This is a work of the US government and distributed under the terms of the Public Domain
NCBI Bookshelf. A service of the National Library of Medicine, National Institutes of Health.
National Toxicology Program. Report on Carcinogens Monograph on Antimony Trioxide: RoC Monograph 13 [Internet]. Research Triangle Park (NC): National Toxicology Program; 2018 Oct.
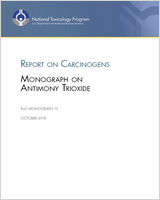
Report on Carcinogens Monograph on Antimony Trioxide: RoC Monograph 13 [Internet].
Show detailsIn the United States, antimony(III) trioxide (Sb2O3) is the most commercially significant form of processed antimony. In nature, Sb2O3 exists in minerals such as valentinite and senarmontite (ATSDR 2017; Roper et al. 2012). Antimony is found in nature in these and other mineral species, often in association with arsenic compounds due to their similar geochemical properties.
Exposure to antimony(III) trioxide is the focus of this section. However, evaluating exposure data specific to antimony(III) trioxide is complicated by the fact that antimony species can be interconverted in the environment and in vivo; thus, people can be exposed to antimony(III) trioxide from sources releasing other forms of antimony and to other forms of antimony from sources releasing antimony(III) trioxide. In addition, environmental and biomonitoring studies generally use methods that measure total elemental antimony (Sb) and not specific species of antimony. Data on exposure for specific antimony compounds are consequently limited. This section starts with antimony and antimony(III) trioxide consumption in the U.S. (Section 2.1), discusses exposure specifically to antimony(III) trioxide, and also briefly reviews exposure to other forms of inorganic antimony that might lead to exposure to antimony(III) trioxide.
Exposure to antimony(III) trioxide primarily results from its production, industrial and consumer uses, recycling, and release into the environment. In industrial processes, antimony(III) trioxide often changes its chemical form during production processes of formulation and processing, which will be discussed in more detail for manufacturing processes (Section 2.1) Occupational exposure from those uses is discussed in Section 2.2 (occupational exposure), and exposure among the general population is discussed in Sections 2.3.1 (consumer products) and 2.3.2 (environmental exposure).
2.1. Manufacturing Processes, Uses, and Production-related Information
2.1.1. Manufacturing Processes
The life cycle of antimony trioxide from raw material to consumer product is depicted in Figure 2-1. Antimony(III) trioxide for manufacturing processes may either be imported in that form (second box by the number 1 in Figure 2-1) or produced in the United States by oxidation of imported antimony metal (box 2 in Figure 2-1). Antimony trioxide is used to make various products and may change forms during the manufacturing of those products (see Section 2.1.2) The life cycle for antimony and antimony(III) trioxide often ends at disposal as waste during either production processes or in the final consumer product.

Figure 2-1
Antimony(III) Trioxide Used in Manufacturing Processes
Antimony(III) trioxide is produced primarily by re-volatilization of crude antimony(III) trioxide or by oxidation of antimony metal (EU 2008). The only domestic producer of primary antimony metal and oxide identified is a company in Montana that uses imported feedstock (USGS 2018), as no marketable antimony has been mined in the United States since 2015 (USGS 2018). The most recent U.S. mine production was in Nevada in 2013 and 2014, when about 800 tons of stibnite (Sb2S3), the principal antimony ore, was extracted. That mine has been on care-and-maintenance status (i.e., production has ceased but management for public health and safety continues) since 2015 (USGS 2018).
Antimony trioxide changes its chemical form during the formulation and processing stages for many products. The changes in chemical form for antimony are illustrated in Figure 2-1 by the grey shading in the boxes, which indicates the likelihood that antimony(III) trioxide is present at that stage of the process as described in the figure legend.
2.1.2. Uses
Antimony(III) Trioxide
The major industrial use of antimony(III) trioxide (NTP 2017a; USEPA 2014) is as a synergist for halogenated flame retardants in plastics, rubber, and textiles, all of which are used in a wide variety of consumer products. Other uses include as a catalyst for polyethylene terephthalate (PET) production, and an additive in art and specialized glasses, pigments, paints, and ceramics.
Flame retardant synergist: The bromine- or chlorine-containing flame retardants work by quenching free radicals in the gas phase of combustion. Hydrogen halides (e.g., hydrogen chloride, HCl; and hydrogen bromide, HBr) released from the halogenated flame retardants react with antimony(III) trioxide to form antimony halides, which are more effective as flame retardants than the hydrogen-containing molecules. The final concentration of antimony(III) trioxide as a flame-retardant synergist is 4% to 6% of the treated textile, but back-coating for textiles may contain up to 24% (EU 2008). Transformation of antimony(III) trioxide does occur if the product is burned (e.g., form antimony halides).
PET production: Antimony(III) trioxide used as a catalyst for polyethylene terephthalate (PET) production in Japan and China has been shown to be present in the finished plastic as antimony(III) glycolate with antimony concentration ranging from below the detection limit to above 300 mg/kg in the PET bottles (Takahashi et al. 2008). While the major current use for PET plastic is in bottles for water and other beverages, often intended for single use and then disposal, the major use for recycled PET is as PET fibers for fleece fabrics for clothing, in soft toys, rugs, carpets, and upholstery, including in automobiles. Antimony is not generally removed from the PET to recycle antimony (Grund et al. 2011).
Specialty glass, paints, and pigments: Antimony(III) trioxide is also used in art and other specialty glasses as a fining agent to remove gaseous inclusions that could leave bubbles in the glass product. Antimony is also used in paints and pigments as a white pigment and an opacifier. The resulting pigments are used in a broad range of industries and consumer products such as plastics, coatings, enamels, ceramics, and building materials. During the production process of specialty glass and pigments, antimony(III) trioxide may be chemically transformed to antimony(V) pentoxide by oxidation, and the resulting antimony(V) form may either present as antimony(V) pentoxide in glass or be chemically bonded in a crystal matrix in pigments. Approximately 0.8% antimony is found in finished glass.
An additional minor use of antimony(III) trioxide is in cement to reduce chromium(VI) to chromium(III). However, only those individuals working with cement as a powder would likely be exposed to antimony(III) trioxide because of the intended chemical reaction, which will change its chemical form (without changing antimony’s trivalent oxidation status) from Sb2O3 to the SbO33− ion (antimonite) in the finished concrete (Mapei Group 2017).
Future uses of antimony(III) trioxide are predicted to grow globally for use as a synergist with flame retardants (2% per year) and in PET production (8% per year) (EU 2008). No prediction for the uses in the U.S. market was found. Antimony(III) trioxide was introduced as a fining agent in glass manufacture to replace the more toxic arsenic, but the form of antimony used is shifting to sodium antimoniate(V) so that use of antimony(III) trioxide will likely decrease in the future.
Other Notable Uses for Major Antimony Forms
Major uses of elemental antimony, i.e., the metal, are to make metal alloys, such as lead-based alloys used in lead-acid batteries, lead pipe, cable sheathing, and ammunition; other alloys are used in electrical equipment, and plumbing. Antimony compounds (e.g., antimony(V) pentoxide and sodium(III) antimonite) are used as synergists for flame-retardant additives in plastics (ATSDR 2017; EU 2008). Other antimony compounds (e.g., lead stibnite and antimony sulfides) are also used as primers for ammunition, and in production of fireworks, pesticides, synthetic rubber, and automobile brake pads and linings. Antimony(III) diamyldithiocarbamate is used in lubricating compositions, such as grease, to provide extreme pressure protection (Hiza et al. 2006).
Medical uses of antimony compounds include as emetics (e.g., potassium antimonyl(III) tartrate or tartar emetic) (NTP 2017a) and to treat leishmaniasis (pentavalent antimonials, such as sodium stibogluconate(V)). However, the use of these drugs in the United States has declined. Pentavalent antimonials are no longer licensed for U.S. commercial use to treat leishmaniasis (CDC 2016b), but sodium stibogluconate(V) can be made available to U.S.-licensed physicians through the Centers for Disease Control and Prevention (CDC) Drug Service under an Investigational New Drug protocol approved by the U.S. Food and Drug Administration (FDA) and by CDC’s Institutional Review Board. In many other countries, the pentavalent antimonials administered by intravenous (i.v.) injection are still widely used.
2.1.3. Production, Consumption, and Trade of Antimony and Antimony(III) Trioxide in the United States
Antimony(III) trioxide, elemental antimony, and several other antimony compounds (e.g., antimony(V) pentoxide, and antimony(III) diamyldithiocarbamate) are high-production-volume chemicals, based on their production in, or import into, the United States in quantities of 1 million pounds or more per year (see Table 2-1 for U.S. antimony(III) trioxide and antimony compound production volumes for 2015 and Table 2-2 for import and export information). Elemental (i.e., metallic) antimony may be converted to antimony(III) trioxide by oxidation, and various forms of antimony, such as antimony(III) trisulfide in brake lubricants oxidize to antimony(III) trioxide at the high temperature achieved during the use of vehicle brakes. Other forms do not generally give rise to the trioxide form except through incineration. The EU (2008) risk assessment report noted that combustion or incineration processes produce antimony(III) trioxide from all forms of pre-incinerated antimony.
Table 2-1
U.S. Antimony(III) Trioxide and Antimony Compound Production Volumes for 2015 Exceeding 1 Million Pounds per Year Ranked by Quantity.
Table 2-2
U.S. Imports and Exports of Antimony Metal and Compounds for 2016a.
Antimony(III) trioxide accounts for 80% of total antimony use in the United States (NTP 2017a; USEPA 2014). Reports under the U.S. Environmental Protection Agency’s (EPA’s) Chemical Data Reporting rule indicate that approximately 1 million to 10 million pounds of antimony(III) trioxide is produced in the United States (see Table 2-1); however, the actual consumption of antimony(III) trioxide is likely much higher. In 2017, U.S. imports for consumption were approximately 52.8 million pounds of antimony oxide (weight of antimony content) (USGS 2018). EPA (2014) reported that most (approximately 87%) of the roughly 70 million pounds (gross weight) of antimony(III) trioxide consumed in the United States each year between 2007 and 2011 was imported (USEPA 2014). The majority of total antimony (83%) used in the United States is also imported, mostly from China, and the remainder (17%) is recovered from antimony-lead batteries (USGS 2018). In 2012, the U.S. EPA identified three companies manufacturing and ten companies importing antimony(III) trioxide (USEPA 2012).
2.2. Occupational Exposure
The highest exposures to antimony(III) trioxide and total antimony occur in the workplace including transportation workers exposed to antimony trioxide in the air. Historic data for the number of workers exposed to antimony were reported for the National Occupational Exposure Survey (NOES) conducted by the National Institute for Occupational Safety and Health (NIOSH) from 1981 to 1983, during which an estimated 209,773 male and female workers were potentially exposed to antimony(III) trioxide (CDC 2017). Although these data are over 30 years old, cancer has a long latency and thus this exposure information is still relevant. In 2010, 273 U.S. facilities likely produced or used antimony(III) trioxide (in flame retardants), based on information from EPA’s Toxics Release Inventory Program (USEPA 2014). Fire fighters may be exposed to antimony in smoke particulates released from combustion of retardant-treated textiles during fires (Fabian et al. 2010).
U.S. monitoring data from the Occupational Safety and Health Administration (OSHA) Chemical Exposure Health Dataset during a period of more than 30 years (1984 to 2017) reported data from 2,126 personal breathing zone samples collected from companies producing or using “antimony and compounds (as Sb)” (forms of antimony not specified) (OSHA 2017). The antimony air levels (measured personal breathing zone values), as total antimony, ranged from 0.2 μg/m3 to 54,500 μg/m3 across all facilities. Facilities with the highest antimony air concentrations were in the following industries: standard industrial classification (SIC) Code 2899, chemicals and chemical preparations, not elsewhere classified (this category would likely include antimony-containing flame retardants) (3.3 μg/m3 to 54,500 μg/m3); SIC Code 3341, secondary smelting and refining of nonferrous metals (this category would likely include antimonial lead refining) (1.8 μg/m3 to 47,700 μg/m3), and SIC Code 3339, primary smelting and refining of nonferrous metals (including antimony) (5 μg/m3 to 18,500 μg/m3). All of these industries are likely to involve exposure to antimony(III) trioxide as either a primary product or through oxidation of elemental antimony during smelting and refining processes; however, the levels most likely reflect other antimony compounds in addition to antimony trioxide.
Workers in the United States and other countries producing or using antimony(III) trioxide, as well as workers in occupations exposed to other antimony compounds, can be exposed to antimony(III) trioxide through inhalation of airborne solid dust or by skin contact resulting in increased excretion in the urine (see Table 2-3). The studies reported in Table 2-3 were identified primarily from the ATSDR (2017) draft toxicological profile for antimony and compounds and supplemented by literature searches. All results are reported from the primary publication cited.
Table 2-3
Air Levels and Urine Levels of Total Antimony in Workers Occupationally Exposed to Various Antimony Compounds in the Air.
Among industries using or producing antimony(III) trioxide, the highest levels (up to 5,000 to 6,000 μg/m3, levels 10 times higher than the threshold limit value [TLV]), are found among smelters or antimony manufacturing industries (see Table 2-3). The European Union (EU) (2008) risk assessment report (RAR) for antimony trioxide (Sb2O3) considered metal smelting and refining to be one of the major anthropogenic sources of antimony release to the atmosphere. U.S. air monitoring data specific for antimony(III) trioxide industries come primarily from NIOSH walk-through surveys of a few smelters or antimony(III) trioxide companies conducted largely in the 1970s, which usually were conducted as part of health hazard evaluations (CDC 2016a) or industrial hygiene surveys, the results for two of which were also reported in an epidemiological study (Schnorr et al. 1995) (see Table 2-3). Workers using or producing other types of antimony, such as elemental antimony used in the battery industry, can also be exposed to antimony(III) trioxide because metallic antimony oxidizes to antimony(III) trioxide in the air (EU 2008).
Workers in the transportation industry can be exposed to antimony trioxide from oxidation of antimony sulfide or sulfate in brake pads. Port workers in Valparaiso City, Chile were exposed to elevated air concentrations of antimony from heavy vehicular traffic (Quiroz et al. 2009) that resulted in very high levels of antimony in the blood (average concentration of 27 ± 9 ng antimony/kg), which were 5 to 10 times higher than in two control groups (1) from another part of the city or (2) from a rural area outside Valparaiso.
Urinary excretion of antimony by exposed workers generally increases with the level of exposure, although relatively few studies have reported both exposure and urinary excretion for the same workers. A few studies that reported both parameters are summarized in Table 2-4 together with studies that reported air levels only. The current TLV for elemental antimony and antimony compounds in air is 500 μg/m3 (ACGIH 2017)) and levels above as well as below this value have been reported. Bailly et al. (1991) measured urine and air concentrations of total antimony for workers manufacturing pentavalent antimony compounds (antimony(V) pentoxide and sodium antimoniate(V)) and reported a significant correlation (r = 0.83, p < 0.0001) between airborne antimony concentrations (log value) and both post-shift urinary antimony concentrations (log value) and an increase in urinary antimony concentrations during the work shift (r = 0.86, p= < 0.0001). Air concentrations and pre-shift and post-shift urinary antimony levels are also reported in Table 2-3.
Table 2-4
Antimony(III) Trioxide Occupational Exposure Level Estimates (as Antimony(III) Trioxide).
Extensive and systematic occupational monitoring data specific to antimony(III) trioxide, or exposures converted to antimony(III) trioxide equivalents, were reported by the EU risk assessment report (EU 2008) (Table 2-4). The industrial processes used in Europe are likely similar to those used in the United States, so data from the EU can help inform potential U.S. exposure. In general, the levels reported in the EU risk assessment report fall within similar ranges to those reported for the most recent U.S. data in Table 2-4 although considerable variability exists for reported values. In addition, the EU risk assessment report data are reported as antimony(III) trioxide; however, this represents only about a 20% difference from the estimates based on total antimony due to the adjustment for the atomic weight of oxygen. Also, the data for the United States are older and, thus, in general, U.S. exposure levels for some industries were higher than the European data. Both U.S. and European data indicate the highest exposures are for antimony(III) trioxide production, followed by the flame-retardant industries. Lower exposures are reported for production of crystal glass and pigment industries.
Inhalation exposure can also occur when antimony(III) trioxide powder is used in cement mixing (or cement powder-based product blending) applications (see Section 2.3) (Mapei Group 2017).
2.3. General Population Exposure
Evidence for exposure of the U.S. general population to antimony is provided by biomonitoring data showing its presence in urine, whole blood, and saliva. Data from the National Health and Nutrition Examination Survey (NHANES) indicate low level of exposure to antimony, with antimony (all forms of antimony) geometric means urine concentration of 0.132 μg/L for years 1999 to 2000 and 0.043 μg/L for years 2013 to 2014 (Table 2-5). Although the mean concentration (not considering the samples with antimony at below detection limit) appeared to be decreasing over time, this could reflect the use of more sensitive analytical methods, primarily inductively coupled plasma mass spectrometry (ICP-MS) in recent years, rather than an actual decrease in exposure, an explanation supported by reports of values close to the lower detection limits for the methods used (Filella et al. 2013a). On the other hand, Pang et al. (2016) (see Table 2-5) analyzing urine samples collected from 1998 to 2003 with a sensitive ICP-MS method reported urine antimony concentrations of 0.1 μg/L or higher, suggesting the exposure was higher.
Table 2-5
Ranges of Geometric Mean and 95th Percentile Antimony Levels in Urine, Blood, and Saliva Samples of U.S. Populations.
Based on analysis of NHANES data, higher urinary antimony levels were found in individuals with lower socioeconomic status, defined as either low income or living in economically deprived neighborhoods (Belova et al. 2013; Gonzales et al. 2016; Tyrrell et al. 2013). Slightly higher urinary antimony levels were reported for smokers than non-smokers in 2013 to 2014 data, as well as for younger people (6 to 11 years old, and 12 to 19 years old) than 20 years and older in 1999 to 2000 data and in 2013 to 2014 data. Total antimony measured in urine as the elemental form can be from various forms of antimony, not just antimony(III) trioxide (see Table 2-5). Antimony concentrations in whole blood (Filella et al. 2013a; 2013b; Whitworth et al. 2017) and saliva (Olmez et al. 1998) were available in only few samples, and the concentrations were much higher than that in urine.
Several studies have reported an association between biomonitoring data in the general population (e.g., urinary antimony, cord blood antimony) and adverse biological effects (Scinicariello and Buser 2016) or non-cancer endpoints, such as cardiovascular-related diseases (e.g., Guo et al. (2016); Shiue and Hristova (2014)) and adverse pregnancy outcomes (Zheng et al. 2014), suggesting that chronic exposure to low levels of antimony may be a potential public health concern.
No U.S. data on total antimony concentrations in breast milk were found, but concentrations (arithmetic means) measured outside the United States ranged from below the detection limit to 13 ng/g [13 μg/L] (Filella et al. 2013a).
The general population is potentially exposed to antimony directly from consumer products (Section 2.3.1)or indirectly from the environment by inhaling contaminated air (Section 2.3.2)or by consuming contaminated food or drinking water (Section 2.3.3)Because antimony can change its form in the environment, the form of antimony to which people are exposed may not be the same form initially released into the environment.
Table 2-6 and Figure 2-1 summarize exposure sources to antimony compounds from exposure to products manufactured with antimony(III) trioxide and the final forms of antimony to which people are exposed.
Table 2-6
Sources of Antimony(III) Trioxide and the Final Forms of Antimony (Antimony(III) Trioxide and Others) to Which People Are Exposed.
2.3.1. Consumer Products
Consumers are potentially exposed to antimony from consumer products as a result of the use of antimony(III) trioxide as a synergist with flame retardants or in PET containers. Exposure of the general population from consumer products is generally to antimony(III) trioxide by inhalation of dust from these products although some exposure could also occur orally to antimony(III) trioxide or other forms of antimony. Exposure is likely higher for children, especially infants, because of their direct skin contact with carpet material containing antimony(III) trioxide as a flame-retardant synergist while crawling, their mouthing of other fabrics containing flame retardants or toys with antimony-containing paint or plastic, and their potential to inhale more dust containing antimony from carpets because they are closer to the floor than adults (see Table 2-7). A 1998 study (Jenkins et al. 1998) reported that antimony could be detected in infant cot mattress covers containing polyvinyl chloride (PVC), and antimony was present in the leachate (extraction fluids) from mattress material.
Table 2-7
Estimated Consumer Exposure to Antimony (as Antimony(III) Trioxide) Directly and Indirectly from Products Containing Antimony(III) Trioxide.
Because antimony(III) trioxide can change its form during the manufacture of many products, exposure may be to other forms of antimony. For instance, if antimony is released in liquid (e.g., water, sweat, or saliva) at near-neutral pH, it will exist as hydrolyzed forms in solution (see Figure 1-1 in Section 1), Sb(III) as Sb(OH)3 or H3SbO3 and Sb(V) as Sb(OH)6− or H2SbO4− rather than as antimony cations (ATSDR 1992). The antimony in house dust is mainly antimony(III) trioxide (from wear and tear of flame-retardant-treated fabric) (EU 2008). Table 2-7 shows exposure levels for consumer products evaluated in the EU antimony trioxide (i.e., antimony(III) trioxide) risk assessment report, which converted all exposure levels to the equivalent mass of antimony(III) trioxide (i.e., converting measured antimony to corresponding antimony(III) trioxide based on molecular weight).
The only U.S. data on indoor air antimony levels are from an elementary school in Arizona (Majestic et al. 2012), where the particles <1 μm in diameter (PM1) fraction of air samples averaged 0.017 μg antimony/m3. Antimony in air was most likely resuspended from flame-retardant-treated carpet by foot traffic.
A study measuring antimony in costume cosmetic products purchased in the San Francisco Bay area reported measurements of antimony in eyeshadows (mean = 0.34 mg/kg; range = 0.13 to 0.57 mg/kg; N = 5) and in body paint (mean = 1.5 mg/kg; range = 0.12 to 6.2 mg/kg; N = 5) (Perez et al. 2017).
A study in the United Kingdom measured antimony in 750 consumer products (rubber, textile, and foamed materials) (Turner and Filella 2017), and detected antimony in 18% of over 800 measurements of those products at approximately 60 μg/g to 60,000 μg/g. Antimony was also detected in another study in the United Kingdom that measured antimony and other toxic metals in paints on public playground structure surfaces; levels ranged from 273 μg/g to 16,000 μg/g (Turner et al. 2016). Similar products in the United States would likely have similar levels.
2.3.2. Environmental Exposure
Antimony enters the environment through releases from industries producing, using, or recycling antimony and from natural sources (e.g., volcanic activity or erosion). An estimate for antimony emissions to the air from natural sources in the 1980s indicated that 41% could be accounted for from wind-borne soil particles, volcanoes, sea salt spray, forest fires, and biogenic sources (ATSDR 2017). Anthropogenic activities such as mining, fossil fuel combustion (coal or petroleum), smelting, waste incineration, and other human activities increase antimony concentrations in the local environment, which may be carried by air or water beyond the immediate area of those activities.
Toxics Release Inventory (TRI) data indicate that production- and use-related releases of antimony and antimony compounds to the environment have occurred at numerous U.S. industrial facilities. In 2014, 542 U.S. facilities that manufactured, processed, and used antimony reported releasing 8.6 million pounds of antimony and antimony compounds into the environment (land, water, and air) (TRI 2016). An EPA Toxic Substances Control Act (TSCA) Work Plan Chemical Risk Assessment for Antimony Trioxide (USEPA 2014) sorted 2010 TRI data by industry codes using the North American Industry Classification System (NAICS) codes to identify a subset of 273 U.S. facilities that likely produced, processed, or used antimony(III) trioxide-containing flame retardants. In addition, 11,635 pounds of antimony per year were released into the air from antimony(III) trioxide plants.
Air
Releases into air are the most relevant source of exposure specifically to antimony(III) trioxide. Increases above background levels result from releases by companies producing or using antimony(III) trioxide and from geogenic emissions by oxidation of antimony as noted above (ATSDR 2017; EU 2008). Individuals living near industrial facilities may be exposed to much higher levels of antimony in the air; a study in the 1970s reported that antimony air levels downstream of a copper smelter in the United States exceeded 300 ppm [300,000 μg/m3] (HSDB 2013). U.S. antimony air particulate matter levels ranged from not detectable (the lower limit of detection was not reported) to 1.21 μg/m3, which was reported for a site close to a lead smelter (Ragaini et al. 1977). Elevated mean air levels of 0.146 μg/m3 were reported in areas near operating mines producing various ores in Kellogg, Idaho in 1970 (an area that includes one of six companies producing antimony in the United States in 1992) and 0.040 μg/m3 in an industrial area in England (ATSDR 2017).
Antimony can change oxidation state in the environment and during industrial use. Aerosolized elemental antimony oxidizes to antimony(III) trioxide through reactions with atmospheric oxidants (ATSDR 1992; ATSDR 2017; EU 2008). During coal combustion, antimony forms antimony oxides, regardless of the form of antimony present in the coal (Health Canada 2010); Pavageau et al. (2004) also reported formation of antimony(V) pentoxide from coal combustion. Similarly, antimony(III) trioxide is the primary species released to the atmosphere from other high-temperature industrial processes, such as smelting, combustion of petroleum and petroleum products, and incineration of products that contain antimony (Health Canada 2010; NTP 2017). Recycling of antimony as part of antimonial lead in automobile batteries, where antimony has historically made up to 2% of the total weight, generally involves oxidation of both metals, with production of antimony(III) trioxide (Dupont et al. 2016; Grund et al. 2011). Antimony(III) trisulfide (used as automobile brake lubricant) and antimony(III) trisulfate (used as automobile brake filler) have been reported to oxidize to antimony(III) trioxide at temperatures reached in the braking process (above 300°C) (EU 2008). Antimony concentration measurements taken at a roadside site in London, England were 6.73 ± 3.49 ng/m3 (0.00673 ± 0.00348 μg/m3) while the background level was 1.31 ± 0.807 ng/m3 (0.00131 ± 0.000807 μg/m3) (Gietl et al. 2010). People thus can inhale antimony(III) trioxide transformed from other antimony compounds.
Antimony is present almost entirely in the particulate matter in air. ATSDR summarized these data from various U.S. cities for 2014, reporting daily mean concentrations as total antimony ranging from 0.00037 to 0.002 μg/m3 for total suspended particulate, 0.0013 to 0.0206 μg/m3 for particles <10 μm in diameter (PM10), and 0.0019 to 0.022 μg/m3 for particles <2.5 μm in diameter (PM2.5) (see Table 6-4 in ATSDR (2017).) Antimony levels in areas unpolluted by anthropogenic activity are low (approximately 0.001 μg/m3) (ATSDR 2017). The EU (2008) estimated that the reasonable worst-case background concentration of antimony in outdoor air is 0.0026 μg/m3.
Water, Rain, and Soil
Antimony(III) trioxide most likely oxidizes to antimony(V) following contact with moisture and oxygen in air (EU 2008; Health Canada 2010) and exposure to antimony in aqueous media like water, rain, and snow are most likely to other forms of antimony. Thermodynamic equilibrium calculations indicate that antimony(V) predominates in oxic systems and antimony(III) in anoxic systems; however, antimony(III) has been detected at higher concentrations than predicted in oxic systems, and antimony(V) has been detected at higher concentrations than predicted in anoxic systems (Filella et al. 2002a).
According to the National Water-Quality Assessment (NAWQA) program, which surveyed groundwater between 1992 and 2003, U.S. groundwater had generally low concentrations of antimony, with a median concentration of <1 μg/L (ATSDR 2017). Mining activities have been shown to increase antimony levels in nearby water systems. For example, waste from antimony mining and smelting activities in the Kellogg district of northern Idaho were dumped into the South Fork River, which had a mean antimony level of 4.3 μg/L while the nearby North Fork River was considered unpolluted with a mean level of 0.9 μg/L (ATSDR 2017). Increased levels of antimony in rainwater likely depend on release of antimony from industrial sites. The mean total antimony concentration in rainwater collected downwind from a copper smelter in Tacoma, Washington was 1.3 ppb while that collected upwind during the same storms was only 0.03 ppb (ATSDR 1992).
Exposure to antimony in the soil is expected to be minimal because of low solubility and mobility of antimony (Li et al. 2014; USEPA 2014). However, both trivalent and pentavalent antimony compounds are present in dust and soil carried into houses (EU 2008). Although the levels of antimony in the earth’s crust average 0.2 μg/g to 0.3 μg/g, levels in soil vary more widely when samples are taken at different locations within the United States. A survey of soils by the United States Geological Survey (USGS) found levels from <1 μg/g to 8.8 μg/g with an average concentration of 0.48 ppm (μg/g), (Shacklette and Boerngen 1984). Proximity to motor vehicle traffic can also result in higher levels of antimony in soil. Levels of antimony in soil 0 cm to 5 cm below the surface at three locations in Austria indicated that the location with very little vehicular traffic had much lower antimony levels (0.64 μg/g) than the other sites with more traffic (6.30 μg/g and 2.74 μg/g) (Amereih et al. 2005).
2.3.3. Food and Drinking Water
Levels of antimony (form not specified) in food in the United States range from not detectable (limit of detection not reported) to 1.7 μg/g of dry weight (Belzile et al. 2011). Antimony(V) is the most prevalent antimony species in drinking water, as the result of oxidative treatments (chlorination or ozonation) used in water disinfection processes. Antimony levels in U.S. drinking water range from 0.02 μg/L to 9.6 μg/L. The value of 9.6 μg/L was reported for bottled water heated in PET bottles at 80°C for 48 hours.
Exposure to antimony can result from consumption of contaminated food or drinking water (see Table 2-8). However, the EU risk assessment report (EU 2008) noted that antimony(III) trioxide in solution will produce the antimony(III) ion, which hydrolyzes to either the trivalent form as neutral Sb(OH)3, or the pentavalent form as charged Sb(OH)6− (see Section 1.2).
Table 2-8
Antimony (as Antimony(III) Trioxide Equivalents) Typical and Worst-case Exposure Levels from Food, Breast Milk, and Drinking Water Based on Data Measured in Europe.
2.4. Summary and Synthesis
A significant number of people in the United States are exposed to antimony(III) trioxide (Sb2O3), as evidenced by occupational exposure data and supporting data on production, consumption, and releases into the environment and exposures from consumer products. In addition to exposure to antimony(III) trioxide in the workplace from its use as a synergist with flame-retardant chemicals, as a catalyst in production of PET plastic, as a pigment and fining agent in glass production, and as a colorant and opacifier in pigments for paints and ceramic glazes, people are potentially exposed from using consumer products containing antimony(III) trioxide, and by breathing contaminated air, or a combination of these sources. The chemical form of antimony changes during manufacturing, in the environment, and in vivo, and detection methods typically measure total antimony rather than specific forms of antimony, so identifying exposure specifically to antimony(III) trioxide is presently difficult.
The highest occupational exposure to antimony(III) trioxide occurs in workplaces that produce or use antimony(III) trioxide (e.g., smelting and refining operations and production of antimony(III) trioxide). During the 1970s, reported levels ranged from 50 to 5,000 μg/m3, compared with the current threshold limit value (TLV) of 500 μg/m3. In the United States, roughly 70 million pounds of antimony(III) trioxide are used annually as a synergist for halogenated flame retardants in plastics, rubber, and textiles, as a catalyst in PET production, and as an additive in optical and art glass, pigments, paints, and ceramics. Workers at an estimated 273 U.S. facilities (based on information from EPA’s Toxics Release Inventory) were exposed to antimony(III) trioxide in 2010. More than 200,000 workers were exposed to antimony(III) trioxide in the 1981 to 1983 U.S. National Occupational Exposure Survey, indicating extensive past exposure to antimony(III) trioxide.
The highest occupational exposure to antimony(III) trioxide in the United States, exceeding current regulatory levels by at least 10-fold, occurred during smelting and refining operations and production of antimony(III) trioxide in the 1970s and 1980s. Antimony is no longer mined in the United States and smelting and refining of metallic antimony and production of antimony(III) trioxide was limited to one company in the United States in 2017. More recent European data suggest that the highest exposure to antimony(III) trioxide occurs during production of antimony(III) trioxide, followed by the flame-retardant industry. Lower levels of exposures occur during the use of Sb2O3 in the glass and PET industries.
Biomonitoring for antimony in urine and environmental data provide evidence of widespread exposure to antimony; however, the proportion that results from exposure to antimony(III) trioxide is usually not known. Antimony in air is expected to be mainly in the form of antimony(III) trioxide with the highest concentrations near facilities, such as mines and smelting operations, that release antimony(III) trioxide into the air. People can also be exposed to antimony(III) trioxide in the air from oxidation of various forms of antimony, such as antimony(III) trisulfide in brake lubricants which is heated to a high temperature during the use of vehicle brakes, various antimony compounds in burning of coal and petroleum, and various forms of antimony in waste that is burned or incinerated. Household products that contain antimony(III) trioxide, particularly flame-retardant-treated textiles, plastics, and rubber, can release particles containing antimony(III) trioxide to the air or dust and antimony ions in liquids leading to dermal or oral exposures, e.g., through mouthing of these products by infants or small children.
- Human Exposure - Report on Carcinogens Monograph on Antimony TrioxideHuman Exposure - Report on Carcinogens Monograph on Antimony Trioxide
- uncharacterized protein CHLRE_16g675413v5 [Chlamydomonas reinhardtii]uncharacterized protein CHLRE_16g675413v5 [Chlamydomonas reinhardtii]gi|2082268694|ref|XP_042916199.1|Protein
- si:ch211-219a15.4 si:ch211-219a15.4 [Danio rerio]si:ch211-219a15.4 si:ch211-219a15.4 [Danio rerio]Gene ID:563138Gene
- ubiquitin carboxyl-terminal hydrolase 22/27/51 [Fusarium oxysporum f. sp. lycope...ubiquitin carboxyl-terminal hydrolase 22/27/51 [Fusarium oxysporum f. sp. lycopersici 4287]gi|902728448|gb|KNB00017.1||gnl|WGS |FOXG_03707T1Protein
Your browsing activity is empty.
Activity recording is turned off.
See more...