This is a work of the US government and distributed under the terms of the Public Domain
NCBI Bookshelf. A service of the National Library of Medicine, National Institutes of Health.
National Toxicology Program. Report on Carcinogens Monograph on Merkel Cell Polyomavirus: RoC Monograph 11 [Internet]. Research Triangle Park (NC): National Toxicology Program; 2016 Aug.
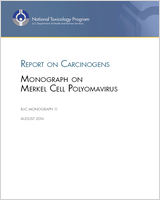
Report on Carcinogens Monograph on Merkel Cell Polyomavirus: RoC Monograph 11 [Internet].
Show detailsTo date, Merkel cell carcinoma is the only neoplasm associated with MCV (see Section 3); however, an etiologic role has been suggested for some cases of small-cell lung cancer (Helmbold et al. 2009), non-small cell lung cancer (Gheit et al. 2012; Hashida et al. 2013; Joh et al. 2010a; Lasithiotaki et al. 2013), and some hematologic malignancies (Teman et al. 2011). MCV was identified as a causal factor in Merkel cell carcinoma after it was found clonally integrated into the cellular DNA of approximately 80% of Merkel cell carcinoma tumors examined (Becker et al. 2009b; Feng et al. 2008; Kassem et al. 2008; Martel-Jantin et al. 2014; Rodig et al. 2012). In contrast, MCV DNA is maintained as a circular episome in the host cell during productive infection (Pastrana et al. 2009). This section reviews the following topics: the general characteristics of MCV and risk factors for Merkel cell carcinoma (Section 4.1), MCV and cancer hallmarks (Section 4.2), the mode of action and evidence for MCV’s role in Merkel cell carcinoma (Section 4.3), and provides a brief synthesis of the mechanistic data (Section 4.4).
4.1. Characteristics and Risk Factors
The biological properties and other characteristics of MCV were described in Section 1. MCV is part of the normal human skin flora as it is chronically shed from human skin (Schowalter et al. 2010). It follows that asymptomatic MCV infection is common (Amber et al. 2013; Chen et al. 2011; IARC 2013; Tolstov et al. 2009). Merkel cell carcinoma is a rare but aggressive primary neuroendocrine carcinoma that is thought to arise in Merkel cells (a type of mechanoreceptor cell located in the stratum basale of the skin) (Stakaitytė et al. 2014). These tumors occur most frequently (>90%) in sun-exposed areas, particularly around the head and neck, but can occur almost anywhere on the body.
Oncogenic viruses, including MCV, generally cause cancer by dysregulation of cell growth and proliferation; however, additional factors (e.g., immunosuppression, chronic inflammation, environmental agents) increase the risk for malignant transformation (Mesri et al. 2014). The infectious nature of oncogenic viruses distinguishes them somewhat from other cancer-causing agents (Ahuja et al. 2014). Although only a small percentage of individuals infected with an oncogenic virus develop cancer (this is typical for exposure to any carcinogenic agent), chronic infection provides the virus with a prolonged opportunity to mount mutagenic and epigenetic events that increases the risk of cell transformation and malignancy. Several critical alterations in a cell’s physiology (i.e., cancer hallmarks) have been identified that are required for malignant transformation (Hanahan and Weinberg 2000; 2011). The following sections discuss cofactors and cancer hallmarks that have been associated with MCV-induced Merkel cell carcinoma.
In addition to MCV, additional risk factors for Merkel cell carcinoma include immunosuppression, UV exposure, and advanced age (Agelli et al. 2010; Amber et al. 2013; Becker et al. 2009a; Dalianis and Hirsch 2013; Spurgeon et al. 2015; Spurgeon and Lambert 2013; Teman et al. 2011). Chronically immunosuppressed individuals (e.g., chronic lymphocytic leukemia, autoimmune disease, organ transplant, and HIV/AIDS patients) are more than 15 times more likely to develop Merkel cell carcinoma than their age- and sex-matched controls (Becker et al. 2009a). Merkel cell carcinoma in immunosuppressed individuals also has a higher mortality rate than in non-immunosuppressed individuals and occurs at a significantly younger age (about 50% <50 years compared with a mean age of 70 for all Merkel cell carcinoma cases). Further, partial regression of metastatic Merkel cell carcinoma has been reported following discontinuation of immunosuppressive therapy. Severe immunosuppression appears to increase the risk of Merkel cell carcinoma more than that of malignant melanoma (ratio of melanoma to Merkel cell carcinoma in the general population of 65:1 compared to 6:1 in the post-transplant population) (Agelli et al. 2010).
UV radiation is both mutagenic and immunosuppressive and may contribute to Merkel cell carcinoma development (see Section 3.3.2). In addition, C to T and CC to TT transition mutations have been identified in the p53 and H-ras genes of Merkel cell carcinoma and are considered diagnostic of UV-induced DNA damage (Agelli et al. 2010; Popp et al. 2002; Van Gele et al. 2000).
4.2. MCV and Cancer Hallmarks
All mammalian cells carry similar molecular machinery that regulates proliferation, differentiation, and cell death (Hanahan and Weinberg 2000; 2011). Transformation of a normal cell into a cancer cell is a multistep process that involves genetic and epigenetic changes that disrupts the cell’s molecular machinery and promotes malignant growth. These changes result in several critical alterations that are recognized as hallmarks of cancer and include: (1) sustained growth factor signaling, (2) evading growth suppressors, (3) resisting cell death, (4) enabling replicative immortality, (5) inducing angiogenesis, (6) activating invasion and metastasis, (7) evading immune destruction, and (8) reprogramming of energy metabolism. Genomic instability and inflammation underlie these changes and foster their acquisition and development. Several of these cancer hallmarks have been identified in the pathogenesis of MCV-positive Merkel cell carcinoma and are briefly reviewed here.
4.2.1. Growth Factor Signaling
Merkel cell carcinoma is a rare but aggressive skin tumor that grows rapidly and, if untreated, may double in size within a week (Becker et al. 2009a; Houben et al. 2009). Normal cells require mitogenic growth signals in order to move from a quiescent state into an active proliferative state (Hanahan and Weinberg 2000). These signals are transmitted into the cell via transmembrane receptors that bind distinctive classes of signaling molecules. In contrast, tumor cells are able to generate their own growth signals and are not as dependent on exogenous growth stimulation. Many oncogenes in cancer cells act by mimicking normal growth factor signals. Further, growth factor receptors are overexpressed or modified in many cancers, resulting in an enhanced response to circulating levels of exogenous growth factor signals or constitutive expression. Relevant growth factor signaling changes reported for Merkel cell carcinoma include a novel single heterozygous base change in exon 10 of the platelet-derived growth factor (PDGF) receptor and heterozygous loss of chromosome 10 or the long arm of chromosome 10 where the tumor suppressor phosphatase and tensin homologue (PTEN) is encoded (Fernández-Figueras et al. 2007; Houben et al. 2009; Swick et al. 2008; Van Gele et al. 2001; Van Gele et al. 1998). However, it was not clear if the base change in PDGF represented a true mutation or a single nucleotide polymorphism (Houben et al. 2009; Swick et al. 2008). No mutations were observed that affected the mitogen activated protein kinase (MAPK) pathway (Houben et al. 2006).
4.2.2. Evading Growth Suppressors
Normal tissues maintain cellular quiescence and homeostasis through multiple antiproliferative signals or growth suppressors (Hanahan and Weinberg 2000; 2011). In addition to inducing and sustaining growth-stimulatory signals, cancer cells must evade powerful antigrowth signals, many of which depend on the actions of tumor suppressor genes. In particular, the retinoblastoma and p53 tumor suppressor pathways are interconnected and operate as central control nodes to regulate cell proliferation, senescence, and apoptosis (Hanahan and Weinberg 2000; 2011; Houben et al. 2009; Yamasaki 2003). Retinoblastoma transduces growth-inhibitory signals originating primarily from outside the cell while p53 receives input from stress sensors, e.g., DNA damage, within the cell (Hanahan and Weinberg 2011; Houben et al. 2009). When in a hypophosphorylated state, retinoblastoma protein blocks cell proliferation by sequestering and altering the function of E2F transcription factors that control the expression of genes essential for progression from G1 to S phase. Phosphorylation of retinoblastoma by cyclin/cyclin-dependent kinase complexes causes dissociation of the retinoblastoma-E2F complex and cell-cycle entry (Sihto et al. 2011). The retinoblastoma and/or p53 pathways are dysregulated in virtually all human tumors (Yamasaki 2003).
Several studies have shown that the retinoblastoma pathway is critical to Merkel cell carcinoma pathogenesis while p53 mutations are rare (Bhatia et al. 2010; Borchert et al. 2014; Cimino et al. 2014; Harms et al. 2013; Higaki-Mori et al. 2012; Houben et al. 2012; Kuwamoto 2011; Lassacher et al. 2008; Sahi et al. 2014; Sihto et al. 2011). Merkel cell carcinoma retinoblastoma expression has a strong positive association with MCV DNA and MCV large T-antigen (LT) expression, suggesting that retinoblastoma inhibition is important for MCV-induced tumorigenesis (Sihto et al. 2011). Although LT expression was not associated with expression of phosphorylated retinoblastoma, LT binds to retinoblastoma, thus reducing retinoblastoma-E2F complex formation and inhibiting its cell-cycle regulation function. The oncogenic role of LT is discussed further in Section 4.3.2.
Cimino et al. (2014) reported that the retinoblastoma pathway was dysregulated in both MCV-negative and MCV-positive Merkel cell carcinoma cases and proposed two separate pathways of Merkel cell carcinoma oncogenesis. In MCV-positive cases, the retinoblastoma protein is functionally inactivated as described above. In MCV-negative cases, Merkel cell carcinoma tumors had truncating, nonsense mutations in the retinoblastoma gene. Mutations in the p53 gene were found primarily in LT- or retinoblastoma-negative tumors suggesting possible involvement of p53 in MCV-negative tumors (Sihto et al. 2011). Additionally, hypermethylation of the p14ARF promoter DNA has been reported in about 40% of Merkel cell carcinoma samples (Lassacher et al. 2008). Silencing of p14ARF could cause inactivation of the p53 pathway. Asioli et al. (2007) also reported that 25 of 47 cases of Merkel cell carcinoma were positive for p63 expression (a member of the p53 family) and that these cases demonstrated a more aggressive clinical course. In contrast, Higaki-Mori et al. (2012) showed no significant correlation of MCV infection and survival with p63 expression. However, the p63 gene is frequently amplified or overexpressed in human cancers (Asioli et al. 2007).
4.2.3. Apoptosis
Apoptosis is controlled by both intrinsic and extrinsic signaling pathways and involves counterbalancing pro- and antiapoptotic members of the Bcl-2 family of regulatory proteins (Adams and Cory 2007; Hanahan and Weinberg 2011). Bcl-2, and related proteins inhibit apoptosis by binding to and suppressing proapoptotic triggering proteins (Bax and Bak) that are embedded in the mitochondrial outer membrane. Overexpression of anti-apoptotic proteins or loss of pro-apoptotic signals results in loss of tissue homeostasis and supports oncogenesis by allowing cancer cells to evade programmed cell death. Bcl-2 overexpression is a common finding in many tumors and has been observed in approximately 67% to 85% of Merkel cell carcinoma tumors examined (Feinmesser et al. 1999; Houben et al. 2009; Kennedy et al. 1996; Sahi et al. 2012). However, Bcl-2 protein expression was not correlated with the MCV status of the tumors (Sahi et al. 2012). The presence of MCV also was associated with deregulated expression of the Bcl-2 gene in several cases of non-small-cell lung cancer (Lasithiotaki et al. 2013). Bcl-2 expression was downregulated in MCV-positive lung tumors compared with MCV-negative tumors (p = 0.05) or healthy tissue (p = 0.047), and the Bax/Bcl-2 ratio was 0.97 for the lung cancer group compared to 8.06 for the controls. A Bax/Bcl-2 ratio of <1 is associated with a lower apoptotic index (Brambilla et al. 1996). In addition, Bcl-2 inhibition was associated with Merkel cell carcinoma tumor shrinkage in an in vivo SCID mouse/human Merkel cell carcinoma xenograft model (Schlagbauer-Wadl et al. 2000).
The antiapoptotic protein survivin was also overexpressed in Merkel cell carcinoma tissue and was found to have a critical role in the survival of MCV-positive Merkel cell carcinoma cells (Arora et al. 2012b; Dresang et al. 2013; Kim and McNiff 2008; Sahi et al. 2012). A sevenfold increase in mRNA encoding the survivin oncoprotein was reported for MCV-positive compared with MCV-negative Merkel cell carcinoma tumors (Arora et al. 2012b). Xie et al. (2014) reported that decreased transcript and protein detection of the survivin gene in MCV-negative Merkel cell carcinoma cells was due to overexpression of microRNA (miRNA) miR-203. miR-203 functions as a tumor suppressor that is downregulated in certain cancers, and its expression was significantly lower in MCV-positive tumors compared with MCV-negative tumors. Nuclear staining for survivin was also associated with an aggressive clinical course and poor prognosis (Kim and McNiff 2008).
4.2.4. Angiogenesis
New blood vessel growth, or angiogenesis, is essential to sustain neoplastic development. To accomplish this growth, an “angiogenic switch” is almost always activated and remains on, causing normally quiescent vasculature to sprout new vessels to supply the growing tumor (Hanahan and Weinberg 2011). Increased expression of vascular endothelial growth factor (VEGF) via activation of hypoxia-inducible factor (HIF-1) is commonly observed in cancer. Although HIF pathway activation was not demonstrated with Merkel cell carcinoma, there was a significant association between metastatic tumor spread and elevated expression of VEGF (Fernández-Figueras et al. 2007). Another study reported that VEGF-A, VEGF-C, and VEGF-receptor 2 were expressed in 91%, 75%, and 88%, respectively, of the 32 Merkel cell carcinomas examined (Brunner et al. 2008).
4.2.5. Immune Evasion
The initial event in MCV-induced Merkel cell carcinoma is most likely a loss of immune surveillance for the virus as evidenced by increased risk in immunosuppressed populations (Amber et al. 2013; Hughes and Gao 2013; Moore and Chang 2010). MCV-related cases of Merkel cell carcinoma display vigorous antibody responses to MCV structural proteins; however, Merkel cell carcinoma tumors do not express detectable amounts of MCV VP1 capsid protein (Pastrana et al. 2009). These data suggest that the strong humoral responses in Merkel cell carcinoma patients are primed by an unusually robust MCV infection rather than Merkel cell carcinoma tumor viral antigen expression and that loss of cellular immune control may allow more extensive viral spread before tumor development (Moore and Chang 2010; Pastrana et al. 2009). Other mechanisms of immune evasions include downregulation of genes associated with the innate immune response (e.g., CCL20, CXCL-9, IL-2, IL-8, TANK) (Moens et al. 2015; Stakaitytė et al. 2014), downregulation of Toll-like receptor 9 (TLR9) (Griffiths et al. 2013), and disruption of inflammatory signaling via inhibition of the NF- κB essential modulator (NEMO) adaptor protein (Shahzad et al. 2013). TLR9 is a key receptor in the host innate immune response that senses viral or bacterial dsDNA. Mouchet et al. (2014) compared transcriptional profiles in MCV-positive Merkel cell carcinoma cells and normal Merkel cells and reported that most of the downregulated genes were related to immune interactions.
A common feature of oncogenic viruses is that they persist in the host as a latent or pseudo-latent infection that generally does not replicate to form infectious virus particles (also known as lytic replication) in tumor cells (Moore and Chang 2010). Latent infection serves as an immune evasion strategy that allows the virus to hide from the immune system by turning off unnecessary viral proteins that might be detected by cell-mediated immunity. When latent viruses switch to lytic infection, virus replication generates pathogen-associated molecular patterns that trigger DNA damage responses and innate immune signaling. These cellular responses result in death of the infected cell and release of infectious virions. Integration of small DNA tumor viruses, such as MCV, into the nascent tumor cell eliminates their ability to replicate as virions (a state of pseudo-latency). Monoclonal integration of viral DNA within individual tumors provides the primary evidence that MCV causes most cases of Merkel cell carcinoma (Moore and Chang 2010; Pastrana et al. 2009). These concepts are discussed further in the following sections.
4.3. Mode of Action and Evidence for Cancer Causation
As discussed in the Overview and Introduction Section, it is difficult to apply stringent criteria, such as Hill’s considerations, for determining that a human tumor virus is oncogenic (Moore and Chang 2010; zur Hausen 2001). Moore and Chang (2010) concluded that the MCV association with Merkel cell carcinoma could not be established strictly by using Hill’s epidemiological considerations for several reasons. First, MCV infection is ubiquitous while Merkel cell carcinoma is very rare and measurement of the total MCV burden does not reflect the tumor-causing form of the virus (i.e., the virus must be mutated and integrated into the host genome). Secondly, many studies report two clinical forms of Merkel cell carcinoma—one type that is MCV infected (predominant form) and one type that is not. These studies also suggest the MCV-positive cases have a better prognosis than MCV-negative cases. In addition to the usual criteria used by epidemiologists to determine causality, other factors, including molecular evidence, should be considered as proposed by IARC (1997) and zur Hausen (1994; 2001). The major lines of evidence supporting the role of MCV in Merkel cell carcinoma include the following: (1) the increased incidence of Merkel cell carcinoma in immunodeficient individuals indicates an infectious etiology, (2) the infectious agent was identified as MCV, (3) the MCV genome was monoclonally integrated in most Merkel cell carcinoma samples, (4) the integration event invariably was associated with truncating mutations in the large T antigen-coding sequence that lead to loss of the helicase domain but retention of the retinoblastoma binding domain and small T antigen coding, (5) expression of T antigens only in tumor cells in MCV-infected tumors, and (6) knockdown of T antigens leading to growth arrest and cell death in MCV-positive Merkel cell carcinoma samples (Gjoerup 2012). As a result, the available data provide strong support that MCV is an etiologic factor in most cases of Merkel cell carcinoma and are reviewed below.
4.3.1. Presence and Persistence of MCV in Merkel Cell Carcinoma
MCV has been detected in approximately 70% to 97% of Merkel cell carcinoma cases (Amber et al. 2013; Feng et al. 2008; IARC 2013; Rodig et al. 2012; Sihto et al. 2009; Spurgeon and Lambert 2013; Stakaitytė et al. 2014). The data suggest that Merkel cell carcinoma can develop through both a virus-mediated pathway in which MCV promotes tumorigenesis and possibly, in a minority of cases, a nonvirus-mediated pathway (Amber et al. 2013; Martel-Jantin et al. 2012). However, some have suggested that the presence of MCV in Merkel cell carcinoma is more common than reported and that improved detection methods might reveal that all Merkel cell carcinoma specimens contain MCV DNA (Rodig et al. 2012). Martel-Jantin et al. (2012) also reported that MCV detection was much higher in fresh-frozen biopsies than in formalin-fixed paraffin-embedded biopsies. Carter et al. (2009) reported that while 77% (24/31) of Merkel cell carcinoma samples were positive for MCV, 92% (22/24) of these patients were positive for antibodies to MCV. These results raise the possibility that MCV is involved in Merkel cell carcinoma initiation but may not be required to maintain the cancer phenotype and may explain why some Merkel cell carcinomas are negative for MCV DNA. Virus-negative carcinomas might indicate advanced tumor stages with a secondary loss of virus genomes (Niller et al. 2011). It is well documented that viral genomes, either inserted into the host cellular DNA or co-replicating with it in episomal form, can be subsequently lost from neoplastic cells (i.e., a “hit and run” mechanism). The number of copies of the MCV genome found integrated in Merkel cell carcinoma ranges widely from less than one to several thousand copies (Bhatia et al. 2010; DeCaprio and Garcea 2013; Laude et al. 2010; Rodig et al. 2012; Shuda et al. 2009).
4.3.2. Viral Oncogenes and Maintenance of the Malignant Phenotype
The MCV genome consists of about 5,400 base pairs that are divided into early and late coding regions separated by a noncoding regulatory region (see Section 1.1.2) (Chang and Moore 2012; Spurgeon and Lambert 2013; Stakaitytė et al. 2014). The early coding region expresses overlapping nonstructural transcripts from a single T antigen locus that are differentially spliced to form small T (sT) and large T (LT) antigens. The late region encodes MCV structural proteins and a miRNA (MCV-miR-M1-5p) that is encoded antisense to the LT coding region. The miRNA is thought to downregulate expression of the early genes and may have a role in cellular transformation (Lee et al. 2011; Seo et al. 2009). Viral miRNA was detected in half of MCV-positive tumors but was not detected in MCV-negative tumors (Lee et al. 2011).
The sT and LT antigens are critical for viral replication, manipulation of the host cell cycle, and cellular transformation (Amber et al. 2013; Angermeyer et al. 2013; Stakaitytė et al. 2014). About 75% of Merkel cell carcinoma tumor samples are positive for LT, while about 92% are positive for sT (Stakaitytė et al. 2014). In normal infections in permissive cells, MCV completes its replication cycle in the cell nucleus to form virions without inducing tumorigenesis. The T antigens are transcribed immediately upon entry into the nucleus of the host cell and induce the cell to enter S-phase. However, mutagenic events can cause MCV integration into host cell DNA and truncation of LT (see In vitro studies Section below). In these circumstances, expression of sT and mutated LT dysregulate cell proliferation and prevent apoptosis primarily through interactions with retinoblastoma and eukaryotic translation initiation factor 4E-binding protein 1 (4E-BP1) (Houben et al. 2012; Hughes and Gao 2013; Shuda et al. 2011). Expression of truncated LT also inhibits key responses to UV radiation-induced DNA damage (i.e., DNA repair and cell-cycle defects) and suggests that progressive MCV-mediated genomic instability contributes to Merkel cell carcinoma (Demetriou et al. 2012). This may explain why most cases of Merkel cell carcinoma occur on chronically sun-exposed skin. Mutational analyses have yet to identify other signature mutations in Merkel cell carcinoma (Erstad and Cusack 2014). However, Van Gele et al. (1998) reported that Merkel cell carcinoma cases showed a characteristic pattern of chromosomal gains and losses that were similar to that seen in small-cell lung carcinoma. The roles of LT and sT in cellular transformation and oncogenesis are discussed in the following sections.
In vivo Studies
Recent studies show that MCV T antigens have oncogenic activity in vivo in transgenic mice (Spurgeon et al. 2015; Verhaegen et al. 2015). Spurgeon et al. (2015) developed a mouse model that used keratin 14-mediated Cre recombinase-induced expression of MCV truncated LT and wild-type sT antigens in the skin (K14Cre-MCPyV168 mice). Expression of Merkel cell carcinoma tumor-derived MCV T antigens promoted hyperplasia, hyperkeratosis, acanthosis, and papilloma formation in the stratified epithelium of the skin with additional abnormalities occurring in footpads, whisker pads, and eyes. Evidence for neoplastic progression included increased cellular proliferation, unscheduled DNA synthesis, increased E2F-responsive genes, disrupted differentiation, and activation of DNA damage response. Similarly, Verhaegen et al. (2015) reported that expression of MCV sT antigen alone was sufficient for rapid neoplastic transformation in vivo in a panel of transgenic mouse models. sT antigen-expressing embryos exhibited hyperplasia, impaired differentiation, increased proliferation, apoptosis, and activation of a DNA damage response in epithelia. Mutation of the sT antigen-binding domain resulted in loss of transforming activity, thus, identifying this domain as critical for in vivo transformation. Mogha et al. (2010) demonstrated that simulated solar radiation caused a dose-dependent increase of sT antigen transcripts in human volunteers infected with variants of MCV in episomal form. These data might explain the association between Merkel cell carcinoma and UV exposure.
In vitro Studies
All LT sequences recovered from primary Merkel cell carcinoma tumors or tumor-derived cell lines harbor signature mutations (Borchert et al. 2014; Schmitt et al. 2012; Shuda et al. 2008; Stakaitytė et al. 2014). These mutations cause premature truncation of the entire C-terminal domain, which leads to the loss of domains associated with viral replication (i.e., origin binding domain and the ATPase/helicase region). Deletions of C-terminal LT sequences appear to be a highly specific surrogate marker for MCV-induced malignancy (Schmitt et al. 2012). Although the sites of mutations are randomly distributed from different tumors, the retinoblastoma-binding motif is preserved (Borchert et al. 2014; Shuda et al. 2008). Integration of MCV genomes with full-length LT capable of initiating host DNA replication would result in unlicensed replication, replication fork collision, DNA breakage, and cytopathic cell death (Shuda et al. 2008; Stakaitytė et al. 2014). Full-length MCV LT also showed a decreased potential to support cellular proliferation, focus formation, and anchorage-independent cell growth via activation of host DNA damage responses and upregulation of p53 downstream target genes (Li et al. 2013).
Infected cells containing a wild-type episomal MCV genome can be transformed into a tumor cell containing multiple copies of an integrated mutant viral genome via two distinct models (DeCaprio and Garcea 2013). LT truncation and amplification of viral genome copy number may occur before or after random integration into the host genome (Figure 4-1). If wild-type MCV is integrated into the host genome, then it must be followed by an LT mutation to disable viral replication. The integrated mutant genome could subsequently undergo copy number amplification. In cases where the LT mutation occurs first, the mutant genome could undergo rolling-circle amplification prior to integration (DeCaprio and Garcea 2013; Stakaitytė et al. 2014). Therefore, at least two mutation events are required prior to tumorigenesis and this may explain why Merkel cell carcinoma is rare. In either case, there is a strong selection pressure within the Merkel cell carcinoma tumors to eliminate viral replication capabilities and retain only replication-deficient copies of MCV.

Figure 4-1
Models of MCV-induced Cell Transformation
Cheng et al. (2013) also demonstrated that truncated LT was more efficient than wild-type LT at inducing cellular proliferation. Knockdown of T antigen expression in MCV-positive Merkel cell carcinoma cell lines induced cell-cycle arrest and apoptosis in vitro and regression of established xenograft tumors in vivo (Houben et al. 2012; Houben et al. 2010). These effects were largely due to the interaction of LT with retinoblastoma. LT also was required for MCV-positive Merkel cell carcinoma cell growth and survival (Angermeyer et al. 2013; Shuda et al. 2011). Borchert et al. (2014) reported that truncated LT antigens exhibit a very high binding affinity for retinoblastoma and that both wild-type and truncated LT antigens could transform baby rat kidney epithelial cells. However, truncated LT antigen did not bind to p53 or reduce p53-dependent transcription. Since the constructs used in this study were likely able to express both LT and sT antigens, sT might have contributed to the transformation events. Liu et al. (2011) also identified human Vam6p (hVam6p) cytoplasmic protein as a novel target for MCV LT. MCV LT translocates hVam6p to the nucleus, sequestering it from its normal function in lysosomal processing. Although this study suggested that hVam6p sequestration was more likely to play a role in MCV replication than in tumorigenesis, the data were insufficient to rule out possible contributions to cell growth and proliferation.
sT expression was sufficient to induce cell transformation, loss of contact inhibition, and anchorage-independent growth in rodent fibroblast, and serum independent growth in human fibroblasts (Angermeyer et al. 2013; Shuda et al. 2011). Silencing of sT expression by sT-specific short hairpin RNAs lead to variable degrees of growth retardation; however, these effects were not sT specific because MCV-negative cell lines were similarly affected. MCV sT-induced cell transformation may be mediated by reducing the turnover of hyperphosphorylated 4E-BP1 (a downstream component of the PI3K-Akt-mTOR signaling pathway) (Shuda et al. 2011; Stakaitytė et al. 2014). The PI3K-Akt-mTOR signaling cascade is an important pathway in cell-cycle regulation that is overactive in many cancers and is often targeted by oncogenic viruses. Hyperphosphorylation prevents 4E-BP1 from sequestering the eukaryotic cap-dependent translation initiation factor 4E (eIF4E), allowing free eIF4E to form the cap assembly on mRNA and initiate translation. eIF4E is part of a multisubunit eIF4F complex (composed of eIF4E, eIF4A, and eIF4G). Overexpression of eIF4F can induce cell transformation in rodent and human cells in vitro (Avdulov et al. 2004; Lazaris-Karatzas et al. 1990; Stakaitytė et al. 2014). An alternative pathway for regulation of cap-dependent translation during mitosis is through cyclin-dependent kinase 1 (CDK1) hyperphosphorylation of 4E-BP1 (Shuda et al. 2015). sT-induced cell transformation was reversed by expression of a constitutively active mutant 4E-BP1 protein that could not be inactivated by MCV sT (Stakaitytė et al. 2014). sT also contributes to LT expression by blocking proteasomal degradation of LT by the cellular SCFFbw7 E3 ligase (Kwun et al. 2015; Kwun et al. 2013). sT inhibits E3 ligase through its LT stabilization domain (LSD) and consequently stabilizes other cellular Fbw7 targets such as the cell-cycle regulators c-myc and cyclin E.
These data suggest a synergistic role for both sT and mutated LT antigens during Merkel cell carcinoma tumorigenesis (Borchert et al. 2014; Houben et al. 2012; Shuda et al. 2011). sT may be essential for initial cell transformation and stabilizing LT, while LT is necessary for subsequent survival and proliferation of transformed cells (Stakaitytė et al. 2014). Some of the primary molecular targets and biological effects associated with MCV LT and sT antigens are illustrated in Figure 4-2.

Figure 4-2
Molecular Targets and Biological Effects of MCV LT and sT Antigens
4.3.3. MCV as a Major Risk Factor for Merkel Cell Carcinoma
Although MCV infection is common, Merkel cell carcinoma is rare (Chang and Moore 2012). Early clinical findings identified immunosuppression as a major risk factor for Merkel cell carcinoma and pointed toward an infectious etiology. Epidemiological studies (see Section 3) support an association of Merkel cell carcinoma cases with MCV infection. MCV antibody levels are significantly higher in MCV-positive Merkel cell carcinoma cases compared with healthy controls that are MCV seropositive which suggests that development of Merkel cell carcinoma is preceded by an unusually robust MCV infection (Agelli et al. 2010; Pastrana et al. 2009). MCV also is monoclonally integrated into the host genome in Merkel cell carcinoma primary tumors and metastases providing strong evidence that viral infection precedes clonal expansion of the neoplastic cell (Feng et al. 2008; Laude et al. 2010). Viral genome integration is a typical feature of virus-mediated oncogenesis and refutes the possibility that MCV is merely a coincidental passenger infection in Merkel cell carcinoma (Chang and Moore 2012; Kuwamoto 2011). These data, combined with several studies showing that expression of MCV T antigens are required to sustain tumor growth (see Section 4.2.2), provide strong evidence that MCV is a major risk factor for Merkel cell carcinoma. The key events identified for MCV-induced Merkel cell carcinoma are shown in Figure 4-3.

Figure 4-3
Key Events Leading from MCV Infection to Merkel Cell Carcinoma
4.4. Synthesis
Human viral oncogenesis is a complex process that involves interactions among many viral, host, and environmental factors. Although MCV infection is common, very few people develop Merkel cell carcinoma. Therefore, as is the case with most oncogenic viruses, several cofactors are associated with a higher risk of developing Merkel cell carcinoma (e.g., immune suppression, chronic UV exposure, and advanced age). The key events associated with MCV-induced Merkel cell carcinoma cases include immunosuppression and immune evasion, monoclonal integration of the MCV genome and expression of T antigens in tumor cells, mutations causing truncation of the LT antigen, and dysregulation of cell-cycle control and apoptosis. The major lines of evidence linking MCV to Merkel cell carcinoma include the following:
- Immunosuppression is an important cofactor based on an increased risk of developing Merkel cell carcinoma in AIDS patients, organ transplant patients, and the elderly, which is consistent with an infectious etiology;
- MCV has been identified as an infectious agent in 80% or more of Merkel cell carcinoma cases;
- The MCV genome is monoclonally integrated in most Merkel cell carcinoma samples;
- A signature feature of MCV-positive Merkel cell carcinoma tumors is the presence of mutations that truncate the LT protein at its carboxy-terminus leading to loss of viral replication while preserving transforming activity;
- Molecular targets for truncated LT antigen include retinoblastoma and TLR9 that promote cell-cycle progression and disrupt immune signaling;
- Molecular targets for sT include 4E-BP1, NEMO, TLR9, and FBW7 that dysregulate CAP-dependent translation, downregulate NF-κB transcription, disrupt immune signaling, and upregulate c-myc and cyclin E;
- MCV T antigens transform cells in vitro and in vivo, are expressed only in tumor cells of MCV-infected tumors, and are required to maintain tumor growth and survival.
- Mechanisms and Other Relevant Data - Report on Carcinogens Monograph on Merkel C...Mechanisms and Other Relevant Data - Report on Carcinogens Monograph on Merkel Cell Polyomavirus
- Introduction and Methods - Report on Carcinogens Monograph on Merkel Cell Polyom...Introduction and Methods - Report on Carcinogens Monograph on Merkel Cell Polyomavirus
- Dinosperma erythrococcaDinosperma erythrococcabiosample
- RecName: Full=Destrin; AltName: Full=Actin-depolymerizing factor; Short=ADFRecName: Full=Destrin; AltName: Full=Actin-depolymerizing factor; Short=ADFgi|46577586|sp|P60981.3|DEST_HUMANProtein
Your browsing activity is empty.
Activity recording is turned off.
See more...