NCBI Bookshelf. A service of the National Library of Medicine, National Institutes of Health.
Interaction Profile for: Lead, Manganese, Zinc, and Copper. Atlanta (GA): Agency for Toxic Substances and Disease Registry (US); 2004 May.
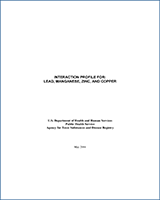
Interaction Profile for: Lead, Manganese, Zinc, and Copper.
Show detailsA.1. Toxicokinetics
Gastrointestinal absorption of soluble lead salts in adult humans can be high during fasting (40–50%), but is about 3–15% when ingested with food. On the basis of dietary balance studies, gastrointestinal absorption of lead in children appears to be higher and may account for 40–50% of the ingested dose. Studies in animals also provide evidence that gastrointestinal absorption of lead is much higher in younger organisms. Absorption is strongly affected by nutritional status, with higher absorption of lead in children who are iron deficient. Calcium deficiency also may increase lead absorption, based on studies in children. Coadministration of calcium with lead decreases lead absorption in adults, and in animal studies. Vitamin D administration has been shown to enhance lead absorption in animal studies. The distribution of lead appears similar across routes of exposure. Initially, lead is distributed to the blood plasma and soft tissues, but under steady state conditions 99% of the lead in blood is found in the erythrocyte, where much of it is bound to hemoglobin. Lead accumulates in blood, such that bone lead accounts for approximately 73% of the body burden in children, increasing to 94% in adults. Inorganic lead is not known to be metabolized, but lead ions are complete by macromolecules. Unabsorbed lead is excreted in the feces; absorbed lead that is not retained is excreted through the urine and bile (ATSDR 1999).
A.2. Health Effects
The effects of lead are similar across inhalation and oral routes of exposure. Lead has been shown to affect virtually every organ and system in the body in both humans and animals. The most sensitive effects of lead appear to be neurological (particularly in children), hematological, and cardiovascular. Epidemiological studies provide evidence for an association between prenatal and postnatal exposure to lead and adverse effects on neurodevelopment in infants and young children, and support the use of PbB as an index of toxicological effect. The neurological effects included impaired cognitive ability and IQ deficits in children. On the basis of several meta-analyses, it appears that a highly significant IQ decrement of 1–3 points is associated with a change in PbB from 10 to 20 μg/dL. In addition, associations between biomarkers of lead exposure and increased problem behavior in the classroom have been reported (ATSDR 1999; Marlowe et al. 1985). In adult humans, slowing of nerve conduction velocity occurs at PbBs of ≥30 μg/dL; peripheral nerve function appears to be affected in children at similar PbBs. Oral studies in animals support the human evidence regarding neurobehavioral toxicity of lead to infants and children from prenatal and postnatal exposure. In animals, lead has been shown to alter a number of neurotransmitter systems including dopamine, norepinephrine, serotonin, and gamma-aminobutyric acid systems (ATSDR 1999).
Lead interferes with the synthesis of heme, resulting in accumulation of ALA in tissues and elevated excretion of ALA in urine, elevation of zinc protoporphyrin in erythrocyte, reductions in blood hemoglobin, and in a hypochromic, normocytic anemia at higher levels of exposure. Many epidemiological studies have found increases in blood pressure to be associated with increases in PbB. The contribution of lead, as compared with other factors, is relatively small, and whether the observed associations represent causality is controversial. Animal data demonstrate that oral exposure to lead increases blood pressure. At higher levels of exposure in humans, lead produces cardiac lesions and electrocardiographic abnormalities. Chronic nephropathy in humans is associated with PbB levels of 40–>100 μg/dL. Oral exposure of animals to lead causes renal damage; histopathology is similar in humans and animals and includes intranuclear inclusion bodies, swollen mitochondria, and tubular damage. Adverse effects on the testes and sperm have been seen in occupationally exposed men with PbBs of 40–50 μg/dL, and the more recent literature suggest that PbB concentrations <40 μg/dL also may be associated with adverse effects on sperm counts and morphology (ATSDR 1999).
A.3. Mechanisms of Action
Lead can affect virtually every organ or system in the body through mechanisms that involve fundamental biochemical processes. These mechanisms include the ability of lead to inhibit or mimic the action of calcium and to interact with proteins. In the interaction with proteins, lead binds with virtually every available functional group, including sulfhydryl, amine, phosphate, and carboxyl groups, with sulfhydryl having the highest affinity. In its binding with sulfhydryl groups, lead may interfere with the activity of zinc metalloenzymes, as zinc binds to a sulfhydryl group at the active site. Lead also binds to metallothionein, a sulfhydryl-rich protein, but does not appear to displace cadmium or zinc. Metallothionein is induced by cadmium, zinc, and arsenic, but apparently not by lead, although metallothionein sequesters lead in the cell. Another lead-binding protein is an acidic, carboxyl-rich protein found in the kidney and brain (ATSDR 1999).
Lead interferes with heme synthesis by altering the activity of several mitochondrial and cytosolic enzymes. One of the most sensitive hematological effects is inhibition of the cytosolic enzyme ALAD, with no threshold apparent through the lowest PbB levels (≈3 μg/dL). Lead’s inhibition of ALAD occurs through binding of lead to vicinal sulfhydryls at the active site of ALAD, where zinc is normally bound to a single sulfhydryl. Lead stimulates the mitochondrial enzyme ALAS, through feedback derepression, with a threshold in human leukocytes at a PbB of about 40 μg/dL. As a result of the inhibition of ALAD and stimulation of ALAS, ALA accumulates in blood, urine, and soft tissues, including brain. ALA is structurally similar to GABA, an inhibitory neurotransmitter. ALA appears to act as a GABA agonist at the presynaptic GABA receptors, causing negative-feedback inhibition of GABA release. In addition, ALA undergoes autooxidation, generating free radicals that may contribute to toxicity, and ALA promotes oxyhemoglobin oxidation. At relatively high levels of lead exposure, anemia may occur due to the interference with heme synthesis and also to red cell destruction. Decreases in tissue heme pools can have deleterious effects throughout the body, not only because heme is a constituent of hemoglobin, but also because heme is a prosthetic group of cytochrome P450 and the cytochromes of cellular energetics (ATSDR 1999; EPA 1986). Lead inhibits the insertion of iron into protoporphyrin by the mitochondrial enzyme ferrochelatase, possibly through binding of lead to the sulfhydryl groups of the active site or indirectly through disruption of mitochondrial structure. Inhibition of ferrochelatase results in elevation of zinc protoporphyrin (ZPP) in erythrocytes; ZPP is a sensitive indicator of lead exposure, occurring in children at PbBs of about 25 μg/dL. Effects on heme synthesis are not restricted to the erythrocyte. A number of studies suggest that lead-impaired heme production itself may be a factor in lead's neurotoxicity (ATSDR 1999). Other potential mechanisms of neurotoxicity include lead acting as a calcium agonist in a number of processes (ATSDR 1999), and lead inhibition of receptor binding to the NMDA receptor channel, which does not appear to occur at the zinc allosteric site and is relatively insensitive (Lasley and Gilbert 1999).
Mechanisms by which lead might affect blood pressure include effects on several hormonal and neural regulatory systems, changes in vascular smooth muscle reactivity, cardiac muscle contractility, changes in cell membrane cation transport systems, and possible effects on vascular endothelial cells (ATSDR 1999).
Lead has been shown to interfere with the DNA binding properties of zinc-finger regions of transcription factors, and this interference could potentially elicit multiple responses, but consequences have not yet been defined (Zawia et al. 2000).
A.4. Health Guidelines
ATSDR (1999) has not derived MRLs for lead. ATSDR (1999) has suggested the use media-specific slope factors and site-specific environmental monitoring data to predict media-specific contributions to blood lead. The predicted contributions from the individual media are summed to yield a total predicted PbB level. The media-specific slope factors were derived from regression analysis of lead concentrations in water, soil, dust, diet, or air and PbBs for various populations.
The CDC determined in 1991 that blood lead levels of >10 μg/dL are to be considered elevated (ATSDR 1999; CDC 1991).
EPA (IRIS 2001) has not developed a reference concentration (RfC) or RfD for lead. EPA stated that it would be inappropriate to develop an RfD for inorganic lead (and lead compounds) because some of the health effects occur at PbBs so low as to be essentially without a threshold. Instead, EPA defines lead risk as the probability of exceeding a PbB of concern (i.e., 10 μg/dL) in children (EPA 1994a) or in fetuses (EPA 1996). This approach is supported by human epidemiological studies that have associated PbBs exceeding 10 μg/dL with impairment or delays in neurobehavioral development and other effects on children (e.g., blood enzymes). EPA estimates lead risk in children using the Integrated Exposure Uptake Biokinetic (IEUBK) model (EPA 1994b). This model translates estimates of site-specific exposure concentrations into estimates of the probability that children’s blood leads will exceed a PbB of concern.
The National Toxicology Program (NTP 2001) has determined that lead acetate and lead phosphate can reasonably be anticipated to be human carcinogens, based on sufficient evidence of carcinogenicity in experimental animals. NTP (2001) considered lead chromate as one of the “Chromium Hexavalent Compounds.” The International Agency for Research on Cancer (IARC 1987) has determined that the animal data are sufficient to classify lead and some lead compounds as possibly carcinogenic to humans (Group 2B). EPA (IRIS 2001) classified lead in Group B2—probable human carcinogen. EPA did not develop an oral slope factor for lead because of the many uncertainties, some of which may be unique to lead. An EPA inhalation unit risk also is not available for lead (IRIS 2001). American Conference of Governmental Industrial Hygienists (ACGIH 2001) classified lead and certain inorganic lead compounds as A3 carcinogens—confirmed animal carcinogen with unknown relevance to humans. Lead chromate, assessed on the basis of both lead and chromate, was classified by ACGIH (2001) as an A2 carcinogen— carcinogenic in animals at doses considered relevant to worker exposure, but with insufficient epidemiological data to confirm risk to humans.
A.5. Derivation of Target-Organ Toxicity Dose (TTD) Values
TTDs for chronic oral exposure to lead were derived for endpoints affected by lead and one or more of the other chemicals in the lead, manganese, zinc, and copper mixture that is the subject of this Interaction Profile. The relevant endpoints for lead and this mixture include neurological and hematological. Relevant endpoints for another metal mixture also included renal, cardiovascular, and testicular. The TTDs derived for those endpoints are retained in this Appendix, but are not relevant for the present mixture. Chronic oral TTDs for lead are derived below, using the methods described in ATSDR (2001a, 2001b). Because ATSDR’s approach to the assessment of lead uses media-specific slope factors and site-specific contributions to PbB, the TTDs for lead are derived based on PbB as well (see rationale in Chapter 3 of this profile). The derivations are based on data provided in ATSDR (1999), and particularly Section 2.2.1 (Effects in Humans Based on Blood Lead (PbB) Levels), Section 2.5 (Relevance to Public Health), and Section 2.7 (Biomarkers of Exposure and Effect). The derivation methods used similar reasoning as for the CDC and EPA levels of concern (see neurological effects).
Neurological Effects
A large number of epidemiological studies and case reports indicate that exposure to lead causes neurological effects. Slowing of nerve conduction velocity is associated with PbBs of ≥30 μg/dL in children and adults. Of greater concern are the inverse linear relationships between IQ and other neurobehavioral measures in children at PbBs extending down through 10 μg/dL or possibly lower. Children appear to be more sensitive to the neurobehavioral toxicity of lead than are adults. Limited data suggest an association between decreased neurobehavioral performance and PbB in aging subjects at relatively low PbBs, indicating that the elderly may be another sensitive population. Although results of the epidemiological studies in children are not entirely consistent, several meta-analyses have indicated that a highly significant IQ decrement of 1–3 points is associated with a change in PbB from 10 to 20 μg/dL in children (IPCS 1995; Needleman and Gatsonis 1990; Pocock et al. 1994; Schwartz 1994). The CDC (1991) determined that blood lead levels of >10 μg/dL are to be considered elevated in children, based largely on concern for the effects of low-level lead exposure on the central nervous system. EPA defines lead risk as the probability of exceeding a PbB of concern (10 μg/dL) in children or fetuses (EPA 1994a, 1996). The CDC level of concern for lead of 10 μg/dL is adopted as the TTD for neurological effects (TTDNEURO).
Renal Effects
Chronic nephropathy is associated with PbB levels of 40–>100 μg/dL in humans exposed to lead occupationally. There are some indications of renal damage in a study of children whose mean PbB was 34.2 μg/dL (increased –acetyl-β-D-glucosaminidase activity in urine, a sensitive indicator) (Verberk et al. 1996). The value for children, supported by the occupational data, and rounded to 34 μg/dL, is taken as the TTD for renal effects (TTDRENAL).
Cardiovascular Effects
At higher levels of exposure, lead produces cardiac lesions and electrocardiographic abnormalities in humans. Many epidemiological studies have reported an association between increases in blood pressure and increases in PbB. The contribution of lead, as compared with other factors, is relatively small, and whether the associations indicate causality is controversial. Animal data demonstrate that oral exposure to lead increases blood pressure ATSDR (1999). The correlation between PbB and blood pressure is apparent at relatively low PbBs extending through 10 μg/dL (e.g., Schwartz 1995). Therefore, the CDC level of concern, 10 μg/dL, is adopted as the TTD for cardiovascular effects (TTDCARDIO).
Hematological Effects
Lead interferes with the synthesis of heme. The consequence at higher levels of exposure is a hypochromic, normocytic anemia. The most sensitive indicator of effect on heme synthesis is the inhibition of ALAD. ALAD activity is inversely correlated with PbB through the lowest levels of PbB in the general population. Even in the absence of detectable effects on hemoglobin levels, there is concern that effects on heme synthesis may have far-reach impacts, particularly on children (ATSDR 1999). Accordingly, the CDC PbB of concern, 10 μg/dL (CDC 1991), is selected as the TTD for hematological effects (TTDHEMATO).
Testicular Effects
Adverse effects of the testes and sperm have been reported in occupationally exposed men with PbBs of 40–50 μg/dL in some studies, but not in others, and are well-established at higher levels of exposure (PbBs ≥66 μg/dL) (ATSDR 1999). The point of departure for increased risk of below normal sperm and total sperm count was 40 μg/dL (Alexander et al. 1996). This value is selected as the TTD for testicular effects (TTDTESTIC).
Summary (TTDs for Lead)
TTDNEURO = 10 μg/dL PbB = CDC level of concern
TTDRENAL = 34 μg/dL PbB
TTDCARDIO = 10 μg/dL PbB
TTDHEMATO = 10 μg/dL PbB
TTDTESTIC = 40 μg/dL PbB
A.6. References
- ACGIH. 2001. 2001 TLVs and BEIs. Threshold limit values for chemical substances and physical agents. Biological exposure indices. American Conference of Governmental Industrial Hygienists, Cincinnati, OH.
- Alexander BH, Checkoway H, van Netten C, et al 1996. Semen quality of men employed at a lead smelter. Occup Environ Med 53:411–416. (As cited in ATSDR 1999). [PMC free article: PMC1128498] [PubMed: 8758037]
- ATSDR. 1999. Toxicological profile for lead. Atlanta, GA: Agency for Toxic Substances and Disease Registry.
- ATSDR. 2001a. Guidance manual for the assessment of joint toxic action of chemical mixtures. Atlanta, GA: Agency for Toxic Substances and Disease Registry.
- ATSDR. 2001b. Guidance manual for the preparation of an interaction profile. Atlanta, GA. Agency for Toxic Substances and Disease Registry.
- CDC. 1991. Preventing lead poisoning in young children. Atlanta, GA: U.S. Department of Health and Human Services, Public Health Service, Centers for Disease Control and Prevention. (As cited in ATSDR 1999).
- EPA. 1986. Air quality criteria for lead. Research Triangle Park, NC: U.S. Environmental Protection Agency, Office of Research and Development, Office of Health and Environmental Assessment, Environmental Criteria and Assessment Office. EPA 600/8–83-028F, 12–34 to 12–37.
- EPA. 1994a. Guidance manual for the integrated exposure uptake biokinetic model for lead in children. U.S. Environmental Protection Agency. EPA/540/R-93/081. PB93–963510. (As cited in ATSDR 1999).
- EPA. 1994b. Technical support document: Parameters and equations used in integrated exposure uptake biokinetic model for lead in children. (v0.99d). U.S. Environmental Protection Agency. EPA/540/R-94/040. PB94–963505. (As cited in ATSDR 1999).
- EPA. 1996. Bioavailability of lead in soil samples from the Jasper County, Missouri superfund site. U.S. Environmental Protection Agency Region 8. Document Control No. 04800–030-0161. (As cited in ATSDR 1999).
- IARC. 1987. IARC monographs on the evaluation of carcinogenic risk to humans. International Agency for Research on Cancer, World Health Organization. Supplement 7, Vols 1 to 47.
- IPCS. 1995. Inorganic lead. Environmental Health Criteria 165 ed. Geneva: World Health Organization. International Programme on Chemical Safety. (As cited in ATSDR 1999).
- IRIS. 2001. Integrated Risk Information System. U.S. Environmental Protection Agency. Http://www
.epa.gov/iris/subst/index.htm. April 17, 2001. - Lasley SM and Gilbert ME. 1999. Lead inhibits the rat N-methyl-D-aspartate receptor channel by binding to a site distinct from the zinc allosteric site. Toxicol Appl Pharmacol 159:224–233. [PubMed: 10486309]
- Marlowe M, Cossairt A, Moon C, et al 1985. Main and interaction effects of metallic toxins on classroom behavior. J Abnorm Child Psychol 13(2):185–198. [PubMed: 4008752]
- Needleman HL, Gatsonis CA. 1990. Low-level lead exposure and the IQ of children: A meta-analysis of modern studies. J Am Med Assoc 263(5):673–678. [PubMed: 2136923]
- NTP. 2001. 9th report on carcinogens. Research Triangle Park, NC: U.S. Department of Health and Human Services. National Toxicology Program. http://ehis
.niehs.nih.gov/roc/toc9.html. September 11, 2001. - Pocock SJ, Smith M, Baghurst P. 1994. Environmental lead and children’s intelligence: A systematic review of the epidemiological evidence. Br Med J 309:1189–1197. (As cited in ATSDR 1999). [PMC free article: PMC2541690] [PubMed: 7987149]
- Schwartz J. 1994. Low-level lead exposure and children’s IQ: A meta-analysis and search for a threshold. Environ Res 65:42–55. (As cited in ATSDR 1999). [PubMed: 8162884]
- Schwartz J. 1995. Lead, blood pressure, and cardiovascular disease in men. Arch Environ Health 50:31–37. (As cited in ATSDR 1999). [PubMed: 7717767]
- Verberk MM, Willems TE, Verplanke AJ, et al 1996. Environmental lead and renal effects in children. Arch Environ Health 51(1):83–87. (As cited in ATSDR 1999). [PubMed: 8629869]
- Zawia NH, Crumpton T, Brydie M, et al 2000. Disruption of the zinc finger domain: A common target that underlies many of the effects of lead. Neurotoxicology 21(6):1069–1080. [PubMed: 11233753]
- Background Information for Lead - Interaction Profile for: Lead, Manganese, Zinc...Background Information for Lead - Interaction Profile for: Lead, Manganese, Zinc, and Copper
- Sphaerolaimus sp. voucher F26 YG-2022 small subunit ribosomal RNA gene, partial ...Sphaerolaimus sp. voucher F26 YG-2022 small subunit ribosomal RNA gene, partial sequencegi|2414522166|gb|OQ065317.1|Nucleotide
- Homo sapiens RNA pseudouridylate synthase domain containing 2, mRNA (cDNA clone ...Homo sapiens RNA pseudouridylate synthase domain containing 2, mRNA (cDNA clone MGC:21638 IMAGE:4420027), complete cdsgi|23271507|gb|BC016967.1|Nucleotide
Your browsing activity is empty.
Activity recording is turned off.
See more...