NCBI Bookshelf. A service of the National Library of Medicine, National Institutes of Health.
Toxicological Profile for Endosulfan. Atlanta (GA): Agency for Toxic Substances and Disease Registry (US); 2015 Aug.
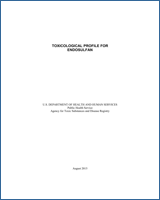
Toxicological Profile for Endosulfan.
Show details3.1. INTRODUCTION
The primary purpose of this chapter is to provide public health officials, physicians, toxicologists, and other interested individuals and groups with an overall perspective on the toxicology of endosulfan. It contains descriptions and evaluations of toxicological studies and epidemiological investigations and provides conclusions, where possible, on the relevance of toxicity and toxicokinetic data to public health.
A glossary and list of acronyms, abbreviations, and symbols can be found at the end of this profile.
Technical-grade endosulfan contains at least 94% α-endosulfan and β-endosulfan. The α- and β-isomers are present in the ratio of 7:3, respectively. The majority of the studies discussed below used technical-grade endosulfan. However, a few examined the effects of the pure α- and β-isomers. Endosulfan sulfate is a reaction product found in technical-grade endosulfan as a result of oxidation, biotransformation, or photolysis. There is very little difference in toxicity between endosulfan and its metabolite, endosulfan sulfate. However, the α-isomer has been shown to be about 3 times as toxic as the β-isomer of endosulfan.
3.2. DISCUSSION OF HEALTH EFFECTS BY ROUTE OF EXPOSURE
To help public health professionals and others address the needs of persons living or working near hazardous waste sites, the information in this section is organized first by route of exposure (inhalation, oral, and dermal) and then by health effect (death, systemic, immunological, neurological, reproductive, developmental, genotoxic, and carcinogenic effects). These data are discussed in terms of three exposure periods: acute (14 days or less), intermediate (15–364 days), and chronic (365 days or more).
Levels of significant exposure for each route and duration are presented in tables and illustrated in figures. The points in the figures showing no-observed-adverse-effect levels (NOAELs) or lowest-observed-adverse-effect levels (LOAELs) reflect the actual doses (levels of exposure) used in the studies. LOAELs have been classified into “less serious” or “serious” effects. “Serious” effects are those that evoke failure in a biological system and can lead to morbidity or mortality (e.g., acute respiratory distress or death). “Less serious” effects are those that are not expected to cause significant dysfunction or death, or those whose significance to the organism is not entirely clear. ATSDR acknowledges that a considerable amount of judgment may be required in establishing whether an end point should be classified as a NOAEL, “less serious” LOAEL, or “serious” LOAEL, and that in some cases, there will be insufficient data to decide whether the effect is indicative of significant dysfunction. However, the Agency has established guidelines and policies that are used to classify these end points. ATSDR believes that there is sufficient merit in this approach to warrant an attempt at distinguishing between “less serious” and “serious” effects. The distinction between “less serious” effects and “serious” effects is considered to be important because it helps the users of the profiles to identify levels of exposure at which major health effects start to appear. LOAELs or NOAELs should also help in determining whether or not the effects vary with dose and/or duration, and place into perspective the possible significance of these effects to human health.
The significance of the exposure levels shown in the Levels of Significant Exposure (LSE) tables and figures may differ depending on the user’s perspective. Public health officials and others concerned with appropriate actions to take at hazardous waste sites may want information on levels of exposure associated with more subtle effects in humans or animals (LOAELs) or exposure levels below which no adverse effects (NOAELs) have been observed. Estimates of levels posing minimal risk to humans (Minimal Risk Levels or MRLs) may be of interest to health professionals and citizens alike.
A User’s Guide has been provided at the end of this profile (see Appendix B). This guide should aid in the interpretation of the tables and figures for Levels of Significant Exposure and the MRLs.
3.2.1. Inhalation Exposure
Limited information is available regarding the effects of endosulfan in humans and animals after inhalation exposure. The reports of effects in humans are limited to case reports of adverse effects noted in workers exposed to large quantities of endosulfan during its manufacture. Exposures in these reports are likely to be a combination of inhalation and dermal exposures. Therefore, the findings from these case reports are also presented in the section on dermal exposures (Section 3.2.3).
3.2.1.1. Death
No studies were located regarding death in humans after inhalation exposure to endosulfan. LC50 (lethal concentration, 50% kill) values of 12.6 and 34.5 mg/m3 for female and male rats, respectively, were obtained after a 4-hour nose-only exposure to aerosolized endosulfan (Hoechst 1983a). No deaths were observed among male and female rats exposed to aerosolized endosulfan (nose-only) at concentrations as high as 2 mg/m3 for 6 hours/day, 5 days/week for a total of 21 out of 29 days (Hoechst 1984c). Acute LC50 values for male and female rats are recorded in Table 3-1 and plotted in Figure 3-1.
3.2.1.2. Systemic Effects
The highest NOAEL values and all reliable LOAEL values for systemic effects for each species and duration category are recorded in Table 3-1 and plotted in Figure 3-1.
Respiratory Effects. No studies were located regarding respiratory effects in humans after inhalation exposure to endosulfan.
Irregular respiration was observed in both male and female rats after a 4-hour nose-only inhalation exposure to aerosolized endosulfan (Hoechst 1983a). In both male and female rats, dyspnea was observed at the lowest concentrations tested (12.3 and 3.6 mg/m3 for males and females, respectively). Necropsy of the rats that died showed dark-red foci the size of a pinhead in the lungs. It is unclear whether these effects represent direct effects of inhaled endosulfan on respiratory tissues or whether they are secondary to central nervous system effects on respiratory function. No treatment-related effects were revealed by routine gross and microscopic examination of the nasal cavity, trachea, and lungs of male and female rats exposed (nose-only) to concentrations of endosulfan of up to 2 mg/m3 for 6 hours/day, 5 days/week for a total of 21 out of 29 days (Hoechst 1984c).
Cardiovascular Effects. No studies were located regarding cardiovascular effects in humans after inhalation exposure to endosulfan. Routine gross and microscopic examination of the heart and aorta of rats exposed (nose-only) to concentrations of up to 2 mg/m3 aerolized technical endosulfan for 6 hours/day, 5 days/week for a total of 21 out of 29 days revealed no treatment-related effects (Hoechst 1984c).
Gastrointestinal Effects. No studies were located regarding gastrointestinal effects in humans after inhalation exposure to endosulfan. Routine gross and microscopic examination of tissues of the gastrointestinal system (parotid and submandibular glands, esophagus, stomach, small and large intestines, and pancreas) revealed no treatment-related effects following exposure to aerosolized technical endosulfan for 6 hours/day, 5 days/week for a total of 21 out of 29 days at concentrations of up to 2 mg/m3 (Hoechst 1984c).
Table 3-1
Levels of Significant Exposure to Endosulfan - Inhalation.

Figure 3-1
Levels of Significant Exposure to Endosulfan - Inhalation.
Hematological Effects. No studies were located regarding hematological effects in humans after inhalation exposure to endosulfan.
Routine gross and microscopic examination of hematopoietic organs (spleen and bone marrow) and routine hematological analyses did not reveal any effects of nose-only exposure of rats to concentrations of technical endosulfan of up to 2 mg/m3 for 6 hours/day, 5 days/week for a total of 21 out of 29 days (Hoechst 1984c).
Musculoskeletal Effects. No studies were located regarding musculoskeletal effects in humans after inhalation exposure to endosulfan.
Routine gross and microscopic examination of skeletal muscle and the diaphragm revealed no treatment-related effects following nose-only inhalation exposure of rats to concentrations of up to 2 mg/m3 aerolized technical endosulfan for 6 hours/day, 5 days/week for a total of 21 out of 29 days (Hoechst 1984c).
Hepatic Effects. No studies were located regarding hepatic effects in humans after inhalation exposure to endosulfan.
Routine gross and microscopic examination of the liver did not reveal any effects of nose-only exposure of rats to concentrations of up to 2 mg/m3 aerolized technical endosulfan for 6 hours/day, 5 days/week for a total of 21 out of 29 days (Hoechst 1984c).
Renal Effects. No studies were located regarding renal effects in humans after inhalation exposure to endosulfan.
Routine gross and microscopic examination of the kidneys and urinary bladder did not reveal any effects of nose-only exposure of rats to concentrations of up to 2 mg/m3 aerolized technical endosulfan for 6 hours/day, 5 days/week for a total of 21 out of 29 days (Hoechst 1984c).
Endocrine Effects. No studies were located regarding endocrine effects in humans after inhalation exposure to endosulfan.
Routine gross and microscopic examination of the pituitary gland did not reveal any effects in rats exposed nose-only to concentrations of up to 2 mg/m3 aerolized technical endosulfan for 6 hours/day, 5 days/week for a total of 21 out of 29 days (Hoechst 1984c).
Dermal Effects. No studies were located regarding dermal effects in humans after inhalation exposure to endosulfan.
Routine gross and microscopic examination of the skin did not reveal any effects of nose-only exposure of rats to concentrations of up to 2 mg/m3 aerolized technical endosulfan for 6 hours/day, 5 days/week for a total of 21 out of 29 days (Hoechst 1984c).
Ocular Effects. No studies were located regarding ocular effects in humans after inhalation exposure to endosulfan.
Routine gross and microscopic examination of the eyes did not reveal any effects of nose-only exposure of rats to concentrations of up to 2 mg/m3 aerolized technical endosulfan for 6 hours/day, 5 days/week for a total of 21 out of 29 days (Hoechst 1984c).
Body Weight Effects. No studies were located regarding body weight effects in humans after inhalation exposure to endosulfan.
On day 20 of exposure, body weight gain slightly reduced (<10%) in male, but not female, rats exposed nose-only to concentrations of up to 2 mg/m3 aerolized technical endosulfan for 6 hours/day, 5 days/week for a total of 21 out of 29 days (Hoechst 1984c). No significant effect was seen at an exposure level of 1 mg/m3. The body weight reduction was associated with a marked reduction in food consumption at that time.
Metabolic Effects. No studies were located regarding metabolic effects in humans after inhalation exposure to endosulfan.
Nose-only exposure of rats to concentrations of up to 2 mg/m3 aerolized technical endosulfan for 6 hours/day, 5 days/week for a total of 21 out of 29 days had no significant effect on serum electrolytes or glucose levels (Hoechst 1984c).
3.2.1.3. Immunological and Lymphoreticular Effects
No studies were located regarding immunological effects in humans after inhalation exposure to endosulfan.
Routine gross and microscopic examination of the lymph nodes, thymus, and spleen did not reveal any effects of nose-only exposure of rats to concentrations of up to 2 mg/m3 aerolized technical endosulfan for 6 hours/day, 5 days/week for a total of 21 out of 29 days (Hoechst 1984c). No studies directly assessing immunologic function were located.
3.2.1.4. Neurological Effects
Neurotoxicity is the primary effect observed in humans following occupational exposure to endosulfan. Convulsions were reported in nine individuals exposed to the endosulfan-containing insecticide, Thiodan®, during bagging (Ely et al. 1967). Other effects noted in at least one of the subjects prior to the onset of convulsions included malaise, nausea, vomiting, dizziness, confusion, and/or weakness. In addition, a case of long-term, possibly permanent brain damage in an industrial worker was attributed by Aleksandrowicz (1979) to endosulfan exposure. This worker was exposed by cleaning vats that contained residues of endosulfan solution. The acute phase of the poisoning was manifested by repeated convulsions and impaired consciousness. After recovery from the repeated seizure episode, the patient became disoriented and agitated. Two years later, he exhibited cognitive and emotional deterioration, memory impairment, and impairment of visual-motor coordination manifested by an inability to perform small tasks. However, modest alcohol consumption (1 L of wine/week) may have been a contributing factor. Limitations associated with these reports include lack of quantitative exposure data, lack of data on the duration of exposure, the possibility of multiple routes of exposure (i.e., oral and dermal, as well as inhalation), and possible concurrent exposure to other chemicals. Therefore, this information can only provide qualitative evidence of neurotoxicity associated with inhalation exposure to endosulfan in humans.
Evidence of neurotoxicity was also observed in animal studies. Nose-only exposure of rats to aerosolized technical endosulfan at concentrations of 3.6 mg/m3 in females and 12.3 mg/m3 in males resulted in trembling and ataxia (Hoechst 1983a). At higher concentrations, tremors, tonic-clonic convulsions, and reduced corneal, pupillary, placing, shock, paw-pinch, and cutaneous reflexes were observed in both sexes. Nose-only exposure of male and female rats to concentrations of up to 2 mg/m3 aerosolized technical endosulfan for 6 hours/day, 5 days/week for a total of 21 out of 29 days resulted in no observed behavioral disturbances (Hoechst 1984c). In addition, routine gross and histopathologic examination of the cerebrum, cerebellum, brain stem, optic nerve, and pituitary demonstrated no treatment-related abnormalities.
3.2.1.5. Reproductive Effects
No studies were located regarding reproductive effects in humans following inhalation exposure to endosulfan.
No studies were located that examined reproductive function in animals after inhalation exposure to endosulfan. However, routine gross and histopathological examination of the reproductive organs (testes, epididymides, seminal vesicles, prostate, ovaries, and uterus) of rats exposed (nose-only) to up to 2 mg/m3 aerosolized technical endosulfan for 6 hours/day, 5 days/week for a total of 21 out of 29 days revealed no adverse effects (Hoechst 1984c).
3.2.1.6. Developmental Effects
No studies were located regarding developmental effects in animals following inhalation exposure to endosulfan.
Roberts et al. (2007) evaluated the hypothesis that maternal residence near agricultural pesticide applications during key periods of gestation could be associated with the development of autism spectrum disorders (ASD) in children. The study population included 269,746 singletons born between 1 January 1996 and 31 December 1998 to mothers residing in 19 counties in California. The final analyses were conducted on 465 cases and 6,975 controls. To exclude associations likely due to multiple testing error, the investigators employed a staged analytical design applying a priori criteria to the results of conditional logistic regressions. A total of 249 combinations of compounds, buffer radii, and temporal periods met a pre-established requirement of five exposed cases and controls per cell. Of these, four that described applications of dicofol and endosulfan that occurred during the period immediately before and concurrent with central nervous system embryogenesis met a priori criteria and were unlikely to be a result of multiple testing. Multivariate a posteriori models comparing children of mothers living within 500 m of field sites with the highest non-zero quartile of organochlorine poundage to those with mothers not living near field sites suggested an odds ratio (OR) for ASD of 6.1 (95% confidence interval [CI] 2.4–15.3). ASD risk increased with the poundage of pesticide applied and decreased with distance from field sites. According to the investigators, strengths of the study included the ability to locate pesticide applications with relatively high resolution in both space and time, which allowed them to test hypotheses referring to specific temporal periods of vulnerability. The primary limitation was thought to be possible misclassification of exposure. Also, since little information was available regarding the mothers and children other than basic demographic characteristics, the investigators were unable to adjust for confounders potentially important for gestational neurodevelopment, such as the use of prenatal vitamins. Finally, the investigators could not dismiss that women in the “exposed” categories may have been disproportionally employed in agriculture and, therefore, subject to occupational exposures to pesticides beyond drift concentrations.
3.2.1.7. Cancer
No studies were located regarding cancer in animals after inhalation exposure to endosulfan.
In a case-control study of the relation between occupational exposures to various suspected estrogenic chemicals and the occurrence of breast cancer among 261 cases and 753 controls, the breast cancer OR was not elevated above unity (OR=0.8; 95% CI 0.2–3.2) for occupational exposure to endosulfan compared to unexposed controls (Aschengrau et al. 1998). However, the sample sizes were very small (three exposed; seven not exposed), and co-exposure to other unreported chemicals also reportedly occurred. Both of these factors may have contributed to the high degree of uncertainty in the OR indicated by the wide confidence interval. Rau et al. (2012) examined the possible association between levels of endosulfan in the bone marrow from children and acute hematological malignancies. The investigators also assessed whether children with high levels of endosulfan in their marrow resided in areas sprayed with endosulfan. The cohort consisted of 26 children with proven hematological malignancy and 26 age-matched controls with benign hematological disease. The major illness among the cases was acute lymphoblastic leukemia (n=23), whereas immune thrombocytopenic purpura occurred in 50% of controls (n=13). Six out of 26 cases tested positive for endosulfan in the bone marrow compared to 1 out of 26 controls; the concentration of endosulfan in the bone marrow was not provided. All of the children with elevated endosulfan in the bone marrow resided in the areas sprayed with the pesticide. The small sample size and the lack of control for potential confounders make this report of questionable value.
3.2.2. Oral Exposure
3.2.2.1. Death
Acute accidental or intentional ingestion of large amounts of endosulfan has resulted in death in humans. Five cases of acute lethal poisoning in humans resulting from ingestion of Thiodan® were reported by Terziev et al. (1974). In two cases of suicide, the ingested dose was reported to be up to 100 mL of Thiodan® (concentration of endosulfan in this particular formulation was not specified); in the other three poisonings, the victims drank liquids containing the pesticide, but the ingested doses were not specified. Initial clinical symptoms of endosulfan poisoning included gagging, vomiting, diarrhea, agitation, writhing, loss of consciousness, cyanosis, dyspnea, foaming at the mouth, and noisy breathing. Autopsies performed in three out of five cases revealed edema of the brain and lungs, hemorrhage of the medullary layer of the kidneys, acute lung emphysema, and chromatolysis of the neurons. Two cases of lethal ingestion of endosulfan-containing formulations were reported by Demeter and Heyndrickx (1978). In one, a 40-year-old man who consumed Posidor (20% endosulfan and 30% dimethoate in xylene) and alcohol died within 3 hours. His body was dark-red/purple, and his face was cyanotic. Autopsy revealed edematous lungs. The authors suggested that death was due to the combined effects of dimethoate (an organophosphate insecticide compound and potent cholinesterase inhibitor) and endosulfan. In the other case, a 28-year-old man ingested a fatal dose of Thiodan® powder (20% endosulfan) in conjunction with alcohol. Postmortem findings included congested and edematous lungs. Death was due to asphyxiation, which the authors suggested was caused by a synergistic effect of alcohol and endosulfan. In neither case was the ingested dose of endosulfan quantified. In the case of a 55-year-old female who died following intentional ingestion of an unspecified amount of an endosulfan formulation containing 35% active ingredient dispersed in a colorless liquid containing 55% xylene, autopsy revealed no gross anatomical or histological abnormalities attributed to endosulfan (Bernardelli and Gennari 1987). According to the investigators, the presence of malignant melanoma and the concurrent ingestion of xylene may have been contributing factors in the death of this woman.
A lethal case of a woman who ingested an unknown amount of endosulfan mistakenly added to food was reported (Blanco-Coronado et al. 1992). One to 4 hours after ingestion, she had tonic-clonic convulsions, nausea, vomiting, headache, and dizziness. On admission to the hospital, the concentrations of endosulfan (both isomers) in the gastric contents, blood, and urine were 55.4, 2.9, and 3 mg/L, respectively. She died 8 days after admission to the hospital following acute renal failure, disseminated intravascular coagulation, thrombi in the pulmonary arteries and aorta, and cardiogenic shock. Postmortem finding included bilateral pleural effusions, congested and edematous lungs with exudative areas and pulmonary edema, hyaline membranes, microatelectasia, polymorphonuclear lymphocytes and red cells in the alveoli, and interstitial fibrosis. A similar lethal case of a man who died 10 days after ingesting an unknown amount of endosulfan was described by Lo et al. (1995). The cause of death was described as cardio-respiratory arrest and heart failure and pulmonary edema. None of these case reports provide sufficient data to estimate a lethal dose of endosulfan in humans.
An estimated oral dose of 260 mg endosulfan/kg (the endosulfan formulation was not specified) caused severe seizures in a 43-year-old man, and brain death from cerebral herniation and massive cerebral edema occurred within 4 days of exposure (Boereboom et al. 1998); there were no signs of myocardial infarction and only slight congestion of the heart, but pulmonary congestion and atelectasis were evident at autopsy. Eyer et al. (2004) described the case of man who intentionally drank 500 mL of Thiodan® 35 containing 180 g of endosulfan (approximately 2.6 g/kg) who presented with repetitive seizures on admission. The patient did not respond to treatment and died from multiorgan failure on day 10. Twenty-five hours after ingestion, the concentration of endosulfan in serum was approximately 0. 8 mg/L; a terminal half-life in blood of 15.2 hours was estimated for endosulfan. Post-mortem examination conducted 1 day after death showed severe brain edema. Parbhu et al. (2009) reported the case of a 2.5-year-old male who ingested an unknown amount of an 11.6% solution of endosulfan and immediately developed generalized tonic-clonic seizure activity, became unresponsive, and died on day 3. Moon and Chun (2009) reported that 16 out of 52 patients admitted to an emergency facility with acute endosulfan poisoning died. Refractory status epilepticus was the most common cause of death. The amount ingested being >35 g of endosulfan was the independent variable that best predicted patient mortality. Moses and Peter (2010) reported 18.75% mortality among 16 patients admitted to a hospital with documented endosulfan exposure in India.
Signs of acute lethal endosulfan poisoning in animals are similar to those observed in humans and include hyperexcitability, dyspnea, decreased respiration, and fine tremors followed by tonic-clonic convulsions. Oral LD50 values for technical-grade endosulfan vary depending on species, sex, formulation tested, and nutritional status of the animal (Gupta and Gupta 1979; WHO 1984). With regard to species sensitivity, mice appear to be quite sensitive to endosulfan’s lethal effects, with a reported LD50 value of 7.36 mg/kg in males (Gupta et al. 1981) and 2 out of 10 male mice dying after administration of 7.5 mg technical endosulfan/kg in the diet for 7 days (Wilson and LeBlanc 1998). In contrast, LD50 values in male rats range between 40 and 121 mg/kg (Boyd and Dobos 1969; Boyd et al. 1970; Hoechst 1990; Lindquist and Dahm 1957). A single oral dose of 20 mg/kg of technical endosulfan killed 3 out of 14 male rats within hours of dosing, following seizure activity (Gilbert and Mack 1995). In a 60-day study, 8 of 19 male rats died following gavage dosing with 7.5 mg technical endosulfan/kg/day (Ansari et al. 1984). An LD50 value of 76.7 mg/kg technical endosulfan has been calculated from the results of a study in dogs (sex and breed not indicated) (Hoechst 1970). However, technical endosulfan causes vomiting in dogs, and one study found that all dogs died that did not vomit after ingesting doses of at least 30 mg/kg (FMC 1958). Thus, the value of the dog LD50 may reflect both the dose and whether or not the dogs vomited.
In rats, exposed males and females appear to have different sensitivities to the lethal effects of endosulfan exposure. Summary data submitted by Hoechst (1990) from experiments conducted mainly with technical endosulfan showed that female LD50 values ranged between 10 and 23 mg/kg, whereas male LD50 values ranged between 40 and 125 mg/kg. Thus, female rats appear to be 4–5 times more sensitive to the lethal effects of endosulfan than male rats. This difference may be related to differences in the toxicokinetics of endosulfan in male and female rats (see also Section 3.4). Insufficient data were available to determine whether differences in sensitivity to lethal effects exist between males and females of species other than the rat.
The effects of protein deficiency on endosulfan toxicity were studied in Wistar rats (Boyd and Dobos 1969; Boyd et al. 1970). Rats fed a diet totally deficient in protein for 28 days prior to administration of a single oral dose of technical endosulfan had an LD50 of 5.1 mg/kg of endosulfan. Rats fed a low-protein diet (3.5% protein) for 28 days had an LD50 of 24 mg/kg of endosulfan. Rats fed standard laboratory chow (26% protein) had an LD50 of 102–121 mg/kg. The immediate cause of death in all animals was respiratory failure following tonic-clonic convulsions. This study demonstrated that, while a protein-deficient diet does not affect the nature of the toxic reaction, it may affect the sensitivity of rats to the lethal effects of endosulfan.
The two isomers of endosulfan (α-and β-) also have different LD50 values in rats. The α-isomer is more toxic than the β-isomer in female rats, with an oral LD50 value of 76 mg/kg versus an LD50 value of 240 mg/kg for β-endosulfan (Hoechst 1975, 1990; Maier-Bode 1968). The same difference was reported in female albino mice; the lethal doses were 11 mg/kg versus 36 mg/kg for α-endosulfan and β-endosulfan, respectively (Dorough et al. 1978). The lethal dose for endosulfan sulfate in mice was comparable to that of the α-isomer, 8 mg/kg (Dorough et al. 1978). Hoechst (1966a, 1966b) reported an LD50 of 14 mg/kg for α-endosulfan and 17 mg/kg for β-endosulfan in female mice.
In rats, daily administration of 5 or 10 mg/kg doses of technical endosulfan by gavage in corn oil during GDs 6–14 produced a dose-related increase in maternal deaths in these test groups (Gupta et al. 1978).
In intermediate-duration studies, rats tolerated 6-day/week gavage doses of 20 mg/kg technical endosulfan for 7 weeks (Garg et al. 1980), whereas increased mortality was observed in male and female mice at doses of 7.3 and 7.52 mg/kg/day, respectively, for 13 weeks (Hoechst 1984b). Two out of 19 rats administered 7.5 mg technical endosulfan/kg/day died in a 60-day study (Ansari et al. 1984). A study also found female rats to be more sensitive than males, since 3 out 10 females died during a 30-day feeding study, but no deaths occurred in male groups (Paul et al. 1995). An additional intermediate-duration study reported that 4 out of 15 male rats (females not tested) died after administration of 10 mg technical endosulfan/kg, 3 times a week for 4–5 weeks (Gilbert 1992). Three out of nine rabbits administered 1.5 mg/kg/day technical endosulfan and five out nine rabbits administered 3 mg/kg/day died in a 30-day gavage study (Hatipoglu et al. 2008). No deaths occurred in rabbits administered 0.75 mg/kg/day. Clinical signs observed in some of the rabbits included hyperexcitability, dyspnea, hyperpnea, tremors and convulsions, depression, forelimb extension, loss of mobility, and eventually death.
Increased mortality was observed in both male rats (at doses of 20.4 mg/kg/day and above) and male mice (at doses of 0.46 mg/kg/day and above) in a 2-year bioassay with technical endosulfan conducted by the National Cancer Institute (NCI 1978). The authors attributed the excessive mortality in the male rats to treatment-related toxic nephropathy. The high mortality in male mice was possibly due to fighting since no other treatment-related cause for the deaths could be determined. Survival in females of both species was unaffected by endosulfan (NCI 1978). However, survival was significantly decreased in female rats that consumed 5 mg/kg/day technical endosulfan for 2 years (FMC 1959b) and in female mice that consumed approximately 2.9 mg technical endosulfan/kg/day for 2 years (Hack et al. 1995; Hoechst 1988b). In these studies, survival in male rats was not affected at 5 mg/kg/day for 2 years (FMC 1959b) and survival in male mice was not affected at 2.5 mg/kg/day for 2 years (Hoechst 1988b).
All reliable LD50 and LOAEL values for death in each species and duration category are recorded in Table 3-2 and plotted in Figure 3-2. In some studies, only the α- or β-isomer of endosulfan was tested. In such cases, a notation regarding the specific isomer tested is included in the effect description.
Table 3-2
Levels of Significant Exposure to Endosulfan - Oral.

Figure 3-2
Levels of Significant Exposure to Endosulfan - Oral.
3.2.2.2. Systemic Effects
Case reports of human poisonings and studies in animals indicate that during acute oral exposure to lethal or near-lethal amounts of endosulfan, involvement of a large number of organ systems (respiratory, cardiovascular, gastrointestinal, hematological, hepatic, and renal) is observed. It cannot be rule out that some systemic effects observed at dose levels that induce frank neurological effects such as tremors and seizures are secondary to the neurological effects. No long-term targets have been identified in humans largely because no studies of humans chronically exposed to endosulfan have been conducted. However, during longer-term exposure, the kidney appears to be the primary systemic target organs in rats.
The highest NOAEL values and all reliable LOAEL values for systemic effects for each species and duration category are recorded in Table 3-2 and plotted in Figure 3-2. In some studies, only the α- or β-isomer of endosulfan was tested. In such cases, a notation regarding the specific isomer tested is included in the effect description.
Respiratory Effects. Respiratory effects have been observed in cases of lethal poisonings from intentional or accidental ingestion of large quantities of endosulfan. Cyanosis, dyspnea, foaming at the mouth, and noisy breathing have been observed in several subjects prior to death (Blanco-Coronado et al. 1992; Terziev et al. 1974). It should be noted that these signs and symptoms may also be secondary to endosulfan-induced cardiac failure and/or to aspiration of the solvent. At autopsy, acute emphysema and/or congested and edematous lungs were frequently observed in persons following ingestion of lethal quantities of endosulfan (Blanco-Coronado et al. 1992; Boereboom et al. 1998; Demeter and Heyndrickx 1978; Lo et al. 1995; Terziev et al. 1974). Respiratory effects were also part of the clinical syndrome displayed by a 20-year-old man who attempted suicide by ingesting 200 mL of a 30% endosulfan formulation (Thionax®) (Shemesh et al. 1988). Although the man was given activated charcoal to limit gastrointestinal absorption during the first 16 hours following ingestion and his stomach was pumped, hypoxia (due to alveolar hypoventilation and pulmonary edema) was evident. In the following 2 weeks, the patient had recurrent aspiration pneumonia and a persistent need for mechanical ventilation. Although it is possible that these respiratory effects were due, in part, to a direct action of endosulfan on the lungs, it is not unlikely that some of the observed effects were caused by aspiration, if the patient was lavaged without the airways being controlled (Eddelston et al. 2007). It is unclear whether other ingredients in the Thionax® contributed to the effects observed. Respiratory effects were also reported in other nonlethal cases of acute intoxication with endosulfan. Pulmonary infiltrate was reported in three out of four cases 4 hours after ingesting an unknown amount of endosulfan, and four of these five cases required mechanical ventilation (Blanco-Coronado et al. 1992). Boereboom et al. (1998) observed minor lung hemorrhaging and atelectasis at autopsy of a man who ingested approximately 260 mg/kg endosulfan 4 days prior to death.
Similar to the data from humans, respiratory effects have been observed in animals almost exclusively in acute, lethal-dose exposure situations. Studies in which a limited number of dogs were given single oral doses of technical endosulfan as low as 10 mg/kg (FMC 1958) or 50 mg/kg (Hoechst 1970) demonstrated respiratory paralysis and death. Autopsy of the dogs revealed congestion of the lungs. It is not clear whether these effects were a result of direct action on the lungs or were associated with the generalized convulsions. In another study in which female rats were given a single gavage dose of β-endosulfan, lung congestion was observed at 70 mg/kg, but not at 63 mg/kg (Hoechst 1988a). These dose levels caused lethality in the rats.
Local inflammation of the lungs and dilated alveoli were observed in rats administered 10 mg/kg/day of technical endosulfan in peanut oil by gavage for 15 days (Gupta and Chandra 1977). However, there was high mortality in this dose group (three of eight animals died prior to study termination), and it is not clear if these effects were observed primarily in the intercurrent deaths or in animals surviving for the full 15 days of exposure. Intraparenchymal hemorrhages, edema, hyperemia, and degeneration of alveolar pneumocytes were reported in rabbits following administration of ≥1.5 mg/kg/day technical endosulfan by gavage in corn oil for 30 days (Hatipoglu et al. 2008). These dose levels also caused lethality in the rabbits. The NOAEL was 0.75 mg/kg/day.
With the exception of the effects reported by Hoechst (1988a) in female rats, no effects on respiratory tissues were observed during gross and histopathological examinations in intermediate- and chronic-duration studies in rats, mice, or dogs at sublethal doses of technical endosulfan (FMC 1959b, 1967; Hack et al. 1995; Hoechst 1984b, 1988b, 1989a, 1989c; NCI 1978).
Cardiovascular Effects. Cardiovascular effects were part of the clinical syndrome displayed by a 20-year-old man who attempted suicide by ingesting 200 mL of a 30% endosulfan formulation (Thionax®) (Shemesh et al. 1988). Although the man’s stomach contents were aspirated and he was given activated charcoal to limit absorption during the first 16 hours following ingestion, episodes of tachycardia and hypertension occurred, followed by cardiogenic shock. It is not clear whether these cardiovascular effects were due to a direct action of endosulfan on the cardiovascular system or a result of a more general toxic insult (e.g., convulsions). It is also unclear whether other ingredients in the Thionax® may have contributed to the effects observed. A similar picture was described in another lethal case of acute intoxication with endosulfan (Lo et al. 1995). Severe cardiovascular effects developed in a woman who ingested an unknown amount of endosulfan mistakenly mixed into food (Blanco-Coronado et al. 1992). On admission to the hospital, she had transient hypotension (60/30 mm Hg). Over the next few days, her hemodynamic parameters remained abnormal, and she died 8 days after admission following acute renal failure, disseminated intravascular congestion, thrombi in the pulmonary arteries and aorta, and cardiogenic shock. A man who ingested approximately 260 mg/kg endosulfan experienced a drop in arterial blood pressure on the day of exposure, and focal cardiac inflammation and “slight” heart congestion on autopsy 4 days after exposure (Boereboom et al. 1998). Persistent tachycardia and severe hypotension were described in a patient who ingested 35 mL of an organochlorine fluid containing a total of 12.3 g endosulfan (Eyer et al. 2004). Hypotension and abnormalities in the EKG were observed in a study of 52 cases of acute poisoning with endosulfan (Moon and Chun 2009).
Administration of 2 mg/kg/day (only dose level tested) endosulfan by gavage in corn oil to male Wistar rats for 28–42 days induced myocardial fiber edema and congestion and degeneration (Kalender et al. 2004a; Jalili et al. 2007) (Kalender et al. [2004a] used technical endosulfan; the formulation used by Jalili et al. [2007] was not specified). Similar observations were reported in pregnant Wistar rats after administration of 1 mg/kg/day (only dose level tested) technical endosulfan by gavage in olive oil on GDs 6–20 and sacrificed on GD 20 (Singh et al. 2007b). Alva et al. (2012) reported decrease activity of antioxidant enzymes and increased lipid peroxidation in homogenates of heart tissue from rats dosed by gavage with ≥0.5 mg/kg/day technical endosulfan for 21 days; however, no microscopic examination of the heart was conducted.
In general, longer-term exposure of animals to sublethal concentrations of technical endosulfan has not resulted in gross or microscopic evidence of cardiovascular toxicity (FMC 1967; Hack et al. 1995; Hoechst 1984b, 1988b, 1989c). However, two rat studies indicated possible toxic effects. Also, male rats that consumed 2.9 mg/kg/day for 2 years had an increased incidence of aneurysms in blood vessels (Hoechst 1989a). Female rats were not similarly affected at doses up to 3.8 mg/kg/day for 2 years (Hoechst 1989a). In light of the large number of negative studies that used similar doses of endosulfan, the biological significance of the isolated observations of blood vessel aneurysms is unknown. An additional chronic study in rats that used larger doses of technical endosulfan (20 and 48 mg/kg/day) reported calcification of the heart and the aorta and mesenteric arteries in male rats (NCI 1978). The calcification was thought to be caused by parathyroid hyperplasia, which in turn was secondary to kidney disease.
Gastrointestinal Effects. Nausea, gagging, vomiting, and diarrhea were part of the clinical syndrome exhibited by persons who consumed high doses (lethal in some cases) of endosulfan either intentionally or accidentally (Blanco-Coronado et al. 1992; Eyer et al. 2004; Karatas et al. 2006; Pradhan et al. 1997; Terziev et al. 1974; Yavuz et al. 2007). However, it is unclear whether these effects were the result of gastrointestinal irritation or were mediated by effects of endosulfan on central nervous system control of gastrointestinal function. Mucosal inflammation of the stomach and the proximal small intestinal were postmortem observations in a man who purposely ingested an unknown amount of endosulfan (Lo et al. 1995). In contrast, a man who ingested endosulfan once at approximately 260 mg/kg did not show any apparent stomach or intestinal lesions at autopsy 4 days later (Boereboom et al. 1998).
Female rats that received a single dose of 63 mg of β-endosulfan/kg by gavage, a dose that was lethal, had blood in the small intestines and mucus in the stomach (Hoechst 1988a). Studies in dogs indicate that acute exposure to relatively high doses of endosulfan may cause stomach irritation and vomiting. Dogs given a single oral dose of 30 mg/kg of technical endosulfan exhibited vomiting and stomach irritation (FMC 1958). Following a single oral dose of 50 mg/kg of technical endosulfan, dogs had congestion in the stomach and small intestine (Hoechst 1970). Similarly, rats given single unspecified doses of technical endosulfan in an LD50 determination showed irritant gastroenteritis (Boyd et al. 1970). Rabbits treated with a single dose of 15.1 mg/kg of a formulation of endosulfan containing 35% of active ingredient had watery diarrhea for 3–4 days after dosing, but eventually recovered (Ceron et al. 1995); this dose level was lethal to some of the treated rabbits. Increased goblet cell activity, blunted villi, and lymphoid depletion were reported in the intestines from female Wistar rats following administration of 1 mg/kg/day (only dose level tested) technical endosulfan on GDs 6–20 and sacrificed on GD 20 (Singh et al. 2007b).
Longer-term exposure of animals to sublethal doses of technical endosulfan has generally not resulted in observable signs of gastrointestinal toxicity. Routine gross and histopathological examination of the gastrointestinal tract revealed no adverse effects in rats, mice, or dogs in such studies (FMC 1959b, 1967; Hack et al. 1995; Hoechst 1984b, 1988b, 1989a, 1989c; NCI 1978). However, convulsive spasms of the abdominal and jaw muscles without vomiting were observed in male and female dogs that consumed 2.0 and 1.8 mg/kg/day, respectively, for 1 year (Hoechst 1989c). No adverse gross or histopathological findings were noted following examination of the gastrointestinal tracts of these animals, indicating that the spasms may have been a neurological effect, rather than the result of gastrointestinal irritation.
Hematological Effects. Leukocytosis and decreased platelet counts were reported in a group of subjects shortly after they ingested an unknown amount of endosulfan (Blanco-Coronado et al. 1992). One subject from that study, who eventually died, had prolonged partial thromboplastin time and prothrombin time with thrombocytopenia, and decreased fibrinogen 2 days after being admitted to the hospital. Elevated white cell count was also observed in an additional case of fatal acute poisoning with endosulfan (Lo et al. 1995). Significantly elevated hemoglobin (61.2 g/100 mL compared to a reference range of 13–18 g/100 mL) and slightly elevated white cell count (12,600/mm3 compared to a reference range of 5000–10,000/mm3), but normal hematocrit, were seen in a male patient at approximately 40 minutes after ingesting 260 mg endosulfan/kg; the man subsequently died (Boereboom et al. 1998). Moon and Chun (2009) noted thrombocytopenia in 11 (21%) of their patients, but Karatas et al. (2006) reported normal complete blood counts in their study of 23 cases of endosulfan intoxication. No further information was located regarding hematological effects in humans after oral exposure to endosulfan.
Limited information is available regarding hematological effects in animals following acute oral exposure to endosulfan. Female Wistar rats treated with a single gavage dose of ≥22 mg/kg of an unspecified endosulfan formulation had decreased hemoglobin at sacrifice 24 hours after dosing; a group treated with a dose of 33 mg/kg showed decreases in red blood cells, hemoglobin, and packed cell volume (Siddiqui et al. 1987b). It should be noted that the resulting values were still within normal limits. In another study, male New Zealand rabbits administered a single gavage dose of 15.1 mg/kg of an endosulfan formulation with 35% active ingredient also showed a decrease in red blood cells, hemoglobin, and packed cell volume; this dose was lethal to five out of seven rabbits (Ceron et al. 1995). Neither one of these studies has been included in Table 3-2, the Siddiqui et al. (1987b) study because it did not specify the type of endosulfan used and the Ceron et al. (1995) study for using a formulation with 35% active ingredient.
Mixed results have been obtained in studies examining longer-term exposures to endosulfan. Adverse hematological effects were observed in a well-conducted study in which rats were administered technical endosulfan in the diet for 13 weeks (Hoechst 1985a). At 6 weeks, effects observed in male rats that consumed 1.9 mg/kg/day included decreases in hemoglobin, red blood cell count, and mean corpuscular hemoglobin concentration, and an increase in mean corpuscular volume. Decreased mean corpuscular hemoglobin concentration was observed in female rats at a similar dose. At higher doses in this study, the magnitude of the effects increased, and effects comparable to those observed in males were observed in females. At 13 weeks, males exhibited decreased hemoglobin concentration at ≥3.8 mg/kg/day, whereas decreased hemoglobin was seen in females at 0.8 mg/kg/day. Following a 4-week withdrawal period, spleen weights were significantly increased in males at ≥1.9 mg/kg/day. Microcytic hypochromic anemia, thrombocytopenia, and leukocytosis were also reported in another study in rats administered 2 mg/kg/day (only dose tested) technical endosulfan by gavage for 4 weeks (Mahran 2013). It should be noted that hematological determinations performed in other intermediate- and chronic-duration studies in rats using doses comparable to those noted above do not support the ability of technical endosulfan to cause anemia (Dikshith et al. 1984; FMC 1959b; Hack et al. 1995; Hoechst 1989a). Also, no significant effects on hematological parameters or on routine gross and histopathological examination of bone marrow and the spleen were observed in mice, dogs, or rabbits during intermediate- and chronic-duration studies with technical endosulfan (FMC 1967; Hatipoglu et al. 2008; Hoechst 1984b, 1988b, 1989c), and in a more recent study in rats (Sheets et al. 2004).
The hematological effects reported in the Hoechst (1985a) and Mahran (2013) studies cannot be totally discounted as spurious. A possible explanation for the discrepancy between the findings in these two studies and the other studies noted above may be provided by the results of the study by Das and Garg (1981). These authors found decreased red blood cells in rats reared on a low-protein diet (3.5% protein) that also provided doses of 0.025 and 5 mg/kg/day of an unspecified formulation of endosulfan for 9–18 weeks. However, no effect was observed at these doses in rats given normal protein diets prior to exposure, indicating that protein deficiency enhances the anemia-inducing capacity of endosulfan. Thus, some subtle stressor may have affected the response of rats in the study by Hoechst (1985a) such that they responded similarly to rats that consumed a protein-deficient diet.
Musculoskeletal Effects. Rhabdomyolysis (destruction of skeletal muscle fibers) was described in 31 out of 52 cases of acute endosulfan poisoning reported by Moon and Chun (2009). Limited information was obtained regarding effects of endosulfan on muscle and/or bone in animals. Routine gross and microscopic examination of samples of bone and/or muscle obtained from animals in intermediate-duration (Hoechst 1984b, 1989c; Sheets et al. 2004) and chronic-duration (FMC 1967; Hoechst 1988b, 1989a, 1989c; NCI 1978) studies revealed no adverse effects of technical endosulfan on these tissues.
Hepatic Effects. Elevated serum alanine aminotransferase (ALT) and aspartate aminotransferase (AST) activities were reported in a woman 2 days after being admitted to the hospital because of ingestion of endosulfan-contaminated food (Blanco-Coronado et al. 1992). The patient died 8 days after admission, following acute renal failure, disseminated intravascular coagulation, thrombi in the pulmonary arteries and aorta, and cardiogenic shock. Postmortem examination revealed dilation and congestion of hepatic sinusoids. Centrilobular congestion and slight prominence of the bile canaliculi were among postmortem observations in an additional fatal case of acute poisoning with endosulfan (Lo et al. 1995). A man who ingested approximately 260 mg/endosulfan/kg showed liver congestion on autopsy 4 days after exposure (Boereboom et al. 1998). Elevated transaminases were also reported in 4 out of 16 cases of acute endosulfan poisoning described by Moses and Peter (2010). Moon and Chun (2009) reported that 36.5% of their patients showed hepatic toxicity (ALT activity >100 U/L). The ALT levels started at more than 100 U/L at 1–4 days and reached their highest levels (median 346 U/L, range 123–807 U/L) at 1–8 days after ingestion. The liver function test results returned to normal within 18 days after ingestion in the survival group. In contrast, the patients in the nonsurvival group continued to show ALT levels >100 U/L. In their study of 23 accidental poisoning cases, Karatas et al. (2006) reported that on day 2, three patients still exhibited abnormal liver function in the form of elevated serum AST and ALT. In two of these patients, enzyme levels returned to normal at the end of the 3rd and 10th day, respectively. In the third patient, AST and ALT increased gradually and the patient developed hepatic encephalopathy and was transferred for liver transplant on day 5. No further information was located regarding hepatic effects in humans after oral exposure to endosulfan.
Studies in experimental animals indicate that both toxic effects and adaptive effects may be seen in the liver following oral exposure to endosulfan.
Necropsy of dogs that ingested single lethal doses of technical endosulfan (10 mg/kg, FMC 1958; 50 mg/kg, Hoechst 1970) revealed liver congestion, possibly secondary to endosulfan-induced right heart failure rather than through a direct effect. Similarly, autopsied rats that received unspecified doses of technical endosulfan in an LD50 study were reported to have liver congestion (Boyd et al. 1970). Rats receiving a single oral dose of 33 mg/kg of an unspecified formulation of endosulfan had increased serum ALT activity, indicating hepatic damage (Siddiqui et al. 1987b). Rabbits that were administered a single gavage dose of 15.1 mg/kg of an endosulfan formulation containing 35% active ingredient, which was lethal to 5 out of 7 rabbits, had significantly increased serum alkaline phosphatase, ALT, and AST activities (suggesting liver damage) in the days following treatment (Ceron et al. 1995); no histopathology was conducted in this study. These observations are consistent with findings in humans acutely exposed to high doses of endosulfan.
Adaptive effects (including increased microsomal enzyme activity, increased liver weight, increased smooth endoplasmic reticulum, and decreased pentobarbital-induced sleeping time) in the absence of any signs of toxicity have also been observed in female rats in acute-duration studies at doses of 2.5 mg/kg/day technical endosulfan for 7 days (Gupta and Gupta 1977a) and in male rats at doses of 5 mg/kg/day (unspecified formulation) for 2 days (Misra et al. 1980) or 10 mg/kg/day technical endosulfan for 14 days (Den Tonkelaar and Van Esch 1974).
Increased liver weight has also been observed in several intermediate-duration studies. For example, increased liver weight has been observed in female rats exposed to 2.5 mg/kg/day technical endosulfan for 15 days (Gupta and Gupta 1977a). In male rats, increased liver weight was observed at doses of 5 mg/kg/day after 15 days (Gupta and Chandra 1977) or 30 days (Dikshith et al. 1984). Doses of 3 mg technical endosulfan/kg/day in the food for 30 days significantly increased serum and liver alkaline phosphatase, AST, and ALT activities in female rats, but not in males (Paul et al. 1995); these effects were seen in the males at a dose level of 6 mg/kg/day. The seemingly greater toxicity in the females was attributed to differences in metabolism between males and females. Similar results regarding ALT and alkaline phosphatase were reported in rats dosed with 2 mg/kg/day (only level tested) technical endosulfan for 4 weeks (Mahran 2013). Evidence of microsomal enzyme induction (decreased pentobarbital-induced sleeping time) was also observed in female rats at 2.5 mg/kg/day for 30 days (Gupta and Gupta 1977a). In general, increases in liver weight have not been accompanied by adverse histopathological changes (Dikshith et al. 1984); however, exposure of male rats to 5 mg/kg/day for 15 days was reported to result in moderate dilation of the sinusoids, areas of focal necrosis, Kupffer cell hyperplasia, and bile duct proliferation with more severe necrosis, inflammation, and dilation at 10 mg/kg/day, a dose that was lethal to three out of eight rats (Gupta and Chandra 1977). Also, increased serum alkaline phosphatase was observed in male and female rats in the study by Dikshith et al. (1984). Treatment of Sprague-Dawley rats for 15 days with 10 mg/kg/day (only level tested) technical endosulfan by gavage increased serum ALT (50%), decreased serum alkaline phosphatase, and increased bilirubin (3–4-fold (Choudhary et al. 2003). Endosulfan induced liver hypertrophy and histopathological changes including dilation of sinusoidal spaces and abnormalities in the nuclei of the hepatocytes. In general, changes after 30 days of treatment were more severe than after 15 days of treatment (Choudhary et al. 2003).
Studies also have reported biochemical changes such as decreased activity of antioxidant enzymes and increased lipid peroxidation in homogenates of liver tissue from rats dosed with ≥0.5 mg/kg/day technical endosulfan for periods ≥21 days (Alva et al. 2012; Mahran 2013).
Treatment of pregnant Wistar rats with 1 mg/kg/day (only dose tested) technical endosulfan by gavage in oil on GDs 6–20 and sacrificed on GD 20 revealed degenerative changes in the liver, karyomegaly, engorged sinusoids, vacuolar degeneration, and double nuclei (Singh et al. 2007b). Treatment of rabbits with 1.5 mg/kg/day technical endosulfan by gavage in oil for 30 days increased the weight of the liver 21% (Hatipoglu et al. 2008). Serum activities of alkaline phosphatase and AST were significantly increased in all treated groups (0.75, 1.5, and 3 mg/kg/day), but serum ALT was not. Microscopic examination of the liver showed alteration mainly in the mid- and high-dose groups consisting of hydropic and granular degeneration and fatty changes in hepatocytes. Other lesions included mononuclear inflammatory cell infiltrations in the portal tract, destruction of portal epithelium, and focal parenchymal lymphocytic cell infiltration with degenerative changes. Since only a qualitative description of these findings was provided, these results are not listed in Table 3-2. The results from these studies suggest that both adaptive and toxic effects may be observed in some intermediate-duration studies.
In studies of somewhat longer duration, effects on liver weight were not observed or were observed only at high doses. Exposure of male rats to doses up to 3.85 mg/kg/day technical endosulfan for 13 weeks via the food had no effect on liver weight (Hoechst 1985a). Increases in liver weight were observed after 13 weeks only at doses of 23.41 mg/kg/day in males and 27.17 mg/kg/day in females (Hoechst 1985a). In this study, granular brown pigment was observed in livers of males at 23.41 mg/kg/day, and centrilobular enlargement was observed in livers of females at 27.17 mg/kg/day; however, these changes were no longer apparent following a 4-week “withdrawal” period during which the animals were no longer exposed to endosulfan. More recently, increased liver weight was reported in male and female rats exposed to ≥13.7 and ≥16.6 mg/kg/day technical endosulfan, respectively, for 13 weeks; however, this was attributed to deposition of endosulfan metabolites and to a tissue reaction (Sheets et al. 2004). Moreover, clinical chemistry tests did not provide any evidence of liver pathology. In mice, an increase in liver weight was observed in females exposed via the diet to 4.6 mg/kg/day but not in males at doses of 3.7 mg/kg/day for 42 days (Hoechst 1985b). No effect on mouse liver weight was observed at doses as high as 7.3 mg/kg/day (males) and 7.52 mg/kg/day (females) technical endosulfan for 13 weeks (Hoechst 1984b). In both mouse studies, no adverse histopathological findings were observed during routine microscopic examination of the livers. Similarly, no adverse histopathological findings were observed during routine microscopic examination of the livers of dogs exposed to TWA doses of 2.9 mg/kg/day (males) and 2.6 mg/kg/day (females) technical endosulfan for 146 days (Hoechst 1989c), but serum alkaline phosphatase was elevated in females treated with the 2.6 mg/kg/day dose.
Chronic-duration studies have generally not shown adaptive or adverse effects on the liver following administration of technical endosulfan. Routine gross and microscopic pathology has not revealed adverse hepatic effects in mice exposed to up to 2.5 mg/kg/day (males) or 2.9 mg/kg/day (females) for 2 years (Hack et al. 1995; Hoechst 1988b; NCI 1978), in rats exposed to ≤5 mg/kg/day (females) or 2.9 mg/kg/day (males) for 2 years (Hack et al. 1995; Hoechst 1989a), or in dogs exposed to 1 mg/kg/day for 2 years (FMC 1967). Serum alkaline phosphatase was, however, elevated in dogs exposed to 0.67 mg/kg/day (males) or 0.6 mg/kg/day (females) for 1 year, suggesting adverse effects on the liver; however, no effects on liver weight, liver function, or microscopic pathology were observed (Hoechst 1989c). An increase in the incidence of hydropic hepatic cells in the liver of male rats exposed to 5 mg/kg/day for 2 years (FMC 1959b) was also observed, indicating that hepatic toxicity may be observed in chronic studies when sufficiently high doses are administered.
Renal Effects. Hemorrhage of the medullary layer of the kidneys was reported in three persons who died following ingestion of endosulfan (Terziev et al. 1974). Acute renal failure was a major contributor to the deaths of two individuals who ingested unknown amounts of endosulfan (Blanco-Coronado et al. 1992; Lo et al. 1995). In both cases, postmortem examination revealed extensive tubular necrosis. In contrast, no kidney lesions were found in a man who died 4 days after ingesting approximately 260 mg endosulfan/kg (Boereboom et al. 1998). In their study of 52 cases of intoxication, Moon and Chun (2009) reported that 26.9% developed acute kidney injury, which resolved without sequelae in the patients who survived. Acute kidney injury is likely to be mostly due to rhabdomyolisis, which in turn, is due to uncontrolled seizures.
A study in mice dosed by gavage with 13 mg/kg/day technical endosulfan for 10 consecutive days reported that kidneys from treated mice showed prominent mitochondrial degeneration in proximal convoluted tubular cells (Caglar et al. 2003). There were also lipofuscin granules and membraneous structures in some of the cells. Some glomeruli also showed changes such as fusion in pedicels and focal thickening at glomerular basal membrane. Catalase activity in kidney tissue was significantly decreased, while the activities of glucose-6-phosphate dehydrogenase and superoxide dismutase were significantly increased, as were the levels of glutathione and malonaldehyde. The authors suggested that oxidative stress may have played a role in the alterations seen.
In intermediate-duration studies in male rats, congestion and focal degeneration in the epithelial lining of kidney tubules, and glomerulonephritis and glomerulonephrosis were observed in animals treated with doses of 10 mg/kg/day (only dose tested) technical endosulfan by gavage for 15–30 days (Choudhary et al. 2003; Gupta and Chandra 1977). No such effects were seen in rats treated with 5 mg/kg/day endosulfan in the Gupta and Chandra (1977) study. Choudhary et al. (2003) also reported an increase in serum urea (66%) and doubling of serum creatinine levels. Increased blood urea nitrogen (50%) and serum creatinine (100%) also were reported in rats following gavage administration of 2 mg/kg/day (only dose tested) technical endosulfan for 4 weeks (Mahran 2013). The latter study also reported decreases in the activities of antioxidant enzymes in homogenates of renal tissue. Administration of 1 mg/kg/day technical endosulfan (only dose level tested) by gavage in oil to pregnant rats on GDs 6–20 induced proximal convoluted epithelial cell degeneration, vacuolization, glomerular congestion, and glomerular monocyte infiltration (Singh et al. 2007b). Also, yellow protein aggregates in the lumen and eosinophilic droplets in the cells of some proximal convoluted tubules were observed in rats following consumption of a diet that provided 3.9 mg/kg/day of technical endosulfan for 13 weeks (Hoechst 1985a). At 23.4 mg/kg/day, males exhibited proteinuria (Hoechst 1985a). At lower doses, however, effects in rats have been limited to increases in kidney weight and changes in cellular pigmentation (Dikshith et al. 1984; FMC 1965; Hoechst 1984a, 1985a). Increases in relative kidney weight have been observed in male rats at doses with 6 mg/kg/day technical endosulfan for 84 days (Hoechst 1984a). Increases in yellow discoloration of the cytoplasm of cells of the proximal convoluted tubules have been observed following consumption of doses as low as 0.64 mg/kg/day for 13 weeks by male rats (Hoechst 1985a). Granular clumped pigment was also observed in cells of the straight portions and occasionally in the proximal convoluted tubules in male rats in this study at doses of 3.85 mg/kg/day at the end of 13 weeks of exposure and at doses of 1.92 mg/kg/day at the end of the 4-week withdrawal period. In mice, consumption of 7.3 mg/kg/day (males) or 7.52 mg/kg/day (females) for 13 weeks resulted in no gross or microscopically evident adverse effects (Hoechst 1984b). Similarly, in dogs given TWA doses of 2.9 mg/kg/day (males) or 2.6 mg/kg/day (females) for 146 days, routine gross and histopathological examination of the kidneys and urinary bladder revealed no adverse effects (Hoechst 1989c).
The toxicological relevance of the yellow discoloration of the cytoplasm of the cells of the proximal convoluted tubules and the increase in relative kidney weight that was observed in the study by Hoechst (1985a) was investigated in a subsequent study (Hoechst 1987) because toxicokinetic studies indicated that endosulfan accumulated in the kidneys of animals following intermediate-duration exposure (Ansari et al. 1984; Dorough et al. 1978; Nath and Dikshith 1979). Thus, the yellow discoloration and increase in kidney weight may have been merely a reflection of endosulfan storage within the cells of the proximal convoluted tubules rather than a toxic effect. Light and electron microscopy of the kidneys of rats that consumed 34 or 68 mg/kg/day for 4 weeks showed pigment deposits and an increase in the number and size of lysosomes in the cells of the proximal convoluted tubules (Hoechst 1987). These changes receded considerably during a 30-day recovery period, suggesting that at the doses and the exposure duration tested, endosulfan and/or metabolites accumulate in the kidney without causing detectable toxicity. Results of residue analysis indicated that endosulfan is stored only temporarily in the kidneys. In addition, only endosulfan-sulfate and endosulfan-lactone were detected in any appreciable quantities in the kidneys. Results similar to those of Hoechst (1987) were reported by Sheets et al. (2004), who reported increased kidney weight in rats dosed via the diet with ≥13.7 mg/kg/day technical endosulfan for 13 weeks; the increase in weight was attributed to the accumulation of an amorphous brown-to-yellow pigment.
Minor changes of questionable biological significance observed in rats administered 5 mg/kg/day of an unspecified endosulfan formulation in a low-protein diet for 9 weeks include a decrease in capsular space and an increase in perirenal adipose tissue (Das and Garg 1981).
Chronic ingestion of endosulfan by rats has been reported to result in nephrotoxicity. Consumption of technical-grade endosulfan by rats for 78 weeks (followed by a 33-week observation period) at TWA doses of 20 mg/kg/day (males) and 11.1 mg/kg/day (females) resulted in toxic nephropathy characterized by degenerative changes in the proximal convoluted tubules at the junction of the cortex and medulla (NCI 1978). Cloudy swelling, fatty degeneration, and necrosis of the tubular epithelium were also evident. Reuber (1981) re-analyzed the histological sections from the NCI study, and found that chronic renal fibrosis was evident in 100% of exposed male rats, and that there was a significantly increased incidence of female rats with acute necrosis of the tubules. Similar results were obtained at lower doses in male rats in studies by FMC (1959b) and Hoechst (1989a). At doses of 5 mg/kg/day for 2 years, an increase in kidney weight, renal tubule dilation, albuminous casts, focal interstitial nephritis, and degeneration of renal tubule epithelium were observed in male rats (FMC 1959b). Doses of approximately 3.8 mg/kg/day for 2 years significantly increased the incidence of marker progressive glomerulonephrosis in female rats (Hack et al. 1995; Hoechst 1989a). It should be noted that in male rats dosed with up to 2.9 mg/kg/day, the increase was less pronounced than in females, but the incidences in all groups, including controls, were considerably higher than in females (Hoechst 1989a). No evidence of renal toxicity was observed in female rats in these studies following consumption of doses of 5 mg/kg/day (FMC 1959b) or 3.8 mg/kg/day (Hack et al. 1995; Hoechst 1989a) for 2 years, other than enlarged kidneys. These results indicate that male rats are more susceptible to the renal toxicity of endosulfan than female rats.
In contrast to the effects seen in rats following chronic-duration exposure, mice and dogs have not shown any evidence of nephrotoxicity at the doses that have been tested. Ingestion by mice of doses of endosulfan of 2.5 mg/kg/day (males) and 2.9 mg/kg/day (females) for up to 2 years resulted in no grossly or microscopically evident adverse effects on the kidneys or urinary bladder (Hack et al. 1995; Hoechst 1988b; NCI 1978). Similarly, ingestion by dogs of doses as high as 2 mg/kg/day (males) and 1.8 mg/kg/day (females) for 1 year (Hoechst 1989c) or 1 mg/kg/day (males and females) for 2 years (FMC 1967) resulted in no evidence of nephrotoxicity. Thus, rats appear to be more sensitive to the nephrotoxic effect of endosulfan.
Endocrine Effects. A cross-sectional study of 303 men and 305 women from Brazil examined the association between exposure to organochlorine pesticides (endosulfan among them) and thyroid status (Freire et al. 2013). The cohort lived in an area where a factory had produced, formulated, and stored pesticides in the past. The factory was closed in 1961. A previous study had indicated that ingestion of local food and dermal absorption through contact with contaminated soil were the main routes of exposure to the pesticides. Blood collected from the participants showed that the prevalence of subclinical hyperthyroidism and the presence of anti-thyroperoxidase antibodies were higher than those reported elsewhere. Median levels of α- and β-endosulfan in men and women were 0.22–0.24 ng/mL. After adjusting for confounders, the results of analyses using multivariate regression models showed that total T3 levels were associated with lower concentrations of β-endosulfan in men and with higher β-endosulfan concentrations in women (associations also existed for other pesticides). No significant associations were seen between serum levels of endosulfan and serum free T4 or TSH. The main limitation of the study is its cross-sectional design, which prevents determining causality. The main strength is the relatively large population sample studied.
Hyperglycemia was reported in a study of six individuals after acute exposure to endosulfan (Blanco-Coronado et al. 1992). A bigger study of 52 subjects with endosulfan intoxication also reported high serum glucose levels on admission (Moon and Chun 2009). However, another study of 23 cases of endosulfan intoxication reported normal glucose in their patients (Karatas et al. 2006).
Administration by gavage of a single oral dose of 5 mg/kg of an unspecified formulation of endosulfan to rats resulted in degranulation of the β-cells of the islets of Langerhans of the pancreas (Barooah et al. 1980). This effect, however, was not observed after the same dose was administered daily for 5 days. Both administration protocols caused dilation of the blood vessels of the islets of Langerhans. Kalender et al. (2004b) administered 2 mg/kg/day (only dose tested) technical endosulfan by gavage in corn oil to male Wistar rats for 6 weeks and reported that after 2 weeks of treatment, B cells in islets of Langerhans in the pancreas showed some swollen mitochondria. After 3 or 4 weeks, there was some mitochondrial swelling and dissolution of mitochondrial matrix, and dilation of the endoplasmic reticulum. Some B cells in islet of Langerhans had weak picnotic nucleus at the end of the 5th week, and large and small vacuoles occurred in the cytoplasm. After 6 weeks of treatment, the number of secretory granules decreased and vacuoles produced by granules were increased. A similar study in male New Zealand rabbits administered 1 mg/kg/day (only dose tested) technical endosulfan for 6 weeks reported that endosulfan induced degenerative changes in the pancreas, especially in the B cells (Ozmen et al. 2010). Immunohistochemistry of the pancreas of treated rabbits showed marked reduction in concentration and distribution of insulin, proinsulin, and amylin. It also showed a marked decrease in proinsulin-, insulin-, and amylin-secreting cells and a slight decrease in glucagon-secreting cells. Administration of up to 5 mg/kg/day technical endosulfan/kg/day by gavage in corn oil for 7 days to rats did not significantly alter the weight of the adrenals (Gupta and Gupta 1977a). Administration also of 5 mg/kg/day (only dose tested) technical endosulfan to rats for 30 days decreased serum T3 by 16%, insulin by 43%, and cortisol by 10%, and increased serum T4 by 16% (Kumar et al. 2011).
Routine gross and/or microscopic examination of the adrenals, pituitary, thyroid, or parathyroid did not reveal any adverse effects following intermediate exposure of rats, mice, or dogs to doses ranging from 2.5 to 10 mg/kg/day technical endosulfan (FMC 1965; Gupta and Chandra, 1977; Hoechst 1984b, 1988b, 1989c). A similar lack of effects was reported in rats administered up to 5 mg endosulfan/kg/day for up to 2 years (FMC 1959b; Hoechst 1989a), dogs treated with up to 1 mg/kg/day for 2 years (FMC 1967) or mice administered 2.9 mg/kg/day for 2 years (Hoechst 1988b). Parathyroid hyperplasia and mineralization (calcium deposits) in several tissues were observed in male rats treated for 74–82 weeks, with estimated doses of 20 mg technical endosulfan/kg/day (NCI 1978). Both of these lesions were secondary to chronic renal failure (NCI 1978). Reuber (1981) re-evaluated the histological sections from the NCI study, and indicated that the incidence of rats with parathyroid hyperplasia was significantly increased at both treatment levels among males, but not among females.
Effects of endosulfan on sex hormones are summarized in Section 3.2.2.5, Reproductive Effects.
Dermal Effects. No studies were located regarding dermal effects in humans following oral exposure to endosulfan.
Only limited information was obtained regarding the effects of endosulfan on the skin in animals. Routine gross and microscopic examination of samples of skin obtained from dogs treated with 2.6 mg technical endosulfan/kg/day in the diet for 147 days revealed no adverse effects (Hoechst 1989c). Female, but not male, rats treated for 13 weeks with 4.6 mg endosulfan/kg/day in the diet exhibited hair loss in the dorsal scapular and cervical regions (Hoechst 1985a). Chronic treatment of rats, mice, or dogs with doses of approximately 2 mg/kg/day technical endosulfan caused no significant alterations in the skin (Hoechst 1988b, 1989a, 1989c; NCI 1978). Male rats dosed with approximately 48 mg/kg/day and females with approximately 22 mg/kg/day technical endosulfan in the diet for 78 weeks did not show treatment-related alterations in the skin (NCI 1978).
Ocular Effects. No studies were located regarding ocular effects in humans following oral exposure to endosulfan.
Only limited information was obtained regarding the effects of technical endosulfan on the eyes in animals. Routine gross and microscopic examination of samples of eyes obtained from rats, mice, and dogs in intermediate-duration (FMC 1965; Hoechst 1984b, 1985b, 1989c) and chronic-duration (Hoechst 1988b, 1989a, 1989c) studies revealed no adverse effects of endosulfan on these tissues. Also, ophthalmoscopy of the eyes revealed no treatment-related effects in rats that consumed doses of up to 23.41 mg/kg/day (males) and 27.17 mg/kg/day (females) for 13 weeks (Hoechst 1985a), 37.2 mg/kg/day (males) and 45.5 mg/kg/day (females) for 13 weeks (Sheets et al. 2004), or 2.9 mg/kg/day (males) and 3.8 mg/kg/day (females) for 2 years (Hack et al. 1995; Hoechst 1989a); in mice that consumed 3.7 mg/kg/day (males) and 4.6 mg/kg/day (females) for 42 days (Hoechst 1985b); or in dogs that consumed TWA doses of 2.9 mg/kg/day (males) and 2.6 mg/kg/day (females) for 146 days or 2 mg/kg/day (males) and 1.8 mg/kg/day (females) for 1 year (Hoechst 1989c).
Body Weight Effects. No studies were located regarding body weight effects in humans following oral exposure to endosulfan.
Administration by gavage of a single dose of up to 100 mg/kg technical endosulfan to male rats or 12 mg/kg to female rats did not significantly affect body weight during a 3-week observation period following dosing (Bury 1997). Body weight was not significantly affected in rats treated with up to 6 mg technical endosulfan/kg/day for 7–8 days (Gupta and Gupta 1977a; Lakshmana and Raju 1994) or in mice treated with up to 15 mg technical endosulfan/kg in the food for 7 days (Wilson and LeBlanc 1998). However, rabbits treated once by gavage with 15.1 mg/kg of a formulation of endosulfan containing only 35% active ingredient and followed for 35 days exhibited a 12% reduction in body weight (Ceron et al. 1995); the dose level was lethal in the rabbit study.
No significant effects on body weight were obtained in intermediate-duration studies with technical endosulfan in which rats were administered 5 mg/kg/day by gavage for 15 days (Gupta and Gupta 1977a), 4 mg/kg/day for 30 days (Hiremath and Kaliwal 2003), 1.5 mg/kg/day by gavage for 30 days (Dikshith et al. 1984), 5 mg/kg/day in the diet for 30 days (Paul et al. 1995), 10 mg/kg by gavage in oil 5 days/week for 90 days (Sinha et al. 1997), or up to 45.5 mg/kg/day for 13 via the diet (Sheets et al. 2004). However, in a study by Gupta and Chandra (1977), rats treated with 10 mg/kg/day technical endosulfan by gavage in oil for 15 days gained 30% less weight than controls; this dose also caused lethality. Also, administration of 1.5 mg/kg/day technical endosulfan to rats for 21 days resulted in a 12% reduction in final body weight relative to controls; doses of 1 mg/kg/day reduced body weight by 7% (Alva et al. 2012). In a study of gestational and lactational exposure of Sprague-Dawley rats, gavage doses of 6.12 mg/kg/day technical endosulfan reduced final body weight on GD 20 by 14% (Cabaleiro et al. 2008); the NOAEL was 0.61 mg/kg/day. In another study of gestational and lactational exposure, dietary administration of 29.8 mg/kg/day technical endosulfan also reduced GD 20 body weight by 14%, and food efficiency appeared to be reduced at this dose level; the NOAEL for changes in body weight was 10.8 mg/kg/day (Gilmore et al. 2006). In a study of only gestational exposure, gavage doses of 1 mg/kg/day technical endosulfan decreased maternal weight gain by 29% (Singh et al. 2007a). Dosing of rabbits with 1 mg/kg/day (only dose tested) technical endosulfan by gavage in oil for 6 weeks (Ata et al. 2007) or 60 days (Ozmen and Mor 2012) did not significantly affect body weight, but a dose of 3 mg/kg/day for 30 days induced an 18% reduction in final body weight (Hatipoglu et al. 2008); the NOAEL was 1.5 mg/kg/day in the latter study. A dose of 2 mg technical endosulfan/kg/day by gavage in water for 90 days was reported to cause significant reduction in weight gain in rats (Paul et al. 1994); in this case, food intake was also suppressed.
Body weight gain was not significantly reduced (<10%) in male or female mice treated in the diet with 2.5 or 2.9 mg/kg/day technical endosulfan, respectively, for 24 months (Hack et al. 1995; Hoechst 1988b). Both male and female rats treated with 3–3.5 mg endosulfan/kg/day in the diet for 24 months also exhibited reduction in body weight gain in the range of 17–18% (Hack et al. 1995). The no-effect-level dose was approximately 0.6–0.7 mg/kg/day. An additional chronic study also reported a significant decrease in weight gain in male rats fed a diet that provided approximately 20 mg of technical endosulfan/kg/day (NCI 1978). In this case, the treated animals were approximately 23% lighter than matched controls after 80 weeks on the experimental diet.
Metabolic Effects. Severe metabolic acidosis with high anion gap was reported in humans after acute poisoning with endosulfan (Blanco-Coronado et al. 1992; Lo et al. 1995). In five of the six cases reported by Blanco-Coronado et al. (1992), the metabolic acidosis was corrected with gastric lavage with activated charcoal and intravenous sodium bicarbonate and diazepam. No further information regarding metabolic effects in humans after exposure to endosulfan was located.
Some studies in animals indicate that endosulfan may affect glucose metabolism and ion permeability of cells. Increased blood glucose and/or decreased hepatic glycogen levels have been observed following acute- and intermediate-duration oral exposure to endosulfan (Chatterjee et al. 1986; Garg et al. 1980; Kiran and Varma 1988). It should be noted that this has been observed in animals exhibiting frank neurotoxicity. Interestingly, the hyperglycemia and decreased hepatic glycogen levels reported by Kiran and Varma (1988) were much more marked in older rats than in younger animals, and older animals, but not younger ones, showed frank neurotoxic effects. None of these studies provided information regarding the composition of the endosulfan formulation used; therefore, they are not presented in Table 3-2. In a study in Wistar rats, dosing males with up to 37.2 mg technical endosulfan/kg/day and females with up to 45.5 mg/kg/day via the diet for 13 weeks did not significantly alter serum levels of phosphorus, potassium, or sodium (Sheets et al. 2004).
Decreased serum calcium has also been observed following a 7-week oral exposure to 5 mg/kg/day of endosulfan (Garg et al. 1980).
Other Systemic Effects. In a group of seven rabbits treated with a single gavage dose of 15.1 mg/kg of a formulation containing only 35% endosulfan, two that recovered from the severe initial neurotoxic effects decreased their food intake by 82% relative to controls during the following weeks after treatment (Ceron et al. 1995). The remaining five rabbits died within hours of dosing. Rats treated for 90 days with daily doses of 2 mg technical endosulfan/kg by gavage in water also reduced their food intake throughout the study (Paul et al. 1994). On the average, the treated rats ate 22% less food than the controls. Although the treated rats did not exhibit severe neurotoxic effects, their spontaneous motor activity was increased relative to controls. Neither food consumption nor water consumption were significantly altered in mice or rats administered technical endosulfan in the diet for 24 months (Hack et al. 1995). In spite of this finding, both mice and rats gained significantly less weight during the study than their matched controls.
3.2.2.3. Immunological and Lymphoreticular Effects
No lesions of the spleen were evident on autopsy of a man who ingested a dose of approximately 260 mg endosulfan/kg (Boereboom et al. 1998).
Studies in male rats indicate that both humoral and cellular immune responses are depressed by endosulfan at doses that do not induce any other overt signs of toxicity. In a series of experiments, Banerjee and Hussain (1986, 1987) administered technical endosulfan in the diet of male rats at concentrations ranging from 5 to 50 ppm (equivalent to 0.45–4.5 mg/kg/day) for 6–22 weeks. The animals were immunized with a subcutaneous injection of tetanus toxin with an equal volume of Freund’s Complete Adjuvant approximately 20 days prior to sacrifice. The animals did not exhibit any overt signs of toxicity, and no changes in body weight or mortality were noted. Serum antibody titer (to tetanus toxin), serum immunoglobulin levels (IgM and IgG), and serum globulin fractions (α-, β-, and γ-globulin) were studied to evaluate humoral immune responses. Serum antibody titer to tetanus toxin, IgG, IgM, and γ-globulin levels were significantly decreased in rats exposed to endosulfan at 4.5 mg/kg/day for 6 weeks and at 0.9 mg/kg/day for longer periods. The effects of endosulfan on cell-mediated immune competence were evaluated with macrophage migration inhibition (MMI) and leukocyte migration inhibition (LMI) tests. The results of both tests indicated that the cell-mediated immune response was significantly depressed in a dose-related manner in animals administered 1.8, 2.7, and 4.5 mg/kg/day. Spleen and thymus weights were not affected by endosulfan treatment in animals treated for 6 weeks, but a significant decrease in spleen weight was observed at 22 weeks in the 1.8 mg/kg/day dose group. These rats also had a significantly increased albumin-to-globulin ratio at week 22. The authors concluded that these results indicate that endosulfan can suppress both humoral and cell-mediated immune responses in rats exposed to levels of endosulfan that induce no other signs of toxicity. This was an apparently well-conducted study that measured sensitive indicators of both humoral and immune function using doses of endosulfan that have not been previously shown to cause toxicity. Male mice exposed to 7.3 mg/kg/day for 13 weeks had significantly decreased spleen weights and decreased neutrophil counts (Hoechst 1984b), indicating that immune activity in mice may also be affected. An intermediate-duration oral MRL of 0.005 mg/kg/day was derived based on the NOAEL of 0.45 mg/kg/day for immunotoxicity identified in the Banerjee and Hussain (1986) study. The intermediate-duration oral MRL was also adopted for the chronic-duration oral MRL for endosulfan.
Other studies have examined the effects of endosulfan on immune function in rats and have not observed effects at higher doses; however, these other studies have examined the effects of endosulfan administration for shorter durations and did not evaluate many of the same end points that showed positive effects in the studies by Banerjee and Hussain (1986, 1987). For example, doses of 4.5 mg/kg/day technical endosulfan given 2 days before and 10 days after infection with Trichinella spiralis larvae resulted in no effect on the number of worms found in the body at sacrifice, no effect on the thymus or spleen weights, and no effect on the percent lymphocytes or white blood cell count (Hoechst 1988c). Also, there were no or marginal effects on the weight and histopathology of the thymus, spleen, or mesenteric and popliteal lymph nodes, or on leukocyte or monocyte counts. Serum IgM and IgG were not affected by 3 weeks of exposure to 5 mg/kg/day (Vos et al. 1982).
Chronic-duration studies have not generally shown adverse effects on organs of the immune system. Routine gross and histopathologic examination of the lymph nodes and thymus of rats, mice, and dogs exposed to technical endosulfan for 2 years at doses of up to 2.9 mg/kg/day (Hoechst 1989a), 2.9 mg/kg/day (Hoechst 1988b), 1 mg/kg/day (FMC 1967), or 48 mg/kg/day (NCI 1978), respectively, revealed no adverse effects. However, these studies did not assess immune function directly.
These results demonstrate that immunotoxicity may be a sensitive end point of endosulfan-induced toxicity following exposure to low doses for sufficient durations. However, since the Banerjee et al. (1986) study was considered preliminary by the authors, it would be reassuring if the results can be replicated by other laboratories. The highest NOAEL value and all reliable LOAEL values for immunological effects in each species in each duration category are recorded in Table 3-2 and plotted in Figure 3-2.
3.2.2.4. Neurological Effects
The most prominent signs of acute overexposure to endosulfan in both humans and animals are hyperactivity, tremors, decreased respiration, dyspnea, salivation, and tonic-clonic convulsions. Five cases of acute lethal poisoning in humans resulting from accidental or intentional ingestion of Thiodan® were reported in an early study by Terziev et al. (1974). The ingested doses were not specified. Initial clinical signs observed in all cases included nervous system effects such as agitation, writhing, and loss of consciousness. Autopsies performed on three of the cases at an unspecified time after ingestion revealed brain edema. Central nervous system stimulation also characterized the clinical syndrome displayed by a 20-year-old man who attempted suicide by ingesting 200 mL of a 30% endosulfan formulation (Thionax®) (Shemesh et al. 1988). Although the patient’s stomach contents were aspirated and he was given activated charcoal to reduce absorption, the patient displayed recurrent convulsions in the first 2 weeks following ingestion. This stage was followed by a slow recovery phase, in which psychomotor function slowly returned. One year after his attempted suicide, his mental activity (presumably psychomotor activity) was still severely impaired, and he required medication to control his seizures. This case report demonstrates that long-term brain damage can occur following acute overexposure to endosulfan in humans. The brain damage may have been caused by hypoxia that accompanied the recurring seizures and respiratory insufficiency seen within the first 2 weeks of ingestion. It is also unclear whether the effects observed may have been due, in part, to other ingredients in the Thionax®.
Similarly, convulsive seizures and a sustained epileptic state persisted after stomach contents were pumped and activated charcoal and anticonvulsive medication were administered to a 43-year-old man who ingested approximately 100 mL of an unspecified endosulfan formulation (18.8 grams of endosulfan or 260 mg/kg according to the investigators) 1 hour earlier (Boereboom et al. 1998). Since endosulfan appeared to have been already absorbed, as judged by the presence of convulsions, the stomach pumping and activated charcoal may have not had the intended effects. Four days after exposure the man was pronounced brain dead and autopsy revealed cerebral hernia from massive cerebral edema. Additional accidental and/or intentional cases of acute poisoning with endosulfan resulting in adverse neurological effects have also been reported in other studies (Blanco-Coronado et al. 1992; Lo et al. 1995; Pradhan et al. 1997). Tonic-clonic convulsions were seen in the Blanco-Coronado et al. (1992) cases, whereas Lo et al. (1995) reported the development of muscle fasciculations and episodes of convulsions in their case. In the case reported by Pradhan et al. (1997), the patient had consumed about 75 mL of liquid endosulfan (35% w/v). In this case, in addition to tonic-clonic seizures and myoclonic jerks, the patient developed psychosis, cortical blindness, and limb rigidity. Magnetic resonance imaging showed reversible lesions of the basal ganglia and occipital cortex. The amount of endosulfan ingested in the Blanco-Coronado et al. (1992) and Lo et al. (1995) reports was unknown.
Eyer et al. (2004) reported two cases of intentional ingestion of endosulfan who presented to the emergency room with persistent seizures. One of the cases, who had consumed 500 mL of a formulation containing 35% active ingredient (180 g of endosulfan according to the investigators), died on day 10 from multiorgan failure. The other case, who responded to treatment, had ingested 35 mL of an organochlorine fluid containing 12.3 g endosulfan. Parbhu et al. (2009) reported that a 2.5-year-old male developed generalized tonic-clonic seizures immediately after ingesting an unknown amount of an 11.6% solution of endosulfan. On day 3, an MRI showed cerebral edema and nuclear medicine flow scan failed to show blood flow to the brain and he was pronounced dead. Kamate and Jain (2011) reported the case of a 2-year-old girl who presented with generalized clonic-status epilepticus to the emergency room after accidentally ingesting endosulfan. Convulsions were controlled by medications and the child gradually regained consciousness after 36 hours of admission. Karatas et al. (2006) reported 23 cases of endosulfan poisoning presenting to an emergency department. On admission, five patients showed seizures and one patient complained of dizziness. The signs and symptoms began 1 hour after ingestion in 12 patients, in the second hour in 9 patients, and in the third hour in 2 patients. Sixteen patients had generalized tonic-clonic seizures and 3 patients had focal seizures. Most patients were discharged within 2 days after intravenous treatment with diazepam. In a study of 52 cases of acute endosulfan poisoning conducted by Moon and Chun (2009), 48 cases experienced seizures and refractory status epilepticus was the most common cause of death. The median latency to seizure onset after ingestion was 70 minutes and the greatest latency was 210 minutes. Refractory status epilepticus was also the cause of death of a case described by Roberts et al. (2004). Among 16 patients with acute endosulfan poisoning identified by Moses and Peter (2010), neurological toxicity predominated, particularly low sensorium (81%) and generalized seizures (75%), including status epilepticus (33%).
A study of 70 patients with Alzheimer’s disease and 75 healthy controls from India found no significant association between the concentration of α- and β-endosulfan in the blood and increased risk of the disease (Singh et al. 2013). Mean values for α- and β-endosulfan in blood from patients with Alzheimer’s disease were higher (2.62 and 2.37 ng/mL) than in controls (1.08 and 1.17 ng/mL), but the differences were not statistically significant. Although no information was provided regarding exposure, it is assumed that both patients and controls were members of the general population, so exposure to pesticides was mainly via ingestion of contaminated food and/or water,
Central nervous system stimulation is the hallmark of acute overexposure to endosulfan in experimental animals. The spectrum of effects includes hyperexcitability, tremors, decreased respiration, tonic-clonic convulsions, and ultimately, death (Boyd and Dobos 1969; Boyd et al. 1970; FMC 1958; Gilbert and Mack 1995; Hoechst 1970, 1975, 1984e; Kiran and Varma 1988). Convulsions have been observed after a single dose of 5 mg/kg in rats (Gilbert and Mack 1995), and after 4 daily doses of 1.8 mg/kg/day in pregnant rabbits (MacKenzie et al. 1981). At 6 mg/kg/day for 14 days, pregnant rats displayed face rubbing, flaccidity, and hyperactivity (FMC 1980). In a study by Bury (1997), female rats administered a single gavage dose of 3 mg/kg had increased incidences of squatting posture, straddled hindlimbs, decreased spontaneous activity, bristle coat, and irregular respiration and panting. Cerebral congestion and edema are often observed at necropsy in animals that die following acute ingestion of endosulfan (Boyd and Dobos 1969; Boyd et al. 1970).
A study in female Wistar rats tested the effectiveness of phenobarbital (Luminal®) and diazepam (Valium®) in reducing the neurotoxicity and lethality of endosulfan (Hoechst 1984e). The rats were administered a single dose of 80 mg/kg technical endosulfan by gavage and were observed for 14 days. Intraperitoneal administration of the therapeutic agents took place 10–20 minutes after treatment with endosulfan at the stage of hyperactivity, before the occurrence of the convulsive phase. Administration of endosulfan alone to 20 rats resulted in the death of 19 rats within 4 hours. Administration of diazepam in doses ranging from 2 to 60 mg/kg was ineffective in preventing death; 24 out of 25 rats died within the first 24 hours after treatment, with only a slight delaying of mortality. Administration of phenobarbital in doses ranging from 50 to 70 mg/kg resulted in a significant reduction both in mortality and clinical signs of intoxication. At the end of the observation period, 12 out of 30 rats tested (40%) survived. Necropsy of dead rats did not reveal chemical-related abnormalities.
Neurological effects have also been described in some intermediate or chronic ingestion studies of technical endosulfan in experimental animals (FMC 1965, 1967; Hoechst 1984b, 1989a). For example, in female rats given daily gavage doses of 1.5 mg/kg/day of endosulfan for 30 days, signs of central nervous system stimulation were observed for the first 3–4 days only and subsided thereafter (Dikshith et al. 1984). However, dietary administration of 3.74 mg/kg/day technical endosulfan to pregnant rats from GD 6 to lactation day 21 did not induce signs of hyperexcitability (Gilmore et al. 2006). The difference in the results from these two studies can probably be attributed to the different mode of administration of endosulfan (i.e., gavage versus dietary). In the Gilmore et al. (2006) study, doses ≥10.8 mg/kg/day increased rearing activity, although without a clear dose-response relationship. In addition, doses of up to 29.8 mg/kg/day did not significantly affect the results of a FOB that included examination of autonomic function, posture and gait, and behavior (Gilmore et al. 2006). Sheet et al. (2004) also did not find significant alterations in tests of motor and locomotor activity and in results of a FOB in Wistar rats administered up to 45.5 mg technical endosulfan/kg/day via the diet for 13 weeks. In that study, microscopic examination of the brain at multiple levels and of the spinal cord and peripheral nerves did not show compound-related alterations. Repeated administration of 1.5 mg/kg/day technical endosulfan by gavage to rabbits induced tremors and convulsions and was lethal to three out of nine rabbits, doses of 0.75 mg/kg/day were without effect (Hatipoglu et al. 2008). In another study, gavage administration of 1 mg/kg/day technical endosulfan (only level tested) for 4 weeks also induced hyperexcitability in rabbits (Mor and Ozmen 2010). Gross examination of the rabbit’s brain showed marked hyperemia at the meningeal vessels and slight hemorrhages in brains and cerebellum in some rabbits. Light microscopy revealed marked lesions including hemorrhage, marked edema, tissue degeneration, and slight glyosis.
Dogs that ingested feed containing 30 ppm technical endosulfan for 54 days, 45 ppm for 52 days, and 60 ppm for up to 40 days (a TWA dose of 2.9 mg/kg/day for males or 2.6 mg/kg/day for females) showed extreme sensitivity to noise, frightened reactions to optical stimuli, and tonic contractions of the muscles of the extremities, face, and jaw (Hoechst 1989c). Animals exhibiting these symptoms were sacrificed to prevent suffering. Prior to sacrifice, the reflexes of these animals were tested. The placing and righting reflexes were absent, but pupillary, flexor, patellar, oral, and cutaneous reflexes were unaffected. At autopsy, results of routine gross and microscopic examination of the cerebral cortex, brain stem, cerebellum, medulla, optic and sciatic nerves, and spinal cord were normal. At slightly lower doses in this study, approximately 2.5–6 hours after consuming 2 mg/kg/day (males) or 1.8 mg/kg/day (females), dogs showed convulsive spasms of the jaws and abdominal muscles without vomiting. Pathology of the gastrointestinal tract did not reveal any adverse effects on these tissues, suggesting that the nervous system may have been the cause of the spasms. However, no effects on reflexes were observed, and gross and microscopic examination of central nervous system tissue revealed no abnormalities. Thus, it is unclear whether the effects observed at this dose were centrally mediated or were responses to gastrointestinal disturbances. No significant neurological effects were observed in studies in rats at doses of 2.9 mg/kg/day (males) and 3.8 mg/kg/day (females) for up to 2 years (Hoechst 1989a) or in rats, mice or dogs in other intermediate- and chronic-duration studies (FMC 1967; Hoechst 1984b, 1988b, 1989c; NCI 1978).
A series of experiments were conducted in male Long-Evans rats to: assess the generality of an increased and persistent susceptibility to seizures following treatment with technical endosulfan; test the bidirectionality of kindling transfer induced by chemical and electrical means; and determine whether chemical kindling reflects cumulative endosulfan toxicity (Gilbert 1992; Gilbert and Mack 1995). The findings can be summarized as follows: (1) a single gavage dose of 2.5 mg/kg of endosulfan reduced the threshold for seizure activity by electrical stimulation in amygdala kindled rats; (2) previous electrical stimulation reduced the threshold for convulsions by a single endosulfan dose; (3) repeated pretreatment with endosulfan followed by a 2-week drug-free period reduced the threshold for seizures by a challenge dose of endosulfan, arguing against cumulative toxicity; and (4) repeated pretreatment with endosulfan reduced the threshold for seizures by electrical stimulation. The positive transfer to electrical kindling suggested a commonality in the mechanism between seizures induced by repeated administration of endosulfan and those produced by repeated electrical stimulation.
The effects of endosulfan on the concentration of neurotransmitter substances in various regions of the brain from rats have been examined (Lakshmana and Raju 1994). These authors found that, relative to controls, treatment of newborn rats by gavage with technical endosulfan (6 mg/kg) for 8 days resulted in changes (increases and decreases) in the levels of noradrenaline, dopamine, and serotonin in the areas of the central nervous system that were examined (olfactory bulb, hippocampus, visual cortex, brainstem, and cerebellum). Treatment for 23 days also resulted in changes in neurotransmitter levels, but either of different magnitude or different direction than those observed in the animals exposed for 8 days, indicating that the duration of exposure is an important parameter to consider when dealing with very young animals. Lakshmana and Raju (1994) also conducted a behavioral test in the rats treated for 23 days and found that treated rats took 29% more time to learn a task than the matched controls. The neurobehavioral effects of endosulfan have also been examined by others. Treatment of immature male rats with 2 mg technical endosulfan/kg/day by gavage for 90 days resulted in inhibition of learning and memory processes, and increased spontaneous motor activity (Paul et al. 1994). Since motor coordination was not significantly altered, Paul et al. (1994) suggested that the impairment in memory and learning was due to a motivation deficit rather than to motor impairment. The learning process, but not the memory process, was re-instated by a serotonin depletor, suggesting that endosulfan produced a learning deficit by increasing serotonergic activity. In a subsequent study by the same group of investigators, in which both male and female rats were tested, it was found that a 30-day treatment with endosulfan in the diet (3 mg/kg/day) increased spontaneous motor activity to a greater degree in males than in females, but there was no sex difference regarding the impairment in memory and learning processes (Paul et al. 1995). The authors speculated that the more marked effect in males may have been due to males preferentially metabolizing endosulfan to a more lipophilic metabolite, endosulfan sulfate, which could have reached the central nervous system. However, other factors cannot be ruled out, in particular since based on the chemical properties described in Chapter 4, endosulfan sulfate does not appear to be significantly more lipophilic than the parent compound.
In summary, endosulfan induces stimulation of the nervous system in humans as evidenced mainly in acute cases of intoxication. Hyperactivity has also been reported in acute-, intermediate-, and chronic-duration studies in animals. If the animal survives the acute toxic effects, then no long-term neurotoxic effects are evident from behavioral, gross, or microscopic observations. However, some impairment may occur that can be detected only by specialized neurobehavioral testing. Endosulfan can also affect the levels of neurotransmitters in the brain.
The highest NOAEL values and all reliable LOAEL values for neurological effects in each species and duration category are recorded in Table 3-2 and plotted in Figure 3-2. In some studies, only the α- or β-isomer of endosulfan was tested. In such cases, a notation regarding the specific isomer tested is included in the effect description.
3.2.2.5. Reproductive Effects
A study was conducted that examined the possible association between levels of some organochlorine pesticides, endosulfan among them, in adipose tissue and infertility in men (Çok et al. 2010). The cohort consisted of 25 infertile men and 21 fertile men. Azoospermic men were chosen among infertile couples who were married for at least 1 year and could not achieve a pregnancy although no contraceptive method was used. The diagnosis of azoospermia was confirmed through two consecutive spermiograms evaluated according to World Health Organization (WHO) criteria. The subjects were asked to complete a questionnaire about their dietary habits, exposure to chemicals in their daily life and at work, home environment, smoking, medical histories, weight, and height. There was no occupational exposure to the chemicals tested, so exposure was assumed to have been predominantly dietary. While the range of endosulfan in adipose tissue (mg/kg of lipid) from infertile men was much wider than in fertile men, the means did not differ statistically. The small number of subjects examined precludes drawing strong conclusions from this study.
A cross-sectional study of 304 men and 300 women from Brazil living near a factory that produced pesticides (endosulfan among them) until 1961 examined associations between sex hormones and levels of pesticides in serum (Freire et al. 2014). In this cohort, exposure occurred through contaminated water, soil, and local food. Testosterone was measured in men and estradiol, progesterone, prolactin, LH, and FSH in women. Results of linear regression analyses showed a significant negative association between serum levels of LH in peri- and postmenopausal women and serum levels of α- and β-endosulfan (as well as for a number of other pesticides) after adjusting for confounders. A significant negative association also was apparent for serum levels of FSH and α-endosulfan in the same groups of women. No significant association was found between levels of pesticides and sex hormone levels in premenopausal women or between endosulfan and levels of testosterone in men. Limitations of the study noted by the investigators included the fact that only one blood collection was conducted, blood samples were not dated according to each woman’s menstrual cycle day, and information on hormonal contraception, hormone replacement therapy, or use of medication that could interfere with hormone levels was not available in the cohort studied. In addition, information on reproductive hormones other than testosterone was not obtained for men. Strengths of the study included a relatively high number of participants.
Numerous studies provide information regarding the effects of endosulfan on reproduction in animals. Exposure of pregnant rats to 1 mg/kg/day technical endosulfan (only level tested) by gavage on GDs 6–20 resulted in a 4-fold increase in the percentage of post-implantation loss relative to controls (Singh et al. 2007b). Cabaleiro et al. (2008) reported an 11% reduction in litter size in rats exposed by gavage to 6.1 mg/kg/day technical endosulfan on GDs 1–21, but not with 0.61 mg/kg/day. In contrast, administration of a much higher dose of technical endosulfan, 29.8 mg/kg/day, via the diet to pregnant rats on GDs 6–21 resulted in only an 8% reduction in mean litter size (Gilmore et al. 2006). The difference between the results of Cabaleiro et al. (2008) and Gilmore et al. (2006) may be in part due to the exposure duration and mode of administration of the chemical (i.e., gavage versus diet). Administration of estimated doses of up to 9 and 8 mg/kg/day of technical endosulfan via the diet to male and female rats, respectively, for 2 weeks prior to mating and continued consumption throughout gestation resulted in no adverse effect on mating performance, pregnancy rate, or gestation (Hoechst 1982). It should be noted that the actual intake of test material was quantified only during the first 2 weeks of exposure. Similarly, administration by gavage of 5 mg/kg/day technical endosulfan to male rats and 1.5 mg/kg/day to female rats for 30 days prior to mating had no adverse effects on fertility when the matings of treated males with control females and treated females with control males were compared to the mating of control males and females (Dikshith et al. 1984). A two-generation reproduction study in rats detected no effect on the size, mortality, or sex ratio of the litters following consumption of doses of 7 mg/kg/day technical endosulfan through the diet for 84 days prior to the F0 mating and 98 days prior to the F1 mating (Hoechst 1984a). However, Jain et al. (2013) reported that exposure of male rats to 10 mg/kg/day (only dose tested) technical endosulfan for 60 days followed by mating with untreated females resulted in reduced fertility as assessed by a significantly reduced number of implantation sites in the females on day 16 of pregnancy. Since there is no indication that statistical tests were applied to the results, this study does not seem reliable and, therefore, is not listed in Table 3-2. Furthermore, according to the text and a graph in the paper, fertility changed from positive 100% in the control group to negative 80% in females mated to treated males, which is unclear.
A number of studies that used dose levels comparable to those described above have not observed adverse effects on the reproductive organs of rats, mice, or dogs. For example, routine gross and histopathological examination of the reproductive organs of male and female rats that ingested doses of technical endosulfan of 5 mg/kg/day for 15 days or 2 years revealed no adverse effects on these organs (FMC 1959b; Gupta and Gupta 1977a; Hack et al. 1995; Hoechst 1989a). Similarly, routine gross and histopathological examination of the reproductive organs of mice that consumed doses of 7.3 mg/kg/day (males) and 7.52 mg/kg/day (females) for 13 weeks (Hoechst 1984b) or 2.5 mg/kg/day (males) and 2.9 mg/kg/day (females) for 2 years (Hack et al. 1995; Hoechst 1988b; NCI 1978) revealed no toxic effects. Also, routine gross and microscopic examination of the reproductive organs of dogs that consumed doses of 2.9 mg/kg/day (TWA dose; males) and 2.6 mg/kg/day (TWA dose; females) for 146 days (Hoechst 1989c) or 2 mg/kg/day (males) and 1.8 mg/kg/day (females) for 1 or 2 years showed no adverse effects (FMC 1967; Hoechst 1989c).
Raizada et al. (1991) examined the potential estrogenic properties of an unspecified endosulfan formulation in ovariectomized rats. Endosulfan administered by gavage at 1.5 mg/kg/day for 30 days to ovariectomized rats did not influence the relative weights or histology of the uterus, cervix, or vagina compared to ovariectomized control rats that did not receive endosulfan. Rats in a positive control group received intraperitoneal injections of estradiol and showed increased relative organ weights and normal development of female reproductive tissues compared to the untreated ovariectomized control rats. Organ weights and tissue development in rats administered simultaneously endosulfan and estradiol were not significantly different from those seen in rats that received estradiol alone. The study results indicated that endosulfan was neither estrogenic nor anti-estrogenic under the conditions of this assay. Hiremath and Kaliwal (2003) examined the potential estrogenic properties of technical endosulfan in ovariectomized mice. Estrogenic activity was assessed using uterine weight and vaginal cornification as end points. Antiestrogenic activity was assessed by administering technical endosulfan along with 17β-estradiol to a group of mice. Ovariectomized control mice showed a prolonged diestrus. Mice treated with 4 mg/kg endosulfan (only level tested) for 30 days did not show vaginal cornification and showed continuous diestrus without any appearance of estrus, indicating its nonestrogenic activity. In addition, there was no significant change in uterine weight. Mice dosed with endosulfan plus 17β-estradiol showed a significant increase in the duration of estrus and increased uterine weight, indicating that endosulfan did not have antiestrogenic activity under the conditions of the study.
Several studies have reported alterations in sperm parameters following exposure to endosulfan. A study in male guinea pigs reported a 28% reduction in sperm count following 14 days of treatment with 2.5 mg/kg/day (the lowest dose tested) technical endosulfan for 14 days (Umar et al. 2012). Male rats given oral doses of 10 mg/kg/day of technical endosulfan for 15 days had decreased weight of the testes (Gupta and Chandra 1977). Gavage doses of 5 mg/kg/day (lowest level tested) of technical endosulfan for 15–30 days reduced sperm motility in the cauda epididymis of rats by 35% (Choudhary and Joshi 2003). Decreased sperm volume and increased abnormal sperm morphology and dead sperm were reported in rabbits dosed by gavage with 1 mg/kg/day technical endosulfan (only level tested) for 6 weeks (Ata et al. 2007). Reduced sperm count and altered testicular enzyme activities, indicating altered spermatogenesis, were also reported in mature rats treated by gavage with 2.5 mg technical endosulfan/kg/day (the lowest dose tested), 5 days/week for 70 days (Sinha et al. 1995). Additional effects seen at 5 and 10 mg/kg/day included reduced intratesticular spermatid count and daily sperm production, and increased incidence of abnormal sperm. All of these effects were also observed in young male rats (3 weeks old) treated by gavage with 2.5 mg technical endosulfan/kg/day (the lowest dose tested), 5 days/week for 90 days, suggesting that the younger animals were more sensitive than the older ones (Sinha et al. 1997). Altered sperm parameters (cauda sperm count, sperm motility and viability, and daily sperm production) were reported in rats dosed with 5 mg/kg/day (only dose tested) technical endosulfan for 15 days (Aly and Khafagy 2014). Increased sperm abnormalities also were reported in rats dosed with 1.5 mg/kg/day (lowest dose tested) technical endosulfan for 45 days (Bharath et al. 2011) and in rats dosed with10 mg/kg/day (only dose tested) technical endosulfan for 60 days (Jain et al. 2013). However, exposure of rats to 2.9 mg/kg/day technical endosulfan (only level tested) in the diet for 8 weeks did not significantly alter the number of mature spermatids in the testes, daily sperm production, number of sperm in the caput/corpus and cauda epididymis, sperm transit time through the epididymis, or sperm morphology (Perobelli et al. 2010). Mice appeared to be more sensitive than other species as doses of 0.8 mg/kg/day (only dose tested) technical endosulfan for 3 weeks decreased sperm count and motility and increased the frequency of sperm abnormalities (Wang et al. 2012, 2014).
Studies have also reported histological alterations in sex organs after exposure to endosulfan. For example, male rats given oral doses of 10 mg/kg/day technical endosulfan by oral gavage for 15 days showed marked degenerative changes in the epithelium of the seminiferous tubules, but no significant effects were reported with 5 mg/kg/day (Gupta and Chandra 1977). Similarly, male rats that consumed a TWA dose of 47.6 mg/kg/day technical endosulfan via the diet for up to 74 weeks had testicular atrophy with degeneration and necrosis of germinal cells lining the seminiferous tubules, multinucleated cells, and calcium deposition resulting in aspermatogenesis (NCI 1978; Reuber 1981). Treatment of mice with 2.45 mg/kg/day (only dose tested) technical endosulfan by gavage for 15 days resulted in degenerative changes in the testes (Nagda et al. 2011). Lower gavage doses of 0.8 mg/kg/day technical endosulfan for 3 weeks induced histological alterations in the testes from male mice (Wang et al. 2014). Daily treatment of male rabbits with 1 mg/kg/day technical endosulfan by gavage for 60 days resulted in testicular alterations that included vascular congestion, interstitial edema, tubular degeneration, and coagulative necrosis of spermatozoa (Ozmen and Mor 2012).
Several studies provide information on levels of sex hormones following exposure to endosulfan. A study by Singh and Pandey (1990) reported a dose-related decrease in testicular testosterone, plasma testosterone, LH, and FSH in groups of male Wistar rats administered technical endosulfan by gavage at 0, 7.5, or 10 mg/kg/day for 15 or 30 days. Testicular microsomal cytochrome P450-dependent monooxygenases were also significantly inhibited at both dose levels after 30 days of exposure. All of the effects from 30 days of exposure were reversible during a 7-day recovery period, except for testicular testosterone, which remained depressed; recovery was not examined following the 15-day exposures. Decreased serum testosterone was also reported in male rats exposed to 15 mg/kg/day technical endosulfan by gavage for 15–30 days; no significant effects were reported at 10 mg/kg/day (Choudhary and Joshi 2003). Similar results have been reported in more recent studies. Serum testosterone was reduced in male rats administered 5 mg/kg/day technical endosulfan for 10 days (Aly and Khafagy 2014) or 30 days (Kumar et al. 2011), in male mice administered 0.8 mg/kg/day technical endosulfan for 3 weeks (Wang et al. 2014), and in male rabbits dosed with 1 mg/kg/day technical endosulfan for 60 days (Ozmen and Mor 2012). In contrast, doses of 2.9 mg/kg/day (only dose tested) technical endosulfan provided to male rats via the diet for 8 weeks had no significant effect on serum levels of testosterone, LH, and FSH (Perobelli et al. 2010). Endosulfan also increased serum prolactin by 25% in male rats administered 5 mg/kg/day technical endosulfan for 30 days (Kumar et al. 2011)
Information regarding testes weight following exposure to endosulfan is available in several studies. In all of these studies, endosulfan was administered by gavage. Singh and Pandey (1990) reported no significant effect on testis weight in rats after administration of up to 15 mg/kg/day technical endosulfan for up to 30 days. More recent studies have reported significant alterations in testes weight in several species following treatment with technical endosulfan. Reduced testes weight was reported in guinea pigs after 14 days of dosing with 2.5 mg/kg/day (Umar et al. 2012). Dosing rats with 5 mg/kg/day for 15 days (Aly and Khafagy 2014) or with 1.5 mg/kg/day for 45 days (Bharath et al. 2013) resulted in significant (15–16%) reduction in testes weight. Dosing mice with 2.45 mg/kg/day for 15 days resulted in an 18% reduction in testes weight (Nagda et al. 2011). In these recent studies, the effective doses were the lowest doses tested. It is worth noting that Dikshith et al. (1984) reported a doubling in testes weight in male rats following administration of 5 mg/kg/day (only dose tested) technical endosulfan for 30 days. No explanation is apparent for the lack of consistency between Dikshith’s results and other studies in rats.
In summary, although the available reproductive studies indicate that endosulfan has no adverse effects on reproductive performance in animals, adverse effects on male reproductive end points have been seen in rats, mice, rabbits, and guinea pigs. The lack of effects seen in the studies that examined reproductive performance (specifically fertility rate) in treated males may be due to the fact that, at least rats, produce and ejaculate 10 times more sperm than are necessary for normal fertility and litter size (Amann 1982; Working 1988).
The highest NOAEL values and all reliable LOAEL values for reproductive effects in rats, mice, and dogs for each duration category are recorded in Table 3-2 and plotted in Figure 3-2.
3.2.2.6. Developmental Effects
No clear geographic association was observed between the level of pesticide use and the locations of homes of children who underwent surgical correction for cryptorchidism (failure of descent of testes) in the Granada region of Spain (García-Rodríguez et al. 1996). Endosulfan exposure levels were unavailable, but another study reported endosulfan isomers and/or metabolites in adipose tissue of 20 of 50 children (40%) who were hospitalized in the Granada hospital for a variety of reasons (Olea et al. 1999), indicating that significant endosulfan exposures occurred in the region. In another study of male newborns from Southern Spain, higher levels of endosulfan sulfate in the placenta (n=220) were associated with lower odds of TSH ≥5 mU/L in cord blood levels (OR=0.36, 95% CI 0.17–0.77, p<0.008) (Freire et al. 2011). The investigators suggested that early exposure to endosulfan sulfate could lead to significant alterations in T4 feedback to the hypothalamus, resulting in decreasing TSH levels at birth. A study of 193 Brazilian children younger than 15 years old who lived near a former pesticide factory reported a significant increasing linear trend between serum endosulfan (1 of 19 pesticides analyzed) and total T3 levels (Freire et al. 2012). Serum levels of FT4 were positively and significantly associated with exposure to p,p’-DDD, α-endosulfan, and dieldrin; no association was found between pesticide exposure and serum TSH. The study controlled for age, gender, and serum lipid content. It should be noted that no child presented clinical or subclinical hyperthyroidism or hypothyroidism. Saiyed et al. (2003) conducted a study of reproductive development in 117 male schoolchildren of a village in India where endosulfan had been aerially sprayed for more than 20 years. Ninety children with no exposure history served as controls. Exposure pathways probably included a combination of the inhalation, oral, and dermal routes. The children were approximately 13 years old. After controlling for age, the study found significantly lower Tanner scores (development of pubic hair, penis, and testes) and serum testosterone level, and higher LH in the exposed group than in controls. Mean serum levels of total endosulfan were 5–6-fold higher in the study group than in controls. Based on results from animal studies, the results of Saiyed et al. (2003) seem biologically plausible; however, it is a small study with relatively high nonparticipation rate in the measurement of the Tanner scores (57% of the exposed group and 33% of controls).
Ren et al. (2011) examined the association between placental levels of some organochlorine compounds, endosulfan among them, and risks for neural tube defects in a rural Chinese population with high incidence of neural tube defects. The cohort consisted of 80 cases and 50 controls. The median level of α-endosulfan in placentas from cases was 0.079 ng/g lipid versus 0.054 ng/g in controls (p=0.03). The investigators reported that increased placental levels of α-endosulfan were associated with increased risks of neural tube defects (OR=2.53, 95% CI 1.03–5.99). When potential confounding factors were adjusted for with an unconditional multivariate logistic model, the association remained (OR=3.26, 95% CI 1.10–9.71). Examining the association between placental levels of α-endosulfan and specific subtypes of neural tube defects showed significant associations with anencephaly and spina bifida. After adjusting for maternal occupation, age, education level, parity, folic acid supplementation, passive smoking, and fever or flu during early pregnancy, season of conception, mother’s residence, and infant sex only the association with spina bifida remained significant. The simultaneous exposure to multiple organochlorine compounds makes it difficult to determine the specific role of endosulfan. Guo et al. (2014) examined the relationship between exposure to organochlorine pesticides (α-endosulfan) and infant birth weight in 81 Chinese mother-infant pairs; exposure was non-occupational and assumed to have been mostly oral. The maximal concentrations of α-endosulfan in maternal serum and cord blood were 1.32 and 6.08 ng/g lipid, respectively; the geometric means and medians were below the limit of detection for the analytical method. Multiple linear regression analyses of data adjusted for confounders showed no significant association between birth weight and maternal exposure to α-endosulfan.
Developmental effects have been observed in rats following oral administration of technical endosulfan to pregnant dams during gestation. Daily administration of endosulfan at doses of 5 or 10 mg/kg/day by gavage in corn oil during GDs 6–14 produced a statistically significant increase in the percentage of resorptions and skeletal variations in the fetuses (e.g., absent fifth sternebrae) (Gupta et al. 1978). A dose-related increase in maternal deaths was observed in both test groups. Thus, embryotoxic effects were observed at doses that also caused maternal toxicity. This study is limited in that dosing was not continued until day 15 and, therefore, did not include the entire period of organogenesis. No statistically significant effect on fetal weight, sex ratio, or skeletal, internal, or external development was observed following administration of doses of 1.5 mg/kg/day of technical endosulfan to pregnant rats by gavage in corn oil during GDs 6–15 (FMC 1972). A slight increase in the incidence of nonossified sternebrae was observed but did not reach statistical significance. Statistically significant skeletal variations (e.g., bipartite and misaligned sternebrae) were observed in fetuses following daily administration of doses of 0.66 mg/kg/day technical endosulfan by gavage to pregnant rats during GDs 6–19 (FMC 1980). However, these variations did not increase with dose, and the incidence observed at the highest dose tested (6 mg/kg/day) did not reach statistical significance. At 6 mg/kg/day, additional fetal toxicity was observed (e.g., decreased fetal weight and length and other skeletal variations). Therefore, 6 mg/kg/day could be considered a LOAEL for developmental toxicity in this study; however, the observation of statistically significant changes at lower doses places uncertainty on this LOAEL. Maternal toxicity (e.g., two deaths, decreased mean corrected body weight gain measured on GD 20, face rubbing, flaccidity, and hyperactivity) was observed in the high-dose group (6 mg/kg/day) and to a lesser extent in the mid-dose group (2 mg/kg/day) (e.g., decreased mean corrected body weight gain measured on GD 20 and face rubbing). Limitations of this study include a number of gavage errors and the unplanned addition of 10 more animals to the high-dose group and 5 more animals to the control group (mated at approximately 30 and 40 days after the initial mating).
Sinha et al. (2001) reported that exposure of pregnant Druckrey rats to 1 mg/kg/day (lowest dose tested) technical endosulfan by gavage in peanut oil on GDs 12–21 resulted in a 53% reduction in sperm count in cauda epididymis and in reductions in absolute and relative weight of the sexual organs from male offspring examined at 100 days of age. Since the offspring were fostered by untreated dams and were not exposed directly after weaning, the effects were attributed solely to gestational exposure. Two studies reported fetal malformations and histological alterations in fetal organs due to gestational exposure to endosulfan. Singh et al. (2007a) reported that administration of 1 mg/kg/day (only dose tested) technical endosulfan by gavage in olive oil to pregnant Wistar rats on GDs 6–20 resulted in a significant decreases in the mean percentage of live fetuses (21%) and mean fetal weight (21%). Also, crown-to-rump length was significantly decreased by 21% in the treated group. Gross, visceral, and skeletal anomalies were significantly increased in the treated group. The most important fetal malformations were internal hydrocephalus, cerebellar hypoplasia, microphthalmia, contracted and notched kidneys, multilobulated liver, dilated renal pelvis, incomplete ossification of skull bones, rib anomalies, and sacral and caudal vertebrae agenesis. Maternal body weight gain during GDs 6–20 was reduced 29% relative to controls. In a later publication, using the same exposure protocol, Singh et al. (2008) conducted microscopic examinations of the liver and kidneys from GD 20 fetuses. The livers from exposed rats showed vacuolar engorgement, sinusoidal dilation, hepatocyte degeneration, and karyomegaly. The kidneys showed tubular dilation, degeneration of tubular epithelium, necrosis of the tubular epithelium, and interstitial fibroblastic proliferation. No alterations were seen in controls. Since only one dose level was tested, the true LOAEL may be lower than 1 mg/kg/day in these two studies.
Exposure of rabbits to endosulfan during GDs 6–28 produced no significant effects on the number of implants, litter size, sex ratio, fetal weight or length, or percentage of live or resorbed fetuses at doses up to 1.8 mg/kg/day technical endosulfan by gavage in corn oil (MacKenzie et al. 1981). However, dams treated with 1.8 mg/kg/day did exhibit neurotoxic signs (e.g., noisy and rapid breathing, hyperactivity, and convulsions) that were considered to be treatment related. Such neurotoxic effects were not observed at 0.7 mg/kg/day.
In addition to the studies noted above that examined the effect of endosulfan administered only during the period of gestation, several studies have examined the effects of endosulfan on fetal development following administration prior to mating and throughout gestation and lactation. For example, administration of 6 mg/kg/day technical endosulfan via the diet from 2 weeks prior to mating through weaning resulted in a significant decrease in mean litter weight during lactation (Hoechst 1982). Increased pup mortality was observed at 8 mg/kg/day. Maternal toxicity (e.g., decreased body weight and increased relative liver weight) was observed in females at ≥6 mg/kg/day. Dietary doses of 7 mg/kg/day of technical endosulfan for 84 days prior to mating through weaning resulted in an 11% decrease in mean litter weight of rats on PND 12 (Hoechst 1984a). Both of these studies are limited in that insufficient information was provided regarding the intake of test material during gestation and lactation. Two additional studies reported significantly reduced (44–46%) male pup weight on PND 21 following maternal exposure to 6.12 mg/kg/day technical endosulfan by gavage in sesame oil during gestation and lactation (Cabaleiro et al. 2008; Caride et al. 2010). A lower dose of 0.61 mg/kg/day reduced pup’s weight by 11% on PND 21. In the high-dose group, maternal body weight at the end of gestation was reduced approximately 14% relative to controls. In both studies, birth weight was not significantly affected suggesting that, for this developmental end point, lactational transfer of endosulfan or a metabolite plays a bigger role than transplacental transfer. In the Caride et al. (2010) study, technical endosulfan was also shown to alter serum levels of prolactin (PL), LH, growth hormone (GH), and TSH measured in offspring on PND 30. In addition, analyses of mRNA in the pituitary showed significantly decreased PL gene expression (high-dose), LH gene expression (both groups), GH gene expression (both groups), and TSH gene expression (both groups). Endosulfan significantly increased nitric oxide synthase 1 and 2 (NOS1, NOS2) gene expression (both groups), but did not significantly affect heme oxygenase-1 (HO-1) gene expression. Nitric oxide is involved in the regulation of pituitary secretion and HO-1 represents a defense mechanism against free radicals. Caride et al. (2010) suggested that nitrosative stress may be implicated in the endocrine toxicity of endosulfan at the pituitary level.
Cabaleiro et al. (2008) also reported that perinatal exposure to technical endosulfan altered the concentration of amino acids and neurotransmitters in the prefrontal cortex from offspring on PND 15, 30, and 60. A later, and similar, study by the same group of investigators showed that maternal exposure to ≥0.61 mg/kg/day technical endosulfan by gavage during gestation and lactation induced alterations (increases and decreases) in the concentration and/or metabolism of dopamine, norepinephrine, and serotonin in the striatum from 60-day-old offspring (Lafuente and Pereiro 2013). Gender differences were evident and suggested that males were more susceptible than females.
Similar studies have been conducted in mice. Wilson et al. (2014a) examined the effects of endosulfan on levels of neurotransmitters (GABA, glutamate, dopamine) in the frontal cortex from 12-week-old male offspring from mice exposed to 1 mg/kg (only dose tested) α-endosulfan every other day during gestation and lactation. The results showed changes in protein markers that indicated: (1) alterations in expression of proteins that are critical to the function of the GABA neurotransmitter system in the frontal cortex; (2) alterations in proteins involved in glutamatergic neurotransmission; and (3) alterations in both presynaptic and postsynaptic dopaminergic proteins that are important in mediating dopaminergic transmission. Wilson et al. (2014b) used the same protocol to determine the effects of α-endosulfan on the dopaminergic neurotransmitter systems in the striatum from male offspring. Perinatal exposure to endosulfan resulted in significant reductions in striatal dopamine transporter and tyrosine hydroxylase (rate-limiting enzyme in the synthesis of dopamine). Treatment of adult mice with 1 mg/kg/day endosulfan for 30 days did not result in the neurochemical changes seen in mice exposed perinatally indicating that the developing mice were more sensitive to endosulfan than adult mice.
The studies of Wilson et al. (2014a, 2014b) and Lafuente and Pereiro (2013) are not presented in Table 3-2 because, in the absence of a measurable functional deficit, it is unclear whether the doses that induced neurochemical changes in the brain from the offspring should be classified as NOAEL or LOAEL.
A study was recently published that reported maternal and developmental effects in mice at doses of endosulfan considerably lower than other studies (Mansour et al. 2014). In that study, pregnant C57BL/6J mice were fed a diet containing 0.03 ppm (30 μg/kg food) technical endosulfan during gestation and lactation (approximately 0.006 mg endosulfan/kg/day using a default food consumption value of 0.0048 kg/food/day and body weight of 0.025 kg for the mice). Both dams and pups were sacrificed at weaning and the liver and kidneys were examined microscopically. Examination of the pups’ livers showed some congested sinusoids and necrotic hepatocytes. Renal glomeruli showed normal structure; however, some tubules showed epithelium detachment or degeneration and interstitial hemorrhage. Similar effects were reported in dams, including alterations of the hepatic lobules associated with hydropic degeneration, fatty change, and foci of necrotic cells. Maternal kidneys showed atrophy of glomeruli and detachment of the epithelium of the renal tubules and interstitial hemorrhage. While no other study has examined the liver or kidneys from pups from mice exposed to endosulfan during gestation and/or lactation, intermediate- and chronic-duration studies (Hack et al. 1995; Hoechst 1984b; NCI 1978) have not reported histological alterations in the liver or kidneys from mice administered endosulfan via the diet in doses up to 3 orders of magnitude higher than those in the Mansour et al. (2014) study. Because of the inconsistency with results of other studies in mice, neither the developmental nor the maternal LOAEL of 0.006 mg/kg/day from Mansour et al. (2014) are presented in Table 3-2. It would be helpful to try to replicate these findings.
Three studies provide information on the sexual maturation of offspring from dams exposed to endosulfan during gestation and lactation. Administration of 1.5 or 3 mg/kg/day technical endosulfan to pregnant Wistar rats by gavage in Tween and distilled water on GDs 15–21 and lactation days 1–21 did not significantly affect number of litters, live births, litter size, birth weight, mortality rate on PND 21, or pup weight on PND21 (Dalsenter et al. 1999). Testes descent and preputial separation occurred slightly earlier in exposed rats (about 1 day) and was not considered biologically significant. Examination of sperm parameters showed a decrease in daily sperm production in the low- (20%) and high-dose (39%) groups on day 65 and in the high-dose group (12%) on day 140, and decreases in percent complete spermatogenesis in the low- (16%) and high-dose group (11%) on day 65. Serum testosterone was not affected. The same group of investigators exposed female Wistar rats to 0.5 or 1.5 mg/kg/day endosulfan by gavage in sunflower oil for 21 days before mating and during gestation and lactation (Dalsenter et al. 2003). Treatment with endosulfan did not significantly affect litter size, birth weight, or weight or viability during PND 1–21. The age of testes descent and preputial separation was comparable among groups. There was no significant effect of exposure to endosulfan on daily sperm production, sperm number per cauda epididymis, sperm transit, percent abnormal sperm, and serum testosterone levels measured at sacrifice on PND 140. In the third study, pregnant Wistar rats were administered doses of 0, 3.74, 10.8, or 29.8 mg/kg/day technical endosulfan via the food on GDs 6–21 and lactation days 1–21 (Gilmore et al. 2006). Offspring were evaluated for clinical signs, body weight, developmental landmarks for sexual maturation, behavioral tests, and ophthalmology. Maternal body weight and weight gain were significantly reduced during gestation, and so was food consumption. Reduced food consumption in the low-dose group was attributed to unpalatability of the diet, but this was less clear for the mid- and high-dose groups. Food efficiency appeared to be reduced in the high-dose group. Mean maternal body weight in the high-dose group on GD 20 was reduced 14% relative to controls. Exposure to endosulfan did not affect neonatal developmental parameters. Pre- and post-weaning weights were lower in exposed pups than in controls. On PND 11, mean pup weight on a litter basis was reduced 8, 11, and 13% in the low-, mid, and high-dose groups, respectively. Preputial separation and vaginal opening were slightly delayed in exposed pups, but the effect on preputial separation was not dose-related, and vaginal opening was within historical controls. The only significant alteration in the results of a FOB was a significant increase in rearing (44% relative to controls) in males from the high-dose group on PND 45. Mean total motor activity was not significantly affected and neither was locomotor activity, auditory startle reflex habituation, and results of learning and memory tests. Ophthalmological examination was unremarkable. At termination on PND 75, analyses of sperm motility, counts and morphology did not reveal treatment-related alterations. There was a statistically significant decrease in fixed perfused brain weight in high-dose males on PND 2, but not in perfused or non-perfused fresh male brain weights on PND 75. Microscopic examination of the brain at various levels, tibial and sciatic nerve, and spinal cord did not show treatment-related effects. Measurement of brain areas showed a 10% decrease in hippocampal gyrus length in high-dose females on PND 21. No differences were seen in males. An additional study in which female Wistar rats were exposed prior to and during mating, gestation, and lactation to 0.5 or 1.5 mg/kg/day technical endosulfan by gavage in sunflower oil reported that treatment with endosulfan did not significantly affect maternal weight, but no data were shown (Silva de Assis et al. 2011). Treatment with endosulfan did not affect litter size, birth weight, or neonatal viability, although it reduced mean pup weight by 6% on PND 21. Neither body weight nor the weights (absolute or relative) of the pups’ liver or kidneys were affected on PND 65 or 140. Serum cholinesterase in high-dose pups was reduced 41% on PND 65 and was not significantly affected on PND 140.
In summary, based on the data available, the evidence for endosulfan-induced embrytoxicity and/or teratogenicity in animals is inconclusive because this has been reported at doses that also affected the mothers mainly in the form of reduced maternal weight gain during pregnancy. However, recent studies have shown that transplacental exposure (and presumably via lactation) to endosulfan and/or metabolites can result in neurochemical alterations in the brain of adult animals; it is unknown whether these alterations translate into behavioral or other functional deficits. In addition, there is weak evidence of endosulfa-induced alterations in sexual maturation in animals. The highest NOAEL value and all reliable LOAEL values for developmental effects in rats and rabbits for the acute- and intermediate-duration categories are recorded in Table 3-2 and plotted in Figure 3-2.
3.2.2.7. Cancer
No studies were located regarding cancer in humans after oral exposure to endosulfan. Carcinogenic effects of endosulfan were investigated in a number of chronic animal bioassays with rats and mice; the available data provide no evidence that endosulfan is carcinogenic.
Carcinogenicity in rats was first assayed in Osborne-Mendel rats by NCI (1978). The assay was flawed because the female rats were given technical endosulfan for less than their entire lifetime (78 out of 110 weeks); high early mortality in the males caused the high- and low-dose males to be terminated at 74 and 82 weeks, respectively, while half of the control males continued on study until 110 weeks; and the doses were changed several times during the study. The poor survival in the male rats precluded drawing a conclusion regarding the carcinogenicity of endosulfan in males because insufficient numbers of animals were alive to demonstrate a risk from late-developing tumors. However, the authors concluded that under the conditions of the assay, endosulfan was not carcinogenic in female rats.
Histological sections from this study were reevaluated by Reuber (1981), who concluded that endosulfan was carcinogenic. By grouping tumors, Reuber (1981) identified statistically significant increases in the total number of malignant tumors in both high-dose females (TWA dose, 22.3 mg/kg/day) and low-dose females (TWA dose, 11.1 mg/kg/day), as well as in the total number of carcinomas and sarcomas in high-dose females and lymphosarcomas in high-dose males (TWA dose, 47.6 mg/kg/day) and high-dose females. No increases in tumor incidence were identified in any specific tissue, and Reuber’s conclusions were not independently confirmed by other scientists.
The carcinogenicity of technical endosulfan was reevaluated in Sprague-Dawley rats using lower doses of endosulfan (Hoechst 1989a). Endosulfan was administered in the diet for 2 years, and no effect on survival was observed in either sex at any dose. Under the conditions of this assay, dietary consumption of doses as high as 3.8 mg/kg/day by females or 2.9 mg/kg/day by males did not result in an increase in the incidence of any neoplastic lesions in these animals. The results from the Hoechst (1989a) bioassay were subsequently published in the open literature (Hack et al. 1995). In an additional study, no increase in neoplastic lesions was observed in Wistar rats that consumed doses of endosulfan as high as 5 mg/kg/day (males) or 1.5 mg/kg/day (females) technical endosulfan for 2 years (FMC 1959b). However, this study is limited in that relatively few rats were used (25/sex/dose), and histopathological evaluation was limited to 5 rats per sex per dose plus any grossly observed lesions.
Carcinogenicity has also been evaluated by NCI using mice (NCI 1968, 1978). Two strains of mice (B6C3F1 and B6AKF1) were tested in the 1968 study. The B6C3F1 mice are the product of mating C57BL/6 females with C3H/Anf males. The B6AKF1 mice are the product of mating C57BL/6 females with AKR males. These hybrids were used because their susceptibility to carcinogenic stimuli was expected to be high. Each treatment group, and each vehicle, positive, and negative control group consisted of 18 males and females of each strain. The animals were administered technical endosulfan in 0.5% gelatin daily by gavage at doses of 1.0 and 2.15 mg/kg/day from 7 to 28 days after birth. From 28 days to 18 months, they were given endosulfan in the diet ad libitum. Concentrations in the diet were calculated so that the endosulfan doses consumed by the animals were the same as those given by gavage. However, these calculations were based on starting body weights, and no adjustments were made to account for growth and changes in food intake throughout the 18-month exposure period. A statistically significant increase (p<0.05) in the incidence of total tumors and pulmonary adenomas was reported for endosulfan; it appears, however, that the data for both strains and doses were combined to perform these statistical analyses. Therefore, it is not possible to assess the validity of these conclusions. Furthermore, the summary data sheets do not clearly indicate the dose, so it appears that the dose level with low survival was not the same dose level that displayed an increase in tumor incidence. Because of the incomplete reporting and the confusion regarding statistical analysis of tumor incidence data, these results cannot be considered adequate evidence of the carcinogenicity of endosulfan.
Carcinogenicity was reassayed by NCI (1978) in B6C3F1 mice. Fifty mice per sex per dose were used. Male mice were given 0, 0.46, or 0.9 mg/kg/day (TWA doses). Female mice were given 0, 0.26, or 0.5 mg/kg/day for 78 weeks. Mice of both sexes were then observed for an additional 14 weeks. No statistically significant increases in tumor incidence were observed in female mice. Because mortality in male mice was high in all groups, a conclusion regarding carcinogenicity in males was not made. Reuber (1981) also reevaluated histological sections from this study and concluded that a significant increase in the incidence of hepatic carcinomas occurred in the low-dose female mice. However, he indicated that histological sections from the liver were inadequate, and his conclusions were not independently verified by other scientists.
The carcinogenicity of technical endosulfan was also evaluated in NMRI mice exposed through the diet to endosulfan for 2 years at doses as high as 2.5 mg/kg/day in males and 2.9 mg/kg/day in females (Hoechst 1988b). Sixty mice per sex per dose were used. No increase in the incidence of any neoplastic lesion was identified in either males or females at any dose. These results were later published in the open literature (Hack et al. 1995).
The ability of technical endosulfan (98.8% pure), α-endosulfan, and β-endosulfan to act as tumor promoters in a two-stage, altered hepatic foci bioassay was examined in male Sprague-Dawley rats (Fransson-Steen et al. 1992). The animals were initiated by intraperitoneal injection of nitrosodiethylamine followed by 2/3 partial hepatectomy. Five weeks later, they were transferred to a diet that provided approximately 1.5, 5, or 15 mg test material/kg/day for 20 weeks. Promoting activity was evaluated for the development of foci of gamma-glutamyltranspeptidase-positive hepatocytes (AHF). Of the three chemicals tested, the α-isomer exhibited the strongest promoting activity; in initiated rats, it caused a significant and dose-related increase in both the volume fraction of liver occupied by AHF and the number of AHF/cm3, and only the highest dose increased the mean foci volume. Technical endosulfan and the β-isomer increased the volume fraction of liver occupied by AHF and the number of AHF/cm3, but the responses were not dose-related, and neither increased mean foci volume. Endosulfan, α-endosulfan, and β-endosulfan induced no or few AHF in rats that were not initiated.
3.2.3. Dermal Exposure
3.2.3.1. Death
No studies were located regarding death in humans after dermal exposure to endosulfan. However, dermal exposure to endosulfan caused death in livestock and experimental animals. Nicholson and Cooper (1977) described the case of five calves that were “dusted liberally” in the late afternoon with a 4% dust formulation of endosulfan to remove lice. The dose was not specified. By 7:00 a.m. the next morning, one calf was dead and the remaining four calves displayed signs of neurotoxicity (muscle tremors, twitching of the ears, snapping of the eyelids, hyperactivity, and tonic-clonic convulsions). By the end of the day, three more calves died and the remaining calf recovered without complications. A necropsy performed on one of the calves revealed no gross lesions.
Lethality data from studies using experimental animals indicate that the lethal dose varies substantially depending on the species and the sex of the animal tested. The dermal LD50 obtained following a single dermal application of endosulfan to the backs of female rabbits was in the range of 167–182 mg/kg of technical endosulfan (Gupta and Chandra 1975). However, two out of three female rats died following exposure to 31.25 mg/kg/day β-endosulfan for 6 hours/day for 5 days (Hoechst 1989b). In contrast, exposure to 250 mg/kg/day during the same 5-day period was not lethal to male rats. At 500 mg/kg/day, two of three males died. This study is limited, however, by the small number of animals tested. Single dermal doses of 1,500 or 2,250 mg/kg of Thionex® applied to clipped skin of pregnant rats (number per exposure group was not clearly reported) on gestation day 1 resulted in the death of at least two females, but no maternal deaths were reported at ≤1,000 mg/kg (EI Dupont deNemours & Co. 1973).
Similar differences in lethality were observed between different sexes and species during slightly longer exposure periods to technical endosulfan (Hoechst 1985c, 1985d). Exposure for a total of 21 out of 30 days for 6 hours/day, 5 days/week, resulted in deaths in males treated with doses of 81 mg/kg/day and in females treated with doses of 27 mg/kg/day (Hoechst 1985c). In contrast, female guinea pigs appeared to be relatively resistant to endosulfan toxicity (Hoechst 1983b). Only 1 of 20 females died when exposed to 587 mg/kg/day for 6 hours/day, 3 days/week for 3 weeks, and it was unclear whether this death was treatment related. In the majority of these reports, the clinical signs observed prior to death (tremors, salivation, and convulsions) were similar to those seen following oral exposure to endosulfan (see Section 3.2.2.1).
All reliable LD50 and LOAEL values for death in each species and duration category are recorded in Table 3-3. In some studies, only the α- or β-isomer of endosulfan was tested. In such cases, a notation regarding the specific isomer tested is included in the effect description.
3.2.3.2. Systemic Effects
The primary systemic targets of endosulfan toxicity in animals following dermal exposure are the liver and kidney. Adverse hematological effects have also been observed following dermal administration of endosulfan. No studies were located regarding musculoskeletal effects in humans or animals after dermal exposure to endosulfan.
The highest NOAEL value and all reliable LOAEL values for systemic effects in each species and duration category are recorded in Table 3-3. In some studies, only the α- or β-isomer of endosulfan was tested. In such cases, a notation regarding the specific isomer tested is included in the effect description.
Respiratory Effects. Increased occurrence of dyspnea and increased respiratory rate were noted in 18 agricultural workers in India who applied endosulfan without protective equipment (both dermal and inhalation exposures probably occurred) (Chugh et al. 1998).
Dyspnea and decreased respiration were observed in female rabbits prior to death following a single dermal application of 225 mg/kg of technical endosulfan (Gupta and Chandra 1975). It is unclear whether similar effects were observed at lower doses in this study. Irregular respiration was also observed in male and female rats as the result of exposure for 6 hours/day for 5 days to β-endosulfan at doses of 16 mg/kg/day (females) and 250 mg/kg/day (males) (Hoechst 1989b). These doses were the highest doses at which no deaths were observed. Acute congestion of the lungs with dilation of alveolar capillaries was observed at necropsy of animals that died as the result of exposure to doses ≥31.25 mg/kg/day (females) and ≥500 mg/kg/day (males) in this study. Congestion of the lungs was also observed at necropsy of rats dying as the result of 30-day, 6-hour/day, 5-day/week exposures to endosulfan at 81 mg/kg/day (males) and 27 mg/kg/day (females) technical endosulfan (Hoechst 1985c). It is probable that these effects are a result of generalized effects on central nervous system activity and attendant sequelae.
Table 3-3
Levels of Significant Exposure to Endosulfan - Dermal.
Cardiovascular Effects. Both tachycardia and bradycardia were noted among 18 agricultural workers in India who applied endosulfan without protective equipment (both dermal and inhalation exposures probably occurred) (Chugh et al. 1998).
Blood vessels were congested and cardiac ventricles were distended with blood in rats that died as the result of exposure to 81 mg/kg/day (males) and 27 mg/kg/day (females) technical endosulfan for 6 hours/day, 5 days/week for 30 days (Hoechst 1985c). However, it is unclear whether these effects were due to a direct action of endosulfan on the blood vessels and heart or were a result of a more general toxic insult (e.g., convulsions). The respective NOAELs for males and females were 27 and 9 mg/kg/day.
Gastrointestinal Effects. Abdominal discomfort after meals, nausea, and vomiting were noted in 18 agricultural workers in India who applied endosulfan without protective equipment (both dermal and inhalation exposures probably occurred) (Chugh et al. 1998). Another study reported gastrointestinal effects in 22 cases of acute poisoning of subjects spraying cotton and rice fields (Singh et al. 1992). Nausea, vomiting, pain in the abdomen, and diarrhea were among the signs and symptoms observed. Singh et al. (1992) assumed that exposure was mainly by the dermal route since subjects who sprayed the rice fields and who suffered cuts over the legs with the sharp leaves of the rice plants exhibited more severe toxicity.
Diarrhea was observed in rats exposed 6 hours/day for 5 days to both lethal and sublethal doses of β-endosulfan (≥250 mg/kg/day for males and ≥16 mg/kg/day for females) (Hoechst 1989b). Autopsy of animals from this study revealed that the mesenteric blood vessels of one of the surviving females exposed to 16 mg/kg/day were distended with blood, and that the small intestines of animals dying as a result of exposure were filled with a reddish fluid (500 mg/kg/day for males and 31.25 for mg/kg/day females). In contrast, no treatment-related effects were revealed by routine gross and histopathological examination of gastrointestinal tissues (stomach, small and large intestines, and pancreas) from rats exposed to doses of 27 mg/kg/day (females) and 81 mg/kg/day (males) technical 6 hours/day, 5 days/week for 30 days (Hoechst 1985c).
Hematological Effects. Normal hemoglobin, hematocrit, white blood cell count, and differential and sedimentation rate were observed in a 35-year-old agricultural pilot approximately 8 hours after a 45-minute dermal exposure (with presumed concurrent inhalation exposure) when his clothing became soaked in endosulfan and methomyl (Cable and Doherty 1999).
Mixed results have been obtained in studies examining hematological effects of dermal exposure to endosulfan in rats. Although decreased hemoglobin was observed in male rats following daily application of 18.75 mg/kg of an unspecified endosulfan formulation for 30 days (Dikshith et al. 1988), similar results have not been observed in female rats or in male rats at similar doses in other studies. For example, no hematological parameters were adversely affected following exposure of females to doses of 32 mg/kg/day for 30 days (Dikshith et al. 1988). In addition, no adverse effects on routine hematological parameters were observed following exposure of rats for 6 hours/day, 5 days/week for 30 days to endosulfan doses of 12–192 mg/kg/day (males) and 3–48 mg/kg/day (females) technical endosulfan (Hoechst 1985d). Similarly negative results were obtained in a comparable 30-day rat study using slightly lower doses of technical endosulfan (Hoechst 1985c). Since Wistar rats were used in the studies by Dikshith et al. (1988) and Hoechst (1985c, 1985d), the different results may be related to the use of different endosulfan formulations: technical grade in the studies by Hoechst (1985c, 1985d) and unknown in the study by Dikshith et al. (31988).
Musculoskeletal Effects. No studies were located regarding musculoskeletal effects in humans or animals after dermal exposure to endosulfan.
Hepatic Effects. Normal serum liver function tests (unspecified) were observed in a 35-year-old agricultural pilot approximately 8 hours after a 45-minute dermal exposure (with presumed concurrent inhalation exposure) when his clothing became soaked in endosulfan and methomyl (Cable and Doherty 1999). Liver function was normal in a 2-year-old girl who developed generalized tonic-clonic seizures after endosulfan was applied on her head to remove lice (Jindal and Sankhyan 2012). The specific liver tests conducted were not mentioned.
Distinct hepatotoxicity has been observed in animal studies following acute-duration exposure to large dermal doses of endosulfan. The livers of female rabbits that survived a single dermal application of 100 mg/kg of technical endosulfan exhibited microscopic evidence of congestion, dilation of sinusoids, hepatocellular degeneration, hyperplastic Kupffer cells, focal necrosis, and portal tract and bile duct proliferation (Gupta and Chandra 1975). In addition, necropsy of rats that died following exposure to doses of technical endosulfan ≥250 mg/kg/day (males) and 31.25 mg/kg/day (females) for 6 hours/day for 5 days revealed darkly discolored livers (Hoechst 1989b).
Subchronic dermal exposures to slightly lower doses of endosulfan have been associated with more mild toxicity and adaptive changes. For example, histopathological examination of livers from male and female rats exposed to doses of 9 mg/kg/day technical endosulfan 6 hours/day, 5 days/week for 30 days revealed slight fatty changes and an increased incidence of cellular hypertrophy and division (Hoechst 1985c). Similar changes were not observed in a repeat 30-day study at 12 or 192 mg/kg/day technical endosulfan in males or 48 mg/kg/day in females (Hoechst 1985d). However, dermal application of 18.75 mg/kg/day of an unspecified endosulfan formulation to male rats for 30 days resulted in decreases in hepatic levels of ALT and AST and increases in serum levels of AST, ALT, and alkaline phosphatase, but no changes in relative organ/body weight or other gross or histopathological evidence of liver damage (Dikshith et al. 1988). Effects in female rats at doses between 9.83 and 32 mg/kg/day were limited to decreases in hepatic AST and ALT and increases in protein, hepatic alkaline phosphatase, and lactate dehydrogenase. The hepatic effects of long-term dermal exposure to endosulfan cannot be evaluated because of lack of data.
Renal Effects. Renal function was normal in a 2-year-old girl who developed generalized tonic-clonic seizures after she received applications of endosulfan on her head to remove lice (Jindal and Sankhyan 2012). The specific renal tests conducted were not mentioned.
The kidneys of female rabbits that received a single dermal application of 100 mg/kg of technical endosulfan exhibited shrunken glomerular tufts, thickened Bowman’s capsules, and necrosis of the tubular epithelial cells (Gupta and Chandra 1975). However, daily application of up to 192 mg/kg/day (males) or 48 mg/kg/day (females) to the skin of rats for 30 days had no effect on kidney weight or histopathology (Dikshith et al. 1988; Hoechst 1985c, 1985d). The discrepancy may reflect species differences, differences in the application vehicle, or different endosulfan formulations. The renal effects of long-term dermal exposure to endosulfan cannot be evaluated because of lack of data.
Endocrine Effects. No studies were located regarding endocrine effects in humans after dermal exposure to endosulfan.
The adrenals of rabbits applied a single dermal dose of 100 mg/kg of technical endosulfan exhibited microscopic changes, including swollen cells with foamy cytoplasm and eccentric nuclei (Gupta and Chandra 1975). Also, release of lipids from the adrenal cortex was observed in rats that died following daily application of 81 mg/kg/day technical endosulfan (males) and 27 mg/kg/day (females) to the skin for 6 hours/day, 5 days/week for 30 days (Hoechst 1985c). However, daily applications of up to 62.5 mg/kg/day (males) or 32 mg/kg/day (females) of an unspecified endosulfan formulation to the skin of rats for 30 days had no effect on adrenal weight or histopathology (Dikshith et al. 1988).
Dermal Effects. No studies were located regarding dermal effects in humans after dermal exposure to endosulfan.
Mild dermal irritation has been observed in two studies following application of highly toxic amounts of endosulfan to the skin. Daily application of β-endosulfan to rat skin for 6 hours/day for 5 days resulted in slight-to-moderate erythema, slight edema, and dry, rough, and scaling skin at 62.5 mg/kg/day in females and 250 mg/kg/day in males (Hoechst 1989b). However, daily application of up to 48 mg/kg/day technical endosulfan (females) or 192 mg/kg/day (males) to the skin of rats for 30 days (5 days/week, 6 hours/day) caused no apparent skin irritation (Hoechst 1985c, 1985d). Dermal application of 587 mg/kg/day of technical endosulfan 3 days/week, 6 hours/day for 3 weeks caused no erythema or edema in guinea pigs (Hoechst 1983b).
Ocular Effects. No studies were located regarding ocular effects in humans after dermal exposure to endosulfan.
Limited information was available regarding ocular irritation by endosulfan. An unspecified amount of a 20% aqueous suspension of endosulfan instilled in the eyes of rabbits did not produce any ocular irritation or congestion (Gupta and Chandra 1975).
Metabolic Effects. Serum electrolytes and blood gases were normal in a 2-year-old girl who developed generalized tonic-clonic seizures after she received applications of endosulfan on her head to remove lice (Jindal and Sankhyan 2012).
3.2.3.3. Immunological and Lymphoreticular Effects
The only study located regarding immunological effects in humans after dermal exposure to endosulfan was an account of the results of patch tests on the backs of 14 farm workers with work-related dermatitis and 8 controls that were not exposed to pesticides (Schuman and Dobson 1985). Skin sensitization was not observed in any of the subjects following a 48-hour, closed-patch exposure to an unspecified amount of 0.1% endosulfan in petrolatum.
Extremely limited information was available regarding immunological effects of endosulfan in animals following dermal exposures. No sensitization was observed after a challenge application of 587 mg/kg/day technical endosulfan to female guinea pigs 16 days following a 6-hour/day, 3-day/week, 3-week exposure to this dose (Hoechst 1983b). In addition, no effect on thymus weight was reported following a 30-day (6 hours/day, 5 days/week) exposure to concentrations of up to 81 mg/kg/day technical endosulfan in males and 27 mg/kg/day in females (Hoechst 31985c). However, one of the male rats that died following exposure to 1,000 mg/kg/day β-endosulfan, 6 hours/day for 5 days had a spleen that was reduced in size (Hoechst 1989b); however, it is unclear whether the reduction in size was an immunotoxic effect or due to some other more generalized toxic insult.
The highest NOAEL and all reliable LOAEL values for immunological effects in rats or guinea pigs following acute- or intermediate-duration dermal exposures are recorded in Table 3-3. In some studies, only the α- or β-isomer of endosulfan was tested. In such cases, a notation regarding the specific isomer tested is included in the effect description.
3.2.3.4. Neurological Effects
As indicated in the section on inhalation exposure, neurotoxicity is the primary effect observed in humans following occupational exposure to endosulfan. Since dermal exposures may comprise a substantial portion of occupational exposure to endosulfan, the results presented in Section 3.2.1.4 are repeated here, along with reports of human exposures that were primarily dermal. Convulsions were reported in nine individuals exposed to the endosulfan-containing insecticide, Thiodan®, during bagging (Ely et al. 1967). In addition, a case of long-term, possibly permanent brain damage in an industrial worker was attributed by Aleksandrowicz (1979) to endosulfan exposure. This worker was exposed while cleaning vats that contained residues of endosulfan solution. The acute phase of the poisoning was manifested by repeated convulsions and impaired consciousness. After recovery, the patient became disoriented and agitated.
Two years later, he exhibited cognitive and emotional deterioration, memory impairment, and impairment of visual-motor coordination manifested by an inability to perform small tasks. However, modest alcohol consumption (1 L of wine/week) may have been a contributing factor directly on the brain or by decreasing metabolism of endosulfan in the liver. A 35-year-old male agricultural pilot experienced nausea, weakness, coldness, and blurred vision after 30 continuous minutes of dermal exposure when his clothes became soaked in endosulfan and methomyl, and tonic-clonic seizures 6 hours after a total of 45 minutes of dermal exposure (with presumed concurrent inhalation exposure) (Cable and Doherty 1999). Serum cholinesterase was within the normal range at 30 hours postexposure. A computed tomography (CT) scan showed no abnormalities, and the patient was discharged after 2 days of neurological observation, but three serial outpatient electroencephalographs (EEGs) showed a persistent nonspecific epileptic focus in the cerebral frontal lobes. It should be noted that methomyl may have contributed to some of the toxic signs reported. In another study, dizziness, nausea, confusion and irritability, muscle twitching, tonic/clonic convulsions, and conduction defects were noted in 18 agricultural workers in India who applied endosulfan without protective equipment (both dermal and inhalation exposures probably occurred) (Chugh et al. 1998). Jindal and Sankhyan (2012) reported the case of a 2-year-old girl who was brought to the emergency room with a history of continuous generalized tonic clonic seizures after her mother applied endosulfan on the head 2 hours prior to the symptoms to remove head lice. After treating the seizures with diazepam and decontaminating the shaved head with soap and water, the girl was asymptomatic 3 days after the episode. Limitations associated with the occupational reports include lack of quantitative exposure data, lack of data on the duration of exposure, the possibility of multiple routes of exposure (i.e., oral and dermal as well as inhalation), and possible concurrent exposure to other chemicals. Therefore, this information can only provide qualitative evidence of neurotoxicity associated with dermal exposure to endosulfan in humans.
Twenty-two cases of endosulfan poisoning were reported in people exposed while spraying cotton and rice fields; the dermal route of exposure was assumed to be the primary route of exposure (Singh et al. 1992). The assumption was based on the fact that those spraying rice fields and who suffered cuts over the legs with the sharp leaves on the rice plants exhibited the more severe toxicity. Three out of the 22 cases exhibited tremors and 11 presented convulsions; all patients recovered.
Central nervous system stimulation similar to that reported for occupational exposure is seen following acute dermal exposure to endosulfan in experimental animals. The spectrum of effects includes hyperexcitability, tremors, decreased respiration, tonic-clonic convulsions, and ultimately death (Gupta and Chandra 1975; Hoechst 1989b; Nicholson and Cooper 1977). In rats, the lowest doses associated with these effects were 16 mg/kg/day in females and 250 mg/kg/day in males during a 6-hour/day, 5-day exposure regimen (Hoechst 1989b).
Similar signs of central nervous system stimulation were observed following exposure to doses of endosulfan as low as 48 mg/kg/day technical endosulfan (females) and 81 mg/kg/day (males) during a 6-hour/day, 5-day/week, 30-day exposure period (Hoechst 1985c, 1985d). Diffuse edema was also observed in the brains of males at the 81-mg/kg/day exposure level. However, daily application of up to 62.5 mg/kg/day (males) or 32 mg/kg/day (females) of an unspecified endosulfan formulation to the skin of rats for 30 days had no effect on brain weight or histopathology (Dikshith et al. 1988). No information was found regarding neurological effects of long-term dermal exposure to endosulfan.
The highest NOAEL and all reliable LOAEL values for neurological effects in rats following acute- and intermediate-duration dermal exposures are recorded in Table 3-3. In some studies, only the α- or β-isomer of endosulfan was tested. In such cases, a notation regarding the specific isomer tested is included in the effect description.
3.2.3.5. Reproductive Effects
No studies were located regarding reproductive effects in humans after dermal exposure to endosulfan.
Limited information was available regarding reproductive effects in animals following dermal exposures to endosulfan. No effects on the reproductive organs were observed during routine gross and histopathological examination following exposure of rats to doses of 81 mg/kg/day technical endosulfan (males) or 27 mg/kg/day (females) for 6 hours/day, 5 days/week for 30 days (Hoechst 1985c). Also, daily application of up to 62.5 mg/kg/day (males) or 32 mg/kg/day (females) of an unspecified endosulfan formulation to the skin of rats for 30 days had no effect on reproductive organ histopathology (Dikshith et al. 1988).
3.2.3.6. Developmental Effects
No studies were located regarding developmental effects in humans after dermal exposure to endosulfan.
An unspecified number of pregnant rats were applied a single dermal dose of 670 or 1,000 mg/kg Thionex® on clipped skin on gestation day 1, and exencephaly was observed in five and three pups, respectively (the total number of live pups at these dose levels was not clearly indicated) (EI Dupont deNemours & Co. 1973). Maternal death was reported at higher dose levels (1,500 and 2,250 mg/kg), no effects were reported at lower dose levels (0 and 450 mg/kg), and no increase in embryolethality was observed at any dose level. Further study details were not provided.
3.2.3.7. Cancer
No studies were located regarding cancer in humans or animals after dermal exposure to endosulfan.
3.3. GENOTOXICITY
Endosulfan has been evaluated for genotoxicity in a variety of in vivo and in vitro assays. As summarized in Tables 3-4 and 3-5, the results of these assays have been mixed, but the majority of mutagenicity tests reported positive results. Certain studies were unsatisfactory, as indicated below.
DNA damage in mononuclear leukocytes, as measured with the alkaline comet assay, was significantly increased in two of four French agricultural workers on the day following the application of pesticide mixtures, including endosulfan, compared to levels of DNA damage prior to application (Lebailly et al. 1998). However, the contribution of endosulfan to the observed effect is uncertain because of co-exposure to fungicides, herbicides, and other insecticides. Evaluations for micronuclei in human peripheral blood lymphocytes provided mixed results, depending on the analytical method used. Both positive (Falck et al. 1999) and negative (Scarpato et al. 1996a, 1996b; Venegas et al. 1998) results were obtained in peripheral lymphocyte micronucleus studies in workers who applied various pesticides, including endosulfan. No increase over control levels was observed in the frequency of micronuclei in peripheral blood lymphocytes of Chilean pesticide sprayers, using the cytochalasin-B method of arresting cytokinesis (Venegas et al. 1998), although endosulfan was reportedly applied by the workers only 3.7% of the time. In Italian greenhouse workers who applied a variety of pesticides including endosulfan, the frequency of micronuclei was increased compared to controls in an assay that used the 5-bromodeoxyuridine DNA-labeling technique (Falck et al. 1999), but not in an assay utilizing cytochalasin-B to arrest cytokinesis (Scarpato et al. 1996a, 1996b). No increase in chromosomal aberrations or sister chromatid exchanges was seen in greenhouse workers in Italy who were exposed to complex mixtures of pesticides that included endosulfan (Scarpato et al. 1996a, 1996b, 1997). Similarly, a study of 50 mother-infant pairs from an agricultural community in Mexico reported no correlation between frequency of micronucleus or DNA damage and levels of organochlorine pesticides (endosulfan among them) in maternal or cord blood (Alvarado-Hernandez et al. 2013). The mixed results in human genotoxicity assays should be treated with caution because coexposure to a variety of other chemicals occurred in each study and the exposure levels of endosulfan were not reported. For these reasons, these studies are not included in Table 3-4.
Table 3-4
Genotoxicity of Endosulfan In Vivo.
Table 3-5
Genotoxicity of Endosulfan In Vitro.
The induction of genotoxic effects in animals following in vivo exposure to endosulfan has been evaluated using the chromosomal aberration test in somatic and germinal cell systems of rats (Dikshith et al. 1978), mice (Kurinnyi et al. 1982; Usha Rani and Reddy 1986), and hamsters (Dzwonkowska and Hubner 1986), the bone marrow micronucleus tests in mice (Usha Rani et al. 1980; Yaduvanshi et al. 2012) and rats (Manjula et al. 2006), and the sex-linked recessive lethal mutation test in Drosophila (Velazquez et al. 1984). Endosulfan enhanced chromosomal aberrations in mouse spermatocytes 60 days post-treatment (Usha Rani and Reddy 1986), in mouse bone marrow (Kurinnyi et al. 1982), and in hamster bone marrow (Dzwonkowska and Hubner 1986). It also and induced micronuclei in rat bone marrow following 60 days of treatment (Manjula et al. 2006), but failed to induce chromosomal aberrations in the spermatogonial cells of rats (Dikshith and Datta 1978; Dikshith et al. 1978).
In male rats, acute exposure to doses of up to 22 mg/kg/day of endosulfan for 5 days did not induce chromosomal aberrations in either bone marrow (somatic) or spermatogonial (germinal) cells. The ratio of mitotic index and frequency of chromatid breaks in the two cell types had no correlation with the doses tested and were not significantly different from the control group (Dikshith and Datta 1978). In mice, a statistically significant increase in chromosomal aberrations was observed 60 days after treatment with oral doses of 6.4 mg/kg/day of endosulfan for 5 days (Usha Rani and Reddy 1986). However, mice administered 21.7 mg/kg/day endosulfan by gavage in water on 2 consecutive days did not show a statistically significant increase in the frequency of micronuclei in bone marrow erythrocytes 6 hours after the second dosing (Usha Rani et al. 1980). In a more recent study, intraperitoneal administration of a single dose of 4.4 or 8.75 mg/kg technical endosulfan to male mice significantly increased the frequency of micronuclei in bone marrow in a dose-related manner 24 hours after dosing (Yadavanshi et al. 2012). The apparent discrepancy between the results from the Usha Rani et al. (1980) and Yadavanshi et al. (2012) studies may be due to the different modes of administration of endosulfan. It should be noted also that the purity and/or formulation of endosulfan used in the former study was not specified.
Oral administration of 11.6 mg/kg/day of endosulfan to rats for up to 30 days also failed to induce chromosomal damage in bone marrow and spermatogonial cell systems, but it is not known how soon after treatment the animals were killed. As shown in mouse studies (Usha Rani and Reddy 1986), a latency period of 60 days was required to see chromosomal aberrations in spermatogonia. However, relatively significant changes were observed for mitotic indices (Dikshith et al. 1978).
In contrast to the 2-day acute mouse study by Usha Rani et al. (1980), a subchronic gavage study in rats administered 3, 6, 9 and 12 mg/kg/day endosulfan for 60 days resulted in increased micronuclei in polychromatic erythrocytes (PCEs) and normachromatic erythrocytes (NCEs) (Manjula et al. 2006). When treated in conjunction with vitamin C, there was a decrease in PCEs and NCEs, which could be attributed to the antimutagenic effect of vitamin C itself. Endosulfan also increased the cytogenetic activity (aberrant metaphases) of mouse bone marrow (L’Vova 1984). In rats, relatively significant changes in mitotic indices (decreased metaphases) in bone marrow and spermatogonial cells have been observed (Dikshith et al. 1978). Endosulfan induced micronuclei in rats following oral administration at 3, 6, 9, and 12 mg/kg/day for 60 days (Manjula et al. 2006); however, it did not induce micronuclei in mice fed 21.7 mg/kg/day for 2 days (Usha Rani et al. 1980). Endosulfan was positive in vivo for the induction of sex-linked recessive lethals and sex-chromosome loss, which indicates that endosulfan is an efficient mutagen in Drosophila (Velazquez et al. 1984). The incidence of in vitro sister chromatid exchanges was increased at least 5-fold compared to controls in cultured postimplantation rat embryos both after direct exposure to endosulfan in powder or microcapsular form, and after cultivation in serum of rats that had been exposed intraperitoneally with either the powder or microcapsulated endosulfan (Popov et al. 1998).
Endosulfan is toxic to yeast but is also mutagenic without activation (Yadav et al. 1982). In vitro, endosulfan induced reverse mutations and mitotic gene conversion and increased the percentage of aberrant colonies in Saccharomyces cerevisiae but did not induce mitotic cross-overs (Yadav et al. 1982). This indicates that endosulfan is capable of inducing chromosome breakage and loss. Endosulfan also induced cytotoxic activity (significant increase in the number of crossover colonies) in the yeast strain S. cerevisiae T2 (deficient in repair system), but not in S. cerevisiae T1 (L’Vova 1984).
Endosulfan, its isomers, and metabolites tested positive for mutagenicity in Salmonella typhimurium strains TA97, TA98, TA100, and TA102 with and without metabolic activation in one study (Bajpayee et al. 2006) and tested positive in strain TA98 without activation in two different studies (Antherieu et al. 2007; Yaduvanshi et al. 2012). However, in other studies, no mutagenic activity was demonstrated in S. typhimurium strains TA97a, TA98, TA100, TA1535, TA1537, or TA1538 without activation (Moriya et al. 1983; Pednekar et al. 1987) or in Escherichia coli WP2 without activation (Moriya et al. 1983). Endosulfan also tested negative in the Salmonella mutagenicity test with or without activation with S9 liver homogenate (Dorough et al. 1978). A forward mutation assay in E. coli K12 showed an endosulfaninduced increase in mutations from ampicillin-sensitive to ampicillin-resistant (Chaudhuri et al. 1999).
Prophage λ was also induced by endosulfan in E. coli, and umu gene expression was induced by endosulfan exposure in S. typhimurium (Chaudhuri et al. 1999).
In cultured human cells, endosulfan was reported positive in sister chromatid exchanges in human lymphoid cells exposed both with and without activation (Sobti et al. 1983) and in HepG2 (human liver hepatoblastoma) cells exposed without activation (Lu et al. 2000). Positive results were also reported in human lymphocyte, HaCaT, and HepG2 cells for DNA damage (strand breaks) in comet assays without metabolic activation (Antherieu et al. 2007; Bajpayee et al. 2006; Jamil et al. 2004). In addition, DNA damage was observed in peripheral blood mononuclear cells (as indicated by the presence of oxidative stress) without activation (Ahmed et al. 2011), and endosulfan was found to induce the formation of DNA adducts in human HepG2 cells, which strongly correlated with high induction of CYP3A gene expression (Dubois et al. 1996). Negative results were reported for mitotic recombination in human lymphocyte cells (L’Vova 1984) and for unscheduled DNA synthesis (UDS) in human lung carcinoma (A 549) cells using liquid scintillation counting (Hoechst 1988d), but the study was inconclusive because no evidence that DNA synthesis was inhibited was presented, and high background levels compromised the sensitivity of the assay.
In mammalian cells, endosulfan produced forward locus gene mutations in mouse lymphoma cells (McGregor et al. 1988) and induced DNA damage in a comet assay in Chinese hamster ovary (CHO) cells in the absence of S9 mix (Bajpayee et al. 2006). Endosulfan also induced the formation of DNA adducts in fetal rat hepatocytes in the presence of S9 mix (Dubois et al. 1996). However, exposure did not induce UDS in primary rat hepatocytes (Hoechst 1984d) and was negative in a micronucleus assay in sheep peripheral lymphocytes (Kovalkovicova et al. 2001)
In summary, genotoxicity studies of endosulfan have provided evidence that this compound can be mutagenic and clastogenic, and that it can induce effects on cell cycle kinetics in different mammalian species. It induced chromosomal aberrations and gene mutations in mice, hamsters, sheep, and Drosophila. However, some of these data may be suspect because some formulations of endosulfan have contained epichlorohydrin, a known genotoxic chemical, as a stabilizer (Hoechst 1990). It should be noted that humans may also be exposed to epichlorohydrin along with endosulfan.
3.4. TOXICOKINETICS
Data regarding toxicokinetics of endosulfan in humans are limited to information from cases of accidental or intentional ingestion of the chemical and cases of occupational exposure in the workplace where inhalation and/or dermal contact may have occurred. The evidence that humans absorb endosulfan by the inhalation and/or dermal routes of exposure is only indirect. Conclusive evidence exists regarding absorption through the gastrointestinal tract, although the extent of absorption is not known. Animals absorb endosulfan by the inhalation, oral, and dermal routes of exposure. Nearly 80% of the administered oral dose may be absorbed and nearly 20% of a dermal dose may be absorbed; the role of the administration vehicle has not been studied. Autopsy data in humans suggest that endosulfan may accumulate in the liver, kidney, and brain at least in the short-term. Data in animals also suggest that following initial distribution to adipose tissue, endosulfan accumulates in liver and kidney, and that the α-isomer accumulates to a greater extent than the β-isomer. There is no information on the metabolism of endosulfan in humans. In animals, endosulfan is metabolized predominantly to polar and nonpolar metabolites by microsomal enzymes. Endosulfan and metabolites have been detected in the urine of humans after ingestion of the chemical. In animals, the main route of excretion of unchanged parent compound and metabolites is in the feces.
3.4.1. Absorption
Health effects in humans and animals provide indirect evidence of absorption of endosulfan following oral, inhalation, and dermal exposures. Endosulfan and metabolites have been detected in tissues of humans and animals following exposure, thus, providing qualitative evidence that endosulfan is absorbed. Endosulfan residues were found in fat of hospitalized Spanish children, indicating that absorption occurs in children (Olea et al. 1999), but no studies were located regarding known or suspected differences between children and adults with respect to endosulfan absorption.
3.4.1.1. Inhalation Exposure
No studies were located regarding the absorption of endosulfan following inhalation in humans and animals. However, Ely et al. (1967) described nine case reports of occupational exposure to endosulfan resulting in neurological effects. Also, neurological effects have been observed in rats following inhalation exposure to endosulfan (Hoechst 1983a). These studies describing the occurrence of neurotoxicity following inhalation exposure to endosulfan provide indirect evidence that endosulfan is absorbed by both humans and animals by this exposure route.
3.4.1.2. Oral Exposure
Although no specific studies were located that quantified the absorption of endosulfan in humans, the onset of seizures approximately 1 hour following ingestion of endosulfan suggests that absorption occurs in the gastrointestinal tract (Karatas et al. 2006; Moon and Chun 2009). Absorption of endosulfan was also evidenced by the appearance of endosulfan in samples of the liver and kidney obtained from poisoning victims at autopsy (Demeter and Heyndrickx 1978; Demeter et al. 1977). In the fatal cases reported by Blanco-Coronado et al. (1992) and Lo et al. (1995), there was little doubt that death was caused by effects triggered by endosulfan. In one fatality, the concentration in the blood was 2.85 mg/L, and this patient died 8 days after ingesting endosulfan accidentally mixed with food (Blanco-Coronado et al. 1992). In five cases of people who survived and eventually recovered, the concentration of endosulfan determined in the blood on admission to the hospital ranged from 0.29 to 0.67 mg/L (Blanco-Coronado et al. 1992). Yavuz et al. (2007) reported that the blood concentration of endosulfan at autopsy of a man who ate a contaminated homemade pastry was 3.15 mg/L. In a lethal case of a man who ingested 500 mL of Thiodan® 35 containing 180 g endosulfan, the peak concentration in blood was approximately 0.86 mg/L 28 hours after ingestion (Eyer et al. 2004). Another case in the same study had a peak blood concentration of endosulfan of 0.12 mg/L 25 hours after ingesting 35 mL of an organochlorine fluid containing 12.3 g endosulfan; this subject survived (Eyer et al. 2004). α-Endosulfan, β-endosulfan, and/or endosulfan sulfate were present in the blood and urine of a 43-year-old man for at least 91 hours after he intentionally ingested approximately 260 mg/kg endosulfan, and the stomach contents contained 3,540 and 1,390 μg/kg of α-endosulfan and β-endosulfan, respectively, upon autopsy 4 days after exposure, indicating that absorption from the gut can occur over a prolonged period after a single oral exposure (Boereboom et al. 1998).
Evidence of the absorption of endosulfan following oral exposure has also been found in animal studies. In metabolic studies with 14C-endosulfan, approximately 65% of the administered radioactivity was recovered from the excreta and tissues of mice 24 hours after ingesting endosulfan in their diet (Deema et al. 1966). In descending order, the highest activities per gram of organ/excreta from two mice were as follows: feces, visceral fat, urine, tissues, respired air, and blood. In an experiment that involved cannulation of the bile duct, approximately 22, 13, and 47% of a 2-mg/kg oral dose of α-endosulfan, and 15, 10, and 29% of a 2 mg/kg oral dose of β-endosulfan were collected in the feces, urine, and bile, respectively, after 48 hours. This indicates that absorption could be as high as 78 and 85% for α- and β-endosulfan, respectively. The rats eliminated 88% of the α-endosulfan (75% in feces, 13% in urine) and 87% of the β-endosulfan (68% in feces, 19% in urine) within 5 days of oral administration (Dorough et al. 1978). A single oral dose of endosulfan given to sheep was almost completely excreted in the feces (50% of the radiolabel) or in the urine (40% of the radiolabel) within 22 days after administration (Gorbach et al. 1968). Administration of a single gavage dose of 5 mg/kg 14C-endosulfan to male rats resulted in rapid absorption through the gastrointestinal tract, as evidenced by an absorption rate constant of 3.07/hour (Chan et al. 2005). Blood concentration reached a maximum of 0.36 mg endosulfan equivalents/L approximately 2 hours after dosing. The terminal elimination half-life of the radioactivity in blood was 193 hours. These studies indicate that absorption occurs in humans and animals following ingestion of endosulfan.
3.4.1.3. Dermal Exposure
Evidence suggesting that humans absorb endosulfan through the skin was presented in a study by Singh et al. (1992), which briefly described 22 cases of acute poisoning among subjects spraying cotton and rice fields. The assumption of dermal absorption was based on the fact that subjects who sprayed rice fields and who suffered cuts over the legs caused by the sharp leaves of the rice plants showed the most severe toxicity. In another case report, serum endosulfan was 4 μg/L 30 hours after an agricultural pilot was exposed dermally (and probably also by inhalation) for approximately 45 minutes in clothing that was “heavily contaminated” with endosulfan and methomyl (Cable and Doherty 1999); the dermal exposure level was not estimated, and no other measures of tissue levels of endosulfan were obtained. Indirect evidence of dermal absorption was provided in the case of a 2-year-old girl who was brought to the emergency room with a history of continuous generalized clonic tonic seizures for 1 hour after her mother applied endosulfan on her head to remove head lice (Jindal and Sankhyan 2012). Neither the exposure concentration nor the levels of endosulfan in blood were available.
Indirect evidence indicates that dermal absorption occurs in animals. Calves dusted with a 4% dust formulation of endosulfan had neurological symptoms (tremors, twitching, convulsions) and died within a day after exposure (Nicholson and Cooper 1977). Neurological effects have also been reported in preclipped rabbits and rats after repeated application of endosulfan to the skin (Dikshith et al. 1988; Gupta and Chandra 1975). Dikshith et al. (1988) reported levels of α-, β-, and total endosulfan in liver, kidney, brain, testes, fatty tissue, and blood 30 days after dermal application of endosulfan.
One animal study provided direct evidence of absorption of endosulfan following dermal exposure by quantifying the rate and extent of dermal absorption in Sprague-Dawley rats (Hoechst 1986). A single dermal application of aqueous suspensions of 0.10, 0.76, and 10.13 mg/kg 14C-endosulfan to male rats resulted in binding of approximately 80% of the test material to the skin at all three dose levels, with the amount bound proportional to the amount applied (Hoechst 1986). After 10 hours, approximately 72% of the applied dose was bound to the skin and 8% of the applied dose was absorbed into the body. After 24 hours, approximately 25% of the bound material was absorbed into the body. Absorption rates were calculated, with the highest rates occurring within the first half hour after application. For the low-, middle-, and high-dose groups, the absorption rates at 0.5 hour were 2.8, 21.7, and 453.9 μg/cm2 of skin/hour, respectively, with the rates being proportional to the amount of endosulfan applied to the skin. The absorption rates decreased with time for all three dose groups. By 24 hours, the absorption rates were 0.1, 0.7, and 6.3 μg/cm2 of skin/hour for the low-, middle-, and high-dose groups, respectively (Hoechst 1986).
3.4.2. Distribution
Studies in animals and autopsy findings of endosulfan and metabolites in various tissues in humans suggest that absorbed endosulfan is most readily distributed to adipose and brain tissue, but that the liver and kidney may be longer-term repositories of endosulfan and its metabolites. No studies were located regarding known or suspected differences between children and adults with respect to endosulfan distribution.
3.4.2.1. Inhalation Exposure
No studies were located regarding the distribution of endosulfan in humans and animals after inhalation exposure to endosulfan.
3.4.2.2. Oral Exposure
α-Endosulfan, β-endosulfan, and the primary metabolite, endosulfan sulfate, have been detected in several human autopsy samples following acute ingestion. In a man who had ingested endosulfan in a single oral dose of approximately 260 mg/kg, postmortem tissue concentrations of α-endosulfan at 4 days postexposure were 4,105 μg/kg, 80 μg/kg, and 59 μg/kg in the fat, brain, and kidney, respectively; the concentration of β-endosulfan in the brain was 69 μg/kg; and the concentrations of endosulfan sulfate were 3,030 μg/kg, 1,350 μg/kg, and 390 μg/kg in the liver, brain, and kidney, respectively (Boereboom et al. 1998). In three other suicides cases, the following concentrations for combined isomers of endosulfan were found in autopsy specimens: blood, 4–8 ppm; liver 0.8–1.4 ppm; kidney, 2.4–3.2 ppm; and brain, 0.25–0.30 ppm (Coutselinis et al. 1978). No information was available on the amount of endosulfan ingested. It is apparent that the highest concentrations were detected in the kidney, liver, and blood. However, it was not possible to determine the specific levels that elicited systemic toxicity prior to death. An autopsy was performed on a 28-year-old man who ingested 20% endosulfan powder (12.4% α-, 8.1% β-) while drunk and was dead on arrival at the hospital. The autopsy revealed the following respective α and β-endosulfan concentrations: blood, 0.06 and 0.015 ppm; urine, 1.78 and 0.87 ppm; liver, 12.4 and 5.2 ppm; and kidney, 2.48 and 1.8 ppm. In another case, endosulfan sulfate was found in the liver at a concentration of 3.4 ppm (Demeter and Heyndrickx 1978; Demeter et al. 1977). In a case reported by Eyer et al. (2004), a man ingested 500 mL of Thiodan® 35 containing 180 g endosulfan and died 10 days later of multiorgan failure. One day after his death, the concentrations of endosulfan (α- plus β-isomers) were 5 μg/g in the bile, 7.5 μg/g in the brain, 26 μg/g in the liver, 0.8 μg/g in the lung, and 110 μg/g in the kidney.
Endosulfan has been detected in breast milk of women environmentally exposed to a number of contaminants in rural Kazakhstan (Lutter et al. 1998), indicating that transfer to children can occur during lactation; the endosulfan concentration in the breast milk was not reported. A study of Turkish urban primiparous women without occupational exposure reported means of 2 and 28 ng/g (lipid basis) for α- and β-endosulfan, respectively, in breast milk (Çok et al. 2011). A study of Spanish women reported concentrations of endosulfan and metabolites in breast milk ranging from 0.60 to 10.70 ng/mL for endosulfan diol and β-endosulfan, respectively (Cerrillo et al. 2005). Isomers and metabolites of endosulfan were detected in the fat of 30–40% of children from agricultural regions of Spain, demonstrating that endosulfan accumulates in adipose tissue of children after presumably repeated dietary exposure (Olea et al. 1999). The mean concentration of endosulfan and metabolites in the placenta from women from southern Spain ranged from 0.33 to 15.62 ng/g for endosulfan ether and endosulfan lactone, respectively (Cerrillo et al. 2005). Another study from the same region reported a geometric mean of 4.02 ng/g for total endosulfan in the placenta (Freire et al. 2011). Cerrillo et al. (2005) also reported concentrations in the range of 1.43–13.23 ng/mL for endosulfan and metabolites in blood from umbilical cord. Yet another study of women from the same region reported mean concentrations of 2.44 and 2.83 ng/mL for α- and β-endosulfan, respectively, in cord blood (Mariscal-Arcas et al. 2010). A study of Mexican women living in a rural area where agriculture is the main activity reported umbilical cord median concentrations of α- and β-endosulfan of 401 and 265 ng/g lipid, respectively (Alvarado-Hernandez et al. 2013).
The distribution of radioactivity 24 hours after a single oral exposure to a food pellet treated with α- and β-14C-endosulfan in male mice was as follows: feces > small intestine > urine > visceral fat > liver > kidney > expired carbon dioxide > blood (Deema et al. 1966). In a more recent study, administration of a single gavage dose of 5 mg/kg 14C-endosulfan to male rats resulted in the following relative amounts of mg of endosulfan equivalents/L in tissues 8 hours after dosing: gastrointestinal tract (20.28) > liver (5.52) > fat (3.61) > thyroid gland (2.50) > kidneys (1.83) > lung (1.31) > serum (0.75) > heart (0.42) > spleen (0.37) > blood (0.28) > brain (0.27) > testes (0.25) > muscle (0.18) (Chan et al. 2005). After administration of three doses of 5 mg/kg, radioactivity decreased in all tissues 25 hours after administration of the last dose.
In a 14-day feeding study with 0.25 mg/kg/day of radiolabeled α- or β-endosulfan, female albino rats had the highest 14C concentrations in liver and kidney (Dorough et al. 1978). The authors reported that endosulfan accumulated in fat tissues during the 14-day exposure period, then declined to undetectable levels by 7 days postexposure. Levels in the fat tissue never reached as high as those seen in the liver and kidney. Concentrations of α-endosulfan, β-endosulfan, and endosulfan sulfate in cattle fatally poisoned after a single exposure due to accidental ingestion of an unknown amount of endosulfan were reported by Braun and Lobb (1976). Respective total endosulfan residues for α-endosulfan, β-endosulfan, and endosulfan sulfate were 0.083, 0.065, and 4.23 ppm in the liver; 0.04, 0.024, and 1.06 ppm in the kidney; 0.031, 0.024, and 0.61 ppm in muscle; and 720, 550, and 0.0 ppm in rumen contents. The surviving calf had 0.025 ppm endosulfan in its blood.
Rats exposed daily via gavage to 5 or 10 mg/kg/day of endosulfan in peanut oil had plasma levels of 2.26 and 0.46 μg/mL for the α- and β-isomers, respectively (Gupta 1978). These levels were measured on the day after the termination of a 15-day gavage dosing regimen. Fifteen days after the last treatment, the plasma concentration of α-endosulfan was 0.05 μg/L, while the β-isomer was not detected. Endosulfan levels were twice as high in fatty tissues as in liver and kidney following 30 days of exposure of male and female Wistar rats to endosulfan (Dikshith et al. 1984). Thirty days of exposure to 11 mg/kg/day produced the highest level in the kidney, while the fatty tissue had a slightly lower concentration (Nath and Dikshith 1979). Male rats exposed daily for 60 days to 2.5 or 3.75 mg/kg/day of endosulfan containing α- and β-isomers in a ratio of 2:1 produced somewhat different distribution patterns for the two isomers (Ansari et al. 1984). For both doses, the highest concentration of the α-isomer was detected in the kidney followed by the epididymis, ventral prostate or spleen, testes, brain, and liver. In descending order, the highest levels of the β-isomer were found in the seminal vesicle, epididymis, heart, ventral prostate, spleen, and liver. Overall, the greatest amounts of both α- and β-isomers of endosulfan were located in the kidneys, seminal vesicle, and epididymis, with the liver having the least amount. Using gas chromatography-mass spectrometry, Chan and Mohd (2005) measured α-endosulfan, β-endosulfan, endosulfan sulfate, and endosulfan diol in plasma, kidneys, and liver from male rats after 15 daily gavage doses of 5 or 10 mg/kg technical endosulfan and after an additional 15-day period without treatment. None of the four chemicals was detected in plasma samples. After 15 days of dosing, α-endosulfan and β-endosulfan were detected in the kidneys, but not in the liver; neither one was detected in the liver or kidneys after the 15-day recovery period. Traces of endosulfan sulfate were detected in liver, but not in the kidneys, at both sampling times.
α-Endosulfan, β-endosulfan, and the endosulfan metabolites, endosulfan sulfate, endosulfan hydroxyether, endosulfan lactone, and endosulfan diol, were measured in blood, liver, and kidney from male rats consuming 34 or 68 mg/kg/day of endosulfan over 4 weeks and from male rats given a 30-day recovery from exposure (Hoechst 1987). Only trace amounts of endosulfan and its metabolites were found in the blood. The predominant substances found in the liver were endosulfan sulfate and endosulfan lactone. Trace amounts of α- and β-endosulfan were measured in the liver; however, there was substantial accumulation of α-endosulfan in the kidneys. Approximately 200 times more α-endosulfan than β-endosulfan was found in the kidney. The predominant metabolites of endosulfan found in the kidney were endosulfan sulfate and endosulfan lactone. Endosulfan-diol was also found, but at much lower concentrations. The amount of endosulfan found in the kidney decreased following a period free from exposure. By the end of the recovery period (4 weeks), α- and β-endosulfan and the endosulfan metabolites were reduced to trace levels in all organs (Hoechst 1987).
Information regarding transfer of endosulfan residues to offspring through breast milk is available from a study in lactating goats (Indraningsih et al. 1993). Goats were administered a daily dose of 1 mg/kg for 28 days and adults and kids were sacrificed at various times (days 1, 8, 15, and 21 posttreatment) after treatment ceased. With the exception of the kidneys, the highest concentrations of residues were recorded in the adults on day 1. In the kidneys, residues increased from day 1 to a maximum on day 8. On day 1, the concentration of residues in the kidneys, liver, and milk were 0.29, 0.20, and 0.02 mg/kg, respectively. No residues could be detected in milk on day 8. α-Endosulfan was the major residue in all tissues except for liver and fat, which contained mainly endosulfan sulfate. No endosulfan residues were detected in the tissues of kids except for α-endosulfan in the liver at a concentration of 0.0011 mg/kg on day 1. These results suggest rapid elimination of residues from tissues and limited transfer to offspring through breast milk. Approximately 1% of the radiolabel from administration of a single oral dose of 14C-endosulfan (65% α-isomer, 35% β-isomer) to milk sheep was recovered in the milk, primarily as endosulfan sulfate (Gorbach et al. 1968).
The distribution in animals after exposure to endosulfan indicates that the α-isomer of endosulfan accumulates throughout the body to a greater extent than the β-isomer (Ansari et al. 1984; Hoechst 1987). Endosulfan is distributed to the fatty tissues initially after exposure, while a greater accumulation of endosulfan reaches the kidney following prolonged exposure. Of all the metabolites of endosulfan, endosulfan sulfate appears to be the one that accumulates predominantly in the liver and kidneys (Hoechst 1987).
3.4.2.3. Dermal Exposure
Serum endosulfan was 4 μg/L at 30 hours after an agricultural pilot was exposed dermally (and probably also by inhalation) for approximately 45 minutes in clothing that was “heavily contaminated” with endosulfan and methomyl (Cable and Doherty 1999); the dermal exposure level was not estimated and no other measures of tissue levels of endosulfan were obtained. A study by Kazen et al. (1974) identified endosulfan residues on the hands of workers after relatively long periods free from exposure. Endosulfan residues were identified on the hands of one worker approximately 30 days after exposure and on the hands of one worker who had not used endosulfan during the preceding season.
Three animal studies were located regarding distribution of endosulfan in animals following dermal exposure (Dikshith et al. 1988; Hoechst 1986; Nicholson and Cooper 1977). Endosulfan was detected in the brain (0.73 ppm), liver (3.78 ppm), and rumen contents (0.10 ppm) of calves that died after dermal exposure to a dust formulation of endosulfan (Nicholson and Cooper 1977). Following a single dermal application of aqueous suspensions of 0.1, 0.83, and 10.13 mg/kg 14C-endosulfan to male Sprague-Dawley rats, low concentrations of endosulfan (ng/g levels) appeared in the blood and tissues (other than skin at and around the application site) after 1 hour (Hoechst 1986). The concentrations of endosulfan in the blood and tissues increased with the time of exposure and were proportional to the dose applied. The liver and kidney appeared to sequester radiolabel relative to the concentrations of radiolabel in the blood or fat. Endosulfan levels were approximately 10 times higher in the liver and kidney than in the fat, blood, and brain throughout the study (Hoechst 1986).
Tissue disposition of the α- and β-isomers has also been quantified in rats after intermediate-duration dermal application of technical-grade endosulfan for 30 days (Dikshith et al. 1988). Male rats were exposed to 18.8, 37.5, or 62.5 mg/kg/day; females were exposed to 9.8, 19.7, or 32.0 mg/kg/day. Fatty tissue contained the highest levels of both α- and β-isomers in both males and females. The levels of the α- and β-isomers, respectively, in animals exposed to the lowest doses was as follows: fatty tissue (0.26 and 0.15 ppb) > kidney (0.16 and 0.01 ppb) > blood (0.03 and 0.015 ppb) > liver (0.02 and 0.004 ppb) > brain (0.03 ppb and not detected) in male rats, and fatty tissue (2.4 and 5.8 ppb) > liver (0.50 and 1.2 ppb) > blood (0.56 and 1.2 ppb) > kidney (0.65 and 0.52 ppb) > brain (0.21 ppb and not detected) in female rats. Generally, these values increased with increased dose. The residue level of both isomers in fatty tissue was much higher in females (8.20–16.13 ng/g) than in males (0.42–0.62 ng/g).
3.4.2.4. Other Routes of Exposure
The distribution of endosulfan and endosulfan sulfate was evaluated in the brains of cats given a single intravenous injection of 3 mg/kg endosulfan (Khanna et al. 1979). Peak concentrations of endosulfan in the brain were found at the earliest time point examined (15 minutes after administration) and then decreased. When tissue levels were expressed per gram of tissue, little differential was observed in distribution among the brain areas studied. However, if endosulfan levels were expressed per gram of tissue lipid, higher initial levels were observed in the cerebral cortex and cerebellum than in the spinal cord and brainstem. Loss of endosulfan was most rapid from those areas low in lipid. Endosulfan sulfate levels peaked in the brain at 1 hour postadministration. In contrast, endosulfan sulfate levels in liver peaked within 15 minutes postadministration. The time course of neurotoxic effects observed in the animals in this study corresponded most closely with endosulfan levels in the central nervous system tissues examined.
3.4.3. Metabolism
No information is available on the metabolism of endosulfan in adult humans or children. Endosulfan is readily metabolized in animals following exposure (Deema et al. 1966; Dorough et al. 1978; Gorbach et al. 1968). It exists in two stable stereoisomeric forms, which can be converted to endosulfan sulfate and endosulfan diol (WHO 1984). These can be further metabolized to endosulfan lactone, hydroxyether, and ether. Using human liver microsomes, Casabar et al. (2006) showed that the formation of endosulfan sulfate from α-endosulfan is catalized primarily by cytochromes CYP2B6 and CYP3A4. A similar study by Lee et al. (2006) reported that the stereoselective formation of endosulfan sulfate from α-endosulfan is mediated by CYP2B6, CYP3A4, and CYP3A5, and that from β-endosulfan is mediated by CYP3A4 and CYP3A5. Figure 3-3 shows the pathway for the degradation of endosulfan. Dorough et al. (1978) indicated that the major portion of residues in the excreta and/or tissues consisted of unidentified polar metabolites that could not be extracted from the substrate, whereas the nonpolar metabolites, including sulfate, diol, α-hydroxyether, lactone, and ether derivatives of endosulfan, represented only minor amounts. Excretion data from an acute dermal study in rats showed that, after 24 hours, a dose-related decrease in excretion occurred at higher doses, suggesting saturation of the metabolic pathway of endosulfan (see Section 3.4.4.3) (Hoechst 1986).

Figure 3-3
Proposed Metabolic Pathway for Endosulfan.
High concentrations of endosulfan sulfate were found primarily in the liver, intestine, and visceral fat 24 hours after mice were exposed to a single dose of 14C-endosulfan (Deema et al. 1966). Five days following a single oral administration of 14C-endosulfan to rats, the diol, sulfate, lactone, and ether metabolites were detected in the feces (Dorough et al. 1978). In sheep, endosulfan sulfate was detected in the feces, and endosulfan alcohol and α-hydroxyether were detected in the urine (Gorbach et al. 1968).
All of the metabolism studies indicate that the parent compound was also found to a large degree in the tissues and excreta. Similar conclusions can be drawn from the work of Gupta and Ehrnebo (1979), who found that almost half of the parent compound was excreted unchanged in rabbits after endosulfan was injected intravenously. The metabolites (e.g., endosulfan sulfate, endosulfan diol) were reported in tissues and excreta following longer exposures to endosulfan (Deema et al. 1966; Dorough et al. 1978). Based on the rapid appearance of endosulfan sulfate in the liver following intravenous administration of endosulfan, it may be concluded that the liver is a site of high metabolic activity in the conversion of endosulfan to endosulfan sulfate (Khanna et al. 1979).
Results of a study in which male rats were fed 34 or 68 mg/kg/day endosulfan over 30 days suggest that metabolism of endosulfan occurs in the kidney (Hoechst 1987). This feeding study was initiated to clarify findings from a previous 13-week feeding study with endosulfan in which a yellow discoloration was observed in the kidneys of rats fed diets containing up to 360 ppm. The results of the 30-day feeding study showed that endosulfan accumulates predominantly in the kidney during exposure and that storage of endosulfan in the kidney is reversible upon removal from exposure (Hoechst 1987). Histological examination of the kidney revealed granular pigmentation and an increase in the number and size of lysosomes in the cells of the proximal convoluted tubules in the kidneys (Hoechst 1987). These changes diminished appreciably during the 30-day recovery period. These lysosomal changes may suggest storage or metabolism of endosulfan in the kidneys (Hoechst 1987). The lysosomal sequestration of endosulfan may account for the yellow pigment seen in the kidneys in a previous toxicity study. The diminishing pigmentation and decreasing endosulfan concentrations, which occurred during the 30-day recovery period, suggest metabolism of the compound in the kidney (Hoechst 1987).
Evidence suggests that endosulfan can induce microsomal enzyme activity. Increased liver microsomal cytochrome P450 activity was observed in male and female rats after single and multiple administrations of endosulfan (Siddiqui et al. 1987a; Tyagi et al. 1984). Increased enzyme activity was observed in hepatic and extrahepatic tissues. Based on the increase in aminopyrine-N-demethylase and aniline hydroxylase activity, endosulfan has been shown to be a nonspecific inducer of drug metabolism (Agarwal et al. 1978).
The available evidence indicates that endosulfan can be metabolized in animals to other lipophilic compounds, which can rapidly enter tissues, and to more hydrophilic compounds that can be excreted.
3.4.4. Elimination and Excretion
Endosulfan and metabolites are eliminated mainly in the feces and urine in humans and animals. Biliary excretion has also been demonstrated to be important in animals. Estimated elimination half-lives ranged between approximately 1 and 7 days in adult humans and animals. Endosulfan can also be eliminated via the breast milk in lactating women and animals, although this is probably a relatively minor elimination route. No studies were located regarding known or suspected differences between children and adults with respect to endosulfan excretion.
3.4.4.1. Inhalation Exposure
No studies were located regarding excretion in animals after inhalation exposure to endosulfan.
The concentration of α- and β-endosulfan in the urine of a pest control worker who wore protective equipment peaked at 0.2 days (approximately 5 hours) after completing a 25-minute application of endosulfan in a greenhouse, declined to control levels by about 1.5 days postexposure, and remained at levels comparable to controls until the end of sampling at 3 days postexposure (Arrebola et al. 1999). Assuming first-order elimination, the urinary elimination half-life was estimated to be 0.94 days for α-endosulfan and 1.16 days for β-endosulfan; no endosulfan metabolite was detected in any urine sample.
3.4.4.2. Oral Exposure
α-Endosulfan and endosulfan sulfate, but not β-endosulfan, were observed in the urine at 0–3.5 hours after exposure, and endosulfan sulfate was also observed in the urine up to 91 hours after exposure in a man who died from ingesting endosulfan in a single oral dose of approximately 260 mg/kg (Boereboom et al. 1998); the terminal half-lives of α- and β-endosulfan in a two-compartment toxicokinetic model were 24.3 and 60.4 hours, respectively. In another case report, endosulfan was quantified in the urine of four patients who ingested endosulfan several hours earlier (Blanco-Coronado et al. 1992). Since the amount ingested was not known, the percentage of the ingested dose that was excreted could not be determined. It is also unknown whether the urine was the main route of excretion.
Endosulfan residues are rapidly eliminated from tissues as suggested by a half-life of approximately 7 days estimated in a 14-day oral study in female rats (Dorough et al. 1978). Rapid elimination was also observed in a 28-day study in goats in which half-lives between 1.1 and 3.1 days were estimated for endosulfan residues in various organs and tissues (Indraningsih et al. 1993).
Orally administered endosulfan is eliminated in both the feces and the urine of mice and rats, with the feces containing most of the pesticide eliminated (Chan et al. 2005; Deema et al. 1966; Dorough et al. 1978). In a study using rats, endosulfan was orally administered as a single gavage dose (2 mg/kg) or in the diet (0.25 or 1.25 mg/kg/day) for 2 weeks (Dorough et al. 1978). The animals given the single oral dose eliminated 19 and 25% of the α- and β-isomer dose, respectively, in the feces and urine in the form of the original compound and its metabolites 24 hours after exposure. After 120 hours, the percentages increased to 88 and 87% of the administered doses, respectively. The cumulative ratio of α-endosulfan eliminated in the feces compared with the urine after 120 hours was 5:1. The ratio for β-endosulfan was somewhat less than 7:2. These ratios are equivalent to excretion of 17% α-endosulfan and <22% β-endosulfan in the urine. The authors found that collection of bile from the rats caused a decrease in the elimination of endosulfan and its metabolites in the feces but had no effect on urinary excretion. Assuming little enterohepatic recirculation, data from bile duct cannulation indicate that about 65–70% of the amount in the feces was due to biliary excretion. Animals administered endosulfan in the diet for 2 weeks showed a similar elimination pattern. The total cumulative percentage of the radiocarbon eliminated from rats given the α- or β-isomers in the diet (5 ppm) was 64 and 65%, respectively. Ratios of the cumulative dose eliminated via the feces compared with the urine for both isomers were approximately 7:1. No major differences were noted in animals given 25 ppm endosulfan in the diet. Extraction and analysis of the feces showed that the residues consisted of the parent compound, five polar metabolites, and unidentified polar material. The urine and feces contained the diol, α-hydroxyether, and lactone of endosulfan. Furthermore, these authors indicated that 47% of the dose was eliminated from the liver via biliary secretion 48 hours following treatment. In mice, endosulfan sulfate and alcohol (diol) were the main metabolites detected, primarily in the feces (Deema et al. 1966). The ratio of radioactivity recovered per gram of excreta for feces and urine was 26:1; however, no corrections were made for quenching or self-absorption. Administration of a single oral dose of 14C-endosulfan (65% α-isomer, 35% β-isomer) to milk sheep resulted in recovery of approximately 50% of the radiolabel in the feces, 4% in the urine, and 1% in the milk (Gorbach et al. 1968). Unmetabolized endosulfan was found in the feces but not in the urine. The main metabolites found in the urine were endosulfan diol and α-hydroxy-endosulfan ether. Most of the 14C activity in the milk of sheep was due to endosulfan sulfate. In a more recent study, male rats were administered a single gavage dose of 5 mg/kg 14C-endosulfan and urine and feces were collected for 96 hours (Chan et al. 2005). Over the 4-day period, 108.6% of the administered radioactivity was recovered in the excreta, fecal elimination being the major route of excretion with 94.4% of the radioactivity. The cumulative urinary excretion was 12.4%. Although these studies suggest variations in the excretion patterns with different species, they do provide evidence that the excretion of endosulfan and its metabolites after oral exposure is rapid and occurs mainly through the fecal route.
Gavage dosing of male and female rats with endosulfan (65.3% α-endosulfan, 33.7% β-endosulfan) for 30 days resulted in a greater accumulation of endosulfan in fatty tissue from females than males (Dikshith et al. 1984). The authors speculated that the difference between males and females was a function of more rapid excretion of endosulfan by males than females, and that this could account for the higher sensitivity of female rats to endosulfan toxicity. However, excretion of endosulfan and its metabolites was not directly measured in this study; therefore, alternative explanations for the differences in residue content and toxicity cannot be discounted.
3.4.4.3. Dermal Exposure
Endosulfan and metabolites were observed in the urine of workers who had prepared and applied endosulfan for 2–5 hours either 1 day or 1 week prior to sampling, without using protective clothing or face mask (thus, exposure was probably both dermal and inhalation) (Vidal et al. 1998). Unchanged α- and β-endosulfan and endosulfan ether were the predominant chemicals excreted 1 day following exposure. One week after exposure, α-endosulfan was detected in urine of four of five workers, but β-endosulfan was detected in only one of five samples and endosulfan ether was not detected at all. Endosulfan sulfate was detected in only one of five samples at 1 week after exposure and in none of the four samples at 1 day postexposure. Endosulfan lactone was detected in one of four and one of five samples at 1 day and 1 week after exposure, respectively.
One study was located regarding excretion in animals after dermal exposure to endosulfan (Hoechst 1986). Following a single dermal application of an aqueous suspension of 14C-endosulfan (at 0.1, 0.83, and 10.13 mg/kg) to male Sprague-Dawley rats, limited excretion of radiolabel (0.5–1.0% of the applied dose) occurred during the first 10 hours of exposure and occurred primarily in the urine. However, between 10 and 24 hours, excretion increased to an average of 10% of the absorbed dose. Elimination was rapid once the endosulfan passed through the skin. Excretion was dose related (13.5% of the absorbed dose at the low dose, 12.4% at the middle dose, and 4.9% at the high dose), with the percentage excreted decreasing with increasing dose. Although excretion was greater in the urine than feces during the first 10 hours of exposure, by 24 hours, excretion in the feces was approximately 2 times greater than in the urine (Hoechst 1986).
3.4.4.4. Other Routes of Exposure
Intravenous administration of endosulfan (7:3 ratio of α- and β-isomers) in rabbits produced slower elimination of the α-isomer (Gupta and Ehrnebo 1979). Excretion of the two isomers occurred primarily via the urine (29%) with much less excreted via the feces (2%). Given the earlier evidence in rats and mice describing the principal route of elimination of endosulfan and its metabolite to be via the feces, the differences in the excretion pattern in this study may be attributable to differences in exposure routes, species differences, or both. Nevertheless, studies in laboratory animals suggest that both renal and hepatic excretory routes are important in eliminating endosulfan from the body. Elimination of small doses is essentially complete within a few days.
3.4.5. Physiologically Based Pharmacokinetic (PBPK)/Pharmacodynamic (PD) Models
Physiologically based pharmacokinetic (PBPK) models use mathematical descriptions of the uptake and disposition of chemical substances to quantitatively describe the relationships among critical biological processes (Krishnan et al. 1994). PBPK models are also called biologically based tissue dosimetry models. PBPK models are increasingly used in risk assessments, primarily to predict the concentration of potentially toxic moieties of a chemical that will be delivered to any given target tissue following various combinations of route, dose level, and test species (Clewell and Andersen 1985). Physiologically based pharmacodynamic (PBPD) models use mathematical descriptions of the dose-response function to quantitatively describe the relationship between target tissue dose and toxic end points.
PBPK/PD models refine our understanding of complex quantitative dose behaviors by helping to delineate and characterize the relationships between: (1) the external/exposure concentration and target tissue dose of the toxic moiety, and (2) the target tissue dose and observed responses (Andersen and Krishnan 1994; Andersen et al. 1987). These models are biologically and mechanistically based and can be used to extrapolate the pharmacokinetic behavior of chemical substances from high to low dose, from route to route, between species, and between subpopulations within a species. The biological basis of PBPK models results in more meaningful extrapolations than those generated with the more conventional use of uncertainty factors.
The PBPK model for a chemical substance is developed in four interconnected steps: (1) model representation, (2) model parameterization, (3) model simulation, and (4) model validation (Krishnan and Andersen 1994). In the early 1990s, validated PBPK models were developed for a number of toxicologically important chemical substances, both volatile and nonvolatile (Krishnan and Andersen 1994; Leung 1993). PBPK models for a particular substance require estimates of the chemical substance-specific physicochemical parameters, and species-specific physiological and biological parameters. The numerical estimates of these model parameters are incorporated within a set of differential and algebraic equations that describe the pharmacokinetic processes. Solving these differential and algebraic equations provides the predictions of tissue dose. Computers then provide process simulations based on these solutions.
The structure and mathematical expressions used in PBPK models significantly simplify the true complexities of biological systems. If the uptake and disposition of the chemical substance(s) are adequately described, however, this simplification is desirable because data are often unavailable for many biological processes. A simplified scheme reduces the magnitude of cumulative uncertainty. The adequacy of the model is, therefore, of great importance, and model validation is essential to the use of PBPK models in risk assessment.
PBPK models improve the pharmacokinetic extrapolations used in risk assessments that identify the maximal (i.e., the safe) levels for human exposure to chemical substances (Andersen and Krishnan 1994). PBPK models provide a scientifically sound means to predict the target tissue dose of chemicals in humans who are exposed to environmental levels (for example, levels that might occur at hazardous waste sites) based on the results of studies where doses were higher or were administered in different species. Figure 3-4 shows a conceptualized representation of a PBPK model.

Figure 3-4
Conceptual Representation of a Physiologically Based Pharmacokinetic (PBPK) Model for a Hypothetical Chemical Substance. Note: This is a conceptual representation of a physiologically based pharmacokinetic (PBPK) model for a hypothetical chemical substance. (more...)
If PBPK models for endosulfan exist, the overall results and individual models are discussed in this section in terms of their use in risk assessment, tissue dosimetry, and dose, route, and species extrapolations.
The Chan et al. (2006) Model
Description of the model. Chan et al. (2006) developed a PBPK model to predict tissue doses of endosulfan in male Sprague-Dawley rats following acute (single or three doses) exposure of the pesticide by gavage. The model comprised nine compartments: gastrointestinal tract, liver, brain, kidneys, fat, testes, well-perfused tissues, poorly-perfused tissues, and the lungs. In the model, the rate of transfer of endosulfan to the tissues was limited by blood flow to the specific tissue, while diffusion was assumed to occur instantaneously in each organ. The model assumed that metabolism occurs only in the liver where the rate of metabolism was described by the Michaelis-Menten equation. Physiological parameters were taken from the literature, whereas partition coefficients and biochemical parameters were determined experimentally and optimized by manual adjustment until the best visual fit of the simulations with experimental data were observed.
Validation of the model. The model was validated by simulating the disposition of 14C-endosulfan in rats following single and repeated gavage doses and comparing the simulated results with the empirical data. Further validation was performed by using experimental data from the literature.
Risk assessment. The rat model developed by Chan et al. (2006) has not been applied to risk assessments.
Target tissues. In general, model simulations for endosulfan concentrations in blood and target tissues (liver, kidneys, brain, and testes) fit reasonably well the shape of the experimental data. However, simulation of total endosulfan disposition from kidneys and liver after repeated oral administration of 5 mg/kg endosulfan resulted in slight overestimation of concentrations at all time points, whereas simulation of total endosulfan disposition from testes resulted in slight underestimation at the last time point.
Sensitivity analysis. The impacts of the physicochemical and biochemical parameters were examined when each parameter was changed from its original value by 1% at the various time points examined. There was no impact of the partition coefficients for all tissues on the concentrations of endosulfan across all time points examined (1, 2, 4, 8, and 24 hours). Parameters such as absorption rate, and biliary and fecal excretion rates for endosulfan had no impact on the blood and tissue concentrations of endosulfan. However, the sensitivity for maximum metabolic rate and urinary excretion rate were consistently negative across tissue time and external exposure concentration, whereas the sensitivity for Michaelis-Menten constant was consistently positive across all time points and external exposure concentrations.
Species extrapolation. Species extrapolation was not attempted in this model. A human model would be needed for dosimetry extrapolation to humans for MRL derivation.
Interroute extrapolation. Interroute extrapolation was not attempted in this model.
3.5. MECHANISMS OF ACTION
3.5.1. Pharmacokinetic Mechanisms
No information was located regarding the mechanism of inhalation, oral, or dermal absorption of endosulfan in humans or animals; however, the lipophilic nature of endosulfan suggests that it is probably absorbed by passive diffusion. Based on results from toxicity studies, the mode of administration of endosulfan (gavage or diet) seems to play a role in the manifestation of the toxicity of endosulfan; effects are manifested at higher dietary dose than gavage doses. No information was located regarding the mechanism by which endosulfan is transported in the blood. However, due to endosulfan’s high solubility in lipids, it is reasonable to assume that it might be associated with a lipid fraction in the blood. Studies in animals suggest that endosulfan initially accumulates in fatty tissues and that relatively high amounts can be found in the liver and kidneys after exposure (Chan and Mohd 2005; Dorough et al. 1978; Gupta 1978; Hoechst 1987; Nath and Dikshith 1979). Rapid accumulation of endosulfan metabolites in the liver (Khanna et al. 1979) and increased lysozymal activity in the kidney (Hoechst 1987) suggest that these may be sites of endosulfan metabolism; however, the role of metabolism in the toxicity of endosulfan has not been well characterized. Although endosulfan induces microsomal cytochrome P450 in the liver (Casabar et al. 2006; Lee et al. 2006; Siddiqui et al. 1987a; Tyagi et al. 1984), it is not clear whether endosulfan thereby induces its own metabolism. Results from a dermal study in rats suggested that the metabolism of endosulfan may be a saturable process (Hoechst 1986). In animals, biliary excretion of endosulfan and metabolites is a main route of elimination of this chemical and may contribute about two-thirds of the endosulfan found in the feces (Dorough et al. 1978). A minor proportion of endosulfan and metabolites is excreted in the urine.
3.5.2. Mechanisms of Toxicity
The neurotoxic effects of endosulfan are well documented in both humans and animals, and extensive research has been conducted in recent years aimed at elucidating its mechanism of neurotoxicity. Although serious neurotoxic effects, including death, generally occur after acute exposure to concentrations much higher than those commonly found in the environment, there is concern about the possibility of accidental exposure of those occupationally exposed such as agricultural workers who apply the pesticide in the fields. In addition to neurotoxicity, exposure to endosulfan in animals has induced a wide array of effects including liver and kidney toxicity, hematological and metabolic effects, alterations in the immune system and reproductive parameters. The possible mechanisms of the effects on organ or systems other than the nervous system have not been as well studied as the mechanism of neurotoxicity. However, there is evidence that endosulfan-induced oxidative stress due to decreased activity of antioxidant enzymes and increased lipid peroxidation are involved in the manifestation of alterations seen in organs and systems following exposure to endosulfan (Alva et al. 2012; Aly and Khafagy 2014; Mahran 2013; Omurtag et al. 2008; Ozdem et al. 2011; Pal et al. 2009; Wang et al. 2014). In addition, it is likely that some of these effects are secondary to the adverse neurological effects. Speculation in this section on the mechanism(s) of action involved in effects that have not been well characterized and/or have been seen inconsistently in animal studies seems inappropriate at this time. Therefore, this section will focus mainly on the mechanism of neurotoxic effects of endosulfan.
Acute exposure to large amounts of endosulfan results in frank effects manifested as hyperactivity, muscle tremors, ataxia, and convulsions. Possible mechanisms of toxicity include interference with the binding of those neurotransmitters to their receptors, alteration of neurotransmitter levels in brain areas by affecting synthesis, degradation, and/or rates of release and re-uptake.
The results from several studies suggest the involvement of GABA receptors in endosulfan-induced neurotoxicity. In a series of in vitro experiments using 3H-dihydropicrotoxinin, Abalis et al. (1986), Cole and Casida (1986), Gant et al. (1987), and Ozoe and Matsumura (1986) showed that endosulfan acts as a noncompetitive GABA antagonist at the chloride channel within the GABA receptor in brain synaptosomes. Antagonism of GABAergic neurons within the central nervous system leads to generalized central nervous system stimulation. Binding of GABA to its receptor opens chloride-selective ion channels leading to influx of chloride into neurons through electrochemical gradient, resulting in hyperpolarization of the membrane and inhibition of cell firing. A reduced inhibitory drive translates into increased activity of the effector neurons. The studies mentioned above found that the ability of endosulfan to induce convulsions correlated with the potency to bind to this site and to inhibit GABA-induced chloride flux, thus providing good evidence for this mechanism of action. An in vitro study that compared sensitivity of GABA receptors from several vertebrate brain preparations (human, dog, mouse, chicken, quail, and salmon) and two insects to α-endosulfan reported little selectivity for the pesticide (Hainzl et al. 1998). IC50 values for the vertebrate preparations ranged between 11 and 33 nM; the IC50 values for the two insect sources were 7 and 3 nM. These values also showed that α-endosulfan is considerably more potent with the two insects than with the vertebrate GABA receptors. Further studies from the same group of investigators with human receptor subtypes expressed individually and in combination in insect Sf9 cells showed that the β3 subunit contains the target for α-endosulfan and other subunits differentially modulate the binding to confer compound-dependent specificity and selective toxicity (Ratra et al. 2001).
A study showed that α-endosulfan blocked the chloride uptake induced by GABA in primary cultures of cortical neurons from 15-day-old mice fetuses by interacting with the t-butylbicyclophosphorothionate (a GABA antagonist) binding site (Pomes et al. 1994). In a subsequent study, the same group of investigators found that α-endosulfan had relatively low cytotoxicity (assessed by disruption of cell membrane integrity) in primary neuronal cultures of cerebellar granule cells, and that it did not increase the formation of intracellular oxidative radicals (Rosa et al. 1996). It did, however, increase mitochondrial transmembrane potential which, according to Rosa et al. (1996), could be linked to a detoxification process of the cell. The authors further stated that their findings were consistent with the view that in vivo neurotoxicity is mediated mainly by inhibition of GABAergic function and that other effects detected in vitro are less important. α-Endosulfan was also reported to inhibit the glycine receptor in cultured cerebellar granule cell from neonatal mice, but less potently than the GABA receptor (Vale et al. 2003). The strychnine-sensitive glycine receptor also regulates chloride flux inhibitory responses. Currently, the GABA-antagonism mechanism of toxicity is the most widely accepted hypothesis.
Several studies reported changes in neurotransmitter levels following exposure to endosulfan. For example, Gupta (1976) found that brain acetylcholinesterase activity was decreased following a single intraperitoneal injection of endosulfan in rats and postulated that the decreased activity of this enzyme resulted in an increase in brain levels of acetylcholine, which could, in turn, be responsible for the central nervous system stimulation observed. However, brain cholinesterase was increased in female rats that consumed ≥4.59 mg/kg/day for 13 weeks (Hoechst 1985a). Thus, it is unclear whether the decrease in brain acetylcholinesterase observed by Gupta (1976) was a representative finding. Neither Paul et al. (1994) nor Lakshmana and Raju (1994) found changes in the activity of acetylcholinesterase in the brain of rats treated with 2 mg endosulfan/kg/day for 90 days or with 6 mg/kg/day for 23 days, respectively. Ansari et al. (1987) also suggested that changes in neurotransmitter levels (specifically serotonin, GABA, and dopamine) in the brain may be partly responsible for the neurotoxicity of endosulfan in rats after observing hyperactivity, tremors, and convulsions following a single intraperitoneal injection of 40 mg/kg of endosulfan. Paul et al. (1994) found significant increases in serotonin concentration in the cerebrum and midbrain of rats after 90 days of treatment with 2 mg/kg/day endosulfan, and in this study, spontaneous motor activity was significantly increased in the treated animals. Furthermore, Paul et al. (1994) also found a correlation between the increase in serotonin and inhibition of a learning paradigm. Lakshmana and Raju (1994) also reported changes in the concentrations of dopamine, noradrenaline, and serotonin in various brain areas of endosulfan-treated rats. In this case, treated rats took 29% more time to learn a behavioral task; however, it was not determined which neurotransmitter(s) change may have been responsible for the behavioral change.
Studies have also examined the role of neurotransmitter receptors in endosulfan-induced neurotoxic effects. For instance, a single intraperitoneal dose of 3 mg/kg of endosulfan or administration of 1 mg/kg/day for 30 days had no effect on frontal cortical 3H-serotonin binding or aggressive behavior in adult rats, but 30 daily injections of 3 mg/kg/day caused a significant increase in 3H-serotonin binding affinity and foot-shock-induced fighting (Agrawal et al. 1983). Serotonin may also play a role in the increase in aggressive behavior (foot-shock-induced fighting) observed in rats following multiple exposures to endosulfan (Agrawal et al. 1983; Zaidi et al. 1985). Rat pups injected with 1 mg/kg/day for 25 days showed a significant increase in frontal cortical 3H-serotonin binding and exhibited a significant increase in foot-shock-induced fighting behavior (Zaidi et al. 1985). These effects were still observed 8 days after cessation of treatment. The authors concluded that endosulfan affects serotonergic function, which in turn induces neurotoxicity in both neonates and adults, as demonstrated by increased 3H-serotonin binding to the frontal cortex and aggressive behavior. A correlation between 3H-serotonin binding and aggressive behavior was also observed. These data also suggest that neonates show a greater sensitivity to endosulfan than adults.
Studies have also shown that perinatal exposure to endosulfan can produce neurochemical alterations in brain of the offspring. In mice, maternal exposure to 1 mg/kg/day endosulfan in the diet during gestation and lactation resulted in alterations in the expression of proteins in the frontal cortex from 12-week-old male offspring (females were not tested) that are critical for the normal functioning of gabaergic, glutamatergic, and dopaminergic pathways (Wilson et al. 2014a). The same exposure protocol also resulted in alteration in dopaminergic pathways in the striatum of 12-week-old offspring. Furthermore, transplacental and/or lactation exposure to endosulfan rendered the offspring more susceptible to the dopaminergic neurotoxin, MPTP, than mice that were exposed to MPTP as adults (Wilson et al. 2014b). Similar exposure of rats to endosulfan also resulted in alterations in the concentration and/or metabolism of dopamine, norepinephrine, and serotonin in the striatum of male and female 60-day-old offspring (Lafuente and Pereiro 2013). Gender differences in response were evident in this study, suggesting that male offspring were more susceptible to early life exposure than females.
As summarized in Section 3.4.3 (Metabolism), the biotransformation of endosulfan can give rise to a number of both polar and nonpolar metabolites. There is little and inconclusive information on whether the toxicological properties of endosulfan are due to the parent compound or to any of its metabolites. One could assume that the more lipophilic substances will cross cell membranes more easily than polar metabolites, accumulate to a greater extent, and perhaps be the most neurotoxic. Differential toxicity could also be related to differential affinity for the GABA receptor. What is known from oral acute-lethality studies in rats and mice is that α-endosulfan is approximately 3 times more toxic than β-endosulfan (Dorough et al. 1978; Hoechst 1975, 1990; Maier-Bode 1968). In addition, in mice, the acute toxicity of endosulfan sulfate was comparable to that of α-endosulfan (Dorough et al. 1978). Also in mice, the metabolites endosulfan α-hydroxy ether, endosulfan lactone, and endosulfan ether had lethal doses 10–20 times higher than the α-or β-isomers; the lethal dose for endosulfandiol was 2 orders of magnitude higher than that of the α- or β-isomer (Dorough et al. 1978). Extrapolation of this information to possible potency differences in longer-term studies is clearly inappropriate since other factors, such as pharmacokinetics and possibly induction of biotransformation enzymes, play a role in longer-term studies.
Evidence from some oral studies in rats suggests that there is a difference in susceptibility to some effects of endosulfan between males and females. For example, the LD50 values in females were up to 3–4 times lower than in males (Hoechst 1990), and similar observations had been made by others (Gupta 1976; Gupta and Chandra 1977). Also, in a 30-day feeding study, 3 out 10 females, but no males, died during the study and the female survivors experienced more pronounced liver toxicity than the males (Paul et al. 1995). The higher sensitivity of females is thought to be due to a greater accumulation and slower elimination of endosulfan residues than the males (Dikshith et al. 1984, 1988). Paul et al. (1995) also conducted a series of motor and neurobehavioral tests in both sexes and found that although endosulfan increased spontaneous motor activity in both sexes, the increase was significantly greater in males. They speculated that males may produce more lipophilic metabolites, such as endosulfan sulfate, than females, which could be responsible for the more marked stimulation of spontaneous activity in males. If this were the case, then endosulfan residues other than the sulfate would be responsible for the adverse liver effects. No consistent differential sensitivity has been observed in species other than the rat.
3.5.3. Animal-to-Human Extrapolations
Almost all of the information regarding the effects of endosulfan in humans is derived from cases of acute exposure to high amounts of the chemical. Some of these cases resulted in death (Bernardelli and Gennari 1987; Blanco-Coronado et al. 1992; Boereboom et al. 1998; Demeter and Heyndrickx 1978; Eyer et al. 2004; Lo et al. 1995; Moon and Chun 2009; Moses and Peter 2010; Parbhu et al. 2009; Terziev et al. 1974). Postmortem examination revealed lesions to a variety of organs and tissues, and this is consistent with findings in animals exposed to lethal doses of endosulfan. In both humans and animals, high doses of endosulfan affect primarily the nervous system and many of the systemic effects observed are secondary to the neurological effects or to aspiration. It is yet to be determined whether effects seen in animals exposed to lower doses of endosulfan for prolonged periods of time would also manifest in humans under similar exposure conditions. Also, there is not enough information to predict whether the metabolism and disposition of endosulfan by humans is similar to those in experimental animals.
3.6. TOXICITIES MEDIATED THROUGH THE NEUROENDOCRINE AXIS
Recently, attention has focused on the potential hazardous effects of certain chemicals on the endocrine system because of the ability of these chemicals to mimic or block endogenous hormones. Chemicals with this type of activity are most commonly referred to as endocrine disruptors. However, appropriate terminology to describe such effects remains controversial. The terminology endocrine disruptors, initially used by Thomas and Colborn (1992), was also used in 1996 when Congress mandated the EPA to develop a screening program for “…certain substances [which] may have an effect produced by a naturally occurring estrogen, or other such endocrine effect[s]…”. To meet this mandate, EPA convened a panel called the Endocrine Disruptors Screening and Testing Advisory Committee (EDSTAC), and in 1998, the EDSTAC completed its deliberations and made recommendations to EPA concerning endocrine disruptors. In 1999, the National Academy of Sciences released a report that referred to these same types of chemicals as hormonally active agents. The terminology endocrine modulators has also been used to convey the fact that effects caused by such chemicals may not necessarily be adverse. Many scientists agree that chemicals with the ability to disrupt or modulate the endocrine system are a potential threat to the health of humans, aquatic animals, and wildlife. However, others think that endocrine-active chemicals do not pose a significant health risk, particularly in view of the fact that hormone mimics exist in the natural environment. Examples of natural hormone mimics are the isoflavinoid phytoestrogens (Adlercreutz 1995; Livingston 1978; Mayr et al. 1992). These chemicals are derived from plants and are similar in structure and action to endogenous estrogen. Although the public health significance and descriptive terminology of substances capable of affecting the endocrine system remains controversial, scientists agree that these chemicals may affect the synthesis, secretion, transport, binding, action, or elimination of natural hormones in the body responsible for maintaining homeostasis, reproduction, development, and/or behavior (EPA 1997h). Stated differently, such compounds may cause toxicities that are mediated through the neuroendocrine axis. As a result, these chemicals may play a role in altering, for example, metabolic, sexual, immune, and neurobehavioral function. Such chemicals are also thought to be involved in inducing breast, testicular, and prostate cancers, as well as endometriosis (Berger 1994; Giwercman et al. 1993; Hoel et al. 1992).
In vivo studies in animals suggest that endosulfan may disrupt normal reproductive hormone levels in male animals, but that it is not an endocrine disrupter in females. Persistent depressed testicular testosterone was seen in male rats after intermediate-duration oral exposures to endosulfan. In ovariectomized female rats, orally administered endosulfan did not induce normal development of female reproductive tissues, and in female mice and immature female rats, acute parenteral exposure to endosulfan did not affect several endocrine-related end points. In vitro studies have evaluated endosulfan for estrogen receptor (ER) and cytosolic protein binding affinity, ER-mediated reporter gene expression, estrogenic induction of cell proliferation, and alteration of relative abundance of active estradiol metabolites. Overall, in vitro evidence in favor of endosulfan estrogenicity indicates relatively weak potency compared to 17β-estradiol . Apparently contradictory results were reported in different studies for several of the assays, indicating that caution should be used in interpreting the collective in vitro results.
Significantly increased serum testosterone and decreased testicular testosterone were reported in male rats after a 7-day exposure to endosulfan using oral doses in the range of 7.5–10 mg/kg/day, but not at ≤5 mg/kg/day (Singh and Pandey 1989). However, results after a 15-day exposure were highly variable and frequently not dose-related, making interpretation of the significance of the study results difficult. A subsequent study (Singh and Pandey 1990) indicated a dose-related decrease in testicular testosterone, and plasma testosterone, LH, and FSH in groups of male Wistar rats orally administered endosulfan at 0, 7.5, or 10 mg/kg/day for 15 or 30 days. In addition, activities of steroidogenic enzymes and testicular cytochrome P450-dependent monooxygenases were depressed after the 30-day exposure at ≥7.5 mg/kg/day. All of the effects from 30 days of exposure were reversible during a 7-day recovery period, except for decreased testicular testosterone, which remained depressed; no recovery period was utilized for the 15-day exposures. Decreased serum testosterone was also reported in male rats exposed to 15 mg/kg/day technical endosulfan by gavage for 15–30 days; no significant effects were reported at 10 mg/kg/day (Choudhary and Joshi 2003). Serum testosterone was reduced in male rats administered 5 mg/kg/day technical endosulfan for 10 days (Aly and Khafagy 2014) or 30 days (Kumar et al. 2011), in male mice administered 0.8 mg/kg/day technical endosulfan for 3 weeks (Wang et al. 2014), and in male rabbits dosed with 1 mg/kg/day technical endosulfan for 60 days (Ozmen and Mor 2012). In contrast, doses of 2.9 mg/kg/day technical endosulfan provided to male rats via the diet for 8 weeks had no significant effect on serum levels of testosterone, LH, and FSH (Perobelli et al. 2010).
Raizada et al. (1991) examined the potential estrogenic properties of an unspecified endosulfan formulation in ovariectomized rats. Endosulfan administered by gavage at 1.5 mg/kg/day for 30 days to ovariectomized rats did not influence the relative weights or histology of the uterus, cervix, or vagina compared to ovariectomized control rats that did not receive endosulfan. Rats in a positive control group received intraperitoneal injections of estradiol and showed increased relative organ weights and normal development of female reproductive tissues compared to the untreated ovariectomized control rats. Organ weights and tissue development in rats administered simultaneously endosulfan and estradiol were not significantly different from those seen in rats that received estradiol alone. The study results indicated that endosulfan was neither estrogenic nor anti-estrogenic under the conditions of this assay. Hiremath and Kaliwal (2003) examined the potential estrogenic properties of technical endosulfan in ovariectomized mice. Estrogenic activity was assessed using uterine weight and vaginal cornification as end points. Antiestrogenic activity was assessed by administering technical endosulfan along with 17β-estradiol to a group of mice. Ovariectomized control mice showed a prolonged diestrus. Mice treated with 4 mg/kg endosulfan (only level tested) for 30 days did not show vaginal cornification and showed continuous diestrus without any appearance of estrus, indicating its nonestrogenic activity. In addition, there was no significant change in uterine weight. Mice dosed with endosulfan plus 17β-estradiol showed a significant increase in the duration of estrus and increased uterine weight, indicating that endosulfan did not have antiestrogenic activity under the conditions of the study. Immature female rats intraperitoneally administered technical grade endosulfan at 3 mg/kg/day for 3 days showed no changes with respect to relative uterine and pituitary weights, uterine peroxidase activity, circulating thyroxine levels, or to levels of FSH, LH, TSH, prolactin, and growth hormone in the pituitary gland (Wade et al. 1997). As an extension of the same assay, endosulfan did not alter relative levels of ER or progesterone receptors compared to controls in crude uterine cytosol prepared from uterine tissue of the rats dosed intraperitoneally. Uterine weight in female mice was not affected by acute subcutaneous administration of technical-grade endosulfan at up to 10 mg/kg/day for 3 days, whereas 17β-estradiol at up to 4 mg/kg/day gave a strong positive response (Shelby et al. 1996). More recently, subcutaneous doses of up to 6 mg/kg/day administered to ovariectomized adult rats did not significantly alter uterine weight or the luminal epithelial cell height (Varayoud et al. 2008). However, doses as low as 6 μg/kg/day of endosulfan mimicked the effect of a non-uterotrophic dose of 17β-estradiol in modifying the expression of estrogen-responsive genes such as ERα, progesterone receptor, and complement factor-3, which according to the investigators, could contribute to an increase in implantation failures. In a later publication, the same group of investigators reported that a subcutaneous injection of 0.6 mg/kg/day of endosulfan given to female rat pups from PND 1 to 7 altered the expression of estrogen-dependent genes that regulate uterine development and differentiation (Milesi et al. 2012).
Using a pituitary cell line known to respond to estrogens by increasing its secretion of prolactin, Rousseau et al. (2002) reported that endosulfan was able to induce a substantial increase of prolacting expression. The investigators suggested that endosulfan could modulate an estrogen-inducible gene such as prolactin by acting via second messenger-mediated cellular mechanisms instead of solely competing with estrogens for the nuclear estrogen receptor sites. In HeLa cells, endosulfan was shown to weakly activate ERα with the 7xAP-1 reporter, but not other reporters, in a nonclassical “tethered” mechanism (Li et al. 2013).
α-Endosulfan had 400 times lower in vitro binding affinity for a recombinant human progesterone receptor than the natural ligand and 85,000 times lower binding affinity for a recombinant human estrogen receptor (Scippo et al. 2004). The corresponding binding affinities for β-endosulfan for the same human recombinant receptors were 1,760 and 78,000 times lower than the natural ligands. Endosulfan (60% α-isomer and 38% β-isomer) at 300 μM had approximately 20 times lower in vitro binding affinity for oviductal cytosolic binding proteins of yellow-bellied turtle (Trachemys scripta) and American alligator (Alligator mississippiensis) compared to 17β-estradiol at 1 μM (Crain et al. 1998). The results of these studies suggest that the relatively low binding affinity of endosulfan for ER may be somewhat offset by a relatively lower binding affinity for cytosolic proteins, producing a relatively greater bioavailability for interacting with intracellular steroid receptors than estradiol. Indeed, in a competitive ER-binding assay, endosulfan significantly inhibited both [3H]17β-estradiol binding to the estrogen receptor and progestin [3H]R5020 binding to the progesterone receptor using receptors prepared from alligator oviduct tissue (Vonier et al. 1996). However, in another competitive binding assay, neither of the endosulfan isomers either singly or in combination with dieldrin inhibited 17β-estradiol binding either to recombinant human ER at concentrations up to 10 μM (Arcaro et al. 1998) or to mouse uterine receptor (Shelby et al. 1996). Similarly, 17β-estradiol-induced foci formation in MCF-7 human breast cancer cells was neither inhibited nor stimulated by cotreatment with endosulfan (Arcaro et al. 1998). In a more recent study, α-endosulfan inhibited the binding of 3H-estradiol to the estrogen receptor in cultured cortical neurons and cerebellar granule cells from mice fetuses and pups; the IC50 was 21.7 μM (Briz et al. 2011).
ER-mediated reporter gene expression was related to endosulfan incubation concentration; in general, 100 μM induced gene expression, while mixed results were obtained at lower concentrations. Endosulfan induced human ER-mediated β-galactosidase (β-gal) activity at 100 μM in an estrogen-responsive reporter system in yeast, but not at ≤10 μM (Ramamoorthy et al. 1997). The endosulfan-induced yeast β-gal activity was about 32% of that induced by estradiol at 0.01 μM. Endosulfan was the only pesticide (among endosulfan, chlordane, toxaphene, and dieldrin) to induce β-gal activity above background; binary mixtures of endosulfan with the other pesticides induced significantly less activity than endosulfan alone.
The test system was considerably less sensitive to endosulfan when mouse ER, rather than human ER, was used to mediate β-gal activity (Ramamoorthy et al. 1997). In similar assays, endosulfan at 10 μM had no effect on β-gal activity in yeast (Saccharomyces) transfected with either the human or rainbow trout ER (Andersen et al. 1999). In addition, no effect was observed on transcriptional activation of HeLa cells transfected with plasmids containing an estrogen receptor as a responsive element (Shelby et al. 1996). Endosulfan also did not induce transient reporter gene expression in MCF-7 human breast cancer cells at an incubation concentration of 2.5 μM (Andersen et al. 1999). Maximum endosulfan-induced ER-mediated luciferase reporter gene expression occurred in vitro in a T47D human breast adenocarcinoma cell line at approximately 10 μM, while 50% expression of luciferase occurred at about 5.9 μM; the maximum expression was approximately 59% of the effect from exposure to 0.03 nM estradiol (0.00003 μM) (Legler et al. 1999). Luciferase expression from combined treatment with endosulfan and dieldrin was additive over concentrations ranging from 3 to 8 μM.
Endosulfan at 10 μM induced in vitro proliferation of MCF-7 human breast cancer cells to between 2- and 5-fold higher than that seen in hormone-free cells, but appeared to be cytotoxic at approximately 100 μM (Andersen et al. 1999). A similar study showed that endosulfan (technical grade) induced cell proliferation in the MCF-7 human breast cancer cell line at exposure levels of 10 and 50 μM between 2 and 4 times control levels, but not at ≤2 μM (Wade et al. 1997). Soto et al. (1994, 1995) also demonstrated MCF-7 proliferation at a dose level of 10–25 μM endosulfan, with the maximum cell growth induced by endosulfan achieving 86% of that induced by estradiol, and cytotoxicity occurring at higher exposure levels. In apparent contradiction of these positive findings, endosulfan (isomeric composition not reported) did not substantially affect the growth of either ER-positive (MCF-7) or ER-negative (SK-BR-3) cultured human breast cancer cell lines at concentrations of ≤35 μM. Endosulfan did severely inhibit cell growth at higher concentrations, and this growth inhibition was synergistic when cultures were incubated with either dieldrin or chlordane (Hsu et al. 1998). In another in vitro assay, both α- and β-endosulfan were weakly estrogenic in inducing foci in MCF-7 cultures at 10 μM (but not at lower concentrations), and showed no estrogenic synergism when incubated in combination with dieldrin (Arcaro et al. 1998). In addition to inducing cell proliferation, endosulfan induced proliferation of the progesterone receptor, which is another estrogen-mimicking effect (Soto et al. 1995). Recently Briz et al. (2011) reported that 5 μM α-endosulfan significantly induced MCF-7 cell proliferation, thus defining a lowest-observed-effect concentration (LOEC) half that defined by Soto et al. (1994). Endosulfan significantly increased cell proliferation and ER transactivation gene response in MCF-7 human breast cancer cells at 1 μM; maximum response was obtained at 25 μM (Andersen et al. 2002). In addition, endosulfan potentiated the 17β-estradiol-induced proliferation when it was tested together with a concentration of 17β-estradiol causing a submaximal response. In the same study, endosulfan exhibited very weak antiandrogenic activity in CHO cells, reducing the response of the synthetic androgen R1881 by 28% (Andersen et al. 2002).
α-Endosulfan and β-endosulfan each altered the relative quantities of estradiol metabolites in vitro in ER-positive MCF-7 human breast cancer cells. The amount of a genotoxic estradiol metabolite, 16α-hydroxyesterone (16α-OHE1), was increased relative to controls and the metabolite, 2-hydroxy-estrone (2-OHE1), which inhibits breast cell proliferation, was decreased relative to controls (Bradlow et al. 1995), resulting in a slight increase in the 16α-OHE1/2-OHE1 ratio. The authors hypothesized that by producing an increase in the 16α-OHE1/2-OHE1 ratio, endosulfan may increase the risk of estradiol-induced abnormal cell growth in ER-positive tissues such as breast tissue.
The overall evidence indicates that endosulfan administered in vivo may be disruptive of reproductive hormone levels in male animals. On the other hand, endosulfan is neither estrogenic nor disruptive of thyroid or pituitary hormone levels in females in vivo, despite its weak estrogenicity in several in vitro test systems.
3.7. CHILDREN’S SUSCEPTIBILITY
This section discusses potential health effects from exposures during the period from conception to maturity at 18 years of age in humans, when all biological systems will have fully developed. Potential effects on offspring resulting from exposures of parental germ cells are considered, as well as any indirect effects on the fetus and neonate resulting from maternal exposure during gestation and lactation. Relevant animal and in vitro models are also discussed.
Children are not small adults. They differ from adults in their exposures and may differ in their susceptibility to hazardous chemicals. Children’s unique physiology and behavior can influence the extent of their exposure. Exposures of children are discussed in Section 6.6, Exposures of Children.
Children sometimes differ from adults in their susceptibility to hazardous chemicals, but whether there is a difference depends on the chemical (Guzelian et al. 1992; NRC 1993). Children may be more or less susceptible than adults to health effects, and the relationship may change with developmental age (Guzelian et al. 1992; NRC 1993). Vulnerability often depends on developmental stage. There are critical periods of structural and functional development during both prenatal and postnatal life, and a particular structure or function will be most sensitive to disruption during its critical period(s). Damage may not be evident until a later stage of development. There are often differences in pharmacokinetics and metabolism between children and adults. For example, absorption may be different in neonates because of the immaturity of their gastrointestinal tract and their larger skin surface area in proportion to body weight (Morselli et al. 1980; NRC 1993); the gastrointestinal absorption of lead is greatest in infants and young children (Ziegler et al. 1978). Distribution of xenobiotics may be different; for example, infants have a larger proportion of their bodies as extracellular water, and their brains and livers are proportionately larger (Altman and Dittmer 1974; Fomon 1966; Fomon et al. 1982; Owen and Brozek 1966; Widdowson and Dickerson 1964). The fetus/infant has an immature (developing) blood-brain barrier that past literature has often described as being leaky and poorly intact (Costa et al. 2004). However, current evidence suggests that the blood-brain barrier is anatomically and physically intact at this stage of development, and the restrictive intracellular junctions that exist at the blood-CNS interface are fully formed, intact, and functionally effective (Saunders et al. 2008, 2012).
However, during development of the blood-brain barrier, there are differences between fetuses/infants and adults which are toxicologically important. These differences mainly involve variations in physiological transport systems that form during development (Ek et al. 2012). These transport mechanisms (influx and efflux) play an important role in the movement of amino acids and other vital substances across the blood-brain barrier in the developing brain; these transport mechanisms are far more active in the developing brain than in the adult. Because many drugs or potential toxins may be transported into the brain using these same transport mechanisms—the developing brain may be rendered more vulnerable than the adult. Thus, concern regarding possible involvement of the blood-brain barrier with enhanced susceptibility of the developing brain to toxins is valid. It is important to note however, that this potential selective vulnerability of the developing brain is associated with essential normal physiological mechanisms; and not because of an absence or deficiency of anatomical/physical barrier mechanisms.
The presence of these unique transport systems in the developing brain of the fetus/infant is intriguing; as it raises a very important toxicological question as to whether these mechanisms provide protection for the developing brain or do they render it more vulnerable to toxic injury. Each case of chemical exposure should be assessed on a case-by-case basis. Research continues into the function and structure of the blood-brain barrier in early life (Kearns et al. 2003; Saunders et al. 2012; Scheuplein et al. 2002).
Many xenobiotic metabolizing enzymes have distinctive developmental patterns. At various stages of growth and development, levels of particular enzymes may be higher or lower than those of adults, and sometimes unique enzymes may exist at particular developmental stages (Komori et al. 1990; Leeder and Kearns 1997; NRC 1993; Vieira et al. 1996). Whether differences in xenobiotic metabolism make the child more or less susceptible also depends on whether the relevant enzymes are involved in activation of the parent compound to its toxic form or in detoxification. There may also be differences in excretion, particularly in newborns who all have a low glomerular filtration rate and have not developed efficient tubular secretion and resorption capacities (Altman and Dittmer 1974; NRC 1993; West et al. 1948). Children and adults may differ in their capacity to repair damage from chemical insults. Children also have a longer remaining lifetime in which to express damage from chemicals; this potential is particularly relevant to cancer.
Certain characteristics of the developing human may increase exposure or susceptibility, whereas others may decrease susceptibility to the same chemical. For example, although infants breathe more air per kilogram of body weight than adults breathe, this difference might be somewhat counterbalanced by their alveoli being less developed, which results in a disproportionately smaller surface area for alveolar absorption (NRC 1993).
The only study of children directly exposed to endosulfan located is that of Rau et al. (2012) who examined the association between levels of endosulfan in the bone marrow from children and acute hematological malignancies. The investigators also assessed whether children with high levels of endosulfan in their marrow resided in areas sprayed with endosulfan. The cohort consisted of 26 children with proven hematological malignancy and 26 age-matched controls with benign hematological disease. The major illness among the cases was acute lymphoblastic leukemia (n=23), whereas immune thrombocytopenic purpura occurred in 50% of controls (n=13). Six out of 26 cases tested positive for endosulfan in the bone marrow, compared to 1 out of 26 controls; the concentration of endosulfan in the bone marrow was not provided. All of the children with elevated endosulfan in the bone marrow resided in the areas sprayed with the pesticide. The small sample size and the lack of control for potential confounders make this report of questionable value.
Studies of adults, mostly cases of accidental or intentional acute exposure (ingestion) to large amounts of endosulfan, indicate that the primary target of endosulfan toxicity is the nervous system. The effects are manifested as hyperactivity and convulsions and, in some cases, have resulted in death (Bernardelli and Gennari 1987; Blanco-Coronado et al. 1992; Boereboom et al. 1998; Demeter and Heyndrickx 1978; Eyer et al. 2004; Lo et al. 1995; Moon and Chun 2009; Moses and Peter 2010; Parbhu et al. 2009; Terziev et al. 1974). Children would likely experience the same health effects seen in adults exposed to endosulfan as evidenced by a case in which a 2-year-old girl developed seizures after her mother applied an unspecified endosulfan formulation on her head to remove lice (Jindal and Sankhyan 2012). An additional case reported clonic status epilepticus in a 2-year-old girl who accidentally ingested an unspecified endosulfan formulation (Kamate and Jain 2011). These effects of endosulfan in humans have been reproduced in experimental animals.
Results from a few animal studies suggest that, for some end points, young and older animals exhibit different susceptibility. For example, a study conducted in rat pups in which the animals were treated intraperitoneally with 1 mg of technical endosulfan/kg/day for 25 days beginning at 1 day of age found a significant increase in the binding of serotonin to frontal cortical membranes, which could have been due to an increase in the maximum number of binding sites or to alterations in receptor affinity (Zaidi et al. 1985). The increased binding correlated well with an increase in aggressive behavior and could be reversed by administration of a serotonin blocker; the NOAEL was 0.5 mg/kg/day. Exposure of adults to 3 mg/kg for 30 consecutive days induced significant increase in binding but of a lesser magnitude than in developing rats (Seth et al. 1986). Without further elaboration, Seth et al. (1986) suggested that the increased sensitivity showed by the pups may be due to the fact that serotonergic receptors develop postnatally. However, adult rats were not tested with 1 mg/kg/day endosulfan for 30 days, so it is not known whether or not toxicity would be comparable. Neither of these studies provided information regarding the purity of the test substance. Kiran and Varma (1988) administered an unspecified endosulfan formulation orally for 4 days at 12.5 mg/kg/day to rats of four different ages (15, 30, 70, and 365 days old) and found that in older animals, endosulfan produced body tremors and muscular contractions, as well as hyperglycemia and reduction in liver glycogen content. None of these effects were observed in the 15-day-old pups, but endosulfan did reduce the activity of erythrocyte Na+-K+-ATPase in this age group. No explanation was offered for this differential effect. If, as discussed in Section 3.5.2, endosulfan-induced convulsive activity is caused by inhibition of GABAergic systems, an immature GABAergic system in the 15-day-old pups may have been responsible for the lack of such activity. The results from these studies suggest that the determination of whether young animals are more susceptible than older ones or vice versa is influenced by the specific neurological response that is affected. The neurological response, in turn, depends on the degree of maturation of the neurotransmitter system(s) responsible for that response.
An additional study reported age-dependent effects. Lakshmana and Raju (1994) found that oral treatment of rat pups with endosulfan from postnatal days 2 to10 resulted in changes in the concentration of noradrenalin, dopamine, and serotonin in various brain areas that differed either in magnitude or direction from changes seen in pups treated from postnatal days 2 to 23. While the results from this study do not necessarily indicate that neonates are more sensitive to the toxic effects of endosulfan, they do show that the duration of exposure in neonates is an important parameter to consider.
Three recent studies, one in rats (Lafuente and Pereiro 2013) and two in mice (Wilson et al. 2014a, 2014b), showed that maternal exposure to endosulfan during gestation and/or lactation can result in changes in levels of neurotransmitters (GABA, glutamate, dopamine, norepinephrine, serotonin) in the brain of young adult offspring. In addition, exposure of rats early during development rendered them more sensitive to the effects of exposure to a dopaminergic neurotoxin later in life (Wilson et al. 2014b).
Differential susceptibility between young and older animals has also been found regarding other end points. Studies by Sinha et al. (1995, 1997) found that oral treatment of 3-week-old male rats with endosulfan for 90 days resulted in reduced intratesticular spermatid count and increased percent of abnormal sperm at doses lower than those that caused similar effects in 3-month-old rats treated for 70 days. This led the authors to conclude that exposure during a period of testicular maturation when spermatogenesis is in progress may result in disturbed spermatogenesis at sexual maturity.
A limited number of studies in humans have provided suggestive evidence of associations between maternal exposure to endosulfan and developmental alterations in the offspring including autism spectrum disorders (Roberts et al. 2007), alterations in thyroid function (Freire et al. 2011, 2012), neural tube defects (Ren et al. 2011), and delayed sexual maturity in male children (Saiyed et al. 2003). After considering the strengths and limitations of the individual studies, no definite conclusions could be drawn. A recent study of Chinese women found no significant association between levels of α-endosulfan in maternal or cord blood and infant birth weight (Guo et al. 2014).
Endosulfan induced adverse developmental effects in animals; most studies were conducted in rats. It should be noted that since not all studies provide information regarding maternal effects, it is not totally clear whether the developmental effects occur only in the presence of maternal toxicity. Perinatal exposure of rats to endosulfan induced skeletal variations (FMC 1980; Gupta et al. 1978), reduced offspring weight (Cabaleiro et al. 2008; Caride et al. 2010; Gilmore et al. 2006; Hoechst 1982, 1984a), and altered sperm parameters in adult male offspring that were not directly exposed, but received gestational and/or lactational exposure to endosulfan (Dalsenter et al. 1999; Sinha et al. 2001). Two of the most recent studies in which Wistar rats were exposed to 1 mg/kg/day endosulfan (only dose level tested) on GDs 6–20 reported significant increases in the incidences of gross, visceral, and skeletal anomalies, and hepatocyte and renal tubule epithelium degeneration in GD 20 fetuses (Singh et al. 2007a, 2008). The lowest developmental LOAEL was 0.61 mg/kg/day for an 11% reduction in body weight from Sprague-Dawley male rat pups on PND 21 after treatment of the dams by gavage during the entire gestation and lactation periods (Cabaleiro et al. 2008; Caride et al. 2010); the 0.61 mg/kg/day dose was the lowest dose tested. Interestingly, treatment of Wistar rats with a much higher dose, 29.8 mg/kg/day endosulfan, via the diet on GDs 6–21 and during lactation resulted in a reduction of 11 and 4% in male and female pup’s weight, respectively, on PND 21 (Gilmore et al. 2006). The apparent difference in sensitivity may be related to the different strains used and to the different mode of administration of the test material (i.e., gavage versus the diet). Endosulfan was not estrogenic in in vivo assays in immature female rats (Raizada et al. 1991; Wade et al. 1997) or mice (Hiremath and Kaliwal 2003; Shelby et al. 1996), and exhibited mixed positive and negative results with respect to estrogenic properties in various in vitro assays (see further details in Section 3.6).
There is no information regarding pharmacokinetics of endosulfan in children or regarding nutritional factors that may influence the absorption of endosulfan. Endosulfan has been detected in the breast milk of women environmentally exposed (Cerrillo et al. 2005; Çok et al. 2011; Lutter et al. 1998), in the placenta (Cerrillo et al. 2005; Freire et al. 2011), and in cord blood (Mariscal-Arcas et al. 2010). The results from these studies indicate that transfer of endosulfan and/or metabolites to the fetus and nursing babies can occur. In a study in which lactating goats were administered endosulfan for 28 days, only trace amounts of endosulfan residues were transferred to the nursing kids (Indraningsih et al. 1993). In milk sheep, approximately 1% of radioactivity administered in a single oral dose of 14C-endosulfan was recovered in the milk as endosulfan sulfate at approximately 1 day postdosing; the concentration in the milk declined to very low levels by 8 days postexposure, but was still detectable in milk at 2 ppb at 22 days postexposure (the end of the study) (Gorbach et al. 1968). There is no information on the metabolism of endosulfan in children. Because endosulfan is rapidly eliminated from the body after exposure, there is little likelihood that the chemical from preconception exposures in women would be present in the body during pregnancy or lactation. Although there is evidence that endosulfan induces microsomal cytochrome P450 in animals (Siddiqui et al. 1987a; Tyagi et al. 1984), the specific mechanism of endosulfan metabolism is not known, and therefore, no conclusion about developmental regulation can be drawn.
There are no biomarkers of exposure or effect for endosulfan that have been validated in children or adults exposed as children. Isomers and metabolites of endosulfan were detected in the fat of 30–40% of children hospitalized in agricultural regions of Spain (Olea et al. 1999). The adipose endosulfan was presumably from recent dietary exposure in the light of evidence in a rat study (Dorough et al. 1978), indicating that endosulfan is rapidly eliminated from fat tissues after cessation of dietary exposure. However, methods for obtaining samples of fat are relatively invasive, so adipose endosulfan may not be practicable as a routine biomarker of recent exposure in children. No studies were located regarding interactions of endosulfan with other chemicals in children. Information regarding interactions of endosulfan with other chemicals in humans is limited to anecdotic reports, and inference to what might occur in children based on those reports might be inappropriate.
No information was located regarding pediatric-specific methods for reducing peak absorption following exposure to endosulfan, reducing body burden, or interfering with the mechanism of action for toxic effects. No data were located regarding whether methods for reducing toxic effects of endosulfan used in adults might be contraindicated in children. No data were available on whether methods for reducing toxic effects of endosulfan used in adults have been validated in children.
There is no information regarding possible transgenerational effects of endosulfan exposure in humans and the limited data in animals are insufficient to establish whether such effects might occur. For example, a statistically significant increase in chromosomal aberrations was observed in mouse spermatocytes 60 days after initial treatment with oral doses of endosulfan of 6.4 mg/kg/day for 5 days (Usha Rani and Reddy 1986). In male rats, acute exposure to doses of up to 22 mg/kg/day of endosulfan for 5 days did not induce chromosomal aberrations in spermatogonial cells (Dikshith and Datta 1978). The ratios of mitotic index and frequency of chromatid breaks in the two cell types had no correlation with the doses tested and were not significantly different from the control group. Oral administration of 11.6 mg/kg/day of endosulfan to rats for up to 30 days also failed to induce chromosomal damage in spermatogonial cell systems, but it is not known how soon after treatment the animals were killed, and as shown in mouse studies (Usha Rani and Reddy 1986), a latency period of 60 days was required to see chromosomal aberrations in spermatogonia. However, relatively significant changes were observed for mitotic indices at doses that also caused lethality (Dikshith et al. 1978).
3.8. BIOMARKERS OF EXPOSURE AND EFFECT
Biomarkers are broadly defined as indicators signaling events in biologic systems or samples. They have been classified as markers of exposure, markers of effect, and markers of susceptibility (NAS/NRC 1989).
A biomarker of exposure is a xenobiotic substance or its metabolite(s) or the product of an interaction between a xenobiotic agent and some target molecule(s) or cell(s) that is measured within a compartment of an organism (NAS/NRC 1989). The preferred biomarkers of exposure are generally the substance itself, substance-specific metabolites in readily obtainable body fluid(s), or excreta. However, several factors can confound the use and interpretation of biomarkers of exposure. The body burden of a substance may be the result of exposures from more than one source. The substance being measured may be a metabolite of another xenobiotic substance (e.g., high urinary levels of phenol can result from exposure to several different aromatic compounds). Depending on the properties of the substance (e.g., biologic half-life) and environmental conditions (e.g., duration and route of exposure), the substance and all of its metabolites may have left the body by the time samples can be taken. It may be difficult to identify individuals exposed to hazardous substances that are commonly found in body tissues and fluids (e.g., essential mineral nutrients such as copper, zinc, and selenium). Biomarkers of exposure to endosulfan are discussed in Section 3.8.1.
Biomarkers of effect are defined as any measurable biochemical, physiologic, or other alteration within an organism that, depending on magnitude, can be recognized as an established or potential health impairment or disease (NAS/NRC 1989). This definition encompasses biochemical or cellular signals of tissue dysfunction (e.g., increased liver enzyme activity or pathologic changes in female genital epithelial cells), as well as physiologic signs of dysfunction such as increased blood pressure or decreased lung capacity. Note that these markers are not often substance specific. They also may not be directly adverse, but can indicate potential health impairment (e.g., DNA adducts). Biomarkers of effects caused by endosulfan are discussed in Section 3.8.2.
A biomarker of susceptibility is an indicator of an inherent or acquired limitation of an organism’s ability to respond to the challenge of exposure to a specific xenobiotic substance. It can be an intrinsic genetic or other characteristic or a preexisting disease that results in an increase in absorbed dose, a decrease in the biologically effective dose, or a target tissue response. If biomarkers of susceptibility exist, they are discussed in Section 3.10, Populations That Are Unusually Susceptible.
3.8.1. Biomarkers Used to Identify or Quantify Exposure to Endosulfan
The primary biomarkers for endosulfan exposure include tissue and excreta concentrations of endosulfan, or its metabolite, endosulfan sulfate. Other metabolites that can be detected include endosulfan diol, hydroxyether, and endosulfan lactone (Hayes 1982; WHO 1984). In animals, the metabolites appear in the tissues and excreta following exposure to endosulfan (Chan et al. 2005; Deema et al. 1966; Dorough et al. 1978). These water-soluble metabolites are rapidly formed and excreted in the urine and feces. Elevated levels of both α- and β-endosulfan, but not endosulfan metabolites, were detected in the urine of a pest control worker (who wore protective equipment) after a single 25-minute exposure to endosulfan in a greenhouse application. Urinary endosulfan declined to control levels by about 1.5 days postexposure (Arrebola et al. 1999). α-Endosulfan and β-endosulfan were detected in the urine of workers who had applied endosulfan on the day prior to urine sampling, and were at lower levels in workers who had been occupationally exposed 1 week prior to sampling (Vidal et al. 1998). Metabolites (endosulfan ether, endosulfan sulfate, and endosulfan lactone) were either infrequently detected or occurred at relatively low levels in the urine. Endosulfan was detected in the serum of an agricultural pilot 30 hours after his clothes became soaked with endosulfan and methomyl (Cable and Doherty 1999). Endosulfan has been detected in breast milk of women exposed environmentally to endosulfan (Cerrillo et al. 2005; Çok et al. 2011; Lutter et al. 1998), and endosulfan sulfate has been detected in sheep breast milk following consumption of large oral doses (Gorbach et al. 1968). Isomers and metabolites of endosulfan were detected in the fat of 30–40% of children hospitalized in agricultural regions of Spain, demonstrating that endosulfan accumulates in adipose tissue of young humans after repeated dietary exposure (Olea et al. 1999). Endosulfan and metabolites have also been detected in placenta (Cerrillo et al. 2005; Freire et al. 2011) and cord blood (Mariscal-Arcas et al. 2010). No other biomarkers of exposure specific for endosulfan were identified in the available literature. No studies were located that quantified the concentrations of endosulfan or its metabolites in relation to specific environmental exposure concentrations.
3.8.2. Biomarkers Used to Characterize Effects Caused by Endosulfan
There are no biomarkers of effect specific for endosulfan in humans. The characteristic effects of acute exposure to high amounts of endosulfan in humans and animals are signs of overactivity of the nervous system such as hyperexcitability, tremors, and convulsions. Although many types of chemicals can induce seizures, non-specific biomarkers of clinical severity after poisoning exist; increased serum creatine kinase activity is a marker of seizure-induced rhabdomyolysis and increased serum lactate is a marker of metabolic acidosis. Other systemic effects observed in these cases are likely to be secondary to neurological effects. There are no studies of humans subjected to long-term exposure to low levels of endosulfan; therefore, no biomarkers for that type of exposure have been identified. The organ most sensitive to longer-term endosulfan exposure of animals appears to be the kidney; however, this effect also is not specific for endosulfan exposure (Hoechst 1989a).
3.9. INTERACTIONS WITH OTHER CHEMICALS
There are two reports that involved death in humans after simultaneous exposure to endosulfan and alcohol, but the nature of the interaction is not totally clear and the evidence is weak at best. In one death (Demeter et al. 1977), the blood alcohol concentration in a subject was 1.81 g/L (which is not considered lethal), and the quantity of endosulfan consumed (although the exact quantity was not reported) was also unlikely to be fatal when ingested alone. Thus, the authors suggested that the endosulfan and alcohol acted synergistically to result in death. It is also possible that alcohol interfered with the metabolism of endosulfan in the liver and, therefore, delayed endosulfan elimination. On the other hand, the presence of alcohol would probably have been protective since it usually increases the threshold for seizures. A second fatal outcome was reportedly caused by ingestion of alcohol and Posidor (20% endosulfan and 30% dimethoate in xylene) (Demeter et al. 1978). Dimethoate is an organophosphate insecticide and a potent inhibitor of the cholinesterase; it was considered by the authors to be much more acutely toxic than endosulfan. It is possible that some of the effects, such as hyperactivity, tremors, and seizures, may have resulted from the combined contribution of the two pesticides, although through different mechanisms. The investigators did not discuss what the role of alcohol might have been in this case.
Endosulfan has been documented to be an enzyme inducer of the cytochrome P450-dependent monooxygenase system in several studies with experimental animals (Agarwal et al. 1978; Den Tonkelaar and Van Esch 1974; Gupta and Gupta 1977a; Kiran and Varma 1988; Siddiqui et al. 1987a; Sriram and Misra 1983). Vitamin A was found to inhibit the activity of cytochrome P450-dependent monooxygenase systems induced by endosulfan. Specific parameters included microsomal protein and cytochrome P450 contents and the activities of NADPH-cytochrome c reductase, aminopyrine N-demethylase, and aniline hydroxylase (Sriram and Misra 1983). Endosulfan and pentobarbital have also demonstrated an interactive effect. Endosulfan reduced the sleeping time induced in male rats by the administration of sodium pentobarbitone (Balasubramamian et al. 1996). The induction of hepatic microsomal enzyme activity and the enhanced metabolism of the pentobarbitone caused by endosulfan are the probable mechanisms, as evidenced by reduced pentobarbitone concentrations in the blood and brain of endosulfan-treated rats (Balasubramamian et al. 1996; Den Tonkelaar and Van Esch 1974; Gupta and Gupta 1977a).
Phenobarbital has a mitigating effect on endosulfan toxicity in rats (Hoechst 1984e). The acute lethal toxicity and neurotoxicity of endosulfan were decreased when phenobarbital (50–70 mg/kg) was given following the appearance of toxic signs. In contrast, diazepam (2–60 mg/kg) delayed death but did not prevent it. It is possible that phenobarbital-induced microsomal enzymes increased the metabolism of endosulfan.
Endosulfan promoted the hypnotic effects of diazepam by prolonging the duration of the loss of righting reflex (Balasubramamian et al. 1996). The investigators speculated that endosulfan increased the potency of diazepam by increasing the binding sites for diazepam in the brain synaptic membranes and/or promoted its biotransformation to a longer-acting metabolite, oxazepam; however, little evidence was presented in support their speculation (Balasubramamian et al. 1996). In the same study, endosulfan promoted the convulsant action of picrotoxin by shortening the convulsion latency and increasing convulsion frequency. It is also possible that endosulfan enhanced the convulsant activity of picrotoxin because both compounds act at the GABA receptor.
Other studies have reported negative findings following the investigation of possible interactions between endosulfan and various compounds. In rats, treatment with endosulfan did not potentiate or aggravate the adverse liver effects induced by pretreatment with carbon tetrachloride (Dikshith and Raizada 1983). The in vitro estrogenic effects of endosulfan and dieldrin were found to be additive but not synergistic (Wade et al. 1997). Endosulfan estrogenicity in transfected yeast was inhibited by coexposure with other pesticides. Endosulfan induced human ER-mediated β-galactosidase (β-gal) activity at 100 μM in an estrogen-responsive reporter system in yeast, but did not induce human ER-mediated β-gal activity at ≤10 μM exposure levels (Ramamoorthy et al. 1997). Binary mixtures of endosulfan with chlordane, toxaphene, and dieldrin induced significantly less activity than endosulfan alone. No additive, antagonistic, or potentiating effects were observed in rats treated with endosulfan and metepa (a chemosterilant used to control insect vectors) (Nath et al. 1978).
Cytotoxic synergism between endosulfan and other organochlorine pesticides was demonstrated in an in vitro assay of growth inhibition of ER-negative SK-BR-3 human breast cancer cells, but was not demonstrated in a parallel assay using ER-positive MCF-7 cells (Hsu et al. 1998). The concentration at which 50% growth inhibition (IC50) was achieved in SK-BR-3 cells was approximately 35 μM for endosulfan and dieldrin individually, but the IC50 was 0.1 μM for the mixture of the two pesticides. Similarly, the IC50 value for chlordane alone was 3.5 μM, but in combination with endosulfan, the IC50 was 0.2 μM. A lack of synergism between α- or β-endosulfan and dieldrin was also seen in a foci-induction assay with MCF-7 cells, in which the endosulfan isomers individually were weak inducers of foci at 10 μM (Arcaro et al. 1998).
Simultaneous exposure of rats to endosulfan and either ochratoxin or citrinin (both nephrotoxic mycotoxins) resulted in additive adverse hepatic, renal, and lymphoreticular effects in females, altered hormone levels (T3, T4, testosterone, prolactin, insulin, cortisol) in males, and embryotoxic and teratogenic effects (Kumar et al. 2011; Singh et al. 2007a, 2007b).
3.10. POPULATIONS THAT ARE UNUSUALLY SUSCEPTIBLE
A susceptible population will exhibit a different or enhanced response to endosulfan than will most persons exposed to the same level of endosulfan in the environment. Reasons may include genetic makeup, age, health and nutritional status, and exposure to other toxic substances (e.g., cigarette smoke). These parameters result in reduced detoxification or excretion of endosulfan, or compromised function of organs affected by endosulfan. Populations who are at greater risk due to their unusually high exposure to endosulfan are discussed in Section 6.7, Populations with Potentially High Exposures.
The limited toxicity data available for endosulfan suggest that two subgroups of the population may be more susceptible to endosulfan exposure than the general population. These subgroups include people with kidney or neurological diseases.
The central nervous system is a major target of endosulfan-induced toxicity in both humans and animals (Bernardelli and Gennari 1987; Blanco-Coronado et al. 1992; Boereboom et al. 1998; Boyd and Dobos 1969; Demeter and Heyndrickx 1978; Eyer et al. 2004; Gilbert and Mack 1995; Hoechst 1970, 1975, 1984e; Lo et al. 1995; Moon and Chun 2009; Moses and Peter 2010; Parbhu et al. 2009; Terziev et al. 1974). Therefore, individuals with seizure disorders, such as epilepsy, may be particularly susceptible because exposure to endosulfan may reduce the threshold for tremors, seizures, and other forms of neurotoxicity, as demonstrated in studies in rats (Gilbert 1992; Gilbert and Mack 1995).
A study of 150 people from India in different stages of chronic kidney disease and 96 healthy controls reported that those with chronic kidney disease had significantly higher serum levels of organochlorine pesticides (α- and β-endosulfan among them) than the controls (Siddharth et al. 2012). Kidney disease was defined as having a glomerular filtration rate ≤60 mL/minute. The subjects had not been occupationally exposed to pesticides. The conclusion of the study was that individuals with an impaired glomerular filtration rate tend to accumulate pesticides (and possibly other substances as well).
Several studies conducted in experimental animals have demonstrated that diets deficient in protein exacerbate the oral toxicity of endosulfan (Boyd 1972; Boyd et al. 1970; Das and Garg 1981). These results suggest that people who consume low-protein diets, such as chronic alcoholics, dieters, food faddists, various cults, some ethnic groups, the elderly, and some people living in depressed areas or underdeveloped countries, may be more susceptible to the toxic effects of endosulfan.
A recent study examined the role of CYP17A1 gene polymorphism on the effect of organochlorine pesticides (endosulfan among them) on the risk of small for gestational age (Chand et al. 2014). The CYP17A1 gene is involved in key steps of estrogen synthesis, which affect fetal growth. The study found a significant association between high levels of endosulfan in cord blood and the wild-type genotype (A1A1) of CYP17A1, which led to an estimated reduction in birth weight of 0.315 kg. It should be noted, however, that a plot of birth weight against levels of endosulfan in cord blood yielded a correlation coefficient (r) of −0.413 for the wild-type genotype. This means that the coefficient of determination (r2) is 0.17, indicating that 17% of the total variation in birth weight in women carrying the wild-type genotype can be attributed to endosulfan in cord blood and 83% to unexplained causes.
A detailed discussion of children’s susceptibility can be found in Section 3.7, Children’s Susceptibility.
3.11. METHODS FOR REDUCING TOXIC EFFECTS
This section will describe clinical practice and research concerning methods for reducing toxic effects of exposure to endosulfan. However, because some of the treatments discussed may be experimental and unproven, this section should not be used as a guide for treatment of exposures to endosulfan. When specific exposures have occurred, poison control centers and medical toxicologists should be consulted for medical advice. No texts were located that provide information about treatment following specific exposure to endosulfan.
3.11.1. Reducing Peak Absorption Following Exposure
The following has been extracted from HSDB (2010). Procedures that have been used in an acute exposure situation to limit absorption of endosulfan include the following. In inhalation and dermal exposures, the exposed person is first removed from the source of exposure. Contaminated clothing should be removed and the skin and hair should be washed three times. An initial soap washing can be followed by an alcohol washing and another soap washing to remove unabsorbed material. Since leather absorbs pesticides, it is recommended that leather not be worn in the presence of pesticides and all contaminated leather should be discarded. If eye exposure occurs, the exposed eyes should be irrigated with copious amounts of room temperature water for at least 15 minutes. If irritation, pain, swelling, lacrimation, or photophobia persists, the patient should be seen in a health care facility. After acute high-dose oral exposures, absorption from the gastrointestinal tract may be limited by gastric lavage, generally within 1 hour of ingestion. Gastric lavage can be followed by administration of activated charcoal as a slurry; the usual dose is 25–100 g in adults/adolescents, 25–50 g in children (1–12 years old), and 1 g/kg in infants <1year old. However, there is no evidence of benefit from either charcoal or gastric lavage and clear evidence of harm if the latter is done badly. Gastric lavage may be indicated in patients who are comatose or at risk of convulsing. Oil-based cathartics may facilitate gastrointestinal absorption and, therefore, are not used.
3.11.2. Reducing Body Burden
The only relevant information located was that administration of cholestyramine resin may increase fecal excretion of endosulfan trapped in the enterohepatic circulation (Dreisbach and Robertson 1987; HSDB 2010).
3.11.3. Interfering with the Mechanism of Action for Toxic Effects
The primary life-threatening effect produced following exposure to high levels of endosulfan is respiratory paralysis resulting from the development of refractory status epilepticus. It is therefore important to urgently control seizures. Diazepam (Aleksandrowicz 1979; Blanco-Coronado et al. 1992), midazolam followed by a 30-minute loading dose of phenytoin (Cable and Doherty 1999), and phenobarbitone (Chugh et al. 1998; Eyer et al. 2004; Yavuz et al. 2007); diazepam followed by phenytoin (Jindal and Sankhyan 2012) have been used to treat tonic-clonic seizures following exposure to high amounts of endosulfan. Lorazepam has also been recommended (HSDB 2010). Phenobarbital or propofol has been suggested if seizures are uncontrollable or recur after diazepam (HSDB 2010). Phenytoin is not recommended for seizures in poisoned patients (Shah and Eddleston 2010). General anesthesia with EEG monitoring should be instigated urgently if diazepam and phenobarbital are not rapidly effective.
Several studies have reported on the amielorating action of antioxidant substances on the effects of endosulfan in animals. The most studied antioxidant appears to be vitamin C. Studies have reported that simultaneous administration of vitamin C and endosulfan reduced alterations induced by endosulfan on reproductive effects in rats and rabbits (Ata et al. 2007; Ozmen and Mor 2012; Rao et al. 2005), on genotoxic effects in rats (Manjula et al. 2006), on neurotoxicity in rabbits (Mor and Ozmen 2010), and in parameters of immune response in rats (Pal et al. 2009). Similar mitigating activity has been reported for vitamin E (Jalili et al. 2007; Kalender et al. 2004a; Mahran 2013; Mansour et al. 2014; Nagda et al. 2011; Pal et al. 2009; Wang et al. 2012; Zervos et al. 2011), melatonin (Omurtag et al. 2008), taurine (Aly and Khafagy 2014), and 5-aminosalicylic acid (Jaiswal et al. 2005). There is strong evidence that the mechanism by which these substances ameliorate the effects of endosulfan is by preventing the endosulfan-induced formation of reactive oxygen species and lipid peroxidation (El-Shenawy 2010; Kannan and Jain 2003; Omurtag et al. 2008; Ozdem et al. 2011; Zervos et al. 2011).
No treatment strategies were located for chronic low-level exposures to endosulfan.
3.12. ADEQUACY OF THE DATABASE
Section 104(I)(5) of CERCLA, as amended, directs the Administrator of ATSDR (in consultation with the Administrator of EPA and agencies and programs of the Public Health Service) to assess whether adequate information on the health effects of endosulfan is available. Where adequate information is not available, ATSDR, in conjunction with the National Toxicology Program (NTP), is required to assure the initiation of a program of research designed to determine the health effects (and techniques for developing methods to determine such health effects) of endosulfan.
The following categories of possible data needs have been identified by a joint team of scientists from ATSDR, NTP, and EPA. They are defined as substance-specific informational needs that if met would reduce the uncertainties of human health assessment. This definition should not be interpreted to mean that all data needs discussed in this section must be filled. In the future, the identified data needs will be evaluated and prioritized, and a substance-specific research agenda will be proposed.
3.12.1. Existing Information on Health Effects of Endosulfan
The existing data on health effects of inhalation, oral, and dermal exposure of humans and animals to endosulfan are summarized in Figure 3-5. The purpose of this figure is to illustrate the existing information concerning the health effects of endosulfan. Each dot in the figure indicates that one or more studies provide information associated with that particular effect. The dot does not necessarily imply anything about the quality of the study or studies, nor should missing information in this figure be interpreted as a “data need”. A data need, as defined in ATSDR’s Decision Guide for Identifying Substance-Specific Data Needs Related to Toxicological Profiles (Agency for Toxic Substances and Disease Registry 1989), is substance-specific information necessary to conduct comprehensive public health assessments. Generally, ATSDR defines a data gap more broadly as any substance-specific information missing from the scientific literature.
Most of the literature reviewed concerning the health effects of endosulfan in humans described case reports and case series of occupational exposure and accidental or intentional ingestion of endosulfan. The cases of occupational exposure to endosulfan concerned exposures of acute-to-intermediate durations, and the cases of oral exposure were exclusively acute-duration exposure situations. The predominant route of exposure in the occupational case reports/series is believed to be inhalation, but the possibility of some degree of dermal exposure cannot be ruled out. The information on human exposure is limited because the possibility of concurrent exposure to other pesticides or other toxic substances cannot be excluded. In addition, the precise duration and level of exposure to endosulfan generally cannot be quantified from the information presented in these reports.
The database for the health effects of endosulfan following ingestion in experimental animals is substantial. However, as can be seen in Figure 3-5, somewhat less information is available on the effects of inhalation and dermal exposure to endosulfan in animals. Furthermore, the health effects associated with acute- and intermediate-duration inhalation and dermal exposure are more fully characterized than those associated with chronic inhalation or dermal exposure. There is no evidence suggesting that the toxicity of endosulfan is route-specific. However, ingested endosulfan should reach the liver sooner.

Figure 3-5
Existing Information on Health Effects of Endosulfan.
People living near hazardous waste sites may be exposed to endosulfan primarily via dermal contact with or ingestion of contaminated soils since endosulfan is found bound to soil particles. Another possible mechanism for oral exposure to endosulfan is the ingestion of pesticide-laden dust from a waste site or treated field carried by the wind and deposited on garden crops. Ingestion of contaminated water is not expected to be a significant route of exposure since endosulfan is not very water soluble and is generally not found in groundwater. Likewise, inhalation exposure to endosulfan is not a major route of exposure since endosulfan is generally detected at low levels in the ambient atmosphere. For the general population, the primary route of exposure to endosulfan is via ingestion of residues on contaminated foods. Therefore, information on the toxicity of endosulfan following ingestion and dermal exposure is most relevant for individuals living in the vicinity of hazardous waste sites.
3.12.2. Identification of Data Needs
Acute-Duration Exposure. Information is available regarding the effects of acute-duration exposure in humans following inhalation, oral, and dermal exposure to high levels of endosulfan (Aleksandrowicz 1979; Bernardelli and Gennari 1987; Blanco-Coronado et al. 1992; Boereboom et al. 1998; Demeter and Heyndrickx 1978; Ely et al. 1967; Eyer et al. 2004; Jindal and Sankhyan 2012; Karatas et al. 2006; Lo et al. 1995; Moon and Chun 2009; Parbhu et al. 2009; Schuman and Dobson 1985; Shemesh et al. 1988; Singh et al. 1992; Terziev et al. 1974). In animals, information is available following exposures by all three routes (Boyd and Dobos 1969; Boyd et al. 1970; Caglar et al. 2003; Den Tonkelaar and Van Esch 1974; FMC 1958, 1972, 1980; Gilbert and Mack 1995; Gupta and Chandra 1975; Gupta and Gupta 1977a; Gupta et al. 1978, 1981; Hoechst 1966a, 1966b, 1970, 1975, 1983a, 1984e, 1988c, 1989b; Hiremath and Kaliwal 2002a; Lakshmana and Raju 1994; Lindquist and Dahm 1957; Misra et al. 1980; Nicholson and Cooper 1977; Siddiqui et al. 1987b; Sinha et al. 2001; Terziev et al. 1974; Umar et al. 2012; Wilson and LeBlanc 1998). Endosulfan may be lethal to humans and animals by all routes of exposure studied, depending on dose (Bernardelli and Gennari 1987; Boereboom et al. 1998; Blanco-Coronado et al. 1992; Boyd and Dobos 1969; Boyd et al. 1970; Demeter and Heyndrickx 1978; Eyer et al. 2004; Gupta and Chandra 1975; Gupta et al. 1978, 1981; Hoechst 1966a, 1966b, 1975, 1983a, 1989b; Lindquist and Dahm 1957; Lo et al. 1995; Nicholson and Cooper 1977; Parbhu et al. 2009; Terziev et al. 1974). The main target of toxicity in humans and animals following acute, high-level exposure by any route is the central nervous system (Aleksandrowicz 1979; Boereboom et al. 1998; Boyd and Dobos 1969; Boyd et al. 1970; Cable and Doherty 1999; Chugh et al. 1998; Ely et al. 1967; Eyer et al. 2004; FMC 1958, 1959a, 1980; Gilbert and Mack 1995; Gupta and Chandra 1975; Hoechst 1970, 1975, 1983a, 1984e, 1989b; Karatas et al. 2006; MacKenzie et al. 1981; Moon and Chun 2009; Mor and Ozmen 2010; Moses and Peter 2010; Nicholson and Cooper 1977; Shemesh et al. 1988; Terziev et al. 1974). Adverse systemic effects (respiratory, cardiovascular, gastrointestinal, hematological, hepatic, renal, and metabolic) reported in most cases of acute exposure to high amounts of endosulfan are likely to be secondary to the serious neurological effects (i.e., tremors, seizures) and to aspiration of the pesticide and its solvent (the latter can be highly damaging to the lung). The same occurs in animals (Boyd et al. 1970; Den Tonkelaar and Van Esch 1974; FMC 1958, 1972; Gupta and Chandra 1975; Gupta and Gupta 1977a; Hoechst 1970, 1983a, 1989b; Misra et al. 1980; Siddiqui et al. 1987b; Terziev et al. 1974).
The data in animals are insufficient to derive an acute inhalation MRL because serious effects were observed at the lowest dose tested (Hoechst 1983a). An acute-duration oral MRL was derived for endosulfan based on adverse neurological signs in rabbits administered the compound by gavage (MacKenzie et al. 1981). Additional acute studies do not seem necessary at this time.
Intermediate-Duration Exposure. No information is available on the toxicity of endosulfan to humans following intermediate-duration exposure by the oral route. Only very limited information is available regarding intermediate-duration occupational exposure (Aleksandrowicz 1979). Information is available regarding the effects of intermediate-duration exposure in animals following inhalation, oral, and dermal exposure (Alva et al. 2012; Banerjee and Hussain 1986, 1987; Choudhary et al. 2003; Dikshith et al. 1984, 1988; Garg et al. 1980; Gilmore et al. 2006; Gupta and Chandra 1977; Gupta and Gupta 1977a; Hatipoglu et al. 2008; Hiremath and Kaliwal 2003; Hoechst 1982, 1983b, 1984a, 1984b, 1984c, 1985a, 1985b, 1985c, 1985d, 1987, 1989c; Kalender et al. 2004a, 2004b; Kumar et al. 2011; Mahran 2013; Ozmen and Mor 2012; Ozmen et al. 2010; Sheets et al. 2004; Singh et al. 2007a, 2007b; Vos et al. 1982). These studies reported adverse effects on the liver, kidney, reproductive (testes), immune systems and nervous system as well as adverse reproductive and developmental effects. The data in animals were not sufficient to derive an intermediate-duration inhalation MRL, but were sufficient to derive an intermediate-duration oral MRL. An intermediate-duration oral MRL of 0.005 mg/kg/day, based on altered immunocompetence in rats, was derived for endosulfan (Banerjee and Hussain 1986). It is worth mentioning that the results of several studies suggest that at comparable doses, endosulfan seems to be more toxic administered by gavage than through the diet. Studies addressing this issue seem necessary since the diet is the most relevant route of exposure for the general population; therefore, risk assessment values based on gavage studies may not be entirely appropriate.
A recent intermediate-duration dietary developmental study in mice reported significant maternal liver and kidney histopathology at a dose level of approximately 0.006 mg endosulfan/kg/day (Mansour et al. 2014). However, other dietary studies in mice administered endosulfan in doses 2 or 3 orders of magnitude higher did not observe histopathological alterations in the liver of mice (Hack et al. 1995; Hoechst 1984b; NCI 1978). It would be helpful to try to replicate the Mansour et al. (2014) study.
Chronic-Duration Exposure and Cancer. No information is available on health effects in humans chronically exposed solely to endosulfan by any route. There are no available data regarding chronic-duration inhalation or dermal exposure in experimental animals. Information is, however, available regarding the effects of chronic-duration exposure in animals following oral exposure (FMC 1959b, 1967; Hack et al. 1995; Hoechst 1984b, 1988b, 1989a, 1989c; NCI 1978). The targets of toxicity in animals following chronic oral exposure appear to be the kidney. A chronic-duration oral MRL was not derived based on these studies because the potential point of departure from the best study available (Hoechst 1989a) yielded a chronic-duration oral MRL slightly higher than the intermediate-duration oral MRL. For this reason, the intermediate-duration oral MRL was also adopted for the chronic-duration oral MRL for endosulfan. Since no data are available for the inhalation route of exposure, a chronic inhalation MRL could not be derived. However, a chronic-duration dermal study might be useful to identify more accurately the end points of toxicity and the concentrations at which these effects are observed. Although there are several chronic-duration oral studies with endosulfan in rats, mice, and dogs, there are issues, particularly in the study in dogs conducted by Hoechst (1989c), that would be helpful to clarify. In that study, serum alkaline phosphatase activity was significantly increased relative to controls in males and females dosed with ≥0.6–0.7 mg/kg/day technical endosulfan; the NOAEL was approximately 0.2 mg/kg/day. In the absence of significant alterations in serum transaminases and liver histopathology, the investigators did not consider the changes in alkaline phosphatase activity toxicologically significant.
Very limited information is available regarding exposure to endosulfan and cancer. An occupational study found no association between exposure to endosulfan and breast cancer (Aschengrau et al. 1998). A study of the general population provided suggestive evidence of an association between levels of endosulfan in the bone marrow from children and hematological malignancies (Rau et al. 2012). A very small number of cases in the former study and lack of control of confounders in the latter study preclude drawing definitive conclusions. No studies or reports of cancer in humans associated with exposure to endosulfan by any route have been found. The carcinogenicity of endosulfan has been studied in chronic oral bioassays using rats (FMC 1959b; Hack et al. 1995; Hoechst 1989a; NCI 1978) and mice (Hack et al. 1995; Hoechst 1988b; NCI 1968, 1978). The available data in experimental animals were negative or inconclusive. The limited information available on the toxic effects of dermally administered endosulfan suggests that this chemical behaves similarly across both the oral and dermal routes of exposure. However, a study assessing the neoplastic potential of chronic-duration dermal exposure to endosulfan might be valuable since skin contact with contaminated soil is an important route of exposure for people living near hazardous waste sites.
Genotoxicity. No reliable data on humans exist to indicate whether endosulfan may act by a genotoxic mechanism. A study of mother-infant pairs from an agricultural community in Mexico reported no correlation between frequency of micronucleus or DNA damage and levels of organochlorine pesticides (endosulfan among them) in maternal blood or cord blood (Alvarado-Hernandez et al. 2013). The results from available in vivo animal studies and in vitro studies are mixed, but generally provide evidence that this compound can be mutagenic, clastogenic, and can induce effects on cell cycle kinetics in two different mammalian species (Ahmed et al. 2011; Antherieu et al. 2007; Bajpayee et al. 2006; Dikshith and Datta 1978; Dikshith et al. 1978; Dorough et al. 1978; Dubois et al. 1996; Dzwonkowska and Hubner 1986; Hoechst 1984d, 1988d; Jamil et al. 2004; Kurinnyi et al. 1982; Lu et al. 2000; L’Vova 1984; Manjula et al. 2006; McGregor et al. 1988; Moriya et al. 1983; Pednekar et al. 1987; Sobti et al. 1983; Usha Rani and Reddy 1986; Usha Rani et al. 1980; Velazquez et al. 1984; Yadav et al. 1982; Yaduvanshi et al. 2012). Some positive results may be suspect, however, because some endosulfan formulations contained epichlorohydrin, a known genotoxic chemical, as a stabilizer. Thus, additional testing verifying the positive results reported by Dikshith and Datta (1978), Dikshith et al. (1978), Dzwonkowska and Hubner (1986), Kurinnyi et al. (1982), L’Vova (1984), Usha Rani and Reddy (1986), Usha Rani et al. (1980), and Velazquez et al. (1984) would be valuable. Also, should populations that have been exposed exclusively to endosulfan be identified, in vivo tests of chromosomal aberrations in would provide valuable information on the genotoxic potential of endosulfan in humans.
Reproductive Toxicity. Very limited information is available regarding reproductive effects in humans exposed to endosulfan. A study reported no significant association between levels of endosulfan in adipose tissue from men and fertility (Çok et al. 2010). Since the cohort consisted of only 25 infertile men and 21 controls, definitive conclusions could not be drawn from the study. A study of a Brazilian population environmentally exposed to endosulfan and other organochlorine pesticides reported associations between altered serum level of LH and FSH in peri- and postmenopausal women and exposure to endosulfan (Freire et al. 2014).
Studies have reported that oral endosulfan had no effect on reproductive performance in rats (Dikshith et al. 1984; Hoechst 1982, 1984a). Adverse effects on the testes were observed in male rats that ingested endosulfan, but no assessment of reproductive performance was made (Gupta and Gupta 1977a; NCI 1978; Wang et al. 2012, 2014). Additional studies that looked at possible effects of endosulfan on spermatogenesis found reduced sperm counts and sperm abnormalities in rats and mice in intermediate-duration studies (Aly and Khafagy 2014; Ata et al. 2007; Bharath et al. 2011; Choudhary and Joshi 2003; Sinha et al. 1995, 1997). Acute- and intermediate-duration oral studies also reported reduced serum testosterone in male rats (Aly and Khafagy 2014; Kumar et al. 2011), in male mice (Wang et al. 2014), and in male rabbits (Ozmen and Mor 2012). With the exception the finding of testicular atrophy observed in rats treated with relatively high doses of endosulfan in the diet for up to 82 weeks (NCI 1978), other chronic-duration studies did not report morphological alterations in the reproductive organs of rats, mice, or dogs (Hoechst 1988b, 1989a, 1989c). No information is available on effects on reproductive performance of inhaled or dermally administered endosulfan, but no such studies seem necessary at this time. The fact that studies in rats indicate that endosulfan has no adverse effects on reproductive performance in animals following oral exposure is not totally unexpected since rats produce and ejaculate 10 times more sperm than are necessary for normal fertility and litter size (Amann 1982; Working 1988).
Developmental Toxicity. A limited number of studies in humans have provided suggestive evidence of associations between maternal exposure to endosulfan and developmental alterations in the offspring including autism spectrum disorders (Roberts et al. 2007), alterations in thyroid function (Freire et al. 2011, 2012), neural tube defects (Ren et al. 2011), and delayed sexual maturity in male children (Saiyed et al. 2003). After considering the strengths and limitations of the individual studies, no definite conclusions could be drawn. A conclusion shared by these studies was that further research is necessary and that the cohorts should be followed up. A study of Chinese women environmentally exposed to organochlorine pesticides (endosulfan among them) did not find an association between infant birth weight and maternal or cord levels of endosulfan (Guo et al. 2014).
No conclusive evidence of developmental toxicity in animals has been presented, mainly because of the questionable quality of the studies available and/or observations of developmental toxicity at maternally toxic doses of endosulfan, or because the studies provided no information regarding maternal effects (Cabaleiro et al. 2008; Caride et al. 2010; FMC 1980; Gupta et al. 1978; Hoechst 1982; Singh et al. 2007a, 2008). Further testing would be helpful to verify the effects that have been observed and to delineate clearly the doses at which these effects may be expected to occur. Any further testing should be by the oral route of exposure because it is the most relevant for humans living in the vicinity of hazardous waste sites, adverse developmental effects have been observed following oral exposure, and the limited information available on the toxic effects of dermally administered endosulfan suggests that this chemical behaves similarly across both routes of exposure. A developmental study in which endosulfan was administered via the diet to rats provided little evidence of developmental toxicity at doses considerably higher than those used in developmental gavage studies (Gilmore et al. 2006). As mentioned before, this issue needs to be addressed with studies of comparative toxicokinetics of gavage and dietary administration of endosulfan. Three recent studies reported neurochemical alterations in the brain from young adult mice and rats born to dams exposed to endosulfan during gestation and lactation (Lafuente and Pereiro 2013; Wilson et al. 2014a, 2104b). Similar studies should be conducted that also conduct behavioral tests to try to determine possible neurochemical bases for specific behaviors. As mentioned above under Intermediate-Duration Exposure, it would be helpful to try to replicate the developmental study of Mansour et al. (2014) that reported maternal and developmental effects (liver and kidney histopathology) in mice at doses considerably lower than in other studies.
Immunotoxicity. There is virtually no information regarding the effects of endosulfan on the human immune system. However, specially designed studies using rats indicate that both humoral and cellular immune responses can be depressed by ingested endosulfan at doses that do not induce any overt signs of toxicity (Banerjee and Hussain 1986, 1987). In vitro studies support the possibility that endosulfan affects immune system function (Das et al. 1988). These results demonstrate that immunotoxicity may be a more sensitive end point of endosulfan-induced toxicity than other end points, which has been the case also for other chemicals (Abadin et al. 2007). Since it is the only study that conducted immunocompetence experiments in rats challenged with a toxin, it would be useful to try to replicate the findings of Banerjee and Hussain (1986). An intermediate-duration oral MRL was derived based on the observation of depressed immune responses (Banerjee and Hussain 1986). Since the limited information available on the effects of dermally administered endosulfan suggests that this chemical behaves similarly across both routes of exposure and that adverse effects on immune function have also been observed in vitro, there is no reason to suspect that the immunotoxic effects observed following oral exposure are route-specific. Tests of immunologic function in exposed human populations would provide information as to whether immunosuppression also occurs in humans or whether this effect may be species-specific. Very limited information suggests that endosulfan can affect macrophage function (Ayub et al. 2003; Han et al. 2007), but further studies investigating the mechanism of endosulfan-induced immunotoxicity are needed.
Neurotoxicity. Information indicates that the central nervous system is the major target of endosulfan-induced toxicity in humans and animals following acute exposure by any route (Aleksandrowicz 1979; Blanco-Coronado et al. 1992; Boereboom et al. 1998; Boyd and Dobos 1969; Boyd et al. 1970; Bury 1997; Cable and Doherty 1999; Chugh et al. 1998; Ely et al. 1967; Eyer et al. 2004; FMC 1958, 1980; Gilbert and Mack 1995; Gupta and Chandra 1975; Hoechst 1970, 1975, 1983a, 1984e, 1989b; Karatas et al. 2006; Lakshmana and Raju 1994; Lo et al. 1995; MacKenzie et al. 1981; Moon and Chun 2009; Moses and Peter 2010; Nicholson and Cooper 1977; Parbhu et al. 2009; Pradhan et al. 1997; Roberts et al. 2004; Shemesh et al. 1988; Terziev et al. 1974). The most prominent signs of acute exposure to endosulfan in humans (oral and occupational) and animals (by all routes) are hyperactivity, tremors, decreased respiration, dyspnea, salivation, and tonic-clonic convulsions, which can lead to death. Neurotoxic effects are not always seen following intermediate- or chronic-duration exposures; however, chronic effects were observed in dogs (Hoechst 1989c). Two reports of humans acutely exposed to high amounts of endosulfan indicated that persistent cognitive brain damage may result following such exposures (Aleksandrowicz 1979; Shemesh et al. 1988). This could be examined in studies in animals under controlled exposures. Some gavage studies in animals have shown changes in neurotransmitter levels and alterations in neurobehavioral processes after exposure to endosulfan (Lakshmana and Raju 1994; Paul et al. 1995). However, dietary studies that provided considerably higher doses of endosulfan found little or no effects on neurobehavioral tests (Gilmore et al. 2006; Sheets et al. 2004), indicating that the mode of administration of the test material plays a role in the effects observed. As indicated before, this issue should be examined with appropriate toxicokinetic studies. Since the limited information available on the effects of dermally administered endosulfan suggests that this chemical behaves similarly across both routes of exposure, and neurotoxicity has been observed following inhalation exposure as well, there is no reason to suspect that the neurological effects observed following oral exposure are route-specific. Further studies investigating the mechanism for endosulfan-induced neurotoxicity would be helpful since this information might help identify special populations at risk for such effects. Furthermore, although neurotoxic effects have not generally been observed in chronic-duration animal studies, sensitive neurological functional end points (e.g., various reflexes, grip strength, sensory function, motor activity, or nerve conduction velocity), extensive histologic neuropathological evaluations of brain, spinal cord, and peripheral nerves, or evaluations of higher functions such as learning and memory have not been done for long-term exposures to endosulfan. This information would be useful to assess the potential for this chemical to cause permanent neurological damage.
Epidemiological and Human Dosimetry Studies. Most of the literature reviewed concerning the health effects of endosulfan in humans described case reports of occupational exposure or accidental or intentional ingestion of endosulfan (Aleksandrowicz 1979; Aschengrau et al. 1998; Bernardelli and Gennari 1987; Blanco-Coronado et al. 1992; Boereboom et al. 1998; Cable and Doherty 1999; Chugh et al. 1998; Demeter and Heyndrickx 1978; Ely et al. 1967; Eyer et al. 2004; Karatas et al. 2006; Lo et al. 1995; Moon and Chun 2009; Moses and Peter 2010; Parbhu et al. 2009; Roberts et al. 2004; Shemesh et al. 1988; Terziev et al. 1974; Yavuz et al. 2007). The predominant routes of exposure in the occupational studies are believed to be inhalation and dermal (workers involved in pesticide manufacture, formulation, and application). There are studies of the general population that examined possible associations between exposure to endosulfan (and additional compounds in some cases) and health outcomes such as cancer (Rau et al. 2012), fertility in men (Çok et al. 2010), sex hormones and thyroid hormones (Freire et al. 2013, 2014), and developmental effects (Freire et al. 2011; Garcia-Rodriguez et al. 1996; Ren et al. 2011; Roberts et al. 2007; Saiyed et al. 2003). The information on human exposure is limited because of the possibility of concurrent exposure to other pesticides or other toxic substances, and the duration and level of exposure to endosulfan generally cannot be quantified from the information presented in these reports. The most likely identifiable subpopulation exposed to endosulfan is pesticide applicators or individuals involved in the production and formulation of endosulfan. Well-designed epidemiological studies of these exposed workers may be useful, specifically examining the effects of endosulfan on the kidney, since this organ appeared to be a sensitive target in long-term studies in rats (Hoechst 1989a). However, the decision to conduct such a study needs to be balanced by the fact that the risk of exposure will diminish since endosulfan is scheduled to be cancelled for all uses by 2016 (EPA 2002, 2012e). The immune system was a sensitive target for endosulfan in an intermediate-duration study in rats (Banerjee and Hussain 1986). If the results of the Banerjee and Hussain (1986) study can be reproduced, immune parameters of populations with known exposure to endosulfan should also be examined. Exposure to endosulfan by the subjects of epidemiological studies should be confirmed by analysis of blood and urine for endosulfan isomers and endosulfan sulfate. If endosulfan is found to be associated with adverse effects in any organ or system, then tests of these specific organs or systems may be useful tools to monitor endosulfan exposure in individuals living near hazardous waste sites.
Biomarkers of Exposure and Effect
Exposure. Endosulfan and metabolites have been detected in the urine from workers exposed to this substance (Arrebola et al. 1999; Cable and Doherty 1999; Vidal et al. 1998). Endosulfan and metabolites have also been detected in the placenta (Cerrillo et al. 2005; Freire et al. 2011), cord blood (Alvarado-Hernandez et al. 2013; Mariscal-Arcas et al. 2010), fat tissue (Olea et al. 1999), and breast milk from the general population (Cerrillo et al. 2005; Çok e t al. 2011; Lutter et al. 1998). Endosulfan has also been detected in blood from subjects who accidentally or intentionally ingested high amounts of the pesticide (Blanco-Coronado et al. 1992; Boereboom et al. 1998; Eyer et al. 2004; Yavuz et al. 2007). The presence of the parent compound and its metabolites are specific biomarkers for endosulfan exposure. Only in the acute, high-exposure cases there have been attempts to quantitate exposure. Estimating exposure in cases of prolonged low-level exposure, as it may occur for the general population, would require reliable exposure characterization and a better knowledge of endosulfan toxicokinetics in humans.
Effect. There are no biomarkers of effect specific for endosulfan in humans or in animals. The typical effect of exposure to high amounts of endosulfan is overactivity of the nervous system such as hyperexcitability, tremors, and seizures, but exposure to numerous other chemicals results in the same type of effects. There are no studies of populations with prolonged exposure to low levels of endosulfan, so no adverse health effects have been reported.
Absorption, Distribution, Metabolism, and Excretion. Indirect evidence describing the occurrence of toxic effects following exposure to endosulfan by all three routes (inhalation, oral, and dermal) indicates that this compound is absorbed by both humans and animals (Bernardelli and Gennari 1987; Boereboom et al. 1998; Blanco-Coronado et al. 1992; Cable and Doherty 1999; Chan et al. 2005; Chugh et al. 1998; Deema et al. 1966; Demeter and Heyndrickx 1978; Dorough et al. 1978; Ely et al. 1967; Eyer et al. 2004; Gorbach et al. 1968; Gupta and Gupta 1979; Karatas et al. 2006; Moon and Chun 2009; Nath and Dikshith 1979; Nicholson and Cooper 1977; Parbhu et al. 2009; WHO 1984). No information is available to assess the relative rates and extent of endosulfan absorption following inhalation or oral exposure in humans or animals or dermal exposure in humans. Limited data are available that assess the relative rates and extent of endosulfan absorption following dermal exposure in animals (Hoechst 1986). The data indicate that endosulfan binds to the skin of rats and is only slowly absorbed in the body, with absorption rates decreasing with time. Only about 25% of the bound material was absorbed into the body by 24 hours (Hoechst 1986). Quantitative information that describes the rate and extent of endosulfan absorption following inhalation, oral, and dermal exposure in humans and/or animals would be useful to assess more fully the hazard presented by exposure to endosulfan at various levels from these different routes. Studies in animals have shown that at comparable doses, endosulfan is more toxic when administered by gavage than via the diet. Of particular interest would be studies that compare the rate of absorption between these two modes of administration.
Limited information from case reports is available regarding the distribution of endosulfan in humans following oral exposure (Boereboom et al. 1998; Coutselinis et al. 1978; Demeter and Heyndrickx 1978; Demeter et al. 1977). Isomers and metabolites of endosulfan were detected in the fat of 30–40% of children hospitalized in agricultural regions of Spain, demonstrating that endosulfan accumulates in adipose tissue of children after presumably repeated dietary exposure (Olea et al. 1999). Endosulfan and metabolites have also been detected in placenta (Cerrillo et al. 2005; Freire et al. 2011), cord blood (Mariscal-Arcas et al. 2010), fat tissue (Olea et al. 1999), and breast milk from the general population (Cerrillo et al. 2005; Çok et al. 2011; Lutter et al. 1998). Animal studies describe the distribution of endosulfan following both short-term and long-term oral exposure (Ansari et al. 1984; Braun and Lobb 1976; Chan et al. 2005; Chan and Mohd 2005; Dikshith et al. 1984; Gupta 1978; Hoechst 1987; Nath and Dikshith 1979). The available evidence indicates that endosulfan tends to distribute initially to the fatty tissues but accumulates in the kidney with prolonged exposure. Studies that compare the concentration of endosulfan and/or metabolites in blood in gavage and dietary studies could provide an explanation for the seemingly different toxic potency of endosulfan between the two modes of administration. No quantitative information is available on the distribution of endosulfan following inhalation exposure, and three studies are available that describe the distribution of endosulfan in animals after dermal exposure (Dikshith et al. 1984; Hoechst 1986; Nicholson and Cooper 1977). Although limited data are available on the rate and extent of endosulfan distribution, the available information indicates the kidney appears to be the organ with the greatest tissue accumulations following both short- and long-term exposure. However, more information on the distribution of endosulfan in humans and animals following exposure to all three routes would be useful in ascertaining whether there are differences across routes of exposure with respect to distribution.
No information is available regarding the metabolism of endosulfan in humans. However, the metabolic pathway of this chemical has been well characterized in several species of experimental animals (Deema et al. 1966; Dorough et al. 1978; Gorbach et al. 1968; WHO 1984). The data indicate that metabolism of endosulfan occurs in both the liver and kidney (Agarwal et al. 1978; Deema et al. 1966; Hoechst 1987; Siddiqui et al. 1987a; Tyagi et al. 1984). Limited data from an acute dermal study showing a dose-related decrease in excretion with increasing dose indicate that the metabolism of endosulfan is saturable (Hoechst 1986).
Information is available regarding excretion of endosulfan and metabolites in humans. Blanco-Coronado et al. (1992) measured total endosulfan in the urine of poisoned individuals shortly after poisoning occurred. However, it could not be ascertained whether the urine was a major or minor excretion route. α-Endosulfan, β-endosulfan, and/or metabolites were present in the urine of humans after intentional oral exposure (Boereboom et al. 1998) and after occupational exposure either with (Arrebola et al. 1999) or without (Vidal et al. 1998) protective clothing.
No information was located regarding excretion of endosulfan residues in animals following inhalation exposure. Limited data were located regarding excretion in animals following dermal exposure (Hoechst 1986). The routes and extent of endosulfan excretion following oral exposure in animals have been characterized (Chan et al. 2005; Deema et al. 1966; Dorough et al. 1978; Gorbach et al. 1968). More data are needed regarding the characterization of the metabolites and the extent of endosulfan excretion following inhalation and dermal exposure in both humans and animals.
Practically all toxicokinetic properties reported were based on results from acute exposure studies. Generally, no information was available regarding intermediate or chronic exposure to endosulfan. Since endosulfan is an enzyme inducer (Siddiqui et al. 1987a; Tyagi et al. 1984), the kinetics of metabolism during chronic exposure probably differ from those seen during acute exposure. Similarly, excretion kinetics may differ with time and dose. Thus, additional studies on the metabolism and excretion of endosulfan during intermediate or chronic exposure would be useful to assess the potential for toxicity following longer-duration exposures. A PBPK model was developed for endosulfan that describes toxicokinetics in rats (Chan et al. 2006). The model simulated gavage dosing of rats. It would be useful to incorporate into the model parameters related to dietary administration of endosulfan since studies have shown that endosulfan is considerably less toxic administered in the diet than by gavage. Dietary intake is the most relevant route of exposure to endosulfan by the general population.
Comparative Toxicokinetics. Most of the reliable data available on the toxicity of endosulfan in humans are from acute exposures where neurotoxicity is the end point of concern, but toxicokinetics have not been studied (Aleksandrowicz 1979; Ely et al. 1967; Moon and Chun 2009; Moses and Peter 2010; Roberts et al. 2004; Shemesh et al. 1988; Terziev et al. 1974). The same spectrum of effects is seen in animals after acute exposure (Boyd and Dobos 1969; Boyd et al. 1970; FMC 1958; Gilbert and Mack 1995; Gupta and Chandra 1975; Hoechst 1970, 1975, 1983a, 1984e, 1989b; Nicholson and Cooper 1977; Terziev et al. 1974). Other systemic effects observed in humans and in animals are likely to be secondary to the adverse neurological effects. Although no toxicokinetic studies have been performed in humans, there is information on some toxicokinetic aspects of endosulfan in several species of experimental animals (rats, mice, rabbits, and sheep), and there appears to be little difference between the species (Ansari et al. 1984; Braun and Lobb 1976; Dikshith et al. 1984; Deema et al. 1966; Dorough et al. 1978; Gorbach et al. 1968; Gupta 1978; Nath and Dikshith 1979; Nicholson and Cooper 1977; WHO 1984). However, substantial differences exist in the doses required to produce toxicity in male and female rats in acute- (Hoechst 1985c, 1985d, 1990) and intermediate-duration studies (Paul et al. 1995). Differences in the rates of excretion were proposed to account for the differences in sensitivity of male and female rats (Dikshith et al. 1984), but excretion was not directly studied by these authors. Therefore, further studies evaluating the reason for this difference may provide valuable information for estimating acutely toxic doses in humans.
Methods for Reducing Toxic Effects. As stated in Section 3.11.1, Reducing Peak Absorption Following Exposure, there is information on the procedures that may be used to limit absorption of endosulfan following ingestion (HSDB 2010). Identification of improved procedures to reduce absorption is always valuable. A number of anticonvulsants and sedatives have been used to manage the seizures induced by exposure to high amounts of endosulfan. These include phenytoin, phenobarbital, valproate, propofol, thiopentane, midazolam, diazepam, lorazepam, and clobazam (Moses and Peter 2010). These drugs have been used in different combinations and sequences as the specific situations required. The effectiveness of these drugs varied from case to case and probably depended on the time elapsed between poisoning and initiation of treatment and on the amount of endosulfan taken. Publishing treatments that have proved to be effective in randomized controlled trials in medical journals could help decrease the number of fatalities resulting from endosulfan poisoning, particularly in countries where it is still widely used.
Children’s Susceptibility. Data needs relating to both prenatal and childhood exposures, and developmental effects expressed either prenatally or during childhood, are discussed in detail in the Developmental Toxicity subsection above.
The information on health effects of endosulfan in humans is derived mainly from cases of accidental or intentional exposure of adults to high amounts of the pesticide, and the main adverse effect is neurotoxicity. Cases of children accidentally exposed to high amounts of endosulfan showed the same types of effects observed in adults (Jindal and Sankhyan 2012; Kamate and Jain 2011; Parbhu et al. 2009). However, based on these high-exposure cases in which quantitative exposure information was not available, it is difficult to determine whether children are more susceptible to endosulfan than adults. Some studies in animals have provided evidence that young animals respond to endosulfan differently than adult animals (Kiran and Varma 1988; Lakshmana and Raju 1994; Sinha et al. 1995, 1997; Wilson et al. 2014b; Zaidi et al. 1985), but there is no conclusive evidence to suggest that young animals are more susceptible than older ones. A study also showed that perinatal exposure to endosulfan may increase the susceptibility of the offspring to other neurotoxins (Wilson et al. 2014b). Further studies that evaluate a number of different end points in young as well as older organisms would provide valuable information.
A few studies in humans provided suggestive evidence of associations between maternal exposure to endosulfan and developmental alterations in the offspring including autism spectrum disorders (Roberts et al. 2007), alterations in thyroid function (Freire et al. 2011, 2012), neural tube defects (Ren et al. 2011), and delayed sexual maturity in male children (Saiyed et al. 2003). After considering the strengths and limitations of the individual studies, no definite conclusions could be drawn. Studies in animals have reported adverse developmental effects (Cabaleiro et al. 2008; Caride et al. 2010; Gilmore et al. 2006; Singh et al. 2007a, 2008; Sinha et al. 2001), but since not all studies provided information regarding maternal effects, it not totally clear whether developmental effects occur in the absence of maternal toxicity. Perinatal administration of endosulfan to rats by gavage resulted in more severe developmental effects than administration of comparable dose via the diet (Cabaleiro et al. 2008; Gilmore et al. 2006). As indicated earlier, this general issue should be examined with appropriate toxicokinetic studies.
No data were located concerning whether pharmacokinetics of endosulfan in children are different from adults. Endosulfan has been detected in human placenta and cord blood (Cerrillo et al. 2005; Freire et al. 2011; Mariscal-Arcas et al. 2010), so it is reasonable to assume that it can reach the fetus. Although endosulfan has been detected in human milk (Cerrillo et al. 2005; Çok et al. 2011; Lutter et al. 1998), some studies in animals showed very little accumulation of endosulfan residues in breast milk (Gorbach et al. 1968; Indraningsih et al. 1993), which is consistent with the rapid elimination of endosulfan from tissues and subsequent excretion via feces and urine. Cross-foster studies in animals could provide information regarding differential transfer of endosulfan and/or metabolites through the placenta and the mother’s milk. There are no PBPK models for endosulfan in either adults or children. There is no information to evaluate whether absorption, distribution, metabolism, or excretion of endosulfan in children is different than in adults.
There are no biomarkers of exposure or effect that have been validated in children. There are no data on interactions of endosulfan with other chemicals in children, and the existing data in adults are inadequate to determine whether the same effects will be observed in children. There are no pediatric-specific methods to reduce peak absorption for endosulfan following exposure, to reduce body burden, or to interfere with the endosulfan’s mechanism of action.
Child health data needs relating to exposure are discussed in Section 6.8.1, Identification of Data Needs: Exposures of Children.
3.12.3. Ongoing Studies
The following ongoing research was identified in the National Institute of Health (NIH) RePORTER (2015) database. Dr. C.S. Watson from the Department of Biochemistry, School of Medicine at the University of Texas Medical Branch in Galveston, Texas, is studying the mechanism by which environmentally relevant chemicals, endosulfan among them, affect through nongenomic pathways the functions initiated by estradiol at the plasma membrane. The research is sponsored by the National Institute of Environmental Health Sciences.
- INTRODUCTION
- DISCUSSION OF HEALTH EFFECTS BY ROUTE OF EXPOSURE
- GENOTOXICITY
- TOXICOKINETICS
- MECHANISMS OF ACTION
- TOXICITIES MEDIATED THROUGH THE NEUROENDOCRINE AXIS
- CHILDREN’S SUSCEPTIBILITY
- BIOMARKERS OF EXPOSURE AND EFFECT
- INTERACTIONS WITH OTHER CHEMICALS
- POPULATIONS THAT ARE UNUSUALLY SUSCEPTIBLE
- METHODS FOR REDUCING TOXIC EFFECTS
- ADEQUACY OF THE DATABASE
- HEALTH EFFECTS - Toxicological Profile for EndosulfanHEALTH EFFECTS - Toxicological Profile for Endosulfan
Your browsing activity is empty.
Activity recording is turned off.
See more...