NCBI Bookshelf. A service of the National Library of Medicine, National Institutes of Health.
Toxicological Profile for White Phosphorus. Atlanta (GA): Agency for Toxic Substances and Disease Registry (US); 1997 Sep.
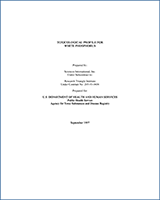
Toxicological Profile for White Phosphorus.
Show details5.1. OVERVIEW
White phosphorus can enter the environment from its production, use, accidental spills during loading and unloading for shipment, and accidental spills during transport. Hazardous wastes sites containing white phosphorus can also be a source of phosphorus in the environment. White phosphorus has been found in at least 77 of the 1,430 current or former EPA National Priorities List (NPL) hazardous waste sites (HazDat 1996). However, the number of sites evaluated for white phosphorus is not known. The frequency of these sites within the United States can be seen in Figure 5-1.
The persistence of elemental phosphorus in the air is very short due to oxidation to phosphorus oxides and ultimately to phosphorus acids. However, the particulate phosphorus aerosol may be coated with a protective oxide layer that may prevent further oxidation and extend the lifetime of particulate phosphorus in air. Both wet and dry deposition remove unreacted elemental phosphorus and the degradation products from the air. Similarly, elemental phosphorus oxidizes and hydrolyzes in water and in soil. A small amount of elemental phosphorus is lost from soil and water by volatilization.
Phosphorus is used as a fumigant in the storage of grain. Because of ease of application, pellets of aluminum or magnesium phosphide are commonly used (Garry et al. 1993). Phosphine, a highly toxic gas, is generated from phosphide. The rate of formation of phosphine (permissible exposure limit [PEL], 0.4 mg/m3) is dependent on the ambient temperature and humidity. Its release is rapid, and it is extremely fatal to the unprotected person (Garry et al. 1989). An accidental death of a pregnant woman was related to phosphine exposure from stored grain which had been fumigated with aluminum phosphide (AIP3) pellets (Garry et al. 1993). Phosphine is also generated when phosphorus is used as a dopant in the processing of microchips, where a small amount of phosphorus is added to another substancesuch as a semi-conductor to alter its properties (Garry et al. 1989). When phosphine is formed, it decomposes to several lesser known intermediates such as P2H4 a potential alkylating agent, finally forming H2PO4 (phosphoric acid), salts, and water. In the presence of oxygen, these breakdown products are formed rapidly from phosphine (Garry et al. 1993). A small fraction of phosphine may undergo oxidation with the formation of phosphates. The half-life of elemental phosphorus in water is 2-20 hours and 3-7 days in soil. However, the half-life of elemental phosphorus in the anoxic zones of water, sediment, and soil can exceed 2 years and may be ≤10,000 years.

FIGURE 5-1
FREQUENCY OF NPL SITES WITH WHITE PHOSPHOROUS CONTAMINATION .
The combustion of white phosphorus felt or red phosphorus butyl rubber will produce smoke. Smoke is an aerosol comprised of oxides of phosphorus (phosphorus pentoxide and phosphorus trioxide), some of their transformation products (see Section 3.2), and a small amount of unburnt phosphorus. The aerosol components in the smoke will undergo dispersion and chemical transformation in air to form acids or phosphorus, and will ultimately deposit from air to the hydrosphere and the lithosphere. The main components of the aerosol deposited over water and soil are acids of phosphorus. Under oxidizing conditions in soil and water, phosphorus acids will be transformed to phosphate or polyphosphates. Under reducing conditions, the disproportionation reaction of phosphorus acid can produce phosphine, and the gas may be released to the atmosphere. The fate of deposited unburnt phosphorus in water and soil has already been discussed in the preceding paragraph.
Elemental phosphorus has been detected in occupational air at levels ≥0.45 mg/m3. It has been detected in water, sediment, and aquatic animals in the vicinity of phosphorus production and user sites. Concentrations of ≤386 µg/kg were detected in freshwater drums collected from Yellow Lake in Pine Bluff, Arkansas. Elemental phosphorus was detected in dead waterfowl in the vicinity of contaminated water at a concentration of 3,501,000 µg/kg (3.5 g/kg). The fat of a dead bald eagle from Eagle River Plats, Alaska, contained 60 µg/kg elemental phosphorus. The typical intake of elemental phosphorus for the general population in the United States resulting from inhalation of air and ingestion of drinking water and food is not known. People who live near phosphorus production sites, user sites (e.g., Pine Bluff Arsenal), WP/F artillery training sites, and dumpsites that contain elemental phosphorus may be exposed to elemental phosphorus at higher levels than the control population. People who live near accidental spill sites may also be exposed to phosphorus at high doses during a spill incident. There is a lack of data providing evidence of these high exposures.
5.2. RELEASES TO THE ENVIRONMENT
5.2.1. Air
White phosphorus can enter air from its production, use, accidental spills during loading and unloading for shipment, and accidental spills during transport. During white phosphorus production, an estimated 0.58 kg total phosphorus/ton of product is emitted into the air (EPA 1989). Part of the air emissions during production of white phosphorus from phosphate rock is expected to be in the form of phosphate. The air emission of elemental phosphorus during production of munitions at the Pine Bluff Arsenal in Arkansas was estimated at 1 mg/kg of phosphorus used (Berkowitz et al. 1981). The amount of elemental phosphorus released to air during the manufacture of phosphoric acid, phosphates, and other phosphorus chemicals is not known because the air emission factor is estimated for total phosphorus (EPA 1989). However, the emission of elemental phosphorus from the manufacture of phosphorus chemicals may be low, because the conversion rate from elemental phosphorus to compounds is high. White phosphorus is also released in air during its use as an incendiary device by the military. It will enter the atmosphere during testing, which produces phosphorus smoke in the field. At a smoke density of 0.1 mg/m3, the estimated concentration of elemental phosphorus in the smoke was 21 ppb (EPA 1991). It has been suggested that elemental phosphorus is released into the air during manufacture and treatment of some semiconductors, such as gallium phosphide (Knizek 1978). Elemental phosphorus also enters air from accidental spills during loading and unloading at the dockside (Idler et al. 1981); but no estimate of the amount of emission from this source was located. Accidental spills during transport also emit white phosphorus to the atmosphere. In one such incident in 1986, the derailment and subsequent fire of a tanker car gradually released 12,000 gallons of white phosphorus, mostly as combustion products, in Miamisburg, Ohio (Scoville et al. 1989). According to the Toxics Release Inventory (TR193 1995), an estimated total of 30.0 thousand pounds of white phosphorus, amounting to 8.3% of the total environmental release, was discharged to the air from manufacturing and processing facilitiesin the United States in 1993 (see Table 5-1). The TRI data should be used with caution since only certain types of facilities are required to report. This is not an exhaustive list.
Table 5-1
Releases to the Environment from Facilities That Manufacture or Process White Phosphorus.
5.2.2. Water
The same sources that emit elemental phosphorus to air are also responsible for its emission to water. In its initial states of operation, the ERCO plant in Newfoundland, Canada, which produced white phosphorus, discharged 68-91 kg/day of colloidal white phosphorus into the Long Harbor inlet of Placentia Bay in Newfoundland. Elemental phosphorus was found in both the effluent water and the bottom sediment of Long Harbor (Davidson et al. 1987; EPA 1991). White phosphorus is also expected to be found in the effluents from user industries where it is converted into products such as phosphoric acid and phosphate, detergents, fireworks, insecticide, rat poisons, flotation agents, and red phosphorus (Idler et al. 1981). However, the concentration of elemental phosphorus in effluents from industries that produce phosphorus compounds is much lower, compared to industries that do not use chemical conversion processes for producing final products (e.g., WP/F production). White phosphorus also enters water when treated or untreated effluents are released from munitions production facilities that use white phosphorus. Before water recycling measures were implemented at the Pine Bluff Arsenal in Arkansas, the effluent water (phossy water) from the facility contained 153.4 mg/L of white phosphorus (Pearson et al. 1976). White phosphorus is usually handled in the molten state (55°C), submerged under water or as an inert gas. Accidental spills of white phosphorus during loading, unloading, or transport can release the element into water directly or by way of washout from terrestrial surfaces. The accident in Miamisburg, Ohio, is an example of such a release (Scoville et al. 1989). Small amounts of elemental phosphorus can be released into water in leachate from sludge heaps (produced during production of phosphorus) or runoff from hazardous waste sites containing elemental phosphorus (Berkowitz et al. 1981; Idler et al. 1981). Leaching from a phosphorus-containing landfill may also transport small amounts of elemental phosphorus to groundwater (Berkowitz et al. 1981). In addition, water deposition of atmospheric phosphorus may release small amounts of phosphorus into water (since most of the phosphorus in air will oxidize with the exception of phosphorus protected from oxidation by an oxide layer) (Berkowitz et al. 1981). According to TRI93 (1995), an estimated total of 5.0 thousand pounds of white phosphorus, amounting to 1.4% of the total environmental release, was discharged to water (including public sewers) from manufacturing and processing facilities in the United States in 1993.
5.2.3. Soil
Two important sources of elemental phosphorus in soil are the creation of slag piles during the production of white phosphorus, and the disposal of solid wastes containing elemental phosphorus in hazardous waste landfills (Berkowitz et al. 1981; Idler et al. 1981). The field use of WP/F and red phosphorus/butyl rubber smoke/obscurant releases elemental phosphorus into soil primarily as unburnt phosphorus in munitions (Berkowitz et al. 1981; Van Voris et al. 1987). During the deployment of ammunition, a significant amount of phosphorus may remain unoxidized in the form of unburnt elemental phosphorus (Spanggord et al. 1985). Elemental phosphorus is also released to soil from accidental spills during the loading, unloading, and transport of white phosphorus. Smaller amounts of particulate phosphorus present as an aerosol enter land as a result of wet and dry deposition of atmospheric phosphorus (Berkowitz et al. 1981). According to TR193 (1995), an estimated total of 328,000 pounds of white phosphorus, amounting to 90.3% of the total environmental release, was discharged to land from manufacturing and processing facilities in the United States in 1993. Additionally, a total of 196,000 pounds of white phosphorus waste (54.1% of the total environmental phosphorus release) from these facilities was transferred to other locations for disposal. Some of these wastes may have been disposed of in hazardous waste sites.
5.3. ENVIRONMENTAL FATE
5.3.1. Transport and Partitioning
The persistence of white phosphorus in air is very short and may range from minutes to days (see Section 5.3.2.1). Particulate white phosphorus present as an aerosol may be coated with a protective layer of oxide and may have a longer lifetime in air (Berkowitz et al. 1981). In addition to aerosol age, phosphorus aerosol speciation is also affected by the humidity of the ambient environment (Van Voris et al. 1987). Washout and rainout processes transport both the reaction products of vapor phase phosphorus and unreacted particles of phosphorus to water and land (Berkowitz et al. 1981). Because of its lower water solubility, physical state (gas), and slower reactivity, phosphine formed during the combustion of white phosphorus or released to the atmosphere from other media persists in the atmosphere longer than other reaction products.
Phosphorus enters water mostly as phosphates; smaller amounts enter as elemental phosphorus. Phosphates in an aquatic system partition between the aquatic phase (10%) and the sediment (90%) (Berkowitz et al. 1981). Elemental phosphorus that remains unoxidized in water can be partially deposited in the sediment after gravitational settling and also partially remains in the colloidal form in water. The rate of gravitational settling depends on the particle size of white phosphorus; larger phosphorus particles settle faster than smaller particles. Elemental phosphorus particles with diameters ranging from 0.015 to 3.0 mm were isolated from pond sediment samples of Eagle River Flats in Alaska (Bird 1991). The estimated soil sorption coefficient (Koc) value of 3.05 (see Table 3-2) indicates that both the water-soluble and colloidal forms of white phosphorus in the water phase may sorb moderately to particulate matter in water (Spanggord et al. 1985; Swann et al. 1983). The particle-sorbed phosphorus is eventually transported to the sediment. Volatilization from water to air is another mode of transport of white phosphorus. The Henry’s law constant (H) of 2.11x10-3 atm·m3/mol (Spanggord et al. 1985) indicates that volatilization of elemental phosphorus from water is significant (Thomas 1982). The estimated volatilization half-life of white phosphorus from water is 48.5 minutes from a stream 4 feet deep, with an eddy diffusivity of 1 cm2/second (Spanggord et al. 1985). Under turbulent conditions, the rate of volatilization is faster and may be an important fate-determining process for white phosphorus.
White phosphorus moderately bioconcentrates in aquatic organisms, but the bioconcentration factors are much lower than those for other toxic organic chemicals. The bioconcentration factors for white phosphorus in a few species of aquatic organisms are as follows: cod (Gadus morhua) whole body, 44, and muscle, 24; salmon (Salvo salur) muscle, 22 (Fletcher 1974); channel catfish (Ictalurus punctutus) muscle, 50-100; fathead minnow (Pimephdes promelas) muscle, 127 (Bentley et al. 1978); cod (G. morhua) muscle, l0-100 (Dyer et al. 1970); clams (Mya arenaria), 23; mussel (Mytilus edulis), 10; periwinkle (Littorinu littroreu), 42; starfish (Asterius vulgaris), 27; lobster (Homurus americanus) muscle, 23-34 (Fletcher 1971). It has been suggested that the bioconcentration factor (BCF) of elemental phosphorus in fish muscle may depend on the concentration in water. The BCF for cod muscle decreased with increasing water concentration, ranging from 34 at 4.4 ug/L to 10 at 29 µg/L (Maddock and Taylor 1976). Depuration was rapid once the contaminated fish were placed in clean water; no elemental phosphorus was observed from within hours to 7 days of exposure (Bentley et al. 1978; Fletcher 1971; Maddock and Taylor 1976).
The two processes involved in the transport of elemental phosphorus from soil are volatilization and leaching. When 35 mg of elemental phosphorus was added to two soils, one acid and the other calcareous, ≈0.004-0.6% of the applied phosphorus found at a depth of ≤ 10 cm volatilized as elemental phosphorus (not as oxides) in 3 days (Warnock 1972). The loss of a maximum amount of 0.6% phosphates was complete in 3-7 days. The amount of phosphorus volatilized appeared to be similar from both soils. The rate of volatilization decreased by increasing the depth to which the phosphorus was applied or by increasing soil moisture content; the rate did not go to zero. The transport of elemental phosphorus from soil by leaching depends on the Koc value. The estimated Koc value of 3.05 (see Table 3-2) indicates that phosphorus may moderately sorb in soil. Therefore, moderate leaching of phosphorus may occur from the anaerobic soil zone where elemental phosphorus will be stable toward chemical oxidation (EPA 1989; Richardson 1992).
5.3.2. Transformation and Degradation
5.3.2.1. Air
WP/F reacted rapidly in air with an estimated half-life of ≈5 minutes (Spanggord et al. 1985). However, the deployment of the military smoke/obscurant in the field may produce an estimated 10% of unburnt phosphorus (Spanggord et al. 1985). Other sources will also emit elemental phosphorus (see Section 5.2.1). Particle size from phosphorus obscurant aerosols is determined by the age of the particles and the amount of water vapor available in the atmosphere. In wind tunnel studies particles increased in diameter by 1.3 times and increased in volume by 2.0 times at a relative humidity of 60%. Similar increases were observed with humidity levels higher than 60%. These increases are due to absorption of water vapor and to a lesser extent by coagulation of the particles. Therefore, in general particles that are smaller have been released more recently than larger particles (Van Voris et al. 1987). In air, elemental phosphorus in the vapor phase reacts rapidly with atmospheric oxygen to produce oxides of phosphorus (Spanggord et al. 1985). Since oxides of phosphorus are highly soluble in water, wet deposition removes them from air (Berkowitz et al. 1981). Particulate elemental phosphorus also reacts at most environmental pressures and at temperatures >5ºC (EPA 1989). The amount of phosphorus remaining in the particles has been observed to decrease with increasing relative humidity from a phosphorus percentage of 25% at low humidities to 15% for humidities near 90% (Van Voris et al. 1987). However, if the particulate phosphorus is coated with a protective layer of oxide, further oxidation may not occur (Berkowitz et al. 1981). This may increase the lifetime of elemental phosphorus in the air. Both wet and dry deposition remove the oxide-coated elemental phosphorus from the atmosphere with the wet deposition rate increasing with increasing relative humidity.
Hydrolysis of elemental phosphorus in the atmosphere can produce phosphine. Phosphine in the presence of oxygen is highly reactive and is rapidly oxidized to, among other things, phosphoric acid. The production of phosphine is inversely related to the oxygen concentration, and is thus favored by low oxygen pressures (Spanggord et al. 1985). Hence, the probability of phosphine formation in the atmosphere is low because the concentration of oxygen is not conducive to it. Wind tunnel studies showed levels of phosphine generated during a white phosphorus aerosol study at levels approaching the detection limit. The highest phosphine concentration observed was 70 µg/m3, representing 0.02% of the total phosphorus in the aerosol. The higher concentrations were more prevalent at higher relative humidities (Van Voris et al. 1987). Such a reaction is more likely in water and soil (EPA 1991).
nOf the several products formed during the use of WP/F obscurant/smoke, phosphine is especially important because of its toxicity (EPA 1991). Therefore, the environmental fate of phosphine is briefly discussed. The important process for the loss of phosphine in the atmosphere is most likely its reaction with hydroxyl radicals. Based on measured rates under simulated conditions, the estimated lifetime of phosphine in the troposphere due to reaction with hydroxyl radicals is <1 day (Fritz et al. 1982). The hydrogen abstraction reaction may produce [PH2], which may react with ozone to produce H2PO. PH2•] is a free radical and is highly reactive, with or without ozone. Ultraviolet (UV) light can induce PH3 (phosphine) to form [PH2•]. H2PO may produce hypophosphorus acid (H2PO2) as a result of a reaction with nitrogen dioxide in air and subsequent hydrolysis. The hypophosphorus acid is ultimately oxidized to phosphorus and phosphoric acid.
5.3.2.2. Water
White phosphorus can exist in water as dissolved phosphorus in amounts ≤3 mg/L, in the colloidal state, as large particles of elemental phosphorus at concentrations >3 mg/L, or in the particle-sorbed state (Bullock and Newlands 1969; EPA 1991). Elemental phosphorus can undergo oxidation and hydrolysis in water. The rate of reactions depends on the dissolved oxygen concentration, temperature, state. of phosphorus in water (dissolved, sorbed, colloidal, or particle form), and possibly the pH of the solution. The rate of reaction grows faster as the temperature of the water increases (Lai and Rosenblatt 1977a). At concentrations well below the solubility limit (3 mg/L), elemental phosphorus disappeared from water by a first-order process with a half-life of 2 hours at ≈10°C and 0.85 hours at 30°C (EPA 1991; Zitko et al. 1970). The rate of phosphorus disappearance in water increased with the oxygen (or air) concentration and the pH of water (Lai and Rosenblatt 1977a). However, the faster initial disappearance half-life of 3.5 hours (compared to distilled water at pH 4.2) observed in river water at 22°C and a pH of 7.6 may have been due to the catalytic effects of ions present in the river water, rather than the pH effect (Lai and Rosenblatt 1977a).
The disappearance half-life of elemental phosphorus in water also depends on the physical state of phosphorus. For example, the disappearance half-life of collodial phosphorus was 80 hours at 30°C and 240 hours at 0°C at concentrations between 10-50 mg/L (Bullock and Newlands 1969), compared to a half-life of 2 hours in solution form at 10°C (Zitko et al. 1970). The half-life of white phosphorus in solution increased from 2 to 20 hours when the phosphorus was present in the sorbed state in sediment (Zitko et al. 1970). In anoxic water, the estimated half-life of a solid chunk of white phosphorus that was protectively coated due to oxidation/hydrolysis at the oxic zone was 2.43 years (Spanggord et al. 1985).
The experiments discussed above determined the rate of disappearance and the half-life of elemental phosphorus in water in open systems. The phosphorus in these experiments disappeared due to hydrolysis/oxidation and evaporation. Spanggord et al. (1985) studied the loss of elemental phosphorus in sealed reaction flasks. In a closed reaction flask with argon-saturated water, the loss of white phosphorus can only be due to hydrolysis. The estimated half-life for hydrolysis at ambient temperatures was 84 hours (Spanggord et al. 1985). The estimated half-lives of white phosphorus at ambient temperatures due to a combination of hydrolysis and oxidation reaction were 42 hours in air-saturated water and 56 hours in nonair-saturated water (Spanggord et al. 1985). Phosphine forms both in the presence and absence of air. However, since phosphine is a gas with a low water solubility (see Table 3-3), it either oxidizes or volatizes rapidly from water (Lai and Rosenblatt 1977a). At a white phosphorus concentration of 0.205 mg/L, approximately 6-9% of initial phosphorus was released as phosphine within 2 days (Lai and Rosenblatt 1977a). Kinetic studies concluded that the rate of phosphine production is inversely proportional to the oxygen concentration, and thus is favored by low oxygen pressure (Spanggord et al. 1985). The products of hydrolysis of white phosphorus are phosphine, hypophosphorus acid, phosphorus acid, and phosphoric acid. The oxidation products are oxides of phosphorus that produce hypophosphorus acid, phosphorus acid, and phosphoric acids in the presence of water (Spanggord et al. 1985).
White phosphorus reacts rapidly with chlorine to form phosphorus trichloride, which finally hydrolyzes and oxidizes to form phosphoric acids (EPA 1991). Chlorination of water, therefore, further shortens the half-life of phosphorus in water.
No data on the biodegradation of white phosphorus in water under aerobic conditions were located. Considering the rapidity with which phosphorus disappears from aerated water as a result of a combination of evaporative and chemical processes, it is unlikely that aerobic biodegradation can compete with these loss processes. Anaerobic biodegradation studies concluded that biotransformation of elemental phosphorus is not rapid in water (Spanggord et al. 1985).
In most natural water, phosphine is very unstable and oxidizes even under anoxic conditions. Depending upon the redox potential of water, the oxidation products are diphosphine (P2H4), phosphorus, hypophosphorus acid, phosphorus acid, and phosphoric acid (Kumar et al. 1985). Based on soil studies (Berck and Gunther 1970; Hilton and Robison 1972), small amounts of phosphine may also be adsorbed (reversible sorption) or chemisorbed (irreversible sorption) to suspended solid and sediments in water. However, based on the estimated Henry’s law constant (H) of 0.09 atm·m3/mol (see Table 3-3) and the expected volatility associated with various ranges of H, volatilization is expected to be the most important loss process for phosphine in water.
5.3.2.3. Sediment and Soil
The exposure of soil to phosphorus aerosols will upset the pH of the soil and create a more acidic layer of soil. This decrease in pH often can exceed the buffering capacity of the surface layer of the soil depending upon the amount of applied phosphorus. This process can be mitigated by a larger soil volume area so that phosphorus speciation on a field scale will be minimal. The interaction of metals with phosphorus condensates could lead to their leachability and possible trace metal migration from the soil (Van Vorris et al. 1987).
White phosphorus exposure to plants results in a variety of deleterious effects which are based upon the species of plant, the smoke concentration, the duration of exposure, the relative humidity, and the wind speed. These effects can include leaf tip burn, leaf curl, leaf abscission and drop, floral abortion, chlorosis, neucrotic spotting, wilting, dessication, and dieback. Other factors influencing the effects of white phosphorus upon plants are whether or not there is a post-exposure rainfall and whether the exposure is a large acute dose or several lower chronic doses (Van Voris et al. 1987).
In soils, oxidation is the predominant route of loss of white phosphorus up to a depth that allows diffusion of oxygen (Bohn et al. 1970). The oxidation of phosphorus to its oxides is usually very rapid. White phosphorus oxidized within 2 days in soil at ambient temperatures (Rodriguez et al. 1972). A soil volatilization study found that the volatilization of elemental phosphorus stopped after 3-7 days (Warnock 1972). If the termination is attributed to oxide formation, it can be concluded that elemental phosphorus oxidized in soil within 3-7 days.
However, the rate of oxidation will be slower if white phosphorus forms a protective coating (Bohn et al. 1970). The results of anaerobic biodegradation studies with soil and sediments are inconclusive (Spanggord et al. 1985). Although white phosphorus transformed faster in some nonsterile than in sterile controls, the slower transformation in sterile soil could be attributed to a change in soil property due to autoclaving (Spanggord et al. 1985); the investigators found that the biotransformation microorganisms were difficult to grow under their test conditions. The lack of growth is an indication of lack of biodegradation. Therefore, in the absence of oxidation and biodegradation reactions in anaerobic zones of soil and sediment, the half-life of elemental phosphorus could be l0-10,000 years (Richardson 1992; Spanggord et al. 1985). It should be mentioned that although microorganisms that biodegrade elemental phosphorus under anaerobic conditions could not be grown, both linear polyphosphates and cyclic metaphosphates could be microbially hydrolyzed to simpler phosphates in water and soil under aerobic and anaerobic conditions (Spanggord et al. 1985).
In sealed tubes, phosphine completely disappeared in less than 40 days from three different types of soil with varying amounts of moisture (Hilton and Robison 1972). The disappearance was attributed to initial sorption, and the subsequent biotic and abiotic oxidation of part of the sorbed compound. The rate of adsorption increased with decreasing moisture content and increasing organic soil content (Hilton and Robison 1972). The study showed that phosphine sorption in soil can occur by both physical and chemical sorption processes, and that the chemisorption process is higher in soils with a low organic matter and high mineral content (Berck and Gunther 1970). Chemisorption irreversibly binds phosphine in soil so that it is not available for volatilization. However, since phosphine is gaseous and is only slightly soluble in water, volatilization from soil may be the most important process by whiclrphosphine is lost from soil when chemisorption is not occurring.
5.4. LEVELS MONITORED OR ESTIMATED IN THE ENVIRONMENT
Phosphorus exists mostly in the phosphate form in the general environment. The levels of phosphorus determined in most environmental samples are reported as total phosphorus and do not distinguish between elemental phosphorus and its compounds. However, elemental phosphorus is far more toxic than other oxidized phosphorus states (oxides and acids of phosphorus). This profile reports the level of elemental phosphorus and not total phosphorus in environmental samples.
5.4.1. Air
The emission of elemental phosphorus into the air from the stacks of a munitions manufacturing plant could cause an estimated exposure level of up to 0.5 mg/m3/hour (worst-case scenario). A more likely exposure level is 0.5µg/m3/hour (Berkowitz et al. 1981). As the elemental phosphorus undergoes dispersion and reaction in air, its level from the stack emission would continue to decrease with increased distance. During field use of a single 155 mm WP/P shell over an area of 100 m2, the estimated maximum ambient white phosphorus and phosphine concentrations were estimated to be 7 µg/m3 and 7 µg/m3, respectively (Berkowitz et al. 1981). If 72 shells are used over the same area for a continuous screen, the maximum ambient white phosphorus and phosphine concentrations would be 0.12 mg/m3 and 0.12 µg/rn3 (Berkowitz et al. 1981). During deployment of WP/F bursting rockets and howitzers where the smoke covered a minimum ground area of 9,500-12,000 m2, the estimated environmental concentration of smoke would be 5-25 mg/m3 (Shinn et al. 1985). On the other hand, the deployment of white phosphorus-based mortars, guns, rockets, and howitzers covering a minimum smoke area of 100-800 m2, may produce an environmental concentration of 1,800-3,500 mg/m3 smoke (Shinn et al. 1985). The concentration of white phosphorus in air from the smoke would be only a small fraction of the smoke concentration.
5.4.2. Water
The concentration of elemental phosphorus in the overflow water of a settling pond of a white phosphorus filling plant in Arkansas was ≤ 84 mg/L (Pearson et al. 1976). The average concentration in the discharge was 10.6 mg/L (Pearson et al. 1976). The mean concentration of elemental phosphorus at the Yellow Lake spillway from this discharge varied from 0.14 to 2.46 mg/L at different periods during 1971-1974. The concentration of elemental phosphorus measured in 1975 at different locations in Yellow Lake ranged from 0.02 to 40.4 µg/L (Pearson et al. 1976). After recycling measures for the phossy water were implemented, the concentration of elemental phosphorus in water from Long Harbour, Placentia Bay, Newfoundland, rarely exceeded 3.0 µg/L (Addison et al. 1972a), the concentration in Yellow Lake ranged from 0.005 to 0.01 µg/L, and the level in the Arkansas River, which is connected to the lake, ranged from 0.003 to 0.004 µg/L (EPA 1989). Similarly, phossy water discharged into adjacent water from the white phosphorus manufacturing plant in Long Harbour contained elemental phosphorus, as indicated by levels of elemental phosphorus in the sediment (see Section 5.4.3).
5.4.3. Sediment and Soil
Before the waste-water treatment facility was instituted, the concentrations of elemental phosphorus in the sediment in the vicinity of the effluent discharge pipe of the phosphorus manufacturing plant in Long Harbour, Placentia Bay, Newfoundland, ranged from 5.5 to 2,910 mg/kg (Idler 1972). After the treatment facility was installed, the overflow of a small settling pond during heavy rain continued to contaminate the adjacent waterways. The concentration of elemental phosphorus in the sediments of Yellow Lake at different locations ranged from 0.02 to 43.4 µg/kg (wet weight) (Pearson et al. 1976). For the last 10 years, the U.S. Army has used Eagle River Flats, a 1,000 hectare estuarine salt marsh near Anchorage, Alaska, for artillery training. Elemental phosphorus was detected at a concentration range of 0.0025-10.2 mg/kg in 30-47% of the sediment samples collected from the salt marsh in the fall of 1990 (Bird 1991; Racine et al. 1992b; Richardson 1992). Sediment samples from Eagle River Flats were again collected during May and August of 1991 and analyzed for elemental phosphorus (Racine et al. 1992a). Depending upon the sampling location and timing of sample collection (before and after an explosion test), elemental phosphorus was detected in 0-100% of sediment samples; the concentration ranged from undetectable (detection limit <0.0001 mg/kg) to 198 mg/kg. Samples of sediments collected before and after explosion tests indicated that the level of elemental phosphorus increased as a result of artillery training. The concentration of elemental phosphorus in soil from a phosphorus dump site at Pine Bluff Arsenal was 5,200 µg/kg (Spanggord et al. 1985). No other data on the level of elemental phosphorus in soils from artillery training sites, phosphorus manufacturing, or disposal sites or arsenal sites where the munitions are loaded into artillery were located.
5.4.4. Other Environmental Media
Elemental phosphorus has been detected in fish and birds from areas in the vicinity of elemental phosphorus production sites (ERCO of Newfoundland), an arsenal production facility (Pine Bluff Arsenal in Arkansas), and artillery training sites (Eagle River Flats, Anchorage, Alaska). The concentrations of elemental phosphorus in fish and birds collected from these sites are given in Table 5-2. The concentrations of elemental phosphorus in the gizzard content of a dead waterfowl and a dead mallard collected outside of the Eagle River Flats in 1991 were 3,501,000 and 103,000 µg/kg, respectively (Racine et al. 1992a). However, the levels of elemental phosphorus in different tissues of live waterfowl collected from the Eagle River Flats in 1991 ranged from undetectable to 2,700 µg/kg (Racine et al. 1992a). Elemental phosphorus at levels similar to those found in dead waterfowl at Eagle River Flats (5 µg/g) were introduced into the diets of American kestrels (Falco sparverius) resulting in accumulation of P4 in the fat deposits and skin at 24 hours postdosing (Nam et al. 1994).
TABLE 5-2
Concentration of Elemental Phosphorus in Fish and Birds.
5.5. GENERAL POPULATION AND OCCUPATIONAL EXPOSURE
No data were located indicating that detectable levels of elemental phosphorus are present in the ambient air, drinking water, or food consumed by the general population not living near sources of elemental phosphorus emission. Therefore, the general population exposure to elemental phosphorus is very low and likely to be below measurable levels. A segment of the general population that lives near sources of emissions (e.g., production sites, user sites, and disposal sites) may be exposed to elemental phosphorus at high levels. This segment of the population is discussed in Section 5.6.
The concentrations of white phosphorus levels in the atmosphere of a wet fill production iine at Pine Bluff Arsenal, Arkansas, were measured in 1975 (Berkowitz et al. 1981). The levels of white phosphorus at this location exceeded the OSHA 8-hour time-weighted average exposure limit of 0.1 mg/m3. At one location, the concentration of elemental phosphorus was 0.45 mg/m3 and may have been underestimated due to poor quality control (Berkowitz et al. 1981). Values well below the OSHA limit (0.7-1.2 µg/m3) were detected during a 1977 survey at a dry fill line of the same plant (Berkowitz et al. 1981). However, the samples for these measurements were collected when the plant was not running at full capacity. Military personnel who use phosphorus-containing ammunitions may be exposed to white phosphorus smoke during warfare, training exercises, and accidents. Vapor samples were taken to measure worker exposure to H3PO4 PH3 and P4 during an Albright & Wilson acid plant decommissioning (June 9-10, 1992) (Albright and Wilson 1992a). The workers were digging out phosphorus residue from beneath storage and metering tanks. According to the decommissioning report, “Three out of three P4 vapor samples exceeded the OSHA permissible exposure limit (PEL) of 0.1 mg/m3 at 0.35, 0.12, and 1.11 mg/m3. Samples for exposure to H3PO4 were below the OSHA PEL of 1.0 mg/m3. Sensitivity for the PH, samples was reduced due to analytical problems. The levels of PH4 were all reported to be below 0.5 ppm. This compares to an OSHA PEL of 0.3 ppm for PH3 exposure. Workers were not using supplied air during their exposure (Albright & Wilson 1992a). During a phosphorus storage tank decontamination from July 13-15, 1992, at an Albright & Wilson plant in Cincinnati, one tank P4 vapor sample out of seven exceeded the OSHA PEL of 0.1 mg/m3. The measured value of the sample was 0.11 mg/m3 and occurred while a worker was using supplied air. Exposures to H3PO4 PH3 and AsH3 were all below the OSHA PELs during the tank decontamination (Albright & Wilson 1992b).
According to the National Occupational Exposure Survey (NOES) conducted by NIOSH, an estimated 208,990 workers were exposed to white phosphorus in the workplace. The NOES database does not contain any information about the concentration levels to which these workers may have been exposed or the frequency and duration of the exposure (NOES 1991).
5.6. POPULATIONS WITH POTENTIALLY HIGH EXPOSURES
The general population that lives near elemental phosphorus production sites, white phosphorus user sites (e.g., Pine Bluff Arsenal in Arkansas), artillery training sites (e.g., Eagle River Flats, Anchorage, Alaska), and dumpsites that contain elemental phosphorus may be exposed to elemental phosphorus at levels higher than the control population. The exposure could occur from inhalation of contaminated air, consumption of contaminated fish or game birds, and skin exposure from bathing in contaminated water. Additionally, children living near phosphorus-containing hazardous waste sites may be exposed to elemental phosphorus by dirt ingestion and/or skin contact while playing at unrestricted dumpsites. People who live near accidental spill sites (e.g., spill in Miamisburg, Ohio) are a likely population with potentially high exposures. However, reports providing evidence of these exposures were not located. As discussed in Section 5.5, people who work in user sites and possibly production sites, waste disposal sites, and military personnel using phosphorus-containing ammunitions are likely populations with potentially high exposures.
5.7. ADEQUACY OF THE DATABASE
Section 104(I)(5) of CERCLA, as amended, directs the Administrator of ATSDR (in consultation with the Administrator of EPA and agencies and programs of the Public Health Service) to assess whether adequate information on the health effects of white phosphorus is available. Where adequate information is not available, ATSDR, in conjunction with the NTP, is required to assure the initiation of a program of research designed to determine the health effects (and techniques for developing methods to determine such health effects) of white phosphorus.
The following categories of possible data needs have been identified by a joint team of scientists from ATSDR, NTP, and EPA. They are defined as substance-specific informational needs that if met would reduce the uncertainties of human health assessment. This definition should not be interpreted to mean that all data needs discussed in this section must be filled. In the future, the identified data needs will be evaluated and prioritized, and a substance-specific research agenda will be proposed.
5.7.1. Identification of Data Needs
Physical and Chemical Properties. Data such a log Kow log Koc and the Henry’s law constant needed for estimating the environmental transport of elemental phosphorus are available (see Table 3-2). However, an experimentally determined and reliable log Koc value for elemental phosphorus would be helpful.
Production, Import/Export, Use, Release, and Disposal. Although the current production capacity of elemental phosphorus in the United States is available (SRI 1992), the amount of elemental phosphorus actually produced is not known. The future trend for the demand of elemental phosphorus is expected to decrease by 1-2% in this decade (CMR 1991). Although some fluctuation has been observed, the export of elemental phosphorus remained constant at 5% of production during the past couple of decades (CMR 1991,1985,1981,1978). The import of elemental phosphorus is expected to be low because the production capacity in the United States is higher than the demand (CMR 1991); but recent import dam were not located in the literature.
The uses of elemental phosphorus in the United States are known (CMR 1991; EPA 1991; Van Wazer 1982). There is no evidence that elemental phosphorus is used in any consumer products other than rat poisons. Fish and game birds collected from the vicinity of production and user locations may contain elemental phosphorus (Addison et al. 1972b; Pearson et al. 1976; Racine et al. 1992a, 1992b). There is evidence that significant exposures could occur in workplaces where elemental phosphorus is handled (Berkowitz et al. 1981). Monitoring data indicate that significant quantities of elemental phosphorus can be found in sediment and in certain game birds near use sites (Pearson et al. 1976; Racine et al. 1992a, 1992b; Spanggord et al. 1985). Although some of the disposal methods used for elemental phosphorus are known (Berkowitz et al. 1981; HSDB 1993; Uhrmacher et al. 1985), the efficiency of these methods for the destruction of elemental phosphorus is not known. Information about the amounts of elemental phosphorus disposed of by each method is not known. EPA does regulate the disposal of elemental phosphorus-containing wastes in water and soil (EPA 1992a, 1992b).
According to the Emergency Planning and Community Right-to-Know Act of 1986,42 U.S.C. Section 11023, industries are required to submit substance release and off-site transfer information to the EPA. The Toxics Release Inventory (TRI), which contains this information for 1993, became available in May of 1995. This database will be updated yearly and should provide a list of industrial production facilities and emissions.
Environmental Fate. Elemental phosphorus partitions from water to sediment (Berkowitz et al. 1981) transporting elemental phosphorus to sediment. Volatilization from water and soil transports small amounts of elemental phosphorus to air (Spanggord et al. 1985; Wamock 1972). Elemental phosphorus quickly oxidizes and hydrolyzes in air and in aerobic zones of water and soil to produce mainly oxides and acids of phosphorus, except when covered by a protective coating of phosphorus oxides (Bohn et al. 1970; Bullock and Newlands 1969; EPA 1991; Lai and Rosenblatt 1977a; Rodriguez et al. 1972; Spanggord et al. 1985; Zitko et al. 1970). However, elemental phosphorus reaching the anaerobic zones of sediment and soil may persist for periods of l0-10,000 years (Richardson 1992; Spanggord et al. 1985). Therefore, anaerobic zones of soil and sediment may act as a sink for elemental phosphorus.
Bioavailability from Environmental Media. The bioavailability of elemental phosphorus following inhalation, oral, and dermal contact is poorly understood (see Section 2.3). The estimated log Koc for elemental phosphorus is 3.05 (See Table 3-2). Therefore, elemental phosphorus is moderately sorbed to aerosol particles in air, to sediment in water, and to soil. However, due to its high reactivity, elemental phosphorus may not be found in aerobic zones of soil and water, unless the element is protected from oxidation by unreactive oxide coating (Berkowitz et al. 1981). Its bioavailability in the sorbed state from inhaled air, ingested soil, and dermal contact with soil and water may be lower than the free form of the element under identical conditions.
Food Chain Bioaccumulation. Elemental phosphorus moderately bioconcentrates in aquatic organisms (Bentley et al. 1978; Fletcher 1971; Maddock and Taylor 1976). The biomagnification potential for elemental phosphorus in predators resulting from consumption of contaminated prey organisms has not been studied systematically. However, high concentrations of elemental phosphorus have been found in tissues of certain kinds of bottom-feeding waterfowls (Bird 1991; Racine et al. 1992a, 1992b). Elemental phosphorus has also been found in a dead bald eagle collected in the vicinity of Eagle River Flats (Bird 1991).
Exposure Levels in Environmental Media. With the exception of occupational air, monitoring data on the concentrations of elemental phosphorus in nonoccupational air, drinking water, and total diet were not located. The estimated value for the total human intake of elemental phosphorus from various environmental media is not available.
Reliable monitoring data for the levels of white phosphorus in contaminated media at hazardous waste sites are needed so that the information obtained on levels of white phosphorus in the environment can be used in combination with the known body burden of white phosphorus to assess the potential risk of adverse health effects in populations living in the vicinity of hazardous waste sites.
Exposure Levels in Humans. Data regarding the levels of elemental phosphorus in human tissues such as, blood, urine, fat, and breast milk, were not located in the literature. Such data, especially for occupationally exposed populations and populations surrounding hazardous waste sites, could be important. This information is necessary for assessing the need to conduct health studies on these populations.
Exposure Registries. No exposure registries for white phosphorus were located. This substance is not currently one of the compounds for which a subregistry has been established in the National Exposure Registry. The substance will be considered in the future when chemical selection is made for subregistries to be established. The information that is amassed in the National Exposure Registry facilitates the epidemiological research needed to assess adverse health outcomes that may be related to exposure to this substance.
5.7.2. On-going Studies
No on-going studies were located.
- POTENTIAL FOR HUMAN EXPOSURE - Toxicological Profile for White PhosphorusPOTENTIAL FOR HUMAN EXPOSURE - Toxicological Profile for White Phosphorus
- RecName: Full=Trafficking protein particle complex subunit 5RecName: Full=Trafficking protein particle complex subunit 5gi|71153352|sp|Q8IUR0.1|TPPC5_HUMANProtein
Your browsing activity is empty.
Activity recording is turned off.
See more...