NCBI Bookshelf. A service of the National Library of Medicine, National Institutes of Health.
Toxicological Profile for Boron. Atlanta (GA): Agency for Toxic Substances and Disease Registry (US); 2010 Nov.
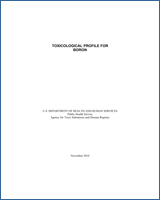
Toxicological Profile for Boron.
Show details3.1. INTRODUCTION
The primary purpose of this chapter is to provide public health officials, physicians, toxicologists, and other interested individuals and groups with an overall perspective on the toxicology of boron. It contains descriptions and evaluations of toxicological studies and epidemiological investigations and provides conclusions, where possible, on the relevance of toxicity and toxicokinetic data to public health.
A glossary and list of acronyms, abbreviations, and symbols can be found at the end of this profile.
3.2. DISCUSSION OF HEALTH EFFECTS BY ROUTE OF EXPOSURE
To help public health professionals and others address the needs of persons living or working near hazardous waste sites, the information in this section is organized first by route of exposure (inhalation, oral, and dermal) and then by health effect (death, systemic, immunological, neurological, reproductive, developmental, genotoxic, and carcinogenic effects). These data are discussed in terms of three exposure periods: acute (14 days or less), intermediate (15–364 days), and chronic (365 days or more).
Levels of significant exposure for each route and duration are presented in tables and illustrated in figures. The points in the figures showing no-observed-adverse-effect levels (NOAELs) or lowest-observed-adverse-effect levels (LOAELs) reflect the actual doses (levels of exposure) used in the studies. LOAELs have been classified into “less serious” or “serious” effects. “Serious” effects are those that evoke failure in a biological system and can lead to morbidity or mortality (e.g., acute respiratory distress or death). “Less serious” effects are those that are not expected to cause significant dysfunction or death, or those whose significance to the organism is not entirely clear. ATSDR acknowledges that a considerable amount of judgment may be required in establishing whether an end point should be classified as a NOAEL, “less serious” LOAEL, or “serious” LOAEL, and that in some cases, there will be insufficient data to decide whether the effect is indicative of significant dysfunction. However, the Agency has established guidelines and policies that are used to classify these end points. ATSDR believes that there is sufficient merit in this approach to warrant an attempt at distinguishing between “less serious” and “serious” effects. The distinction between “less serious” effects and “serious” effects is considered to be important because it helps the users of the profiles to identify levels of exposure at which major health effects start to appear. LOAELs or NOAELs should also help in determining whether or not the effects vary with dose and/or duration, and place into perspective the possible significance of these effects to human health.
The significance of the exposure levels shown in the Levels of Significant Exposure (LSE) tables and figures may differ depending on the user’s perspective. Public health officials and others concerned with appropriate actions to take at hazardous waste sites may want information on levels of exposure associated with more subtle effects in humans or animals (LOAELs) or exposure levels below which no adverse effects (NOAELs) have been observed. Estimates of levels posing minimal risk to humans (Minimal Risk Levels or MRLs) may be of interest to health professionals and citizens alike.
A User’s Guide has been provided at the end of this profile (see Appendix B). This guide should aid in the interpretation of the tables and figures for Levels of Significant Exposure and the MRLs.
3.2.1. Inhalation Exposure
Most of the inhalation toxicity data for boron involves human or animal exposure to borate dusts such as boric acid, boron oxide, or various hydration states of sodium borate salts (anhydrous; pentahydrate; and decahydrate; also referred to as borax) or other borate salts (e.g., calcium borate). In aqueous media, boron oxide is rapidly transformed to boric acid and, depending on the pH of the media, to borate salts. Equivalent boron doses have been calculated throughout this assessment by multiplying reported doses by an adjustment factor relating the molar mass ratio of boron in a given compound to the total molar mass. Thus, the dose adjustment factors are 0.175 for boric acid, 0.113 for disodium borate decahydrate, 0.151 for disodium borate pentahydrate, 0.215 for anhydrous borate, and 0.155 for boron oxide. In addition, animal studies were located reporting the effects of inhaled diborane, a flammable boron-containing gas. Because of the differences in environmental prevalence, industrial uses, likelihood of exposure, and respiratory toxicity, the effects of diborane exposure are discussed separately from other boron compounds in Section 3.2.1.2, Respiratory Effects.
3.2.1.1. Death
No studies were located regarding death in humans after inhalation exposure to boron. The 4-hour LC50 for boric acid, borax, and disodium borates is >2 mg boron/m3 (Hubbard 1998). No fatalities were observed in rats exposed for 6 hours/day, 5 days/week, to 470 mg boron oxide/m3 (73 mg boron/m3) for 10 weeks, 175 mg boron oxide/m3 (27 mg boron/m3) for 12 weeks, or 77 mg boron oxide/m3 (12 mg boron/m3) for 24 weeks, or dogs exposed to 57 mg boron oxide/m3 (9 mg boron/m3) for 23 weeks (Wilding et al. 1959).
3.2.1.2. Systemic Effects
No studies were located regarding cardiovascular, gastrointestinal, hematological, musculoskeletal, or renal effects in humans after inhalation exposure to boron. No studies were located regarding dermal effects after acute inhalation exposure in humans or animals for any duration category, but eye irritation has been reported in sodium-borate mining and processing workers. Information on respiratory, cardiovascular, gastrointestinal, hematological, musculoskeletal, and renal effects in animals and respiratory effects and dermal/ocular effects in humans is discussed below. The highest NOAEL values and all reliable LOAEL values for these systemic effects for each species and duration category involving exposure to boric acid, borates, or boron oxide are recorded in Table 3-1 and plotted in Figure 3-1. Results from a few mouse inhalation studies of diborane gas indicate that it is a potent respiratory toxicant, much more potent than borates or boron oxide. Since it is not expected to be as important an environmental compound as borates or boron oxide, NOAEL and LOAEL values from these mouse studies of diborane gas are not included in Table 3-1 or Figure 3-1.
Respiratory Effects. Occupational studies of workers exposed to dusts of sodium borates, the most important commercial forms of boron, have identified irritation of the respiratory tract and eyes, without measurable changes in pulmonary function. In an early cross-sectional surveillance of 629 U.S. workers in a sodium borate open-pit mining and production plant, past occurrence of symptoms of respiratory irritation such as dryness of the mouth, nose, or throat, dry cough, nose bleeds, and sore throat were reported at elevated frequencies in workers in areas with mean dust concentrations of 8.4 and 14.6 mg particulates/m3 (1.8 and 3.1 mg boron/m3, respectively), compared with workers in areas with lower mean dust levels of 4.0 and 1.1 mg particulate/m3 (0.9 and 0.2 mg boron/m3) (Garabrant et al. 1984; 1985). These boron concentrations are upper bound estimates, assuming that all of the sampled dust is anhydrous borax. A reduction in forced expiratory volume in 1 second (FEV1) was measured in a subgroup of smoking workers with estimated high cumulative exposure (≥80 mg particulate/m3, ≥9 mg boron/m3) to sodium borate dusts, but not in groups of less-exposed smoking workers or in nonsmoking workers. However, a subsequent surveillance of FEV1 in 303 of the original 629 borax workers, 7 years after the original surveillance, , some of whom were exposed to ≥15 mg boron/m3, found no exposure-related changes in FEV1 over this period, when adjustments were made for the effects of age, height, and smoking on FEV1 (Wegman et al. 1991, 1994). Although the prevalence of workers reporting acute respiratory irritation symptoms increased with the mean dust concentrations for different job categories in the plant, the cross-sectional design of the Garabrant et al. (1985) study prevented the determination of whether the elevation of acute respiratory symptoms was a consequence of acute or repeated exposure to sodium borate dusts. Thus, the effect levels associated with the measured prevalence of acute respiratory symptoms in this study are not included in Table 3-1 or Figure 3-1.
Table 3-1
Levels of Significant Exposure to Boron - Inhalation.

Figure 3-1
Levels of Significant Exposure to Boron - Inhalation.
The occurrence of acute respiratory symptoms as a possible consequence of acute exposure to dusts of sodium borate (the decahydrate, pentahydrate, and anhydrous tetraborates) was studied in a later study by interviewing workers about acute irritation symptoms before the work shift began and at regular hourly intervals during the work shift, and by measuring personal air concentrations of particulates at concurrent intervals for 4 consecutive days (Hu et al. 1992; Wegman et al. 1991, 1994). Seventy-nine exposed production workers and 27 nonexposed workers were included in the study. In the latest analysis of the collected data, the incidence rates for irritation symptoms in exposed workers were statistically significantly higher than those in nonexposed workers, with exposed workers 9-, 5-, and 3-fold more likely to report incidents of nasal, eye, and throat irritation, respectively, than comparison workers (Hu et al. 1992; Wegman et al. 1991, 1994). For the unexposed groups, the arithmetic means of the 6-hour TWA daily dust concentration was 0.45 mg particulates/m3 (0.02 mg boron/m3), with 100% of samples ≤1.0 mg particulates/m3 and about 90% of samples ≤0.5 mg particulates/m3. The arithmetic mean of the 6-hour TWA daily dust concentrations for the exposed group was 5.72 mg particulates/m3 (0.44 mg boron/m3), with a majority of air concentrations between 1.0 and 10.0 mg particulates/m3 (Wegman et al. 1991, 1994). Several factors make it difficult to identify a NOAEL or LOAEL for this study, precluding its inclusion in Table and Figure 3-1. Study participants rated their perception of individual episodes of eye, throat, and nasal irritancy on a scale of 0 (not at all) to 10 (very, very much), with ratings of 1, 2, and 3 representing “very little”, “fairly little”, and “moderate” irritation, respectively. Unexposed responders to the pre-shift survey reported a mean rating of 1.9 for all symptoms, while the mean rating for nasal irritation (the most commonly reported effect) among exposed workers was just slightly higher at 2.2. Thus, the cutoff for boron dust-induced effects is likely to be for ratings of ≥3. In the exposed group, 91% of reported symptoms were rated with severity scores ≤3 and 96% of symptoms were rated with severity scores of ≤4 (“pretty much”). For incidences in which the workers depressed a counter button to record time of symptom onset, and the study surveyor recorded an occurrence of effect, the probability of reporting an effect did not change markedly until 0.25-hour TWA exposure levels reached 0.8–1.2 mg boron/m3 (Wegman et al. 1991). There was no significant difference in reporting of symptoms based on the type of borate dust, which differ in boron content by almost a factor of two (i.e., 4.7 mg anhydrous borax/m3 and 8.8 mg/m3 of the decahydrate provide 1 mg boron/m3). Finally, the data do not indicate a temporal increase in effect intensity, as would be expected for a local irritant. Further, since no chronic effects (i.e., reduced FEV1) were observed in workers assessed by Wegman et al. (1994), 7 years after being assessed by Garabrant et al. (1985), the acute-duration inhalation MRL of 0.3 mg/m3 should also be health-protective for intermediate- and chronic-duration exposures.
Acute-duration laboratory exposures of volunteers to boric acid and sodium borate (sodium borate pentahydrate) dust provide more precise estimates of effect levels than the occupational studies. Male and female volunteers were trained to recognize the difference in chemesthetic feel (pungency or irritancy) of various levels of CO2 offered to the eyes, nose, and throat (Cain et al. 2004, 2008). Exposures of ≥17.7% CO2 resulted in a “feel” described by the volunteers as irritating. Male volunteers exposed to ≤3.0 mg boron/m3 (≤20 mg sodium borate/m3) for 20 minutes reported increasingly higher magnitude of feel of the dust in eyes, nose, and throat. However, the mean reported level of feel, compared to equivalent CO2 levels, was not considered irritating by the study subjects. The mean reported perception of feel at ≥4.5 mg boron/m3 (≥30 sodium borate/m3) was reported to feel irritating to the nose (Cain et al. 2004). Significantly increased nasal secretions (by mass) occurred at 1.5 mg boron/m3 (10 mg sodium borate/m3), but not 0.8 mg boron/m3 (5 mg sodium borate/m3) (Cain et al. 2004). In a similar experiment, male and female volunteers exposed to 1.5 mg boron/m3 (10 mg sodium borate/m3) or 1.8 mg boron/m3 (10 mg boric acid/m3) for 47 minutes while exercising reported a mean sense of feel that initially increased and peaked at the equivalent of slightly less than 17.7% CO2. These boron exposures, with a mean reported feel equivalent to <17.7% CO2, were considered non-irritating by the study subjects, although increases in nasal secretions were observed at 1.8 mg boron/mg3 (10 mg boric acid/m3), but not at 0.9 mg boron/mg3 (5 mg boric acid/m3) (Cain et al. 2008). The volume of nasal secretions increased above controls for the first 20 minutes of exposure, but did not continue to increase thereafter, suggesting that increased secretions resulted from possible dust-induced changes in osmolarity rather than an irritation response. No dose-related changes in nasal airway resistance or breathing patterns were observed. Together, these studies were used to identify a minimal LOAEL of 1.5 mg boron/m3, with an associated NOAEL of 0.8 mg/m3, for significantly increased mass of nasal secretions, from which an acute-duration inhalation MRL of 0.3 mg boron/m3 was derived (see Appendix A).
Corroborating evidence in animals for the respiratory irritation potential of inhaled boron compounds is sparse. Mild respiratory irritation, indicated by a 20% reduction in breathing rate, occurred in mice inhaling 53 mg boron/m3 (300 mg boric acid/m3) for 3 hours (Krystofiak and Schaper 1996). Rats were exposed to aerosols of boron oxide (6 hours/day, 5 days/week) at concentrations of 470 mg boron oxide/m3 (73 mg boron/m3) for 10 weeks, 175 mg boron oxide/m3 (27 mg boron/m3) for 12 weeks, or 77 mg boron oxide/m3 (12 mg boron/m3) for 24 weeks, and dogs were exposed to 57 mg boron oxide/m3 (9 mg boron/m3) for 23 weeks (Wilding et al. 1959). In rats, histological examination of a comprehensive set of tissues revealed no differences between the tissues of exposed and control animals. Signs of gross toxicity were restricted to the appearance of a reddish exudate from the nose of some of the rats exposed to 470 mg boron oxide/m3.
Mice (ICR) exposed to 0.7 ppm diborane gas (0.2 mg boron/m3) for 2 or 4 weeks exhibited slight infiltration of polymorphous neutrophil in peribronchiolar regions of the respiratory tract (Nomiyama et al. 1995). Mice exposed to 5 ppm diborane gas (1.7 mg boron/m3) for 2 weeks exhibited increased lung weight, nasal cavity changes, diffuse panbrochiolitis-like lesions, cellular infiltration of the bronchioles and perivascular area, appearance of alveolar macrophages, perivascular lymphoid hyperplasia, lung congestion, bleeding, and edema (Uemura et al. 1995).
Comparison of the results from the studies of mice exposed to diborane gas (Nomiyama et al. 1995; Uemura et al. 1995) and those from studies of rats and dogs exposed to boron oxide (Wilding et al. 1959) indicate that diborane gas is much more potent as a respiratory toxicant than boron oxide. Diborane gas is expected to have a very short half-life in the environment because of its reactivity. Thus, it is not expected to be a significant environmental toxicant, except in workplaces where it might be used and accidentally released.
Cardiovascular Effects. Rats exposed to aerosols of boron oxide at a concentration of 470 mg boron oxide/m3 (73 mg boron/m3) for 10 weeks, 175 mg boron oxide/m3 (27 mg boron/m3) for 12 weeks, or 77 mg boron oxide/m3 (12 mg boron/m3) for 24 weeks showed no gross or microscopic effects in the cardiovascular system (Wilding et al. 1959).
Gastrointestinal Effects. No gross or microscopic changes were seen in the gastrointestinal tract of rats exposed to aerosols of boron oxide at a concentration of 470 mg boron oxide/m3 (73 mg boron/m3) for 10 weeks, 175 mg boron oxide/m3 (27 mg boron/m3) for 12 weeks, or 77 mg boron oxide/m3 (12 mg boron/m3) for 24 weeks (Wilding et al. 1959).
Hematological Effects. Rats exposed to aerosols of boron oxide at concentrations of 470 mg boron oxide/m3 (73 mg boron/m3) for 10 weeks, 175 mg boron oxide/m3 (27 mg boron/m3) for 12 weeks, or 77 mg boron oxide/m3 (12 mg boron/m3) for 24 weeks, and dogs exposed to aerosols of boron oxide at concentrations of 57 mg boron oxide/m3 (9 mg boron/m3) for 23 weeks showed no significant changes in total red and white blood cell count, hemoglobin, hematocrit, or differential count (Wilding et al. 1959).
Musculoskeletal Effects. No gross or microscopic effects of exposure were observed in the femur, rib, and muscle of rats exposed to concentrations of 470 mg boron oxide/m3 (73 mg boron/m3) for 10 weeks, 175 mg boron oxide/m3 (27 mg boron/m3) for 12 weeks, or 77 mg boron oxide/m3 (12 mg boron/m3) for 24 weeks (Wilding et al. 1959).
Hepatic Effects. No gross or microscopic hepatic effects were observed in rats exposed to aerosols of boron oxide at concentrations of 470 mg boron oxide/m3 (73 mg boron/m3) for 10 weeks, 175 mg boron oxide/m3 (27 mg boron/m3) for 12 weeks, or 77 mg boron oxide/m3 (12 mg boron/m3) for 24 weeks. No hepatic effects were indicated from serum chemistry of dogs exposed to aerosols of boron oxide at concentrations of 57 mg boron oxide/m3 (9 mg boron/m3) for 23 weeks (Wilding et al. 1959).
Renal Effects. No gross or microscopic renal effects were observed in rats exposed to aerosols of boron oxide at concentrations of 470 mg boron oxide/m3 (73 mg boron/m3) for 10 weeks, 175 mg boron oxide/m3 (27 mg boron/m3) for 12 weeks, or 77 mg boron oxide/m3 (12 mg boron/m3) for 24 weeks. No renal effects were indicated from serum chemistry of dogs exposed to aerosols of boron oxide at concentrations of 57 mg boron oxide/m3 (9 mg boron/m3) for 23 weeks (Wilding et al. 1959).
Ocular Effects. Human occupational exposure to a mean concentration of 0.44 mg boron/m3 (5.72 mg particulates/m3) (Hu et al. 1992; Wegman et al. 1994) and 1.8–3.1 mg boron/m3 (8.4–14.6 mg particulates/m3) (Garabrant et al. 1984, 1985) as sodium borate dust produced eye irritation following acute-duration exposures. The cross-sectional study design of Garabrant et al. (1984, 1985) made it difficult to determine whether the observed effects were caused by acute or repeated exposures; however, the design employed by Wegman et al. (1994), which included 6-hour TWA air samples and worker reports of irritancy before the start of the work shift and during the shift, allowed the determination that this acute ocular irritation was due to acute exposure. No ocular effects were observed in rats exposed to aerosols of boron oxide at concentrations of 470 mg boron oxide/m3 (73 mg boron/m3) for 10 weeks, 175 mg boron oxide/m3 (27 mg boron/m3) for 12 weeks, or 77 mg boron oxide/m3 (12 mg boron/m3) for 24 weeks (Wilding et al. 1959).
3.2.1.3. Immunological and Lymphoreticular Effects
No gross or microscopic effects on immunological or lymphoreticular tissues (lymph nodes and spleens) were observed in rats exposed to aerosols of boron oxide at concentrations of 470 mg boron oxide/m3 (73 mg boron/m3) for 10 weeks, 175 mg boron oxide/m3 (27 mg boron/m3) for 12 weeks, or 77 mg boron oxide/m3 (12 mg boron/m3) for 24 weeks (Wilding et al. 1959).
3.2.1.4. Neurological Effects
No gross or microscopic effects on the brain were observed in rats exposed to aerosols of boron oxide at concentrations of 470 mg boron oxide/m3 (73 mg boron/m3) for 10 weeks, 175 mg boron oxide/m3 (27 mg boron/m3) for 12 weeks, or 77 mg boron oxide/m3 (12 mg boron/m3) for 24 weeks (Wilding et al. 1959).
3.2.1.5. Reproductive Effects
A study of 28 male boric acid production workers occupationally exposed to 22–80 mg/m3 boron aerosols (boron form uncertain) for ≥10 years (Tarasenko et al. 1972) revealed low sperm counts, reduced sperm motility, and elevated fructose content of seminal fluids, compared to controls. These effects are consistent with high-dose animal exposures. However, this study is limited by the small number of subjects and limited data reporting. Furthermore, a cross-sectional survey of 753 employees working for at least 9 months at a borax production facility in California found worker fertility rates to be higher than the U.S. national average (Whorton et al. 1994). However, this study is limited by lack of exposure data.
In animals, no gross or microscopic effects were found on the ovary or testes of rats exposed to aerosols of boron oxide at concentrations of 470 mg boron oxide/m3 (73 mg boron/m3) for 10 weeks, 175 mg boron oxide/m3 (27 mg boron/m3) for 12 weeks, or 77 mg boron oxide/m3 (12 mg boron/m3) for 24 weeks (Wilding et al. 1959).
3.2.1.6. Developmental Effects
No studies were located regarding developmental effects in humans or animals after inhalation exposure to boron.
3.2.1.7. Cancer
No studies were located regarding cancer in humans or animals after inhalation exposure to boron.
3.2.2. Oral Exposure
Data for boron oral toxicity in humans involves exposure to the borates or boric acid. These boron-containing compounds are primarily found in food and water and have been implicated in numerous accidental or intentional poisonings in human case reports. Similarly, the boron toxicity studies in animals have utilized exposures to borates or boric acid.
3.2.2.1. Death
Case reports of lethal oral exposure of humans to boron primarily involve accidental or intentional exposures to high levels of boric acid. Similar clinical signs have been seen in adults and children. A review of 784 primarily acute boric acid exposures in adults and children found that 88% of cases were asymptomatic. The reports did not contain information on the dose response for boric acid, as symptomatic cases had doses ranging from 100 mg to 55 g boric acid (18 mg–10 g boron), while asymptomatic cases had doses ranging from 10 mg to 89 g boric acid (2 mg–16 g boron) (Litovitz et al. 1988). Nonetheless, death occurred in some children following oral doses in this wide dose range. Five infants who ingested formula accidentally prepared with 2.5% aqueous solution of boric acid became lethargic, developed vomiting and diarrhea, and died within 3 days after exposure (Wong et al. 1964). The estimated boric acid consumption ranged from 4.51 to 14 g (0.8–2.5g boron). In two infants who ingested 9.25 g boric acid (505 mg boron/kg/day) and 14 g boric acid (765 mg boron/kg/day), degenerative changes were seen in the liver, kidney, and brain (Wong et al. 1964). In a food poisoning incident in Malaysia, 13 children died after consuming Chinese noodles contaminated with boric acid (Chao et al. 1991a, 1991b). The deaths were determined by the study authors to be caused by unknown levels of aflatoxin and boric acid in the noodles. Clinical signs included vomiting, pyrexia, diarrhea, abdominal pain, anorexia, giddiness, seizures, and eventual coma. Postmortem examination revealed coagulative necrosis of the liver, proliferative metaplasia of the hepatocytes, giant cell formation, central vein sclerosis, bile stasis, and steatosis, acute renal tubule necrosis, upper gastrointestinal erosion, and encephalopathy. However, the relative contribution of boric acid to these effects could not be determined.
A common suite of symptoms was presented in case reports of adult oral exposures that resulted in death. A 77-year-old man ingested a single dose of 30 g of boric acid (85 mg boron/kg) to cure hiccups (Ishii et al. 1993). Effects included vomiting, diarrhea, erythema, cyanotic extremities, acute renal failure, cardiopulmonary hypotension, and death from cardiac insufficiency. Almost identical clinical signs and death occurred in a 45-year-old man ingesting approximately 49 g of boron (280 g boric acid, 700 mg boron/kg assuming a body weight of 70 kg) (Restuccio et al. 1992).
In animals, rats appear to be more sensitive than dogs or mice to the lethal effects of acute boron exposures. Oral LD50 values for respective boron equivalents of boric acid or borax were 898 and 642 mg/kg in an unspecified rat strain (Smyth et al. 1969), 600 and 510 mg/kg in Sprague-Dawley rats (Weir and Fisher 1972), and 550 and 690 mg/kg in Long Evans rats (Weir and Fisher 1972). No deaths were reported in dogs exposed to a single dose of 696 mg boron/kg (3,977 mg boric acid/kg) and 738 mg boron/kg (5,549 mg borax/kg) (Weir and Fisher 1972). No single-dose LD50 studies in mice were available; however, mortality rates of 20 and 60% were observed in males given 14 daily doses of 2,251 and 3,671 mg boron/kg/day (12.9 or 21.0 g boric acid/kg/day) in the diet, respectively (NTP 1987), but not at 926 mg boron/kg/day (5.3 g boric acid/kg/day). These animals were lethargic and exhibited discolored spleen, liver, and renal medullae and hyperplasia and dysplasia of the forestomach (NTP 1987).
With intermediate-duration oral exposure, rats appear to be slightly more sensitive than mice to the lethality of boric acid. There was 100% mortality in Sprague-Dawley rats fed 450 mg boron/kg/day within a 6-week period (Weir and Fisher 1972). Congestion of liver and kidneys, small gonads, and brain swelling were reported. Eighty percent of male and 60% of female B6C3F1 mice died in a study involving exposure to 577 mg boron/kg/day (3,298 mg boric acid/kg/day) in the diet for up to 90 days (NTP 1987). Hyperkeratosis and/or acanthosis in the stomach and extramedullary hematopoiesis of the spleen in both sexes of mice were observed at the same dose level.
With chronic exposure to boric acid in the diet, mortality at 103 weeks was ≥40 and ≥30% in male and female B6C3F1 mice, respectively, exposed to ≥79 mg boron/kg/day (≥450 mg boric acid/kg/day), compared to 18 and 34% in untreated male and female controls, respectively (NTP 1987). No clinical signs were reported for either sex; however, boron caused an increased incidence of testicular atrophy and interstitial hyperplasia in male mice exposed to doses ≥201 mg boron/kg/day (1,150 mg boric acid/kg/day).
LD50 values and, in some cases, the lowest levels at which death was reported in humans and animals and the duration categories are recorded in Table 3-2 and plotted in Figure 3-2.
3.2.2.2. Systemic Effects
No studies were located regarding respiratory effects in animals or musculoskeletal effects in humans or animals after oral exposure to boron.
Information on respiratory, gastrointestinal, hematological, cardiovascular, hepatic, renal, and dermal/ocular effects is discussed below. The highest NOAEL values and all reliable LOAEL values for these systemic effects for each species and duration category are recorded in Table 3-2 and plotted in Figure 3-2.
Respiratory Effects. Widespread vascular congestion and hemorrhages in the lungs were reported in one infant who ingested an estimated dose of 505 mg boron/kg/day for 3–5 days (Wong et al. 1964).
Cardiovascular Effects. Ingestion of 85 mg boron/kg (as 30 g of boric acid) by a 77-year-old man (Ishii et al. 1993) resulted in cardiopulmonary hypotension and death from cardiac insufficiency.
Gastrointestinal Effects. Ingestion of boron in humans can cause gastrointestinal effects. Nausea, persistent vomiting, diarrhea, and colicky abdominal pain in infants were associated with acute ingestion of a total of ≥184 mg boron/kg/day (based on 1.9 kg body weight) as boric acid, which was accidentally incorporated in infant formula (Wong et al. 1964). Vomiting and diarrhea occurred following ingestion of 85 mg boron/kg (as 30g of boric acid) by a 77-year-old man (Ishii et al. 1993). Vomiting was the only sign of boron toxicity in two adult females who ingested 14 g boron (80 g boric acid) in a fungicide and 52 g boron (297 g boric acid) in a suicide attempt. In the absence of body weight data, doses for these cases could not be estimated. The subjects were hospitalized for 24–96 hours and did not develop further symptoms following release (Linden et al. 1986). Food poisoning of 13 children with unknown levels of aflatoxin and boric acid (Chao et al. 1991a, 1991b) resulted in vomiting, diarrhea, abdominal pain, anorexia, and upper gastrointestinal erosion.
Table 3-2
Levels of Significant Exposure to Boron - Oral.

Figure 3-2
Levels of Significant Exposure to Boron - Oral.
In B6C3F1 mice, dietary exposure to ≥2,251 mg boron/kg/day as boric acid for 14 days resulted in gastric hyperplasia and dysplasia (NTP 1987). Dogs given a single dose of 1,000 mg boron/kg as boric acid vomited (Weir and Fisher 1972).
Hematological Effects. In animals orally exposed for intermediate or chronic durations, effects on hematological end points have been observed sporadically in dogs and consistently in mice; they did not occur in rats exposed for 90 days. Mongrel dogs fed 60.5 mg boron/kg/day for 90 days in the diet as borax (but not as boric acid) had decreased packed cell volume and hemoglobin values, but no hematological effects were seen in dogs fed 81 mg boron/kg/day as borax or boric acid for 2 years (Weir and Fisher 1972). Erythrocyte count and total and differential leukocyte counts were comparable to control levels (Weir and Fisher 1972). Splenic extramedullary hematopoiesis occurred in mice fed 72 mg boron/kg/day as boric acid for 90 days and 79 mg boron/kg/day for 2 years (Dieter 1994; NTP 1987), while no hematological effects were observed in rats fed 450 mg boron/kg/day as borax or boric acid for 90 days (Weir and Fisher 1972). Decreased packed cell volume and hemoglobin values were seen in rats fed 81 mg boron/kg/day as borax for 2 years (Weir and Fisher 1972).
Hepatic Effects. Case reports in humans suggest that the liver is susceptible to boron toxicity at high dose levels (Wong et al. 1964). Jaundice has been reported, and there were mild alterations at histological examination in infants who ingested 505 or 765 mg boron/kg/day as boric acid (accidentally incorporated in infant formula) for 3–5 days (Wong et al. 1964). In the same incident, congestion and fatty changes were observed, and there was parenchymatous degeneration in newborn infants who ingested 505 or 765 mg boron/kg as boric acid for 3–5 days (Wong et al. 1964). Coagulative necrosis of the liver, proliferative metaplasia of the hepatocytes, giant cell formation, central vein sclerosis, bile stasis, and hepatic steatosis were observed in children ingesting unknown levels of aflatoxin and boric acid in Chinese noodles (Chao et al. 1991a, 1991b).
In mice, the liver appears to be a toxicity target of repeated oral exposure to boric acid, but it is not a target in rats and dogs repeatedly exposed to boric acid or borates. Two-year dietary exposures of ≥79 mg boron/kg/day as boric acid to B6C3F1 mice resulted in chronic inflammation and coagulative necrosis in the liver (NTP 1987; Dieter 1994), but no exposure-related hepatic lesions were found in Sprague-Dawley rats fed 81 mg boron/kg/day as boric acid or borax in the diet for 2 years or in mongrel dogs fed 6.8 mg boron/kg/day as boric acid or borax in the diet for 2 years (Weir and Fisher 1972). No exposure-related liver lesions were seen in mice fed 577 mg boron/kg/day as boric acid for 13 weeks (Dieter 1994; NTP 1987); in Sprague-Dawley rats fed 450 mg boron/kg/day as boric acid or borax for 90 days (Weir and Fisher 1972); or in mongrel dogs fed 60.5 mg boron/kg/day as boric acid or borax for 90 days (Weir and Fisher 1972).
Boron-related changes in liver weights have not been consistently observed in animal studies. Reduced liver weights were observed in rats fed 86 mg boron/kg/day as borax for 30 days (Dixon et al. 1979) and fed 150 mg boron/kg/day as borax or boric acid for 90 days (Weir and Fisher 1972), while increased relative liver weights were seen in mongrel dogs fed 60.5 mg boron/kg/day as boric acid for 90 days (Weir and Fisher 1972).
In liver microsomal fractions from rats given approximately 20.8 mg boron/kg/day as borax in drinking water, NADPH-cytochrome C reductase activity and cytochrome b5 content decreased in the liver microsomal fraction after 10 and 14 weeks of exposure (Settimi et al. 1982). There was also a reduction in the cytochrome P-450 concentration detected at 14 weeks (Settimi et al. 1982). The toxicological significance of these biochemical changes is not clear, especially since intermediate- and chronic-duration feeding studies with Sprague-Dawley rats fed boric acid or borax did not report exposure-related hepatic lesions (Weir and Fisher 1972).
Renal Effects. Human case reports involving high accidental ingestion levels show that boron can cause injury to the kidney. Acute renal failure was observed in a 77-year-old man ingesting 85 mg boron/kg (as 30 g of boric acid) (Ishii et al. 1993). Degenerative changes in parenchymal cells with oliguria and albuminuria have been demonstrated in two newborn infants after ingestion of 505 and 765 mg boron/kg/day as boric acid in an evaporated milk formula over a period of 3–5 days (Wong et al. 1964). Acute renal tubule necrosis was seen in children dying from ingestion of unknown levels of aflatoxin and boric acid in Chinese noodles (Chao et al. 1991a, 1991b).
No exposure-related renal lesions were observed in Sprague-Dawley rats fed up to 450 mg boron/kg/day as borax or boric acid for 90 days (Weir and Fisher 1972), in mice fed 577 mg boron/kg/day as boric acid for 13 weeks (NTP 1987; Dieter 1994), or in dogs fed up to 60.5 mg boron/kg/day as borax or boric acid for 90 days (Weir and Fisher 1972). With 2 years of dietary exposure, no exposure-related renal lesions were observed in Sprague-Dawley rats fed 81 mg boron/kg/day as borax or boric acid (Weir and Fisher 1972), in B6C3F1 mice fed up to 201 mg boron/kg/day as boric acid (Dieter 1994; NTP 1987), or in mongrel dogs fed 6.8 mg boron/kg/day as borax or boric acid (Weir and Fisher 1972). The available data indicate that the kidney is not a sensitive toxicity target of oral exposure to boric acid or borates.
Endocrine Effects. Human data for endocrine effects from orally ingested boron are limited to studies of low-dose boron nutritional supplementation. Postmenopausal women ingesting 0.4 mg boron/day (as 3.25 mg sodium borate/day) in the diet had a 3-fold increase in plasma testosterone levels compared to those ingesting 0.03 mg boron/day (as 0.25 mg sodium borate/day) (Nielsen et al. 1987). However, bodybuilders taking daily supplements of 2.5 mg boron (approximately 0.03 mg/kg; calculated using mean body weights from study data) for 7 weeks did not exhibit differences from controls in free or total plasma testosterone levels (Ferrando and Green 1993).
Rats fed diets with 61 mg boron/kg/day as boric acid (9000 ppm) for 28 days had decreases of approximately 60–78% in plasma testosterone levels beginning on day 4 of exposure (Treinen and Chapin 1991). Follicle stimulating hormone (FSH) and leutenizing hormone (LH) levels in the blood of rats fed 26–68 mg boron/kg/day as boric acid (3,000–9,000 ppm; doses estimated by study authors) in the diet were increased, possibly in response to testicular atrophy observed in the 52 and 68 mg boron/kg/day groups (Ku et al. 1993a). No histologic lesions were observed in thyroid, adrenals, or pituitary tissues of Sprague-Dawley rats fed 450 or 81 mg boron/kg/day as boric acid or borax in the diet for 90 days or 2 years, respectively, or in mongrel dogs fed 60.5 or 6.8 mg boron/kg/day as boric acid or borax in the diet for 90 days or 2 years, respectively (Weir and Fisher 1972) or in pituitary tissues of mice fed 577 or 201 mg boron/kg day as boric acid for 90 days or 2 years, respectively (Dieter 1994; NTP 1987).
Dermal Effects. Skin effects can occur following ingestion of boron (as boric acid) in humans. Extensive exfoliative dermatitis began in infants as an erythema involving palms, soles, and buttocks. It eventually became generalized with subsequent bulbous formation, massive desquamation, and sloughing (Wong et al. 1964). These changes were associated with ingestion of 505 mg boron/kg/day; however, skin lesions were lacking following ingestion of 765 mg boron/kg/day. Similarly, extensive erythema with desquamation was observed in an adult who ingested single doses of boric acid powder (Schillinger et al. 1982). The exact amount ingested was not stated. However, 14 g (equivalent to 22.5 mg boron/kg based on 109 kg body weight) was measured as missing from a container from which the patient admitted consuming half its contents.
In animals studies, skin lesions were observed in Sprague-Dawley rats fed 150 mg boron/kg/day as borax or boric acid in the diet for 90 days (skin desquamations on the paws and tails) or 81 mg boron/kg/day for 2 years (scaly tails and desquamation on footpads) (Weir and Fisher 1972), but dermal lesions were not observed in B6C3F1 mice exposed to boric acid in the diet for 13 weeks or 2 years (Dieter 1994; NTP 1987) or in mongrel dogs exposed to boric acid or borax in the diet for 90 days or 2 years (Weir and Fisher 1972).
Ocular Effects. There are no reports of ocular effects in humans following oral exposure to humans.
Sprague-Dawley rats fed 150 mg boron/kg/day as borax or boric acid for 90 days exhibited inflammation of the eyes, while 81 mg boron/kg/day as borax or boric acid in the diet for 2 years resulted in bloody ocular discharge (Weir and Fisher 1972). No ocular effects were seen in dogs fed 60.5 mg boron/kg/day as borax or boric acid for 90 days or 6.8 mg boron/kg/day borax or boric acid for 2 years (Weir and Fisher 1972). Likewise, mice fed 201 mg boron/kg/day as boric acid for 2 years exhibited no ocular effects.
Body Weight Effects. Fisher 344 rats fed 68 mg boron/kg/day as boric acid (9,000 ppm) for 9 weeks had 6% lower body weight gain than controls (Ku et al. 1993a). Male and female B6C3F1 mice fed 288 and 577 mg boron/kg/day as boric acid (0.5% of diet) for 13 weeks had 17 and 23% decreased weight gains, respectively, while 2-year exposures to 201 mg boron/kg/day as boric acid resulted in 19% lower weight gains in both sexes (NTP 1987, Dieter 1994).
3.2.2.3. Immunological and Lymphoreticular Effects
No studies were located regarding immunological effects in humans or animals after oral exposure to boron.
3.2.2.4. Neurological Effects
Case reports in humans have indicated neurological effects after accidental ingestion of high levels of boron (as boric acid). Newborn infants who ingested 4.5–14 g boric acid showed central nervous system involvement manifested by headache, tremors, restlessness, and convulsions followed by weakness and coma (Wong et al. 1964). Histological examination of 2 of 11 infants revealed congestion and edema of brain and meninges with perivascular hemorrhage and intravascular thrombosis at a dose ≥505 mg boron/kg/day (Wong et al. 1964). Seizure disorders have been associated with boron exposures (as borax) in infants who ingested 12–120 g borax for 4–10 weeks (O’Sullivan and Taylor 1983) and 9–125 g borax over a period of 5–12 weeks (Gordon et al. 1973). Estimates of boron dose could not be determined since the authors did not provide body weight data. Blood boron levels in the infants exposed who ingested borax ranged from 2.6 to 8.5 µg/mL (O’Sullivan and Taylor 1983). In one infant with a seizure disorder who ingested (via pacifier dipped in honey and borax mixture) approximately 125 g borax over 3 months, the blood boron level was 1.64 mg/100 mL (Gordon et al. 1973).
Existing animal studies do not provide evidence that the nervous system is a toxicity target of repeated oral exposure to boric acid or borates, but no studies have examined batteries of neurological end points in animals following exposure to boron compounds. Relative brain weights were increased in mongrel dogs fed 60.5 mg boron/kg/day as borax, but not boric acid, for 90 days and in rats fed 170 or 81 mg boron/kg/day as borax or boric acid for 90 days or 2 years, respectively (Weir and Fisher 1972). No histological lesions were observed in these animals or in the brain or spinal cord of mice fed 577 or 201 mg boron/kg/day as boric acid for 13 weeks or 2 years, respectively (NTP 1987; Dieter 1994).
In Wistar rats, exposure to approximately 20.8 mg boron/kg/day as borax (based on weight of 0.35 kg and average water consumption of 20.7 mL) in drinking water for up to 14 weeks caused increased cerebral succinate dehydrogenase activity after 10 and 14 weeks of exposure (Settimi et al. 1982). Increased ribonucleic acid (RNA) concentration and increased acid proteinase activity in brain occurred after 14 weeks (Settimi et al. 1982). The neurological significance of these biochemical changes is unclear.
All LOAEL values for neurological effects in humans and animals are recorded in Table 3-2 and plotted in Figure 3-2.
3.2.2.5. Reproductive Effects
A survey of Turkish subpopulations compared fertility rates of 1,068 families living in two Turkish villages having drinking water boron levels of 2–29 mg/liter (from nearby geological deposits of calcium borate) with 610 families living in three other villages having drinking water boron levels of 0.03–0.4 mg/liter (Sayli 1998a, 1998b; Sayli et al. 1998). Assuming 70-kg body weights and 2-L/day drinking water consumption, these boron levels would results in an estimated range of daily doses of 0.06–0.8 mg/kg/day. Three generations of families were represented. No significant differences in frequencies of infertility were observed between high- (2.34% infertility) and low-exposure (2.62% infertility) village groups. A separate analysis of the same subpopulation found no association of higher drinking water borate concentrations with increased rates of spontaneous abortions, stillbirths, or infant death (Tuccar et al. 1998). A follow-up study of this population reported no significant differences in infertility frequencies between the two populations (Sayli 2003).
A questionnaire was administered to 542 male workers at a borax and boric acid production facility in California inquiring about the ability to conceive a child after at least 9 months of employment at the facility (Whorton et al. 1994). The worker population was categorized as having low, mid, or high exposures to borax or boric acid (exposure levels were not reported). A standardized birth ratio (SBR) was calculated as the number of children born to wives compared to the number of births expected in the same fraction of the U.S. population. The calculated SBR of 113 indicated higher birth rates among the borate workers relative to the U.S. population. Thus, this survey provided no evidence for association between occupational exposure to borax or boric acid and impaired fertility; however, the study is limited by non-rigorous survey design, lack of quantitative exposure data, and lack of a comparable comparison (control) group.
A cross-sectional survey of 1,187 Chinese boron mining and processing workers examined the association of boron exposure and various lifestyle factors to multiple reproductive indices (Chang et al. 2006). No exposure estimates were reported, but boron levels in drinking water and staple foodstuffs were significantly higher for surveyed workers compared to a comparison population. After correcting for age, race, diet, alcohol consumption, smoking, and education, there were no statistically significant differences between workers and the comparison population for ability to sire offspring, delayed pregnancy, multiple births, miscarriages, induced abortions, stillbirths, or tubal or ectopic pregnancies.
Liu et al. reported two studies of 176 (2006a) and 195 (2006b) Chinese men exposed to between 13 and 430 mg boron/day. The investigators found no significant difference in semen quality and sperm motility, speed, or departure from a straight-line path in groups of borate workers, non-occupationally exposed men from a nearby community with high environmental levels of borates, and men from a community with low environmental levels of borates. However, data for estimated boron exposures for each group were not available.
A study of 146 Chinese men comprising borate workers, a community group, and controls were assessed for chromosomal shifts in the ratio of Y:X chromosomes in sperm (Robbins et al. 2008). A significant correlation was found for Y:X ratios and internal boron doses in blood, semen, and urine. Estimated daily boron exposures of 41.2, 4.3, and 2.3 mg/day in the borate workers, community group, and controls were associated with mean Y:X sperm ratios of 0.93, 0.96, and 0.99, respectively. However, no dose-response relationship was observed in the reported percentage of men who had fathered “more boys than girls”, which was 57.7, 42.3, and 76.7% in the borate workers, community group, and controls, respectively. There were several possible confounding factors in this study, including (1) the exclusion of men who had fathered equal numbers of boys and girls, (2) the average number of children/subject was <2 (approximately 1.3), and (3) a high rate of elective abortions across groups (49.2, 28.2, and 50.0% for borate workers, community group, and controls, respectively), in which some men may have favored raising male offspring while complying with China’s one-child-only policy. Thus, it cannot be determined from these data if a boron-associated shift in the Y:X sperm ratio resulted in a change in sex of offspring.
While the human survey database is extensive in that large populations with chronic exposure durations were sampled from multiple locations (Chang et al. 2006; Liu et al. 2006a, 2006b; Robbins et al. 2008; Sayli 1998a, 1998b, 2003; Sayli et al. 1998; Whorton et al. 1994), they are limited for informing dose response relationships for reproductive effects. Specifically, the reliance on questionnaires and lack of clinical observations, absence of an appropriate comparison population (Robbins et al. 2008; Whorton et al. 1994), or low confidence in estimates of personal or group boron exposure (Liu et al. 2006a, 2006b) preclude these data from providing a basis for deriving an oral MRL.
Animal studies of acute-, intermediate-, and chronic- oral exposure to boric acid or borax consistently identified testicular atrophy and histological lesions and the associated impacts on spermatogenesis as the most sensitive reproductive effect. The majority of studies were performed in rats; however, the effects observed in rats were also observed in mice and dogs. The effects of boron on animal testes appear to be dose- and duration-related.
Acute-duration (2-week) oral gavage or dietary exposures of Wistar rats to 88 mg boron/kg/day as boric acid produced significant damage to male reproductive tissues, but doses of 53 or 44 mg boron/kg/day were without effect (Fukuda et al. 2000; Ku et al. 1993a; Kudo et al. 2000). Exposure to 88 mg boron/kg/day resulted in 12 and 13% reduction in absolute and relative testes weights, respectively, multinucleated giant cell formation, increased residual body-like structures in the testes, degeneration/necrosis of germ cells, increased cellular debris in the epididymal ducts, exfoliation of round spermatids, and mild inhibition of spermiation (retention of step 19 spermatids at stages IX–XI) (Fukuda et al. 2000; Kudo et al. 2000).
Intermediate-duration gavage studies in rats resulted in similar effects as observed in the acute studies, but at lower exposure levels. Wistar rats given gavage doses of 26 mg boron/kg/day as boric acid for 3 weeks exhibited decreased sperm motility, morphologically-abnormal sperm heads and tails, and increased preimplantation fetal loss when treated males were mated with untreated females; no reproductive effects occurred in this study with exposure to 9 mg boron/kg/day (Yoshizaki et al. 1999). Four-week daily gavage doses of 44 mg boron/kg/day as boric acid resulted in exfoliation of testicular and epididymal germ cells in Wistar rats (Kudo et al. 2000). Fukuda et al. (2000) gave gavage doses of 53 mg boron/kg/day to Wistar rats for 4 weeks and observed 13 and 15% reductions in absolute and relative testes weights, respectively, cellular debris in the testes, caudal and caput epididymis, focal atrophy of the seminiferous tubules, and decreased number of sperm in the ducta lumina. Rats given 4-week daily gavage doses of 88 mg boron/kg/day group exhibited reduced sperm motility, reduced total sperm in caudal epididymis, atrophy of seminiferous tubules, atypical residual bodies, multinucleated giant cell formation, and the inability to impregnate females (Yoshizaki et al. 1999).
Intermediate-duration feeding studies in rats reported effects similar to those of the gavage studies. Rats fed 100 mg boron/kg/day as borax in the diet, but not 50 mg boron/kg/day, for 30 and 60 days showed testicular atrophy (Lee et al. 1978). Sprague-Dawley rats fed 86 mg boron/kg/day as borax in the diet for 30 or 60 days were infertile for 3 or 5 weeks after exposure, respectively (Dixon et al. 1979). Exposure to 43 mg boron/kg/day as borax for 60 days produced reduced testicular and epididymal weights and diameter of seminiferous tubules occurred, and reduced testicular levels of hyaluronidase, sorbitol dehydrogenase, and lactic acid dehydrogenase (isoenzyme-X) at 30 days (Dixon et al. 1979). Mildly inhibited spermiation was observed in Fischer 344 rats exposed to 26 mg boron/kg/day in the diet for 5–9 weeks (Ku et al. 1993a). Fischer 344 rats fed 61 mg boron/kg/day as boric acid for 4 weeks showed inhibited spermiation, appearance of peripheral spermatid nuclei, and spermatocyte sloughing/epithelial disorganization (Treinen and Chapin 1991), while severe inhibition of spermiation and testicular atrophy were observed in Fischer 344 rats exposed to 68 or 52 mg boron/kg/day as boric acid in the diet for 6 or 9 weeks (Ku et al. 1993a). Full recovery from inhibition of spermiation was observed in a 38 mg boron/kg/day group by 16 weeks after cessation of exposure for 9 weeks, but no recovery from testicular atrophy was observed in the 52 and 68 mg boron/kg/day groups up to 32 weeks after exposure ended (Ku et al. 1993a). Dose-related elevations of FSH and LH suggested that boron exposure did not affect the compensatory response to atrophy (Ku et al. 1993a). In a recent study, Sprague-Dawley rats fed 136 mg boron/kg/day as boric acid for 60 days exhibited decreased weights of testes, epididymes, seminal vesicles, prostate, and vas deferens; decreased sperm motility, spermatocyte, spermatid, and Leydig cell numbers; decreased testosterone levels, sexually aggressive behavior, sexual mounts, number of females impregnated, and viable pups/impregnated female; and increased cellular degeneration, ejaculation time, postejaculatory interval, and fetal resorptions in impregnated females (Nusier and Bataineh 2005).
In intermediate-duration drinking water studies in rats, no reproductive effects (reproductive organ weight or histolopathology) were evident in Sprague-Dawley rats following exposure to 0.6 mg boron/kg/day as borax for 90 days (Dixon et al. 1976). In another study, impaired spermatogenesis was observed in Long-Evans rats given 44.7 mg boron/kg/day as borax in drinking water for 70 days (Seal and Weeth 1980). Complete sterility was observed in Sprague-Dawley rats fed 1,170 ppm boron equivalents in the diet as boric acid or borax (at an estimated dose level of 101 and 116 mg boron /kg/day for males and females); sterility was associated with a lack of viable sperm in atrophied testes in males and decreased ovulation in females (Weir and Fisher 1972). Rats were exposed for 14 weeks before mating in this study. No pregnancies occurred when female rats exposed to this dose level were mated with non-exposed male rats. At lower exposure levels (10 or 30 mg boron/kg/day for males and 12 or 35 mg boron/kg/day for females), no exposure-related adverse effects were found on overall fertility indices in three successive generations (Weir and Fisher 1972).
Studies in mice and dogs support the observations of reproductive effects seen in rats. In a study in which male CD-1 mice were exposed to gavage doses of boric acid of 0, 21, 70, or 210 mg boron/kg/day for 21 days (5 days before mating, during 5 days of mating, and extending to 21 total days of exposure), average testes weights were decreased at doses ≥70 mg boron/kg/day, and exfoliation/disruption of seminiferous tubules and inhibited spermiation were observed at 210 mg boron/kg/day (Harris et al. 1992). Exposed male mice were mated to similarly exposed female mice (except that females were exposed for 8 days before mating), but no exposure-related effects were found on the percentage of females who became pregnant, the number of live pups per litter, or the weight of pups at birth (Harris et al. 1992). Degeneration of the seminiferous tubules was seen in mice exposed to 288 mg boron/kg/day as boric acid in the diet for 13 weeks, while 103-week dietary exposure to 201 mg boron/kg/day as boric acid resulted in testicular atrophy, degeneration of the seminiferous tubules, and interstitial hyperplasia (Dieter 1994; NTP 1987).
In a 2-generation (27-week) feeding study in CD-1 mice using a continuous breeding protocol, seminiferous tubule degeneration, impaired spermatogenesis, and reduced sperm motility resulted from ≥111 mg boron/kg/day as boric acid (Fail et al. 1991). These doses were also associated with reduced litter size and fetal body weight. No effects were observed in a 27 mg boron/kg/day dose group.
In mongrel dogs fed boric acid or borax for 90 days, severe testicular atrophy was seen at 60.5, but not at 6 mg boron/kg/day (Weir and Fisher 1972). With 2 years of exposure, testicular atrophy and spermatogenic arrest were observed in dogs exposed to 22.8, but not in dogs exposed to 6.8 mg boron/kg/day (Weir and Fisher 1972).
Effects of boric acid or borates on female reproductive organs and their functions are less clearly identified and studied in animals than effects on male reproductive organs. When pregnant CD-1 mice were exposed to gavage doses of 210 mg boron/kg/day on gestation days 8–14, all dams failed to deliver litters (Harris et al. 1992). Exposure to 21 or 70 mg boron/kg/day during the same period did not affect littering ability, average litter weight, or the number of live neonates at postnatal days 0, 1, and 4 (Harris et al. 1992). Mechanistic aspects of this effect of gestational exposure on littering capability of pregnant rats are unstudied. As discussed earlier, female Sprague Dawley rats exposed for 14 weeks to 1,170 ppm boron equivalents in the diet as boric acid or borax (estimated dose level of 116 mg boron/kg/day) did not become pregnant when mated with non-exposed males (Weir and Fisher 1972). The female sterility response at this dose level was associated with decreased ovulation.
The highest NOAEL values and all reliable LOAEL values for reproductive effects in animals and duration category are recorded in Table 3-2 and plotted in Figure 3-2.
3.2.2.6. Developmental Effects
No studies were available identifying developmental toxicity in humans from exposure to boron. However, several types of developmental effects (e.g., decreased fetal body weight, increased incidence of skeletal abnormalities) in animals were observed in standard developmental toxicity studies involving oral exposure of pregnant mice, rats, and rabbits to boric acid of borate salts. In addition, reduced pup weight at birth has been observed in animals receiving intermediate-duration exposures.
In mice, reduced fetal body weight and skeletal malformations were seen following acute- and intermediate-duration maternal oral exposures to boric acid.
Pregnant CD-1 mice fed 79 mg boron/kg/day as boric acid on gestation days 0–17 had fetuses with 33% lower body weight compared with controls, while fetal skeletal effects (e.g., short rib XIII, agenesis of lumbar vertabra, fused ribs) were reported at 175.3 mg boron/kg/day on gestation days 0–17 (Heindel et al. 1992, 1994). No effects on fetal development were observed in the 43.4 mg boron/kg/day dose group (Heindel et al. 1992, 1994).
No effects on implantation sites, littering, number of live pups per litter, or postnatal pup weight were observed following gavage exposure of pregnant CD-1 mice to 70 mg boron/kg/day as boric acid on gestation days 8–14 (Harris et al. 1992).
In an acute-duration study, pregnant CD-1 mice were given gavage doses of boric acid on various gestation days to examine the influence of stage of fetal development on skeletal malformations caused by boric acid (Cherrington and Chernoff 2002). When two gavage doses of 70 mg boron/kg as boric acid were given on gestation day 6, 7, 8, 9, or 10, increased incidence of fetuses with cervical rib and rib agenesis were observed in the groups treated on gestation day 8, but not with exposure on gestation days 6, 7, 9, or 10 (Cherrington and Chernoff 2002). Similarly, increased incidences of fetuses with cervical rib, rib agenesis, reduced rib length, and fused ribs were seen after twice daily doses of 70 mg boron/kg on gestation days 6–8. Reduction in length of fetal rib XIII was seen in groups dosed once daily with 88 mg boron/kg/day on gestation days 6–10. A single dose of 131 mg boron/kg on gestation day 8 resulted in increased incidence of fetuses with cervical ossification, while two doses of 131 mg boron/kg on gestation day 8 caused multiple thoracic skeletal malformations. Reduced fetal weight was observed in all treated groups (Cherrington and Chernoff 2002). This study did not identify acute NOAELs for fetal skeletal effects in mice. The study authors suggested that boric acid may alter gastrulation and presomitic mesoderm formation in CD-1 mice, which are key gestational milestones for axial skeletal development.
In rats, acute-duration developmental toxicity data were not available. However, intermediate-duration oral exposure of pregnant rats exhibited effects on fetal skeletal development and fetal weight, similar to those observed in mice. Pregnant Sprague-Dawley rats fed 13.6 boron/kg/day as boric acid in the diet on gestation days 0–20 had reduced fetal body weight, and increased incidence of fetuses with skeletal abnormalities at gestation day 20 occurred in groups of dams fed 28.4 mg boron/kg/day (Heindel et al. 1992). Skeletal abnormalities observed in groups fed 28.4 mg boron/kg/day included agenesis or shortening of rib XIII, increased incidence of fetuses with enlargement of the lateral ventricles of the fetal brain, and increased resorptions (Heindel et al. 1992). Pregnant Sprague-Dawley rats fed 10 mg boron/kg/day as boric acid on gestation days 0–20 exhibited no developmental effects, but exposures of 13 mg boron/kg/day as boric acid resulted in decreased fetal body weight and skeletal abnormalities seen on gestation day 0. However, in a second phase of this study, identically treated dams were allowed to litter and pups were observed through postnatal day 21. Upon necropsy, these pups did not exhibit significantly different body weights or incidences of skeletal abnormalities seen and fetuses examined on gestation day 0 (Price et al. 1996a, 1998).
Developmental toxicity data in rabbits are available only for acute-duration oral exposures (Price et al. 1996b). Pregnant New Zealand white rabbits given gavage doses of 44 mg boron/kg/day as boric acid on gestation days 6–19 exhibited increased maternal body weight (corrected for gestation) and reduced maternal kidney weight, gravid uterine weight, fetal body weight, number of ovarian corpora lutea, number of implantation sites, and live fetuses, compared with controls. Resorptions and fetal external (cleft palate), visceral (enlarged lateral ventricle of the brain), skeletal (cleft sternum, fused sternebrae), and cardiovascular (enlarged aorta, interventricular septal defect) malformations were increased, compared with controls. No significant maternal or fetal effects were observed following gavage doses of 22 mg boron/kg/day as boric acid. The observed effects are consistent with those seen in acute-, intermediate-, and chronic-duration oral exposures in other animals. These data represent the most sensitive adverse effects observed in any species following acute-duration oral exposures. Thus, an acute-duration MRL of 0.2 mg boron/kg/day was derived based on a NOAEL of 22 mg boron/kg/day and a LOAEL of 44 mg boron/kg/day for developmental effects in New Zealand white rabbits (Price et al. 1996b).
With intermediate-duration oral exposure to boric acid, reduced fetal body weight and skeletal abnormalities were consistently observed in developmental toxicity assays of mice, rats, and rabbits. Skeletal malformations increased in variety and severity with dose. However, reductions in fetal body weight appear to occur at lower exposure levels than those associated with skeletal abnormalities. With intermediate-duration exposure during gestation, the most sensitive developmental effect identified across all three species was reduced fetal weight in pregnant Sprague-Dawley rats fed 13.6 mg boron/kg/day in the diet on gestation days 0–20. This effect was seen at lower intermediate-duration exposure levels than the lowest intermediate-duration oral dose associated with reproductive effects in rats (i.e., a LOAEL of 26 mg boron/kg/day was identified for inhibition of spermiation in rats) (Ku et al. 1993a). An intermediate oral MRL of 0.2 mg boron/kg/day was calculated as described in the footnote on Table 3-2, based on a benchmark dose analysis (Allen et al. 1996) of combined data sets (Heindel et al. 1992; Price et al. 1996a) for reduced fetal body weight in rats.
The highest NOAEL values and all reliable LOAEL values for developmental effects in animals and duration category are recorded in Table 3-2 and plotted in Figure 3-2.
3.2.2.7. Cancer
Three epidemiological studies have associated increased boron intake in drinking water with decreased incidences of prostate and vaginal cancer. Cui et al. (2004) used the cross-sectional data from the NHANES III study, conducted from 1988 to 1994, which contained health and diet information for the non-institutionalized U.S. population. These investigators reported that men with mean intakes of ≥1.54 mg boron/day had significantly less risk of developing prostate cancer than men ingesting ≤0.52 mg/day. This study was limited by its cross-sectional design and reliance on 1-day recall of diet information to estimate boron exposure. A second study (Barranco et al. 2007) on a Texas population correlated increased boron in groundwater with reduced prostate cancer incidence rates. However, the observed correlation appeared to be driven primarily by 2–3 specific cases. Korkmaz et al. (2007) studied 1,059 rural Turkish women and associated higher boron intake (as evidenced by approximately 8-fold higher urinary boron concentration) with lower incidences of cervical cytopathology (0 findings in the high-boron group, 15 cases in the low-boron group). While this study did attempt to correct for lifestyle factors and other genotoxic confounders, it was cross-sectional in design. The hypotheses drawn from these studies are interesting; however, no clinical studies in humans or animals are available to substantiate effects of anti-tumor protection offered by boron.
No evidence of exposure-related cancer was observed in rats exposed to 81 mg boron/kg/day as boric acid or borax for 2 years (Weir and Fisher 1972), dogs exposed to 6.8 mg boron/kg/day as boric acid or borax for 2 years (Weir and Fisher 1972), or mice exposed to 201 mg boron/kg/day as boric acid for 2 years (Dieter 1994; NTP 1987). In nude mice subcutaneously injected with human LNCaP cells (prostate tumor clones), oral gavage doses of boric acid were given for 8 weeks (Gallardo-Williams et al. 2004) to determine if boron offered protection against prostate tumor growth. Although there was no significant difference between control and boron-treated mice in tumor incidences, the tumor sizes in mice given 1.7 mg boron/kg/day were significantly smaller and the serum level of tumor specific antigen (PSA) was significantly less than controls.
3.2.3. Dermal Exposure
Information on dermal toxicity in humans involves exposure to the borates (as boric acid or borax), while the animal data involves exposure to boron oxide, which easily converts to boric acid in humid air or upon entering the mucosal layer of tissues.
3.2.3.1. Death
No studies were located regarding death in humans or animals after dermal exposure to boron.
3.2.3.2. Systemic Effects
No studies were located regarding hematological and dermal/ocular effects in humans or regarding respiratory, cardiovascular, gastrointestinal, musculoskeletal, hepatic, or renal effects in humans or animals after dermal exposure to boron.
Hematological Effects. Draize and Kelley (1959) reported the application of 25–200 mg/kg/day boric acid in aqueous solution did not produce hematological changes when rubbed onto intact skin during a 90-day rabbit study. No quantitative data were provided; therefore, these results could not be evaluated.
Dermal Effects. Human data are limited to case reports of accidental exposure of the head. Three male workers (59-year-old waste handler and 34- and 36-year-old automotive mechanics) presented with general or focal alopecia of the scalp, presumably from spillage or wiping of boric acid or borax, respectively, onto the head (Beckett et al. 2001). In the case of the waste handler, the concentration of boric acid in the milieu of other known solvents in the waste tank was unknown. In the cases of the automotive workers, exposure was determined to arise from under-the-chassis flushing of automobile radiators which contained coolant solutions of ethylene glycol and 1–5% borax. Actual exposures could not be determined. Blood sample analysis revealed no elevated blood boron levels in any of the subjects. Gradual and full hair re-growth occurred.
In animals, application of 1 g boron oxide dust to a 25 cm2 area of the skin of four rabbits produced erythema that lasted for 2–3 days (Wilding et al. 1959).
Ocular Effects. Instillation of boron oxide dust (50 mg) into the eyes of four rabbits produced conjunctivitis (Wilding et al. 1959).
No studies were located regarding the following effects in humans or animals after dermal exposure to boron:
3.2.3.3. Immunological and Lymphoreticular Effects
3.2.3.4. Neurological Effects
3.2.3.5. Reproductive Effects
3.2.3.6. Developmental Effects
3.2.3.7. Cancer
3.2.4. Other Routes of Exposure
Direct application of a solution containing 6.3 mg boron (as sodium perborate monohydrate) onto the cornea of rabbits resulted in mild irritancy of the epithelium and superficial stroma (Maurer et al. 2001).
3.3. GENOTOXICITY
No studies were located regarding genotoxic effects of boron by inhalation, oral, or dermal exposure in humans.
Results were predominantly negative in bacterial assays and in in vitro (Table 3-3) mammalian assays, including tests for chromosomal aberrations, gene mutation (Benson et al. 1984; Demerec et al. 1951; Haworth et al. 1983; Landolph 1985; NTP 1987), and cell transformation (Landolph 1985). Induction of β-galactosidase as part of the SOS response was observed in Escherichia coli PQ37, both with and without S-9 metabolic activation (Odunola 1997). Structural chromosomal aberrations, but not sister chromatid exchanges, were observed in cultured human lymphocytes exposed to boric acid (Arslan et al. 2008).
Table 3-3
Genotoxicity of Boron In Vitro.
3.4. TOXICOKINETICS
3.4.1. Absorption
3.4.1.1. Inhalation Exposure
Reports of upper respiratory tract symptoms of irritation following exposure to boron oxide and boric acid dusts suggest that boron can deposit in the upper airway (Garabrant et al. 1984, 1985). Borax production workers were found to have approximately an order of magnitude higher blood and urine concentrations of boron at the end of a work shift compared to the beginning, suggesting that inhaled boron is absorbed and systemically distributed (Culver et al. 1994a).
3.4.1.2. Oral Exposure
Near-complete gastrointestinal absorption was indicated in humans as evidenced by the urinary recovery of 93.9% of the ingested dose of boric acid over a 96-hour collection period (Jansen et al. 1984a). Dourson et al. (1998) reviewed data from the literature to estimate oral absorption fractions of 81–92% for humans and 95% for animals (rats).
3.4.1.3. Dermal Exposure
Volunteers exhibited 0.23 and 0.21% percutaneous absorption of 1.8 mL of 5% solutions of boric acid and borax (Wester et al. 1998). Urinary excretion studies in humans (Section 3.4.4.3) suggest there is very little absorption of boron through intact skin. Excretion studies (Section 3.4.4.3) in rabbits suggest that boron is readily absorbed following contact with damaged skin (Draize and Kelley 1959).
3.4.2. Distribution
No quantitative studies were located regarding distribution in humans.
3.4.2.1. Inhalation Exposure
No studies were located regarding distribution of boron in animals after inhalation exposure.
3.4.2.2. Oral Exposure
Boron evenly distributed to liver, kidney, brain, muscle, adrenals, epididymis, testes, seminal vesicles, and blood, but not fat, of male rats fed 61 mg boron/kg/day as boric acid (9,000 ppm) for 1–28 days (Ku et al. 1991; Moseman 1994; Treinen and Chapin 1991), reaching steady-state by 4 days. Blood and testes boron levels were similar in rats fed 26–68 mg boron/kg/day as boric acid (3,000–9,000) for 9 weeks (Ku et al. 1991). However, boron accumulated in bone in male rats fed 61 mg boron/kg/day (as boric acid) for 9 weeks, with achievement of steady-state at 4 weeks. Bone levels were approximately 3-fold higher than soft tissue levels (Moseman 1994).
3.4.2.3. Dermal Exposure
No studies were located regarding distribution of boron in animals after dermal exposure.
3.4.3. Metabolism
As an inorganic chemical, boron is not expected to be metabolized by humans or animals. Studies of inhalation and oral exposure of animals and humans to borates have consistently reported recovery of the parent borate only in the blood, tissues, and urine (Culver et al. 1994a; Draize and Kelley 1959; Jansen et al. 1984a; Ku et al. 1991; Moseman 1994; Treinen and Chapin 1991).
3.4.4. Elimination and Excretion
3.4.4.1. Inhalation Exposure
Over 94% of estimated total boric acid (mean 11.84 mg boron/day) inhaled and ingested by Chinese borate workers was eliminated 24 hours later in the urine (Xing et al. 2005) as determined by the percent estimated daily dose recovered in the urine. In rats that inhaled average concentrations of 77 mg/m3 boron oxide aerosols over a 22-week period, an average of 11.90 mg boron/kg/day was detected in the urine compared to 0.24 mg/kg/day in untreated control groups (Wilding et al. 1959).
3.4.4.2. Oral Exposure
Over 93% of the administered dose was excreted in the urine of six male volunteers 96 hours after administration of a single oral dose of 1.9 mg boron/kg (as boric acid) (Jansen et al. 1984a). An analysis of nine cases involving boric acid poisoning revealed a mean half-life of 13.4 hours (range 4–27.8 hours).
There was no correlation between half-life and calculated serum boric acid level at to (r=0.08, p=0.84) (Litovitz et al. 1988). Boric acid was detected in urine of patients 23 days after a single ingestion (Wong et al. 1964). Renal clearance of dietary boron from fifteen pregnant women was calculated to be 1.02 mL/minute/kg, or 66.1 mL/minute (Pahl et al. 2001).
In rabbits, 50–66% of the administered dose was recovered in urine after ingestion of 17.1–119.9 mg boron/kg/day as boric acid (Draize and Kelley 1959). In rats fed 26–68 mg boron/kg/day as boric acid (3,000–6,000 ppm) for 9 weeks, boron concentrations in bone began decreasing after cessation of exposure; however, bone levels remained approximately 3-fold higher than controls for up to 32 weeks (Chapin et al. 1997; Moseman 1994). Blood levels in these same animals returned to control levels within 7 days of exposure cessation (Ku et al. 1991). Using literature data, Dourson et al. (1998) estimated the fraction eliminated of absorbed boron to be 67–98% in humans and 99% in rats. These investigators also calculated clearance values of 40 mg/kg/hour in humans, 163 mg/kg/hour in rats, and 397 mg/kg/hour in pregnant rats. Pregnancy did not affect renal clearance (0.2 L/hour/kg or 1.0 mL/minute) or elimination half-life (3.2 hours) in rats given gavage doses of 0.05–5 mg boron/kg/day (as boric acid) on gestation day 16 (Vaziri et al. 2001).
3.4.4.3. Dermal Exposure
Limited data in humans suggest that very little absorption of boron occurs through intact skin. There was no increase in the urinary excretion of boron in one human subject following the application of 15 g boric acid (37.5 mg boron/kg body weight) on the forearm for 4 hours (Draize and Kelley 1959). In volunteers having 1.8 mL of 5% boric acid or borax solutions applied to the back and left for 24 hours, urinary boron levels increased to peak values of <0.1% above background levels at 14 days following exposure (Wester et al. 1998).
Animal studies support human findings. Draize and Kelley (1959) applied 200 mg/kg as boric acid to intact, abraded or burnt, and partially denuded skin of rabbits. Net urinary excretion of boric acid per 24 hours during 4 consecutive days of compound treatment was 1.4, 7.6, and 21.4 mg/kg, respectively (0.25, 1.3, and 3.7 mg boron/kg, respectively).
3.4.4.4. Other Routes of Exposure
In eight adult volunteers administered a single dose of boric acid (562–611 mg) by intravenous infusion, 98.7% of the administered dose was recovered in urine 120 hours after injection (Jansen et al. 1984b).
Renal blood clearance averaged 39.1 mL/minute per 1.73 m2 surface area in eight adult human subjects administered intravenous injections of 35 mg boron/kg (as sodium pentaborate). Urine boron concentrations on the day of administration averaged 1.19 mg/mL (Farr and Konikowski 1963). In rats administered an intravenous infusion of 86 mg boron/kg (as borax) (Tagawa et al. 2000), boron distributed rapidly to the extravascular tissues, giving a steady state volume of distribution of 1.19 L/kg. Excretion of boron was rapid, with 87.6% eliminated in the urine by 2 hours after infusion. Pharmacokinetic analysis of the blood time course data resulted in an estimated elimination rate constant (Kel) of 0.15 hour-1 and a clearance rate of 0.11 L/hour/kg. The elimination half-life was 8.43 hours. Guinea pigs given intra-tympanic doses of 0.2 mL/day of 4% boric acid in saline did not exhibit changes in hearing level (Oztukcan et al. 2008).
3.4.5. Physiologically Based Pharmacokinetic (PBPK)/Pharmacodynamic (PD) Models
Physiologically based pharmacokinetic (PBPK) models use mathematical descriptions of the uptake and disposition of chemical substances to quantitatively describe the relationships among critical biological processes (Krishnan et al. 1994). PBPK models are also called biologically based tissue dosimetry models. PBPK models are increasingly used in risk assessments, primarily to predict the concentration of potentially toxic moieties of a chemical that will be delivered to any given target tissue following various combinations of route, dose level, and test species (Clewell and Andersen 1985). Physiologically based pharmacodynamic (PBPD) models use mathematical descriptions of the dose-response function to quantitatively describe the relationship between target tissue dose and toxic end points.
PBPK/PD models refine our understanding of complex quantitative dose behaviors by helping to delineate and characterize the relationships between: (1) the external/exposure concentration and target tissue dose of the toxic moiety, and (2) the target tissue dose and observed responses (Andersen and Krishnan 1994; Andersen et al. 1987). These models are biologically and mechanistically based and can be used to extrapolate the pharmacokinetic behavior of chemical substances from high to low dose, from route to route, between species, and between subpopulations within a species. The biological basis of PBPK models results in more meaningful extrapolations than those generated with the more conventional use of uncertainty factors.
The PBPK model for a chemical substance is developed in four interconnected steps: (1) model representation, (2) model parameterization, (3) model simulation, and (4) model validation (Krishnan and Andersen 1994). In the early 1990s, validated PBPK models were developed for a number of toxicologically important chemical substances, both volatile and nonvolatile (Krishnan and Andersen 1994; Leung 1993). PBPK models for a particular substance require estimates of the chemical substance-specific physicochemical parameters, and species-specific physiological and biological parameters. The numerical estimates of these model parameters are incorporated within a set of differential and algebraic equations that describe the pharmacokinetic processes. Solving these differential and algebraic equations provides the predictions of tissue dose. Computers then provide process simulations based on these solutions.
The structure and mathematical expressions used in PBPK models significantly simplify the true complexities of biological systems. If the uptake and disposition of the chemical substance(s) are adequately described, however, this simplification is desirable because data are often unavailable for many biological processes. A simplified scheme reduces the magnitude of cumulative uncertainty. The adequacy of the model is, therefore, of great importance, and model validation is essential to the use of PBPK models in risk assessment.
PBPK models improve the pharmacokinetic extrapolations used in risk assessments that identify the maximal (i.e., the safe) levels for human exposure to chemical substances (Andersen and Krishnan 1994). PBPK models provide a scientifically sound means to predict the target tissue dose of chemicals in humans who are exposed to environmental levels (for example, levels that might occur at hazardous waste sites) based on the results of studies where doses were higher or were administered in different species. Figure 3-3 shows a conceptualized representation of a PBPK model.
If PBPK models for boron exist, the overall results and individual models are discussed in this section in terms of their use in risk assessment, tissue dosimetry, and dose, route, and species extrapolations.
There are no PBPK models available for boron.

Figure 3-3
Conceptual Representation of a Physiologically Based Pharmacokinetic (PBPK) Model for a Hypothetical Chemical Substance. Note: This is a conceptual representation of a physiologically based pharmacokinetic (PBPK) model for a hypothetical chemical substance. (more...)
3.5. MECHANISMS OF ACTION
3.5.1. Pharmacokinetic Mechanisms
Absorption. Boron is absorbed across pulmonary tissues into the blood, as seen in workers exposed to borate dusts, who were found to have higher blood and urine boron concentrations at the end of a work shift compared to the beginning of the shift (Culver et al. 1994a). Boron is almost completely absorbed in the gastrointestinal tract, with up to 92 and 95% of ingested dose being recovered in the urine (Dourson et al. 1998). No data are available to indicate whether boron is actively transported or passively diffused across pulmonary or gastrointestinal tissues. Diet may influence the rate of boron absorption in the gut, as higher initial boron levels were found in the urine of humans given boron in an ointment vehicle, compared to administration via a water vehicle (Schou et al. 1984). Boron was found to be minimally absorbed across intact human or animal skin (Draize and Kelley 1959; Wester et al. 1998).
Distribution. Boron is distributed readily to all body tissues. Tissue levels from daily doses were observed to achieve steady-state with plasma in all tissues examined, including neurological and reproductive tissues, with the exception of bone and adipose tissues (Ku et al. 1991. Bone serves as a storage depot for boron, while adipose tissue has a lower affinity for boron than other soft tissues. The mechanism(s) of transport across tissue membranes and into bone are not known. No data were available identifying binding of boron to a carrier protein in the blood or plasma membranes.
Metabolism. Boron is a trace element and is not metabolized in the body. Borates exist in the body as boric acid, the only form of boron recovered in the urine.
Excretion. Excretion of systemically absorbed born is accomplished primarily through renal elimination, with minor fractions excreted in the saliva, sweat, and feces (Jansen et al. 1984a). No data are available regarding the contribution of tubular absorption of boron in the kidney. Glomerular filtration rate is likely the dominant factor in renal elimination of boron. As such, the systemic elimination of boron may be compromised in populations with reduced glomerular filtration rates (Dourson et al. 1998), such as preeclamptic women. This assumption is used in deriving chemical-specific uncertainty factors, which themselves are used for deriving the intermediate-duration oral MRL (Appendix A).
3.5.2. Mechanisms of Toxicity
No studies were available in humans describing a mechanism of toxicity for neurological, gastrointestinal, hepatic, or renal effects observed in case reports of high-dose poisoning incidents. In animals, reproductive and developmental effects have been the most sensitive toxic end points observed.
Although several studies have examined possible mechanisms for reproductive toxicity, the actual toxic mechanism remains unknown. In rats, delayed spermiation (inhibited release of mature sperm) appears to be the hallmark event in testicular toxicity, followed by exfoliation of germ epithelium and atrophy at higher doses (Treinen and Chapin 1991). Leydig and Sertoli cell cultures exposed to 10 mM boric acid did not exhibit reduced responsiveness to induction of testosterone production, but exhibited reduced intracellular cAMP levels following FSH stimulation (Ku et al. 1993b). Further, lactate and pyruvate (the primary energy sources for Sertoli cells) production (possibly from boronation of NAD cofactors [Ku et al. 1993b]) and deoxyribonucleic acid (DNA) synthesis were significantly reduced, suggesting that germ epithelial sloughing and testicular atrophy may result from impaired energy production and mitosis/meiosis in the Sertoli cells (Fail et al. 1998). However, in vivo delayed spermiation appears to occur at lower Sertoli cell exposure levels than disruption of energy production and DNA synthesis, making it difficult to conclude whether reproductive effects are hormonally or metabolically-mediated (Fail et al. 1998; Ku et al. 1993a).
The mechanism of toxicity for developmental effects is also unknown. Fail et al. (1998) suggest that the reduction in fetal body weight (the most sensitive end point observed in rats [Heindel et al. 1992]) may be due to mitotic inhibition observed in viruses, bacteria, insects, yeasts, and animals. Hyperacetylation of embryonic mouse tissues is highly associated with skeletal malformations following exposure to histone deacetylase inhibitors such as valproic acid and trichostatin A. Mice given intraperitoneal doses of 175 mg boron/kg (as boric acid) on gestation day 8 exhibited hyperacetylation of embryonic somites, inhibition of histone deacetylase, and increased incidences of skeletal malformations (fused ribs and vertebra, changes in the typical number of axial segments in different axial districts). The association of these biochemical and morphological effects suggest that boric acid-induced skeletal malformations may result from inhibition of histone deacetylase. Wery et al. (2003) reported a cranial shift in the anterior limits of the hoxa6 and hoxc6 genes in the fetuses of pregnant rats given two gavage doses of 88 mg/boron/kg/day (as boric acid) on gestation day 9. The control of position and development of the fetal vertebrae have been associated with the activity of these genes (see Section 3.3). It is not known whether inhibition of histone deacetylase, as suggested by Fail et al. (1998) is involved with the response of the hoxa6 and hoxc6 genes to in utero exposure to high doses of boron.
3.5.3. Animal-to-Human Extrapolations
Similarities in rodents and humans for boron toxicokinetics and reproductive physiology suggest that the animal toxicity data are relevant for human risk assessment. Animal and rodents studies indicate that boron is readily absorbed (particularly via the oral route), not metabolized, and extensively eliminated via urinary excretion (Section 3.4). Male humans and rodents share similar physiological structures and hormonal control mechanisms of the reproductive system, suggesting a similar target of toxicity for reproductive effects. Similarly, the sequence of fetal developmental steps is similar between the species. Lack of human data, particularly reproductive and developmental data, for effects observed in animal studies introduce uncertainty into the extrapolation of animals data to humans.
3.6. TOXICITIES MEDIATED THROUGH THE NEUROENDOCRINE AXIS
Recently, attention has focused on the potential hazardous effects of certain chemicals on the endocrine system because of the ability of these chemicals to mimic or block endogenous hormones. Chemicals with this type of activity are most commonly referred to as endocrine disruptors. However, appropriate terminology to describe such effects remains controversial. The terminology endocrine disruptors, initially used by Thomas and Colborn (1992), was also used in 1996 when Congress mandated the EPA to develop a screening program for “…certain substances [which] may have an effect produced by a naturally occurring estrogen, or other such endocrine effect[s]…”. To meet this mandate, EPA convened a panel called the Endocrine Disruptors Screening and Testing Advisory Committee (EDSTAC), and in 1998, the EDSTAC completed its deliberations and made recommendations to EPA concerning endocrine disruptors. In 1999, the National Academy of Sciences released a report that referred to these same types of chemicals as hormonally active agents. The terminology endocrine modulators has also been used to convey the fact that effects caused by such chemicals may not necessarily be adverse. Many scientists agree that chemicals with the ability to disrupt or modulate the endocrine system are a potential threat to the health of humans, aquatic animals, and wildlife. However, others think that endocrine-active chemicals do not pose a significant health risk, particularly in view of the fact that hormone mimics exist in the natural environment. Examples of natural hormone mimics are the isoflavinoid phytoestrogens (Adlercreutz 1995; Livingston 1978; Mayr et al. 1992). These chemicals are derived from plants and are similar in structure and action to endogenous estrogen. Although the public health significance and descriptive terminology of substances capable of affecting the endocrine system remains controversial, scientists agree that these chemicals may affect the synthesis, secretion, transport, binding, action, or elimination of natural hormones in the body responsible for maintaining homeostasis, reproduction, development, and/or behavior (EPA 1997). Stated differently, such compounds may cause toxicities that are mediated through the neuroendocrine axis. As a result, these chemicals may play a role in altering, for example, metabolic, sexual, immune, and neurobehavioral function. Such chemicals are also thought to be involved in inducing breast, testicular, and prostate cancers, as well as endometriosis (Berger 1994; Giwercman et al. 1993; Hoel et al. 1992).
No studies were located regarding endocrine disruption in humans after exposure to boron.
While depressed testosterone blood levels was observed in rats fed diets with 61 mg boron/kg/day as boric acid (Treinen and Chapin 1991), GnRH challenge in boron-fed rats (Fail et al. 1998) resulted in LH responses similar to controls and an exacerbated FSH response relative to controls, suggesting that peripheral hormonal changes were not due to neuroendocrine toxicity, but possibly to Leydig and Sertoli cell-specific effects (Fail et al. 1998).
No in vitro studies were located regarding endocrine disruption of boron.
3.7. CHILDREN’S SUSCEPTIBILITY
This section discusses potential health effects from exposures during the period from conception to maturity at 18 years of age in humans, when all biological systems will have fully developed. Potential effects on offspring resulting from exposures of parental germ cells are considered, as well as any indirect effects on the fetus and neonate resulting from maternal exposure during gestation and lactation. Relevant animal and in vitro models are also discussed.
Children are not small adults. They differ from adults in their exposures and may differ in their susceptibility to hazardous chemicals. Children’s unique physiology and behavior can influence the extent of their exposure. Exposures of children are discussed in Section 6.6, Exposures of Children.
Children sometimes differ from adults in their susceptibility to hazardous chemicals, but whether there is a difference depends on the chemical (Guzelian et al. 1992; NRC 1993). Children may be more or less susceptible than adults to health effects, and the relationship may change with developmental age (Guzelian et al. 1992; NRC 1993). Vulnerability often depends on developmental stage. There are critical periods of structural and functional development during both prenatal and postnatal life, and a particular structure or function will be most sensitive to disruption during its critical period(s). Damage may not be evident until a later stage of development. There are often differences in pharmacokinetics and metabolism between children and adults. For example, absorption may be different in neonates because of the immaturity of their gastrointestinal tract and their larger skin surface area in proportion to body weight (Morselli et al. 1980; NRC 1993); the gastrointestinal absorption of lead is greatest in infants and young children (Ziegler et al. 1978). Distribution of xenobiotics may be different; for example, infants have a larger proportion of their bodies as extracellular water, and their brains and livers are proportionately larger than adults (Altman and Dittmer 1974; Fomon 1966; Fomon et al. 1982; Owen and Brozek 1966; Widdowson and Dickerson 1964). The infant also has an immature blood-brain barrier (Adinolfi 1985; Johanson 1980) and probably an immature blood-testis barrier (Setchell and Waites 1975). Many xenobiotic metabolizing enzymes have distinctive developmental patterns. At various stages of growth and development, levels of particular enzymes may be higher or lower than those of adults, and sometimes unique enzymes may exist at particular developmental stages (Komori et al. 1990; Leeder and Kearns 1997; NRC 1993; Vieira et al. 1996). Whether differences in xenobiotic metabolism make the child more or less susceptible also depends on whether the relevant enzymes are involved in activation of the parent compound to its toxic form or in detoxification. There may also be differences in excretion, particularly in newborns who all have a low glomerular filtration rate and have not developed efficient tubular secretion and resorption capacities (Altman and Dittmer 1974; NRC 1993; West et al. 1948). Children and adults may differ in their capacity to repair damage from chemical insults. Children also have a longer remaining lifetime in which to express damage from chemicals; this potential is particularly relevant to cancer.
Certain characteristics of the developing human may increase exposure or susceptibility, whereas others may decrease susceptibility to the same chemical. For example, although infants breathe more air per kilogram of body weight than adults breathe, this difference might be somewhat counterbalanced by their alveoli being less developed, which results in a disproportionately smaller surface area for alveolar absorption (NRC 1993).
Human data of boron toxicity in children and humans due to high-dose exposures are not adequate to identify the presence or lack of children’s susceptibility. Normal boron blood levels in children and infants range from 0 to 1.25 μg/mL (Fisher and Freimuth 1958; O’Sullivan and Taylor 1983). Infants exhibiting adverse effects after ingestion of boric acid in infant formula had boron blood levels (reported as borate) of 20–150 μg/mL, with fatal cases having blood levels of 200–1,600 μg/mL (Wong et al. 1964). Comparatively, adult serum boron level (as boric acid) of 2,320 μg/mL was not associated with significant toxicity (Linden et al. 1986).
No animal studies were located regarding susceptibility of immature animals to boron toxicity. However, oral exposure studies in pregnant animals have identified developmental effects in fetus exposed to boron (as borax or boric acid) in utero. No developmental effects were observed in animals following inhalation exposures. The observed effects from oral exposure include skeletal malformations and cardiovascular abnormalities. The most sensitive effect identified was reduced fetal body weight, which consistently occurred in animals at lower doses than required for skeletal malformations or other effects. The acute- and intermediate-duration oral MRLs are based on reduced fetal body weight in rats and should be protective of children developing in the womb.
3.8. BIOMARKERS OF EXPOSURE AND EFFECT
Biomarkers are broadly defined as indicators signaling events in biologic systems or samples. They have been classified as markers of exposure, markers of effect, and markers of susceptibility (NAS/NRC 1989).
A biomarker of exposure is a xenobiotic substance or its metabolite(s) or the product of an interaction between a xenobiotic agent and some target molecule(s) or cell(s) that is measured within a compartment of an organism (NAS/NRC 1989). The preferred biomarkers of exposure are generally the substance itself, substance-specific metabolites in readily obtainable body fluid(s), or excreta. However, several factors can confound the use and interpretation of biomarkers of exposure. The body burden of a substance may be the result of exposures from more than one source. The substance being measured may be a metabolite of another xenobiotic substance (e.g., high urinary levels of phenol can result from exposure to several different aromatic compounds). Depending on the properties of the substance (e.g., biologic half-life) and environmental conditions (e.g., duration and route of exposure), the substance and all of its metabolites may have left the body by the time samples can be taken. It may be difficult to identify individuals exposed to hazardous substances that are commonly found in body tissues and fluids (e.g., essential mineral nutrients such as copper, zinc, and selenium). Biomarkers of exposure to boron are discussed in Section 3.8.1.
Biomarkers of effect are defined as any measurable biochemical, physiologic, or other alteration within an organism that, depending on magnitude, can be recognized as an established or potential health impairment or disease (NAS/NRC 1989). This definition encompasses biochemical or cellular signals of tissue dysfunction (e.g., increased liver enzyme activity or pathologic changes in female genital epithelial cells), as well as physiologic signs of dysfunction such as increased blood pressure or decreased lung capacity. Note that these markers are not often substance specific. They also may not be directly adverse, but can indicate potential health impairment (e.g., DNA adducts). Biomarkers of effects caused by boron are discussed in Section 3.8.2.
A biomarker of susceptibility is an indicator of an inherent or acquired limitation of an organism’s ability to respond to the challenge of exposure to a specific xenobiotic substance. It can be an intrinsic genetic or other characteristic or a preexisting disease that results in an increase in absorbed dose, a decrease in the biologically effective dose, or a target tissue response. If biomarkers of susceptibility exist, they are discussed in Section 3.10, Populations That Are Unusually Susceptible.
3.8.1. Biomarkers Used to Identify or Quantify Exposure to Boron
Boron in blood and urine can be used as an indicator of exposure to boron. Normal dietary concentrations of boron in the blood of humans range from 0 to 1.25 μg/mL in children and infants (Fisher and Freimuth 1958; O’Sullivan and Taylor 1983). Boron blood levels (reported as borate) of 20–150 μg/mL have been associated with adverse systemic effects in infants who ingested boric acid in infant formula (Wong et al. 1964). Boron concentrations, expressed as borate, reported in fatal cases vary from 200 to 1,600 μg/mL in infants (Wong et al. 1964). In adults, a serum boron level (as boric acid) of 2,320 μg/mL was not associated with significant toxicity (Linden et al. 1986).
Urinary excretion levels can also be useful indicators of elevated total body burden of boron. Concentrations of boron in the normal population range from 0.07 to 0.15 mg/100 mL (Vignec and Ellis 1954) and from 0.004 to 0.66 mg/100 mL (Imbus et al. 1963). In one infant, the urine contained 13.9 mg boron/liter as borax or 1.38 mg boron/mL of boric acid following ingestion of a borax and honey mixture over a period of 12 weeks (Gordon et al. 1973). Virtually complete urinary excretion was indicated by the recovery of 93.9% (over a 96-hour collection period) of a boric acid solution ingested by three volunteers (Jansen et al. 1984a).
Neurological, dermal, gastrointestinal, liver, and kidney effects in humans have been associated with exposure to boron. Studies in animals have demonstrated gonadal injury. Various clinical and biochemical tests exist that may provide useful information on exposure. However, similar effects are caused by a variety of other substances and are, therefore, not specific for boron exposure.
3.8.2. Biomarkers Used to Characterize Effects Caused by Boron
Central nervous system injury, gastrointestinal effects, and skin damage are characteristic manifestations of boron toxicity in humans. Liver and kidneys in humans and testes in animals can also be affected. Various clinical and biochemical changes associated with these effects may be measured to detect the extent of exposure to boron. There is no single biological indicator of boron exposure; consequently, several parameters must be measured including boron levels in urine and blood and biochemical changes for systemic and neurological effects.
Neurological damage has been reported in humans. Neurological effects reported in humans have focused primarily on histopathological alterations. No data were provided on biochemical changes. In animals, testicular atrophy and reduced sperm production have been demonstrated following chronic boron exposure. There are clinical and biochemical tests to detect neurological and gonadal injury, but these are not specific for boron exposure. Sparse data in animals suggest some biochemical changes; for instance, cerebral succinate dehydrogenase was increased in rats after boron exposure. Animal data further demonstrate biochemical alterations following gonadal injury. Dose-dependent reduction in hyaluronidase, sorbitol dehydrogenase, and lactic acid dehydrogenase (isoenzyme-X) were observed in rats following boron exposure.
3.9. INTERACTIONS WITH OTHER CHEMICALS
No studies were located regarding the influence of other chemicals on the toxicity of boron.
3.10. POPULATIONS THAT ARE UNUSUALLY SUSCEPTIBLE
A susceptible population will exhibit a different or enhanced response to boron than will most persons exposed to the same level of boron in the environment. Reasons may include genetic makeup, age, health and nutritional status, and exposure to other toxic substances (e.g., cigarette smoke). These parameters result in reduced detoxification or excretion of boron, or compromised function of organs affected by boron. Populations who are at greater risk due to their unusually high exposure to boron are discussed in Section 6.7, Populations with Potentially High Exposures.
No data were located identifying a population that is unusually susceptible to boron toxicity. Case reports in humans suggest that large variability exists with the human population to the lethal effect of boron. However, there are no data to suggest which segment of the population is more susceptible to boron.
3.11. METHODS FOR REDUCING TOXIC EFFECTS
This section will describe clinical practice and research concerning methods for reducing toxic effects of exposure to boron. However, because some of the treatments discussed may be experimental and unproven, this section should not be used as a guide for treatment of exposures to boron. When specific exposures have occurred, poison control centers and medical toxicologists should be consulted for medical advice. The following texts provide specific information about treatment following exposures to boron:
Ellenhorn MJ, Schonwald S, Ordog G, et al., eds. 1997. Ellenhorn’s medical toxicology. Diagnosis and treatment of human poisoning. 2nd ed. Baltimore, MA: Williams & Wilkins, 1098–1100, 160t, 162t.
Goldfrank LR, Flomenbaum NE, Lewin NA, et al., eds. 2002. Goldfrank’s toxicologic emergencies. 7th ed. New York, NY: McGraw-Hill, 1282, 1289–1290, 1134.
Viccellio P, Bania T, Brent J, et al., eds. 1998. Emergency toxicology. 2nd ed. Philadelphia, PA: Lippincott-Raven, 448–449, 470, 1141.
Human exposure to boron may occur by inhalation, ingestion, or dermal contact (see Chapter 6). Boron in the form of boric acid or borate dust is an upper respiratory tract irritant following inhalation and may also irritate the eyes and skin. Ingestion of boron may cause gastrointestinal, neurological, hepatic, renal, and dermal effects (see Section 3.2). General recommendations for reducing absorption of boron following exposure have included removing the exposed individual from the contaminated area and removing the contaminated clothing. If the eyes and skin were exposed, then they should be flushed with water.
3.11.1. Reducing Peak Absorption Following Exposure
Nausea, vomiting, and diarrhea have been induced by ingestion of boron in humans. Some authors recommend reducing absorption of boron from the gastrointestinal tract by administration of emetics (e.g., syrup of ipecac) and cathartics (e.g., magnesium sulfate) (Stewart and McHugh 1990). Caution should be taken, however, not to induce further damage to the esophageal mucosa or to cause aspiration of the vomit into the lungs during emesis. There is disagreement regarding the efficiency of activated charcoal in preventing absorption of boron from the gastrointestinal tract following oral exposure (Ellenhorn and Barceloux 1988; Stewart and McHugh 1990). It has been suggested that activated charcoal be administered following gastric evacuation, but its effectiveness has not been established (Ellenhorn and Barceloux 1988). Administration of intravenous fluids may be required if severe dehydration or shock develop and local skin care may be necessary if skin desquamation occurs (Stewart and McHugh 1990). In addition, the treatment of boron poisoning may require a control for convulsions.
3.11.2. Reducing Body Burden
Elemental boron is not metabolized (see Section 3.4). Studies in volunteers indicated that most of the administered dose is excreted in the urine within few days (Jansen et al. 1984a). Saline diuresis has been suggested to enhance urinary excretion of boron (Goldfrank et al. 1990). Exchange transfusions, peritoneal dialysis, or hemodialysis may be employed to lower plasma boron levels following either acute or chronic intoxication. There are indications that hemodialysis is the most effective of these procedures (Goldfrank et al. 1990; Naderi and Palmer 2006; Stewart and McHugh 1990).
3.11.3. Interfering with the Mechanism of Action for Toxic Effects
No studies were available to support measures to interfere with the mechanism of action for boron once it has been absorbed.
3.12. ADEQUACY OF THE DATABASE
Section 104(I)(5) of CERCLA, as amended, directs the Administrator of ATSDR (in consultation with the Administrator of EPA and agencies and programs of the Public Health Service) to assess whether adequate information on the health effects of boron is available. Where adequate information is not available, ATSDR, in conjunction with the National Toxicology Program (NTP), is required to assure the initiation of a program of research designed to determine the health effects (and techniques for developing methods to determine such health effects) of boron.
The following categories of possible data needs have been identified by a joint team of scientists from ATSDR, NTP, and EPA. They are defined as substance-specific informational needs that if met would reduce the uncertainties of human health assessment. This definition should not be interpreted to mean that all data needs discussed in this section must be filled. In the future, the identified data needs will be evaluated and prioritized, and a substance-specific research agenda will be proposed.
3.12.1. Existing Information on Health Effects of Boron
The existing data on health effects of inhalation, oral, and dermal exposure of humans and animals to boron are summarized in Figure 3-4. The purpose of this figure is to illustrate the existing information concerning the health effects of boron. Each dot in the figure indicates that one or more studies provide information associated with that particular effect. The dot does not necessarily imply anything about the quality of the study or studies, nor should missing information in this figure be interpreted as a “data need”. A data need, as defined in ATSDR’s Decision Guide for Identifying Substance-Specific Data Needs Related to Toxicological Profiles (Agency for Toxic Substances and Disease Registry 1989), is substance-specific information necessary to conduct comprehensive public health assessments. Generally, ATSDR defines a data gap more broadly as any substance-specific information missing from the scientific literature.
Most of the information concerning health effects of oral exposure to boron in humans is found in case reports of accidental acute and intermediate ingestion of boron. Controlled-exposure studies of volunteers and cross-sectional surveys of borate mining and production workers have identified acute upper respiratory and ocular irritation as an effect of concern from acute inhalation exposure. Epidemiology studies of intermediate- to chronic-duration exposures (involving repeated occupational exposure to dusts of borates or repeated exposure to boron in drinking water) have not clearly identified a toxic effect in humans, but have found no associations between boron exposure and impaired pulmonary function or impaired fertility. Information on acute dermal exposure exists, but none was found on effects after intermediate- and chronic-duration exposure.
In animals, information exists on health effects following acute, intermediate, and chronic oral exposure to boric acid or borax. Comprehensive acute toxicity studies involving inhalation exposure to boron compounds are not available, with the exception of a study of mice exposed by inhalation to diborane gas for 2 or 4 weeks. Diborane gas is expected to have a very short half-life in the environment because of its reactivity. Thus, it is not expected to be a significant environmental toxicant, except in workplaces where it might be used and accidentally released. Health effects have been examined in rats and dogs exposed by inhalation to boric oxide aerosols for intermediate durations. Studies of health effects in animals exposed by inhalation to boron compounds for chronic durations are not available. Health effects have been observed in animals following acute dermal exposure, but no toxicity studies of animals dermally exposed for intermediate and chronic durations are available.

Figure 3-4
Existing Information on Health Effects of Boron.
3.12.2. Identification of Data Needs
Acute-Duration Exposure. Associations between acute inhalation exposure to borate dusts and increased prevalence of self-reported symptoms of irritation of the eyes, nose, and throat of volunteers under controlled exposure conditions (Cain et al. 2004, 2008) form the basis of an acute-duration inhalation MRL. These data are supported by self-reported data for mild upper respiratory tract irritation in workers under workplace conditions (Garabrant et al. 1984, 1985; Hu et al. 1992; Wegman et al. 1991, 1994). Dose-response relationships for mild respiratory and ocular irritation from studies of animals exposed by inhalation for acute durations are not available.
Case reports of acute oral poisonings with boric acid do not clearly identify toxicity targets and dose-response relationships in humans, but reported effects in fatal cases include degenerative changes in the liver, kidney, and brain (Chao et al. 1991a, 1991b; Wong et al. 1964). In animal studies, acute-duration oral exposure to boric acid or borates has been associated with effects on the male reproductive organs (e.g., decreased testicular weight and altered sperm morphology) in Wistar rats exposed to gavage boric acid doses of 88 mg boron/kg/day for 2 weeks (Fukuda et al. 2000; Kudo et al. 2000) and developmentally toxic effects (including reduced fetal weight and increased skeletal variations or malformations) in CD-1 mice exposed during gestation to boric acid doses as low as 70 mg boron/kg (2 times/day) (Cherrington and Chernoff 2002) and in New Zealand rabbits exposed during gestation (days 6–19) to doses of 44 mg boron/kg/day (Price et al. 1996b). A NOAEL of 22 mg boron/kg/day for developmental effects in rabbits (Price et al. 1996b) serves as the basis of the acute-duration oral MRL for boron.
Dermal/ocular effects have been associated with dermal exposure in humans (Beckett et al. 2001) and animals (Wilding et al. 1959). The irritation effects observed in animals may have been due to the exothermic rehydration reaction of the anhydride boron oxide.
No further acute-duration health effect studies are recommended at this time.
Intermediate-Duration Exposure. No studies are available that establish associations between intermediate-duration oral exposures to boron compounds and health effects in humans. Case reports of intermediate-duration exposure to humans are limited to reports on infants exhibiting neurological effects after ingestion of high levels of boron as boric acid. Seizure disorders occurred during oral exposures of infants to borax lasting 4–12 weeks, with cumulative approximate doses of 12–120 g (Gordon et al. 1973; O’Sullivan and Taylor 1983). No associations were found between elevated levels of borates in drinking water and fertility rates in surveys of Turkish subpopulations expected to have intermediate- to chronic-duration exposures to boron (Sayli 1998a, 1998b, 2003; Sayli et al. 1998). Likewise, cross-sectional surveys of boron mining and processing workers in California (Whorton et al. 1994) and China (Chang et al. 2006; Liu et al. 2006a, 2006b) failed to find associations between boron exposure (which may have included oral exposure to boron) and adverse effects on indices of fertility. Robbins et al. (2008) associated a shift in Y:X sperm and male:female offspring ratios with boron exposure; however, this study had multiple confounders limiting the interpretation of reported results.
Studies in animals indicate that reproductive and developmental effects are the most sensitive effects associated with intermediate-duration oral exposures to boric acid or borates. Testicular atrophy and histopathology, sperm abnormalities, and reduced sperm production have been observed in mice, rats and dogs after intermediate-duration ingestion of doses ≥26 mg boron/kg/day as boric acid (Dieter 1994; Dixon et al. 1976, 1979; Fail et al. 1991; Fukuda et al. 2000; Harris et al. 1992; Ku et al. 1993a; Kudo et al. 2000; Lee et al. 1978; NTP 1987; Nusier and Bataineh 2005; Seal and Weeth 1980; Weir and Fisher 1972; Yoshizaki et al. 1999). Complete sterility was observed in Sprague-Dawley rats fed boric acid or borax in the diet (101 and 116 mg boron/kg/day for males and females, respectively) for 14 weeks before mating; sterility was associated with a lack of viable sperm in atrophied testes in males and decreased ovulation in females (Weir and Fisher 1972). No pregnancies occurred, when female rats exposed to this dose level were mated with non-exposed male rats. At lower exposure levels (10 or 30 mg boron/kg/day for males and 12 or 35 mg boron/kg/day for females), no exposure-related adverse effects were found on overall fertility indices in three successive generations (Weir and Fisher 1972). Developmental effects (including decreased fetal weight, increased incidence of skeletal variations and malformations, and increased resorptions) have been observed in offspring of rat and mouse dams exposed to 13–79 mg boron/kg/day as boric acid during gestation (Heindel et al. 1992, 1994; Price et al. 1996a). Multiple developmental end point data from the rat studies by Heindel et al. (1992) and Price et al. (1996a) were pooled and subjected to benchmark dose analyses (Allen et al. 1996) to derive a benchmark-dose point of departure of 10.3 mg boron/kg/day for the intermediate-duration oral MRL for boron.
Studies of Chinese borate workers have evaluated specific clinical end points of semen quality, but do not provide adequate estimation of magnitude or duration of boron exposure. Additional cross-sectional or prospective surveys of reproductive health end points in populations exposed to elevated levels of boron compounds in drinking water may help to better identify reproductive toxicity and developmental toxicity as potential health effects in humans with elevated oral exposure to boron for intermediate or chronic durations.
Increased frequencies of symptoms of acute upper respiratory and ocular irritation have been reported in workers exposed repeatedly by inhalation to boron oxide and borate dusts at average concentrations of 1.8 and 3.1 mg boron/m3, and employed in the borax industry for a mean duration of 11.4 years (SD 8.1 years), compared with a reference population (Garabrant et al. 1984, 1985); however, it is uncertain if these acute symptoms were due to acute or repeated exposure. Later studies of a different design indicated that acute irritation symptoms in these workers are due to acute exposures, and that pulmonary function variables (e.g., FEV1) were not significantly influenced by exposure over a 7-year period of employment (Hu et al. 1992; Wegman et al. 1994). These results are adequate to form the basis of an acute inhalation MRL for boron, but do not clearly indicate whether the dose-response relationships for acute boron-induced upper respiratory and ocular irritation symptoms are changed with intermediate or chronic durations of exposure. Because exposure-related effects on pulmonary function variables were not evident during a 7-year period between the studies of Garabrant et al. (1984, 1985) and Wegman et al. (1994), the results provide some confidence that the acute-duration inhalation MRL may by protective for intermediate- and chronic-duration exposures.
Intermediate-duration inhalation exposure studies in animals are restricted to a series of studies in which albino rats were exposed to boric oxide aerosols for 6 hours/day, 5 days/week for 10 weeks at an average concentration of 73 mg boron/m3 (n=20 rats), 12 weeks at 27 mg boron/m3 (n=4), or 24 weeks at 12 mg boron/m3 (n=70) (Wilding et al. 1959). Histopathologic examination of a comprehensive set of tissues (lung, trachea, pancreas, thyroid, adrenal, eye, femur, rib, bone marrow, liver, heart, spleen, kidney, brain, stomach, intestine, ovary, testis, lymph node, and muscle) in exposed and control rats revealed no exposure-related lesions, with the exception that some rats exposed to the highest concentration (73 mg boron/m3) showed a reddish nasal exudate. Although dogs were included in this study (three dogs were exposed to 9 mg boron/m3 for 23 weeks), end points were restricted to clinical signs of toxicity, body weight, hematological end points, and sulfobromophthalein retention, a measure of liver function (Wilding et al. 1959). No exposure-related effects on these end points were found in exposed dogs, compared with controls. Because the NOAELs identified in the rat and dog studies were higher than concentrations associated with acute respiratory and ocular irritation in humans acutely exposed to boron (Cain et al. 2004; Wegman et al. 1994), the intermediate-duration inhalation database was considered inadequate for derivation of an MRL.
Additional prospective health evaluations of respiratory function and reproductive and developmental variables in borate mining and processing workers with intermediate- and chronic-duration exposures may help to better identify impaired respiratory function, impaired reproductive performance, and developmental effects as critical effects in humans from intermediate- or chronic duration exposure to boron compounds.
Chronic-Duration Exposure and Cancer. No studies are available that establish associations between chronic-duration oral exposures to boron compounds and noncancer or cancer effects in humans. As discussed in the previous section, no associations were found between exposure to borates or boric oxide and indices of fertility in surveys of Turkish subpopulations expected to have intermediate- to chronic-duration oral exposures to boron (Sayli 1998a, 1998b, 2003; Sayli et al. 1998), or in surveys of boron mining and processing workers in California (Whorton et al. 1994) and China (Chang et al. 2006; Liu et al. 2006a, 2006b). Chronic-duration oral toxicity studies in animals include 2-year toxicity studies in Sprague-Dawley rats and beagle dogs exposed to boric acid or borax in the diet (Weir and Fisher 1972), and a 2-year toxicity and cancer bioassay in B6C3F1 mice (Dieter 1994; NTP 1987). Histopathological examination of a comprehensive set of tissues from exposed and control rats and dogs (brain, pituitary, thyroids, lung, heart, liver spleen, kidneys, adrenals, pancreas, intestines, urinary bladder testes, ovary (rat only), bone, and bone marrow) found no exposure-related non-neoplastic or neoplastic lesions at dose levels up to 6.8 mg boron/kg/day in dogs or 81 mg boron/kg/day in rats, with the exception that rats exposed to 81 mg boron/kg/day, but not 24 mg boron/kg/day, showed testicular atrophy, decreased growth, decreased packed blood cell and hemoglobin levels, and scaly tails and desquamated footpad skin (Weir and Fisher 1972). In the mouse bioassay, no cancer responses were observed at dose levels up to 201 mg boron/kg/day (Dieter 1994; NTP 1987). Noncancer effects in mice included testicular atrophy and interstitial hyperplasia, lung hemorrhage, and 10–17% decreased body weight at 201 mg boron/kg/day and splenic hematopoiesis and chronic hepatic inflammation and coagulative necrosis at 79 and 201 mg boron/kg/day, the only dose levels included in the study.
Although data are sufficient to develop a chronic oral MRL, a value was not derived. Because intermediate-duration LOAELs for developmental toxicity in rats (13.6 and 13 mg boron/kg/day [Heindel et al. 1992; Price et al. 1996a, 1998]) were lower than the NOAEL (24 mg boron/kg/day) for testicular atrophy and other non-cancer effects in chronically exposed rats (Weir and Fisher 1972), the intermediate MRL, which is based on developmental toxicity, should be protective against reproductive toxicity following chronic exposure. In mice, the intermediate-duration oral NOAEL (43.4 mg boron/kg/day) and LOAEL (79 mg boron/kg/day) for developmental toxicity in CD-1 mice (Heindel et al. 1992) were lower than the chronic-duration LOAEL for testicular atrophy and decreased body weight in B6C3F1 mice (201 mg boron/kg/day) and overlapped with the LOAEL (79 mg boron/kg/day) for splenic hematopoiesis and chronic hepatic inflammation and necrosis (Dieter 1994; NTP 1987). Additional studies may be useful in assessing the level of confidence in existing intermediate-duration NOAEL and LOAEL values for developmental toxicity in rats and mice. Additional chronic-duration oral exposure studies in animals do not seem necessary at this time.
As discussed in the previous section, symptoms of acute upper respiratory and ocular irritation have been reported in workers employed in the borax industry for an average of >10 years, but pulmonary function variables were not significantly influenced by exposure over a 7-year period of employment (Garabrant et al. 1984, 1985; Hu et al. 1992; Wegman et al. 1994). These results are the basis of an acute inhalation MRL for boron, but do not clearly indicate whether the dose-response relationships for acute boron-induced upper respiratory and ocular irritation effects are changed with intermediate or chronic durations of exposure. Because exposure-related effects on pulmonary function variables were not evident during a 7-year period of employment, the results provide some confidence that the acute-duration inhalation MRL may by protective for intermediate- and chronic-duration exposures. Additional prospective health evaluations of respiratory function and reproductive and developmental variables in borate mining and processing workers with intermediate- and chronic-duration exposures may help to better identify impaired respiratory function, impaired reproductive performance, and developmental effects as critical effects in humans from intermediate- or chronic-duration inhalation exposure to boron compounds.
The available chronic oral bioassays in rats and dogs exposed to boric acid or borax (Weir and Fisher 1972) and mice exposed to boric acid (Dieter 1994; NTP 1987) found no evidence for exposure-related cancer. Although no epidemiological studies have been conducted to examine possible associations between boron exposure and cancer, the results from the animal studies provide no strong impetus to conduct such studies.
Genotoxicity. No in vivo human data were located. Bacterial and limited mammalian assays were negative for mutagenicity (Benson et al. 1984; Landolph 1985; Demerec et al. 1951; Haworth et al. 1983; NTP 1987) or cell transformation (Landolph 1985). However, the in vivo animal study of Wery et al. (2003) reported specific alterations in gene expression in embryos at critical points of development that may be associated with skeletal malformations seen in several animal species. Thus, additional in vivo studies may be useful to establish whether direct genetic changes or indirect factors are responsible for the observed changes in gene expression associated with observed skeletal effects in animals.
Reproductive Toxicity. No associations were found between exposure to borates or boric oxide and indices of fertility in surveys of Turkish subpopulations expected to have intermediate- to chronic-duration oral exposures to boron (Sayli 1998a, 1998b, 2003; Sayli et al. 1998), or in surveys of boron mining and processing workers in California (Whorton et al. 1994) and China (Chang et al. 2006). Studies of Chinese borate workers and populations living in environments with high boron exposure did not find effects on semen quality (Liu et al. 2006a, 2006b); however, adequate estimation magnitude or duration of boron exposure for each study group was not available. One study of Chinese borate workers associated high boron exposure with a shift toward males in the ratio the sex of offspring (Robbins et al. 2008); however, several confounding factors in this study limit the interpretation of study data. Effects on the male and female reproductive organs have been clearly demonstrated in rats orally exposed to boric acid or borax; supporting evidence for effects on the male reproductive organs from oral exposure to boron has been reported in mice and dogs. Testicular atrophy and histopathology, sperm abnormalities, and reduced sperm production have been observed in mice, rats, and dogs after intermediate-duration ingestion of doses ≥26 mg boron/kg/day as boric acid (Dieter 1994; Dixon et al. 1976, 1979; Fail et al. 1991; Fukuda et al. 2000; Harris et al. 1992; Ku et al. 1993a; Kudo et al. 2000; Lee et al. 1978; NTP 1987; Nusier and Bataineh 2005; Seal and Weeth 1980; Weir and Fisher 1972; Yoshizaki et al. 1999). Complete sterility was observed in male and female Sprague-Dawley rats fed boric acid or borax in the diet for 14 weeks before mating at 101 and 116 mg boron/kg/day for males and females, respectively (Weir and Fisher 1972). Sterility was associated with a lack of viable sperm in males and decreased ovulation in females, and no pregnancies occurred when female rats exposed to this dose level were mated with non-exposed male rats. At lower exposure levels (10 or 30 mg boron/kg/day for males and 12 or 35 mg boron/kg/day for females), no exposure-related adverse effects were found on fertility indices in three successive generations (Weir and Fisher 1972). With chronic-duration oral exposure, testicular atrophy occurred at 201, but not 79, mg boron/kg/day in B6C3F1 mice (Dieter 1994; NTP 1987) and at 81, but not 24, mg boron/kg/day in Sprague-Dawley rats (Weir and Fisher 1972). No testicular atrophy was found in dogs exposed for 2 years to dietary doses of boric acid or borax at levels up to 6.8 mg boron/kg/day (Weir and Fisher 1972). In the chronic-duration oral exposure studies, no histologic effects on the ovaries were found in rats (Weir and Fisher 1972) or mice (Dieter 1994; NTP 1987), but the ovaries of chronically exposed female dogs were not examined (Weir and Fisher 1972).
With inhalation exposure to boron compounds, reproductive effects do not appear to be an effect of concern. In intermediate-duration inhalation studies with rats, no histologic effects on the testes or ovaries were found in albino rats exposed to boric oxide aerosols for 6 hours/day, 5 days/week for 10 weeks at an average concentration of 73 mg boron/m3, 12 weeks at 27 mg boron/m3, or 24 weeks at 12 mg boron/m3 (Wilding et al. 1959).
Additional prospective health evaluations of reproductive variables in borate mining and processing workers with intermediate- and chronic-duration exposures may help to better identify impaired reproductive performance as a critical effects in humans from intermediate- or chronic-duration inhalation exposure to boron compounds.
Developmental Toxicity. No studies were found on the developmental effects of boron and compounds in humans following inhalation, oral, or dermal exposure. In acute-duration oral exposure animal studies, developmentally toxic effects (including reduced fetal weight and increased skeletal variations or malformations) have been reported in CD-1 mice exposed during gestation to boric acid doses as low as 70 mg boron/kg (2 times/day) (Cherrington and Chernoff 2002) and in New Zealand rabbits exposed during gestation (days 6–19) to doses of 44 mg boron/kg/day (Price et al. 1996b). Developmental effects (including decreased fetal weight, increased incidence of skeletal variations and malformations, and increased resorptions) have been observed in offspring of rat and mouse dams exposed to 13–79 mg boron/kg/day as boric acid during gestation for intermediate durations (Heindel et al. 1992, 1994; Price et al. 1996a). Developmental effects observed in these animal studies are the critical effects for the acute- and intermediate-duration oral MRLs for boron.
Developmental toxicity studies in animals exposed to boron compounds by inhalation are not available. Such studies may be useful to determine if developmental effects are a critical effect from inhalation exposure to boron compounds.
Immunotoxicity. No studies were found in humans or animals on the effects of boron on the immune system by any route of exposure. Results of chronic studies do not suggest that the immune system is a potential target for boron toxicity. Additional studies are not needed at this time.
Neurotoxicity. Case reports in humans, primarily infants, indicate that neurological effects occur after ingestion of boron at high dose levels (Wong et al. 1964). Degenerative changes in brain cells, perivascular hemorrhage, and intravascular thrombosis have been reported in fatal case reports in infants, but neurochemical or neurophysiological changes have not been reported (Settimi et al. 1982; Wong et al. 1964). No studies are available on neurotoxic effects of boron following inhalation or dermal exposure in humans. Animal data are limited to increased brain enzyme activity (Settimi et al. 1982), but no histopathological data are available. Since data on effects are limited primarily to acute oral exposures at high dose levels, additional studies in animals evaluating other dose levels and exposure routes and durations may be useful in evaluating potential risk to humans who may be exposed to low levels of boron compounds near hazardous waste sites.
Epidemiological and Human Dosimetry Studies. Information exists on the adverse health effects of boron compounds in humans. Symptoms of acute upper respiratory and ocular irritation have been reported in workers employed in the borax industry for an average of >10 years, but pulmonary function variables were not significantly influenced by exposure over a 7-year period of employment (Garabrant et al. 1984, 1985; Hu et al. 1992; Wegman et al. 1991, 1994). More precise quantitation of the irritation potential of airborne boron compounds comes from controlled exposure studies of human volunteers (Cain et al. 2004, 2008). No associations were found between exposure to borates or boric oxide and indices of fertility in surveys of Turkish subpopulations expected to have intermediate- to chronic-duration oral exposures to boron (Sayli 1998a, 1998b, 2003; Sayli et al. 1998), or in surveys of boron mining and processing workers in California (Whorton et al. 1994) and China (Chang et al. 2006). Studies of Chinese borate workers and populations living in environments with high boron exposure did not find effects on semen quality (Liu et al. 2006a, 2006b). Other human studies involve case reports of accidental or intentional ingestion of large quantities of boron compounds (Wong et al. 1964; Litovitz et al. 1988; Locatelli et al. 1987). The case report studies identified key health effects (gastrointestinal, respiratory, renal, neurological, hepatic) associated with boron exposure (Wong et al. 1964).
Results from animal studies indicate that the testes and developing fetus as the most sensitive targets following acute, intermediate, or chronic oral exposure to boron. Epidemiological studies that look for associations between boron exposure and reproductive and/or developmental toxicity end points would be useful to better identify impaired reproductive performance and developmental effects as critical effects in humans from intermediate- or chronic-duration exposure to boron compounds.
Biomarkers of Exposure and Effect.
Exposure. Blood and urine borate concentrations may be useful biomarkers of exposure (Jansen et al. 1984a; Litovitz et al. 1988). Normal dietary concentrations of boron in the blood of humans range from 0 to 1.25 μg/mL in children and infants (Fisher and Freimuth 1958; O’Sullivan and Taylor 1983). Elevated blood levels of boron have been reported in cases of acute ingestion of boric acid (Linden et al. 1986; Wong et al. 1964). Urinary concentrations of boron in the normal population range from 0.07 to 0.15 mg/100 mL (Vignec and Ellis 1954) and from 0.004 to 0.66 mg/100 mL (Imbus et al. 1963), whereas elevated concentrations have been measured in humans orally exposed to borax or boric acid (Gordon et al. 1973; Jansen et al. 1984a). Additional studies of exposure levels and blood or urinary levels of boron in borate mining and production workers may help to better characterize quantitative relationships between occupational exposure levels and blood or urinary levels of boron.
Effect. The most clearly identified effects in humans exposed to boron compounds are acute respiratory and ocular irritation from acute inhalation exposure to boron compounds. Several other types of effects, including degenerative changes in brain cells, gastrointestinal irritation, degenerative liver or kidney lesions, and skin changes (erythema involving palms, soles, and buttocks), have been observed in some, but not all, cases of acute- or intermediate-duration oral poisoning with boric acid or borax. In orally exposed animals, effects include testicular atrophy in males and decreased ovulation in females, and developmental effects (fetal body weight deficits and skeletal malformations) with gestational exposure. None of these effects, however, are necessarily specific to boron. To date, a specific biomarker of effect for boron has not been developed for humans or animals.
Absorption, Distribution, Metabolism, and Excretion. Limited quantitative information is available on the absorption, distribution, metabolism, and elimination of boron compounds following oral (Dourson et al. 1998; Ku et al. 1991; Moseman 1994; Treinen and Chapin 1991), inhalation (Wilding et al. 1959), and dermal (Draize and Kelley 1959; Wester et al. 1998) exposure. Since data on toxicokinetics of boron are limited, additional studies are needed by all routes of exposure that will provide data on absorption rates, amount and rate of accumulation in various tissues, and clearance rates. Limited data from oral and dermal studies suggest that boron is primarily excreted in urine. Since boron can deposit in the upper respiratory tract, additional excretion studies by this route would be useful in determining if excretion patterns are similar across all routes of exposure.
Comparative Toxicokinetics. Existing evidence from human and animal studies do not indicate whether or not boron compounds affect the same target tissues. Animal studies indicate the testes as a target tissue (Dieter 1994; Dixon et al. 1979; Fukuda et al. 2000; Ku et al. 1993a; Kudo et al. 2000; Lee et al. 1978; NTP 1990; Price et al. 1998; Seal and Weeth 1980; Weir and Fisher 1972). However, studies of Chinese borate workers found no effect of boron exposure on multiple indices of semen quality (Lui et al. 2006a, 2006b). Data exist on excretion of boron compounds. Based on excretion studies, boron compounds are absorbed by the gastrointestinal tract. There are no available quantitative toxicokinetics data on absorption, distribution, and metabolism. Additional toxicokinetics studies may provide a better basis for extrapolation of animal data to human exposure risk.
Methods for Reducing Toxic Effects. Methods for the mitigation of acute effects of boron poisoning include prevention of absorption of boron from the gastrointestinal tract and standard procedures used to prevent convulsions, severe dehydration, or shock (Stewart and McHugh 1990). Saline diuresis, exchange transfusions, peritoneal dialysis, or hemodialysis may be employed to enhance removal of absorbed boron from the body (Goldfrank et al. 1990; Stewart and McHugh 1990). No additional information was located concerning mitigation of effects of lower-level or longer-term exposure to boron. Further information on techniques to mitigate such effects may be useful in determining the safety and effectiveness of possible methods for treating boron-exposed populations in the vicinity of hazardous waste sites.
Children’s Susceptibility. Data needs relating to both prenatal and childhood exposures, and developmental effects expressed either prenatally or during childhood, are discussed in detail in the Developmental Toxicity subsection above.
The comparative susceptibility of children to the acute respiratory and ocular irritation potential of aerosols of boric acid or other boron compounds has not been examined. Results from animal studies indicate that developmental effects associated with gestational exposure are the most sensitive effects associated with acute- or intermediate-duration oral exposures. Degenerative changes in the male (e.g., testicular atrophy and altered spermiation) and female (decreased ovulation) have also been identified as a sensitive effect from boron, but no studies were located that examined the relative susceptibility of young animals (or children) to these effects.
Child health data needs relating to exposure are discussed in Section 6.8.1, Identification of Data Needs: Exposures of Children.
3.12.3. Ongoing Studies
Wendie A. Robbins of the University of California Los Angeles is being funded by the NIOSH to perform an epidemiologic study investigating the relationship between workplace exposure to boron-containing compounds (including boric acid, borax) and adverse male reproductive effects. Two published human studies on reproductive effects of occupational boron exposure found no effect on fertility or development, while one study reported positive testicular atrophy and sterility and another associated a shift in the ratio of offspring sex with boron exposure. All of these studies have been criticized for inadequacies in study design exposure assessment. The specific aims of this research are to: (1) describe the relationship between boron exposure and direct measures of toxicity on male reproduction, (2) describe the relationship between boron exposure and indirect measures of toxicity on male reproduction, and (3) describe the relationship between workplace, environmental, and dietary sources of boron with biomarkers of exposure and reproductive effect. The goal is to contribute critical information on the exposure level at which boron causes adverse effects on human male reproduction.
- INTRODUCTION
- DISCUSSION OF HEALTH EFFECTS BY ROUTE OF EXPOSURE
- GENOTOXICITY
- TOXICOKINETICS
- MECHANISMS OF ACTION
- TOXICITIES MEDIATED THROUGH THE NEUROENDOCRINE AXIS
- CHILDREN’S SUSCEPTIBILITY
- BIOMARKERS OF EXPOSURE AND EFFECT
- INTERACTIONS WITH OTHER CHEMICALS
- POPULATIONS THAT ARE UNUSUALLY SUSCEPTIBLE
- METHODS FOR REDUCING TOXIC EFFECTS
- ADEQUACY OF THE DATABASE
- HEALTH EFFECTS - Toxicological Profile for BoronHEALTH EFFECTS - Toxicological Profile for Boron
Your browsing activity is empty.
Activity recording is turned off.
See more...