By agreement with the publisher, this book is accessible by the search feature, but cannot be browsed.
NCBI Bookshelf. A service of the National Library of Medicine, National Institutes of Health.
Gilbert SF. Developmental Biology. 6th edition. Sunderland (MA): Sinauer Associates; 2000.
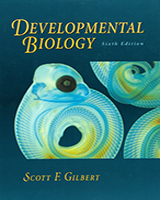
Developmental Biology. 6th edition.
Show detailsIn recent years, the teleost fish Danio rerio, known as the zebrafish, has become a favorite organism of those who wish to study vertebrate development. Zebrafish have large broods, breed all year, are easily maintained, have transparent embryos that develop outside the mother (an important feature for microscopy), and can be raised so that mutants can be readily screened and propagated. In addition, they develop rapidly, so that at 24 hours after fertilization, the embryo has formed most of its tissues and organ primordia and displays the characteristic tadpole-like form (see Granato and Nüsslein-Volhard 1996; Langeland and Kimmel 1997). Therefore, much of the description of fish development below is based on studies of this species
WEBSITE
11.1 Gene manipulation in the zebrafish. Zebrafish can be bred so that they express altered genes rapidly. This technology makes Danio an important contributor to developmental studies. http://www.devbio.com/chap11/link1101.shtml
Cleavage in Fish Eggs
In fish eggs, cleavage occurs only in the blastodisc, a thin region of yolk-free cytoplasm at the animal cap of the egg. Most of the egg cell is full of yolk. The cell divisions do not completely divide the egg, so this type of cleavage is called meroblastic (Greek, meros, “part”). Since only the cytoplasm of the blastodisc becomes the embryo, this type of meroblastic cleavage is called discoidal. Scanning electron micrographs show beautifully the incomplete nature of discoidal meroblastic cleavage in fish eggs (Figure 11.1). The calcium waves initiated at fertilization stimulate the contraction of the actin cytoskeleton to squeeze non-yolky cytoplasm into the animal pole of the egg. This converts the spherical egg into a more pear-shaped structure, with an apical blastodisc (Leung et al. 1998). Early cleavage divisions follow a highly reproducible pattern of meridional and equatorial cleavages. These divisions are rapid, taking about 15 minutes each. The first 12 divisions occur synchronously, forming a mound of cells that sits at the animal pole of a large yolk cell. These cells constitute the blastoderm. Initially, all the cells maintain some open connection with one another and with the underlying yolk cell so that moderately sized (17-kDa) molecules can pass freely from one blastomere to the next (Kimmel and Law 1985).

Figure 11.1
Discoidal cleavage in a zebrafish egg. (A) 1-cell embryo. The mound atop the cytoplasm is the blastodisc region. (B) 2-cell embryo. (C) 4-cell embryo. (D) 8-cell embryo, wherein two rows of four cells are formed. (E) 32-cell embryo. (F) 64-cell embryo, (more...)
Beginning at about the tenth cell division, the onset of the midblastula transition can be detected: zygotic gene transcription begins, cell divisions slow, and cell movement becomes evident (Kane and Kimmel 1993). At this time, three distinct cell populations can be distinguished. The first of these is the yolk syncytial layer (YSL). The YSL is formed at the ninth or tenth cell cycle, when the cells at the vegetal edge of the blastoderm fuse with the underlying yolk cell. This fusion produces a ring of nuclei within the part of the yolk cell cytoplasm that sits just beneath the blastoderm. Later, as the blastoderm expands vegetally to surround the yolk cell, some of the yolk syncytial nuclei will move under the blastoderm to form the internal YSL, and some of the nuclei will move vegetally, staying ahead of the blastoderm margin, to form external YSL (Figure 11.2A,B). The YSL will be important for directing some of the cell movements of gastrulation. The second cell population distinguished at the midblastula transition is the enveloping layer (EVL; Figure 11.2A). It is made up of the most superficial cells of the blastoderm, which form an epithelial sheet a single cell layer thick. The EVL eventually becomes the periderm, an extraembryonic protective covering that is sloughed off during later development.

Figure 11.2
Fish blastula. (A) Prior to gastrulation, the deep cells are surrounded by the EVL. The animal surface of the yolk cell is flat and contains the nuclei of the YSL. Microtubules extend through the yolky cytoplasm and through the external region of the (more...)
Between the EVL and the YSL are the deep cells. These are the cells that give rise to the embryo proper. The fates of the early blastoderm cells are not determined, and cell lineage studies (in which a nondiffusible fluorescent dye is injected into one of the cells so that the descendants of that cell can be followed) show that there is much cell mixing during cleavage. Moreover, any one of these cells can give rise to an unpredictable variety of tissue descendants (Kimmel and Warga 1987; Helde et al. 1994). The fate of the blastoderm cells appears to be fixed shortly before gastrulation begins. At this time, cells in specific regions of the embryo give rise to certain tissues in a highly predictable manner, allowing a fate map to be made (Figure 11.2C; see also Figure 1.6; Kimmel et al 1990).
Gastrulation in Fish Embryos
The first cell movement of fish gastrulation is the epiboly of the blastoderm cells over the yolk. In the initial phase, the deep blastoderm cells move outwardly to intercalate with the more superficial cells (Warga and Kimmel 1990). Later, these cells move over the surface of the yolk to envelop it completely (Figure 11.3). This movement is not due to the active crawling of the blastomeres, however. Rather, the movement is provided by the autonomously expanding YSL “within” the animal pole yolk cytoplasm. The EVL is tightly joined to the YSL and is dragged along with it. The deep cells of the blastoderm then fill in the space between the YSL and the EVL as epiboly proceeds. This can be demonstrated by severing the attachments between the YSL and the EVL. When this is done, the EVL and deep cells spring back to the top of the yolk, while the YSL continues its expansion around the yolk cell (Trinkaus 1984, 1992). The expansion of the YSL depends on a network of microtubules in the YSL, and radiation or drugs that block the polymerization of tubulin inhibit epiboly (Strahle and Jesuthasan 1993; Solnica-Krezel and Driever 1994).

Figure 11.3
Cell movements during gastrulation of the zebrafish. (A) The blastoderm at 30% completion of epiboly (about 4.7 hours). (B) Formation of the hypoblast, either by involution of cells at the margin of the epibolizing blastoderm or by delamination of cells (more...)
During migration, one side of the blastoderm becomes noticeably thicker than the other. Cell-labeling experiments indicate that the thicker side marks the site of the future dorsal surface of the embryo (Schmidt and Campos-Ortega 1995).
The formation of germ layers
After the blastoderm cells have covered about half the zebrafish yolk cell (and earlier in fish eggs with larger yolks), a thickening occurs throughout the margin of the epibolizing blastoderm. This thickening is called the germ ring, and it is composed of a superficial layer, the epiblast, and an inner layer, the hypoblast. We do not understand how the hypoblast is made. Some research groups claim that the hypoblast is formed by the involution of superficial cells under the margin followed by their migration toward the animal pole (see Figure 11.3C). The involution begins at the future dorsal portion of the embryo, but occurs all around the margin. Other laboratories claim that these superficial cells ingress to form the hypoblast (see Trinkaus 1996). It is possible that both mechanisms are at work, with different modes of hypoblast formation predominating in different species. Once formed, however, the cells of both the epiblast and hypoblast intercalate on the future dorsal side of the embryo to form a localized thickening, the embryonic shield (Figure 11.4). As we will see, this shield is functionally equivalent to the dorsal blastopore lip of amphibians, since it can organize a secondary embryonic axis when transplanted to a host embryo (Oppenheimer 1936; Ho 1992). Thus, as the cells undergo epiboly around the yolk, they are also involuting at the margins and converging anteriorly and dorsally toward the embryonic shield (Trinkaus 1992). The hypoblast cells of the embryonic shield converge and extend anteriorly, eventually narrowing along the dorsal midline of the hypoblast. This movement forms the chordamesoderm, the precursor of the notochord (Figure 11.4B,C). The cells adjacent to the chordamesoderm, the paraxial mesoderm cells, are the precursors of the mesodermal somites (Figure 11.4D,E). The concomitant convergence and extension in the epiblast brings the presumptive neural cells from all over the epiblast into the dorsal midline, where they form the neural keel. The rest of the epiblast becomes the skin of the fish. The zebrafish fate map, then, is not much different from that of the frog or other vertebrates (as we will soon see). If one conceptually opens a Xenopus blastula at the vegetal pole and stretches the opening into a marginal ring, the resulting fate map closely resembles that of the zebrafish embryo at the stage when half of the yolk has been covered by the blastoderm (see Figure 1.9; Langeland and Kimmel 1997).

Figure 11.4
Convergence and extension in the zebrafish gastrula. (A) Dorsal view of the convergence and extension movements during zebrafish gastrulation. Epiboly spreads the blastoderm over the yolk; involution or ingression generates the hypoblast; convergence (more...)
Axis Formation in Fish Embryos
Dorsal-ventral axis formation: the embryonic shield
The embryonic shield is critical in establishing the dorsal-ventral axis in fishes. It can convert lateral and ventral mesoderm (blood and connective tissue precursors) into dorsal mesoderm (notochord and somites), and it can cause the ectoderm to become neural rather than epidermal. This was shown by transplantation experiments in which the embryonic shield of one early-gastrula embryo was transplanted to the ventral side of another (Figure 11.5; Oppenheimer 1936; Koshida et al. 1998). Two axes formed, sharing a common yolk cell. Although the prechordal plate and the notochord were derived from the donor embryonic shield, the other organs of the secondary axis came from host tissues that would have formed ventral structures. The new axis has been induced by the donor cells. In the embryo that had had its embryonic shield removed, no dorsal structures formed, and the embryo lacked a nervous system. These experiments are similar to those performed on amphibian gastrulae by Spemann and Mangold (1924; see Chapter 10), and they demonstrate that the embryonic shield is the homologue of the dorsal blastopore lip, the amphibian organizer.

Figure 11.5
The embryonic shield as organizer in the fish embryo. A donor embryonic shield (about 100 cells from a stained embryo) is transplanted into a host embryo at the same early-gastrula stage. The result is two embryonic axes joined to the host's yolk cell. (more...)
Like the amphibian dorsal blastopore lip, the embryonic shield forms the prechordal plate and the notochord of the developing embryo. The precursors of these two regions are responsible for inducing the ectoderm to become neural ectoderm. Moreover, the presumptive notochord and prechordal plate appear to do this in a manner very much like that of their homologous structures in amphibians.* In both fishes and amphibians, BMP proteins made in the ventral and lateral regions of the embryo would normally cause the ectoderm to become epidermis. The notochord of both fishes and amphibians secretes factors that block this induction and thereby allow the ectoderm to become neural. In fishes, the BMP that ventralizes the embryo is BMP2B. The protein secreted by the chordamesoderm that binds with and inactivates BMP2B is a chordin-like paracrine factor called Chordino (Figure 11.6B; Kishimoto et al. 1997; Schulte-Merker et al. 1997). If the chordino gene is mutated, the neural tube fails to form. It is hypothesized (Nguyen et al. 1998) that different concentrations of BMP2B pattern the ventral and lateral regions of the zebrafish ectoderm and mesoderm, and that the ratio between Chordino and BMP2B may specify the position along the dorsal-ventral axis. In fishes, however, the notochord may not be the only structure capable of producing the proteins that block BMP2B. If the notochord fails to form (as in the floating head or no tail mutations), the neural tube will still be produced. It is possible that the notochordal precursor cells (which are produced in these mutations) are still able to induce the neural tube, or that the dorsal portion of the somite precursors can compensate for the lack of a notochord (Halpern et al. 1993; 1995; Hammerschmidt et al. 1996).

Figure 11.6
Axis formation in the zebrafish embryo. (A) Prior to gastrulation, the zebrafish blastoderm is arranged with the presumptive ectoderm near the animal pole, the presumptive mesoderm beneath it, and the presumptive endoderm sitting atop the yolk cell. The (more...)
The embryonic shield appears to acquire its organizing ability in much the same way as its amphibian counterparts. In amphibians, the endoderm cells beneath the dorsal blastopore lip (i.e., the Nieuwkoop center) accumulate β-catenin. This protein is critical in amphibians for the ability of the endoderm to induce the cells above them to become the dorsal lip (organizer) cells. In zebrafish, the nuclei in that part of the yolk syncytial layer that lies beneath the cells that will become the embryonic shield similarly accumulate β-catenin. This protein distinguishes the dorsal YSL from the lateral and ventral YSL regions (Figure 11.7; Schneider et al. 1996). Inducing β-catenin accumulation on the ventral side of the egg causes dorsalization and a second embryonic axis (Kelly et al. 1995). In addition, just prior to gastrulation, the cells of the dorsal blastopore margin synthesize and secrete Nodal-related proteins. These induce the precursors of the notochord and prechordal plate to activate goosecoid and other genes (Sampath et al. 1998; Gritsman et al. 2000.) Thus, the embryonic shield is considered equivalent to the amphibian organizer, and the dorsal part of the yolk cell can be thought of as the Nieuwkoop center of the fish embryo.

Figure 11.7
Nuclear localization of β-catenin marks the dorsal side of the Xenopus blastula (the larger one) and helps form its Nieuwkoop center beneath the organizer. In the zebrafish late blastula, nuclear localization of β-catenin is seen in the (more...)
Anterior-posterior axis formation: two signaling centers
As is evident from Figure 11.5, when a second dorsal-ventral axis is experimentally induced in zebrafish eggs, both the regular and the induced axes have the same anterior-posterior polarity. Both heads are at the former animal cap, and both tails are located vegetally. Indeed, the anterior-posterior axis is specified during oogenesis, and the animal cap marks the anterior of the embryo. This axis becomes stabilized during gastrulation through two distinct signaling centers. First, a small group of anterior neural cells at the border between the neural and surface ectoderm (a region that become the pituitary gland, nasal placode, and anterior forebrain) secrete compounds that cause anterior development. If these anterior neural cells are experimentally placed more posteriorly in the embryo, they will cause the neural cells near them to assume the characteristics of forebrain neurons. The second signaling center, in the posterior of the embryo, consists of lateral mesendoderm precursors at the margin of the gastrulating blastoderm. These cells produce caudalizing compounds , most likely Nodal-related proteins and activin (Figure 11.6C; Woo and Fraser 1997; Houart et al. 1998; Thisse et al. 2000). If transplanted adjacent to anterior neural ectoderm, this tissue will transform the presumptive forebrain tissue into hindbrain-like structures.
WEBSITE
11.2 The fish Hox complexes. In birds and mammals, Hox genes play important roles in the identity of different parts of the body. Studies of fishes show that the Hox genes may similarly specify somite identity along the anterior-posterior axis. These studies also show important evolutionary changes that may have led to the evolution of the vertebrates. http://www.devbio.com/chap11/link1102.shtml
Left-right axis formation
Little is known about left-right axis formation in fishes. In zebrafish, a gene called nodal-related-2 is expressed solely in the left side of the lateral plate mesoderm† (Rebagliati et al. 1998). Nodal-related-2 is a paracrine factor of the TGF-β family. As we will see later in this chapter, proteins of the TGF-β family are critical in the establishment of the left-right axis throughout the vertebrate classes.
Footnotes
- *
Another similarity between the amphibian and fish organizers is that they can be duplicated by rotating the egg and changing the orientation of the microtubules (Fluck et al. 1998). One difference in the axial development of these groups is that in amphibians (see Chapter 10), the prechordal plate is necessary for inducing the anterior brain to form. In Danio, the prechordal plate appears to be necessary for forming ventral neural structures, but the anterior regions of the brain can form in its absence (Schier et al. 1997; Schier and Talbot 1998).
- †
The lateral plate mesoderm, discussed in Chapter 15, is the region that forms the heart and body cavities.
- Early Development in Fish - Developmental BiologyEarly Development in Fish - Developmental Biology
Your browsing activity is empty.
Activity recording is turned off.
See more...