NCBI Bookshelf. A service of the National Library of Medicine, National Institutes of Health.
National Cancer Institute’s Nanotechnology Characterization Laboratory Assay Cascade Protocols [Internet]. Bethesda (MD): National Cancer Institute (US); 2005 May 1-. doi: 10.17917/W63A-GR02

National Cancer Institute’s Nanotechnology Characterization Laboratory Assay Cascade Protocols [Internet].
Show detailsIntroduction
Novel nanoformulated chemotherapeutics and diagnostics require demonstration of efficacy and safety in appropriate animal models prior to clinical translation of the concept. Pharmacokinetic studies are also important to optimize dosing regimen and dose escalation strategy, and identify potential species differences. NCL’s in vivo experiments are tailored specifically to the individual nanoparticle under study. As such, a standard experimental protocol cannot be generated. Instead of a protocol, we offer the following general guidance for experimental design and data analysis. The following description is not a protocol and does not describe all of the relevant experimental parameters necessary to conduct the experiment. If you would like additional information, please feel free to contact us.
Note : FNLCR is accredited by AAALAC International and follows the Public Health Service Policy for the Care and Use of Laboratory Animals (Health Research Extension Act of 1985, Public Law 99-158, 1986). Animal care is provided in accordance with the procedures outlined in the Guide for Care and Use of Laboratory Animals (National Research Council, 1996; National Academy Press, Washington, D.C.). All animal protocols are approved by the FNL Institutional Animal Care and Use Committee (IACUC). Any experiments performed are scientifically justified and are not an unnecessary duplication of previous work by others.
Efficacy Studies
1. NCL Capabilities: In Vivo Efficacy Studies Utilizing Rodent Models
In vivo efficacy studies at NCL are performed in collaboration with FNL’s Laboratory Animal Science Program. NCL efficacy studies utilize transgenic, chemically induced, syngeneic, or xenograft tumor models to provide independent verification of collaborators’ proof-of-concept studies. Here are some of the models we have experience with:
- Tumor Models
- Subcutaneous Models:
- LS174T colon cancer model
- MDA-MB-231 breast cancer model
- MCF-7 estrogen-dependent breast cancer model
- LOX IMVI melanoma model
- HCT116 colon cancer model
- We also have access to the following xenografts under our current animal study protocols (ASP): B16, BALB/3T3, NIH/3T3, N87, SKOV3, KB-DTP, KB-ATCC, and UM SCC-81B. We can obtain approval for other cell lines, but this process can take several weeks.
- Orthotopic Models:
- MDA-MB-231 breast cancer model
- 4T1 breast cancer model
- Intracranial injection of U87 (human)/ TRE (murine) glioma cell lines (in collaboration with FNL’s Center for Advance Preclinical Research (CAPR))
- Metastatic Models:
- Metastasis of mammary pad MDA-MB-231-Luc/4T1-Luc cell line to lymph node and lung
- Metastasis of intradermal B16-F10 to lymph node
- Lung metastasis model (via intravenous injection of B16-F10-Luc cancer cell line)
- Peritoneal cavity metastasis model (via intraperitoneal injection of ID8-Luc ovarian cancer cell line)
- Metastasis to the brain (via left ventricle injection of brain seeking MDA-MB-231 Br breast cancer cell line)
- Chemically Induced Tumor Model:
- Colon cancer induced by chemical treatment with azoxymethane & dextran sulfate salt
- Transgenic Models (in collaboration with CAPR):
- Glioblastoma model (pRb/Kras/PTEN; Tamoxifen/Adeno-Cre; orthotopic)
- Lung cancer model (EGFR-L858R/T790M; KrasG12D/Lkb1; KrasG12D/p53EML4-ALK)
- Pancreatic ductal adenocancer model (KrasG12D/p53)
- Serous ovarian carcinoma (pRb/p53c/c or m/-;pRb/p53c/c or m/- /Brca1;prb/p53c/c or m/- /Brca2)
- Melanoma (HGF/MET; BRAFV600E)
- ImagingIn collaboration with FNL’s Small Animal Imaging Program (SAIP), NCL has the capability to conduct efficacy studies in orthotopic and metastatic tumor models and noninvasively image tumor growth and measure therapeutic response using various imaging modalities, including bioluminescence and fluorescence imaging, PET/SPECT/CT, MRI, and ultrasound. Both in vivo/in situ and ex vivo/individual organ imaging is available upon request. In addition, efficacy of novel diagnostic or theragnostic nanoconstructs containing contrast agents can also be assessed utilizing the above-mentioned capabilities available at the SAIP.
2. General Guidelines for Performing an Efficacy Study
To determine the maximum tolerated dose and optimal dosing regimen of a therapeutic agent, it is ideal to perform dose-range finding studies and preliminary pharmacokinetic evaluation before conducting an in vivo efficacy experiment. These studies assist in estimating appropriate dosing schedules to attain therapeutic concentrations, while avoiding high toxic concentrations.
All cancer cell lines should be tested for human and rodent pathogens (HIV-1, HIV-2, HTLV-1, HTLV-2, Hepatitis B, Hepatitis C, CMV, EBV, JCV, and MoMuLV) prior to use in animal models, and animal study protocols (ASP) should be approved by an Institutional Animal Care and Use Committee (IACUC) prior to initiation of an animal study.
Appropriate control agents should be included in the study, such as standard-of-care treatment for a particular cancer type, free (unformulated, legacy) drug control, vehicle (formulation) control, or unloaded nanoparticle control (where applicable). Cytotoxic nanoformulations and comparator treatments should be dosed at equivalent doses and equitoxic MTD doses (Table 1; MTD defined as dose producing >20% body weight loss in 10% of animals). Doses should be based on initial dose-range finding study. An example of efficacy study design is shown below. Investigational nanoformulated API at two concentrations are compared with clinical formulation of API at an equitoxic and equivalent doses to reasonably estimate the therapeutic window of the nanoformulated API. If the nanoformulation has an attached targeting ligand, the inclusion of an untargeted nanoformulation will help identify targeting advantages. If the nanoformulation is a species-specific gene therapy (e.g. siRNA, plasmid DNA, antisense RNA, etc.), or contains species specific antibodies or ligands, utilizing a mouse ortholog of the relevant gene, antibody or ligand is important to allow assessment of side effects related to manipulation of the gene/protein/receptor in off target tissues. All preclinical studies should be performed using the clinical route and regimen of administration to best represent eventual clinical drug distribution and related efficacy and toxicities.
Depending on the cancer cell line and mouse strain, the tumor take rate and growth profile can vary significantly. A pilot tumor growth study is recommended to characterize the take rate and tumor growth profile prior to therapeutic evaluation. Once the tumors are of palpable size, the animals are randomized based on tumor volume and weight (Tables 2 and 3). The randomization process will remove any tumor size or body weight bias so the average tumor volume between treatment groups is not significant. The route of drug administration should reflect the anticipated clinical route of administration and regimen. The number of animals per treatment group required to achieve statistical power to detect a significance difference for a given treatment depends on the variability in tumor growth/survival and magnitude of the treatment response. Therefore, it is necessary to perform a pilot study to identify the variability in tumor growth/survival and anticipated magnitude of the treatment response. Assuming type I error (alpha) and type II error (Beta) are fixed at 5% (p<0.05) and <20%, respectively, then a simplified estimate of sample size for a tumor volume data (continuous variable) is given by [1]:
A simplified estimate of sample size for survival data (dichotomous variable) is given by:
The sample size based on the above calculations are generally not greater than 10 animals for an implanted tumor model with a potent treatment response.
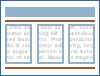
Table 2
Example of Successful Animal Randomization by Tumor Volume.
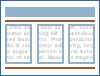
Table 3
Example of Successful Animal Randomizatio.
3. General Guidelines for Data Analysis From an Efficacy Study
Trends can be observed by plotting tumor volume and body weight data as mean ± standard deviation or as percent change from initial volume/body weight (100%). Statistical differences in tumor volume and body weight values between groups can be determined using ANOVA, with appropriate post-hoc comparisons. Group time-to-endpoint or survival data are commonly analyzed by the Kaplan-Meier analysis, with the log-rank (Mantel-Cox) test to determine statistical significance.
Tumor volume and body weight analysis (Table 4) for study time points which contain equal numbers of animals in each group can be analyzed by ANOVA, with post-hoc comparison using either Dunnett’s Test for statistical significance in comparison to the control (Table 5) or the Duncan’s Test for statistical significance between all treatment groups (Table 6).
For study time points which contain unequal numbers of animals, tumor volume and body weight measurements over the study can be analyzed for statistical significance using ANOVA, with post-hoc comparisons by Tukey’s HSD Test (Table 7). Unequal sample sizes are often observed in survival studies, or time-to-endpoint studies in which animals are terminated at set neoplasia (tumor diameter reaching ≥2 cm or tumor ulceration) or morbidity (loss of ≥20% of an initial body weight and or immobility) criteria, as opposed to a set termination day. In this case, the number of animals per treatment group will vary over the course of the study due to differences in animal survival, or time to reach neoplasia or morbidity-related endpoints.
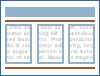
Table 4
Example of Tumor Volume and Animal Body Weight Data. Tumor volume and body weight data for all the treatment groups are shown below and statistical analysis was conducted on this dataset (Tables 5 and 6 show statistical outputs).
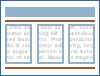
Table 5
Example of Statistical Output Using Dunnett’s Test (significance in comparison to control group).
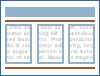
Table 6
Example of Statistical Output Using Duncan’s Test (significance in comparison to other treatment groups).
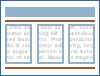
Table 7
Example of Statistical Output Using Tukey HSD Test.
Kaplan-Meier analyses of time-to-endpoint and survival data allows for censoring of data. Censoring of non-neoplasia-related endpoints, such as body weight endpoint (loss of ≥20% of an initial body weight) data, eliminates drug-related endpoints from the analysis of neoplasia-related endpoints (tumor diameter reaching ≥2 cm or tumor ulceration) (Table 8; Figure 1).
Toxicity Studies
1. NCL Capabilities: Single or Repeat-Dose Toxicity Studies in Rodents
In vivo toxicity studies at NCL are performed in collaboration with FNL’s Laboratory Animal Science Program. Each NCL in vivo study is tailored to the particular compound being tested, so each toxicity study is different, but in general we conduct single or repeat-dose toxicity tests, generally in both male and female rats or mice, generally by i.v. administration via tail vein injection. We monitor the animals usually for up to 14-days post dose, although sometimes longer if we expect delayed toxicities (28 days for immunotoxicity) or to accommodate longer treatment regimens (Table 9). We look for in-life changes in body weight or animal behavior, or changes, upon comprehensive necropsy, in organ weight, gross pathology, clinical chemistry, hematology, and histology of treatment groups relative to free/active drug, and vehicle or blank nanomedicine control groups. We evaluate major tissues (see list below) by gross pathology and histology, and conduct statistical analysis of lesion incidence and severity to determine differences between treatment and control groups. Advanced histological techniques such as special stains, immunohistochemistry, and in situ hybridization assays are also available, when applicable. We also conduct statistical analysis of body weight, organ weight (absolute, and body and brain weight normalized), and clinical chemistry and hematology parameters (see list below) to determine differences between groups. Regardless of the stealth properties of the nanomedicine, such as a PEGylated surface, there is often some distribution to organs of the RES, such as liver, spleen, lung, kidney, and these organs should receive special consideration in nanomedicine toxicity studies. There is concern about the long-term consequences of RES accumulation for non-biodegradable platforms, such as carbon-based, metallic and polymeric nanoparticles. In the case of selective distribution, such as RES sequestration or selective distribution of targeted nanoparticle, it may be necessary to perform repeat dose tissue distribution studies to identify accumulation [2].
Tissues Evaluated by Histopathology
- adrenal
- brain
- cecum
- coagulating gland
- colon
- duodenum
- epididymis
- esophagus
- eye
- femoral artery
- femur
- gall bladder
- Harderian gland
- heart
- ileum
- jejunum
- kidney
- liver
- lung
- lymph node
- mammary gland
- muscle
- nasal sections
- ovary
- pancreas
- parathyroid
- pituitary
- prostate
- rectum
- salivary gland
- seminal vesicle
- skin/subcutis
- spinal cord
- spleen
- stomach (glandular/nonglandular)
- testis
- thymus
- thyroid
- tongue
- trachea
- urinary bladder
- uterus
- vertebra
- and any additional tissue with gross findings at necropsy
Hematology Parameters Evaluated
- blood smear
- differential leukocyte count (NE, LY, MO, EO, BA)
- erythrocyte count (RBC)
- hematocrit (HCT)
- hemoglobin (Hb)
- mean corpuscular hemoglobin (MCH)
- mean corpuscular hemoglobin concentration (MCHC)
- mean corpuscular volume (MCV)
- nucleated red blood cell count
- red blood cell distribution width (RDW)
- platelet count (PLT)
- mean platelet volume (MPV)
- reticulocyte count (RETIC)
- total leukocyte count (WBC)
Clinical Chemistry Parameters Evaluated
- alanine aminotransferase (ALT)
- albumin (ALB)
- albumin/globulin ratio (A/G)
- alkaline phosphatase (ALP)
- amylase (AMS)
- bilirubin, total (TBIL)
- blood urea nitrogen (BUN)
- calcium (Ca)
- chloride (Cl)
- creatinine (Cr)
- globulin (GLOB)
- glucose (GLUC)
- phosphate (PHOS)
- potassium (K)
- sodium (Na)
- total protein (TP)
2. General Guidelines for Performing a Toxicity Study
The doses used in the study depend on the expected clinical therapeutic dose, the available material, and the amount of material we can feasibly administer based on drug formulation concentration and administered volume limited by route. Ideally, we test the expected clinical dose (scaled by body weight or surface area to rats) and 10- and 100-fold times that dose. All NCL studies are conducted under non-GLP conditions, but ICH guidance indicates that non-GLP acute toxicity studies can be sufficient for an IND inclusion as long as repeat dose toxicity studies are conducted under GLP conditions.
Pharmacokinetic Studies
1. NCL Capabilities: Pharmacokinetic Studies in Rodents
The ability of nanoformulations to alter drug pharmacokinetics and tissue distribution, and thereby change drug efficacy and toxicity, is the most fundamental concept in the field of nanomedicine. Thus, understanding the pharmacokinetics and biodistribution of nanomedicines is very important, because it allows for formulation optimization, selection of dose and dosing regimens, establishment of PKPD relationships, and identification of likely target organs of toxicity to concentrate on in toxicity studies. The FDA has recommended that nanomedicines undergo comparative pharmacokinetic and mass balance studies with marketed formulations in order to identify differences in drug disposition [3], and we will often compare the nanoformulated drug to the conventionally formulated, marketed version of the drug.
We typically conduct pharmacokinetic, and biodistribution studies, in male double-jugular-catheterized Sprague Dawley rats, although we have also conducted studies in intact (non-catheterized) mice when appropriate (Tables 10 and 11). Catheters allow for accurate dose administration and easy blood draws. We choose rats as our primary model, as rats are the most common rodent species used in IND-enabling GLP toxicity and toxicokinetic studies. Rats also allow for more blood volume to be removed than mice, and mouse PK studies often utilize a single mouse per time point as opposed to multiple blood draws from the same mouse Although techniques like “tail tipping” or submandibular vein collection can allow for multiple murine blood collections, the actual resulting plasma volumes can be quite small, ~50 μL, and this can limit bioanalytical assay sensitivity. It is, however, important to note that mouse PK studies, with a single time point per mouse, does allow for tissue collection at each time point as well, and this would be advantageous for a combined PK biodistribution study. Also, importantly, as the average mouse weighs 1/10th the average rat, even if you have to dose one mouse per time point, the overall dose would be the same assuming 10 time points and the same animal number, N, at each time point. The primary draw back with the one mouse per time point study is the data at each time point will be more variable than if the data had come from a rat study with multiple time points from the same rat.
2. General Guidelines for Performing a Pharmacokinetic Study
The dose for a PK study should be non-toxic (unless this is a toxicokinetic study to relate toxicity to drug exposure as part of a toxicity study), but high enough to allow for drug quantification, which is dependent on the bioanalytical assay sensitivity. Additionally, pharmacokinetic studies should use at least two doses, 5-10 fold apart, in order to estimate the linearity of the pharmacokinetics with respect to dose.
When selecting time points for the study, it is important to try to capture all the distribution phases, absorption (if extravascular route), tissue distribution and terminal elimination. A good rule of thumb is that the area under the time concentration curve all time points, AUCall, should be within 15% of the extrapolated to time infinity AUC, AUCinf; this requires that sufficient time points be selected at the terminal phase to cover the majority of that phase. Capturing each phase sufficiently with the time points selected will also allow better estimation of the slopes of these phases and allow determination if there are multiple phases for each process, such as two tissue distribution phases, as can often occur.
The primary complexity of nanomedicine pharmacokinetics in comparison to conventional drug formulation, is the necessity to measure both the nanomedicine-encapsulated drug, i.e. the encapsulated drug fraction, as well as the unencapsulated drug fraction. All too often, nanomedicine pharmacokinetic studies only measure the total drug systemically, or in tissue, which is a composite of both the encapsulated and unencapsulated drug. Generally, for a stable nanomedicine drug complex, the blood/plasma and tissue will be dominated by the encapsulated drug fraction, which will be primarily represented in the total drug concentration estimate.
Methods to fractionate PK samples and allow determination of encapsulated and unencapsulated drug include dialysis, liquid-liquid extraction, solid phase extraction, size exclusion chromatography, and ultrafiltration (for review see [4]). The method most often used by our laboratory, which was invented in our lab, is the stable isotope tracer ultrafiltration assay, or SITUA [5]. Apart from these methods to measure drug fractions, dual labeling, and complementary and orthogonal techniques to measure both drug and nanoplatform have also been used to determine platform stability and drug release, and estimate encapsulated and unencapsulated drug fractions. These alternative methods include dual labeling and modeling to estimate drug fractions; dual labels can include radioactive of fluorescent labels, such as tritium labeled platform and 14C labeled drug. Similar PK of the nanoplatform and drug labels would suggest platform stability, while disparate PK would suggest instability [6].
Complementary analysis involves complementary techniques, such as quantitative chemical and/or biochemical analysis, for measurement of the platform and payload, such as enzyme linked immunosorbent assay (ELISA) to measure a biological payload and inductively coupled plasma mass spectrometry (ICP-MS) measure a metallic platform. By modeling disparate platform and drug profiles, with knowledge of the free drug PK, the encapsulated and unencapsulated drug profiles can be estimated, as well as the drug release kinetics [7]. Modeling and simulation of nanomedicine metabolite data can also be used to estimate encapsulated and unencapsulated drug profiles [8].
References
- 1.
- H. Shah, How to calculate sample size in animal studies? Natl J Physiol Pharm Pharmacol. 1 (2015) 35–39.
- 2.
- Guideline for Industry, Pharmacokinetics: Guidance for Repeated Dose Tissue Distribution Studies. U.S. Department of Health and Human Services, Food and Drug Administration, Center for Drug Evaluation and Research (CDER); Rockville, MD: Mar 1995. Link.
- 3.
- Guidance for Industry, Liposome Drug Products: Chemistry, Manufacturing, and Controls; Human Pharmacokinetics and Bioavailability; and Labeling Documentation. U.S. Department of Health and Human Services, Food and Drug Administration, Center for Drug Evaluation and Research (CDER); Rockville, MD: Apr 2018. Link.
- 4.
- V.V. Ambardekar, S.T. Stern, NBCD Pharmacokinetics and Drug Release Methods, in: D.J.A. Crommelin, J.S.B. de Vlieger (Eds.) Non-Biological Complex Drugs; The Science and the Regulatory Landscape, Springer International Publishing 2015, pp. 261–287.
- 5.
- S. Skoczen, S.E. McNeil, S.T. Stern, Stable isotope method to measure drug release from nanomedicines, J Control Release, 220 (2015) 169–174. [PMC free article: PMC4688069] [PubMed: 26596375]
- 6.
- B.S. Zolnik, S.T. Stern, J.M. Kaiser, Y. Heakal, J.D. Clogston, M. Kester, S.E. McNeil, Rapid distribution of liposomal short-chain ceramide in vitro and in vivo, Drug Metab Dispos, 36 (2008) 1709–1715. [PubMed: 18490436]
- 7.
- S.T. Stern, J.B. Hall, L.L. Yu, L.J. Wood, G.F. Paciotti, L. Tamarkin, S.E. Long, S.E. McNeil, Translational considerations for cancer nanomedicine, J Control Release, 146 (2010) 164–174. [PMC free article: PMC2921639] [PubMed: 20385183]
- 8.
- S.T. Stern, P. Zou, S. Skoczen, S. Xie, B. Liboiron, T. Harasym, P. Tardi, L.D. Mayer, S.E. McNeil, Prediction of nanoparticle prodrug metabolism by pharmacokinetic modeling of biliary excretion, J Control Release, 172 (2013) 558–567. [PMC free article: PMC3788091] [PubMed: 23664969]
- ContactFor more information or to discuss questions pertaining to your specific nanoformulation, please reach out to NCL’s Head of Pharmacology & Toxicology, Dr. Stephan Stern:Stephan T. Stern, PhD, DABTDirector of Research & Development, Head of Pharmacology & Toxicology SectionNanotechnology Characterization Laboratory
- Folic acid supplementation and malaria susceptibility and severity among people taking antifolate antimalarial drugs in endemic areas.[Cochrane Database Syst Rev. 2022]Folic acid supplementation and malaria susceptibility and severity among people taking antifolate antimalarial drugs in endemic areas.Crider K, Williams J, Qi YP, Gutman J, Yeung L, Mai C, Finkelstain J, Mehta S, Pons-Duran C, Menéndez C, et al. Cochrane Database Syst Rev. 2022 Feb 1; 2(2022). Epub 2022 Feb 1.
- Designing an In Vivo Efficacy Study of Nanomedicines for Preclinical Tumor Growth Inhibition.[Methods Mol Biol. 2018]Designing an In Vivo Efficacy Study of Nanomedicines for Preclinical Tumor Growth Inhibition.Adiseshaiah PP, Stern ST. Methods Mol Biol. 2018; 1682:241-253.
- Planning Implications Related to Sterilization-Sensitive Science Investigations Associated with Mars Sample Return (MSR).[Astrobiology. 2022]Planning Implications Related to Sterilization-Sensitive Science Investigations Associated with Mars Sample Return (MSR).Velbel MA, Cockell CS, Glavin DP, Marty B, Regberg AB, Smith AL, Tosca NJ, Wadhwa M, Kminek G, Meyer MA, et al. Astrobiology. 2022 Jun; 22(S1):S112-S164. Epub 2022 May 19.
- Review Safety and nutritional assessment of GM plants and derived food and feed: the role of animal feeding trials.[Food Chem Toxicol. 2008]Review Safety and nutritional assessment of GM plants and derived food and feed: the role of animal feeding trials.EFSA GMO Panel Working Group on Animal Feeding Trials. Food Chem Toxicol. 2008 Mar; 46 Suppl 1:S2-70. Epub 2008 Feb 13.
- Review The use of pharmacokinetics as an interpretive and predictive tool in chemical toxicology testing and risk assessment: a position paper on the appropriate use of pharmacokinetics in chemical toxicology.[Regul Toxicol Pharmacol. 1994]Review The use of pharmacokinetics as an interpretive and predictive tool in chemical toxicology testing and risk assessment: a position paper on the appropriate use of pharmacokinetics in chemical toxicology.Frantz SW, Beatty PW, English JC, Hundley SG, Wilson AG. Regul Toxicol Pharmacol. 1994 Jun; 19(3):317-37.
- Guide to NCL In Vivo Studies: Efficacy, Pharmacokinetics & Toxicology - National...Guide to NCL In Vivo Studies: Efficacy, Pharmacokinetics & Toxicology - National Cancer Institute’s Nanotechnology Characterization Laboratory Assay Cascade Protocols
- Ultrafiltration Drug Release Assay Utilizing a Stable Isotope Tracer - National ...Ultrafiltration Drug Release Assay Utilizing a Stable Isotope Tracer - National Cancer Institute’s Nanotechnology Characterization Laboratory Assay Cascade Protocols
- beta-defensin 129 precursor [Mus musculus]beta-defensin 129 precursor [Mus musculus]gi|83776583|ref|NP_001033022.1|Protein
- Mus musculus zinc finger, CCHC domain containing 14, mRNA (cDNA clone IMAGE:3153...Mus musculus zinc finger, CCHC domain containing 14, mRNA (cDNA clone IMAGE:3153047), complete cdsgi|13542974|gb|BC005674.1|Nucleotide
Your browsing activity is empty.
Activity recording is turned off.
See more...