NCBI Bookshelf. A service of the National Library of Medicine, National Institutes of Health.
Cappellini MD, Farmakis D, Porter J, et al., editors. 2021 Guidelines: For the Management of Transfusion Dependent Thalassaemia (TDT) [Internet]. 4th edition. Nicosia (Cyprus): Thalassaemia International Federation; 2023.
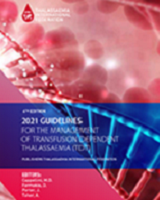
2021 Guidelines: For the Management of Transfusion Dependent Thalassaemia (TDT) [Internet]. 4th edition.
Show detailsHaemoglobin Types
Oxygen is transported from the lungs to the tissues by a highly specialised protein molecule, haemoglobin, which is located in the red cells of the blood. Each red blood cell contains approximately 300 million molecules of this protein, totalling about 30 picograms in weight per cell. Each molecule of haemoglobin is formed by two pairs of identical sub-units, the globin chains. These chains are named with letters of the Greek alphabet and belong to two groups: the α globin cluster, comprising the ζ and α globin chains, and the β globin cluster, comprising the globin chains ε, γ, β and δ. The globin chains appear sequentially during ontogeny and, after pairing, form the following four major types of haemoglobin:
The process of different haemoglobin species being produced and stopping production at certain periods of human development is known as ‘haemoglobin switching’ as shown in Figure 1. Under normal conditions, the red cells of the adult human contain approximately 97-98% of Hb A, 2-3% of Hb A2 and traces of Hb F.

Figure 1
Globin synthesis at various stages of embryonic, fetal and adult erythroid.
Globin genes and globin synthesis
The globin chains have an extremely precise structure, ensuring their prompt loading with oxygen in the lung alveoli and the controlled gradual delivery of the gas into the tissues. The precise structure of the globin chains is coded by genes contained in the DNA of chromosomes 16 (the α gene cluster) and 11 (the β gene cluster). Flanking the structural genes, i.e. in front (on the 5’ side of the DNA sequence, ‘upstream’) and following them (on the 3’ side of the DNA sequence, ‘downstream’), lie several nucleotide sequences which have a ‘regulatory’ role, i.e. they determine which gene is to be turned on and which is turned off, as well as how efficient its expression will be. In adult life, most of the globin synthesis occurs in erythroblasts in the bone marrow. Globin chains must have the correct structure and be trimmed in such a way that the number of α chains precisely matches that of the β chains. When the above conditions are not met, the result is a complete or partial defect in one or both ‘allelic’ globin genes.
The thalassaemias
The term ‘thalassaemia’ refers to a group of blood diseases characterised by decreased or absent synthesis of one or more of the normal globin chains. According to the chain whose synthesis is impaired, the thalassaemias are called α, β, γ, δ, δβ or εγδβ thalassaemias. Most thalassaemias are inherited as recessive traits. These primary quantitative defects are no longer rigidly differentiated by the structural variants produced at reduced rate (such as Hb E and Hb Lepore). From a clinical point of view, the most relevant types are α and β thalassaemias, resulting from the decrease of one of the two types of polypeptide chains (α or β) that form the normal adult human haemoglobin molecule (Hb A, α2β2).
The present book mainly addresses the latter group of thalassaemias, which constitute a major problem in the countries around the Mediterranean Sea, the Middle East and Trans-Caucasus, India and the Far East. The highest carrier frequency of β thalassaemia is reported in the Maldives (18%), Cyprus (14%), Sardinia (10.3%) and Southeast Asia (3-5%). The high gene frequency in these regions is most likely related to the selective pressure from Plasmodium falciparum malaria. However, population migration and intermarriage between different ethnic groups has introduced thalassaemia in almost every country of the world, including Northern Europe, North and South America, the Caribbean and Australia. As for α thalassaemia, it is commonly encountered in Southeast Asia and China with up to 40% of the regional population being carriers, and less commonly in India, the Gulf region, the Middle East, Greece, Italy and Northern Europe. In Southeast Asia the frequency is so high as to cause a major public health problem because of the elevated number of patients with severe Hb H disease and of fetuses with Hb Bart’s hydrops fetalis.
As autosomal recessive conditions, heterozygotes of either α or β thalassaemia are usually asymptomatic and require no treatment. Homozygotes and compound heterozygotes for thalassaemia alleles result in thalassaemia syndromes or diseases. In addition, interactions of thalassaemia and corresponding haemoglobinopathies e.g. Hb E, Hb C or Hb S with β thalassaemia or Hb Constant Spring (Hb CS) with α thalassaemia also give rise to various thalassaemia syndromes. Currently, based on their clinical severity and transfusion requirement, these thalassaemia syndromes can be classified phenotypically into two main groups; 1. Transfusion-Dependent Thalassaemias (TDTs) and 2. Non-Transfusion-Dependent Thalassaemias (NTDTs) as shown in Figure 2.

Figure 2
Phenotypic classification of thalassaemia syndromes based on clinical severity and transfusion requirement.
The TDTs require regular blood transfusion to survive and without adequate transfusion support, they would suffer several complications and a short life span. This category includes patients with β thalassaemia major, severe Hb E/β thalassaemia, transfusion -dependent Hb H disease or Hb H hydrops fetalis and surviving Hb Bart’s hydrops fetalis. This TDT group is the main focus of this present clinical practice guideline (CPG). The groups of NTDT patients include β thalassaemia intermedia, Hb E/β thalassaemia and Hb H disease. The CPG for this category of patients has been separately prepared and published recently by TIF (2013).
β thalassaemia
β thalassaemia includes three main forms: thalassaemia major variably referred to as ‘Cooley’s anaemia’ and ‘Mediterranean anaemia’, thalassaemia intermedia and thalassaemia minor also called ‘β thalassaemia carrier’, ‘β thalassaemia trait’ or ‘heterozygous β thalassaemia’. Apart from the rare dominant forms, subjects with β thalassaemia major are homozygotes or compound heterozygotes for β0 or β+ genes, subjects with thalassaemia intermedia are mostly homozygotes or compound heterozygotes and subjects with thalassaemia minor are mostly heterozygotes.
Pathophysiology
The basic defect in β thalassaemia is a reduced or absent production of β globin chains with relative excess of α chains which accumulate and precipitate in the erythroid precursors forming inclusion bodies that, bound to the membrane skeleton, cause oxidative membrane damage and extensive premature destruction by apoptosis of the red blood cell precursors in the bone marrow (ineffective erythropoiesis). Haemolysis plays a secondary role and is less prominent in thalassaemia major than in thalassaemia intermedia. The first response to ineffective erythropoiesis and anaemia is an increased production of erythropoietin which, in turn, may produce hyperplasia of erythroid marrow in medullary and extramedullary sites with characteristic deformities of the skull and face, cortical thinning and pathological fractures of long bones, extramedullary erythropoietic tissue masses and splenomegaly. The lipid membrane composition of abnormal red blood cells may result in thrombotic complications, especially in splenectomised patients. Moreover, untreated or undertreated thalassaemia major patients may have retarded growth as a result of anaemia and the excessive metabolic burden imposed by erythroid expansion. Anaemia may produce cardiac enlargement and sometimes severe cardiac failure. In non-transfused patients, erythropoiesis, anaemia, and hypoxia downregulate production of hepcidin, a 25-amino acid peptide produced by hepatocytes that plays a central role in the regulation of iron homeostasis, with increased intestinal iron absorption and resulting iron overload. The pathophysiology of β thalassaemia is summarised in Figure 3.

Figure 3
Effects of excess production of free α globin chains in β thalassaemia.
The degree of globin chain imbalance is determined by the nature of the mutation of the β-gene. β0 refers to the complete absence of production of β globin directed by the affected allele. β++ refers to alleles with some residual production of β globin (often around 10%). In β+ the reduction in β globin production is very mild. Almost 300 beta thalassemia alleles have now been characterised. A complete, current list is available at the Globin Gene Server (http://globin.cse.psu.edu/). The large majority are missense, nonsense or frameshift variants. Rarely, the β thalassaemias are the result of gross gene deletion. Despite marked molecular heterogeneity, the prevalent molecular defects are limited in each at-risk population, in which 4 to 10 variants usually account for most of HBB disease–causing alleles.
Table 1 summarises the most common types of β thalassaemia mutations according to their ethnic distribution and severity.
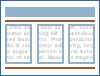
Table 1
Common types of β thalassaemia, their severity and ethnic distribution.
Clinical diagnosis
Carriers of thalassaemia are usually clinically asymptomatic but sometimes have mild anaemia. Clinical presentation of β thalassaemia major usually occurs between 6 and 24 months of age with severe microcytic anaemia, mild jaundice and hepatosplenomegaly. Affected infants fail to thrive and become progressively pale. Feeding problems, irritability, recurrent bouts of fever due to a hypermetabolic state or intercurrent infection, and progressive enlargement of the abdomen caused by spleen and liver enlargement may occur. In developed countries, if prenatal diagnosis has not been performed, the diagnosis of β thalassaemia major is established at this stage and a regular transfusion program is initiated. The classic clinical picture of β thalassaemia major is currently seen only in some developing countries in which the resources for performing long-term transfusion programmes are not available. The clinical picture in patients who are untreated or poorly transfused, is characterised by growth retardation, pallor, jaundice, poor musculature, genu valgum, hepatosplenomegaly, leg ulcers, development of masses from extramedullary haematopoiesis, and skeletal changes resulting from expansion of the bone marrow. Skeletal changes include deformities in the long bones of the legs and typical craniofacial changes: thalassaemic facies (bossing of the skull, prominent malar eminence, depression of the bridge of the nose and hypertrophy of the maxillae, which tends to expose the upper teeth). If a chronic transfusion regimen is not started, patients with thalassaemia major usually die within the first few years of life from high-output heart failure.
Individuals with thalassaemia intermedia present later than those with thalassaemia major, have milder anaemia and, by definition, do not require or only occasionally require transfusions. At the severe end of the clinical spectrum, patients are brought to medical attention between the ages of 2 and 6 years with retarded growth and development. At the other end of the spectrum are patients who are completely asymptomatic until adult life with only mild anaemia. Hypertrophy of erythroid marrow with the possibility of extramedullary haematopoiesis (EMH) is common. Its consequences are characteristic deformities of the bone and face, osteoporosis with pathological fractures of long bones, formation of erythropoietic masses that primarily affect the spleen, liver, lymph nodes, chest and spine and a tendency to thrombotic complications. Enlargement of the spleen is also a consequence of its major role in clearing damaged red cells from the bloodstream. Leg ulcers are frequent. Cardiac involvement in thalassaemia intermedia is mainly characterised by a high-output state and pulmonary hypertension, with systolic left ventricle function usually preserved. Myocardial siderosis is rare. Without appropriate treatment,the incidence of the comorbiditieincreases with advancing age. Iron overload in non-transfused patients mainly occurs because of increased intestinal iron absorption due to greatly expanded but ineffective erythropoiesis. Although the rate of iron loading is slower in thalassaemia intermedia than in thalassaemia major, patients with thalassaemia intermedia can eventually develop complications similar to those of patients with thalassaemia major, including hepatic, endocrine and cardiac dysfunction.
Haematological diagnosis
Heterozygous carriers of β thalassaemia, usually display a low mean cell haemoglobin (MCH), low mean cell volume (MCV) and an increased level of Hb A2, which may be associated with low normal or slightly subnormal haemoglobin levels. A peripheral blood film shows less severe erythrocyte morphological changes than affected individuals and erythroblasts are normally not seen. β thalassaemia major is characterised by reduced haemoglobin level (<70 g/d), MCV >50 fl and <70 fl and MCH >12 and <20 pg. Thalassaemia intermedia is characterised by Hb level between 70 and 100 g/l, MCV between 50 and 80 fl and MCH between 16 and 24 pg. The peripheral blood film in affected individuals demonstrates red blood cell morphological changes with microcytosis, hypochromia, anisocytosis, poikilocytosis and nucleated red blood cells (erythroblasts, NRBC). The number of erythroblasts is related to the degree of anaemia and is markedly increased after splenectomy. In general, these abnormal red blood cell morphological features are shared among different types of thalassaemia syndrome including interactions with haemoglobin variants such as Hb E/β thalassaemia (see below).
Qualitative and quantitative haemoglobin analysis
Cellulose acetate electrophoresis or capillary electrophoresis (CE) and DE-52 microchromatography or high pressure liquid chromatography (HPLC) identify the amount and type of haemoglobin present. The Hb pattern varies by β thalassemia type. In β0 thalassaemia homozygotes, Hb A is absent and Hb F constitutes 92-95% of the total Hb. In β+ thalassaemia homozygotes and β+/β0 compound heterozygotes Hb A levels are between 10 and 30% according to the variable degree of reduction of β globin chain synthesis and Hb F is 70-90%. Hb A2 is variable in β thalassaemia homozygotes and it is enhanced in β thalassaemia minor. Hb F can readily be detected by an acid elution test (F-cell staining) and alkali denaturation.
Molecular analysis
Since the prevalent pathogenic variants are limited in each at-risk population, targeted analysis for pathogenic variants based on ancestry may be considered first. Commonly occurring mutations of the β globin gene are detected by polymerase chain reaction (PCR)-based procedures. The most commonly used methods are reverse dot blot analysis or primer-specific amplification, with a set of probes or primers complementary to the most common mutations in the population from which the affected individual originated. β globin gene sequence analysis may be considered first if the affected individual is not of an ancestry at high risk or if targeted analysis reveals only one or no pathogenic variant. If the results are inconclusive, gene-targeted deletion/duplication analysis can follow.
Correlation genotype-phenotype
The extent of globin chain imbalance is the main determinant of clinical severity in β thalassaemia. Therefore, any factor capable of reducing this imbalance results in a lesser degree of α globin chain precipitation and may ameliorate the clinical picture. One of the most common and consistent mechanisms is homozygosity or compound heterozygosity for two β+ thalassaemia mild or silent mutations. Examples of these alleles are the silent -101 C T and the mild IVS-1–6 T C mutation in the Mediterranean population, the -28 A G in Southeast Asian population and the –29 A G in Africans.
Other factors able to ameliorate the phenotype are the coinheritance of α thalassaemia or of genetic determinants that increase gamma chain production. Deletional and non-deletional hereditary persistence of fetal haemoglobin (HPFH) mutations, associated with a high Hb F level in carriers, when in genetic compounds with severe β thalassaemia alleles, result in mild thalassaemia intermedia. A mild phenotype may also be determined by co-inheritance of genetic determinants associated with gamma chain production, mapping outside the β globin cluster. Recently, several studies using genome-wide association studies (G-WAS) have identified two quantitative trait loci (BCL11A on chromosome 2p16 and HBS1L-MYB intergenic region on chromosome 6q23) that account for 20%-30% of the common variation in Hb F levels in healthy adults and that are associated with the mild thalassaemia intermedia phenotype and with a delayed need for transfusion in patients with homozygous β0 thalassaemia. Furthermore, BCL11A seems to be involved in the regulation of the haemoglobin switching process.
In some instances, heterozygous β thalassaemia can lead to the thalassaemia intermedia phenotype instead of the asymptomatic carrier state. Most of these patients have excess functional α globin genes (α gene triplication or quadruplication) which increases the imbalance in the ratio of α/non-α globin chain synthesis.In addition, rare mutations that result in the synthesis of extremely unstable β globin variants which precipitate in erythroid precursors causing ineffective erythropoiesis may be associated with thalassaemia intermedia in the heterozygotes (dominant thalassaemia).
Several secondary genetic modifiers able to modify the clinical expression of the thalassaemia syndrome have been identified in recent years. The most studied is the presence of (TA)7 polymorphism in the promoter region of the uridine diphosphate-glucuronosyltransferase gene, which in the homozygous state is associated with Gilbert syndrome, and is associated with the development of cholelithiasis in thalassaemia major and intermedia. Other candidate genes are the apolipoprotein E ε4 allele, which seems to be a genetic risk factor for left ventricular failure in homozygous β thalassaemia. Less defined modifying factors are genes coding for HFE-associated hereditary haemochromatosis and genes involved in bone metabolism.
β structural haemoglobin variants relevant to thalassaemia management
- 1.
Haemoglobin E disorder is the most common structural variant with thalassaemic properties.
Hb E is characterised by the substitution of lysine for glutamic acid at position 26 of the β globin chain. The mutation G-A at codon 26 of the β globin genes not only produces the amino acid substitution but also activates a cryptic splice site at codon 24-25, leading to an alternative splicing pathway. The overall result is the production of reduced amounts of the variant haemoglobin (Hb E); Hb E constitutes 25-30% of total haemoglobin in Hb E carriers, instead of the expected 50%. In other words, the codon 26 G-A mutation results both in a qualitative and a quantitative β globin gene defect.
Hb E is the most common abnormal haemoglobin in Southeast Asia, with a carrier frequency of up to 50% in some regions. It is also prevalent in parts of the Indian subcontinent, including India, Pakistan, Bangladesh and Sri Lanka. Heterozygotes for Hb E are clinically normal and manifest only minimal changes in red blood cell indices, with the presence of Hb E on haemoglobin analyses. Hb E can be easily detected using a special dye: dichlorophenolindophenol (DCIP). Homozygotes for Hb E are clinically silent and may be only mildly anaemic. The peripheral blood film shows microcytosis with 20-80% of target cells, while Hb electrophoresis or HPLC shows 85-95% Hb E and 5-10% Hb F. However, a few individuals with homozygous Hb E with up to 20% of Hb F have been identified.
Genetic compounds for Hb E and β thalassaemia, which are also common in Southeast Asia, have clinical manifestations that are variable in severity – from thalassaemia intermedia to severe transfusion-dependent thalassaemia major. These can be classified into three categories:
- Mild Hb E/β thalassaemia: this is observed in about 15% of all cases in Southeast Asia. This group of patients maintain Hb levels between 90 and 120 g/l and usually do not develop clinically significant problems at an early age. However some patients suffer from growth failure, iron overload and other complications similar to those of NTDT patients.
- Moderately severe Hb E/β thalassaemia: the majority of Hb E/β thalassaemia cases fall into this category. The Hb levels remain at 60-70 g/l and the clinical symptoms are similar to β thalassaemia intermedia or NTDT. Transfusions are not required unless an infection precipitates further anaemia. Iron overload may occur.
- Severe Hb E/β thalassaemia: The Hb level can be as low as 40-50 g/l. Patients in this group manifest symptoms similar to those of β thalassaemia major and are treated as thalassaemia major or TDT patients.
The reasons for this variability have only partially been defined including type of β thalassaemia mutation (β+ or β0 thalassaemia), coinheritance of α thalassaemia and an innate propensity to produce post natal γ globin expression, and subjects with seemingly identical genotypes may have clinical manifestations very different in severity.
- 2.
Hb Lepore is another structural β variant resulting from a fusion of the δ and β globin genes. The homozygous state of Hb Lepore or Hb Lepore coinherited with β thalassaemia can result in moderate to severe transfusion-dependent β thalassaemia syndromes.
- 3.
Haemoglobin S (Hb S) is the most common haemoglobin variant in the world, resulting from a substitution of valine for glutamic acid at position 6 of the β globin chain. The interaction of β thalassaemia with Hb S results in a syndrome that closely resembles other sickling disorders. The management of sickle/β thalassaemia should follow the existing National Institutes of Health (NIH) guidelines for management of sickle cell disorders (See http://www
.nhlbi.nih .gov/health/prof/blood/sickle/sick-mt .htm for more information.)
α thalassaemia
α-thalassaemias are inherited disorders characterised by reduced or suppressed production of α globin chains. The human α globin genes are duplicated and located at the telomeric end of the short arm of chromosome 16. α thalassaemia is caused most commonly by deletions of large DNA fragments that involve one or both α globin genes.
Silent carrier state: the presence of a single α globin gene deletion or deletional α+ thalassaemia results in the silent carrier state. Heterozygotes for one missing α globin gene are not anaemic and have normal red blood cell indices. Two major types of this deletional α+ thalassaemia, 3.7 and 4.2 kb deletions, are wide spread throughout the globe and they have been identified even in the population in the Pacific.
α thalassaemia trait: subjects with two residual functional α genes either by deletions that remove two linked α globin genes from the same chromosome or α0 (– –/αα) or combination of deletional α+ thalassaemia (–α/–α), have mild hypochromia and microcytosis. Their MCV and MCH are usually lower than 80 fl and 27 pg, respectively. Less commonly, mutations caused by single or a few nucleotide deletions or alterations known as non-deletional α thalassaemia (αTα/ or ααT/) have been identified in several populations from Mediterranean countries to Southeast Asia and China. Haemoglobin Constant Spring (Hb CS) and Hb Paksé, two abnormal Hbs characterised by elongated alpha globin chains resulting from mutations of the termination codon in the alpha2 globin gene, are the most prevalent non-deletional alpha thalassaemias in Southeast Asia. Heterozygotes for non-deletional α thalassaemia have borderline MCV and MCH therefore they might not be detected in most of the programmes for thalassaemia prevention and control that use red blood cell indices as a screening tool.
Hb H disease: When there are deletions or non-deletional abnormalities of three globin genes, the affected individual would have only one functional gene and this hereditary disorder is known as HbH disease. It is usually characterised by a moderate haemolytic anaemia, splenomegaly and acute haemolytic crisis in response to oxidant drugs and infections. In general, patients with non-deletional Hb H disease have more severe disease than patients with deletional Hb H disease. For example, co-inheritance of Hb Constant Spring and the deletion of two α genes results in a severe form of Hb H disease in which up to 20% of patients require frequent blood transfusion and splenectomy. Most patients with Hb H disease can be managed as recommended in the NTDT guideline.
Rarely, Hb H disease patients with certain non-deletional mutations, specifically Hb Pak Num Po (ααPNP), Hb Quong Sze (αQZα) or Hb Adana (αCD59α) have a severe phenotype that mimics that of α thalassaemia major: early onset of anaemia (at birth or within 6 month of birth), marked anaemia (Hb <50 g/l), huge hepatosplenomegaly and failure to thrive. This condition is known as transfusion-dependent Hb H disease or Hb H hydrops fetalis since some patients even developed severe anaemia and hydropic changes in utero.
Hb Bart’s hydrops fetalis, the most severe clinical manifestation of α thalassaemia, is generally associated with the absence of all four α globin genes, severe fetal anaemia and death in utero. In addition, several maternal complications including preeclampsia, ante partum haemorrhage and dystocia are common in pregnant women with Hb Bart’s hydrops fetalis. Absence of α globin genes in ‘cis’ position in the same chromosome (α0 thalassaemia, – –/) is common in Southeast Asia and the Far East, while it is rare in the Mediterranean area and very rare in Africa. This different distribution explains why the Hb Bart’s hydrops fetalis syndrome and Hb H disease are common in Southeast Asian countries and China, rare in Mediterranean populations and almost absent in the African population. The complete loss of α globin production from the fetal stage results in formation of a tetramer of unpaired α globin chains (α4) that is designated Hb Bart’s. With advanced fetal medicine including intrauterine transfusion, several affected fetuses with Hb Bart’s hydrops have survived their intrauterine ordeals. The number of these surviving Hb Bart’s hydrops is increasing as the practice of IVT increases. However, these individuals will remain transfusion dependent and require life-long blood transfusion. Therefore patients with Hb Bart’s or Hb H hydrops or transfusion-dependent Hb H disease must be treated as TDT patients and follow the recommendations of this present guideline.
Pathophysiology
Reduction of α globin synthesis results in decreased production of Hb A (α2β2) and reduced haemoglobin synthesis. In addition, excess unpaired β globin chains can form tetramers (β4) that are not physically stable and precipitate, attaching to the red cell surface membrane and causing oxidative damage, thus shortening of red cell survival. The formation of β globin tetramers (Hb H) can be detected by haemoglobin analysis. The presence of Hb H increases during acute febrile illness due to increase body temperature. In non-deletional α thalassaemia and in particular in the case of mutations that generate α globin variants such as Hb CS, Hb H can directly precipitate at the membrane surface and generate reactive oxygen species even in the steady state. Therefore, patients with non-deletional Hb H disease are usually have more severe disease than those with deletional Hb H disease. Thalassaemia intermedia and Hb H disease may have similar degrees of anaemia, but haemolysis rather than ineffective erythropoiesis is the primary mechanism in Hb H disease. Indeed, iron loading is much more common inthalassaemia intermedia than in Hb H disease.
Haematological diagnosis
Similarly to β thalassaemia syndromes, patients with Hb H disease have a hypochromic microcytic anaemia with a baseline of haemoglobin of 40-130 g/l. Increased polychromasia and reticulocytosis are observed and can be further augmented during acute infectious episode or haemolytic crises. Nucleated red blood cells and basophilic stippling are commonly present in more severe phenotypes such as Hb Bart’s hydrops and severe non-deletional Hb H disease. Detection of Hb H as Hb H inclusion bodies in a peripheral blood film using supravital staining (brilliant cresyl blue) is the hallmark of this condition.
Qualitative and quantitative haemoglobin analysis
Identification of fast moving haemoglobin species by electrophoresis representing Hb H (β4) and Bart’s (γ4) is characteristic of α thalassaemia syndromes. The levels of Hb H measured can vary from < 1% up to 40% (usual range 10-15%) due to sensitivity of tests, laboratory expertise, type of instruments and the quality of blood samples. Hb H might not be readily identified through some platforms of liquid chromatography; a manual identification using the presence of haemoglobin species at a specific retention time (RT) is required. Due to a lack of available α globin chains, Hb A2 (α2δ2) is reduced. In patients with non-deletional Hb H disease especially Hb H/Hb CS, Hb CS variant can be detected at a very low level (1-4%).
Molecular diagnosis
Molecular testing approaches can include targeted deletion analysis for common deletions, sequence analysis, and deletion analysis of the α1 and α2 globin genes and the HS -40 regulatory region (LCRA). Targeted deletion analysis for specific deletions within the α globin gene cluster can be performed first. The method commonly used to target a deletion is GAP-PCR. The most commonly used methods for known non-deletional mutations are reverse dot blot analysis, primer-specific amplification or PCR following by enzymatic digestion. Sequence analysis of the α1 and α2 globin genes can be performed next if a common deletion is not identified. Typically, exon or whole-gene deletions/duplications are not detected. Deletion analysis of α1 and α2 globin genes and the HS -40 regulatory region located 40 kb upstream from the α globin cluster can be performed next to detect uncommon deletions associated with α thalassaemia if pathogenic variants have not been identified by targeted deletion testing or sequence analysis. Methods that may be used to detect rare or unknown deletions include: Southern blotting (now fallen into abeyance), quantitative PCR, long-range PCR and, above all, multiplex ligation-dependent probe amplification (MLPA). The same methods may be used to detect duplications of the α cluster. Further testing for genes associated with genetic disorders similar to α thalassemia, such as ATRX and HBB may also be considered if clinically indicated.
Since almost all thalassaemic conditions as aforementioned present with hypochromic microcytic anaemia, a diagnosis of thalassaemia should be considered in all those who have such abnormal red blood cell features. However, it is important to exclude iron deficiency anaemia as a possible cause since it remains common in several part of the world. A summary of diagnostic measures for patients with hypochromia and microcytosis and further with diagnostic features of common thalassaemia syndromes are shown in Figures 4, 5 and 6.

Figure 4
Flow chart for the diagnosis of haemoglobin disorders; steps in carrier screening and disease diagnosis. (MCH, mean cell haemoglobin; MCV, mean cell volume; ZnPP, zinc protoporphyrin).

Figure 5
Summary of diagnostic measures for thalassaemia and haemoglobinopathies. MLPA, multiplex ligation-dependent probe amplification; QTL, quantitative locus; TI, thalassaemia intermedia; TM, thalassaemia major.
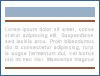
Box
Summary.
References
- Camaschella C., Pagani A. Advances in understanding iron metabolism and its cross-talk with erythropoiesis. British Journal of Haematology. [Online] 2018;182(4):481–494. Available from: doi: https://doi
.org/10.1111/bjh.15403. [PubMed: 29938779] - Danjou F., Anni F., Galanello R. Beta-thalassemia: from genotype to phenotype. Haematologica. [Online] 2011;96(11):1573–1575. Available from: doi: [PMC free article: PMC3208672] [PubMed: 22058279] [CrossRef]
- Danjou F., Francavilla M., Anni F., Satta S., et al. A genetic score for the prediction of beta-thalassemia severity. Haematologica. [Online] 2015;100(4):452–457. Available from: doi: [PMC free article: PMC4380717] [PubMed: 25480500] [CrossRef]
- Fucharoen S., Viprakasit V. Hb H disease: Clinical course and disease modifiers. Hematology / the Education Program of the American Society of Hematology. American Society of Hematology. Education Program. [Online] 2009;1:26–34. Available from: doi: [PubMed: 20008179] [CrossRef]
- Galanello R., Cipollina M.D., Dessi C., Giagu N., et al. Co-inherited Gilbert’s syndrome: a factor determining hyperbilirubinemia in homozygous beta-thalassemia. Haematologica. [Online] 1999;84(2):103–105. Available from: doi: [PubMed: 10091405] [CrossRef]
- Galanello R., Origa R. Beta-thalassemia. Orphanet Journal of Rare Diseases. [Online] 2010;5(1):11. Available from: doi: [PMC free article: PMC2893117] [PubMed: 20492708] [CrossRef]
- Galanello R., Sanna S., Perseu L., Sollaino M.C., et al. Amelioration of Sardinian β0 thalassemia by genetic modifiers. Blood. [Online] 2009;114(18):3935–3937. Available from: doi: [PMC free article: PMC2925722] [PubMed: 19696200] [CrossRef]
- Ganz T. Hepcidin—a regulator of intestinal iron absorption and iron recycling by macrophages. Best Practice & Research Clinical Haematology. [Online] 2005;18(2):171–182. Available from: doi: [PubMed: 15737883] [CrossRef]
- Giardine B., Borg J., Viennas E., Pavlidis C., et al. Updates of the HbVar database of human hemoglobin variants and thalassemia mutations. Nucleic Acids Research. [Online] 2014;42(D1):D1063–D1069. Available from: doi: [PMC free article: PMC3964999] [PubMed: 24137000] [CrossRef]
- Laosombat V., Viprakasit V., Chotsampancharoen T., Wongchanchailert M., et al. Clinical features and molecular analysis in Thai patients with HbH disease. Annals of hematology. [Online] 2009;88:1185–1192. Available from: doi: [PubMed: 19390853] [CrossRef]
- Martyn G.E., Wienert B., Yang L., Shah M., et al. Natural regulatory mutations elevate the fetal globin gene via disruption of BCL11A or ZBTB7A binding. Nature Genetics. [Online] 2018;50(4):498–503. Available from: doi: [PubMed: 29610478] [CrossRef]
- Origa R., Cazzola M., Mereu E., Danjou F., et al. Differences in the erythropoiesis-hepcidin-iron store axis between hemoglobin H disease and β-thalassemia intermedia. Haematologica. [Online] 2015;100(5):e169–e171. Available from: doi: [PMC free article: PMC4420223] [PubMed: 25596269] [CrossRef]
- Origa R., Galanello R., Ganz T., Giagu N., et al. Liver iron concentrations and urinary hepcidin in beta-thalassemia. Haematologica. [Online] 2007;92(5):583–588. Available from: doi: [PubMed: 17488680] [CrossRef]
- Origa R., Sollaino M.C., Borgna-Pignatti C., Piga A., et al. α-Globin Gene Quadruplication and Heterozygous β-Thalassemia: A Not So Rare Cause of Thalassemia Intermedia. Acta Haematologica. [Online] 2014;131(3):162–164. Available from: doi: [PubMed: 24217654] [CrossRef]
- R O., R G. Pathophysiology of beta thalassaemia. Pediatric Endocrinology Reviews : PER. 2011;8 Suppl 2:263–270. [PubMed: 21705976]
- Taher A., Weatherall D.J., Cappellini M.D. Thalassaemia. The Lancet. [Online] 2018;391(10116):155–167. Available from: doi: [PubMed: 28774421] [CrossRef]
- Traivaree C., Monsereenusorn C., Rujkijyanont P., Prasertsin W., et al. Genotype–phenotype correlation among beta-thalassemia and beta-thalassemia/HbE disease in Thai children: predictable clinical spectrum using genotypic analysis. Journal of Blood Medicine. [Online] 2018;9:35–41. Available from: doi: [PMC free article: PMC5905821] [PubMed: 29695942] [CrossRef]
- Uda M., Galanello R., Sanna S., Lettre G., et al. Genome-wide association study shows BCL11A associated with persistent fetal hemoglobin and amelioration of the phenotype of -thalassemia. Proceedings of the National Academy of Sciences. [Online] 2008;105(5):1620–1625. Available from: doi: [PMC free article: PMC2234194] [PubMed: 18245381] [CrossRef]
- Viprakasit V. Alpha-thalassemia syndromes: from clinical and molecular diagnosis to bedside management. 2013;10
- Viprakasit V., Limwongse C., Sukpanichnant S., Ruangvutilert P., et al. Problems in determining thalassemia carrier status in a program for prevention and control of severe thalassemia syndromes: a lesson from Thailand. Clinical Chemistry and Laboratory Medicine. [Online] 2013;51(8):1605–1614. Available from: doi: [PubMed: 23525874] [CrossRef]
- Viprakasit V., Tanphaichitr V.S., Chinchang W., Sangkla P., et al. Evaluation of alpha hemoglobin stabilizing protein (AHSP) as a genetic modifier in patients with β thalassemia. Blood. [Online] 2004;103(9):3296–3299. Available from: doi: [PubMed: 14715623] [CrossRef]
- Weatherall David. J. The inherited diseases of hemoglobin are an emerging global health burden. Blood. [Online] 2010;115(22):4331–4336. Available from: doi: [PMC free article: PMC2881491] [PubMed: 20233970] [CrossRef]
- Weatherall D.J. The Evolving Spectrum of the Epidemiology of Thalassemia. Hematology/Oncology Clinics of North America. [Online] 2018;32(2):165–175. Available from: doi: [PubMed: 29458724] [CrossRef]
- Haemoglobin Types
- Globin genes and globin synthesis
- The thalassaemias
- β thalassaemia
- Pathophysiology
- Clinical diagnosis
- Haematological diagnosis
- Qualitative and quantitative haemoglobin analysis
- Molecular analysis
- Correlation genotype-phenotype
- β structural haemoglobin variants relevant to thalassaemia management
- α thalassaemia
- References
- Review Pathophysiology and therapy for haemoglobinopathies. Part II: thalassaemias.[Expert Rev Mol Med. 2006]Review Pathophysiology and therapy for haemoglobinopathies. Part II: thalassaemias.Urbinati F, Madigan C, Malik P. Expert Rev Mol Med. 2006 May 9; 8(10):1-26. Epub 2006 May 9.
- Review Molecular epidemiology of the thalassaemias (including haemoglobin E).[Baillieres Clin Haematol. 1992]Review Molecular epidemiology of the thalassaemias (including haemoglobin E).Hill AV. Baillieres Clin Haematol. 1992 Jan; 5(1):209-38.
- [Molecular characterization of thalassemias in the Valencia community and its relationship with the hematological phenotype].[Sangre (Barc). 1998][Molecular characterization of thalassemias in the Valencia community and its relationship with the hematological phenotype].Pérez Sirvent M, Moreno Miralles I, Boluferx Gilabert P, Lerma Alejos E, Gómez Rejas MA, Vayá Montaña A, Tascón Astigarraga A, Dasí Carpio MA, Martínez Silvestre M, López Espinosa AR, et al. Sangre (Barc). 1998 Oct; 43(5):392-8.
- Molecular defects in beta-thalassaemias in the population of Saudi Arabia.[Hum Hered. 1995]Molecular defects in beta-thalassaemias in the population of Saudi Arabia.el-Hazmi MA, al-Swailem AR, Warsy AS. Hum Hered. 1995 Sep-Oct; 45(5):278-85.
- Comparison of erythrocyte indices to differentiate between iron deficiency and alpha-thalassaemias in children with microcytosis and/or hypochromia.[East Mediterr Health J. 2010]Comparison of erythrocyte indices to differentiate between iron deficiency and alpha-thalassaemias in children with microcytosis and/or hypochromia.Narchi H, Basak RB. East Mediterr Health J. 2010 Sep; 16(9):966-71.
- Genetic basis, pathophysiology and diagnosis of thalassaemias - 2021 GuidelinesGenetic basis, pathophysiology and diagnosis of thalassaemias - 2021 Guidelines
- LIM homeobox transcription factor 1-beta isoform 1 [Homo sapiens]LIM homeobox transcription factor 1-beta isoform 1 [Homo sapiens]gi|292494911|ref|NP_002307.2|Protein
- Winner3Winner3biosample
- Aj_body_1Aj_body_1biosample
- Avascular necrosis of the head of femurAvascular necrosis of the head of femurMedGen
Your browsing activity is empty.
Activity recording is turned off.
See more...