NCBI Bookshelf. A service of the National Library of Medicine, National Institutes of Health.
Toxicological Profile for Molybdenum. Atlanta (GA): Agency for Toxic Substances and Disease Registry (US); 2020 May.
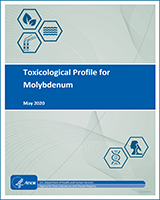
Toxicological Profile for Molybdenum.
Show detailsMRLs are derived when reliable and sufficient data exist to identify the target organ(s) of effect or the most sensitive health effect(s) for a specific duration for a given route of exposure. An MRL is an estimate of the daily human exposure to a hazardous substance that is likely to be without appreciable risk of adverse noncancer health effects over a specified route and duration of exposure. MRLs are based on noncancer health effects only; cancer effects are not considered. These substance-specific estimates, which are intended to serve as screening levels, are used by ATSDR health assessors to identify contaminants and potential health effects that may be of concern at hazardous waste sites. It is important to note that MRLs are not intended to define clean-up or action levels.
MRLs are derived for hazardous substances using the NOAEL/uncertainty factor approach. They are below levels that might cause adverse health effects in the people most sensitive to such chemical-induced effects. MRLs are derived for acute (1–14 days), intermediate (15–364 days), and chronic (≥365 days) durations and for the oral and inhalation routes of exposure. Currently, MRLs for the dermal route of exposure are not derived because ATSDR has not yet identified a method suitable for this route of exposure. MRLs are generally based on the most sensitive substance-induced endpoint considered to be of relevance to humans. Serious health effects (such as irreparable damage to the liver or kidneys, or birth defects) are not used as a basis for establishing MRLs. Exposure to a level above the MRL does not mean that adverse health effects will occur.
MRLs are intended only to serve as a screening tool to help public health professionals decide where to look more closely. They may also be viewed as a mechanism to identify those hazardous waste sites that are not expected to cause adverse health effects. Most MRLs contain a degree of uncertainty because of the lack of precise toxicological information on the people who might be most sensitive (e.g., infants, elderly, nutritionally or immunologically compromised) to the effects of hazardous substances. ATSDR uses a conservative (i.e., protective) approach to address this uncertainty consistent with the public health principle of prevention. Although human data are preferred, MRLs often must be based on animal studies because relevant human studies are lacking. In the absence of evidence to the contrary, ATSDR assumes that humans are more sensitive to the effects of hazardous substance than animals and that certain persons may be particularly sensitive. Thus, the resulting MRL may be as much as 100-fold below levels that have been shown to be nontoxic in laboratory animals.
Proposed MRLs undergo a rigorous review process: Health Effects/MRL Workgroup reviews within the Division of Toxicology and Human Health Sciences, expert panel peer reviews, and agency-wide MRL Workgroup reviews, with participation from other federal agencies and comments from the public. They are subject to change as new information becomes available concomitant with updating the toxicological profiles. Thus, MRLs in the most recent toxicological profiles supersede previously published MRLs. For additional information regarding MRLs, please contact the Division of Toxicology and Human Health Sciences, Agency for Toxic Substances and Disease Registry, 1600 Clifton Road NE, Mailstop S102-1, Atlanta, Georgia 30329-4027.
MINIMAL RISK LEVEL (MRL) WORKSHEET
Download PDF (11K)
Chemical Name: | Molybdenum |
CAS Numbers: | 7439-98-7 |
Date: | May 2020 |
Profile Status: | Final |
Route: | Inhalation |
Duration: | Acute |
MRL Summary: There are insufficient data for derivation of an acute-duration inhalation MRL for molybdenum due to the limited number of endpoints examined in the only available animal studies.
Rationale for Not Deriving an MRL: The database on the acute inhalation toxicity of molybdenum is limited to several 4-hour studies in rats exposed to ammonium dimolybdate (Jackson et al. 1991a), molybdenum trioxide (Jackson et al. 1991b, 1991d; Leuschner 2010), or sodium molybdate (Jackson et al. 1991c) and a 14-day study in rats and mice exposed to molybdenum trioxide (NTP 1997). No effects on lethality or the respiratory tract (most only examined the lungs) were observed at concentrations of 1,200 mg molybdenum/m3 and higher (Jackson et al. 1991a, 1991b, 1991c, 1991d; Leuschner 2010); several of the studies reported decreases in body weight on days 2–3 post-exposure (Jackson et al. 1991b, 1991c, 1991d). The NTP (1997) study evaluated the effect of molybdenum trioxide on the nasal cavity and on body weight in rats and mice exposed 6 hours/day, 5 days/week for 14 days. No adverse effects were observed in the nasal cavity. However, weight loss was observed at the highest concentration tested (200 mg molybdenum/m3); decreases in body weight gain were observed in male rats exposed to 67 mg molybdenum/m3 and in female rats and mice exposed to 200 mg/m3. Given the limited number of endpoints examined, the decrease in body weight gain was not considered a suitable basis for an acute-duration inhalation MRL because the database is inadequate for identifying the critical target of molybdenum toxicity following acute-duration inhalation exposure.
Agency Contacts (Chemical Managers): G. Daniel Todd
MINIMAL RISK LEVEL (MRL) WORKSHEET
Download PDF (10K)
Chemical Name: | Molybdenum |
CAS Numbers: | 7439-98-7 |
Date: | May 2020 |
Profile Status: | Final |
Route: | Inhalation |
Duration: | Intermediate |
MRL Summary: There are insufficient data for derivation of an intermediate-duration inhalation MRL for molybdenum due to the lack of studies identifying a critical target of toxicity.
Rationale for Not Deriving an MRL: Information on the intermediate-duration toxicity of molybdenum is limited to 90-day studies of molybdenum trioxide in rats and mice conducted by NTP (1997) that examined a wide range of potential targets, including reproductive endpoints. No toxicologically significant alterations were observed at concentrations of molybdenum trioxide as high as 67 mg/m3. Consistent with ATSDR’s practice of not using free-standing NOAELs as a POD, an intermediate-duration inhalation MRL was not derived.
Agency Contacts (Chemical Managers): G. Daniel Todd
MINIMAL RISK LEVEL (MRL) WORKSHEET
Download PDF (61K)
Chemical Name: | Molybdenum trioxide |
CAS Numbers: | 1313-27-5 |
Date: | May 2020 |
Profile Status: | Final |
Route: | Inhalation |
Duration: | Chronic |
MRL | 0.002 mg molybdenum/m3 |
Critical Effect: | Respiratory effect, squamous metaplasia of the epiglottis in female rats |
Reference: | NTP 1997 |
Point of Departure: | BMCL10 of 1.60 mg molybdenum/m3 (BMCLHEC of 0.071 mg Mo/m3) |
Uncertainty Factor: | 30 |
LSE Graph Key: | 11 |
Species: | Rat |
MRL Summary: A chronic-duration inhalation MRL of 0.002 mg molybdenum/m3 was derived for molybdenum trioxide based on an increased incidence of squamous metaplasia of the epiglottis in female rats exposed to 6.7 mg molybdenum/m3 as molybdenum trioxide 6 hours/day, 5 days/week for 2 years (NTP 1997). The MRL is based on a BMCL10 of 1.60 mg molybdenum/m3 (human equivalent concentration [HEC] of 0.071 mg molybdenum/m3) and a total uncertainty factor of 30 (3 for extrapolation from animals to humans with dosimetric adjustments and 10 for human variability).
Selection of the Critical Effect: There are limited data on the toxicity of inhaled molybdenum in humans. A study of workers at a molybdenite roasting facility exposed to molybdenum trioxide and other oxides found no alterations in lung function but did find increases in serum uric acid levels (Walravens et al. 1979); the TWA molybdenum concentration was 9.46 mg molybdenum/m3. Another study of workers exposed to ultrafine molybdenum trioxide dust reported respiratory symptoms (dyspnea and cough), radiographic abnormalities, and impaired lung function (Ott et al. 2004); the study did not provide monitoring data. Confidence in these cohort studies was considered very low (see Appendix C for additional information).
Data on the chronic toxicity of molybdenum in laboratory animals is limited to 2-year studies in rats and mice exposed to molybdenum trioxide (NTP 1997). In these studies, NTP (1997) examined a wide range of potential targets of toxicity. Adverse effects were limited to the respiratory tract, specifically the nasal respiratory and olfactory epithelium, epiglottis, and lungs. The respiratory tract was considered the critical target of molybdenum trioxide toxicity.
Selection of the Principal Study: The NTP (1997) study was selected as the principal study.
Summary of the Principal Study:
NTP. 1997. Toxicology and carcinogenicity studies of molybdenum trioxide (CAS No. 1313-27-5) in F344/N rats and B6C3F1 mice (inhalation studies). National Toxicology Program, Research Triangle Park, NC. NT PTR 462.
Groups of male and female F344/N rats and B6C3F1 mice (50/sex/species/group) were exposed to target concentrations of 0, 10, 30, or 100 mg/m3 molybdenum trioxide (0, 6.7, 20, and 67 mg molybdenum/m3) 6 hours/day, 5 days/week for 106 (rats) or 105 (mice) weeks; actual concentrations were within 15% of the target level. The average mass median aerodynamic diameter particle sizes (and geometric standard deviation, σg) were 1.5 (1.8), 1.6 (1.8), and 1.7 (1.8) μm for the 6.7, 20, and 67 mg/m3 concentrations, respectively. The following parameters were used to assess toxicity: twice daily cage-side observations, body weights (weekly for 12 weeks, at 15 weeks, monthly thereafter, and at termination), and histopathological examination of major tissues and organs. In addition, bone density and femoral curvature studies were conducted in 10 animals/sex/species/group.
No significant alterations in survival rates or body weight gain and no toxicologically significant alterations in bone density or curvature were found. Non-neoplastic lesions were only observed in the nose, larynx, and lungs; a summary of the type of lesions and incidences is presented in Table A-1. The severity of the respiratory lesions was concentration related. Significant increases in the incidence of alveolar/bronchiolar carcinoma and/or adenoma were observed in mice: carcinoma in male mice at ≥6.7 mg/m3, adenoma or carcinoma (combined) in male mice at 6.7 and 20 mg/m3, adenoma in female mice at ≥20 and 67 mg/m3, and adenoma or carcinoma (combined) in female mice at 67 mg/m3. In rats, the incidence of alveolar/bronchiolar adenoma or carcinoma (combined) was increased in males; however, the incidences (0/50, 1/49, 1/49, 4/60) were within the range of historical controls and NTP considered this to be equivocal evidence of carcinogenic activity.
Table A-1Incidence of Non-Neoplastic Respiratory Tract Lesions in Rats and Mice Exposed to Molybdenum Trioxide for 2 Years
Download PDF (59K)
Concentration (mg molybdenum/m3) | ||||
---|---|---|---|---|
0 | 6.7 | 20 | 67 | |
Male rats | ||||
Hyaline degeneration of nasal respiratory epithelium | 2/50 | 7/49 | 48/49a | 49/50a |
Squamous metaplasia of epiglottis | 0/49 | 11/48a | 16/49a | 39/49a |
Chronic lung inflammation in alveolus | 2/50 | 3/50 | 25/50a | 47/50a |
Female rats | ||||
Hyaline degeneration of nasal respiratory epithelium | 1/48 | 13/49a | 50/50a | 50/50a |
Hyaline degeneration of nasal olfactory epithelium | 39/48 | 47/49b | 50/50a | 50/50a |
Squamous metaplasia of epiglottis | 0/49 | 18/49a | 29/49a | 49/50a |
Chronic lung inflammation | 14/50 | 13/50 | 43/50a | 49/50a |
Male mice | ||||
Nasal suppurative inflammation | 2/50 | 6/50 | 10/49b | 8/50b |
Nasal olfactory epithelium atrophy | 3/50 | 5/50 | 3/49 | 10/50b |
Hyaline degeneration of nasal respiratory epithelium | 11/50 | 13/50 | 11/49 | 41/50a |
Squamous metaplasia of epiglottis | 0/50 | 26/49a | 37/48a | 49/50a |
Laryngeal hyperplasia | 1/50 | 3/49 | 6/48 | 41/50 |
Histiocyte infiltration in the lungs | 2/50 | 16/50a | 9/49b | 9/50b |
Alveolar epithelial metaplasia | 0/50 | 32/50a | 36/49a | 49/50a |
Female mice | ||||
Hyaline degeneration of nasal respiratory epithelium | 26/49 | 23/50 | 28/49 | 48/49a |
Hyaline degeneration of nasal olfactory epithelium | 22/49 | 14/50 | 14/49 | 36/49a |
Squamous metaplasia of epiglottis | 1/49 | 36/50a | 43/49a | 49/50a |
Laryngeal hyperplasia | 1/49 | 1/50 | 7/49 | 35/50 |
Alveolar epithelial metaplasia | 2/50 | 26/50a | 39/49a | 46/49b |
- a
Significantly different from controls; p≤0.01.
- b
Significantly different from controls; p≤0.05.
Source: NTP 1997
Selection of the Point of Departure for the MRL: The MRL was based on a BMCL10 of 1.60 mg molybdenum/m3 for squamous metaplasia of the epiglottis in female rats.
Benchmark dose (BMD) modeling was conducted for the respiratory tract lesions with statistically significant increases in incidence at ≥6.7 mg/m3 (squamous metaplasia of the epiglottis in male and female rats and mice, hyaline degeneration of the nasal respiratory and olfactory epithelium in female rats, histiocyte infiltration in the lungs in male mice, and alveolar epithelial metaplasia in male and female mice). The incidence data (Table A-1) provided adequate fit for four endpoints (squamous metaplasia in male rats, female rats, and female mice and hyaline degeneration of the nasal respiratory epithelium in female rats). The results of the BMD modeling are presented in the Benchmark Dose Modeling subsection and are summarized in Table A-2.
Table A-2Summary of Benchmark Dose Modeling
Download PDF (15K)
Endpoint | Selected model | BMC10 (mg Mo/m3) | BMCL10 (mg Mo/m3) |
---|---|---|---|
Squamous metaplasia of the epiglottis in male rats | Multistage, 2-degree (Table A-4 and Figure A-1) | 4.36 | 3.53 |
Hyaline degeneration of the respiratory epithelium in female rats | Log-logistic (Table A-5 and Figure A-2) | 5.87 | 4.82 |
Squamous metaplasia of the epiglottis in female rats | Weibull (Table A-6 and Figure A-3) | 1.97 | 1.60 |
Squamous metaplasia of the epiglottis in male mice | Gamma (Table A-7 and Figure A-4) | 1.30 | 1.06 |
BMC = benchmark concentration; BMCL = 95% lower confidence limit on the benchmark concentration
A summary of the potential POD values is presented in Table A-3. Because there are dosimetric differences in regional respiratory tract deposition of aerosols between animal species, a comparison was made between the human equivalent concentration PODs (PODHEC). The lowest PODHEC, BMCLHEC of 0.071 mg molybdenum/m3 for squamous metaplasia of the epiglottis in female rats, was selected as the POD for the MRL.
Table A-3Summary of PODs and HECs
Download PDF (14K)
Endpoint | PODs (mg Mo/m3) | RDDR valuesa | HECsb (mg Mo/m3) |
---|---|---|---|
Squamous metaplasia of the epiglottis in male rats | 3.53 (BMCL) | 0.459 | 0.28 |
Hyaline degeneration of the respiratory epithelium in female rats | 4.82 (BMCL) | 0.248 | 0.21 |
Hyaline degeneration of the olfactory epithelium in female rats | 6.7 (LOAEL) | 0.248 | 0.30 |
Squamous metaplasia of the epiglottis in female rats | 1.60 (BMCL) | 0.248 | 0.071 |
Squamous metaplasia of the epiglottis in male mice | 1.06 (BMCL) | 0.441 | 0.08 |
Histiocyte infiltration in the lungs of male mice | 6.7 (LOAEL) | 1.046 | 1.3 |
Alveolar epithelial metaplasia in male mice | 6.7 (LOAEL) | 1.046 | 1.3 |
Squamous metaplasia of the epiglottis in female mice | 6.7 (LOAEL) | 0.367 | 0.44 |
Alveolar epithelial metaplasia in female mice | 6.7 (LOAEL) | 3.067 | 3.7 |
- a
RDDR values specific for each region of the respiratory tract (extrathoracic, tracheobronchial, and pulmonary) were calculated using EPA’s RDDR calculator with reference body weights of 0.40, 0.25, 0.040, and 0.035 kg for male rats, female rats, male mice, and female mice, respectively, and reported particle sizes and particle size distributions.
- b
HEC calculated by multiplying the duration-adjusted POD (POD × 6 hours/24 hours × 5 days/7days) by the RDDR value.
BMCL = 95% lower confidence limit on the benchmark concentration; HEC = human equivalent concentration; LOAEL = lowest observed adverse effect level; POD = point of departure; RDDR = regional deposited dose ratio for the specific region of the respiratory tract
Adjustment for Intermittent Exposure: The PODs were adjusted for intermittent exposure (6 hours/day, 5 days/week).
Calculation of Human Equivalent Concentration: HECs were calculated for each potential POD by multiplying the duration-adjusted POD by the regional deposited dose ratio (RDDR) for the specific region of the respiratory tract. The RDDR is a factor used to adjust particulate exposure concentration in animals to a predicted concentration in humans that would be associated with the same dose delivered to a specific region of the respiratory tract or to the blood (EPA 1994). The RDDRs were calculated using EPA’s RDDR calculator with reference body weights of 0.40, 0.25, 0.040, and 0.035 kg for the male rats, female rats, male mice, and female mice, respectively, the reported particle sizes, and particle size distributions. The particles were assumed to be monodispersed given that the σg was 1.8.
Uncertainty Factor: The BMCLHEC is divided by a total uncertainty factor (UF) of 30.
- 3 for extrapolation from animals to humans with dosimetric adjustments
- 10 for human variability
- BMCLHEC ÷ UFs = MRL
- 0.071 mg molybdenum/m3 ÷ 30 = 0.002 mg molybdenum/m3
Other Additional Studies or Pertinent Information that Lend Support to this MRL: This MRL is specific to molybdenum trioxide; there are insufficient data to evaluate the health effects associated with inhalation exposure to other molybdenum compounds.
Benchmark Dose Modeling: The incidence data (Table A-1) for respiratory tract lesions, which had significant increases in incidence at ≥6.7 mg/m3 (squamous metaplasia of the epiglottis in male and female rats and mice, hyaline degeneration of the nasal respiratory and olfactory epithelium in female rats, histiocyte infiltration in the lungs in male mice, and alveolar epithelial metaplasia in male and female mice), were fit to all available dichotomous models in EPA’s Benchmark Dose Software (BMDS, version 3.1) using the extra risk option. Adequate model fit was judged by three criteria: goodness-of-fit statistics (p-value >0.1), visual inspection of the dose-response curve, and scaled residual at the data point (except the control) closest to the predefined benchmark response (BMR). Among all of the models providing adequate fit to the data, the lowest BMCL was selected as the POD when the difference between the BMCLs estimated from these models was >3-fold; otherwise, the BMCL from the model with the lowest Akaike’s Information Criterion (AIC) was chosen. For all lesion types, a BMR of 10% was used. Since the incidence of hyaline degeneration in the olfactory epithelium of female rats was essentially the same response level across groups, the data were not modeled since they provide limited information on the dose-response relationship. The incidence data for histiocyte infiltration in the lungs in male mice, alveolar epithelial metaplasia in male mice, squamous metaplasia in female mice, and alveolar epithelial metaplasia in female mice did not fit any of the available dichotomous models. The model predictions for the other endpoints are presented in Tables A-4, A-5, A-6, and A-7 and the fits of the selected models are presented in Figures A-1, A-2, A-3, and A-4.
Table A-4Model Predictions for Squamous Metaplasia of the Epiglottis in Male Rats Exposed to Molybdenum Trioxide (NTP 1997)
Download PDF (100K)
Model | DF | χ2 | χ2 Goodness-of-fit p-valuea | Scaled residualsb | AIC | BMC10 (mg/m3) | BMCL10 (mg/m3) | ||
---|---|---|---|---|---|---|---|---|---|
Dose below BMC | Dose above BMC | Overall largest | |||||||
Gammac | 2 | 3.07 | 0.22 | 0.00 | 1.55 | 1.55 | 169.98 | 4.36 | 3.53 |
Logistic | 2 | 9.45 | 0.01 | 1.50 | 0.93 | −2.47 | 181.70 | ND | ND |
LogLogisticd | 2 | 3.56 | 0.17 | 0.00 | 0.98 | −1.42 | 170.75 | 3.80 | 2.23 |
LogProbitd | 2 | 3.74 | 0.15 | −0.00 | 0.93 | −1.51 | 170.95 | ND | ND |
Multistage (1-degree)e | 3 | 3.07 | 0.38 | 0.00 | 1.55 | 1.55 | 167.98 | 4.36 | 3.53 |
Multistage (2-degree) e , f | 3 | 3.07 | 0.38 | 0.00 | 1.55 | 1.55 | 167.98 | 4.36 | 3.53 |
Multistage (3-degree)e | 3 | 3.07 | 0.38 | 0.00 | 1.55 | 1.55 | 167.98 | 4.36 | 3.53 |
Probit | 2 | 9.17 | 0.01 | 1.60 | 0.90 | −2.37 | 181.01 | ND | ND |
Weibullc | 2 | 3.07 | 0.22 | 0.00 | 1.55 | 1.55 | 169.98 | 4.36 | 3.53 |
- a
Values <0.1 fail to meet conventional goodness-of-fit criteria.
- b
Scaled residuals at doses immediately below and above the BMC; also the largest residual at any dose.
- c
Power restricted to ≥1.
- d
Slope restricted to ≥1.
- e
Betas restricted to ≥0.
- f
Selected model. BMCLs for models providing adequate fit were sufficiently close (differed by <3-fold). Therefore, the model with the lowest AIC was selected.
AIC = Akaike Information Criterion; BMC = maximum likelihood estimate of the exposure concentration associated with the selected benchmark response; BMCL = 95% lower confidence limit on the BMC (subscripts denote benchmark response: i.e., 10 = exposure concentration associated with 10% extra risk); DF = degrees of freedom; ND = not determined, goodness-of-fit criteria, p<0.10
Figure A-1Fit of 2-Degree Multistage Model to Data on Incidence of Squamous Metaplasia of the Epiglottis in Male Rats Exposed to Molybdenum Trioxide
Table A-5Model Predictions for Hyaline Degeneration of the Nasal Respiratory Epithelium in Female Rats Exposed to Molybdenum Trioxide (NTP 1997)
Download PDF (101K)
Model | DF | χ2 | χ2 Goodness-of-fit p-valuea | Scaled residualsb | AIC | BMC10 (mg/m3) | BMCL10 (mg/m3) | ||
---|---|---|---|---|---|---|---|---|---|
Dose below BMC | Dose above BMC | Overall largest | |||||||
Gammac | 2 | 4.41 | 0.11 | 0.14 | −1.03 | 1.82 | 77.98 | 3.69 | 2.85 |
Logistic | 3 | 5.04 | 0.17 | −1.20 | −0.37 | 1.86 | 77.15 | 3.78 | 2.95 |
LogLogistic d , e | 2 | 0.02 | 0.99 | 0.00 | −0.00 | 0.13 | 70.45 | 5.87 | 4.82 |
LogProbitd | 1 | 0.00 | 0.99 | −0.00 | −0.00 | −0.00 | 72.42 | 5.92 | 4.73 |
Multistage (1-degree)f | 2 | 18.41 | 0.00 | 0.28 | −3.28 | −3.28 | 95.80 | ND | ND |
Multistage (2-degree)f | 2 | 2.81 | 0.24 | 0.20 | −1.21 | −1.21 | 74.57 | 3.40 | 2.54 |
Multistage (3-degree)f | 2 | 0.02 | 0.99 | 0.01 | −0.05 | 0.15 | 70.46 | 4.77 | 2.39 |
Probit | 2 | 0.48 | 0.79 | 0.49 | −0.28 | 0.49 | 71.03 | 4.09 | 3.12 |
- a
Values <0.1 fail to meet conventional goodness-of-fit criteria.
- b
Scaled residuals at doses immediately below and above the BMC; also the largest residual at any dose.
- c
Power restricted to ≥1.
- d
Slope restricted to ≥1.
- e
Selected model. BMCLs for models providing adequate fit were sufficiently close (differed by <3-fold). Therefore, the model with the lowest AIC was selected.
- f
Betas restricted to ≥0.
AIC = Akaike Information Criterion; BMC = maximum likelihood estimate of the exposure concentration associated with the selected benchmark response; BMCL = 95% lower confidence limit on the BMC (subscripts denote benchmark response: i.e., 10 = exposure concentration associated with 10% extra risk); DF = degrees of freedom; ND = not determined, goodness-of-fit criteria, p<0.10
Figure A-2Fit of Log-logistic Model to Data on Incidence of Hyaline Degeneration of the Nasal Respiratory Epithelium in Female Rats Exposed to Molybdenum Trioxide
Table A-6Model Predictions for Squamous Metaplasia of the Epiglottis in Female Rats Exposed to Molybdenum Trioxide (NTP 1997)
Download PDF (100K)
Model | DF | χ2 | χ2 Goodness-of-fit p-valuea | Scaled residualsb | AIC | BMC10 (mg/m3) | BMCL10 (mg/m3) | ||
---|---|---|---|---|---|---|---|---|---|
Dose below BMC | Dose above BMC | Overall largest | |||||||
Gammac | 2 | 2.05 | 0.36 | 0.00 | 1.00 | 1.00 | 146.51 | 1.97 | 1.60 |
Logistic | 2 | 15.55 | 0.00 | −2.67 | 2.17 | −2.67 | 163.85 | ND | ND |
LogLogisticd | 1 | 5.02 | 0.03 | −0.00 | 0.82 | −1.58 | 152.04 | ND | ND |
LogProbite | 2 | 4.16 | 0.12 | −0.00 | 0.79 | −1.51 | 148.92 | 2.76 | 1.41 |
Multistage (1-degree)e | 3 | 2.05 | 0.56 | −0.00 | 1.00 | 1.00 | 144.51 | 1.97 | 1.60 |
Multistage (2-degree)e | 1 | 2.05 | 0.15 | −0.00 | 1.04 | 1.04 | 148.50 | 1.99 | 1.60 |
Multistage (3-degree)e | 1 | 1.98 | 0.16 | −0.00 | 1.11 | 1.11 | 148.42 | 2.02 | 1.61 |
Probit | 2 | 17.51 | 0.00 | −2.85 | 2.00 | −2.13 | 166.05 | ND | ND |
Weibull f | 3 | 2.05 | 0.56 | −0.00 | 1.00 | 1.00 | 144.51 | 1.97 | 1.60 |
- a
Values <0.1 fail to meet conventional goodness-of-fit criteria.
- b
Scaled residuals at doses immediately below and above the BMC; also the largest residual at any dose.
- c
Power restricted to ≥1.
- d
Slope restricted to ≥1.
- e
Betas restricted to ≥0.
- f
Selected model. BMCLs for models providing adequate fit were sufficiently close (differed by <3-fold). Therefore, the model with the lowest AIC was selected.
AIC = Akaike Information Criterion; BMC = maximum likelihood estimate of the exposure concentration associated with the selected benchmark response; BMCL = 95% lower confidence limit on the BMC (subscripts denote benchmark response: i.e., 10 = exposure concentration associated with 10% extra risk); DF = degrees of freedom; ND = not determined, goodness-of-fit criteria, p<0.10
Figure A-3Fit of Weibull Model to Data on Incidence of Squamous Metaplasia of the Epiglottis in Female Rats Exposed to Molybdenum Trioxide
Table A-7Model Predictions for Squamous Metaplasia of the Epiglottis in Male Mice Exposed to Molybdenum Trioxide (NTP 1997)
Download PDF (102K)
Model | DF | χ2 | χ2 Goodness-of-fit p-valuea | Scaled residualsb | AIC | BMC10 (mg/m3) | BMCL10 (mg/m3) | ||
---|---|---|---|---|---|---|---|---|---|
Dose below BMC | Dose above BMC | Overall largest | |||||||
Gamma c , d | 3 | 5.55 | 0.14 | −0.00 | 1.60 | −1.65 | 135.46 | 1.30 | 1.06 |
Logistic | 2 | 61.77 | 0.00 | −3.19 | 2.80 | −6.62 | 164.85 | ND-1 | ND-1 |
LogLogistice | 1 | 1.42 | 0.23 | −0.00 | 0.34 | −0.85 | 134.73 | ND-2 | ND-2 |
LogProbitd | 1 | 0.88 | 0.35 | −0.00 | 0.31 | −0.70 | 136.12 | ND-2 | ND-2 |
Multistage (1-degree)f | 2 | 5.55 | 0.06 | −0.00 | 1.60 | −1.65 | 137.46 | ND-1 | ND-1 |
Multistage (2-degree)f | 3 | 5.55 | 0.14 | −0.00 | 1.60 | −1.65 | 135.46 | 1.30 | 1.06 |
Multistage (3-degree)f | 3 | 5.55 | 0.14 | −0.00 | 1.60 | −1.65 | 135.46 | 1.30 | 1.06 |
Probit | 2 | 90.03 | 0.00 | −3.63 | 2.65 | −8.24 | 171.89 | ND-1 | ND-1 |
Weibullc | 3 | 5.55 | 0.14 | −0.00 | 1.60 | −1.65 | 135.46 | 1.30 | 1.06 |
- a
Values <0.1 fail to meet conventional goodness-of-fit criteria.
- b
Scaled residuals at doses immediately below and above the BMC; also the largest residual at any dose.
- c
Power restricted to ≥1.
- d
Selected model. BMCLs for models providing adequate fit were sufficiently close (differed by <3-fold). Therefore, the model with the lowest AIC was selected.
- e
Slope restricted to ≥1.
- f
Betas restricted to ≥0.
AIC = Akaike Information Criterion; BMC = maximum likelihood estimate of the exposure concentration associated with the selected benchmark response; BMCL = 95% lower confidence limit on the BMC (subscripts denote benchmark response: i.e., 10 = exposure concentration associated with 10% extra risk); DF = degrees of freedom; ND-1 = not determined, goodness-of-fit criteria, p<0.10; ND-2 = not determined, BMCL was 10 times lower than lowest non-zero dose
Figure A-4Fit of Gamma Model to Data on Incidence of Squamous Metaplasia of the Epiglottis in Male Mice Exposed to Molybdenum Trioxide
Agency Contacts (Chemical Managers): G. Daniel Todd
MINIMAL RISK LEVEL (MRL) WORKSHEET
Download PDF (13K)
Chemical Name: | Molybdenum |
CAS Numbers: | 7439-98-7 |
Date: | May 2020 |
Profile Status: | Final |
Route: | Oral |
Duration: | Acute |
MRL Summary: There are insufficient data for derivation of an acute-duration oral MRL for molybdenum due inadequate information on the molybdenum and copper intake in the acute-duration studies reporting adverse reproductive effects and the conflicting results between the acute-duration studies and high-quality, intermediate-duration studies.
Rationale for Not Deriving an MRL: A small number of studies have evaluated the acute toxicity of molybdenum. One human study (Deosthale and Gopalan 1974) examining a limited number of potential endpoints did not find alterations in urinary uric acid levels in subjects exposed to doses as high as 0.022 mg molybdenum/kg/day for 10 days. In rabbits, exposure to 1.2 mg molybdenum/kg/day as ammonium heptamolybdate in the diet for 14 days resulted in a 60% increase in serum triglyceride levels (Bersenyi et al. 2008); no histological alterations were observed in the liver or kidneys. The toxicological significance of this finding is not known and has not been reported in a study of male rabbits exposed to 0.58 mg molybdenum/kg/day as ammonium heptamolybdate (Bersenyi et al. 2008) or rats exposed to 60 mg molybdenum/kg/day as sodium molybdate for 90 days (Murray et al. 2014a).
Reproductive effects have been observed in male and female mice and rabbits. In female mice, an increased rate of abnormal MII oocytes was observed at 11 mg molybdenum/kg/day (Zhang et al. 2013). A second acute-exposure study in rabbits exposed to 1.2 mg molybdenum/kg/day as ammonium heptamolybdate for 14 days (Bersenyi et al. 2008) and an intermediate-duration oral study in rats exposed to 60 mg molybdenum/kg/day as sodium molybdate for 90 days (Murray et al. 2014a) did not find histological alterations in the ovaries. In males, a significant decrease in sperm concentration and motility and an increase in sperm abnormalities were observed at 25 mg molybdenum/kg/day in mice (Zhai et al. 2013). A rabbit study reported a reduction in mature spermatocytes in rabbits exposed to 0.58 mg molybdenum/kg/day, but did not report the incidence or statistical significance (Bersenyi et al. 2008). Intermediate-duration studies in rats did not find significant alterations in sperm parameters in rats exposed to 60 mg molybdenum/kg/day as sodium molybdate for 90 days (Murray et al. 2014a) or in rats exposed to 40 mg molybdenum/kg/day as sodium molybdate in a 2-generation study (Murray et al. 2019). Interpretation of the Zhang et al. (2013) and Zhai et al. (2013) studies is limited by the lack of information on the copper content of the “commercial standard pellet” diet used in these studies and the lack of information on molybdenum doses. ATSDR estimated doses using the reported molybdenum concentration in the drinking water and reference values for water consumption and body weight (Zhang et al. 2013) or the midpoint of the reported body weights and an estimated water consumption based on this body weight (Zhai et al. 2013).
The acute-duration oral database was not considered suitable for derivation of an MRL due to the limited information on the molybdenum and copper intake and the conflicting results between the findings of the Zhang et al. (2013) and Zhai et al. (2013) studies with the intermediate-duration Murray et al. (2014a, 2019) studies.
Agency Contacts (Chemical Managers): G. Daniel Todd
MINIMAL RISK LEVEL (MRL) WORKSHEET
Download PDF (8.0K)
Chemical Name: | Molybdenum |
CAS Numbers: | 7439-98-7 |
Date: | May 2020 |
Profile Status: | Final |
Route: | Oral |
Duration: | Intermediate |
MRL | 0.06 mg molybdenum/kg/day |
Critical Effect: | Renal effect, proximal tubule hyperplasia |
Reference: | Murray et al. 2014a |
Point of Departure: | NOAEL of 17 mg molybdenum/kg/day |
Uncertainty Factor: | 100 |
Modifying Factor: | 3 |
LSE Graph Key: | 14 |
Species: | Rat |
MRL Summary: An intermediate-duration oral MRL of 0.06 mg molybdenum/kg/day was derived for molybdenum based on an increased incidence of renal proximal tubule hyperplasia in rats exposed to sodium molybdate in the diet for 90 days (Murray et al. 2014a). The MRL is based on a NOAEL of 17 mg molybdenum/kg/day, a total uncertainty factor of 100 (10 for extrapolation from animals to humans, and 10 for human variability), and a modifying factor of 3 (to address concern that reproductive/developmental alterations may be sensitive outcomes in populations with marginal copper intakes). The MRL is calculated based on the assumption of healthy dietary levels of molybdenum and copper and represents the level of exposure above and beyond the normal diet.
Selection of the Critical Effect: Several adverse effects have been reported in intermediate-duration oral studies in laboratory animals. Observed effects include kidney damage (Bompart et al. 1990; Murray et al. 2014a, 2019), decreased body weight gain (Bompart et al. 1990; Lyubimov et al. 2004; Mills et al. 1958; Murray et al. 2014a; Van Reen and Williams 1956; Williams and Van Reen 1956), hematological effects (Arrington and Davis 1953; Lyubimov et al. 2004), reproductive effects (Fungwe et al. 1990; Jeter and Davis 1954; Lyubimov et al. 2004; Murray et al. 2014a; Pandey and Singh 2002; Wang et al. 2016), and developmental effects (Pandey and Singh 2002).
The toxicity of molybdenum can be influenced by several factors including animal species; previous dietary history; relative amounts of dietary molybdenum, copper, and sulfur; and the form of molybdenum. Copper nutritional status is particularly important in evaluating the relevance of animal toxicity studies for establishing an MRL. Marked differences in the distribution of molybdenum and copper and the toxicity of molybdenum have been observed in rats exposed to high doses of molybdenum and maintained on a copper-deficient diet compared to those maintained on a copper-adequate diet (Brinkman and Miller 1961; Johnson et al. 1969; Nederbragt 1980, 1982; Sasmal et al. 1968). Since the average copper intake of the U.S. population exceeds the dietary requirements (NAS 2001), studies in which animals were fed inadequate levels of copper were not considered relevant for MRL derivation and were excluded from further consideration. Similarly, studies in which the molybdenum was administered as ammonium tetrathiomolybdate were also excluded since administration of tetrathiomolybdate compounds can result in shifts in the copper levels in rats fed copper-adequate diets (increases in serum and kidney copper levels and decreases in liver copper levels) (Mills et al. 1981a), and copper supplementation of rats exposed to ammonium tetrathiomolybdate resulted in a reversal of adverse effects (Lyubimov et al. 2004). A summary of the NOAEL and LOAEL values for studies with adequate copper in the diet is presented in Table A-8.
Table A-8Summary of Health Effects Following Intermediate-Duration Oral Exposure to Molybdenum
Download PDF (62K)
Species, duration (route) | NOAEL | LOAEL | Effect | Reference (compound) | ||||
---|---|---|---|---|---|---|---|---|
Body weight | ||||||||
Rat 147–158 days (diet) | 40 | 22% decrease in maternal body weight gain on GDs 0–7; <10% decrease over entire study | Murray et al. 2019 (sodium molybdate) | |||||
Rat 90 days (diet) | 17 | 60 | Decrease in body weight gain in males; terminal weights 15.2% less than controls | Murray et al. 2014a (sodium molybdate) | ||||
Rat 5 weeks (diet) | 74 | 36% decrease in body weight gain | Mills et al. 1958 (sodium molybdate) | |||||
Rat 8 weeks (gavage) | 40 | 80 | Decrease in body weight gain; terminal body weight was 26% lower than in controls | Bompart et al. 1990 (ammonium heptamolybdate) | ||||
Rat 6 weeks (diet) | 85 | Williams and Van Reen 1956 (sodium molybdate) | ||||||
Rat 6 weeks (diet) | 90 | 22% decrease in body weight gain | Williams and Van Reen 1956 (sodium molybdate) | |||||
Rat 4–5 weeks (diet) | 110 | 46–48% decrease in body weight gain | Van Reen and Williams 1956 (sodium molybdate) | |||||
Rat 147–158 days (drinking water) | 40 | Murray et al. 2019 (sodium molybdate) | ||||||
Hematological effects | ||||||||
Rabbit 30–84 days (diet) | 25 | 54 | Anemia | Arrington and Davis 1953 (sodium molybdate) | ||||
Rabbit ≥8 weeks (diet) | 7 | Jeter and Davis 1954 (sodium molybdate) | ||||||
Rat 90 days (diet) | 60 | Murray et al. 2014a (sodium molybdate) | ||||||
Rat 6 weeks (diet) | 70 | Gray and Daniel 1954 (sodium molybdate) | ||||||
Kidney effects | ||||||||
Rat 90 days (diet) | 17 | 60 | Slight diffuse hyperplasia in proximal tubules | Murray et al. 2014a (sodium molybdate) | ||||
Rat 8 weeks (gavage) | 40 | 80 | Diuresis and creatinuria and decreases in creatinine clearance | Bompart et al. 1990 (ammonium heptamolybdate) | ||||
Rat 147–158 days (diet) | 40 | Murray et al. 2019 (sodium molybdate) | ||||||
Rats 147–158 days (drinking water) | 40 | Murray et al. 2019 (sodium molybdate) | ||||||
Reproductive effects | ||||||||
Rat 8 weeks (drinking water) | 0.76 | 1.5 | Prolonged estrus phase; no effect on female fertility | Fungwe et al. 1990 (sodium molybdate) | ||||
Rat 60 days (gavage) | 3.4a | 10a | Decreases in sperm count and motility; increases in sperm abnormalities | Pandey and Singh 2002 (sodium molybdate) | ||||
Rat 60 days (gavage) | 10a | Decreases in male fertility | Pandey and Singh 2002 (sodium molybdate) | |||||
Mouse 100 days (drinking water) | 100 | Decreased sperm density and motility | Wang et al. 2016 (unspecified molybdenum compound) | |||||
Rat 90 days (diet) | 60 | No treatment-related alterations in sperm parameters; no alterations in vaginal cytology, estrus cycle, or histology of male or female reproductive tissues | Murray et al. 2014a (sodium molybdate) | |||||
Rat ≥8 weeks (diet) | 7 | No effect on fertility | Jeter and Davis 1954 (sodium molybdate) | |||||
Rat 2 generations (diet) | 40 | No effects on sperm parameters, estrous cycling, or fertility | Murray et al. 2019 (sodium molybdate) | |||||
Rat 2 generations (drinking water) | 40 | No effects on sperm parameters, estrous cycling, or fertility | Murray et al. 2019 (sodium molybdate) | |||||
Developmental effectsb | ||||||||
Rat (males only) 60 days (gavage) | 10a | Increased post-implantation losses, increased resorptions, decreased number of live fetuses, and decreases in fetal weight and crown-rump length | Pandey and Singh 2002 (sodium molybdate) | |||||
Rat ≥8 weeks (diet) | 7 | Jeter and Davis 1954 (sodium molybdate) | ||||||
Rat GDs 6–20 (diet) | 37.5 | Murray et al. 2014b (sodium molybdate) | ||||||
Rat 2 generations (diet) | 40 | Murray et al. 2019 (sodium molybdate) | ||||||
Rat 2 generations (drinking water) | 40 | Murray et al. 2019 (sodium molybdate) |
- a
Adjusted for intermittent exposure (5 days/week).
- b
The copper content of the basal diet (6 g/kg diet) in the Fungwe et al. (1990) study is below the recommended level of 8 g/kg required for pregnancy and lactation. Thus, the observed developmental effects are not included in this table.
GD = gestation day; LOAEL = lowest-observed-adverse-effect level; NOAEL = no-observed-adverse-effect level
The Fungwe et al. (1990) study identified the lowest LOAEL value: prolonged estrus phase without an effect on fertility in rats exposed to 1.5 mg molybdenum/kg/day as sodium molybdate in drinking water for 8 weeks (Fungwe et al. 1990). However, this finding was not selected as the critical effect because other high-quality studies have not reported estrus cycle alterations in a 90-day (Murray et al. 2014a) study or 2-generation study (Murray et al. 2019). Additionally, confidence in this study is decreased by the limited information on doses. The study reported molybdenum drinking water concentrations but did not calculate doses. ATSDR estimated doses using reference values for body weight and drinking water consumption. As presented in Table A-9, a comparison with the molybdenum liver concentrations in this study with levels reported in the Murray et al. (2014a, 2019) studies suggested that these estimated doses may have underestimated the actual doses. In the Fungwe et al. (1989) study, the average liver molybdenum level was 10.76 μg/g in the 15 mg/kg/day group; in the Murray et al. (2014a, 2014b) studies, the liver molybdenum level was 4.10–4.92 μg/g in the 17 mg/kg/day groups.
Table A-9Comparison of Molybdenum Liver Concentrations in Female Rats
Download PDF (39K)
Study | Dose Liver molybdenum concentration | ||||
---|---|---|---|---|---|
Murray et al. 2019a (water exposure, unless noted) | 0 mg/kg/day | 5 mg/kg/day | 17 mg/kg/day | 40 mg/kg/day | 40 mg/kg/day (dietary exposure) |
2.96 μg/g | 3.18 μg/g | 4.10 μg/g | 6.48 μg/g | 7.23 μg/g | |
Murray et al. 2014ab (dietary exposure) | 0 mg/kg/day | 5 mg/kg/day | 17 mg/kg/day | 60 mg/kg/day | |
2.46 μg/g | 3.51 μg/g | 4.92 μg/g | 13.0 μg/g | ||
Murray et al. 2014ab (dietary exposure) | 0 mg/kg/day | 0.76 mg/kg/day | 1.5 mg/kg/day | 7.6 mg/kg/day | 15 mg/kg/day |
2.63 μg/g | 5.01 μg/g | 5.03 μg/g | 7.77 μg/g | 10.76 μg/g |
- a
Parental generation.
- b
Liver concentrations reported in Murray et al. (2019).
- c
Liver concentrations from a study by Fungwe et al. (1990) utilizing the same water concentrations as Fungwe et al. (1989).
The next highest LOAEL is 14 mg molybdenum/kg for decreases in sperm count and motility, increased sperm abnormalities, decreased male fertility, increased post-implantation losses, decreased number of live fetuses, and decreased fetal weight in a study of male rats receiving gavage doses of sodium molybdate 5 days/week during a 60-day period (Pandey and Singh 2002). The reliability of this LOAEL is uncertain due to the lack of information on the copper content of the diet and because decreases in fertility and alterations in sperm parameters have not been observed in other high-quality studies involving exposure to 40 mg molybdenum/kg/day via the diet or drinking water in a 2-generation study (Murray et al. 2019) or 60 mg molybdenum/kg/day via the diet in a 90-day study (Murray et al. 2014a). Additionally, no developmental effects were observed in single-generation (Murray et al. 2014b) or 2-generation (Murray et al. 2019) studies in rats exposed to 37.5–40 mg/kg/day.
As with the reproductive and developmental effects, only one study reported hematological effects. Anemia was reported in rabbits exposed to 54 mg molybdenum/kg/day as sodium molybdate in the diet for 30–84 days (Arrington and Davis 1953). This is considered a low-quality study because the molybdenum was sprayed on the food pellets but there was no measurement of actual dietary concentrations, only 2–5 animals per group were tested, and no information was provided on which hematological parameters were altered. Additionally, the diet may not have provided adequate copper levels since copper supplementation was administered to the 54 mg/kg/day group to prevent deaths in the 3/5 animals that exhibited “severe toxic symptoms” characteristic of copper deficiency.
If the reproductive, developmental, and hematological effects are excluded because they were reported in lesser-quality studies and were not confirmed in higher-quality studies, then the lowest LOAEL is 60 mg/kg/day for body weight and renal effects (Murray et al. 2014a). A 15% decrease in body weight gain was observed in male rats; no significant alterations were observed in females. Although a decrease in food consumption was also observed at this dose level, decreases in food efficiency observed at this dose suggest that the decrease in body weight was not solely related to the decreased food intake. The renal effects consisted of slight diffuse hyperplasia in the renal proximal tubules of 2/10 female rats. The investigators (Murray et al. 2014a) noted that this effect is an uncommon background finding in rats of this age and considered it to be treatment related; they also suggested that the effect may be due to the high levels of copper in the kidneys. Kidney effects (degeneration followed by regeneration) have been observed in rats exposed to high levels of copper in the diet (Haywood 1985). A second molybdenum study (Bompart et al. 1990) reported diuresis, creatinuria, decreases in creatinine clearance, and increases in daily excretion of immunoreactive kallikrein in rats administered 80 mg molybdenum/kg/day via gavage for 8 weeks. These alterations are suggestive of decreased glomerular function and distal tubule damage; the absence of changes in the brush border enzymes alanine aminopeptidase and γ-glutamyl transpeptidase suggests no damage to the proximal tubule functional capacity. The study did not include histopathological examination of the kidneys. Although the incidence of proximal tubular hyperplasia was not statistically significant in the high-dose females in the Murray et al. (2014a) study, support for identifying this as the critical effect comes from the Bompart et al. (1990) study, which found evidence of impaired renal function in rats exposed to a slightly higher dose.
Several studies have reported decreases in body weight gain; the lowest LOAEL for this effect was 40 mg molybdenum/kg/day as sodium molybdate in the diet in a 2-generation study (Murray et al. 2019). This study reported a 22% decrease in body weight gain on GDs 0–7 in the parental-generation females. The difference in body weight gain over the length of the study was <10% lower than the controls. This was not observed in the F1 generation and was not observed in P or F1 generation rats similarly exposed to 40 mg molybdenum/kg/day as sodium molybdate in the drinking water (Murray et al. 2019). Decreases in body weight have also been observed at higher molybdenum doses (Bompart et al. 1990; Mills et al. 1958; Murray et al. 2014a; Van Reem and Williams 1956). The decrease in body weight gain observed in the Murray et al. (2019) study was not selected as the basis of the MRL because it was not replicated in the F1 generation or in rats exposed via drinking water (Murray et al. 2019).
Selection of the Principal Study: The Murray et al. (2014a) study was selected as the principal study because it identified the lowest LOAEL for renal effects.
Summary of the Principal Study:
Murray FJ, Sullivan FM, Tiwary AK, et al 2014a. 90-Day subchronic toxicity study of sodium molybdate dihydrate in rats. Regul Toxicol Pharmacol 79:579–588.
Groups of 10 male and 10 female Sprague-Dawley rats were exposed to 0, 5, 17, or 60 mg molybdenum/kg/day (actual concentrations were 0, 4.5, 15.1, and 54.8 mg/kg/day, respectively, in males and 0, 5.4, 19.0, and 65.2 mg/kg/day, respectively, in females and the average overall intakes were 0, 5.0, 17.1, and 60.0 mg/kg/day, respectively) as sodium molybdate dihydrate in the diet for 91 and 92 days; additional groups of rats (10/sex/group) were similarly exposed to 0 or 60 mg/kg/day for 91–92 days and then continued on the basal diet for 60 days. The basal diet contained 906.5 μg/kg molybdenum and 14.23 mg/kg copper; the investigators estimated that the control group received 0.08 mg molybdenum/kg/day. The following parameters were used to assess toxicity: cage-side observations, weekly clinical examinations, ophthalmic examination, weekly body weight measurements, measurement of hematological (hemoglobin, hematocrit, erythrocyte, platelet, mean corpuscular hemoglobin concentration, mean corpuscular volume, red cell distribution width, total and differential leukocyte, reticulocyte, and prothrombin time) and serum chemistry (aspartate aminotransferase, alanine aminotransferase, alkaline phosphatase, blood urea nitrogen, creatinine, glucose, cholesterol, triglycerides, total protein, albumin, uric acid, total bilirubin, sodium, potassium, chloride, calcium, and inorganic phosphorus) parameters, organ weights (adrenal glands, brain, epididymides, heart, kidneys, liver, ovaries, pituitary gland, prostate gland and seminal vesicles, spleen, testes, thymus, thyroid/parathyroid glands, and uterus with cervix), and histopathology examination of major tissues and organs in control and 60 mg/kg/day groups (primary and recovery groups) and the adrenal glands from males and kidneys from females in the 5 and 17 mg/kg/day groups. Additionally, sperm counts and sperm mobility and vaginal cytology and estrus cycles were evaluated.
Significant decreases in body weight gain were observed at 60 mg/kg/day in males starting at week 1 and in females starting at week 6. Terminal body weights were 15.2 and 5.6% less than controls, with only the males being significantly different from controls. At the end of the recovery period, the 60 mg/kg/day males weighed significantly less (9.5%) than controls. Decreases in food consumption were observed on numerous occasions in the males exposed to 60 mg/kg/day; a decrease in food conversion efficiency was also observed in this group. No significant or treatment-related alterations in hematological or serum chemistry parameters were observed. Significant decreases in absolute brain, liver, heart, spleen, and pituitary weights were observed in males exposed to 60 mg/kg/day; however, there were no significant alterations in relative organ weights. Treatment-related histopathological alterations were limited to a slight diffuse hyperplasia in the renal proximal tubules in 2/10 females in the 60 mg/kg/day group; the investigators considered it to be treatment-related because it is an uncommon finding at this age. No significant alterations in vaginal cytology or estrus cycles were observed. Similarly, no significant alterations in spermatid or sperm counts or sperm morphology were observed in males. A slight decrease in sperm motility was observed at 60 mg/kg/day; however, this was likely attributable to the control group having a value that approached the upper limit among historical controls and was not considered treatment related. No alterations in reproductive organ weights or histological alterations were observed.
Selection of the Point of Departure for the MRL: The NOAEL of 17 mg molybdenum/kg/day was selected as the POD for the MRL. BMD modeling was not considered because a response was only observed at the highest dose tested. A dataset exhibiting a response only at the highest dose level would likely provide limited information regarding the shape of a dose-response curve.
Calculations: The investigators estimated doses using body weight and food consumption data.
Intermittent Exposure: Not applicable.
Uncertainty Factor and Modifying Factor: The NOAEL is divided by a total uncertainty factor (UF) of 100 and a modifying factor (MF) of 3
- 10 UF for extrapolation from animals to humans
- 10 UF for human variability
- 3 MF for concern that reproductive and/or developmental effects may be a more sensitive endpoint than kidney effects in populations with marginal copper intakes. The copper content of the Murray et al. (2014b, 2019) reproductive/developmental studies used a commercial diet with a fairly high copper content. In contrast, the Fungwe et al. (1990) study, which reported reproductive effects, utilized a diet that was slightly higher than the dietary requirement. The differences in the copper contents of the diet may explain differences between the study results.
- MRL = NOAEL ÷ (UFs × MF)
- 0.06 mg molybdenum/kg/day = 17 mg molybdenum/kg/day ÷ ((10 × 10) × 3)
Other Additional Studies or Pertinent Information that Lend Support to this MRL: Selection of the POD is supported by the Bompart et al. (1990) study, which found decreases in kidney function in rats administered sodium molybdate.
The MRL is calculated based on the assumption of healthy dietary levels of molybdenum and copper and represents the level of exposure above and beyond the normal diet.
Agency Contacts (Chemical Managers): G. Daniel Todd
MINIMAL RISK LEVEL (MRL) WORKSHEET
Download PDF (59K)
Chemical Name: | Molybdenum |
CAS Numbers: | 7439-98-7 |
Date: | May 2020 |
Profile Status: | Final |
Route: | Oral |
Duration: | Chronic |
MRL Summary: There are insufficient data for derivation of a chronic-duration oral MRL for molybdenum. The only available experimental study was considered a low-quality study that was not considered a suitable basis for an MRL.
Rationale for Not Deriving an MRL: Data on the chronic toxicity of molybdenum come from several population-based studies; most of these studies looked for associations between background exposure to molybdenum and adverse health outcomes. No laboratory animal studies were identified.
Koval’skiy et al. (1961) found increases in blood uric acid and symptoms of gout in residents living in Armenia with high levels of molybdenum in the soil and food; the investigators estimated that the residents were exposed to 10–15 mg/day (0.14–0.21 mg/kg/day). A series of small studies of residents living in areas of Colorado with high levels of molybdenum in the drinking water did not find significant increases in uric acid levels; one study estimated that molybdenum intake was 500 μg/day (0.007 mg/kg/day) (EPA 1979). Other studies have found significant associations between serum or urinary molybdenum levels and the severity of complications from diabetes (Rodriguez Flores et al. 2011), high blood pressure (Yorita Christensen 2013), semen quality (Meeker et al. 2008), testosterone levels (Meeker et al. 2010), and psychomotor index in infants (molybdenum levels were measured in the mothers) (Vazques-Salas et al. 2014). However, none of these studies established causality, and the molybdenum levels accounted for only a small percentage of the variance.
Although the Koval’sky et al. (1961) study provided an estimated dose, the study was not considered suitable for derivation of a chronic-duration oral MRL for molybdenum. The study has a number of deficiencies that limit the interpretation of the results: (1) the control group consisted of 5 individuals compared to 52 subjects in the exposed group; (2) no information was provided on the controls to assess whether they were matched to the exposed group; (3) it does not appear that the study controlled for potential confounders, such as diet and alcohol, which can increase uric acid levels; and (4) NAS (2001) noted that there were potential analytical problems with the measurement of serum and urine copper levels.
Agency Contacts (Chemical Managers): G. Daniel Todd
- ATSDR MINIMAL RISK LEVEL WORKSHEETS - Toxicological Profile for MolybdenumATSDR MINIMAL RISK LEVEL WORKSHEETS - Toxicological Profile for Molybdenum
Your browsing activity is empty.
Activity recording is turned off.
See more...