiction. Neuropsychopharmacology. 2010;35(1):217–238. [PMC free article: PMC2805560] [PubMed: 19710631]
- 15.
- Koob GF, Le Moal M. Drug addiction, dysregulation of reward, and allostasis. Neuropsychopharmacology. 2001;24(2):97–129. [PubMed: 11120394]
- 16.
- Nestler EJ. Is there a common molecular pathway for addiction? Nature Neuroscience. 2005;8(11):1445–1449. [PubMed: 16251986]
- 17.
- Clapp P, Bhave SV, Hoffman PL. How adaptation of the brain to alcohol leads to dependence: A pharmacological perspective. Alcohol Research & Health. 2008;31(4):310–339. [PMC free article: PMC2923844] [PubMed: 20729980]
- 18.
- Moreira FA, Dalley JW. Dopamine receptor partial agonists and addiction. European Journal of Pharmacology. 2015;752:112–115. [PubMed: 25724788]
- 19.
- Swift RM, Aston ER. Pharmacotherapy for alcohol use disorder: Current and emerging therapies. Harvard Review of Psychiatry. 2015;23(2):122–133. [PMC free article: PMC4790835] [PubMed: 25747925]
- 20.
- Uslaner JM, Acerbo MJ, Jones SA, Robinson TE. The attribution of incentive salience to a stimulus that signals an intravenous injection of cocaine. Behavioural Brain Research. 2006;169(2):320–324. [PubMed: 16527365]
- 21.
- Schultz W, Dayan P, Montague PR. A neural substrate of prediction and reward. Science. 1997;275(5306):1593–1599. [PubMed: 9054347]
- 22.
- Phillips PE, Stuber GD, Heien ML, Wightman RM, Carelli RM. Subsecond dopamine release promotes cocaine seeking. Nature. 2003;422(6932):614–618. [PubMed: 12687000]
- 23.
- Volkow ND, Wang G-J, Telang F, Fowler JS, Logan J, Childress A-R, Wong C. Cocaine cues and dopamine in dorsal striatum: Mechanism of craving in cocaine addiction. The Journal of Neuroscience. 2006;26(24):6583–6588. [PMC free article: PMC6674019] [PubMed: 16775146]
- 24.
- Wong DF, Kuwabara H, Schretlen DJ, Bonson KR, Zhou Y, Nandi A, Kumar A. Increased occupancy of dopamine receptors in human striatum during cue-elicited cocaine craving. Neuropsychopharmacology. 2006;31(12):2716–2727. [PubMed: 16971900]
- 25.
- Volkow ND, Tomasi D, Wang GJ, Logan J, Alexoff DL, Jayne M, Du C. Stimulant-induced dopamine increases are markedly blunted in active cocaine abusers. Molecular Psychiatry. 2014;19(9):1037–1043. [PMC free article: PMC4827430] [PubMed: 24912491]
- 26.
- Belin D, Jonkman S, Dickinson A, Robbins TW, Everitt BJ. Parallel and interactive learning processes within the basal ganglia: Relevance for the understanding of addiction. Behavioural Brain Research. 2009;199(1):89–102. [PubMed: 18950658]
- 27.
- Volkow ND, Morales M. The brain on drugs: From reward to addiction. Cell. 2015;162(4):712–725. [PubMed: 26276628]
- 28.
- Volkow ND, Wang G-J, Fowler JS, Logan J, Gatley SJ, Hitzemann R, Pappas N. Decreased striatal dopaminergic responsiveness in detoxified cocaine-dependent subjects. Nature. 1997;386(6627):830–833. [PubMed: 9126741]
- 29.
- Volkow ND, Fowler JS, Wang GJ, Hitzemann R, Logan J, Schlyer DJ, Wolf AP. Decreased dopamine D2 receptor availability is associated with reduced frontal metabolism in cocaine abusers. Synapse. 1993;14(2):169–177. [PubMed: 8101394]
- 30.
- Koob GF, Le Moal M. Plasticity of reward neurocircuitry and the ‘dark side’ of drug addiction. Nature Neuroscience. 2005;8(11):1442–1444. [PubMed: 16251985]
- 31.
- Vendruscolo LF, Estey D, Goodell V, Macshane LG, Logrip ML, Schlosburg JE, Hunt HJ. Glucocorticoid receptor antagonism decreases alcohol seeking in alcohol-dependent individuals. The Journal of Clinical Investigation. 2015;125(8):3193–3197. [PMC free article: PMC4563748] [PubMed: 26121746]
- 32.
- Parsons LH, Hurd YL. Endocannabinoid signalling in reward and addiction. Nature Reviews Neuroscience. 2015;16(10):579–594. [PMC free article: PMC4652927] [PubMed: 26373473]
- 33.
- Koob GF, Arends MA, Le Moal M. Drugs, addiction, and the brain. Waltham, MA: Academic Press; 2014.
- 34.
- Goldstein RZ, Volkow ND. Dysfunction of the prefrontal cortex in addiction: Neuroimaging findings and clinical implications. Nature Reviews Neuroscience. 2011;12(11):652–669. [PMC free article: PMC3462342] [PubMed: 22011681]
- 35.
- Crunelle CL, Kaag AM, van den Munkhof HE, Reneman L, Homberg JR, Sabbe B, van Wingen G. Dysfunctional amygdala activation and connectivity with the prefrontal cortex in current cocaine users. Human Brain Mapping. 2015;36(10):4222–4230. [PMC free article: PMC6869379] [PubMed: 26220024]
- 36.
- Goldstein RZ, Volkow ND. Drug addiction and its underlying neurobiological basis: Neuroimaging evidence for the involvement of the frontal cortex. American Journal of Psychiatry. 2002;159(10):1642–1652. [PMC free article: PMC1201373] [PubMed: 12359667]
- 37.
- Volkow ND, Wang G-J, Telang F, Fowler JS, Logan J, Jayne M, Wong C. Profound decreases in dopamine release in striatum in detoxified alcoholics: Possible orbitofrontal involvement. The Journal of Neuroscience. 2007;27(46):12700–12706. [PMC free article: PMC6673312] [PubMed: 18003850]
- 38.
- Rando K, Hong K-I, Bhagwagar Z, Li C-SR, Bergquist K, Guarnaccia J, Sinha R. Association of frontal and posterior cortical gray matter volume with time to alcohol relapse: A prospective study. American Journal of Psychiatry. 2011;168(2):183–192. [PMC free article: PMC3668974] [PubMed: 21078704]
- 39.
- Mahan AL, Ressler KJ. Fear conditioning, synaptic plasticity and the amygdala: Implications for posttraumatic stress disorder. Trends in Neurosciences. 2012;35(1):24–35. [PMC free article: PMC3206195] [PubMed: 21798604]
- 40.
- Koob GF, Karde DB, Baler RD, Volkow ND. Pathopsychology of addiction. In: Tasman A, Kay J, Lieberman JA, First MB, Riba M, editors. Psychiatry. 4th ed. Vol. 1. New York, NY: Wiley-Blackwell; 2015.
- 41.
- Connor JP, Gullo MJ, White A, Kelly AB. Polysubstance use: Diagnostic challenges, patterns of use and health. Current Opinion in Psychiatry. 2014;27(4):269–275. [PubMed: 24852056]
- 42.
- Heishman SJ, Singleton EG, Liguori A. Marijuana craving questionnaire: Development and initial validation of a self-report instrument. Addiction. 2001;96(7):1023–1034. [PubMed: 11440613]
- 43.
- Lundahl LH, Johanson CE. Cue-induced craving for marijuana in cannabis-dependent adults. Experimental and Clinical Psychopharmacology. 2011;19(3):224–230. [PMC free article: PMC5113719] [PubMed: 21480734]
- 44.
- Buckner JD, Zvolensky MJ, Ecker AH, Jeffries ER. Cannabis craving in response to laboratory-induced social stress among racially diverse cannabis users: The impact of social anxiety disorder. Journal of Psychopharmacology. 2016;30(4):363–369. [PMC free article: PMC5147424] [PubMed: 26839322]
- 45.
- Dube SR, Felitti VJ, Dong M, Chapman DP, Giles WH, Anda RF. Childhood abuse, neglect, and household dysfunction and the risk of illicit drug use: The adverse childhood experiences study. Pediatrics. 2003;111(3):564–572. [PubMed: 12612237]
- 46.
- Najavits LM, Hyman SM, Ruglass LM, Hien DA, Read JP. Substance use disorder and trauma. In: Gold S, Cook J, Dalenberg C, editors. Handbook of trauma psychology. Washington, DC: American Psychological Association; (In press)
- 47.
- Teicher MH, Samson JA. Childhood maltreatment and psychopathology: A case for ecophenotypic variants as clinically and neurobiologically distinct subtypes. American Journal of Psychiatry. 2013;170(10):1114–1133. [PMC free article: PMC3928064] [PubMed: 23982148]
- 48.
- Giedd JN, Blumenthal J, Jeffries NO, Castellanos FX, Liu H, Zijdenbos A, Rapoport JL. Brain development during childhood and adolescence: A longitudinal MRI study. Nature Neuroscience. 1999;2(10):861–863. [PubMed: 10491603]
- 49.
- Squeglia LM, Tapert SF, Sullivan EV, Jacobus J, Meloy MJ, Rolfng T, Pfefferbaum A. Brain development in heavy-drinking adolescents. American Journal of Psychiatry. 2015;172(6):532–542. [PMC free article: PMC4451385] [PubMed: 25982660]
- 50.
- Substance Abuse and Mental Health Services Administration, & Center for Behavioral Health Statistics and Quality. The TEDS Report: Age of substance use initiation among treatment admissions aged 18 to 30. Rockville, MD: Substance Abuse and Mental Health Services Administration; 2014. [PubMed: 27631064]
- 51.
- Hanson KL, Medina KL, Padula CB, Tapert SF, Brown SA. Impact of adolescent alcohol and drug use on neuropsychological functioning in young adulthood: 10-year outcomes. Journal of Child and Adolescent Substance Abuse. 2011;20(2):135–154. [PMC free article: PMC3083020] [PubMed: 21532924]
- 52.
- Prescott CA, Kendler KS. Genetic and environmental contributions to alcohol abuse and dependence in a population-based sample of male twins. American Journal of Psychiatry. 1999;156:34–40. [PubMed: 9892295]
- 53.
- Schuckit MA, Edenberg HJ, Kalmijn J, Flury L, Smith TL, Reich T, Foroud T. A genome-wide search for genes that relate to a low level of response to alcohol. Alcoholism: Clinical and Experimental Research. 2001;25(3):323–329. [PubMed: 11290841]
- 54.
- Dick DM, Agrawal A. The genetics of alcohol and other drug dependence. Alcohol Research & Health. 2008;31(2):111–119. [PMC free article: PMC3860452] [PubMed: 23584813]
- 55.
- Drgonova J, Walther D, Singhal S, Johnson K, Kessler B, Troncoso J, Uhl GR. Altered CSMD1 expression alters cocaine-conditioned place preference: Mutual support for a complex locus from human and mouse models. PLOS ONE. 2015;10(7) [PMC free article: PMC4501703] [PubMed: 26171607]
- 56.
- Zhong X, Drgonova J, Li CY, Uhl GR. Human cell adhesion molecules: Annotated functional subtypes and overrepresentation of addiction-associated genes. Annals of the New York Academy of Sciences. 2015;1349(1):83–95. [PMC free article: PMC4564344] [PubMed: 25988664]
- 57.
- Grant BF, Stinson FS, Dawson DA, Chou SP, Dufour MC, Compton W, Kaplan K. Prevalence and co-occurrence of substance use disorders and independent mood and anxiety disorders: Results from the National Epidemiologic Survey on Alcohol and Related Conditions. Archives of General Psychiatry. 2004;61(8):807–816. [PubMed: 15289279]
- 58.
- Grant BF, Stinson FS, Dawson DA, Chou SP, Ruan WJ, Pickering RP. Co-occurrence of 12-month alcohol and drug use disorders and personality disorders in the United States: Results from the National Epidemiologic Survey on Alcohol and Related Conditions. Archives of General Psychiatry. 2004;61(4):361–368. [PubMed: 15066894]
- 59.
- Center for Behavioral Health Statistics and Quality. Results from the 2015 National Survey on Drug Use and Health: Detailed tables. Rockville, MD: Substance Abuse and Mental Health Services Administration; 2016.
- 60.
- Chilcoat HD, Menard C. Epidemiological investigations: Comorbidity of posttraumatic stress disorder and substance use disorder. In: Ouimette P, Brown PJ, editors. Trauma and substance abuse: Causes, consequences, and treatment of comorbid disorders. Washington, DC: American Psychological Association; 2003. pp. 9–28.
- 61.
- Kessler RC, Berglund P, Demler O, Jin R, Merikangas KR, Walters EE. Lifetime prevalence and age-of-onset distributions of DSM-IV disorders in the National Comorbidity Survey Replication. Archives of General Psychiatry. 2005;62(6):593–602. [PubMed: 15939837]
- 62.
- Jacobsen LK, Southwick SM, Kosten TR. Substance use disorders in patients with posttraumatic stress disorder: A review of the literature. American Journal of Psychiatry. 2001;158(8):1184–1190. [PubMed: 11481147]
- 63.
- Leeies M, Pagura J, Sareen J, Bolton JM. The use of alcohol and drugs to self-medicate symptoms of posttraumatic stress disorder. Depression and Anxiety. 2010;27(8):731–736. [PubMed: 20186981]
- 64.
- Kumari V, Postma P. Nicotine use in schizophrenia: The self medication hypotheses. Neuroscience and Biobehavioral Reviews. 2005;29(6):1021–1034. [PubMed: 15964073]
- 65.
- Anthenelli RM. Focus on: Comorbid mental health disorders. Alcohol Research & Health. 2010;33(1-2):109–117. [PMC free article: PMC3887501] [PubMed: 23579941]
- 66.
- Holmes A, Fitzgerald PJ, MacPherson KP, DeBrouse L, Colacicco G, Flynn SM, Marcinkiewcz CA. Chronic alcohol remodels prefrontal neurons and disrupts NMDAR-mediated fear extinction encoding. Nature Neuroscience. 2012;15(10):1359–1361. [PMC free article: PMC3471649] [PubMed: 22941108]
- 67.
- Kelley ME, Wan CR, Broussard B, Crisafio A, Cristofaro S, Johnson S, Walker EF. Marijuana use in the immediate 5-year premorbid period is associated with increased risk of onset of schizophrenia and related psychotic disorders. Schizophrenia Research. 2016;171(1-3):62–67. [PMC free article: PMC4929616] [PubMed: 26785806]
- 68.
- Keyes KM, Grant BF, Hasin DS. Evidence for a closing gender gap in alcohol use, abuse, and dependence in the United States population. Drug and Alcohol Dependence. 2008;93(1):21–29. [PMC free article: PMC3163892] [PubMed: 17980512]
- 69.
- Hasin DS, Grant BF. The National Epidemiologic Survey on Alcohol and Related Conditions (NESARC) Waves 1 and 2: Review and summary of findings. Social Psychiatry and Psychiatric Epidemiology. 2015;50(11):1609–1640. [PMC free article: PMC4618096] [PubMed: 26210739]
- 70.
- Brady KT, Randall CL. Gender differences in substance use disorders. Psychiatric Clinics of North America. 1999;22(2):241–252. [PubMed: 10385931]
- 71.
- Greenfield SF, Back SE, Lawson K, Brady KT. Substance abuse in women. Psychiatric Clinics of North America. 2010;33(2):339–355. [PMC free article: PMC3124962] [PubMed: 20385341]
- 72.
- Kosten TA, Gawin FH, Kosten TR, Rounsaville BJ. Gender differences in cocaine use and treatment response. Journal of Substance Abuse Treatment. 1993;10(1):63–66. [PubMed: 8450576]
- 73.
- Chanraud S, Pitel A-L, Müller-Oehring EM, Pfefferbaum A, Sullivan EV. Remapping the brain to compensate for impairment in recovering alcoholics. Cerebral Cortex. 2013;23(1):97–104. [PMC free article: PMC3513953] [PubMed: 22275479]
- 74.
- Becker JB, Koob GF. Sex differences in animal models: Focus on addiction. Pharmacological Reviews. 2016;68(2):242–263. [PMC free article: PMC4813426] [PubMed: 26772794]
- 75.
- Okuyemi KS, Powell JN, Savage CR, Hall SB, Nollen N, Holsen LM, Ahluwalia JS. Clinical and imaging study: Enhanced cue-elicited brain activation in African American compared with Caucasian smokers: An fMRI study. Addiction Biology. 2006;11(1):97–106. [PubMed: 16759342]
- 76.
- Brooks PJ, Enoch M-A, Goldman D, Li T-K, Yokoyama A. The alcohol flushing response: An unrecognized risk factor for esophageal cancer from alcohol consumption. PLOS Medicine. 2009;6(3) [PMC free article: PMC2659709] [PubMed: 19320537]
- 77.
- Yokoyama A, Omori T. Genetic polymorphisms of alcohol and aldehyde dehydrogenases and risk for esophageal and head and neck cancers. Japanese Journal of Clinical Oncology. 2003;33(3):111–121. [PubMed: 12672787]
- 78.
- Fukuda K, Yuzuriha T, Kinukawa N, Murakawa R, Takashima Y, Uchino A, Hirano M. Alcohol intake and quantitative MRI findings among community dwelling Japanese subjects. Journal of the Neurological Sciences. 2009;278(1):30–34. [PubMed: 19059611]
- Chapter 2 Preview
- Conducting Research on the Neurobiology of Substance Use, Misuse, and Addiction
- The Primary Brain Regions Involved in Substance Use Disorders
- The Addiction Cycle
- Binge/Intoxication Stage: Basal Ganglia
- Withdrawal/Negative Affect Stage: Extended Amygdala
- Preoccupation/Anticipation Stage: Prefrontal Cortex
- Different Classes of Substances Affect the Brain and Behavior in Different Ways
- Factors that Increase Risk for Substance Use, Misuse, and Addiction
- Use of Multiple Substances and Co-occurring Mental Health Conditions
- Biological Factors Contributing to Population-based Differences in Substance Misuse and Substance Use Disorders
- Recommendations for Research
- References
- THE NEUROBIOLOGY OF SUBSTANCE USE, MISUSE, AND ADDICTION - Facing Addiction in A...THE NEUROBIOLOGY OF SUBSTANCE USE, MISUSE, AND ADDICTION - Facing Addiction in America
Your browsing activity is empty.
Activity recording is turned off.
See more...