NCBI Bookshelf. A service of the National Library of Medicine, National Institutes of Health.
National Research Council (US) Panel on Effects of Past Global Change on Life. Effects of Past Global Change on Life. Washington (DC): National Academies Press (US); 1995.
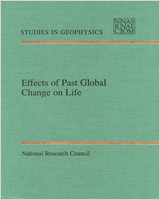
Effects of Past Global Change on Life.
Show detailsWilliam A. Dimichele
National Museum of Natural History, Smithsonian Institution
Tom L. Phillips
University of Illinois
Abstract
Carboniferous coal-forming swamps are an excellent system in which to evaluate the effects of regional to global climatic changes on ecosystem structure and dynamics. Stressful physical conditions restrict the access of most species, creating semiclosed conditions in which the signal-to-noise ratio should be high. We examine patterns of change in coal-swamp systems during the Pennsylvanian at three levels: landscapes, habitats within landscapes, and species within habitats. The timing and extent of turnover at these three levels suggest a hierarchial organization, in the sense that patterns at one level emerge from interactions among elements at a lower level, and can have subsequent reciprocal effects on the dynamics at that lower level.
Changes in the species composition of coals, and in the dominance-diversity structure they define, occur continuously throughout the Pennsylvanian. However, there are distinct breakpoints that allow us to recognize five basic organizational themes. Each of these breakpoints corresponds to an independently inferred change in regional or global climate. Examination of species-level turnover patterns reveals highest values at the landscape-level breakpoints, suggesting at first that climate change may be affecting species turnover, which then scales upward directly into landscape changes. Estimation of the relationship between species turnover and habitat patterns, however, suggests an intermediate level of organization. Species turnover throughout the Westphalian occurs mostly within habitats and on strongly ecomorphic themes; species of the same or closely related and morphologically similar genera tend to replicate each other through time. It is the proportion of habitats that changes at the landscape-breakpoint boundaries, and habitats contain the ecomorphic elements that give the landscape its apparent dominance-diversity structure.
At the Westphalian-Stephanian boundary, high levels of extinction eliminated sufficient numbers of species that Westphalian ecomorphic patterns were destroyed and a new set of species-habitat dynamics was established. In combination with replacement patterns during the Westphalian, the extinction suggests that biotic interactions among species assist in creating a "fabric" or multiniche system that helps constrain the ecomorphic nature of species replacements, whether such replacements occur through evolution within or migration into the system. Loss or decline of a species creates a vacant niche, whose limits are partially defined by the remaining biota. Major disruption of this system by extrinsically induced extinction permits the system to reestablish the interaction fabric based on the biologies of the new suite of species.
Introduction
One of the most important tenets of conventional wisdom currently dominant in plant community ecology is the concept of individualism. This is a highly reductionist principle that attributes all apparent structure in plant communities to the properties of individual organisms; associations of species are a consequence only of their similar tolerances to physical conditions and similar resource requirements. Such a view allows no "emergent" properties, characteristics of ecosystems that result from the interactions of coexisting species populations (avatars in the terminology of Damuth, 1985). Some plant ecologists have turned to the fossil record, almost exclusively that of Quaternary pollen, to test the predictions of this hypothesis when dynamics are viewed over a longer time scale (e.g., Delcourt and Delcourt, 1987; Overpeck et al., 1992) and have found much congruence. Consequently, the pollen record of the past 10,000 yr has come to stand as proxy for the past 420 million years (m.y.) of terrestrial plant history. What about the rest of the record?
Most of the terrestrial fossil record is less complete than that of the Quaternary. This is not to imply that it cannot be sampled at the same scale. It can, as studies of spores and pollen from very ancient sediments indicate (e.g., Smith and Butterworth, 1967; Mahaffy, 1985; Farley, 1989; Eble and Grady, 1993). The major difference lies in our understanding of contemporaneous, potentially causative changes in climate and paleogeography, resolved much more coarsely as one looks deeper into time. Yet the older record preserves patterns of change over a much broader span of time intervals than 10,000 to 100,000 yr.
Where studied in detail, the past does not reveal a pattern of ever-changing community structure and composition. Rather, remarkable periods of persistence of species associations and ecomorphic patterns are found, in which species turnover may occur but structure and dynamics remain largely unchanged for a few to many millions of years. Examples do not abound because little detailed research on community paleoecological patterns has been pursued outside the Late Carboniferous (e.g.; Scott, 1978; Phillips and DiMichele, 1981; Gastaldo, 1987; Raymond, 1988) or the early Tertiary (e.g., Wing, 1988, Farley, 1989). However, these vastly different ecosystems show remarkably similar patterns and suggest that the Quaternary record cannot be used reliably as a blanket model of terrestrial plant community dynamics through time.
In this chapter we focus on changes in the taxonomic composition and structural properties of late Paleozoic plant communities from wetland habitats, in particular those of peat-forming swamps. Peat-forming communities of the Late Carboniferous (Pennsylvanian) provide some of the best opportunities to characterize and analyze an ancient ecosystem. Each of the five major plant groups (lycopsids, ferns, sphenopsids, pteridosperms, and cordaites) is highly distinctive morphologically and anatomically, the generic and species diversities are quite low, and taxa were largely pantropical in distribution within the wetlands. Particularly important in resolving the plants and their intraswamp habitats are coal balls, which are essentially in situ accumulations of plant litter preserved relatively uncompacted in concretions within coal seams. Coal balls occur in many coals, mostly in the upper Carboniferous. Coal balls, miospores, and compression fossils have been studied from across the ancient Euramerican paleocontinent largely as a consequence of their exposure during coal mining and because of their relevance to coal geology as well as paleobotany. Similarly, studies of depositional patterns, and interpretations of regional and global climate have engendered considerable debate (e.g., Wanless et al., 1969; Horne et al., 1978; Phillips and Cecil, 1985; Heckel, 1986; Klein and Willard, 1989; Cecil, 1990). Despite disagreements, the data, and varying conclusions drawn from them, have yielded greater insight than for any pre-Tertiary systems.
The implications of this research may go far beyond the late Paleozoic. Paleoecology provides a baseline against which we can examine, for greater generality, our concepts formulated almost entirely from extant ecosystems. The fossil record is our only access to long-term patterns and their implications for questions of community persistence, stability, and response to major extrinsic perturbations. By examining the response of ancient ecosystems to major disruptions we hope to gain insights into the ways in which plant communities of today may behave—not the relatively trivial specifics of change, but the general mechanistic basis underlying change. We need to learn how to recognize responses of ancient ecosystems that are unique products of times and circumstances, as opposed to inherent qualities that reflect principles of organization that transcend evolutionary diversification of the biota. When comparing modern plant communities to any from the Late Carboniferous we must recognize that the levels of Carboniferous species diversity were at least three orders of magnitude lower than those at present, that there are difficulties in recognizing those environments that are most stable and geologically persistent, particularly in extant systems, and that differences in temporal scale can confound comparisons of ecological patterns.
Late Carboniferous Wetlands
The Carboniferous was the first and most extensive coal age. The pantropical wetlands of the Late Devonian and Early Carboniferous from which the coal-forming forests evolved were the Earth's primeval forests (Cleal, 1987). Most of the major lineages of plants that dominated these environments originated during the Late Devonian and earliest Carboniferous. In taxonomic terms, all the generally recognized major class-level groups of vascular plants (save the flowering plants) and many of the major ordinal-level groups originated during this narrow window of time. In the establishment of all basic life history and architectural patterns in vascular plants, the Late Devonian and earliest Carboniferous parallels the Cambrian radiation of marine invertebrates.
The vascular plant radiation involved a strong association of classand ordinal-level clades with specific ecological conditions. In effect, the basic ecologies and habitats of the landscape were strongly partitioned along taxonomic lines. This pattern of ecological partitioning along taxonomic and life history lines was to distinguish the Carboniferous from all subsequent times. The landscape partitioning of the Carboniferous began to break down over an extended time interval, from the end of the Late Carboniferous through the Early Permian. During this time there were major extinctions in the wetlands (Phillips et al., 1974, 1985; Pfefferkorn and Thomson, 1982), accompanied by expansion of seed plants into vacated resource space (Knoll, 1984). The result, by the Late Permian, was dominance of the landscape by one major life history, the seed habit, which ushered in the pattern still prevalent today.
The lowlands were partitioned, in the broadest sense, between seed plants and lycopsids as the dominant tree groups. Seed plants occupied mainly the better-drained environments; lycopsids grew in swampy, semiflooded habitats. These lineages had very different life histories and morphologies, and the ecosystems they dominated had widely differing dynamics (Phillips and DiMichele, 1992). Today the seed plants, particularly flowering plants, dominate nearly all terrestrial ecosystems; despite the diversity of dynamics encompassed by extant plants and ecosystems, they do not include some of the structure and dynamical properties that were part of the Carboniferous ecological spectrum, the lycopsid-dominated swamps, for example.
Eco-Taxonomic Patterns
Peat Swamps
Peat-forming coal swamps had organic substrates, suggesting chelation of mineral nutrients, typically low pH, and periodic flooding. Such environments had high levels of abiotic stress, a result of the combination of low nutrient levels, anaerobic conditions within water-saturated rooting zones, water-table fluctuations, and regular disturbance. The high abiotic stress (sensu Grime, 1979; DiMichele et al., 1987) appears to have selected strongly against most species, leading to a specialized flora. The tolerant species tended to be parts of peat swamp-centered evolutionary lineages, such as many of the lycopsid genera (DiMichele and Phillips, 1985), or specialized offshoots that became somewhat isolated from main line of the clade, such as cordaitean gymnosperms and some of the marattialean ferns (Rothwell and Warner, 1984; Costanza, 1985; Trivett and Rothwell, 1985; Lesnikowska, 1989).
Late Carboniferous peat swamps were not a single monotonous habitat, as depicted in most museum dioramas. Rather they encompassed a range of subhabitats controlled largely by flooding regime and influenced by disturbances such as incursions of mineral matter, brackish water, and wildfires. Some swamps or parts of swamps may have been domed (ombrotrophic), as in modern areas of Sumatra and Borneo (Cecil et al., 1985; Esterle and Ferm, 1986; Kvale and Archer, 1990; Staub and Esterle, 1992; Eble and Grady, 1993). Others were clearly planar (rheotrophic) swamps, subject to flooding and introduction of clastics in floodwaters (Eggert, 1982; Grady and Eble, 1990; Calder, 1993). Both kinds of swamp, which may have coexisted within a single peat body, were sufficiently variable in physical conditions to support a diversity of habitats and the species specific to them.
Knowledge of the plant composition and paleoecology of peat swamps is provided by coal balls and miospore studies. Coal balls (Figure 8.1) are concretions of the original peat fabric petrified (permineralized) before the peat was coalified. They occur within coal seams, generally as calcium carbonate and occasionally as siliceous concretions. The plants in coal balls are anatomically preserved, often in exquisite detail, and range in size from tiny fragments to large axes. Coal balls occur in more than 65 upper Carboniferous coal seams, but may be confined largely to planar peats (Cecil et al., 1985).

Figure 8.1
Coal balls in situ; Herrin (No.6) Coal, Illinois. Large, light-colored masses are calcium carbonate permineralized coal balls, within the darker coal.
Miospores are approximate indicators of the flora of coal forests. They can be recovered from most coals that have not been subject to intense metamorphosis because of the decay-resistant chemical composition of their outer walls. Correlation of spores with source plants (e.g., Courvoisier and Phillips, 1975; Willard, 1989) provides a baseline for inferring paleoecological patterns from palynological data. In this chapter we focus on the macrofossil (coal-ball) data base we have constructed, although palynological data will be referenced where appropriate.
Clastic Wetlands
The wetlands surrounding peat swamps were a typically diverse array of lowland settings including marshes, clastic (muddy) swamps, levees, splays, and channel margins, all part of larger flood basins. Most wetlands preserved in the fossil record were periodically flooded or had wet substrates (Scott, 1978; Gastaldo, 1987). The dominant plants included pteridosperms (seed ferns), ferns, and other groups that were locally important, such as sphenopsids (horsetails) or lycopsids. Such lowland areas encompassed a much wider array of habitats than did coal swamps. Consequently, there was much more potential for species migrations, habitat gradients, and generally broadly transitional boundaries (ecotones) between habitats, resulting in considerable floristic variation on basic themes.
Among the wetland habitats were swamps with large clastic influxes. These are often preserved as dark, organic shales. Many such swamps were lycopsid-dominated, like the peat swamps, and provide us with unusual information on spatial relationships of the plants, tree sizes, and gross morphology (Wnuk, 1985; DiMichele and Gastaldo, 1986; DeMaris, 1987; Wnuk and Pfefferkorn, 1987), important in the estimation of areal variability of peat-swamp assemblages (e.g., DiMichele and Nelson, 1989). In other cases, pteridosperm dominance of some clastic swamps and wet mineral substrates occurred in the Late Devonian and throughout the Carboniferous.
Plants of the clastic wetlands are generally preserved as compressions and impressions in relatively inorganic sediments, such as shales and sandstones, which provide external morphological features. Coal balls, on the other hand, preserve anatomical structure. Consequently, there are constraints in comparing taxonomic identifications from the two fossil forms. To the extent that identifying characteristics can be compared, the plants of peat deposits, and to a lesser degree those of flooded mineral-rich substrates, mostly represent different species from those in
the moist lowlands (DiMichele et al., 1986, 1991). This is a consequence of the edaphic barriers created by the physical character of swamps. Overlap is greatest among medullosan pteridosperms, many of which apparently colonized those parts of peat swamps subject to flood and fire disturbance, areas with the greatest similarity to parts of the clastic wetlands.
Coal Swamps as Hierarchically Organized Systems
Coal swamps were an integral part of Carboniferous lowland environments and have become the epitome of the "coal age" as generally presented to nonspecialists. In fact, coal swamps represent a special subset of the total range of environments existing during that time. Although this subset is more diverse and heterogeneous than dioramas depict, it constitutes a distinctive basis for making ecological comparisons through Late Carboniferous time. Peat-forming environments are a recognizable environmental subset, providing a taphonomically comparable basis for pattern analysis. In addition, the system was semiclosed to species introduction because of the edaphic constraints imposed on plants by the usually saturated peat substrate. This limitation increases the resolution of ecological patterns through time. The effort of dozens of paleobotanists, for more than a century, has provided the taxonomic and morphological baseline for us to generate a large quantitative data base from approximately 40 coals in western Europe and the United States (Figure 8.2).

Figure 8.2
Global paleogeography during the Late Carboniferous. Coal balls come from the shaded area of the tropical Euramerican subcontinent. Map adapted from 1988 version of Terra Mobilis produced by C. R. Scotese and C. R. Denham.
Organization of Coal-Swamp Ecosystems
The organization of coal-swamp ecosystems is complex, and can be examined at a variety of spatial and temporal scales (Figure 8.3). Each of these scales reveals different elements of the overall organizational hierarchy. In most instances the relationships of organizational patterns and dynamics at different levels are more clearly seen when a larger view of the system is taken.

Figure 8.3
Spatial and temporal scales of resolution available from coal-ball data analysis. SQ.M.= square meters. Small capitals refer to fossil samples, large, bold capitals refer to inferences made from fossil samples.
Resolution at 100- to 104-yr Time Scales: Habitats and Species Assemblages
Coal-ball occurrences vary from scattered specimens to distinct zones or massive aggregates, in situ within coal seams (Figure 8.1). Although many deposits are locally extensive, distinct zones of coal balls can be followed laterally no more than a few meters in most instances. Such "zones" of coal balls can be treated as the litter layer of a single forest stand, preserving a time period of <1 yr (instantaneous events such as fires preserved in fusain layers) to 100 yr. Study of the species composition and relative species abundances in individual zones (Phillips et al., 1977; Phillips and DiMichele, 1981) is the basic unit for further quantitative studies. Inferences can be drawn regarding the vegetational structure of the forest from which the litter sample was drawn, and the dynamics of that forest can be deduced from analysis of the relative proportions, life histories, and habits of the plants.
Aspects of the physical habitat also can be determined or deduced for individual zones, revealing the conditions under which the parent forest grew. Physical evidence of mineral matter in coal, either through ash determinations (Eble and Grady, 1993) or petrography (Johnson, 1979; Esterle and Ferm, 1986; Grady and Eble, 1990), suggests susceptibility of the swamp to floods, that introduce clastics, or peat decay, which may concentrate mineral matter (Cecil et al., 1979; Ruppert et al., 1985). Relative abundances of mineral charcoal (fusain) indicate the presence of fires in the swamp and can point to particular plant groups or tissues that are most frequently preserved as charcoal, or to patterns of preservation that suggest fire intensity (charred outer rinds on tree bark) (Phillips and DiMichele, 1981; DiMichele and Phillips, 1988). The proximity of a coal-ball zone to the underclay, or to thick mineral partings in the coal bed, indicates significant changes in edaphic conditions to or from those characteristic of the peat substrate. In many cases, the mineral partings represent disturbances by clastic-laden floodwaters. Specific plant groups or assemblages have been found to have a disproportionate association with these indicators.
Taphonomic criteria, that is, the characteristics of the preservation of the coal-ball peat, also provide insights into the physical character of the habitat associated with a particular zonal assemblage (Scott and Rex, 1985; Raymond, 1987; Phillips and DiMichele, 1989). Peat exposure can be gauged from the degree to which the peat is rotted and the plant remains poorly preserved. Exposure is also indicated by detritivore activity, such as frass-filled borings in wood, periderm, or soft tissues, or extensive fecal pellet deposits left by surface feeders. The framework-to-matrix ratio of the peat is an indicator of the degree to which fine, particulate material has been flushed from the peat fabric by water through-flow, which may also have affected peat acidity.
Finally, habitat inferences can be drawn from the biotic character of the assemblage itself, through ecomorphic analysis. For example, the relative proportion of ground cover and free-sporing life histories (the "fern" life cycle) can suggest the frequency or length of surface water cover. Relative proportions of opportunistic/invasive life histories, in combination with high species richness and high ecomorphic diversity of an assemblage, can point to recent disturbance and subsequent colonization. The proportions of monocarpic (one reproduction at the end of the lifetime) versus polycarpic (multiple reproductions during lifetime) lycopsid life histories suggest the relative extent to which the environment was stable and "predictable."
These basic patterns are the fundamental building blocks of our higher-order inferences about coal-swamp structure and temporal dynamics. We extend them through time and space to construct a larger, more complex picture.
Resolution at the 103- to 105-yr Time Scale: Landscape Patterns
Coal-ball occurrences in multiple layers, or profiles, provide access to changes in vegetation, vegetational dynamics, and habitats through time. The time interval may vary from 103 to 105 yr, depending on how thick the coal seam is and how much of it was preserved as coal-ball peat.
Dominance and diversity patterns on a site through time can be quantified through profile analysis. In many cases, patterns of vegetational change are associated with physical markers that can be correlated between coal-ball masses within a single seam, such as clastic partings, fusain layers, or distinctive petrographic composition. The evidence we have accumulated to date suggests little directional succession at a whole-seam level, consistent with a planar-peat origin for most coal-ball bearing coals, and no discernible evolutionary change in the morphology of the component species within the time necessary to accumulate a 1- to 2-m thick coal seam.
Landscape patterns within a single coal swamp can be reconstructed from profile data, using single or multiple profiles, or from random sample data. Within any one profile the succession of zones can be taken to represent an atemporal record or sample of the kinds of habitats and assemblages that existed within the swamp. Ordination (see Digby and Kempton, 1987) of individual zones, or of individual coal balls in the case of random samples, provides a base map of landscape-level variability within the coal, including the relative proportions of habitats, dominance-diversity patterns, and the relative similarity of assemblages from different subenvironments. The patterns at the landscape level, including the relative proportions of habitats, plant assemblages, life histories, and community dynamics, are characteristic of a coal or group of coals. These provide a "fingerprint" of a particular coal swamp, and more broadly of a time and geographic region.
Resolution at the 105- to 107-yr Time Scale: Interseam Patterns
It is on the longest time scales that evolutionary change can be detected, and on which the effects of climatic or other extrinsic factors on swamp community dynamics and structure are seen most clearly. Long-term trends in landscape, habitat, and species composition must be examined among coals. Each can be evaluated independently, or the relationships among them can be investigated. For example: Is the timing of change similar at all levels of observation? Are there relationships among levels of organization that are detectable only on the longer time scales? Do events or changes at one level appear to dictate or constrain those at other levels?
Climatic change is often brought about by geological phenomena that develop over millions of years, such as the movement of continental plates, the elevation of mountain ranges, or the waxing and waning of ice sheets and associated eustatic sea-level fluctuations. It is extremely difficult to correlate such events, which have their own considerable margins of error, with specific changes in community composition. Broadly based correlations can be developed, however, and there is great likelihood that widespread biotic changes are the consequence of events of global or at least regional scale. Thus, the long-term analysis of swamp patterns is a scale at which such phenomena can be examined. Certainly, glacial eustatic and climatic effects occur at time scales approximately the same as those over which we believe coal seams accumulated. However, the peat accumulation may have required a relatively narrow range of conditions, such that coal seams occur between the "events," which may mark their beginnings and ends. Consequently, it is only on the interseam level of observation that we can make a case for the climatic changes that affected the dynamics observable at lower levels.
Coal-Swamp Species and Ecomorphs
Well over 100 whole-plant species have been identified in coal balls from the upper Carboniferous of Europe and United States, across a time span of 12 to 15 m.y. Most of these can be assigned broadly to several ecomorphic groups, based on their habit, reproductive morphology, and life history attributes (Figure 8.4). The ecomorphic groups, particularly trees, conform fairly strictly to taxonomic groups or clades, that is, to groups of closely related taxa, which is the basic pattern of the Carboniferous. Much of what we know of evolution based on studies of modern plants and animals suggests that this should not be the case; ecological overlap between groups of plants with different ancestries is the rule in modern forests. It was not an expected result when we began to look for ecomorphic patterns among coal-swamp plants. The explanation for this pattern may lie partly in the ecological structure of the Carboniferous world, including the origin of that pattern.

Figure 8.4
Major tree ecomorphs in Late Carboniferous coal swamps. Left to right: Mature monocarpic lycopsid about 20 m in height; juvenile lycopsid; polycarpic lycopsid (these came in a wide range of sizes); medullosan pteridosperm (seed fern); small Psaronius (more...)
Habits of the major ecomorphic tree groups are illustrated in Figure 8.4. Ecomorphic determination is based mainly on critical life history attributes: timing of reproduction; approximate reproductive output; morphology of, and energy investment in, disseminules; and dispersibility of disseminules. Habit and vegetative morphology also are important: size at maturity; adult shape; growth habit (tree, vine, shrub, ground cover); degree of xeromorphy; and expense of construction. The latter two are comparative, and hence relative, depending on the spectrum of morphologies present in Carboniferous swamps.
Major Intraswamp Habitats
Habitats within a peat-forming swamp are all highly modified from extraswamp analogues by the presence of the predominantly organic substrate. The edaphic qualities of this substrate are variable, and these variations define many of the intraswamp habitats.
Flooded habitats are recognized mainly on biotic or ecomorphic criteria: low species richness, little ground cover, few free-sporing plants (which need an exposed substrate to complete their life cycle), and dominance by plants with specialized semiaquatic or flood-tolerant morphologies. Such habitats also are relatively low in charcoal. Lycopsids were the most common elements of these environments in the Westphalian.
Peat-to-clastic ecotonal habitats cover a wide range of conditions, recognizable mainly on physical criteria, although they encompass plants with corroborative life history strategies. There are several variants. Peat-to-mud transitional environments are associated with underclays, clastic partings, or usually high ash and mineral matter in coal; they are populated in the Westphalian by a small arboreous lycopsid (Paralycopodites), and often by medullosan seed ferns. Fire-prone habitats are associated with elevated levels of fusain and often with increased clastic material; the most common components of these environments are medullosan pteridosperms, sphenopsids, and in some cases, small, scrambling cordaitean gymnosperms. Habitats with long periods of exposure and presumed drying of the peat surface are characterized by heavily rotted and rerooted peats, often with evidence of extensive invertebrate burrowing; such environments are associated with larger cordaites, some sigillarian lycopsids, and medullosan pteridosperms.
Cryptic, or irregular disturbance, habitats are recognizable by physical and ecomorphic attributes. They generally have little fusain or mineral matter. Evidence from an unusual buried forest deposit (Wnuk and Pfefferkorn, 1987), drawn from species compositional similarities, suggests irregular floods. In some swamps, storms associated with the influx of marine waters may have been a major disturbance agent, suggested by multiple coal-ball and coal layers containing marine invertebrates. Such habitats are generally dominated by polycarpic, long-lived trees, lycopsids in the Westphalian, and possibly tree ferns in the Stephanian. Species richness is intermediate, growth architectures often are very variable among the subdominants, and a ground cover component is generally important.
Patterns of Change in Coal-Swamp Communities during the Pennsylvanian Period
Objectives
In this section we focus on the temporal patterns of change in coal swamps. This pattern is examined first at the landscape level-changes that are the easiest to describe and thus to relate to larger questions of environmental influence. The timing and extent of landscape-level changes is compared with patterns in habitat and species composition of successive swamps. We examine the relative timing and extent of change in these elements, and the relationship between species turnover and habitat persistence. We then argue that these relationships suggest a hierarchical structure in which biotic factors influence patterns of species replacement.
Changes at the Landscape Level
Change in the relative abundance of the major plant groups comprising swamp communities is the major, and simplest, indicator of community change and has been discussed elsewhere (Phillips and Peppers, 1984; Phillips et al., 1985). Because the major plant groups (lycopsids, ferns, pteridosperms, sphenopsids, cordaites) are broadly distinct in habitat preference, changes in their relative abundances also reflect changes in the physical characteristics of swamps. Figure 8.5 summarizes the changes by geographic region, with the general pattern summarized in the right-hand column.

Figure 8.5
Patterns of change in the abundance of the major plant groups in 28 selected upper Carboniferous coals. Summary at right includes coals from western Europe (labeled with an asterisk).
Biomass distribution is spread among enough major tree groups, and the patterns are sufficiently distinct, that some important generalities can be resolved at this level. We use mostly western European chronostratigraphic terminology because that of the United States varies widely among geographic regions.
1. Lycopsids, of several ecomorphic forms, dominate most coal swamps for the 9 m.y. (using the Hess and Lippolt, 1986, time scale) of the Westphalian (late early and middle Pennsylvanian). Major extinctions in North America occurred near the Westphalian-Stephanian (middle-upper Pennsylvanian) boundary, eliminating most of the lepidodendrids and removing the lycopsids from a position of ecological dominance in swamps (Phillips et al., 1974).
2. Cordaitean gymnosperms are the major subdominants or dominants during the midportion of this time interval, from the Westphalian B to the early Westphalian D. Two distinct phases are represented. During the Westphalian B and C, cordaitean taxa that produced Mitrospermum-type seeds (ovules) were the most common
forms. During the late Westphalian C and early Westphalian D the most abundant cordaites produced Cardiocarpus type seeds; these may have had a more shrubby or scrambling habit than earlier coal-swamp forms (Cridland, 1964; Costanza, 1985).
3. Tree ferns replace lycopsids as the ecological dominants of the Stephanian (Late Pennsylvanian). Tree fern abundances begin to rise during the Westphalian C, and especially during the Westphalian D. However, systematic studies suggest that largely different suites of Psaronius species and ecomorphs occupied Westphalian and Stephanian swamps (Lesnikowska, 1989).
4. Pteridosperms (seed ferns) fluctuate in abundance during the Westphalian, but attain uniformly higher and less variable abundances in the Stephanian. Studies of seed morphology (Taylor, 1965) suggest substantial species turnover at the Westphalian-Stephanian boundary.
5. Sphenopsids are present in coal swamps throughout the Late Carboniferous, but generally at low levels. Palynology suggests local times and regions of abundance (Peppers, 1979).
The existence of breakpoints, marked by minor to major extinctions of swamp-centered lineages, is one of the most conspicuous aspects of this data summary. These breakpoints also are associated with landscape-level changes in dominance-diversity patterns (e.g., the rise and decline of cordaites, and the dramatic expansion of tree ferns). Palynological analyses (Peppers, 1979; Phillips et al., 1985) reveal the same basic patterns as the coarser megafossil data and point particularly to the Westphalian A-B boundary and the Westphalian-Stephanian boundary as times of major species turnover (Peppers, 1979; Phillips et al., 1974). The patterns at this level led us to suggest a typological classification of coal swamps some time ago (DiMichele and Phillips, 1981). This classification was based on the premise that there were periods of little change in dominance and diversity from one coal swamp to the next in stratigraphic sequence—periods of persistence—punctuated by much shorter time intervals of abrupt vegetational change. Our original formulation was based on a smaller data base, but the basic pattern has remained as further data have accrued.
In order to evaluate this pattern more formally we have used some statistical methods to examine the data for floristic breakpoints (Figure 8.6). Jaccard coefficients and sign tests were used to assess similarity based on species presence or absence; through inspection we examined the data for points of low similarity. Although these are weak tests, used on the weakest manifestation of the data (presence-absence of species), they strongly corroborate the breakpoints at the Westphalian A-B boundary and at the Westphalian/Stephanian boundary. They support less strongly the presence of breakpoints at other boundaries where proportions of dominant tree taxa shift but are associated with few extinctions.

Figure 8.6
Quantitative assessment of landscape-level patterns of change through the Westphalian and Stephanian. Dotted lines mark breakpoints in coal bed average vegetational composition; breakpoints were determined by inspection and statistical analysis. Jaccard (more...)
A more intuitive, or subtle, way to analyze landscape-level vegetational patterns is to use ordinations of zones or coals balls to examine the structure within individual coals. Patterns within successive coals then can be compared. Ordinations cluster the stands, be those stands individual coal balls or zones of coal balls from profiles, based on their quantitative taxonomic similarity. In two or three dimensions they serve as base maps on which additional information can be superimposed: for example, life history occurrences, diversity, physical attributes associated with assemblages, and ecomorphic characteristics. Ordinations thus serve as a transition between landscape-level and habitat-level patterns in that they illustrate the biotic and physical variability of the landscape in detail.
Because of the nature of this review, only selected coals can be illustrated to show the basic patterns of vegetational, landscape-level changes through time (Figure 8.7). The nature of landscape changes detected in ordination again corroborates the pattern detected at the level of average coal seam composition: change in swamp organization at the Westphalian A-B boundary, minor changes between the Westphalian B and early Westphalian D, substantial restructuring during the early Westphalian D, and total overhaul of swamp community organization near the Westphalian-Stephanian (middle-upper Pennsylvanian) boundary.

Figure 8.7
Detrended correspondence analysis ordinations of six Westphalian swamps. Oldest to youngest in stratigraphic order: Union seam (Westphalian A); Rock Springs coal (Westphalian C); Murphysboro equivalent coal (early Westphalian D); Fleming coal (early Westphalian (more...)
Changes in the Habitat Composition of Landscapes
Ordination patterns reveal how the species assemblages of landscapes changed during the Late Carboniferous. During this time there is a close correlation between physical attributes of swamps and species assemblages, particularly in the Westphalian. Because physical attributes of swamps, such as fusain or mineral matter, can be mapped onto ordinations, as can taphonomic, life history, and structural patterns, it is possible to translate ordinations based on taxa into maps of habitat diversity. This permits us to infer patterns of habitat change through time.
Westphalian A swamps had complex habitat organization, harboring a variety of taxa with different physical preferences. The Union Seam of England (Figure 8.7) had four basic assemblages—each, we believe, characteristic of a distinct subset of swamp environments: assemblages dominated by monocarpic lycopsids (Lepidophloios harcourtii or Lepidodendron hickii), growing in areas with long periods of standing water sufficient to reduce the abundance and diversity of ground cover and free-sporing plants; ecotonal assemblages (Paralycopodites and medullosan seed ferns) enriched in fusain, and ecomorphically distinct (no data exist on their position within the seam); cryptic disturbance habitats dominated by polycarpic lycopsids (Diaphorodendron vasculare and Sigillaria spp.), with a diversity of growth architectures, including abundant ground cover; Lyginopteris assemblages for which the physical attributes are unclear, but which overlap to some extent with Diaphorodendron vasculare-dominated assemblages and may have been part of the broader cryptic disturbance set of environments. Other Namurian and Westphalian A swamps offer variations on these themes (Holmes and Fairon-Demaret, 1984; Bertram, 1989).
The transition from the Westphalian A to the Westphalian B was marked by changes in the dominance-diversity composition of swamps. The patterns that appeared persisted through the Westphalian B and C, and into the early Westphalian D. The most notable changes were the extinction of Lyginopteris and the increase in the abundance of cordaitean gymnosperms. The cause of the extinction of Lyginopteris is not clear; the genus disappeared both in coal swamps and in surrounding clastic lowland settings of Euramerica.
Post-Westphalian A swamps not only lacked Lyginopteris but differed in other major aspects from swamps of the Westphalian A. In general, there was a decline in the habitat diversity of any one coal swamp, with the habitats present representing a subset of those in the Westphalian A, complemented by the addition of cordaitean-dominated assemblages. Cordaites appeared initially in ecotonal assemblages characterized by heavily rotted peats and abundant fusain, sometimes associated with medullosans, ultimately becoming a locally dominant component of some swamps (Phillips et al., 1985). Later in the interval, in the late Westphalian C and early Westphalian D, opportunistic cordaites and tree ferns began to expand in importance. The tree ferns transcended habitats, appearing in ecotonal assemblages in numbers following one of the minor breakpoints, becoming interstitial opportunists by the early Westphalian D, and eventually occurring in all but persistently flooded assemblages during the Westphalian.
Despite the breakup of Westphalian A patterns of landscape organization, the basic habitats of the earlier time are recognizable in Westphalian B to early Westphalian D swamps. The persistent habitats, recognizable by the physical, taphonomic, and structural attributes (as discussed earlier), retained their ecomorphic character, although the component species were largely different. Any one of the several possible habitats may have been either absent from a given coal, or present in such low frequency that it was not sampled, including those characterized by cordaitean dominance. Various combination of habitats thus appear throughout the interval (Figure 8.8).

Figure 8.8
Patterns of change in physically determined habitats through time. Habitat characteristics discussed in text. Dotted lines are breakpoints determined by inspection and statistical analysis. MONO = habitats dominated by monocarpic lycopsids; POLY = habitats (more...)
Late Westphalian D coal swamps represent a return to the basic habitat organization characteristic of the Westphalian A: flooded habitats dominated by monocarpic lycopsids, ecotonal habitats dominated by the lycopsid Paralycopodites and medullosans, and cryptic disturbance habitats dominated by polycarpic lycopsids with structurally complex vegetation. Cordaiteans disappeared as an ecologically significant component, associated with the extinctions of several numerically important species. The reassembly of the Westphalian A type of swamp habitat organization is modified by the persistence and further expansion of tree ferns as interstitial opportunists. Identifiably opportunistic species equivalent to the tree ferns were rare in Westphalian A swamps. Psaronius expansion in coal swamps correlates with a similar expansion in lowland wetlands in general (Pfefferkorn and Thomson, 1982).
The habitats of the late Westphalian D each have an ecomorphic character that had persisted throughout the 9 m.y. of the Westphalian. This character persisted through landscape-level breakpoint boundaries, despite species-level changes within the habitats. In relatively few instances, the same species can be identified within these habitats over the entire 9-m.y. period. Species replacements within habitats were largely on ecomorphic themes, especially for trees. The pattern suggests that landscape-level patterns of persistence and change are driven fundamentally by changes in the proportions of the basic habitats represented, not by species-level turnover, which occurred largely within habitats during the Westphalian.
Major extinctions of coal-swamp plants occurred during the Westphalian-Stephanian transition. These were first recognized in miospore studies as the loss of the major Lycospora-producing lepidodendrids (Phillips et al., 1974). Recent systematic studies of the tree ferns (Lesnikowska, 1989) revealed a nearly complete extinction of species in this group in North America at the end of the Westphalian; Stephanian swamps presumably were recolonized from surrounding lowlands, where major extinctions probably also occurred, given that there is limited overlap between Westphalian and Stephanian species (e.g., Corsin, 1951; Remy and Remy, 1977). Examination of the literature on pteridosperms (e.g., Taylor, 1965; Rothwell, 1981; Pigg, 1987) and small ferns (e.g., Phillips, 1974) suggests substantial species level turnover in the coal-swamp members of these groups as well.
In North America this was the first Pennsylvanian extinction to eliminate whole ecomorphic groups of trees entirely. It was these trees that defined the biotic or ecomorphic aspect of most intraswamp habitats. They also defined the biotic limits to habitat space, that is, the nature of the biotic partitioning of the habitat resources. Thus, with this extinction, the fabric of Westphalian swamp communities—the persistent ecomorphic organization of several specific physical habitats—was destroyed.
Stephanian swamps were reorganized into a different set of norms of reaction between the plants and the physical environment. We do not fully understand these at present, in part because vertical profile analysis of Stephanian coal swamps has begun only recently. The swamps did become heavily tree fern dominated and thus generally more like the surrounding clastic lowlands. Tree fern-dominated areas may have had a great deal of local spatial heterogeneity, with cordaites, sphenopsids, and pteridosperms liberally intermixed (Willard and Phillips, 1993). The lycopsids Sigillaria, a tree, and Chaloneria, a robust herb, were locally abundant in parts of many of these swamps (DiMichele et al., 1979). The degree to which any of the Stephanian habitats overlap with those of the Westphalian has yet to be determined; however, preliminary evidence suggests different patterns of resource partitioning.
Changes in the Species-Level Composition of Habitats
The species composition of coal swamps underwent considerable turnover during the Late Carboniferous. In analyzing the distribution and extent of that turnover through time, our objective was to relate it to changes in structure at the other levels of organization and to evaluate the locus of change with respect to habitats. In this analysis stratigraphic range data are used, which we believe is justified because most species are swamp-centered and therefore had potential access to peat swamp ecosystems throughout their existence. However, our sampling may miss some of the smaller or rarer taxa. There are numerous problems with these data: inadequate sampling, inadequate taxonomy for many groups, low sampling density in the Westphalian B and C, and regional patterns that obscure the overall pattern. Nonetheless, the basic message is consistent with the pattern seen at the landscape and habitat levels. We expect to be able to refine the data as our studies proceed.
The data are drawn from our own profile and random sample analyses in order to avoid problems of inflation in the numbers of taxa known from particularly well studied coals, and to provide a uniform basis in taxonomic usage and sampling design. Thus, even though a species is known from a particular coal, if it did not appear in profile or random sample studies it was listed as absent in the raw data compilation; a species will appear as present in the data used for the turnover calculation, however, if the coal from which it is known falls between first and last occurrences in our samples. Species turnover was calculated as
O t0+1 + E t0
1/2(nt0 + nt0+1)
Where E t0 = species disappearing after a coal at time t 0 ; O t0+1 = species first appearing in the next coal at time t 0 + 1 ; n t0 = number of species at time 0; and n t0+1 = number of species in next coal in sequence.
Turnover pattern is illustrated in Figure 8.9, with landscape-level breakpoint boundaries shown as dotted lines. Note that the highest turnovers occur around the major breakpoints, the Westphalian A-B boundary and the Westphalian-Stephanian boundary. Turnover within any one of the five major interbreakpoint intervals is much lower. The Westphalian D has notably higher diversity than the Westphalian A-C or the Stephanian.

Figure 8.9
Patterns of turnover of species between coal swamps; first occurrences and last occurrences of species, and total species refer to individual coals. Similarity (Jaccard) and turnover percentage are comparisons between successive coals.
Analysis of species replacements by habitat suggests that species replacement patterns are not random across the landscape during the Westphalian. Species with similar ecomorphic characteristics may have complementary stratigraphic distributions, or newly appearing species may replace older ones as ecological dominants. These species tend to replace one another within a given type of biotic assemblage and habitat. As a result the ecomorphic character of the basic habitats is maintained throughout the Westphalian and, presumably, will prove to be maintained on a different set of themes during the Stephanian. Although species turnover tends to occur in greatest numbers at the breakpoints, where landscape patterns also change, the replacements that occur at these times are on ecomorphic themes, except at the Westphalian-Stephanian boundary.
At the Westphalian-Stephanian boundary the basic pattern changes. Many of the dominant tree ecomorphs are eliminated in the swamp extinctions. As a consequence, the dynamics of species interactions alone appear to dictate the initial patterns of swamp reorganization in the early Stephanian. Great variation in the suite of dominant species is detected palynologically (Phillips et al., 1974, 1985) among the coals that occur successively after the extinction. Ultimately, the system appears to reequilibrate, with fern dominance and pteridosperm subdominance becoming the rule for the remainder of the Stephanian. These patterns suggest that following the disruption of Westphalian ecosystem structure, coal-swamp dynamics became more stochastic for a relatively short period, until a new system of interactions was established.
Climate Change and Causation
Evidence for Climatic Variability
The morphological characteristics of tropical Euramerican floras indicate a humid, warm climate, lacking seasonality for much of the time: large, evergreen leaves; trunks and leaves with only minor armoring; woods lacking growth rings; trees and shrubs with structure suggesting rapid growth. The characteristics of Late Carboniferous plants are magnified as climate indicators by plants of the younger Permian Period in Euramerica, many of which are much more xeromorphic in character and suggest growth under periodic moisture limitation. One of the most extreme examples of xeromorphy in the Permian is the Hermit Shale flora of Arizona (White, 1929), which contains only seed plants, many of which are armored with spines and have thick, highly sclerotic leaves; the flora is associated with clear sedimentological indicators of seasonal rainfall, and may have inhabited a dry coastal area on the margin of the craton (Stevens and Stone, 1988). Such botanical evidence has led paleobotanists (e.g., White, 1933) to suggest climatic factors as the driving force behind floristic change.
Over the past ten years there has been a reawakening of interest in late Paleozoic climate prompted by studies of paleogeography (Scotese et al., 1979; Ziegler et al., 1981), and the resulting models of climatic dynamics (Parrish, 1982; Parrish et al., 1989). Most of the focus has been on climatic fluctuations during the time of Pennsylvanian coal formation. The most explicit of these have focused on the availability of moisture in the lowland tropics. Inferences have been based on the stratigraphic patterns of several indicators: coal resource abundance (Phillips and Peppers, 1984), coal sulfur and ash (Cecil et al., 1985), coal underclay mineralogy (Dulong and Cecil, 1989), chemical characteristics of rocks associated with coal-bearing strata (Cecil et al., 1985; Cecil, 1990), and the abundances of environmentally sensitive fossil plants in coal (Winston and Stanton, 1989).
Additional evidence for climatic changes during the Pennsylvanian and Permian comes from smaller-scale studies of rocks with depositional histories indicating alternation of wet and dry conditions on a regional level. Examples are underclay mineralogies indicative of long periods of subaerial exposure within coal-bearing sequences (Prather, 1985; Spears and Sezgin, 1985), complex paleosols indicating marine regression and increasingly drier climate (Prather, 1985; Goebel et al., 1989), alternating wetter and drier intervals in ancient dune deposits (Driese, 1985), and mixed sequences including both fluvial and eolian deposits (Johnson, 1987, 1989a,b). Deposits that indicate alternation of wet and dry conditions are generally attributed to changes in base level and associated changes in regional rainfall patterns. Eustasy, linked to South Polar glaciation, has been cited in most instances as the proximal cause of climatic variations (e.g., Wanless and Shepard, 1936; Heckel, 1986, 1989; Rust et al., 1987; Rust and Gibling, 1990; and virtually all of the above citations in this paragraph), and there is considerable evidence of Gondwanan glaciation in the late Paleozoic (Veevers and Powell, 1987). Regional and global climate may have been affected by a variety of additional factors related to regional tectonics. The effects of plate collisions on crustal deformation (Klein and Willard, 1989), changes in circulation patterns associated with the uplift of mountain ranges (Parrish, 1982), and the movement of continents and climatic belts relative to each other (Ziegler, 1990) all complicate climatic patterns.
The principal difficulty with most climatic scenarios is correlation. It is extremely difficult, some would say impossible, to correlate identifiable glacial deposits with identifiable changes in base level half the world away. We sympathize with such concerns and recognize that local, autocyclic depositional factors will overprint and often confound climatic interpretation; how does one differentiate dry climate from locally well-drained conditions based on the limited exposures usually available? Nonetheless, it is clear that one cannot turn to a ''default" climate. It is equally clear that climate has a major, if not the major, impact on many aspects of sedimentation and the distribution of biotas. Ziegler (1990) demonstrates this well in his summary of an enormous amount of physical and paleontological data on Permian climatic and biogeographic patterns. He notes that sharp biogeographic boundaries, often attributed by paleontologists to physiographic barriers, can be the result of subtle climatic variability, a phenomenon well marked in the modern world and in the postglacial (Holocene) migrational patterns of plants (e.g., Delcourt and Delcourt, 1987).
Relationships of Climatic Patterns to Vegetational Patterns
The landscape-level breakpoint boundaries detected in Late Carboniferous coal swamps correspond closely to times of inferred climatic change during the Westphalian and Stephanian (DiMichele et al., 1986). In eastern North America, comparison of three Pennsylvanian climatic scenarios with points of vegetational reorganization is illustrated in Figure 8.10. The correspondence is remarkably close, and the data bases are independent, suggesting a correlation-causation relationship. The differences in the inferred climatic patterns are not as important as the points at which departures from a norm are detected. It is these departures, or major inflections in the climate curves, that appear to dictate the times of vegetational change.

Figure 8.10
Comparison of three climate curve diagrams. The Winston and Stanton (1989) curve is based on abundances in Appalachian basin coals of plants thought to be climatically diagnostic; the Cecil et al. (1985) curve is based on geochemical characteristics (more...)
The vegetational changes at the landscape and species levels appear to scale approximately to the magnitude of inferred climatic variability. The largest inferred climatic changes were near the Westphalian A-B boundary and the Westphalian-Stephanian boundary, also the times of the greatest vegetational changes. However, habitat-level changes offer a different perspective. Habitats persist through several climatic excursions during the Westphalian, retaining ecomorphic attributes and basic generic composition. It is only during the major climatic changes at the end of the Westphalian that habitat structure crumbles. This suggests that there are aspects of the structure of at least some ecosystems that do not follow climatic patterns in a linear manner. Rather, thresholds may exist, and once exceeded, a breakdown in organization or a reduction in the number of hierarchical levels within the system may occur rapidly.
Examination of compression-impression floras from the Pennsylvanian and Permian suggests a similar pattern. There are no climate curves for the Stephanian and Early Permian. However, analyses of Pfefferkorn and Thomson (1982), Ziegler (1990), and DiMichele and Aronson (1992) indicate discontinuous vegetational changes, ultimately resulting in a tropical vegetation with highly xeromorphic aspect. It appears that lowland-wetland vegetation persisted as a unit into the Early Permian and was replaced rather than displaced by what came to be the "Mesophytic" flora (Knoll, 1984; DiMichele and Aronson, 1992). The evidence of increasingly seasonally dry climate into the Early Permian is consistent with both the vegetational and the morphological patterns. The compression floras also suggest a level of organization above that of the landscapes observed in coal swamps, at which taxonomic composition is highly conserved. It may be at this level that the ultimate effect of evolutionary ancestry is manifested.
Summary and Implications
The relationships of turnover at the landscape, habitat and species levels in coal swamps are summarized in Figure 8.11. Turnovers in landscape organization—breakpoints—appear to be a consequence of changes in the proportions of several major types of intraswamp habitats. Turnover in the species composition of swamps correlates with turnover at the landscape level. Consequently, the reshuffling of habitat proportions may reflect periods of intraswamp disruption and dislocation, leading to increased, but usually not catastrophic, levels of species extinction. Species replacements across breakpoints are on strongly ecomorphic themes and, therefore, occur largely within the confines of habitats. For this reason, species turnover does not appear to be the major underlying mechanism of change at the landscape level. Intervals of very high extinction result in the loss of framework ecomorphic species. The result is the breakdown of intrahabitat species replacement patterns, followed by ecosystem reorganization along different lines. During the Pennsylvanian, such major periods of extinction were rare, permitting the biotic-abiotic linkage to persist for millions of years.

Figure 8.11
Comparison of species-, habitat-, and landscape-level changes in coal swamps during the upper Carboniferous. Habitat distributions are listed in a "range-through" fashion, based on first and last occurrence. Swamp type is a typological characterization (more...)
Hierarchical Organization
These patterns are consistent with an interpretation of coal-swamp plant communities as hierarchically organized. Species have characteristic ecological amplitudes, which are more likely to be shared with closely related than distantly related species, at least in the less diverse, pre-angiosperm world. In a given habitat, the entire plexus of species defines a biotic network of evolved interactions. Loss of a few species from this network (extinction or extirpation) can be accommodated because the system has sufficient biotic linkages to be buffered; this notion runs counter to the findings of ecosystem models, where the greater the number of linkages, the lower is the stability of the system. Released resources and severed patterns of interaction are most likely to be utilized by species with similar morphological attributes. Particularly during the Carboniferous, in which ecosystems are strongly partitioned taxonomically, this means by a species related closely to the earlier occupant, and with similar growth form and life history. Thus, the ecomorphic-biotic structure of a habitat, given sufficient time to evolve, may strongly constrain the nature and dynamics of species replacement.
Breakdown of the biotic habitat structure will occur during catastrophic extinction, as near the Westphalian-Stephanian boundary. The result is that the biotic fabric collapses and no longer can constrain the selection of replacement species. At such times the system may go into a lottery-like period of species interactions, perhaps controlled largely by interspecific competition (admittedly, this is almost impossible to document in the fossil record). The new system may reequilibrate and establish a new set of biotic limits to species replacement dynamics.
The coal-swamp floras of the Stephanian bear close resemblance to Westphalian floras of the clastic wetlands (e.g., Pfefferkorn and Thomson, 1982). This suggests that many of the species or clades in Stephanian coal swamps may have had a prior history of cohabitation in the tropical wetlands, which may have reduced the levels of interspecific competition necessary to establish a stable system of biotic interactions within swamps. The ultimate dominance of tree ferns, with subdominant pteridosperms, is a pattern shared by Stephanian coal swamps and the clastic wetlands. We do not know if the same species occupied both kinds of habitats, but it seems unlikely, because of the edaphic constraints imposed by peat substrates. If these plants were capable of tolerating disturbances, they may have established a very different set of equilibrium interactions than those of Westphalian swamps.
Ecosystem Persistence
In a system with organization like that of Pennsylvanian coal swamps, ecosystem persistence is a real property, empirically measurable. The biotic interactions within a system tend to conserve its ecomorphic structure and dynamics, even if there is species turnover. This generality may be true only during times when physical or extrinsic conditions remain relatively uniform for millions of years. It may also require sufficient time for a system of interactions among component species to evolve.
The concept of persistence may need to be evaluated at hierarchical levels above species composition. Although species associations can persist for millions of years, as our data suggest, these patterns may not reveal the major structural attributes of the ecosystem. To view all ecosystems as organized strictly as happenstance associations of species with similar resource requirements may reflect a bias that has grown out of studying short time intervals available to neoecology, complemented by the somewhat longer patterns of Recent palynology. The climatic fluctuations of the last 10,000 to 100,000 yr are not typical of all of Earth history (moreover, the Northern Hemisphere is not a good proxy for the tropics). The dogma of "individualism" should be reevaluated for generality.
Long-Term Species Replacement Dynamics: Evolutionary Implications
Species with opportunistic life histories may have a significant advantage during intervals of ecosystem disruption. During a postextinction lottery, high reproductive output, high dispersibility, tolerance of a diversity of physical conditions, ability to grow in disturbed areas, and a tendency to spawn peripheral isolates (due to dispersal capacities), all favor invasive opportunists. During the Late Carboniferous this is seen in the marked expansion of tree ferns and scrambling cordaites following breakpoint boundaries (Phillips and Peppers, 1984) and by the massive expansion of tree ferns following the terminal Westphalian extinctions. Tree ferns were cheaply constructed; had massive reproductive output, causing vast overrepresentation in the miospore record (Willard, 1993); produced small and widely dispersed isospores capable of founding a population from one spore; and underwent a major radiation in coal swamps and clastic lowlands during the late Westphalian and Stephanian. Similar patterns have been detected at the Cretaceous-Tertiary boundary, the now famous "fern spike" (Tschudy et al., 1984), and the great radiation of angiosperms in the early Tertiary may have been made possible in part by their fundamentally opportunistic biologies during a period of ecosystem disruption (Wolfe and Upchurch, 1986; Wing and Tiffney, 1987).
A secondary dynamic to result from this process is the evolution of larger, longer-lived, presumably more competitive species from opportunistic ancestors. It is the larger forms that ultimately dominate subsequent ecosystems. The tree ferns of the Stephanian again exhibit this clearly, and hints of similar patterns are found among the pteridosperms, lycopsids, and sphenopsids. Most Westphalian marattialeans of coal swamps were not truly large trees; Lesnikowska (1989) has reconstructed scramblers and small trees in which virtually all measurable indicators of size are smaller than descendant forms in the Stephanian, in some instances up to an order of magnitude smaller. Gigantism in Stephanian coal-swamp plants has been noted for sigillarians, which our studies suggest were much larger than intraswamp sigillarians of the Westphalian. Galtier and Phillips (1985) and Willard and Phillips (1993) note record large sizes for medullosan and sphenopsid stems in Stephanian peat deposits. Large sizes point to longer-lived plants in Stephanian coal swamps, and taxonomy points to a new suite of species. Together, these observations suggest that Stephanian descendant forms, which had become ecosystem dominants, were growing in more long-persistent habitats than were Westphalian forms, which had been largely subdominant to lycopsids or ecologically restricted by them during the Westphalian.
The fabric created by the biotic interactions among organisms plays a large role in dictating evolutionary dynamics. Different kinds of opportunities for establishment of divergent phenotypes appear to exist during times of environmental stability than during times of instability and disruption. This is strongly suggested by species turnover, both its magnitude and the ecomorphic nature of species replacement. We suggest that during periods of environmental stability the targets of opportunity for divergent forms are strictly defined by both biotic and abiotic factors. Occupied niches are for the most part not available to new phenotypes, creating a very fine-meshed selective filter. In contrast, during times of environmental change, and some degree of consequent disruption of the ecological status quo, the role of the existing biotic structure is reduced. This may be a threshold response (i.e., the biotic fabric exists or it does not). If the fabric is severely disrupted, as evidently happened during the early Stephanian within peat swamps, the selective filter becomes coarse meshed as numerous opportunities are created for the survival of divergent forms. It is during such times that the role of interspecific competition would be expected to be most powerful as an active agent of selection.
This scenario integrates environmental change with the evolving lineages. It is in effect the model presented by Valentine (1980) for the origin of putative higher taxa. Higher taxa may certainly have a greater chance of survival during times of ecosystem disruption than during periods of long-term stability; however, the model applies to speciation in general. The patterns of the fossil record may require little more than an understanding that ecosystems have not been in constant flux for the past 600 m.y. Recognition that opportunities for survival of divergent phenotypes have varied enormously through time may help reconcile mechanisms based on studies of living organisms with patterns in the fossil record.
References
- Bertram, U. (1989). Untersuchungun an coal balls aus dem Namur A von Ostrau Unter Spezieller Berucksichtigung der Gattungen Heterangium, Lyginopteris, und Microspermopteris , Palaeontographica 214B , 125-244.
- Calder, J. (1993). The evolution of a ground water-influenced (Westphalian B) peat-forming ecosystem in a piedmont setting: The No. 3 seam, Springhill Coal Field, Cumberland Basin, Nova Scotia, Geological Society of America, Special Paper .
- Cecil, C. B. (1990). Paleoclimate controls on stratigraphic repetition of chemical and siliciclastic rocks, Geology 18 , 533-536.
- Cecil, C. B., J. C. Renton, R. W. Stanton, and F. T. Dulong (1979). Some geologic factors controlling mineral matter in coal, in Carboniferous Coal Short Course Guidebook , A. C. Donaldson, editor; , M. W. Presley, editor; , and J. J. Renton, editor. , eds., West Virginia Geological and Economic Survey Bulletin B-37-1, pp.224-239.
- Cecil, C. B., R. W. Stanton, S. G. Neuzil, F. T. Dulong, L. F. Ruppert, and B. S. Pierce (1985). Paleoclimate controls on late Paleozoic sedimentation and peat formation in the central Appalachian Basin (U.S.A.), International Journal of Coal Geology 5 , 195-230.
- Cleal, C. J. (1987). This is the forest primaeval, Nature 326 , 828.
- Corsin, P. (1951). Flore Fossile du Bassin de la Sarre et de la Lorraine , 4me Fascicule, Pecopteridees. Etudes des Gites Mineraux de la France, 370 pp.
- Costanza, S. H. (1985). Pennsylvanianioxylon of middle and upper Pennsylvanian coals from the Illinois Basin, and its comparison with Mesoxylon , Palaeontographica 197B , 81-121.
- Courvoisier, J. M, and T. L. Phillips (1975). Correlation of spores from Pennsylvanian coal-ball fructifications with dispersed spores, Micropaleontology 21 , 45-59.
- Cridland, A. A. (1964). Amyelon in American coal balls, Palaeontology 7 , 186-209.
- Damuth, J. (1985). Selection among "species": A formulation in terms of natural functional units, Evolution 39 , 1132-1146. [PubMed: 28561507]
- Delcourt, P. A., and H. R. Delcourt (1987). Long-Term Forest Dynamics of the Temperate Zone , Springer-Verlag, New York, 439 pp.
- Digby, P. G. N., and R. A. Kempton (1987). Multivariate Analysis of Ecological Communities , Chapman and Hall, London, 206 pp.
- DiMichele, W. A., and R. B. Aronson (1992). The Pennsylvanian-Permian vegetational transition: A terrestrial analogue to the onshore-offshore hypothesis, Evolution 46 , 807-824. [PubMed: 28568663]
- DiMichele, W. A., and P. J. DeMaris (1987), Structure and dynamics of a Pennsylvanian-age Lepidodendron forest: Colonizers of a disturbed swamp habitat in the Herrin (No. 6) Coal of Illinois, PALAIOS 2 , 146-157.
- DiMichele, W. A., and W. J. Nelson (1989). Small-scale spatial heterogeneity in Pennsylvanian-age vegetation from the roof shale of the Springfield Coal (Illinois Basin), Palaios 4 , 276-280.
- DiMichele, W. A., and T. L. Phillips (1981). Stratigraphicgeographic patterns of change in Pennsylvanian coal-swamp vegetation, Botanical Society of America, Miscellaneous Series Publication 160 , 44 (Abstract).
- DiMichele, W. A., and T. L. Phillips (1985). Arborescent lycopod reproduction and paleoecology in a coal-swamp environment of late middle Pennsylvanian age (Herrin Coal, Illinois, U.S.A.), Review of Palaeobotany and Palynology 44 , 1-26.
- DiMichele, W. A., and T. L. Phillips (1988). Paleoecology of the middle Pennsylvanian-age Herrin coal swamp (Illinois) near a contemporaneous river system, the Walshville paleochannel, Review of Palaeobotany and Palynology 56 , 151-176.
- DiMichele, W. A., J. F. Mahaffy, and T. L. Phillips (1979). Lycopods of Pennsylvanian age coals: Polysporia , Canadian Journal of Botany 57 , 1740-1753.
- DiMichele, W. A., T. L. Phillips, and R. G. Olmstead (1987). Opportunistic evolution: Abiotic environmental stress and the fossil record of plants, Review of Palaeobotany and Palynology 50 , 151-178.
- DiMichele, W. A., T. L. Phillips, and D. A. Willard (1986). Morphology and paleoecology of Pennsylvanian coal-swamp plants, University of Tennessee, Department of Geological Sciences, Studies in Geology 15 , 97-114.
- DiMichele, W. A., T. L. Phillips, and G. E. McBrinn (1991). Quantitative analysis and paleoecology of the Secor coal and roof shale floras (Pennsylvanian-age, Oklahoma), Palaios 6 , 390-409.
- Driese, S. G. (1985). Interdune pond carbonates, Weber Sandstone (Pennsylvanian-Permian), northern Utah and Colorado, Journal of Sedimentary Petrology 55 , 187-195.
- Dulong, F. T., and C. B. Cecil (1989). Stratigraphic variation in bulk sample mineralogy of Pennsylvanian underclays from the Central Appalachian Basin, Carboniferous Geology of the Eastern United States , 28th International Geological Congress, Field Trip Guidebook T143, pp.112-118.
- Eble, C. F., and W. C. Grady (1993). Paleoecological interpretation of two middle Pennsylvanian coal beds in the central Appalachian Basin, Geological Society of America, Special Paper .
- Eggert, D. L. (1982). A fluvial channel contemporaneous with deposition of the Springfield Coal Member (V), Petersburg Formation, Northern Warrick County, Indiana, Indiana Geological Survey Special Report 28 , 20 pp.
- Esterle, J. S., and J. C. Ferm (1986). Relationship between petrographic and chemical properties and coal seam geometry, Hance Seam, Breathitt Formation, southeastern Kentucky, International Journal of Coal Geology 6 , 199-214.
- Farley, M. B. (1989). Palynological facies fossils in nonmarine environments in the Paleogene of the Bighorn Basin, Palaios 4, 565-573.
- Galtier, J., and T. L. Phillips (1985). Swamp vegetation from Grand Croix (Stephanian) and Autun (Autunian), France and comparisons with coal-ball peats of the Illinois Basin, C.R. 9 th International Congress of Carboniferous Stratigraphy and Geology 4 , 13-24.
- Gastaldo, R. A. (1986). Implications on the paleoecology of autochthonous lycopods in clastic sedimentary environments of the early Pennsylvanian of Alabama, Palaeogeography, Palaeoclimatology, Palaeoecology 53 , 191-212.
- Gastaldo, R. A. (1987). Confirmation of Carboniferous clastic swamp communities, Nature 326 , 869-871.
- Goebel, K. A., E. A. Bettis III, and P. H. Heckel (1989). Upper Pennsylvanian paleosol in Stranger Shale and underlying Iatan Limestone, southwestern Iowa, Journal of Sedimentary Petrology 59 , 224-232.
- Grady, W. C., and C. F. Eble (1990). Relationships among macerals, miospores and paleoecology in a column of the Redstone coal (upper Pennsylvanian) from north-central West Virginia (U.S.A.), International Journal of Coal Geology 15 , 1-26.
- Grime, J. P. (1979). Plant Strategies and Vegetation Processes , John Wiley & Sons, New York, 222 pp.
- Heckel, P. H. (1986). Sea-level curve for Pennsylvanian eustatic marine transgressive-regressive depositional cycles along midcontinent outcrop belt, North America, Geology 14 , 330-334.
- Heckel, P. H. (1989). Updated middle-upper Pennsylvanian eustatic sea-level curve for midcontinent North America and preliminary biostratigraphic characterization, Compte Rendu, XI International Congress of Carboniferous Stratigraphy and Geology, Beijing 4 , 160-185.
- Hess, J. C., and H. J. Lippolt (1986). 40Ar/39Ar ages of tonstein and tuff sanidines: New calibration points for the improvement of the upper Carboniferous time scale, Isotope Geoscience 59 , 143-154.
- Holmes, J. C., and M. Fairon-Demaret (1984). A new look at the flora of the Bouxharmont coal balls from Belgium, Annals de la Societe Geologique de Belgique 107 , 73-87.
- Horne, J. C., J. C. Ferm, F. T. Caruccio, and B. P. Baganz (1978). Depositional models in coal exploration and mine planning in Appalachian region, American Association of Petroleum Geologists Bulletin 62 , 2379-2411.
- Johnson, P. R. (1979). Petrology and environments of deposition of the Herrin (No. 6) Coal Member, Carbondale Formation, at the Old Ben Coal Company Mine No. 24, Franklin County, Illinois, M.S. thesis, University of Illinois, Urbana-Champaign, 169 pp.
- Johnson, S. Y. (1987). Sedimentology and paleogeographic significance of six fluvial sand bodies in the Maroon Formation, Eagle Basin, northwest Colorado, U.S. Geological Survey Bulletin 1787-A , 1-18.
- Johnson, S. Y. (1989. a). Significance of loessites in the Maroon Formation (middle Pennsylvanian to lower Permian), Eagle Basin, northwest Colorado, Journal of Sedimentary Petrology 59 , 782- 791.
- Johnson, S. Y. (1989. b). The Frying Pan Member of the Maroon Formation, Eagle Basin, northwest Colorado, U.S. Geological Survey Bulletin 1787-1 , 1-11.
- Klein, G. deV., and D. A. Willard (1989). Origin of the Pennsylvanian coal-bearing cyclothems of North America, Geology 17 , 152-155.
- Knoll, A. H. (1984). Patterns of extinction in the fossil record of vascular plants, in Extinctions , M. Nitecki, editor. , ed., University of Chicago Press., Chicago, pp.21-68.
- Kvale, E. P., and A. W. Archer (1990). Tidal deposits associated with low-sulfur coals, Brazil Fm. (lower Pennsylvanian), Indiana, Journal of Sedimentary Petrology 60 , 563-574.
- Lesnikowska, A. D. (1989). Anatomically preserved Marattiales from coal swamps of the Desmoinesian and Missourian of the midcontinent United States: Systematics, ecology and evolution. Ph.D. thesis, University of Illinois, Urbana-Champaign, 227 pp.
- Mahaffy, J. F. (1985). Profile patterns of coal and peat palynology in the Herrin (No. 6) Coal Member, Carbondale Formation, middle Pennsylvanian of southern Illinois, Ninth International Congress of Carboniferous Stratigraphy and Geology, C.R. 5 , 25-34.
- Overpeck, J. T., R. S. Webb, and T. Webb III (1992). Mapping eastern North American vegetation change of the past 18 ka: No-analogs and the future, Geology 20 , 1071-1074.
- Parrish, J. M., J. T. Parrish, and A. M. Ziegler (1989). Permian-Triassic paleogeography and paleoclimatology and implications for therapsid distributions, in The Biology and Ecology of Mammal-Like Reptiles , J. Roth, editor; , C. Roth, editor; , and N. Hotton III, editor. , eds., Smithsonian Institution Press, Washington, D.C.
- Parrish, J. T. (1982). Upwelling and petroleum source beds, with reference to the Paleozoic, American Association of Petroleum Geologists Bulletin 66 , 750-774.
- Peppers, R. A. (1979). Development of coal-forming floras during the early part of the Pennsylvanian in the Illinois Basin, Ninth International Congress of Carboniferous Stratigraphy and Geology , Guidebook to Field Trip 9 , part 2, 8-14.
- Pfefferkorn, H. W., and M. C. Thomson (1982). Changes in dominance patterns in upper Carboniferous plant-fossil assemblages, Geology 10 , 641-644.
- Phillips, T. L. (1974). Evolution of vegetative morphology in coenopterid ferns, Annals of the Missouri Botanical Garden 61 , 427-461.
- Phillips, T. L., and C. B. Cecil (1985). Paleoclimatic controls on coal resources of the Pennsylvanian System of North America: Introduction and overview of contributions, International Journal of Coal Geology 5 , 1-6.
- Phillips, T. L., and W. A. DiMichele (1981). Paleoecology of middle Pennsylvanian age coal swamps in southern Illinois/Herrin Coal Member at Sahara Mine No. 6, in Paleobotany, Paleoecology, and Evolution , Volume I , K. J. Niklas, editor. , ed., Praeger Press, New York, pp.205-255.
- Phillips, T. L., and W. A. DiMichele (1989). From plants to coal: Peat taphonomy of upper Carboniferous coals, 28 th International Geological Congress Abstracts 2 , 605 (Abstract).
- Phillips, T. L., and W. A. DiMichele (1992). Comparative ecology and life-history biology of arborescent lycopods in Late Carboniferous swamps of Euramerica, Annals of the Missouri Botanical Garden 79 , 560-588.
- Phillips, T. L., and R. A. Peppers (1984). Changing patterns of Pennsylvanian coal-swamp vegetation and implications of climatic control on coal occurrence, International Journal of Coal Geology 3 , 205-255.
- Phillips, T. L., R. A. Peppers, M. J. Avcin, and P. F. Laughnan (1974). Fossil plants and coal: Patterns of change in Pennsylvanian coal swamps of the Illinois Basin, Science 184 , 1367-1369. [PubMed: 17810464]
- Phillips, T. L., A. B. Kunz, and D. J. Mickish (1977). Paleobotany of permineralized peat (coal balls) from the Herrin (No. 6) Coal Member of the Illinois Basin, Geological Society of America, Microform Publication 7 , 18-49.
- Phillips, T. L., R. A. Peppers, and W. A. DiMichele (1985). Stratigraphic and interregional changes in Pennsylvanian coalswamp vegetation: Environmental inferences, International Journal of Coal Geology 5 , 43-109.
- Pigg, K. B. (1987). Paleozoic seed ferns: Heterangium kentuckyensis sp. nov., from the upper Carboniferous of North America, American Journal of Botany 74 , 1184-1204.
- Prather, B. E. (1985). An upper Pennsylvanian desert paleosol in the D-zone of the Lansing-Kansas City Groups, Hitchcock County, Nebraska, Journal of Sedimentary Petrology 55 , 213-221.
- Raymond, A. (1987). Interpreting ancient swamp communities: Can we see the forest in the peat? Review of Palaeobotany and Palynology 52 , 217-231.
- Raymond, A. (1988). Paleoecology of a coal-ball deposit from the middle Pennsylvanian of Iowa dominated by cordaitalean gymnosperms, Review of Palaeobotany and Palynology 53 , 233-250.
- Remy, W., and R. Remy (1977). Die Floren des Erdaltertums , Verlag Gluckauf GMBH, Essen, 468 pp.
- Rothwell, G. W. (1981). The Callistophytales (Pteridospermopsida): Reproductively sophisticated gymnosperms, Review of Palaeobotany and Palynology 32 , 103-121.
- Rothwell, G. W., and S. Warner (1984). Cordaixylon dumusum n. sp. (Cordaitales) I. Vegetative structures, Botanical Gazette 145 , 275-291.
- Ruppert, L. F., C. B. Cecil, R. W. Stanton, and R. P. Christian (1985). Authigenic quartz in the upper Freeport coal bed, west-central Pennsylvania, Journal of Sedimentary Petrology 55 , 334-339.
- Rust, B. R., and M. R. Gibling (1990). Braidplain evolution in the Pennsylvanian South Bar Formation, Sydney Basin, Nova Scotia, Canada, Journal of Sedimentary Petrology 60 , 59-72.
- Rust, B. R., M. R. Gibling, M. A. Best, S. J. Dilles, and A. G. Masson (1987). A sedimentological overview of the coal-bearing Morien Group (Pennsylvanian), Sydney Basin, Nova Scotia, Canada, Canadian Journal of Earth Sciences 24 , 1869-1885.
- Scotese, C. R., R. K. Bambach, C. Barton, R. Van der Voo, and A. M. Ziegler (1979). Paleozoic base maps, Journal of Geology 87 , 217-277.
- Scott, A. C. (1978). Sedimentological and ecological control of Westphalian B plant assemblages from West Yorkshire, Proceedings of the Yorkshire Geological Society 41 , 461-508.
- Scott, A. C., and G. Rex (1985). The formation and significance of Carboniferous coal balls, Philosophical Transactions of the Royal Society of London B 311 , 123-137.
- Smith, A. V. H., and M. A. Butterworth (1967). Miospores in the coal seams of the Carboniferous of Great Britain, Palaeontological Society, Special Paper No. 1 .
- Spears, D. A., and H. I. Sezgin (1985). Mineralogy and geochemistry of the Subcrenatum Marine Band and associated coalbearing sediments, Langsett, South Yorkshire, Journal of Sedimentary Petrology 55 , 570-578.
- Staub, J. R., and J. S. Esterle (1992). Evidence for a tidally influenced upper Carboniferous ombrogeneous mire system: Upper bench, Beckley bed (Westphalian A), southern West Virginia, Journal of Sedimentary Petrology 62 , 411-428.
- Stevens, C. H., and P. Stone (1988). Early Permian thrust faults in east-central California, Geological Society of America Bulletin 100 , 552-562.
- Taylor, T. N. (1965). Paleozoic seed studies: A monograph of the genus Pachytesta , Palaeontographica 117B , 1-46.
- Trivett, M. L., and G. W. Rothwell (1985). Morphology, systematics and paleoecology of Paleozoic fossil plants: Mesoxylon priapi, sp. nov. (Cordaitales), Systematic Botany 10 , 205-233.
- Tschudy, R. H., C. L. Pillmore, C. J. Orth, J. S. Gilmore, and J. D. Knight (1984). Disruption of the terrestrial plant ecosystem at the Cretaceous-Tertiary boundary, western interior, Science 225 , 1030-1032. [PubMed: 17783047]
- Valentine, J. W. (1980). Determinants of diversity in higher taxonomic categories, Paleobiology 6 , 444-450.
- Veevers, J. J., and C. M. Powell (1987). Late Paleozoic glacial episodes in Gondwanaland reflected by transgressive-regressive depositional sequences in Euramerica, Geological Society of America Bulletin 98 , 475-487.
- Wanless, H. R., and F. P. Shepard (1936). Sea-level and climatic changes related to late Paleozoic cycles, Geological Society of America Bulletin 47 , 1177-1206.
- Wanless, H. R., J. R. Baroffio, and P. C. Trescott (1969). Conditions of deposition of Pennsylvanian coal beds, Geological Society of America, Special Paper 114 , 105-142.
- White, D. (1929). Flora of the Hermit Shale, Grand Canyon, Arizona , Carnegie Institute of Washington, Publication No. 405, 221 pp.
- White, D. (1933). Some features of the Early Permian flora of North America, 16 th International Geological Congress 1 , 679-689.
- Willard, D. A. (1989). Source plants for Carboniferous microspores: Lycospora from permineralized Lepidostrobus , American Journal of Botany 76 , 820-827.
- Willard, D. A. (1993). Vegetational patterns in the Springfield Coal (Middle Pennsylvanian; Illinois Basin): Comparison of miospore and coal-ball records, in Modern and Ancient Coal-Forming Environments , J. C. Cobb, editor; and C. B. Cecil, editor. , eds., Geological Society of America Special Paper 286, Boulder, Colo., pp.139-152.
- Willard, D. A., and T. L. Phillips (1993). Paleobotany and palynology of the Bristol Hill Coal Member (Bond Formation) and Friendsville Coal Member (Mattoon Formation) of the Illinois Basin (upper Pennsylvania), Palaios 8 , 574-586.
- Wing, S. L. (1988). Taxon-free paleoecological analysis of Eocene megafloras from Wyoming, American Journal of Botany 75 (6-part 2), 120 (Abstract).
- Wing, S. L., and B. H. Tiffney (1987). The reciprocal interaction of angiosperm evolution and tetrapod herbivory, Review of Palaeobotany and Palynology 50 , 179-210.
- Winston, R. B., and R. W. Stanton (1989). Plants, coal, and climate in the Pennsylvanian of the central Appalachians, in Carboniferous Geology of the Eastern United States , 28th International Geological Congress, Field Trip Guidebook T143, pp.118-126.
- Wnuk, C. (1985). The ontogeny and paleoecology of Lepidodendron rimosum and Lepidodendron bretonense trees from the middle Pennsylvanian of the Bernice Basin, Sullivan County, Pennsylvania, Palaeontographica 195B , 153-181.
- Wnuk, C., and H. W. Pfefferkorn (1987). A Pennsylvanian-age terrestrial storm deposit: Using plant fossils to characterize the history and process of sediment accumulation, Journal of Sedimentary Petrology 57 , 212-221.
- Wolfe, J. A., and G. R. Upchurch (1986). Vegetation, climatic and floral changes at the Cretaceous-Tertiary boundary, Nature 324 , 148-152.
- Ziegler, A. M. (1990). Phytogeographic patterns and continental configurations during the Permian Period, in Palaeozoic Palaeogeography and Biogeography , W. S. McKerrow, editor; and C. R. Scotese, editor. , eds., Geological Society of London Memoir, pp.363-379.
- Ziegler, A. M., R. K. Bambach, J. T. Parrish, S. F. Barrett, E. H. Gierlowski, W. C. Parker, A. Raymond, and J. J. Sepkoski (1981). Paleozoic biogeography and climatology, in Paleobotany, Paleoecology and Evolution , Volume 2,K.J. Niklas, editor. , ed., Praeger, New York, pp.231-266.
- PubMedLinks to PubMed
- The Response of Hierarchially Structured Ecosystems to Long-Term Climatic Change...The Response of Hierarchially Structured Ecosystems to Long-Term Climatic Change: A Case Study using Tropical Peat Swamps of Pennsylvanian Age - Effects of Past Global Change on Life
- RIPOR family member 3 isoform X1 [Danio rerio]RIPOR family member 3 isoform X1 [Danio rerio]gi|688561987|ref|XP_009300972.1|Protein
- Danio rerio Unc119c (unc119c) mRNA, complete cdsDanio rerio Unc119c (unc119c) mRNA, complete cdsgi|14587077|gb|AF387342.1|Nucleotide
- Zebrafish DNA sequence from clone DKEY-47M24 in linkage group 13, complete seque...Zebrafish DNA sequence from clone DKEY-47M24 in linkage group 13, complete sequencegi|57977123|emb|CR788247.9|Nucleotide
- inpp5l inositol polyphosphate-5-phosphatase L [Danio rerio]inpp5l inositol polyphosphate-5-phosphatase L [Danio rerio]Gene ID:641571Gene
Your browsing activity is empty.
Activity recording is turned off.
See more...