NCBI Bookshelf. A service of the National Library of Medicine, National Institutes of Health.
Fitridge R, Thompson M, editors. Mechanisms of Vascular Disease: A Reference Book for Vascular Specialists [Internet]. Adelaide (AU): University of Adelaide Press; 2011.
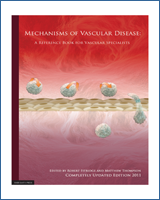
Mechanisms of Vascular Disease: A Reference Book for Vascular Specialists [Internet].
Show detailsIntroduction
Atherosclerosis continues to cause consider able morbidity and mortality, particularly in the western world. While risk factors have been clearly identified, their precise roles in early atherogenesis are complex. The early development of the plaque is dependent upon interactions between damaged endothelial cells, vessel wall smooth muscle cells and circulating inflammatory cells mediated by the release of cytokines, growth factors and cell adhesion molecules. Plaque formation may represent a cell mediated immune phenomenon, with a variety of potential antigenic agents identified. Shear stress and flow considerations also play a part.
Atherosclerosis begins in childhood, but it takes decades for atherosclerosis to evolve into the mature plaques responsible for the onset of ischaemic symptoms. Whilst plaque growth due to smooth muscle cell proliferation, matrix synthesis and lipid accumulation may narrow the arterial lumen and ultimately limit blood flow, uncomplicated atherosclerosis is essentially a benign disease. The final clinical outcome depends on whether a plaque becomes unstable, leading to acute disruption of its surface and exposure of its thrombogenic core to the luminal blood flow. The concept of a ‘vulnerable plaque’ was initially described in 19901,2 and though this initially gained wide acceptance, many authors now favour the broader concept of a ‘vulnerable patient’, whereby certain systemic and haematological conditions (e.g. relative hypercaogulability) must also be met before plaque rupture will result in symptomatic thrombosis.3
Mature atherosclerotic plaques are composed of a lipid core that is separated from the vessel lumen by a cap composed of fibrillar collagen. Disruption of this cap exposes the plaque’s underlying thrombogenic core to the bloodstream, resulting in thromboembolism. This process of ‘plaque rupture’ is responsible for the majority of acute coronary syndromes (unstable angina, MI)4-6,7 and ischaemic cerebral events (stroke, TIA, amaurosis fugax).8-10
Unravelling the complex biochemical and haemodynamic factors leading to plaque rupture is one of the greatest challenges facing contemporary medical research. The vital question in plaque pathogenesis is why, after years of indolent growth, life threatening disruption and subsequent thrombosis should suddenly occur. Plaque stabilisation may prove to be an important clinical strategy for preventing the development of complications.6 Identification of ‘vulnerable plaques’ (i.e., those most at risk of rupture) and ‘vulnerable patients’ (i.e. those with predisposition to atherothrombotic occlusion) would allow pharmacotherapy to be targeted more effectively. Furthermore, a greater understanding of the mechanisms involved in plaque rupture will lead to improvements in preventative therapy.
Evidence for the ‘Plaque Rupture’ Theory’
Coronary circulation
Evidence that plaque rupture leads to acute coronary syndromes has been provided from a number of sources. Early pathological studies using post mortem specimens from fatal cases of acute myocardial infarction have revealed that virtually all cases of coronary thrombosis are related to rupture or fissuring of atheromatous plaques, along with evidence of distal embolisation.7,11-13 Angioscopic findings in patients with stable angina have identified smooth atheroma within their coronary arteries, but disrupted irregular atheroma in the arteries of those with unstable angina.14,15
Radiological and histological studies have demonstrated that patients with a plaque morphology consisting of large lipid cores and thin fibrous caps are at increased risk of cardiovascular events.16-18 In addition, these ‘unstable’ plaques are not necessarily the ones causing severely stenotic lesions.19-21
Cerebral circulation
A similar association between carotid plaque rupture and cerebrovascular events has been shown. In patients undergoing multiple TIAs or stroke progression, microemboli can be detected in the middle cerebral artery by transcranial Doppler.10,22 Surface ulceration of carotid plaques seen on ultrasound imaging correlates well with symptoms23 and echolucent (lipid-rich) plaques are at increased risk of causing future cerebrovascular events.
Early work utilising carotid plaques retrieved at carotid endarterectomy, highlighted the relationship between the presence of throm bus and the clinical status of patients.24,25 This supported the theory that ischaemic attacks resulted from embolism rather than reduction in cerebral blood flow, particularly as few strokes occur in watershed areas.26
A number of subsequent studies demonstrated a relationship between the presence of intraplaque haemorrhage and patient symptoms.27 Persson et al found that intra plaque haemorrhage appeared more frequently in symptomatic patients than asymptomatic patients,28 while Lusby suggested a relationship between the onset of neurological symptoms and development of plaque haemorrhage.29 Intraplaque haemorrhage may potentially arise after cap rupture, though it now seems most likely that it occurs prior to plaque breakdown30 and may play an important role in disruption of the fibrous cap.
The most compelling evidence for an association between carotid plaque rupture and ischaemic cerebral events, is that carotid endarterectomy specimens removed from symptomatic patients are more likely to show histological evidence of rupture, compared to those from asymptomatic patients.8,9 Van Damme and colleagues showed that 53% of complicated carotid plaques (intraplaque haemorrhage, haematoma, thrombus or ulceration) were symptomatic with a corresponding neurological deficit, compared to 21% of simple uncomplicated plaques.31
The Role of Individual Components of the Arterial Wall
A number of intrinsic and extrinsic factors have been identified that determine plaque vulnerability: the size and consistency of the plaque core, the thickness and collagen content of the fibrous cap, and inflammation within the plaque. Further factors such as haemodynamic stress upon the plaque may ultimately contribute to cap disruption.
The evolution of a stable to unstable plaque with cap rupture and thrombosis can be outlined in the following simplistic terms (Figure 4.1): Endothelial damage allows passage of inflammatory cells and LDL into the vessel intima; free radicals are responsible for oxidation of the deposited LDL, and oxidized-LDL promotes cytokine and protease release from macrophages; proteases (in addition to other factors) degrade the fibrous cap causing disruption, allowing exposure of thrombogenic material to the blood; local thrombotic and fibrinolytic activity determine the degree of thrombus progression or dissolution.

FIGURE 4.1
The stages of plaque rupture.
Each component contributing to plaque rupture will be discussed in further detail. The relevant processes occur in the endothelium, the lipid core, the fibrous cap and the vessel lumen.
The endothelium
The origin of plaque destabilization can be traced back to endothelial dysfunction, or ‘activation’. The endothelium is a single layer of highly specialised cells lining the vessel wall/lumen interface. It plays a vital role in modulating vascular permeability, perfusion, contraction and haemostasis. Leukocytes do not bind to normal endothelium. However, endothelial activation leads to the early surface expression of cell adhesion molecules, including VCAM-1, ICAM-1, E-selectin and P-selectin, which permit leukocyte binding. Many of the known atherosclerosis risk factors (e.g., smoking, hyperlipidaemia, hyperglycaemia, hypertension, hyperhomocysteinaemia) exert their damaging effects by causing endothelial activation.32-37
Activated endothelial cells express chemo attractant cytokines such as MCP-1, M-CSF, IL-1, IL-6 and TNF-α, as well as cell adhesion molecules. This pro inflammatory environment, in conjunction with the altered permeability of the dysfunctional endothelium, mediates the migration and entry of leucocytes (mainly monocytes and lymphocytes) into the intima.38-40
The degree of endothelial dysfunction depends upon the balance between endothelial activation and endothelial ‘passivation’ (see Figure 4.2). Nitric oxide is the predominant molecule responsible for passivation, and the endothelium acts as an autocrine organ in its production.41 Nitric oxide is an antioxidant, but has other plaque-stabilizing properties including reducing cell adhesion molecule expression,42 platelet aggregation and SMC proliferation. Endothelial nitric oxide synthase, the enzyme responsible for nitric oxide production, is increased in people undergoing regular physical exertion, which may partly explain the benefits of exercise in atherosclerosis prevention.43

FIGURE 4.2
Factors affecting endothelial activity.
Endothelial cells are exposed to 3 different types of mechanical force. Hydrostatic forces (generated by the blood) and circumferential stress (generated by the vessel wall) are responsible for endothelial injury and activation. The third force is haemodynamic shear stress (generated by the flow of blood), which is inversely related to atherosclerosis formation – areas of high shear stress being relatively protected.44 Despite the systemic nature of atherosclerosis, it is an anatomically focal disease with certain sites having a propensity for plaque formation. Arterial bifurcations exhibit slow blood flow, sometimes even bi-directional flow, resulting in decreased shear stress. The activity of endothelial nitric oxide synthase is decreased in these areas of nonlaminar blood flow.45,46 In addition, there is increased oscillatory and turbulent shear stress at bifurcations, associated with an increase in oxygen free radical production47 and monocyte adhesion.48
According to Laplace’s law, the higher the blood pressure and the larger the luminal diameter, the more circumferential tension develops in the wall.49 This phenomenon combined with a radial compression of the vessel wall may lead to excessive stress in vulnerable regions of the plaque, particularly the cap and shoulder.50 For fibrous caps of the same tensile strength, those caps covering moderately stenotic plaques are probably more prone to rupture than those covering severely stenotic plaques, because the former have to bear a greater circumferential tension.51
Tthe propagating pulse wave causes cyclic changes in lumen size and shape with deformation and bending of plaques, particularly those with a large soft plaque core. Eccentric plaques typically bend at the junction between the relatively stiff plaque and the compliant vessel wall.52 the force applied to this region is accentuated by changes in vascular tone.
High blood velocity within stenotic lesions may shear the endothelium away, but whether high wall stress alone may disrupt a stenotic plaque is questionable.4 The absolute stresses induced by wall shear are usually much smaller than the mechanical stresses imposed by blood and pulse pressure.53
It is clear that the endothelium is much more than an inert arterial wall lining. It is, in fact, a dynamic autocrine and paracrine organ responsible for the functional regulation of local haemodynamics. Factors that disturb this delicate balance are responsible for the initiation of a cascade of events eventually leading to plaque rupture.
The lipid core
The size and consistency of the atheromatous core is variable and critical to the stability of individual lesions, with a large volume lipid core being one of the constituents of the vulnerable plaque (Figure 4.3). It appears that the accumulation of lipids in the intima renders the plaque inherently unstable.

FIGURE 4.3
Longitudinal section of carotid plaque demonstrating a large volume lipid core.
Although extremely variable, the ‘average’ coronary plaque is predominantly sclerotic with the atheromatous core making up <30% of the plaque volume.54 The variability in plaque composition is poorly understood, with no relationship to any of the identified risk factors for atherosclerosis. Gertz and Roberts examined the histological composition of postmortem plaques from 17 infarct-related coronary arteries.55 They found much larger proportions of the disrupted plaques to be occupied by atheromatous gruel in comparison to the intact plaques. Davies found a similar relationship in aortic lesions, with 91% of thrombosing plaques versus 11% of intact plaques exhibiting a lipid core that occupied >40% of the total plaque volume.56
Histological data regarding the necrotic core of carotid plaques is limited. There is, however, considerable evidence to link ultrasound detected echolucent plaques (deemed to contain more soft or amorphous tissue) with symptomatology.57,58 Feeley and colleagues demonstrated that symptomatic carotid plaques contained a significantly higher proportion of amorphous material than asymptomatic plaques,59 with the lipid-rich core constituting 40% of overall plaque volume.60
LDL plays a more complex role in plaque instability than can be explained simply by the ‘spaceoccupying’ effect of accumulated lipid. A large core may produce a greater luminal narrowing, but plaque rupture sites are often characterized by ‘outward remodelling’ whereas those stenoses causing stable angina are more likely to be associated with ‘inward remodelling’.61 Indeed, it has been shown that in patients suffering acute coronary syndromes who had undergone angiography in the preceding months, the responsible lesion was recorded as causing a <70% stenosis in the majority of cases.19,20,61 This is perhaps not surprising since, as mentioned earlier, a larger lumen places increased circumferential stress on the plaque, predisposing it to rupture.
As inflammatory cells cross the dysfunctional endothelium, cholesterol also enters in the form of LDL, and becomes trapped in the subendothelial space. This LDL is oxidized by free radicals creating a proinflammatory compound.62 Oxidized LDL is taken up by intimal macrophages – the process being mediated via receptors expressed on the macrophage surface,63 although endocytosis of native LDL has also been demonstrated.64 This process initially protects the surrounding smooth muscle and endothelial cells from the direct cytotoxic effects of oxidised-LDL, but leads to the formation of ‘foam cells’ (lipid-laden macrophages). Uptake of oxidized LDL stimulates the expression of cytokines and proteolytic enzymes, propagating the cycle of inflammation.
The formation of a lipid core is a balance between LDL deposition of cholesterol in the damaged intima and removal by HDL (Figure 4.4). HDL and its carrier, apolipoprotein A-I, are responsible for so-called ‘reverse cholesterol transport’ – moving cholesterol from cells into the blood (from where it can be transferred to the liver for excretion in the bile).65 However, it may also be capable of effecting lipid removal directly from the plaque, one of the possible explanations for plaque regression seen with increased HDL levels.66 HDL may have other beneficial effects also, such as improving endothelial function,67 decreasing cell adhesion molecule expression,68 and inhibiting oxidation of LDL.69

FIGURE 4.4
Factors affecting plaque lipid content.
In addition to the potential proinflammatory role of oxidised LDL, it has recently been proposed that cholesterol accumulation may lead to plaque rupture via a more direct physical pathway. Changes in local biological milieu such as decreased temperature or increased pH may cause in vitro precipitation of cholesterol into solid crystals. This alteration in state not only leads to a significant volume increase of up to 45%, but also leads to formation of sharp tipped crystals that might be capable of damaging surrounding tissues and initiating plaque rupture. Using electron microscopy, Abela et al demonstrated that such crystal could be seen perforating the luminal surface of ruptured plaques from human coronary arteries.70,71
The cap of the plaque
The cap of the atherosclerotic plaque plays a vital role in isolating the plaque’s thrombogenic core from the bloodstream. Since the thickness and collagen content of this cap are important determinants of overall plaque stability,51 many authors now use the term ‘thincap fibroatheroma’ (TCFA) to identify those plaques most at risk of rupture. The accepted definition of TCFA is any plaque with a cap thickness of less than 65µm. Though the exact mechanisms that underlie progression from stable plaque to TCFA remain somewhat uncertain, it has been suggested that endothelial shear stress may play an important role, since TCFAs most often arise at sites of low endothelial shear stress (such as bifurcations and the concave side of arterial bends).72
Whatever the thickness of the fibrous cap, it is composed largely of fibrillar collagens (type I and type III68), though the relative proportion of collagen decreases as the cap thins. The fibrillar collagens have a lower thrombogenicity than the underlying core, but their exposure can be responsible for thrombus formation following erosion of the overlying endothelium.73,74 This phenomenon accounts for one third of acute coronary syndromes,75 and the subsequent healing process of erosions can account for rapid and stepwise progression in plaque growth, leading to sudden increases in stenosis or occlusion.76
The most vulnerable area of the plaque is the shoulder region, where the cap is often at its thinnest.7 Studies have shown a reduction in the collagen content of the cap around areas of plaque disruption, as well as steep transverse gradients of connective tissue constituents across ulcerated plaques.77 This may result from a reduction in matrix production by smooth muscle cells, which exhibit diminished numbers in areas of plaque disruption,56 or from increased degradation of matrix by proteolytic enzymes. It is most likely, of course, that a combination of excessive matrix degradation and reduced matrix production are responsible for cap thinning (Figure 4.5). A reduction in SMCs within the fibrous cap would certainly undermine its strength.78 Recently there has been interest in the role of smooth muscle cell apoptosis in plaque cap weakening, caused by a combination of intrinsic and extrinsic factors, particularly macrophage and lipid derived products.79,80

FIGURE 4.5
Factors affecting plaque collagen content.
More recently it has been suggested that plaque integrity may also be influenced by the development of minute, spherical microcalcifications within the fibrous cap. These microcalcifications are thought to represent accumulations of calcified macro phages or even post-apoptotic smooth muscle cells and result in highly focal increases in physical stress. The increased stress leads to areas of facial debonding, weakening the infrastructure of the cap and contributing to subsequent plaque disruption.81
Smooth muscle cells and collagen production
The SMC has a paradoxical role in plaque instability. On the one hand, SMCs are responsible for plaque matrix production and adverse arterial remodelling, while on the other, they produce collagens that give the plaque intrinsic strength. SMC inhibition therefore has potentially detrimental and beneficial effects.
In the normal arterial wall, SMCs are present in the media and express a differentiated phenotype. They are contractile and do not divide or migrate.82 In atherosclerosis, when stimulated by the milieu of growth factors and cyokines, they ‘dedifferentiate’ and express a synthetic phenotype.83 In the media, SMCs are surrounded by a basal lamina consisting of type IV collagen. Proteolytic enzymes secreted by macrophages are responsible for digestion of this supporting framework. The released SMCs are then able to migrate to the intima, where they secrete new extracellular matrix.84 SMCs play a crucial role in stabilising atherosclerotic plaques, as they are responsible for the production of the cap fibrillar collagens.82 In this respect, SMCs are important not only in initial formation of the fibrotic cap, but also in repair of subclinical plaque rupture. SMCs accumulate at the rupture site and secrete fibrous proteins. This restores plaque integrity, but may also lead to rapid growth of the plaque causing vessel stenosis. Certain platelet factors, including PDGF and TGF-β, are felt to be particularly important in stimulating collagen synthesis by SMCs, whereas γ-interferon (from activated T-cells) has the opposite effect.85
Since SMCs are the only cells producing fibrous tissue for inclusion in the atherosclerotic plaque, the balance between recruitment and degradation of these cells is clearly of great significance in plaque stability. It had previously been accepted that all SMCs involved in atherosclerosis were derived from the local vessel media or intima. However, many groups are now examining the possibility that they may also be recruited from a circulating pool of SMC progenitor cells.86 The prospect of manipulating the activity of these progenitor cells to increase plaque stability is an attractive therapeutic target, though further work is still required in this area.
Whatever the true origin of plaque SMCs, they play a vital role in maintaining the structure of the plaque and SMC apoptosis leads to decreased collagen production, thinning of the fibrous cap and increased volume of the necrotic core.87-89 A recent, though small, study demonstrated that the proportion of SMCs undergoing apoptosis and the frequency of cytoplasmic remnants of apoptotic cells were significantly increased in unstable versus stable angina atherectomy specimens.90 Apoptosis of SMCs and macrophages has been identified within plaques, but only in advanced disease with dense macrophage infiltration. Apoptotic cells are deemed to have become susceptible to a form of cell death which is distinct from necrosis and is characterised by a series of morphological changes, starting with shrinkage of the cell membrane and leading on to condensation of nuclear chromatin, cellular fragmentation and eventually engulfment of apoptotic bodies by surrounding cells.79
Pro-apoptotic proteins are present in advanced plaques, and it has been observed that cells derived from the plaque, but not the adjacent media, die when brought into culture.80,91 Intimal cell apoptosis may account for the low density of smooth muscle cells in unstable plaques, and may contribute to the events leading up to plaque disruption. Though further study is still required, prevention of smooth muscle cell apoptosis may prove to be an important therapeutic target in the treatment of atherosclerotic disease.
Macrophages and collagen degradation
It is now known that inflammation plays a major role in plaque progression and especially in the period just prior to its rupture.92 Macrophages control many of the inflammatory processes within the plaque,93 and are responsible for the production of proteolytic enzymes capable of degrading the extracellular matrix.94,95 The predominant proteolytic enzymes involved in plaque disruption are the matrix metalloproteinases or MMPs.96
The MMPs are a family of proteolytic enzymes characterised by the presence of zinc ions at their active sites. All degrade components of the extracellular matrix, and are divided into 4 main classes on the basis of their substrate specificity (Table 4.1).
TABLE 4.1
The Matrix Metalloproteinase Family.
MMPs are essential in normal healthy individuals, playing a key role in processes such as wound healing.97,98 However there is growing interest in their role in disease states where ECM breakdown plays a pre dominant role.99 Early interest focused on a pathological role for MMPs in the resorption of periodontal structures in periodontal disease,100 the destruction of joints in rheumatoid arthritis,101 and the local invasive behaviour of malignancies.102 In vascular disease, they have been implicated in many of the stages of atherosclerosis but most particularly in acute plaque disruption.103 The site of rupture is characterised by an intense inflammatory infiltrate consisting predominantly of macrophages,94 that undergoes activation resulting in increased MMP expression. This shifts the delicate equilibrium towards proteolysis and away from matrix accumulation, making plaque disruption more likely (Figure 4.5).
MMP activity is tightly controlled at several levels and expression of MMPs is determined at the transcriptional level by various cytokines and growth factors.104
In a variety of tissue types, IL-1, PDGF and TNF-α stimulate expression,105,106 while heparin, TGF-β and corticosteroids inhibit expression.107,108 In recent years, there has also been considerable interest in the regulatory role of extracellular matrix metalloproteinase inducer (EMMPRIN). EMMPRIN was initially identified as a tumour-derived protein that facilitated cancer cell invasion by stimulating MMP production in epithelial cells and fibroblasts.109 However, subsequent studies have demonstrated that EMMPRIN also stimulates production of MMPs by smooth muscle cells and monocytes, making it highly relevant in atherosclerosis and plaque instability. In addition, EMMPRIN may also lead to increased production of inflammatory cytokines which further augment MMP activity as described above.110
MMPs are initially secreted as latent inactive proenzymes and converted to the active state by cleavage of a propeptide domain.111 The major physiological activator is plasmin, which in turn is regulated by PAI.112 Thrombin has been shown to activate MMP-2 in vitro113 and could provide a mechanism for MMP activation at sites of vascular injury. Reactive oxygen species also modulate enzyme activation.114,115
Metalloproteinase activity is further governed by naturally occurring MMP inhibitors. These ‘tissue inhibitors of metallo proteinases’ (TIMPs) provides a further level of control and overall proteolytic activity depends on the ratio of activated MMPs to TIMPs.116
Early studies showed that MMPs were present at increased levels in atherosclerotic arteries. Raised levels of gelatinase activity were demonstrated in the aortas of patients with occlusive disease compared to healthy controls, and zymography revealed that this was predominantly MMP-9.117 Subsequently, quantitative studies using ELISA revealed a six-fold increase in MMP-9 levels in atherosclerotic aortas.118 The level and expression of MMP-2 is also increased in atherosclerotic aortic tissue compared with normal aorta.119 While expression of MMP-2 has been detected in normal arteries, it appears that most MMPs are expressed only in atherosclerotic tissue.120 The colocalisation of MMP-1, -2, -3 and -9 to the vulnerable shoulder of the plaque provided further evidence of their potential role in acute disruption.120
More recent studies have demonstrated an association between MMP levels and markers of plaque instability. Increased immunostaining for MMP-9 was seen in 12 atherectomy specimens retrieved from patients with unstable angina compared to the stable form.121 A larger study, involving 75 carotid endarterectomy specimens, demonstrated a close association between raised plaque levels of MMP-9 and a number of indicators of plaque instability, including symptomatology, cerebral embolisation and histological features of plaque rupture.9
Convincing evidence therefore exists of increased levels of MMP-2 and -9 in unstable plaques. However, intact type I and type III collagen molecules, which account for the load bearing strength of the plaque cap, are not substrates for MMP-2 and -9. While it has been reported that high concentrations of MMP-2 can degrade type I collagen in an in vitro environment devoid of TIMPs,122 it is likely that in vivo only the collagenases, MMP-1, -8 and -13, are capable of degrading fibrillar collagens.
MMP-1 and -13 levels are higher in ‘atheromatous’ compared to ‘fibrous’ plaques,123 and MMP-8 has been demonstrated in atheroma but not normal arteries.124 The expression of MMP-1 is increased in areas of high circumferential stress.125 It is likely that both mechanics and proteolysis play a role in the degradation and weakening of the collagen-rich extracellular matrix, and understanding their interaction may be crucial.126
Evidence from our laboratories suggests that active MMP-8 is significantly raised in unstable plaques retrieved at carotid endarterectomy (Figure 4.6). The ratio of active MMP-8 to TIMP-1 and -2 (its naturally occurring inhibitors) were also significantly higher in the more unstable plaques of the 159 specimens collected in this study. This implies net proteolysis of the types of collagen found in the cap of the plaque by MMP-8. Immunohistochemistry confirmed the presence of MMP-8 protein within the plaque, which colocalised with macrophages (Figure 4.7).

FIGURE 4.6
Plaque concentrations of active MMP-8 are significantly higher in symptomatic compared to asymptomatic carotid plaques: (a) from patients suffering carotid territory symptoms in the 6 months prior to surgery (p-value 0.0002), (b) from patients with pre-operative (more...)

FIGURE 4.7
Histological sections taken from the shoulder region of a symptomatic carotid plaque. Some sections show disruption of the friable plaque. (a) Low power H&E section with boxed area delineating high power view shown in (b-e). (b) High power H&E (more...)
Genetic variation in the genes controlling MMPs could theoretically be responsible for the susceptibility of some individuals to atherosclerotic plaque rupture. Early work has identified a number of polymorphisms that may be influential in this regard. Price et al have identified a novel genetic variation in the MMP-2 gene,127 Ye and colleagues detected a polymorphism in the promoter region of the MMP-3 gene that may lead to increased systemic levels.128 This polymorphism was subsequently found to be more common in patients suffering MI, compared to a control group.129 A single nucleotide polymorphism (C to T transition at position-1562) has been shown to influence MMP-9 transcription.130 In this study by Zhang and co-workers, triple-vessel coronary artery disease was detected by angiography in 26% of patients with this polymorphism compared to 15% of those without.130 Presenting a coherent picture of the interactions between various polymorphisms and the corresponding gene expression is difficult, and further complicated by environmental effects. However, it is clear that the potential exists to identify ‘at risk’ individuals in such a manner.
The vessel lumen
Disruption alone would not precipitate ischaemic syndromes without thrombus formation on the plaque surface, so plaque instability and thrombogenicity in tandem predispose to acute clinical events. Platelet adherence to the sub-endothelium after surface disruption leads to activation, with ADP and serotonin release stimulating further platelet recruitment and activation.
Once formed, thrombus can behave in three ways, dependent on the physical nature of the rupture and the balance between local fibrinolytic and coagulation processes. Firstly, the initial thrombus may progress to cause occlusion of the vessel. Secondly, the thrombus may disintegrate resulting in distal embolisation. Thirdly, the clot can undergo rapid dissolution, with the healed rupture resulting in a variable decrease in vessel lumen diameter.76
Tissue factor is a major regulator of haemostasis.131 It is the most thrombogenic component of atherosclerotic plaques132 and is expressed by numerous cell types, including endothelial cells. The level of tissue factor in coronary plaques from patients with unstable angina is more than twice the value observed in those plaques from stable angina patients.133 Positive immunostaining for tissue factor correlates with areas of intense macrophage infiltration and SMCs, suggesting a cell-mediated increased thrombogenicity in unstable plaques. The increase in tissue factor levels seems to be linked to expression of the CD-40 receptor on the macrophage cell surface. The CD-40 ligand is expressed on activated T-lymphocytes, and other atheroma-associated cells,134 which can therefore induce tissue factor production by macrophages via this signalling system. Expression is also regulated by cytokines and oxidised LDL.135,136 It has been reported that a blood-borne pool of tissue factor exists,137 though in the context of plaque disruption, macrophage production of tissue factor is predominantly responsible for plaque thrombogenicity.133,138,139 It is interesting to note that many of the recognised cardiovascular risk factors increase the expression of tissue factor.140,141
The Role of Angiogenesis in Plaque Rupture
Angiogenesis is essential for normal growth and development. Neovascularisation has been observed in plaques142 and it is postulated that it may play a role in atherosclerosis by providing growth factors and cytokines to regions of plaque development.
A study of coronary atherectomy specimens revealed the presence of neovascularisation in 50% of specimens from patients with unstable angina compared to 10% of specimens from patients with stable angina,29 suggesting a possible role in plaque instability. Angiogenesis may contribute to plaque instability by causing intraplaque haemorrhage or extravasation of erythrocytes and inflammatory mediators into the centre of the plaque. Once red blood cells have leaked into the plaque, cholesterol from the cell membrane may become incorporated into the lipid core increasing its volume.143 This is supported by the finding that lipid-rich plaques have a significantly higher microvessel density than fibrous plaques.144 The associated delivery of inflammatory cells may also lead to plaque degradation by stimulating MMP activity as described earlier in this chapter.
Perhaps more importantly, most neovascularisation occurs at the vulnerable shoulder area of the plaque. Immunostaining for inflammatory cells showed a close association between angiogenesis and inflammatory infiltration. In addition, a parallel increase in the expression of leukocyte adhesion molecules in the same vulnerable areas was demonstrated.144
Angiogenesis involves interactions between endothelial cells and components of the basement membrane matrix. MMP activity is required for such interactions, especially MMP-2 and MT1-MMP.145 TIMPs have been shown to reduce angiogenesis, while up regulation of MMP activity stimulates its increase.146 However, whilst neovascularisation may promote and sustain inflammatory infiltration, the converse may also be true, whereby changes in the plaque associated with inflammation may themselves promote angiogenesis. Further work in this area is required.
The Role of Infectious Agents in Plaque Rupture
The role of infectious agents in atherosclerosis and plaque rupture is controversial. Definitive proof of a causal relationship is lacking, although studies have reported associations between plaque development and Chlamydia pneumoniae,147-149 Helicobacter pylori,150 cytomegalovirus,151-152 Herpes simplex virus types 1 and 2,153 and hepatitis A virus.154
Certain infectious agents can evoke cellular and molecular changes supportive of a role in atherogenesis.155 Work has shown that Chlamydial interaction with monocytes results in upregulation of TNF-α and IL-1β,156,157 both of which are associated with plaque development. Chlamydial production of the HSP-60 antigen activates human vascular endothelium, and increases TNF-α and MMP expression in macrophages.158,159 Once again, these are factors that influence plaque stability.
It has also been proposed that infective pathogens may exert their effects via direct infection of cells in the vessel wall. This establishes localised inflammation, leading to increased smooth muscle cell migration and greater uptake of oxidised low-density lipoprotein.160
There is some doubt about the methods employed for Chlamydia detection,161 and also the role of potential confounding factors in epidemiological studies.162 A large-scale prospective study of 15,000 healthy men in the United States which was controlled for age, smoking, socio-economic status and other cardiovascular risk factors, failed to show any association between Chlamydia seropositivity and the risk of MI.163
More recently, the staMIna trial164 demonstrated that eradication therapy (amoxicillin/azithromycin, metronidazole and omeprazole) administered for 1-week after an acute coronary syndrome, significantly reduced cardiac death and acute coronary syndrome readmission rates over the following 12 months. These effects were unrelated to Chlamydia pneumoniae or Helicobacter pylori seropositivity, however, suggesting that the trial therapy prevented lesion progression by a mechanism unrelated to its antibiotic action.
Though the role of infection in atherosclerosis is still unclear, it seems that any causal relationship is likely to be highly complex and involve both direct and indirect pathways. Important factors may also include the patient’s susceptibility to infection and their innate inflammatory and immune responses.165
Risk Prediction of Plaque Instability
Imaging
Angiography can demonstrate ulceration166 but does not appear to be able to adequately distinguish between stable and unstable plaques.167 In addition, the degree of stenosis detected by angiography does not correlate well with the future risk of events19-21 because, as already discussed, it is often not the most stenotic plaques that are at highest risk of rupture.
Conventional ultrasound studies have shown an association between carotid plaque morphology and neurological symptoms168 but have been unable to predict the risk of future events.23 Intravenous ultrasound (IVUS), however, has been shown to have much greater resolution (100 µm) and provides detailed crosssectional images of the arterial wall. It is also able to identify the increased echolucence of lipid--rich plaques and for a time it was thought that it might prove useful in detection of rupture prone plaques.169 Unfortunately, sensitivity and specificity were found to be low with this technique and it has largely been superseded by intravenous ultrasound virtual histology (IVU-SVH). The improved spectral analysis offered by this technology allows more detailed plaque characterization and can provide detail on lipid content, calcification and volume of the necrotic core.170 Recent studies have shown this may be a clinically useful tool and demonstrated that IVU-SVH identified more TCFAs in patients with acute coronary syndrome than in those with stable angina pectoris.171
In parallel with the development of IVUS-VH, many groups have now begun to use optical coherence tomography (OCT). Also an intravenous modality, OCT is analogous to ultrasound imaging (using light rather than sound waves) and provides excellent spatial resolution (10-15 µm). This allows detailed assessment of the arterial wall and can identify those plaques with a fibrous cap less than 65µm thick (i.e. TCFA) as well as areas of increased echolucency.172 Whilst this technique has yielded very encouraging results in the identification of culprit atherosclerotic lesions, it is not without its limitations. Since blood attenuates the optical signal, the vessel under investigation must be proximally occluded for considerable periods to allow accurate imaging. An updated version of the technology has therefore been developed in recent years. This second generation of OCT is known as optical frequency domain imaging (OFDI) and involves much higher frame rates (>100 frames/sec). The higher frame rate allows rapid three dimensional imaging of long arterial segments using high speed pull back of the probe. This means there is no need for proximal occlusion of the vessel and the artery can simply be purged with saline just prior to imaging173 Further investigation will be needed to assess the true clinical utility of this technique.
Since increased inflammatory activity occurs prior to plaque rupture, attempts have been made to detect this increase, using local temperature measurements. Thermography studies have shown that temperature correlates well with macrophage cell density in human carotid plaques.174 The temperature of coronary vessels in patients with ischaemic heart disease, in particular acute coronary syndromes, is higher than in normal controls.175 In addition, increased local plaque temperature has been shown to be an independent predictor of adverse clinical outcome.176
High-resolution MRI appears to characterize the atherosclerotic plaque better than other imaging techniques.177 It is more accurate then angiography in measuring the degree of stenosis and, unlike angiography and IVUS, is non-invasive. However, a multicentre trial of imaging in coronary artery disease found whilst that MRI could reliably identify significant intraluminal lesions and rule out proximal or three-vessel disease, specificity was low.178 This led to the suggestion that MRI may be more sensitive and specific if combined with intravascular enhancing agents such as gadolinium. Using such a marker improved MRI specificity and facilitated identification of carotid TCFA.179
MRI is still technically limited in many cases by small vessel size and movement arte fact, and studies have not yet demonstrated the ability to predict risk of future cardiovascular events. Nonetheless, advances in the technique suggest a potential future role for MRI in detection of the high-risk plaque.
Just as enhancing agents may increase the accuracy of MRI, they may also prove useful in identifying atherosclerotic lesions using positron emission tomography (PET) and there has been increasing interest in the use of18 fluorodeoxyglucose (18FDG). Uptake of this glucose analogue is increased in metabolically active cells and early animal studies suggest it enriches in plaque macrophages and indicates areas of neovascularisation.180 However, the clinical application of this technique has yet to be demonstrated.
Blood markers
It has long been established that adverse lipid profiles correlate with increased risk of MI and stroke though this is not a direct predictor of plaque rupture. Raised CRP levels have also been associated with increased cardiovascular risk in apparently healthy patients,181,182 though its use as a prognostic marker of clinically significant thrombosis remains controversial.
MMP-2 and MMP-9 are raised in the peripheral blood of patients suffering from acute coronary syndromes,183 while plasma MMP-9 is raised in patients with unstable carotid plaques.184 A recent study of 1127 patients with coronary artery disease identified baseline plasma MMP-9 levels to be a novel predictor of cardiovascular mortality.185
Similarly, raised serum levels of soluble intercellular adhesion molecule-1 (slCAM-1) have been shown to be an independent predictor of future coronary event in patients with coronary heart disease.186
Many other molecules have also been investigated as potential prognostic markers in progression of atherosclerosis, including cytokines, lipoproteins, myeloperoxidases and placental growth factor. Though some have yielded promising results, none has yet been widely accepted as a reliable predictor of plaque rupture or clinical events.187
Therapy Aimed at Plaque Stabilisation
Pharmacotherapy to induce plaque stabilisation could be targeted at different aspects of the complex pathway leading up to plaque rupture, in particular:
- the endothelium – by increasing endothelial passivation
- the lipid core – by reducing LDL deposition/augmenting LDL removal
- the fibrous cap – by increasing collagen deposition/preventing collagen degradation
- the vessel lumen – by altering the thrombogenicity of the local environment.
Most recent interest has focussed on the role of HMG Co-a reductase inhibitors, which appear capable of influencing plaque stabilisation at all these levels.
HMG Co-A Reductase Inhibitors
HMG Co-A reductase inhibitors, or statins, are well known for their lipid-lowering action. They are the most effective group of therapeutic agents for lowering LDL and raising HDL levels. However, recent evidence suggests that they are also capable of decreasing cardiovascular events in those with normal cholesterol levels.188,189 The Oxford Heart Protection Study189 was a randomised controlled trial of simvastatin versus placebo in 20,536 individuals at high-risk of cardiovascular disease. Coronary death rate and other vascular events were significantly reduced in the simvastatin groups, even in patients with lipid levels below currently recommended targets (<5mmol/l total cholesterol and <3mmol/l LDL-cholesterol).
In the lipid lowering arm of the Anglo-Scandinavian Cardiac Outcomes Trial (ASCOT),188 10,305 individuals with total cholesterol levels <6.5mmol/l were randomised to either atorvastatin or placebo. The trial was stopped 1.7 years before the planned 5-year follow-up target was reached, as there were significantly fewer cardiovascular events in the atorvastatin group. The observed clinical benefit is probably a combination of lipid lowering below levels previously considered ‘normal’ and additional lipid-independent plaque stabilising actions. Several studies have reported effects other than lipid-lowering properties, including anti-proteolytic and anti-inflammatory mechanisms.190,191
Statins increase nitric oxide synthase activity192 and encourage endothelial passivation (Figure 4.2). As discussed earlier, nitric oxide causes vasodilatation, inhibition of SMC proliferation and platelet aggregation and has widespread anti-inflammatory and anti-oxidant properties. Statins also reduce the expression of cell adhesion molecules,193 interfering with the adherence of monocytes to the endothelium.
Statins may also have direct anti inflammatory and antiproteolytic actions, which contribute to increased plaque stability. In cell culture and animal models, statins have been shown to reduce macrophage secretion of MMP-1, -2, -3 and -9,194 and increase the collagen content of the plaque.195 Also, CRP levels are decreased by statins in a lipid-independent manner.191,196
Work from our laboratories suggests that statin therapy stabilises carotid plaques by lowering the levels of MMP-1, MMP-9 and IL-6. In an observational non-randomised study of 137 patients, we found that patients on statin therapy were significantly less likely to have suffered carotid territory symptoms within the month prior to carotid endarterectomy. The number of patients undergoing spontaneous pre-operative cerebral embolization was also significantly lower in the statin group.
HMG Co-a reductase inhibitors also have the potential to reduce thrombogenicity by decreasing tissue factor activity197 and lowering levels of PaI-1.198,199
MMP Inhibition
The realisation that tissue remodelling due to increased MMP activity plays a key role in disease states has led to considerable interest in the potential for MMP inhibition. Most clinical and preclinical data regarding therapeutic manipulation of the extracellular matrix has been in the fields of arthritis, periodontal disease and cancer.103 MMP inhibition aimed at plaque stabilisation aims to redress the imbalance between enzymes and inhibitors, which causes excessive tissue degradation. Potential methods of MMP inhibition include the administration of:
Tissue Inhibitors of Metalloproteinases (TIMPs)
The level of TIMPs can be increased either by the exogenous administration of recombinant TIMPs or by stimulating their local production through gene therapy. Increased TIMP-1 raised the collagen, elastin and smooth muscle content of atherosclerotic lesions in animal models,200 while local gene transfer of TIMP-2 has been shown to decrease vascular remodelling in conjunction with lowered MMP activity (experimental models).201
It is difficult to extrapolate these data to potential applications in humans. The major drawback associated with TIMPs would be tissue delivery, since exogenous products would be metabolised and denatured with minimal tissue penetration at the intended site of action. Systemic stimulation of TIMPs would almost certainly have significant side effects precluding clinical use. Therefore, treatment would have to take the form of local tissue delivery or gene therapy. Clearly either system will be very expensive to develop, so more interest has concentrated on the development of synthetic MMP inhibitors.
Synthetic MMP inhibitors
Synthetic peptides work by binding to the zinc ion at the active site of the MMP, thus preventing cleavage of substrate collagen molecules.202 Batimastat showed promise in decreasing tumour development and metastasis (animal models)203 and limiting aneurysm expansion (experimental models),204 but is not available in an oral form. Marimastat, which is available orally, was shown to limit intimal hyperplasia205 and aneurysm expansion in vivo.206 It also showed promise in early human cancer studies, but caused significant musculoskeletal side effects in 30% of patients.207 Recent studies of MMI270, a more specific inhibitor (of MMP-2, MMP-8 and MMP-9), have shown a similar side effect profile.208 Furthermore, recent animal studies of broad-spectrum synthetic MMP inhibitors have found them to be generally deleterious in terms of both plaque growth and plaque stability.209
Doxycycline
Doxycycline, a member of the tetracycline antibiotic family, is also a non selective MMP inhibitor,210 with a proven safety profile. Clinical trials have shown that doxycycline is capable of decreasing cartilage MMP levels when given to patients prior to hip surgery.211 It has also been shown to limit intimal hyperplasia212 and aneurysm expansion in vivo,213 by reducing MMP-9 activity. Furthermore, when given to patients prior to AAA repair the expression of MMP-2 and MMP-9 was reduced in the aortic wall.214
A randomised clinical trial of doxycycline versus placebo in patients prior to carotid endarterectomy demonstrated decreased plaque MMP-1 levels and a potential for clinical benefit.215 A phase II study of doxycycline administration to patients with small AAAs recently showed that it was reasonably well-tolerated (92% completed the 6-month course) and reduced plasma MMP-9 levels.216 Further studies are on going to evaluate its effects on small aneurysm expansion.
ACE Inhibitors
ACE inhibitors (ACEI) and angiotensin II receptor antagonists decrease cardiovascular events, independently of their effects on blood pressure control. The ACEI, trandalopril, and the experimental angiotensin receptor antagonist, HR720, decrease the area of atherosclerotic lesions in the thoracic aorta of cholesterolfed monkeys.217 This was achieved without alteration of mean blood pressure or cholesterol levels. The Heart Outcomes Prevention Evaluation (HOPE) study demonstrated a decrease in cardiovascular events in high-risk patients given ramipril as opposed to placebo.218 This effect could only be partly explained by the modest decrease in mean blood pressure seen between the 2 groups (3/2mmHg).
Angiotensin II promotes endothelial activation,219 and therefore the mechanism of action of ACEIs could be through endothelial passivation (leading to a reduction in cell adhesion molecule expression and macrophage infiltration). ACEIs may also exert their effects through bradykinin potentiation, resulting in decreased smooth muscle cell migration, decreased inflammation and decreased production of oxygen free radicals (COPPOLA 2008). Navalkar et al provided biochemical evidence to support these hypotheses by demonstrating that irbesartan (an angiotensin II receptor blocker) can decrease plasma levels of VCAM-1, TNF-α and superoxide.220
With ever more detailed understanding of the human genome, gene therapies have also come under investigation in the search for antiatherosclerotic therapies. Hans et al have demonstrated that polya DP ribose polymerase (PARP 1), a DNA-repair protein, stimulates apoptosis in the presence of local inflammation and plays an important role in plaque dynamics. They went on to show that inhibition of PARP-1 resulted in a reduction in plaque size, decreased collagen degradation and increased plaque smooth muscle content in ApoE(-/-) mice.221 These findings suggest that PARP-1 inhibition may also represent a valuable therapeutic tool, though its applicability in humans has yet to be demonstrated.
Since the clinical significance of any plaque rupture is also governed by the intravascular environment, investigators continue to seek new therapies that may decrease the thrombogenicity of blood. Until now, this has been achieved with a combination of aspirin and another antiplatelet agent – most commonly clopidogrel – but drug resistance and side effect profiles can limit its applicability. The latest class of antiplatelet drugs is the P2Y12 blockers, which inhibits platelet activation via blockade of the P2Y12 ADP-receptor. Though these drugs (such as prasugrel and ticagrelor) may still have significant side effect profiles, they seem to be associated with far less unwanted bleeding and may be effective in patients who do not respond to clopidogrel.222
Tough a number of therapeutic targets have shown promise in preventing plaque rupture, substantial work is still needed in this area since many of the potential therapeutic targets (such as smooth muscle cells and macrophages) have the ability to play both detrimental and beneficial roles in the complex process of atherosclerosis.
Summary
Acute plaque disruption precedes the onset of clinical ischaemic syndromes. Exposure of the highly thrombogenic core to luminal blood results in platelet adherence and thrombosis. Inflammation is clearly involved in the process of plaque development and acute disruption, though the precise mechanism by which the inflammatory process is initiated remains unclear. The roles of angiogenesis, cellular apoptosis and infectious agents also require further clarification. Unstable plaques have a large lipid core and a thin fibrous cap with reduced collagen content. A major component of plaque destabilisation appears to be increased matrix degradation, the primary regulators of which are the MMPs and their inhibitors. There are a number of potential therapeutic options aimed at preventing plaque disruption. In particular, MMP inhibition is an attractive target for such pharmacotherapy.
References
- 1.
- Casscells W, Naghavi M, Willerson JT. Vulnerable atherosclerotic plaque: a multifocal disease. Circulation 2003; 107(16): 2072–5. [PubMed: 12719287]
- 2.
- Little WC. Angiographic assessment of the culprit coronary artery lesion before acute myocardial infarction. The American journal of cardiology 1990; 66(16): 44G–47G. [PubMed: 2239714]
- 3.
- Naghavi M, Libby P, Falk E, Casscells SW, Litovsky S, Rumberger J, et al. From vulnerable plaque to vulnerable patient: a call for new definitions and risk assessment strategies: Part I. Circulation 2003; 108(14): 1664–72. [PubMed: 14530185]
- 4.
- Falk E, Shah PK, Fuster V. Coronary plaque disruption. Circulation 1995; 92(3): 657–71. [PubMed: 7634481]
- 5.
- Libby P. Current concepts of the pathogenesis of the acute coronary syndromes. Circulation 2001; 104(3): 365–72. [PubMed: 11457759]
- 6.
- Shah PK. Plaque disruption and thrombosis: potential role of inflammation and infection. Cardiology in review 2000; 8(1): 31–9. [PubMed: 11174871]
- 7.
- Falk E. Unstable angina with fatal outcome: dynamic coronary thrombosis leading to infarction and/or sudden death. Autopsy evidence of recurrent mural thrombosis with peripheral embolization culminating in total vascular occlusion. Circulation 1985; 71(4): 699–708. [PubMed: 3971539]
- 8.
- Carr S, Farb A, Pearce WH, Virmani R, Yao JS. Atherosclerotic plaque rupture in symptomatic carotid artery stenosis. J Vasc Surg 1996; 23(5): 755–65; discussion 65–6. [PubMed: 8667496]
- 9.
- Loftus IM, Naylor AR, Goodall S, Crowther M, Jones L, Bell PR, et al. Increased matrix metalloproteinase-9 activity in unstable carotid plaques. A potential role in acute plaque disruption. Stroke 2000; 31(1): 40–7. [PubMed: 10625713]
- 10.
- Sitzer M, Müller W, Siebler M, Hort W, Kniemeyer HW, Jäncke L, et al. Plaque ulceration and lumen thrombus are the main sources of cerebral microemboli in high-grade internal carotid artery stenosis. Stroke 1995; 26(7): 1231–3. [PubMed: 7604420]
- 11.
- Davies MJ, Thomas A. Thrombosis and acute coronary artery lesions in sudden cardiac ischemic death. N Engl J Med 1984; 310(18): 1137–40. [PubMed: 6709008]
- 12.
- Falk E. Plaque rupture with severe preexisting stenosis precipitating coronary thrombosis. Characteristics of coronary atherosclerotic plaques underlying fatal occlusive thrombi. Br Heart J 1983; 50(2): 127–34. [PMC free article: PMC481384] [PubMed: 6882602]
- 13.
- Friedman M. Pathogenesis of coronary thrombosis, intramural and intraluminal hemorrhage. Advances in cardiology 1970; 4: 20–46. [PubMed: 4924082]
- 14.
- Forrester JS, Litvack F, Grundfest W, Hickey A. A perspective of coronary disease seen through the arteries of living man. Circulation 1987; 75(3): 505–13. [PubMed: 3815762]
- 15.
- Sherman CT, Litvack F, Grundfest W, Lee M, Hickey A, Chaux A, et al. Coronary angioscopy in patients with unstable angina pectoris. N Engl J Med 1986; 315(15): 913–9. [PubMed: 3489893]
- 16.
- Davies MJ. The pathophysiology of acute coronary syndromes. Heart 2000; 83(3): 361–6. [PMC free article: PMC1729334] [PubMed: 10677422]
- 17.
- Felton CV, Crook D, Davies MJ, Oliver MF. Relation of plaque lipid composition and morphology to the stability of human aortic plaques. Arterioscler Thromb Vasc Biol 1997; 17(7): 1337–45. [PubMed: 9261265]
- 18.
- Kolodgie FD, Burke AP, Farb A, Gold HK, Yuan J, Narula J, et al. The thin cap fibroatheroma: a type of vulnerable plaque: the major precursor lesion to acute coronary syndromes. Curr Opin Cardiol 2001; 16(5): 285–92. [PubMed: 11584167]
- 19.
- Ambrose JA, Winters SL, Stern A, Eng A, Teichholz LE, Gorlin R, et al. Angiographic morphology and the pathogenesis of unstable angina pectoris. Journal of the American College of Cardiology 1985; 5(3): 609–16. [PubMed: 3973257]
- 20.
- Giroud D, Li JM, Urban P, Meier B, Rutishauer W. Relation of the site of acute myocardial infarction to the most severe coronary arterial stenosis at prior angiography. The American journal of cardiology 1992; 69(8): 729–32. [PubMed: 1546645]
- 21.
- Hackett D, Davies G, Maseri A. Preexisting coronary stenoses in patients with first myocardial infarction are not necessarily severe. European Heart Journal 1988; 9(12): 1317–23. [PubMed: 3229426]
- 22.
- Markus HS, Thomson ND, Brown MM. Asymptomatic cerebral embolic signals in symptomatic and asymptomatic carotid artery disease. Brain 1995; 118 (Pt 4): 1005–11. [PubMed: 7655877]
- 23.
- Golledge J, Cuming R, Ellis M, Davies AH, Greenhalgh RM. Carotid plaque characteristics and presenting symptom. Br J Surg 1997; 84(12): 1697–701. [PubMed: 9448618]
- 24.
- Gunning A, Pickering G, Robbs-Smith A, Russell R. Mural thrombosis of the subclavian artery and subsequent embolism in cervical rib Q J Med 1964; 33: 133–54. [PubMed: 14116852]
- 25.
- Harrison MJ, Marshall J. The finding of thrombus at carotid endarterectomy and its relationship to the timing of surgery. Br J Surg 1977; 64(7): 511–2. [PubMed: 922314]
- 26.
- Bogousslavsky J, Van Melle G, Regli F. The Lausanne Stroke Registry: analysis of 1,000 consecutive patients with first stroke. Stroke 1988; 19(9): 1083–92. [PubMed: 3413804]
- 27.
- Imparato AM, Riles TS, Gorstein F. The carotid bifurcation plaque: pathologic findings associated with cerebral ischemia. Stroke 1979; 10(3): 238–45. [PubMed: 462508]
- 28.
- Persson AV, Robichaux WT, Silverman M. The natural history of carotid plaque development. Arch Surg 1983; 118(9): 1048–52. [PubMed: 6615213]
- 29.
- Lusby RJ, Ferrell LD, Ehrenfeld WK, Stoney RJ, Wylie EJ. Carotid plaque hemorrhage. Its role in production of cerebral ischemia. Arch Surg 1982; 117(11): 1479–88. [PubMed: 6182861]
- 30.
- Tenaglia AN, Peters KG, Sketch MH, Annex BH. Neovascularization in atherectomy specimens from patients with unstable angina: implications for pathogenesis of unstable angina. American heart journal 1998; 135(1): 10–4. [PubMed: 9453515]
- 31.
- Van Damme H, Vivario M. Pathologic aspects of carotid plaques: surgical and clinical significance. Int Angiol 1993; 12(4): 299–311. [PubMed: 8207303]
- 32.
- Barua RS, Ambrose JA, Srivastava S, DeVoe MC, Eales-Reynolds L-J. Reactive oxygen species are involved in smoking-induced dysfunction of nitric oxide biosynthesis and upregulation of endothelial nitric oxide synthase: an in vitro demonstration in human coronary artery endothelial cells. Circulation 2003; 107(18): 2342–7. [PubMed: 12707237]
- 33.
- Barua RS, Ambrose JA,Saha DC, Eales-Reynolds L-J. Smoking is associated with altered endothelial-derived fibrinolytic and antithrombotic factors: an in vitro demonstration. Circulation 2002; 106(8): 905–8. [PubMed: 12186791]
- 34.
- Hanratty CG, McGrath LT, McAuley DF, Young IS, Johnston GD. The effects of oral methionine and homocysteine on endothelial function. Heart 2001; 85(3): 326–30. [PMC free article: PMC1729651] [PubMed: 11179277]
- 35.
- Lüscher TF, Tanner FC, Noll G. Lipids and endothelial function: effects of lipid-lowering and other therapeutic interventions. Current opinion in lipidology 1996; 7(4): 234–40. [PubMed: 8883499]
- 36.
- Salt IP, Morrow VA, Brandie FM, Connell JMC, Petrie JR. High glucose inhibits insulin-stimulated nitric oxide production without reducing endothelial nitric-oxide synthase Ser1 177 phosphorylation in human aortic endothelial cells. J Biol Chem 2003; 278(21): 18791–7. [PubMed: 12644458]
- 37.
- Taddei S, Virdis A, Ghiadoni L, Sudano I, Salvetti A. Endothelial dysfunction in hypertension. J Cardiovasc Pharmacol 2001; 38 Suppl 2: s11–4. [PubMed: 11811368]
- 38.
- Cybulsky MI, Gimbrone MA. Endothelial expression of a mononuclear leukocyte adhesion molecule during atherogenesis. Science 1991; 251(4995): 788–91. [PubMed: 1990440]
- 39.
- van der Wal AC, Das PK, Tigges AJ, Becker AE. Adhesion molecules on the endothelium and mononuclear cells in human atherosclerotic lesions. Am J Pathol 1992; 141(6): 1427–33. [PMC free article: PMC1886778] [PubMed: 1281621]
- 40.
- Vanhoutte PM. Endothelial dysfunction and atherosclerosis. European Heart Journal 1997; 18 Suppl E: E19–29. [PubMed: 9402468]
- 41.
- De Caterina R, Libby P, Peng HB, Thannickal VJ, Rajavashisth TB, Gimbrone MA, et al. Nitric oxide decreases cytokine-induced endothelial activation. Nitric oxide selectively reduces endothelial expression of adhesion molecules and proinflammatory cytokines. J Clin Invest 1995; 96(1): 60–8. [PMC free article: PMC185173] [PubMed: 7542286]
- 42.
- Moncada S, Higgs A. The L-arginine nitric oxide pathway. N Engl J Med 1993; 329(27): 2002–12. [PubMed: 7504210]
- 43.
- Kingwell BA, Sherrard B, Jennings GL, Dart AM. Four weeks of cycle training increases basal production of nitric oxide from the forearm. Am J Physiol 1997; 272(3 Pt 2): H1070–7. [PubMed: 9087577]
- 44.
- Malek AM, Alper SL, Izumo S. Hemodynamic shear stress and its role in atherosclerosis. Jama 1999; 282(21): 2035–42. [PubMed: 10591386]
- 45.
- MA Gimbrone, Resnick N, Nagel T, Khachigian LM, Collins T, Topper JN. Hemodynamics, endothelial gene expression, and atherogenesis. Ann N Y Acad Sci 1997; 811: 1–10; discussion 10–1. [PubMed: 9186579]
- 46.
- Nadaud S, Philippe M, Arnal JF, Michel JB, Soubrier F. Sustained increase in aortic endothelial nitric oxide synthase expression in vivo in a model of chronic high blood flow. Circ Res 1996; 79(4): 857–63. [PubMed: 8831511]
- 47.
- De Keulenaer GW, Chappell DC, Ishizaka N, Nerem RM, Alexander RW, Griendling KK. Oscillatory and steady laminar shear stress differentially affect human endothelial redox state: role of a superoxide-producing NADH oxidase. Circ Res 1998; 82(10): 1094–101. [PubMed: 9622162]
- 48.
- Chappell DC, Varner SE, Nerem RM, Medford RM, Alexander RW. Oscillatory shear stress stimulates adhesion molecule expression in cultured human endothelium. Circ Res 1998; 82(5): 532–9. [PubMed: 9529157]
- 49.
- Lee RT, Kamm RD. Vascular mechanics for the cardiologist. Journal of the American College of Cardiology 1994; 23(6): 1289–95. [PubMed: 8176085]
- 50.
- Cheng GC, Loree HM, Kamm RD, Fishbein MC, Lee RT. Distribution of circumferential stress in ruptured and stable atherosclerotic lesions. A structural analysis with histopathological correlation. Circulation 1993; 87(4): 1179–87. [PubMed: 8462145]
- 51.
- Loree HM, Kamm RD, Stringfellow RG, Lee RT. Effects of fibrous cap thickness on peak circumferential stress in model atherosclerotic vessels. Circ Res 1992; 71(4): 850–8. [PubMed: 1516158]
- 52.
- MacIsaac AI, Thomas JD, Topol EJ. Toward the quiescent coronary plaque. Journal of the American College of Cardiology 1993; 22(4): 1228–41. [PubMed: 8104965]
- 53.
- Grønholdt ML, Dalager-Pedersen S, Falk E. Coronary atherosclerosis: determinants of plaque rupture. European Heart Journal 1998; 19 Suppl C: C24–9. [PubMed: 9597422]
- 54.
- Kragel AH, Reddy SG, Wittes JT, Roberts WC. Morphometric analysis of the composition of atherosclerotic plaques in the four major epicardial coronary arteries in acute myocardial infarction and in sudden coronary death. Circulation 1989; 80(6): 1747–56. [PubMed: 2598434]
- 55.
- Gertz SD, Roberts WC. Hemodynamic shear force in rupture of coronary arterial atherosclerotic plaques. The American journal of cardiology 1990; 66(19): 1368–72. [PubMed: 2244569]
- 56.
- Davies MJ, Richardson PD, Woolf N, Katz DR, Mann J. Risk of thrombosis in human atherosclerotic plaques: role of extracellular lipid, macrophage, and smooth muscle cell content. Br Heart J 1993; 69(5): 377–81. [PMC free article: PMC1025095] [PubMed: 8518056]
- 57.
- el-Barghouti N, Nicolaides AN, Tegos T, Geroulakos G. The relative effect of carotid plaque heterogeneity and echogenicity on ipsilateral cerebral infarction and symptoms of cerebrovascular disease. Int Angiol 1996; 15(4): 300–6. [PubMed: 9127769]
- 58.
- Reilly LM, Lusby RJ, Hughes L, Ferrell LD, Stoney RJ, Ehrenfeld WK. Carotid plaque histology using real-time ultrasonography. Clinical and therapeutic implications. Am J Surg 1983; 146(2): 188–93. [PubMed: 6192733]
- 59.
- Feeley TM, Leen EJ, Colgan MP, Moore DJ, Hourihane DO, Shanik GD. Histologic characteristics of carotid artery plaque. J Vasc Surg 1991; 13(5): 719–24. [PubMed: 2027212]
- 60.
- Grønholdt M-LM, Nordestgaard BG, Bentzon J, Wiebe BM, Zhou J, Falk E, et al. Macrophages are associated with lipid-rich carotid artery plaques, echolucency on B-mode imaging, and elevated plasma lipid levels. J Vasc Surg 2002; 35(1): 137–45. [PubMed: 11802145]
- 61.
- Takano M, Mizuno K, Okamatsu K, Yokoyama S, Ohba T, Sakai S. Mechanical and structural characteristics of vulnerable plaques: analysis by coronary angioscopy and intravascular ultrasound. Journal of the American College of Cardiology 2001; 38(1): 99–104. [PubMed: 11451303]
- 62.
- Steinberg D. Low density lipoprotein oxidation and its pathobiological significance. J Biol Chem 1997; 272(34): 20963–6. [PubMed: 9261091]
- 63.
- Nicholson AC, Han J, Febbraio M, Silversterin RL, Hajjar DP. Role of CD36, the macrophage class B scavenger receptor, in atherosclerosis. Ann N Y Acad Sci 2001; 947: 224–8. [PubMed: 11795270]
- 64.
- Kruth HS, Huang W, Ishii I, Zhang W-Y. Macrophage foam cell formation with native low density lipoprotein. J Biol Chem 2002; 277(37): 34573–80. [PubMed: 12118008]
- 65.
- de la Llera Moya M, Atger V, Paul JL, Fournier N, Moatti N, Giral P, et al. A cell culture system for screening human serum for ability to promote cellular cholesterol efflux. Relations between serum components and efflux, esterification, and transfer. Arterioscler Thromb 1994; 14(7): 1056–65. [PubMed: 8018660]
- 66.
- Badimon JJ, Badimon L, Fuster V. Regression of atherosclerotic lesions by high density lipoprotein plasma fraction in the cholesterolfed rabbit. J Clin Invest 1990; 85(4): 1234–41. [PMC free article: PMC296557] [PubMed: 2318976]
- 67.
- Spieker LE, Sudano I, Hürlimann D, Lerch PG, Lang MG, Binggeli C, et al. High-density lipoprotein restores endothelial function in hypercholesterolemic men. Circulation 2002; 105(12): 1399–402. [PubMed: 11914243]
- 68.
- Barter PJ. Inhibition of endothelial cell adhesion molecule expression by high density lipoproteins. Clin Exp Pharmacol Physiol 1997; 24(3–4): 286–7. [PubMed: 9131299]
- 69.
- Lin K-Y, ChenY-L, ShihC-C, PanJ-P, Chan W-E, Chiang A-N. Contribution of HDL-apolipoproteins to the inhibition of low density lipoprotein oxidation and lipid accumulation in macrophages. J Cell Biochem 2002; 86(2): 258–67. [PubMed: 12111995]
- 70.
- Abela GS, Aziz K. Cholesterol crystals rupture biological membranes and human plaques during acute cardiovascular events--a novel insight into plaque rupture by scanning electron microscopy. Scanning 2006; 28(1): 1–10. [PubMed: 16502619]
- 71.
- Vedre A, Pathak DR, Crimp M, Lum C, Koochesfahani M, Abela GS. Physical factors that trigger cholesterol crystallization leading to plaque rupture. Atherosclerosis 2009; 203(1): 89–96. [PubMed: 18703195]
- 72.
- Koskinas KC, Chatzizisis YS, Baker AB, Edelman ER, Stone PH, Feldman CL. The role of low endothelial shear stress in the conversion of atherosclerotic lesions from stable to unstable plaque. Curr Opin Cardiol 2009; 24(6): 580–90. [PubMed: 19809311]
- 73.
- Farb A, Burke AP, Tang AL, Liang TY, Mannan P, Smialek J, et al. Coronary plaque erosion without rupture into a lipid core. A frequent cause of coronary thrombosis in sudden coronary death. Circulation 1996; 93(7): 1354–63. [PubMed: 8641024]
- 74.
- van der Wal AC, Becker AE, van der Loos CM, Das PK. Site of intimal rupture or erosion of thrombosed coronary atherosclerotic plaques is characterized by an inflammatory process irrespective of the dominant plaque morphology. Circulation 1994; 89(1): 36–44. [PubMed: 8281670]
- 75.
- Virmani R, Kolodgie FD, Burke AP, Farb A, Schwartz SM. Lessons from sudden coronary death: a comprehensive morphological classification scheme for atherosclerotic lesions. Arterioscler Thromb Vasc Biol 2000; 20(5): 1262–75. [PubMed: 10807742]
- 76.
- Burke AP, Kolodgie FD, Farb A, Weber DK, Malcom GT, Smialek J, et al. Healed plaque ruptures and sudden coronary death: evidence that subclinical rupture has a role in plaque progression. Circulation 2001; 103(7): 934–40. [PubMed: 11181466]
- 77.
- Burleigh MC, Briggs AD, Lendon CL, Davies MJ, Born GV, Richardson PD. Collagen types I and III, collagen content, GAGs and mechanical strength of human atherosclerotic plaque caps: span wise variations. Atherosclerosis 1992; 96(1): 71–81. [PubMed: 1418104]
- 78.
- Davies MJ. Stability and instability: two faces of coronary atherosclerosis. The Paul Dudley White Lecture 1995. Circulation 1996; 94(8): 2013–20. [PubMed: 8873680]
- 79.
- Kockx MM. Apoptosis in the atherosclerotic plaque: quantitative and qualitative aspects. Arterioscler Thromb Vasc Biol 1998; 18(10): 1519–22. [PubMed: 9763521]
- 80.
- Kockx MM, De Meyer GR, Muhring J, Jacob W, Bult H, Herman AG. Apoptosis and related proteins in different stages of human atherosclerotic plaques. Circulation 1998; 97(23): 2307–15. [PubMed: 9639374]
- 81.
- Vengrenyuk Y, Carlier S, Xanthos S, Cardoso L, Ganatos P, Virmani R, et al. A hypothesis for vulnerable plaque rupture due to stress induced debonding around cellular microcalcifications in thin fibrous caps. Proc Natl Acad Sci USA 2006; 103(40): 14678–83. [PMC free article: PMC1595411] [PubMed: 17003118]
- 82.
- Barnes MJ, Farndale RW. Collagens and atherosclerosis. Exp Gerontol 1999; 34(4): 513–25. [PubMed: 10817807]
- 83.
- Dilley RJ, McGeachie JK, Prendergast FJ. A review of the proliferative behaviour, morphology and phenotypes of vascular smooth muscle. Atherosclerosis 1987; 63(2–3): 99–107. [PubMed: 3548737]
- 84.
- Newby AC. Molecular and cell biology of native coronary and veingraft atherosclerosis: regulation of plaque stability and vessel-wall remodelling by growth factors and cell extracellular matrix interactions. Coron Artery Dis 1997; 8(3–4): 213–24. [PubMed: 9237032]
- 85.
- Amento EP, Ehsani N, Palmer H, Libby P. Cytokines and growth factors positively and negatively regulate interstitial collagen gene expression in human vascular smooth muscle cells. Arterioscler Thromb 1991; 11(5): 1223–30. [PubMed: 1911708]
- 86.
- Bentzon JF, Falk E. Circulating smooth muscle progenitor cells in atherosclerosis and plaque rupture: current perspective and methods of analysis. Vascul Pharmacol 2010; 52(1–2): 11–20. [PubMed: 19951745]
- 87.
- Clarke MCH, Littlewood TD, Figg N, Maguire JJ, Davenport AP, Goddard M, et al. Chronic apoptosis of vascular smooth muscle cells accelerates atherosclerosis and promotes calcification and medial degeneration. Circ Res 2008; 102(12): 1529–38. [PubMed: 18497329]
- 88.
- Geng YJ. Biologic effect and molecular regulation of vascular apoptosis in atherosclerosis. Curr Atheroscler Rep 2001; 3(3): 234–42. [PubMed: 11286645]
- 89.
- Geng YJ. Libby P. Evidence for apoptosis in advanced human atheroma. Colocalization with interleukin 1 beta converting enzyme. Am J Pathol 1995; 147(2): 251–66. [PMC free article: PMC1869820] [PubMed: 7639325]
- 90.
- Bauriedel G, Hutter R, Welsch U, Bach R, Sievert H, Lüderitz B. Role of smooth muscle cell death in advanced coronary primary lesions: implications for plaque instability. Cardiovasc Res 1999; 41(2): 480–8. [PubMed: 10341848]
- 91.
- Bennett MR, Evan GI, Schwartz SM. Apoptosis of human vascular smooth muscle cells derived from normal vessels and coronary atherosclerotic plaques. J Clin Invest 1995; 95(5): 2266–74. [PMC free article: PMC295839] [PubMed: 7738191]
- 92.
- Buja LM, Willerson JT. Role of inflammation in coronary plaque disruption. Circulation 1994; 89(1): 503–5. [PubMed: 8281687]
- 93.
- Libby P, Simon DI. Inflammation and thrombosis: the clot thickens. Circulation 2001; 103(13): 1718–20. [PubMed: 11282900]
- 94.
- Moreno PR, Falk E, Palacios IF, Newell JB, Fuster V, Fallon JT. Macrophage infiltration in acute coronary syndromes. Implications for plaque rupture. Circulation 1994; 90(2): 775–8. [PubMed: 8044947]
- 95.
- Shah PK, Falk E, Badimon JJ, Fernandez-Ortiz A, Mailhac A, Villareal-Levy G, et al. Human monocyte-derived macrophages induce collagen breakdown in fibrous caps of atherosclerotic plaques. Potential role of matrix-degrading metalloproteinases and implications for plaque rupture. Circulation 1995; 92(6): 1565–9. [PubMed: 7664441]
- 96.
- Loftus IM, Naylor AR, Bell PRF, Thompson MM. Matrix metalloproteinases and atherosclerotic plaque instability. Br J Surg 2002; 89(6): 680–94. [PubMed: 12027977]
- 97.
- Agren MS, Jorgensen LN, Andersen M, Viljanto J, Gottrup F. Matrix metalloproteinase 9 level predicts optimal collagen deposition during early wound repair in humans. Br J Surg 1998; 85(1): 68–71. [PubMed: 9462387]
- 98.
- Wysocki AB, Staiano-Coico L, Grinnell F. Wound fluid from chronic leg ulcers contains elevated levels of metalloproteinases MMP-2 and MMP-9. J Invest Dermatol 1993; 101(1): 64–8. [PubMed: 8392530]
- 99.
- Krane SM. Clinical importance of metalloproteinases and their inhibitors. Ann NY Acad Sci 1994; 732: 1–10. [PubMed: 7978783]
- 100.
- Page RC. The role of inflammatory mediators in the pathogenesis of periodontal disease. J Periodont Res 1991; 26(3 Pt 2): 230–42. [PubMed: 1679130]
- 101.
- Harris ED. Rheumatoid arthritis. Pathophysiology and implications for therapy. N Engl J Med 1990; 322(18): 1277–89. [PubMed: 2271017]
- 102.
- Parsons SL, Watson SA, Brown PD, Collins HM, Steele RJ. Matrix metalloproteinases. Br J Surg 1997; 84(2): 160–6. [PubMed: 9052425]
- 103.
- Dollery CM, McEwan JR, Henney AM. Matrix metalloproteinases and cardiovascular disease. Circ Res 1995; 77(5): 863–8. [PubMed: 7554139]
- 104.
- Mauviel A. Cytokine regulation of metalloproteinase gene expression. J Cell Biochem 1993; 53(4): 288–95. [PubMed: 8300745]
- 105.
- Rajavashisth TB, Xu XP, Jovinge S, Meisel S, Xu XO, Chai NN, et al. Membrane type 1 matrix metalloproteinase expression in human atherosclerotic plaques: evidence for activation by proinflammatory mediators. Circulation 1999; 99(24): 3103–9. [PubMed: 10377072]
- 106.
- Singer CF, Marbaix E, Lemoine P, Courtoy PJ, Eeckhout Y. Local cytokines induce differential expression of matrix metallo proteinases but not their tissue inhibitors in human endometrial fibroblasts. Eur J Biochem 1999; 259(1–2): 40–5. [PubMed: 9914473]
- 107.
- Chen H, Li D, Saldeen T, Mehta JL. TGF beta 1 attenuates myocardial ischemia-reperfusion injury via inhibition of upregulation of MMP 1. Am J Physiol Heart Circ Physiol 2003; 284(5): H1612–7. [PubMed: 12679326]
- 108.
- Gogly B, Hornebeck W, Groult N, Godeau G, Pellat B. Influence of heparin(s) on the interleukin-1-beta induced expression of collagenase, stromelysin-1, and tissue inhibitor of metalloproteinase-1 in human gingival fibroblasts. Biochem Pharmacol 1998; 56(11): 1447–54. [PubMed: 9827576]
- 109.
- Biswas C, Zhang Y, DeCastro R, Guo H, Nakamura T, Kataoka H, et al. The human tumor cell-derived collagenase stimulatory factor (renamed EMMPRIN) is a member of the immunoglobulin superfamily. Cancer Res 1995; 55(2): 434–9. [PubMed: 7812975]
- 110.
- Schmidt R, Bültmann A, Fischel S, Gillitzer A, Cullen P, Walch A, et al. Extracellular matrix metalloproteinase inducer (CD147) is a novel receptor on platelets, activates platelets, and augments nuclear factor kappaB-dependent inflammation in monocytes. Circ Res 2008; 102(3): 302–9. [PubMed: 18048771]
- 111.
- Ngase H. Activation mechanisms of matrix metalloproteinases. Biol Chem 1997; 378(3–4): 151–60. [PubMed: 9165065]
- 112.
- Lijnen HR. Plasmin and matrix metalloproteinases in vascular remodeling. Thromb Haemost 2001; 86(1): 324–33. [PubMed: 11487021]
- 113.
- Galis ZS, Kranzhöfer R, Fenton JW, Libby P. Thrombin promotes activation of matrix metalloproteinase-2 produced by cultured vascular smooth muscle cells. Arterioscler Thromb Vasc Biol 1997; 17(3): 483–9. [PubMed: 9102166]
- 114.
- Rajagopalan S, Meng XP, Ramasamy S, Harrison DG, Galis ZS. Reactive oxygen species produced by macrophage derived foam cells regulate the activity of vascular matrix metalloproteinases in vitro. Implications for atherosclerotic plaque stability. J Clin Invest 1996; 98(11): 2572–9. [PMC free article: PMC507715] [PubMed: 8958220]
- 115.
- Xu XP, Meisel SR, Ong JM, Kaul S, Cercek B, Rajavashisth TB, et al. Oxidized low density lipoprotein regulates matrix metalloproteinase-9 and its tissue inhibitor in human monocyte-derived macrophages. Circulation 1999; 99(8): 993–8. [PubMed: 10051290]
- 116.
- Gomez DE, Alonso DF, Yoshiji H, Thorgeirsson UP. Tissue inhibitors of metalloproteinases: structure, regulation and biological functions. Eur J Cell Biol 1997; 74(2): 111–22. [PubMed: 9352216]
- 117.
- Vine N, Powell JT. Metalloproteinases in degenerative aortic disease. Clin Sci 1991; 81(2): 233–9. [PubMed: 1653668]
- 118.
- Thompson RW, Holmes DR, Mertens RA, Liao S, Botney MD, Mecham RP, et al. Production and localization of 92 kilodalton gelatinase in abdominal aortic aneurysms. An elastolytic metalloproteinase expressed by aneurysm infiltrating macrophages. J Clin Invest 1995; 96(1): 318–26. [PMC free article: PMC185203] [PubMed: 7615801]
- 119.
- Li Z, Li L, Zielke HR, Cheng L, Xiao R, Crow MT, et al. Increased expression of 72-kd type IV collagenase (MMP-2) in human aortic atherosclerotic lesions. Am J Pathol 1996; 148(1): 121–8. [PMC free article: PMC1861591] [PubMed: 8546199]
- 120.
- Galis ZS, Sukhova GK, Lark MW, Libby P. Increased expression of matrix metalloproteinases and matrix degrading activity in vulnerable regions of human atherosclerotic plaques. J Clin Invest 1994; 94(6): 2493–503. [PMC free article: PMC330083] [PubMed: 7989608]
- 121.
- Brown DL, Hibbs MS, Kearney M, Loushin C, Isner JM. Identification of 92-kD gelatinase in human coronary atherosclerotic lesions. Association of active enzyme synthesis with unstable angina. Circulation 1995; 91(8): 2125–31. [PubMed: 7697840]
- 122.
- Aimes RT, Quigley JP. Matrix metalloproteinase-2 is an interstitial collagenase. Inhibitor-free enzyme catalyzes the cleavage of collagen fibrils and soluble native type I collagen generating the specific 3/4- and 1/4-length fragments. J Biol Chem 1995; 270(11): 5872–6. [PubMed: 7890717]
- 123.
- Sukhova GK, Schönbeck U, Rabkin E, Schoen FJ, Eoole AR, Billinghurst RC, et al. Evidence for increased collagenolysis by interstitial collagenases-1 and -3 in vulnerable human atheromatous plaques. Circulation 1999; 99(19): 2503–9. [PubMed: 10330380]
- 124.
- Elerman MP, Sukhova GK, Libby P, Gerdes N, Tang N, Horton DB, et al. Expression of neutrophil collagenase (matrix metalloproteinase-8) in human atheroma: a novel collagenolytic pathway suggested by transcriptional profiling. Circulation 2001; 104(16): 1899–904. [PubMed: 11602491]
- 125.
- Lee RT, Schoen FJ, Loree HM, Lark MW, Libby P. Circumferential stress and matrix metalloproteinase 1 in human coronary atherosclerosis. Implications for plaque rupture. Arterioscler Thromb Vasc Biol 1996; 16(8): 1070–3. [PubMed: 8696948]
- 126.
- Arroyo LH, Lee RT. Mechanisms of plaque rupture: mechanical and biologic interactions. Cardiovasc Res 1999; 41(2): 369–75. [PubMed: 10341836]
- 127.
- Price SJ, Greaves DR, Watkins H. Identification of novel, functional genetic variants in the human matrix metalloproteinase2 gene: role of Sp1 in allele-specific transcriptional regulation. J Biol Chem 2001; 276(10): 7549–58. [PubMed: 11114309]
- 128.
- Ye S, Watts GF, Mandalia S, Humphries SE, Henney AM. Preliminary report: genetic variation in the human stromelysin promoter is associated with progression of coronary atherosclerosis. Br Heart J 1995; 73(3): 209–15. [PMC free article: PMC483800] [PubMed: 7727178]
- 129.
- Terashima M, Akita H, Kanazawa K, Inoue N, Yamada S, Ito K, et al. Stromelysin promoter 5A /6A polymorphism is associated with acute myocardial infarction. Circulation 1999; 99(21): 2717–9. [PubMed: 10351963]
- 130.
- Zhang B, Henney A, Eriksson P, Hamsten A, Watkins H, Ye S. Genetic variation at the matrix metalloproteinase 9 locus on chromosome 20q12.2 13.1. Hum Genet 1999; 105(5): 418–23. [PubMed: 10598806]
- 131.
- Nemerson Y. Tissue factor and hemostasis. Blood 1988; 71(1): 1–8. [PubMed: 3275472]
- 132.
- Fernández-Ortiz A, Badimon JJ, Falk E, Fuster V, Meyer B, Mailhac A, et al. Characterization of the relative thrombogenicity of atherosclerotic plaque components: implications for consequences of plaque rupture. Journal of the American College of Cardiology 1994; 23(7): 1562–9. [PubMed: 8195515]
- 133.
- Moreno PR, Bernardi VH, López-Cuéllar J, Murcia AM, Palacios IF, Gold HK, et al. Macrophages, smooth muscle cells, and tissue factor in unstable angina. Implications for cell-mediated thrombogenicity in acute coronary syndromes. Circulation 1996; 94(12): 3090–7. [PubMed: 8989114]
- 134.
- Mach F, Schönbeck U, Libby P. CD40 signaling in vascular cells: a key role in atherosclerosis? Atherosclerosis 1998; 137 Suppl: S89–95. [PubMed: 9694547]
- 135.
- Aikawa M, Voglic SJ, Sugiyama S, Rabkin E, Taubman MB, Fallon JT, et al. Dietary lipid lowering reduces tissue factor expression in rabbit atheroma. Circulation 1999; 100(11): 1215–22. [PubMed: 10484543]
- 136.
- Bevilacqua MP, Schleef RR, Gimbrone MA, Loskutoff DJ. Regulation of the fibrinolytic system of cultured human vascular endothelium by interleukin 1. J Clin Invest 1986; 78(2): 587–91. [PMC free article: PMC423598] [PubMed: 3090105]
- 137.
- Giesen PL, Rauch U, Bohrmann B, Kling D, Roqué M, Fallon JT, et al. Blood-borne tissue factor: another view of thrombosis. Proc Natl Acad Sci USA 1999; 96(5): 2311–5. [PMC free article: PMC26780] [PubMed: 10051638]
- 138.
- Meisel SR, XU XP, Edgington TS, Dimayuga P, Kaul S, Lee S, et al. Differentiation of adherent human monocytes into macrophages markedly enhances tissue factor protein expression and procoagulant activity. Atherosclerosis 2002; 161(1): 35–43. [PubMed: 11882315]
- 139.
- Toschi V, Gallo R, Lettino M, Fallon JT, Gertz SD, Fernández-Ortiz A, et al. Tissue factor modulates the thrombogenicity of human atherosclerotic plaques. Circulation 1997; 95(3): 594–9. [PubMed: 9024145]
- 140.
- Matetzky S, Tani S, Kangavari S, Dimayuga P, Yano J, Xu H, et al. Smoking increases tissue factor expression in atherosclerotic plaques: implications for plaque thrombogenicity. Circulation 2000; 102(6): 602–4. [PubMed: 10931797]
- 141.
- Sambola A, Osende J, Hathcock J, Degen M, Nemerson Y, Fuster V, et al. Role of risk factors in the modulation of tissue factor activity and blood thrombogenicity. Circulation 2003; 107(7): 973–7. [PubMed: 12600909]
- 142.
- Barger AC, Beeuwkes R, Lainey LL, Silverman KJ. Hypothesis: vasa vasorum and neovascularization of human coronary arteries. A possible role in the pathophysiology of atherosclerosis. N Engl J Med 1984; 310(3): 175–7. [PubMed: 6197652]
- 143.
- Virmani R, Kolodgie FD, Burke AP, Finn AV, Gold HK, Tulenko TN, et al. Atherosclerotic plaque progression and vulnerability to rupture: angiogenesis as a source of intraplaque hemorrhage. Arterioscler Thromb Vasc Biol 2005; 25(10): 2054–61. [PubMed: 16037567]
- 144.
- de Boer OJ, van der Wal AC, Teeling P, Becker AE. Leucocyte recruitment in rupture prone regions of lipid-rich plaques: a prominent role for neovascularization? Cardiovasc Res 1999; 41(2): 443–9. [PubMed: 10341843]
- 145.
- Haas TL, Madri JA. Extracellular matrix driven matrix metalloproteinase production in endothelial cells: implications for angiogenesis. Trends Cardiovasc Med 1999; 9(3–4): 70–7. [PubMed: 10578520]
- 146.
- Kostoulas G, Lang A, Nagase H, Baici A. Stimulation of angiogenesis through cathepsin B inactivation of the tissue inhibitors of matrix metalloproteinases. FEBS Lett 1999; 455(3): 286–90. [PubMed: 10437790]
- 147.
- Muhlestein JB, Anderson JL, Hammond EH, Zhao L, Trehan S, Schwobe EP, et al. Infection with Chlamydia pneumoniae accelerates the development of atherosclerosis and treatment with azithromycin prevents it in a rabbit model. Circulation 1998; 97(7): 633–6. [PubMed: 9495296]
- 148.
- Saikku P, Leinonen M, Tenkanen L, Linnanmäki E, Ekman MR, Manninen V, et al. Chronic Chlamydia pneumoniae infection as a risk factor for coronary heart disease in the Helsinki Heart Study. Ann Intern Med 1992; 116(4): 273–8. [PubMed: 1733381]
- 149.
- Saikku P, Leinonen M, Mattila K, Ekman MR, Nieminen MS, Mäkelä PH, et al. Serological evidence of an association of a novel Chlamydia, TWAR, with chronic coronary heart disease and acute myocardial infarction. Lancet 1988; 2(8618): 983–6. [PubMed: 2902492]
- 150.
- Ossei-Gerning N, Moayyedi P, Smith S, Braunholtz D, Wilson JI, Axon AT, et al. Helicobacter pylori infection is related to atheroma in patients undergoing coronary angiography. Cardiovasc Res 1997; 35(1): 120–4. [PubMed: 9302355]
- 151.
- Nieto FJ, Adam E, Sorlie P, Farzadegan H, Melnick JL, Comstock GW, et al. Cohort study of cytomegalovirus infection as a risk factor for carotid intimal-medial thickening, a measure of subclinical atherosclerosis. Circulation 1996; 94(5): 922–7. [PubMed: 8790026]
- 152.
- Span AH, Grauls G, Bosman F, van Boven CP, Bruggeman CA. Cytomegalovirus infection induces vascular injury in the rat. Atherosclerosis 1992; 93(1–2): 41–52. [PubMed: 1317707]
- 153.
- Sorlie PD, Adam E, Melnick SL, Folsom A, Skelton T, Chambless LE, et al. Cytomegalovirus/herpesvirus and carotid atherosclerosis: the ARIC Study. J Med Virol 1994; 42(1): 33–7. [PubMed: 8308517]
- 154.
- Zhu J, Quyyumi AA, Norman JE, Costello R, Csako G, Epstein SE. The possible role of hepatitis A virus in the pathogenesis of atherosclerosis. J Infect Dis 2000; 182(6): 1583–7. [PubMed: 11069227]
- 155.
- Epstein SE, Zhu J, Burnett MS, Zhou YF, Vercellotti G, Hajjar D. Infection and atherosclerosis: potential roles of pathogen burden and molecular mimicry. Arterioscler Thromb Vasc Biol 2000; 20(6): 1417–20. [PubMed: 10845851]
- 156.
- Heinemann M, Susa M, Simnacher U, Marre R, Essig A. Growth of Chlamydia pneumoniae induces cytokine production and expression of CD14 in a human monocytic cell line. Infect Immun 1996; 64(11): 4872–5. [PMC free article: PMC174462] [PubMed: 8890256]
- 157.
- Kaukoranta-Tolvanen SS, Ronni T, Leinonen M, Saikku P, Laitinen K. Expression of adhesion molecules on endothelial cells stimulated by Chlamydia pneumoniae. Microb Pathog 1996; 21(5): 407–11. [PubMed: 8938646]
- 158.
- Kol A, Bourcier T, Lichtman AH, Libby P. Chlamydial and human heat shock protein 60s activate human vascular endothelium, smooth muscle cells, and macrophages. J Clin Invest 1999; 103(4): 571–7. [PMC free article: PMC408102] [PubMed: 10021466]
- 159.
- Kol A, Sukhova GK, Lichtman AH, Libby P. Chlamydial heat shock protein 60 localizes in human atheroma and regulates macrophage tumor necrosis factor-alpha and matrix metalloproteinase expression. Circulation 1998; 98(4): 300–7. [PubMed: 9711934]
- 160.
- Kalayoglu MV, Libby P, Byrne GI. Chlamydia pneumoniae as an emerging risk factor in cardiovascular disease. Jama 2002; 288(21): 2724–31. [PubMed: 12460096]
- 161.
- Weiss SM, Roblin PM, Gaydos CA, Cummings P, Patton DL, Schulhoff N, et al. Failure to detect Chlamydia pneumoniae in coronary atheromas of patients undergoing atherectomy. J Infect Dis 1996; 173(4): 957–62. [PubMed: 8603977]
- 162.
- Hahn DL, Golubjatnikov R. Smoking is a potential confounder of the Chlamydia pneumoniae-coronary artery disease association. Arterioscler Thromb 1992; 12(8): 945–7. [PubMed: 1637792]
- 163.
- Ridker PM, Kundsin RB, Stampfer MJ, Poulin S, Hennekens CH. Prospective study of Chlamydia pneumoniae IgG seropositivity and risks of future myocardial infarction. Circulation 1999; 99(9): 1161–4. [PubMed: 10069783]
- 164.
- Stone AFM, Mendall MA, KaskiJ-C, Edger TM, Risley P, Poloniecki J, et al. Effect of treatment for Chlamydia pneumoniae and Helicobacter pylori on markers of inflammation and cardiac events in patients with acute coronary syndromes: South Thames Trial of Antibiotics in Myocardial Infarction and Unstable Angina (STAMINA). Circulation 2002; 106(10): 1219–23. [PubMed: 12208796]
- 165.
- Epstein SE, Zhu J, Najafi AH, Burnett MS. Insights into the role of infection in atherogenesis and in plaque rupture. Circulation 2009; 119(24): 3133–41. [PubMed: 19546396]
- 166.
- Eliasziw M, Streifler JY, Fox AJ, Hachinski VC, Ferguson GG, Barnett HJ. Significance of plaque ulceration in symptomatic patients with high-grade carotid stenosis. North American Symptomatic Carotid Endarterectomy Trial. Stroke 1994; 25(2): 304–8. [PubMed: 8303736]
- 167.
- Rothwell PM, Salinas R, Ferrando LA, Slattery J, Warlow CP. Does the angiographic appearance of a carotid stenosis predict the risk of stroke independently of the degree of stenosis? Clin Radiol 1995; 50(12): 830–3. [PubMed: 8536392]
- 168.
- Grønholdt ML. Ultrasound and lipoproteins as predictors of lipid-rich, rupture-prone plaques in the carotid artery. Arterioscler Thromb Vase Biol 1999; 19(1): 2–13. [PubMed: 9888860]
- 169.
- Ehara S, Kobayashi Y, Yoshiyama M, Shimada K, Shimada Y, Fukuda D, et al. Spotty calcification typifies the culprit plaque in patients with acute myocardial infarction: an intravascular ultrasound study. Circulation 2004; 110(22): 3424–9. [PubMed: 15557374]
- 170.
- Nair A, Kuban BD, Tuzcu EM, Schoenhagen P, Nissen SE, Vince DG. Coronary plaque classification with intravascular ultrasound radiofrequency data analysis. Circulation 2002; 106(17): 2200–6. [PubMed: 12390948]
- 171.
- HongM-K, Mintz GS, Lee CW, LeeJ-W, ParkJ-H, ParkD-W, et al. A three-vessel virtual histology intravascular ultrasound analysis of frequency and distribution of thin-cap fibroatheromas in patients with acute coronary syndrome or stable angina pectoris. The American journal of cardiology 2008; 101(5): 568–72. [PubMed: 18308000]
- 172.
- JangI-K, Tearney GJ, MacNeill B, Takano M, Moselewski F, Iftima N, et al. In vivo characterization of coronary atherosclerotic plaque by use of optical coherence tomography. Circulation 2005; 111(12): 1551–5. [PMC free article: PMC2785437] [PubMed: 15781733]
- 173.
- Tearney GJ, Waxman S, Shishkov M, Vakoc BJ, Suter MJ, Freilich MI, et al. Three-dimensional coronary artery microscopy by intracoronary optical frequency domain imaging. JACC Cardiovasc Imaging 2008; 1(6): 752–61. [PMC free article: PMC2852244] [PubMed: 19356512]
- 174.
- Casscells W, Hathorn B, David M, Krabach T, Vaughn WK, McAllister HA, et al. Thermal detection of cellular infiltrates in living atherosclerotic plaques: possible implications for plaque rupture and thrombosis. Lancet 1996; 347(9013): 1447–51. [PubMed: 8676628]
- 175.
- Stefanadis C, Diamantopoulos L, Vlachopoulos C, Tsiamis E, Dernellis J, Toutouzas K, et al. Thermal heterogeneity within human atherosclerotic coronary arteries detected in vivo: A new method of detection by application of a special thermography catheter. Circulation 1999; 99(15): 1965–71. [PubMed: 10208999]
- 176.
- Stefanadis C, Toutouzas K, Tsiamis E, Stratos C, Vavuranakis M, Kallikazaros I, et al. Increased local temperature in human coronary atherosclerotic plaques: an independent predictor of clinical outcome in patients undergoing a percutaneous coronary intervention. Journal of the American College of Cardiology 2001; 37(5): 1277–83. [PubMed: 11300435]
- 177.
- Botnar RM, Stuber M, Kissinger KV, Kim WY, Spuentrup E, Manning WJ. Noninvasive coronary vessel wall and plaque imaging with magnetic resonance imaging. Circulation 2000; 102(21): 2582–7. [PubMed: 11085960]
- 178.
- Kim WY, Danias PG, Stuber M, Flamm SD, Plein S, Nagel E, et al. Coronary magnetic resonance angiography for the detection of coronary stenoses. N Engl J Med 2001; 345(26): 1863–9. [PubMed: 11756576]
- 179.
- Wasserman BA, Smith WI, Trout HH, Cannon RO, Balaban RS, Arai AE. Carotid artery atherosclerosis: in vivo morphologic characterization with gadolinium-enhanced double oblique MR imaging initial results. Radiology 2002; 223(2): 566–73. [PubMed: 11997569]
- 180.
- Aziz K, Berger K, Claycombe K, Huang R, Patel R, Abela GS. Noninvasive detection and localization of vulnerable plaque and arterial thrombosis with computed tomography angiography/positron emission tomography. Circulation 2008; 117(16): 2061–70. [PubMed: 18391115]
- 181.
- Ridker PM, Buring JE, Ahih J, Matias M, Hennekens CH. Prospective study of C-reactive protein and the risk of future cardiovascular events among apparently healthy women. Circulation 1998; 98(8): 731–3. [PubMed: 9727541]
- 182.
- Ridker PM, Cushman M, Stampfer MJ, Tracy RP, Hennekens CH. Inflammation, aspirin, and the risk of cardiovascular disease in apparently healthy men. N Engl J Med 1997; 336(14): 973–9. [PubMed: 9077376]
- 183.
- Kai H, Ikeda H, Yasukawa H, Kai M, Seki Y, Kuwahara F, et al. Peripheral blood levels of matrix metalloproteases -2 and -9 are elevated in patients with acute coronary syndromes. Journal of the American College of Cardiology 1998; 32(2): 368–72. [PubMed: 9708462]
- 184.
- Loftus IM, Naylor AR,Bell PR,Thompson MM. Plasma MMP-9 – a marker of carotid plaque instability. Eur J Vasc Endovasc Surg 2001; 21(1): 17–21. [PubMed: 11170872]
- 185.
- Blankenberg S, Rupprecht HJ, Poirier O, Bickel C, Smieja M, Hafner G, et al. Plasma concentrations and genetic variation of matrix metalloproteinase 9 and prognosis of patients with cardiovascular disease. Circulation 2003; 107(12): 1579–85. [PubMed: 12668489]
- 186.
- Haim M, Tanne D, Boyko V, Reshef T, Goldbourt U, Leor J, et al. Soluble intercellular adhesion molecule-1 and long-term risk of acute coronary events in patients with chronic coronary heart disease. Data from the Bezafibrate Infarction Prevention (BIP) Study. Journal of the American College of Cardiology 2002; 39(7): 1133–8. [PubMed: 11923036]
- 187.
- Koenig W, Khuseyinova N. Biomarkers of atherosclerotic plaque instability and rupture. Arterioscler Thromb Vase Biol 2007; 27(1): 15–26. [PubMed: 17082488]
- 188.
- Sever PS, Dahlöf B, Poulter NR, Wedel H, Beevers G, Caulfield M, et al. Prevention of coronary and stroke events with atorvastatin in hypertensive patients who have average or lower-than-average cholesterol concentrations, in the Anglo-Scandinavian Cardiac Outcomes Trial--Lipid Lowering Arm (ASCOT-LLA): a multicentre randomised controlled trial. Lancet 2003; 361(9364): 1149–58. [PubMed: 12686036]
- 189.
- Group HPSC. MRC/BHF Heart Protection Study of cholesterol lowering with simvastatin in 20,536 high-risk individuals: a randomised placebo-controlled trial. Lancer 2002; 360(9326): 7–22. [PubMed: 12114036]
- 190.
- Bellosta S, Via D, Canavesi M, Pfister P, Fumagalli R, Paoletti R, et al. HMG-CoA reductase inhibitors reduce MMP-9 secretion by macrophages. Arterioscler Thromb Vasc Biol 1998; 18(11): 1671–8. [PubMed: 9812903]
- 191.
- Scalia R, Gooszen ME, Jones SP, Hoffmeyer M, Rimmer DM, Trocha SD, et al. Simvastatin exerts both anti-inflammatory and cardio-protective effects in apolipoprotein E-deficient mice. Circulation 2001; 103(21): 2598–603. [PubMed: 11382730]
- 192.
- Endres M, Laufs U, Huang Z, Nakamura T, Huang P, Moskowitz MA, et al. Stroke protection by 3-hydroxy-3-methylglutaryl (HMG)-CoA reductase inhibitors mediated by endothelial nitric oxide synthase. Proc Natl Acad Sci USA 1998; 95(15): 8880–5. [PMC free article: PMC21171] [PubMed: 9671773]
- 193.
- Shovman O, Levy Y, Gilburd B, Shoenfeld Y. Anti-inflammatory and immunomodulatory properties of statins. Immunol Res 2002; 25(3): 271–85. [PubMed: 12018465]
- 194.
- Luan Z, Chase AJ, Newby AC. Statins inhibit secretion of metalloproteinases-1, -2, -3, and -9 from vascular smooth muscle cells and macrophages. Arterioscler Thromb Vasc Biol 2003; 23(5): 769–75. [PubMed: 12663370]
- 195.
- Crisby M, Nordin-Fredriksson G, Shah PK, Yano J, Zhu J, Nilsson J. Pravastatin treatment increases collagen content and decreases lipid content, inflammation, metalloproteinases, and cell death in human carotid plaques: implications for plaque stabilization. Circulation 2001; 103(7): 926–33. [PubMed: 11181465]
- 196.
- Ridker PM, Rifai N, Lowenthal SP. Rapid reduction in C-reactive protein with cerivastatin among 785 patients with primary hypercholesterolemia. Circulation 2001; 103(9): 1191–3. [PubMed: 11238259]
- 197.
- Aikawa M, Rabkin E, Sugiyama S, Voglic SJ, Fukumoto Y, Furukawa Y, et al. An HMG-CoA reductase inhibitor, cerivastatin, suppresses growth of macrophages expressing matrix metalloproteinases and tissue factor in vivo and in vitro. Circulation 2001; 103(2): 276–83. [PubMed: 11208689]
- 198.
- Bourcier T, Libby P. HMG CoA reductase inhibitors reduce plasminogen activator inhibitor-1 expression by human vascular smooth muscle and endothelial cells. Arterioscler Thromb Vasc Biol 2000; 20(2): 556–62. [PubMed: 10669656]
- 199.
- Libby P, Aikawa M. Effects of statins in reducing thrombotic risk and modulating plaque vulnerability. Clin Cardiol 2003; 26(1 Suppl 1): I11–4. [PMC free article: PMC6654646] [PubMed: 12539817]
- 200.
- Rouis M, Adamy C, Duverger N, Lesnik P, Horellou P, Moreau M, et al. Adenovirus-mediated overexpression of tissue inhibitor of metalloproteinase-1 reduces atherosclerotic lesions in apolipoprotein E-deficient mice. Circulation 1999; 100(5): 533–40. [PubMed: 10430768]
- 201.
- Hu Y, Baker AH, Zou Y, Newby AC, Xu Q. Local gene transfer of tissue inhibitor of metalloproteinase-2 influences vein graft remodeling in a mouse model. Arterioscler Thromb Vasc Biol 2001; 21(8): 1275–80. [PubMed: 11498453]
- 202.
- Schwartz MA, Venkataraman S, Ghaffari MA, Libby A, Mookhtiar KA, Mallya SK, et al. Inhibition of human collagenases by sulfur-based substrate analogs. Biochem Biophys Res Commun 1991; 176(1): 173–9. [PubMed: 1850255]
- 203.
- Watson SA, Morris TM, Parsons SL, Steele RJ, Brown PD. Therapeutic effect of the matrix metalloproteinase inhibitor, batimastat, in a human colorectal cancer ascites model. Br J Cancer 1996; 74(9): 1354–8. [PMC free article: PMC2074768] [PubMed: 8912529]
- 204.
- Bigatel DA, Elmore JR, Carey DJ, Cizmeci-Smith G, Franklin DP, Youkey JR. The matrix metalloproteinase inhibitor BB-94 limits expansion of experimental abdominal aortic aneurysms. J Vasc Surg 1999; 29(1): 130–8; discussion 38–9. [PubMed: 9882797]
- 205.
- Porter KE, Loftus IM, Peterson M, Bell PR, London NJ, Thompson MM. Marimastat inhibits neointimal thickening in a model of human vein graft stenosis. Br J Surg 1998; 85(10): 1373–7. [PubMed: 9782017]
- 206.
- Treharne GD, Boyle JR, Goodall S, Loftus IM, Bell PR, Thompson MM. Marimastat inhibits elastin degradation and matrix metalloproteinase 2 activity in a model of aneurysm disease. Br J Surg 1999; 86(8): 1053–8. [PubMed: 10460642]
- 207.
- Talbot DC, Brown PD. Experimental and clinical studies on the use of matrix metalloproteinase inhibitors for the treatment of cancer. Eur J Cancer 1996; 32A(14): 2528–33. [PubMed: 9059343]
- 208.
- Levitt NC, Eskens FA, O’Byrne KJ, Propper DJ, Denis LJ, Owen SJ, et al. Phase I and pharmacological study of the oral matrix metalloproteinase inhibitor, MMI270 (CGs27023a), in patients with advanced solid cancer. Clin Cancer Res 2001; 7(7): 1912–22. [PubMed: 11448904]
- 209.
- Johnson JL, Fritsche-Danielson R, Behrendt M, Westin-Eriksson A, Wennbo H, Herslof M, et al. Effect of broad-spectrum matrix metalloproteinase inhibition on atherosclerotic plaque stability. Cardiovasc Res 2006; 71(3): 586–95. [PubMed: 16759648]
- 210.
- Greenwald RA, Golub LM, Ramamurthy NS, Chowdhury M, Moak SA, Sorsa T. In vitro sensitivity of the three mammalian collagenases to tetracycline inhibition: relationship to bone and cartilage degradation. Bone 1998; 22(1): 33–8. [PubMed: 9437511]
- 211.
- Smith GN, Yu LP, Brandt KD, Capello WN. Oral administration of doxycycline reduces collagenase and gelatinase activities in extracts of human osteoarthritic cartilage. J Rheumatol 1998; 25(3): 532–5. [PubMed: 9517776]
- 212.
- Loftus IM, Porter K, Peterson M, Boyle J, London NJ, Bell PR, et al. MMP inhibition reduces intimal hyperplasia in a human vein graft stenosis model. Ann N Y Acad Sci 1999; 878: 547–50. [PubMed: 10415769]
- 213.
- Petrinec D, Liao S, Holmes DR, Reilly JM, Parks WC, Thompson RW. Doxycycline inhibition of aneurysmal degeneration in an elastase-induced rat model of abdominal aortic aneurysm: preservation of aortic elastin associated with suppressed production of 92 kD gelatinase. J Vasc Surg 1996; 23(2): 336–46. [PubMed: 8637112]
- 214.
- Thompson RW, Baxter BT. MMP inhibition in abdominal aortic aneurysms. Rationale for a prospective randomized clinical trial. Ann N Y Acad Sci 1999; 878: 159–78. [PubMed: 10415728]
- 215.
- Axisa B, Loftus IM, Naylor AR, Goodall S, Jones L, Bell PRF, et al. Prospective, randomized, double-blind trial investigating the effect of doxycycline on matrix metalloproteinase expression within atherosclerotic carotid plaques. Stroke 2002; 33(12): 2858–64. [PubMed: 12468782]
- 216.
- Baxter BT, Pearce WH, Waltke EA, Littooy FN, Hallett JW, Kent KC, et al. Prolonged administration of doxycycline in patients with small asymptomatic abdominal aortic aneurysms: report of a prospective (Phase II) multicenter study. J Vasc Surg 2002; 36(1): 1–12. [PubMed: 12096249]
- 217.
- Miyazaki M, Sakonjo H, Takai S. Anti-atherosclerotic effects of an angiotensin converting enzyme inhibitor and an angiotensin II antagonist in Cynomolgus monkeys fed a high-cholesterol diet. Br J Pharmacol 1999; 128(3): 523–9. [PMC free article: PMC1571675] [PubMed: 10516628]
- 218.
- Yusuf S, Sleight P, Pogue J, Bosch J, Davies R, Dagenais G. Effects of an angiotensin-converting-enzyme inhibitor, ramipril, on cardiovascular events in high-risk patients. The Heart Outcomes Prevention Evaluation Study Investigators. N Engl J Med 2000; 342(3): 145–53. [PubMed: 10639539]
- 219.
- Tummala PE, Chen XL, Sundell CL, Laursen JB, Hammes CP, Alexander RW, et al. Angiotensin II induces vascular cell adhesion molecule-1 expression in rat vasculature: A potential link between the renin-angiotensin system and atherosclerosis. Circulation 1999; 100(11): 1223–9. [PubMed: 10484544]
- 220.
- Navalkar S, Parthasarathy S, Santanam N, Khan BV. Irbesartan, an angiotensin type 1 receptor inhibitor, regulates markers of inflammation in patients with premature atherosclerosis. Journal of the American College of Cardiology 2001; 37(2): 440–4. [PubMed: 11216960]
- 221.
- Hans CP, Zerfaoui M, Naura AS, Catling A, Boulares AH. Differential effects of PARP inhibition on vascular cell survival and ACAT-1 expression favouring atherosclerotic plaque stability. Cardiovasc Res 2008; 78(3): 429–39. [PubMed: 18245064]
- 222.
- Marczewski MM, Postula M, Kosior D. Novel antiplatelet agents in the prevention of cardiovascular complications – focus on ticagrelor. Vasc Health Risk Manag 2010; 6: 419–29. [PMC free article: PMC2882894] [PubMed: 20539844]
- Mechanisms of Plaque Rupture - Mechanisms of Vascular DiseaseMechanisms of Plaque Rupture - Mechanisms of Vascular Disease
- Eubrachyura glyceraldehyde 3-phosphate dehydrogenase (GAPDH) gene, partial cds.Eubrachyura glyceraldehyde 3-phosphate dehydrogenase (GAPDH) gene, partial cds.PopSet: 2286223158PopSet
- RecName: Full=Forkhead box protein C1; AltName: Full=Forkhead-related protein FK...RecName: Full=Forkhead box protein C1; AltName: Full=Forkhead-related protein FKHL7; AltName: Full=Forkhead-related transcription factor 3; Short=FREAC-3gi|13638267|sp|Q12948.3|FOXC1_HUMANProtein
- Foxc1 forkhead box C1 [Mus musculus]Foxc1 forkhead box C1 [Mus musculus]Gene ID:17300Gene
Your browsing activity is empty.
Activity recording is turned off.
See more...