This is a work of the US government and distributed under the terms of the Public Domain
NCBI Bookshelf. A service of the National Library of Medicine, National Institutes of Health.
National Toxicology Program. Report on Carcinogens Monograph on Merkel Cell Polyomavirus: RoC Monograph 11 [Internet]. Research Triangle Park (NC): National Toxicology Program; 2016 Aug.
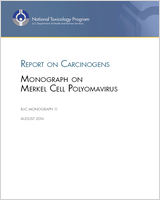
Report on Carcinogens Monograph on Merkel Cell Polyomavirus: RoC Monograph 11 [Internet].
Show detailsThis section reviews the biological properties (Section 1.1) and methods for detection (Section 1.2) of the Merkel cell polyomavirus (MCV, MCPyV). The material presented in Sections 1.1 and 1.2 is summarized in Section 1.3.
1.1. Biological Properties
1.1.1. Family and Type
The Merkel cell polyomavirus (MCV) was discovered in 2008 when non-human DNA was detected in human Merkel cell carcinoma cells. The novel sequences had high identity with other known polyomaviruses (IARC 2013; Spurgeon and Lambert 2013). Polyomaviruses were discovered in multiple rodent tumors in the 1950s, thus the term polyoma, meaning multiple tumors (Dalianis and Hirsch 2013; Moens et al. 2015). Polyomaviruses have also been found in birds, fish, cattle, and primates, humans included. MCV shares a high degree of similarity (50% nucleotide identity) with murine polyomavirus and other members of the recently proposed “Almipolyomavirus” genus (Carter et al. 2013; IARC 2013; Moens et al. 2015; Moore and Chang 2010; Spurgeon and Lambert 2013). MCV is more distantly related (35% nucleotide identity) to a cluster of highly related primate polyomaviruses that include simian virus 40 (SV40), African Green Monkey lymphotropic polyomavirus, BK polyomavirus (BKV), and JC polyomavirus (JCV). There are 13 polyomaviruses that infect humans, these include MCV, BKV, and JCV (Dalianis and Hirsch 2013; Moens et al. 2015). Serological and PCR-based studies indicate that, like other human polyomaviruses, MCV establishes a chronic lifelong infection in a large majority of healthy individuals (IARC 2013; Moore and Chang 2010). The skin appears to be a primary site of MCV infection.
1.1.2. Virus Structure and Genome
MCV is a non-enveloped virus (40 to 55 nm in diameter) composed of the capsid proteins, virus capsid protein 1 (VP1), 2 (VP2), and the genome (see Figure 1-1) (Dalianis and Hirsch 2013; IARC 2013; Spurgeon and Lambert 2013). The outside layer is composed of only VP1, which will spontaneously self-assemble into virus-like particles with icosahedral symmetry (IARC 2013). The minor capsid protein, VP, associates with pockets on the interior surface of VP1. The surface of the virion has a knobby appearance with indentations where the N- and C-termini of VP1 are located (Dalianis and Hirsch 2013; IARC 2013). These termini form disulfide bridges that give the virus stability, even after heating to 75°C (167°F) for an hour. This stability supports the idea that transmission could occur through environmental exposures, such as contact with sewage, rivers, and surfaces.

Figure 1-1
Merkel Cell Polyomavirus Particle
Within the capsid is a circular double-stranded (dsDNA) genome of about 5 kb that is wrapped around host cell-derived histones (Dalianis and Hirsch 2013; IARC 2013; Moens et al. 2015; Spurgeon and Lambert 2013). There is little genetic diversity among wild-type MCV, with isolates having genomes with >98.5% nucleotide identity. A single non-coding regulatory region, which contains the origin of replication as well as promoter and enhancer sequences for two flanking protein-coding regions. The two coding regions transcribe in opposite directions and are regulated temporally, with one coding region containing the early regulatory genes (LT, sT, alternate frame of the large T open reading frame [ALTO], 57kT) and the other containing late structural genes (VP1, VP2) which are created by alternative splicing of their respective early or late gene transcripts (Figure 1-2).

Figure 1-2
Genome Schematic
The early genes, LT and sT, regulate gene expression of viral and host genes, while the function of a multiply spliced LT isoform called 57kT (not shown in the figure) is not well understood (IARC 2013; Moens et al. 2015; Spurgeon and Lambert 2013). Besides the capsid proteins (VP1 and VP2), the late coding strand also expresses a regulatory micro-RNA (miRNA) (IARC 2013; Spurgeon and Lambert 2013). The 3' ends of both transcriptional units are separated by bi-directional polyadenylation sequences, which in the murine polyomaviruses and possibly MCV, cause inefficient termination. This allows for some transcripts to continue into the other transcription unit where the miRNA is located. The miRNA is 22 nucleotides long and appears to negatively regulate LT expression.
1.1.3. Replication
Entry of MCV into the cell requires receptor-mediated endocytosis using a cellular glycan receptor with at least one sialic acid residue binding to VP1 on the surface of the viral capsid and an interaction with heparan sulfate (IARC 2013) (see Figure 1-3). Although it is currently unclear how the non-enveloped MCV virion traverses host cell membranes, other polyomaviruses are thought to traffic to the endoplasmic reticulum, where host-cell chaperone proteins facilitate entry of the polyomavirus into the cytoplasm. The viral genome then enters the nucleus and early genes become expressed, using cellular transcription factors. The early genes promote viral DNA episomal replication. LT binds to the origin of replication, has helicase activity, and also binds host DNA polymerase alpha-primase to initiate viral DNA replication (IARC 2013; Moens et al. 2015; Spurgeon and Lambert 2013). While LT is necessary and sufficient to drive viral DNA replication, sT is not sufficient to drive replication, but may be essential for initial cell transformation and stabilizing LT (Stakaitytė et al. 2014). The ability of LT to bind host DNA polymerase alpha-primase is thought to play a major role in determining tissue and host tropism as the cellular receptors needed for endocytosis of the virus are commonly found on many types of cells. The LT early gene can also promote the expression of late genes, allowing for virus formation that can lead to cell lysis. The function of miRNA is believed to be to suppress LT expression and to keep the late and early phase gene expressions temporally separate. The expression of the late genes can become blocked causing the virus to enter a latent phase where a low copy number of viral DNA is maintained episomally and viral genes are not expressed. With little to no viral gene expression, the virus can evade immune detection. The regulation of latent or lytic phases of MCV infection are not fully understood.

Figure 1-3
MCV Infection and Replication Cycle
MCV DNA has been found integrated into the host DNA of 80% of Merkel cell carcinomas, where it is clonally passed along to daughter cells of both the primary tumor and metastatic tumors (Dalianis and Hirsch 2013; IARC 2013; Moens et al. 2015; Moore and Chang 2010). Integration is thought to occur by non-homologous recombination (Moore and Chang 2010). Because it is clonally expressed in Merkel cell carcinoma, its integration into the host genome is thought to be an early step in carcinogenesis. When integrated into the host genome, the helicase activity of LT would cause uncontrolled replication of the surrounding cellular chromosome, triggering rapid cell death. However, in tumors, integrated MCV genomes contain a truncated LT gene deficient in helicase activity and are unable to replicate, allowing for cell viability.
1.2. Detection
MCV exposure is commonly found in the general population, starting in newborns and increasing in prevalence as age increases, and at a high prevalence in Merkel cell carcinoma patients (Dalianis and Hirsch 2013; IARC 2013). MCV infections can be identified by detecting viral DNA and antibodies against MCV. These biomarkers can be examined in the blood, saliva, urine, or specific tissues.
1.2.1. Detection in Fluids
Antibodies
Detection of MCV antibodies in the blood can be achieved by several different immunoassay methods (IARC 2013; Xu et al. 2015). Anti-MCV antibodies are detected by enzyme-linked immunosorbent assays (ELISA), luminex-based multiplex serological assays using VP1 or VP1 plus VP2 virus-like particles (VLP) produced in insect cells, 299 TT cells, glutathione S-transferase (GST)-VP1 recombinant protein (capsomeres), or neutralization assays using MCV pseudovirions produced in human embryonic kidney 293TT cells (Coursaget et al. 2013). High levels of VP1 antibodies are seen in only 7% of people without Merkel cell carcinoma, but they are detected in 65% of Merkel cell carcinoma patients. However, serological tests to detect anti-VP1 antibodies are not equivalent; e.g., assays using VP1 monomers have been shown to underestimate MCV seroprevalence compared with assays using VLPs (Coursaget et al. 2013; Kean et al. 2009). Neutralization assays using MCV pseudovirions have been used to confirm the specificity of the MCV reactivity (Coursaget et al. 2013; Pastrana et al. 2009). Antibody assays have low cross reactivity for other polyomaviruses, like JCV, BKV, or lymphotrophic papovavirus. The level of antibodies correlates with the viral load on the skin and active viral shedding and increases in Merkel cell carcinoma patients.
Detection of antibodies in the blood against early gene products, such as LT or sT, are rare in MCV-infected people who do not have Merkel cell carcinoma (0.9%) compared with Merkel cell carcinoma patients (41%) (Dalianis and Hirsch 2013; IARC 2013). The levels of LT and sT antibodies in patients with Merkel cell carcinoma change with the severity of the cancer and could be used to predict prognosis (IARC 2013).
DNA
MCV DNA can be detected in the blood, saliva, or urine by PCR, nested PCR, real-time PCR, quantitative PCR, and rolling circle amplification (IARC 2013). Common MCV-specific genes used for detection include early genes, LT and sT as well as the late gene VP1. Detection of MCV DNA in bodily fluids indicates an active infection, but will not clearly identify the tissue that is infected (IARC 2013; Xu et al. 2015).
1.2.2. Detection in Cells
Antigens
Antibodies specific for MCV early gene products, sT and LT, have identified MCV by immunohistochemical staining in Merkel cell carcinoma cells and tumor biopsy specimens, producing similar findings as DNA detection (IARC 2013). Viral LT protein expression in Merkel cell carcinoma was demonstrated using monoclonal antibody to a conserved epitope of LT. sT antigen is found in Merkel cell carcinoma more often than LT, and some Merkel cell carcinomas express sT without detectable LT. It appears that Merkel cell carcinoma patients whose tumors score robustly positive for T antigen expression have better survival than MCV-negative Merkel cell carcinoma patients (Moore and Chang 2014; Paulson et al. 2010). Therefore, antibodies to sT and LT could be used to predict the prognosis of Merkel cell carcinoma patients.
DNA
MCV DNA can be detected in tissues and tumor specimens (Dalianis and Hirsch 2013; IARC 2013; Moens et al. 2015; Moore and Chang 2010). PCR can be used on tissues that have been fixed in formaldehyde and embedded in paraffin, though formaldehyde can lead to DNA fragmentation and may give misleading results in tissues with very low viral loads. Therefore, DNA in fixed tissue might not accurately indicate viral load.
Specific MCV genes (LT and miRNA) have been used for DNA detection in tissue. MCV DNA has been detected in about 80% of Merkel cell carcinoma tumors and most have a truncated LT gene (Dalianis and Hirsch 2013; Moens et al. 2015; Moore and Chang 2010). Since a truncated LT gene that lacks helicase activity is needed for stable host genomic integration and carcinogenesis, the detection of truncated LT gene might be a biomarker specific for carcinogenic risk. The miRNA has been found in about 50% of Merkel cell carcinoma patients and the level of expression correlates with the number of copies of MCV DNA.
1.3. Summary
Merkel cell polyomavirus is a very stable non-enveloped DNA virus found in the skin and integrated into the genome of most Merkel cell carcinomas. Once MCV enters a host cell, its genome is maintained in a form that allows it either to replicate independently or to integrate into the host cell’s genetic material for replication. MCV can exist in either a lytic phase (in which the infected cell is destroyed and viral particles are released) or a latent phase (in which the virus does not replicate). During the latent phase, little viral gene expression occurs, and the virus can evade immune detection. MCV establishes a chronic lifelong infection in a large majority of healthy individuals. The skin appears to be a primary site of MCV infection, and healthy individuals have been shown to chronically shed MCV DNA from the skin surface (Schowalter et al. 2010). MCV has also been reported to infect saliva and mouth, esophagus, and colon (Loyo et al. 2010). MCV is stable at temperatures up to 167°F, so infection can occur from contact with the virus left on surfaces or in water.
- Properties and Detection - Report on Carcinogens Monograph on Merkel Cell Polyom...Properties and Detection - Report on Carcinogens Monograph on Merkel Cell Polyomavirus
Your browsing activity is empty.
Activity recording is turned off.
See more...