NCBI Bookshelf. A service of the National Library of Medicine, National Institutes of Health.
National Research Council (US) Panel on Effects of Past Global Change on Life. Effects of Past Global Change on Life. Washington (DC): National Academies Press (US); 1995.
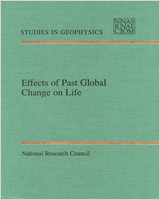
Effects of Past Global Change on Life.
Show detailsGerta Keller
Princeton University
Katharina V. Salis Perch-Nielsen
Swiss Federal Institute of Technology
Abstract
The effects of the Cretaceous-Tertiary (K/T) boundary global change on calcareous nannoplankton and planktic foraminifera are most severe in low latitudes and negligible in high latitudes. In low latitudes, species extinctions are complex and prolonged beginning during the final 100,000 to 300,000 yr of the Cretaceous, accelerating across the K/T boundary, and reaching maximum negative conditions between 10,000 and 40,000 yr into the Tertiary accompanied by low primary productivity. In high latitudes, no significant species extinctions occurred at or near the K/T boundary, and all dominant species thrived well into the early Tertiary. Return to a more stable ecosystem and to increased marine productivity in low latitudes does not occur until about 250,000 to 350,000 yr after the K/T boundary, coincident with the extinction of Cretaceous survivors in high latitudes. Within this transition interval, habitats of deep- and intermediate-dwelling tropical planktic foraminiferal species are gradually and selectively eliminated in low latitudes, and by K/T boundary time only cosmopolitan surface dwellers survive. This implies the disruption of the water-mass structure, change in the thermocline, and a drop in surface productivity. Although no single cause is likely to account for these different prolonged and dramatic faunal and environmental changes between low and high latitudes, long-term oceanic instability associated with sea-level, temperature, salinity, and productivity fluctuations may account for most of the faunal changes observed in planktic foraminifera. However, other environmental changes (e.g., volcanism, bolide impact) may have accelerated the demise of the low latitude Cretaceous fauna already on the decline.
Introduction
The Cretaceous-Tertiary boundary transition is marked by one of the most dramatic environmental changes in the Earth's history, with both cause and effect still vigorously disputed. Presently the most popular theory of the cause of this global change is a large extraterrestrial bolide impact. Supporters of this theory cite anomalously high concentrations of noble elements and shocked mineral grains in a thin boundary clay layer in the marine and terrestrial realm as sufficient evidence of a bolide impact. In addition, Alvarez et al. (1980) viewed the reported sudden extinction of all but one Cretaceous planktic foraminiferal species as evidence of the catastrophic effect of this impact on Earth's biota. Alternative theories invoke long-term Earth-derived causes such as large-scale volcanism (McLean, 1985; Officer and Drake, 1985), mantle plume activity (Loper and McCartney 1986, 1988), sea-level fluctuations, and climate changes (Hallam, 1989) as seen in the geological record spanning the K/T boundary transition, as well as the decline and extinction of many fossil groups during the late Maastrichtian (Kauffman, 1984; Sloan et al., 1986; Keller, 1888, 1989b; Keller and Barrera, 1990; Canudo et al., 1991). Although no evidence presented to date conclusively supports either extraterrestrial or Earth-derived causes, the magnitude of this global change remains undisputed. What is unclear, however, is the nature, tempo, and geographic extent of this mass extinction.
Regardless of the ultimate cause of this global change, the effect on life and especially on calcareous marine microplankton can be evaluated from the geological record. Shells of calcareous nannofossils and planktic foraminifera contribute the bulk of marine sediments and provide a record not only of species extinction and evolution, but also of population dynamics in response to environmental perturbations. A record of climate and productivity changes is retained in the carbon and oxygen isotope ratios of their shells. No other fossil group provides as much information on the changing environment across the K/T boundary transition.
Despite the rich information contained in the calcareous marine microplankton, specialists still differ considerably in their interpretation of the extinction record, with one group arguing for catastrophic extinctions of nearly the entire fauna and flora at the K/T boundary and the other group arguing for an extended extinction period. Among nannofossil experts, the difference in opinion centers on whether Cretaceous species in Tertiary sediments are reworked or survivors (Perch-Nielsen, 1979a,b; Perch-Nielsen et al., 1982; Thierstein, 1982; Jiang and Gartner, 1986). Unfortunately, the very small size of nannofossils, and their easy transportation and redeposition, make determination of in situ assemblages a nontrivial problem, and neither view can be proven beyond reasonable doubt.
Among foraminiferal experts, differences rest largely on two apparently contradictory extinction records, the deep-sea versus continental shelf or neritic environments. Deep-sea records show all but one Cretaceous species extinct at the K/T boundary, apparently supporting a catastrophic impact (e.g., Smit and Romein, 1985; D'Hondt and Keller, 1991). In contrast, neritic sections show an extended period of extinctions beginning before the K/T boundary and continuing well into the Tertiary; thereby providing only weak support for a geologically instantaneous catastrophic event (Keller, 1988, 1989a,b; Canudo et al., 1991; Huber, 1991). Impact supporters reconcile these different records by interpreting Cretaceous species present above the K/T boundary as reworked (e.g., Smit, 1982, 1990; Smit and Romein, 1985).
Unlike the unresolved problem of reworked nannofossils, reworked Cretaceous foraminiferal species in Tertiary deposits can be positively identified by their physical and preservational characteristics, as well as by their δ13C isotope signal (Barrera and Keller, 1990; Keller et al., 1993). Based on these criteria, up to one-third of the Cretaceous species may have survived the K/T boundary event. In contrast, the near total extinction of Cretaceous species in the deep-sea appears to be an artifact of a temporally incomplete stratigraphic record as discussed below (MacLeod and Keller, 1991a,b). Recently, the study of marine K/T sections in northern and southern high latitudes has revealed no significant species extinctions and the survival of nearly all species well into the Tertiary, which indicates that the effects of the K/T mass extinction may have been limited to lower latitudes (Keller, 1993; Keller et al., 1993).
In this chapter we examine the effects of global environmental changes on calcareous marine microplankton across the K/T boundary in deep-sea and continental shelf sequences. We have chosen the temporally most complete sections known to date: El Kef in Tunisia, Caravaca in Spain, Brazos River in Texas, Deep-Sea Drilling Program (DSDP) Site 528 in the South Atlantic, and Ocean Drilling Program (ODP) Site 738C in the Indian Antarctic Ocean (Figure 4.1). These four sections span bathyal to neritic environments from high to low latitudes and thus provide a cross section of marine ecosystems. We address four critical and interrelated topics:

Figure 4.1
Locations of K/T boundary sections examined for this study plotted on a paleogeographic reconstruction of continental positions at the time of the K/T boundary (66.4 Ma). White = ocean basins; light stipple = continental platforms; black = inferred extent (more...)
- 1.
How complete are K/T boundary sequences?
- 2.
What is the nature of species extinctions?
- 3.
What is the effect on dominant species populations?
- 4.
Are specific habitats selectively destroyed?
How Complete Are K/T Boundary Sections?
To test competing causal hypotheses or to evaluate the effect of global change on marine microplankton, accurate estimates of the stratigraphic and temporal completeness of individual K/T boundary sections are needed. In current stratigraphic analysis the blueprint for judging complete and continuously deposited K/T boundary sequences seems to be the presence and relative thickness of a boundary clay, the presence of nannofossil zones Micula prinsii and Neobiscutum romeinii and planktic foraminiferal Zone Guembelitria cretacea (P0) followed by the Parvularugoglobigerina eugubina Zone (Pla) (Perch-Nielsen et al., 1982; Smit, 1982; Smit and Romein, 1985). By this definition, many K/T boundary sections have been judged as "relatively complete" even though the earliest Tertiary Zone P0 or the N. romeinii Zone are missing. Moreover, this method of judging chronostratigraphic completeness does not allow the recognition of the existence of intrazonal hiatuses, and may thus lead to erroneous interpretations of the nature and rate of environmental change.
Foraminiferal workers have generally examined deep-sea sections, which were believed to be more complete than shallower continental shelf sections, and found that virtually all planktic foraminiferal species disappeared simultaneously at the K/T boundary. This pattern of species extinctions is illustrated in Figure 4.2 for DSDP Site 528, which contains a relatively continuous sedimentary record with a thin laminated boundary interval (in the core catcher) (D'Hondt and Keller, 1991). Yet, as in nearly all deep-sea sections, the basal Tertiary Zone P0 and probably part of Zone Pla are missing. By judging DSDP Site 528, as well as all other low- to mid-latitude deep-sea sites that show the same sudden mass extinction and absence of the basal Tertiary Zone P0 as representing a temporally complete record, the obvious interpretation was a geologically instantaneous catastrophic event such as a bolide impact. This interpretation has even been applied to the stratigraphically more complete K/T boundary sequences at El Kef and Caravaca by Smit (1982, 1990), based on the assumption that all Cretaceous species present above the K/T boundary (except G. cretacea) are reworked (see Canudo et al., 1991, for a discussion of this problem).

Figure 4.2
Stratigraphic ranges of planktic foraminifera at DSDP Site 528 (data from D'Hondt and Keller, 1991). Note that the abrupt termination of all Cretaceous species and sudden appearance of Tertiary species are the result of a hiatus that encompasses Zone (more...)
Among nannofossil workers, Worsley (1974) speculated that major hiatuses characterized all deep-sea sections and shorter hiatuses were present in marine-shelf sections. This observation tended to be supported by the later discovery of the new uppermost Maastrichtian nannofossil Zone Micula prinsii and an unnamed basal Tertiary interval below the Neobiscutum romeinii subzone at El Kef by Perch-Nielsen (1979a). Despite this early recognition of an incomplete K/T boundary record in the deep-sea by both foraminiferal and nannofossil workers, few attempts at systematic chronostratigraphic analysis have been made and only for a single or a few sections (Thierstein, 1982; Herbert and D'Hondt, 1990), largely because high-resolution stratigraphic records have not been available.
Thus, what has been missing from the K/T controversy is a comprehensive chronostratigraphic and biostratigraphic data synthesis for all K/T transitions in marine depositional settings that would allow determination of the temporal completeness of each section and thereby clarify the nature of the extinction record. Such an analysis has recently been completed by MacLeod and Keller (1991a,b), who used the graphic correlation method of Shaw (1964) to compare the distribution of biostratigraphic and lithostratigraphic datums in 15 relatively complete boundary sections. These comparisons resulted in a composite estimate of 76 latest Maastrichtian to Early Paleocene (Zones P0-P1c) biostratigraphic datums for planktic foraminiferal and calcareous nannofossil species that corrects for intersequence diachroneity and allows correlations for individual sections to be made within a common chronostratigraphic model. Based on this method, analysis of 29 K/T boundary sections suggests that short, global, intrazonal hiatuses of varying duration are present in virtually all sections previously considered relatively complete. Moreover, dramatically different patterns of sediment accumulation appear to characterize neritic and deep-sea depositional environments following the K/T boundary.
Figure 4.3 illustrates this pattern for the sections discussed in this chapter along with the sea-level curve of Brinkhuis and Zachariasse (1988) based on dinoflagellate data from El Kef. Minimum estimates for hiatuses are given in black; maximum estimates are shown in stippled pattern. At DSDP Sites 528 and 577 as well as 10 other deep-sea sections examined (MacLeod and Keller, 1991a,b) an interval of nondeposition or short hiatus, which includes Zone P0 and the lower part of Zone P1a, characterizes the earliest Tertiary. This hiatus is coincident with a rapid transgression following the pre-K/T boundary maximum regression. Sediment accumulation occurs at this time, mainly in mid to outer shelf and upper bathyal environments as indicated by the presence of Zone P0, although a condensed interval or very short hiatus may be present in these sections also (Brazos, Caravaca, Agost). This sediment pattern suggests that the rapid sea-level transgression in Zone P0 trapped terrigenous sediment and organic carbon on continental shelves and temporarily deprived deep ocean basins of an inorganic sediment source and enhanced carbonate dissolution (Berger, 1970; Berger and Winterer, 1974; Loutit and Kennett, 1981; Haq et al., 1987; Donovan et al., 1988). Increased carbonate dissolution in the basal Tertiary (Zone P0) is widely recognized in virtually all marine K/T boundary sequences (Keller, 1988, 1993; Canudo et al., 1991; D'Hondt and Keller, 1991; Schmitz et al., 1992). A second short hiatus is frequently present near the P1a/P1b Zone boundary (Figure 4.3), and like the P0/P1a, a hiatus may mark a sea-level lowstand. With the possible exception of El Kef, these two hiatuses appear to be present in the Spanish, Texan, and Israeli sections (Keller et al., 1990; MacLeod and Keller, 1991a,b). At El Kef an interval of reduced sedimentation (stippled pattern) may be present. A similar hiatus and dissolution pattern was observed at ODP Site 738 in the Indian Antarctic Ocean, where deposition occurred at about 1000-m depth (Keller, 1993).

Figure 4.3
Extent and temporal distribution of intrazonal hiatuses plotted against the eustatic sea-level curve of Brinkhuis and Zachariasse (1988). Biostratigraphic zonation from Keller (1988) as modified by Keller and Benjamini (1991).
It is evident from these analyses that neritic sequences such as those at El Kef and Brazos River, upper bathyal to outer neritic sequences such as those at Agost and Caravaca, and the upper slope Antarctic Ocean Site 738 contain the most detailed records of biological and environmental changes for the K/T transitions. Therefore, it is imperative that the effect of global change on marine microplankton be examined in these temporally most complete K/T boundary transitions.
Species Response To K/T Disturbance
Planktic Foraminifera
Are species extinctions across the K/T boundary geologically instantaneous or successive and taxonomically selective? If species extinctions appear geologically instantaneous as illustrated for planktic foraminifera in deep-sea Site 528 (Figure 4.2), then the environmental change must have been caused by a major catastrophe, provided there is no hiatus in the sediment record. However, as discussed in the previous section, a geologically instantaneous mass extinction at the K/T boundary must now be ruled out because this pattern is a result of truncation by a hiatus (Figures 4.2 and 4.3). If species extinctions appear systematic, sequentially eliminating certain morphologies, geographically limited taxa, or habitats over an extended time interval, then long-term environmental changes such as changes in climate, sea-level, rates of seafloor spreading, volcanism, and atmospheric pCO2 are likely causes. However, an extended species extinction pattern does not necessarily rule out the possibility that an extraterrestrial impact (albeit of lesser magnitude than that proposed by the impact theory) occurred some time during this interval. However, recognizing such an impact, and separating its effects on marine microplankton from already ongoing environmental changes, are difficult, if not impossible.
The pattern of planktic foraminiferal species extinctions in low latitudes across the K/T boundary transition is best exemplified by the Tunisian El Kef section, which contains the most complete boundary sequence known to date. Figure 4.4 illustrates the sequence of species extinctions at El Kef along with illustrations of species and their sizes relative to each other. By comparing the apparent species extinction pattern in deep-sea Site 528 (Figure 4.2) with that of the mid to outer neritic El Kef section, it is immediately obvious that the patterns of extinction and evolution are dramatically different. Whereas species first and last appearances cluster at the K/T boundary at Site 528, an extended pattern beginning at least 25 cm below the boundary and ending about 4 m above it (Zone P1a) is observed at El Kef. (Note that the scale is reduced for the late Maastrichtian interval, relative to the Paleocene and hence concentrates these extinctions near the boundary.) Fourteen species or 33% disappear at 25 cm (6 species) and 7 cm (8 species) below the K/T boundary. Twelve species extinctions (28%) coincide with the K/T boundary, and 6 species (14%) disappear 15 cm above the boundary. Of the remaining 16 Cretaceous species (38%), 8 species disappear in Zones P0 or basal Zone P1a; the remaining 8 species die out gradually in Zones P1a or basal P1b. This extended species extinction pattern is not unique to El Kef, but has also been observed at other low latitude sections including Brazos River (Keller, 1989a,b), Agost, and Caravaca (Canudo et al., 1991). Such similar extinction patterns in regions as widely separated as Tunisia, Spain, and Texas argue against this being an artifact of sample spacing or reworking.

Figure 4.4
Stratigraphic ranges of planktic foraminifera across the K/T boundary at El Kef. Note the early disappearance of tropical, large, complex, deep, and intermediate water dwellers at or before the K/T boundary and the survival of smaller cosmopolitan surface (more...)
Moreover, species extinctions appear to be systematic, affecting tropical complex, large, and highly ornamented morphologies first (globotruncanids, racemiguembelinids, planoglobulinids) followed by subtropical and somewhat smaller morphologies (pseudotextularids, rugoglobigerinids; Figure 4.4). Still, smaller, less ornamented, robust cosmopolitan species with triserial, biserial, and rotalid morphologies (guembelitrids, heterohelicids, globigerinellids) survive the K/T boundary event and disappear gradually during the first 200,000 yr of the Tertiary. Within Tertiary sediments, these survivor species are generally dwarfed, with specimen size averaging about half the size of their Cretaceous ancestors. Their preservation is indistinguishable from the Tertiary fauna in the same samples, indicating that their origin is not likely due to redeposited Cretaceous sediments (Keller, 1988, 1989a,b). Moreover, stable isotopic signatures of the Cretaceous species Guembelitria cretacea and Heterohelix globulosa, unambiguously measure Tertiary values (Barrera and Keller, 1990; Keller et al., 1993). However, despite their ubiquitous presence in lower Danian sediments of continental shelf sections, these Cretaceous foraminifers have been routinely interpreted as reworked and consequently ignored or eliminated from faunal distribution lists (Hofker, 1960; Olsson, 1960; Berggren, 1962; Smit, 1982, 1990; Olsson and Liu, 1993). This interpretation has led to erroneously depicting a sudden extinction of all but one (G. cretacea) Cretaceous species exactly at the K/T boundary, as most recently illustrated by Smit (1990) and challenged by Canudo et al. (1991). Moreover, recent studies of high latitude sequences in Denmark (Nye Klov) and the Indian Antarctic Ocean (ODP Site 738C) have shown that virtually all species survived the K/T boundary and thrived 200,000 to 300,000 yr into the Tertiary (Keller, 1993; Keller et al., 1993). This Cretaceous survivor fauna includes the same cosmopolitan taxa that survived the K/T boundary in low latitudes. In contrast to the more specialized tropical and subtropical taxa, these cosmopolitan species were probably tolerant of wide-ranging temperature, oxygen, salinity, and nutrient conditions.
Evolution of Tertiary species begins immediately after the K/T boundary with the appearance of very small, unornamented, and "primitive" morphologies (Figure 4.4). In these aspects they are similar to the surviving Cretaceous fauna. Cretaceous survivors gradually disappeared as Tertiary species diversified and somewhat larger morphotypes appeared. Species diversity, which declined near the K/T boundary from an average of 45 species to about 10 species in the earliest Tertiary Zone P0 and increased to about 15 species in Zone P1a (Figure 4.4) failed to recover until Zone P1c or about 300,000 yr after the K/T boundary. In high latitudes, this recovery coincides with the disappearance of the Cretaceous survivor taxa (Keller, 1993; Keller et al., 1993).
Calcareous Nannoplankton
Detection of the calcareous nannoplankton species extinction pattern across the K/T boundary transition is seriously hampered by the ease with which these specimens are reworked and the fact that there is usually no way to tell a reworked from an in situ nannofossil based on the state of preservation. For instance, Jiang and Gartner (1986) noted that of nearly 70 species considered to be "vanishing Cretaceous species," only 2 were not found in at least one of up to 60 samples from the 6 m of sediments representing Tertiary Zones NP1 and NP2. The relative abundance of common Cretaceous species, however, decreases gradually up-section as illustrated in Figure 4.5 (Jiang and Gartner, 1986). Recently, a quantitative study of Antarctic Ocean ODP Site 690 by Pospichal and Wise (1990) has revealed a very similar gradual decrease of Cretaceous species in the early Tertiary (Zone CPla; Figure 4.6). A similar pattern is also observed at El Kef where a rapid decrease of Cretaceous species also occurs in nannofossil Zone NP1, but few are still present in Zones NP2 to NP3 (Figure 4.7; Perch-Nielsen et al., 1982). No quantitative data are available from DSDP Sites 577 and 528. However, at the South Atlantic Site 524, Percival (1984) also noted a gradual decline and subsequent disappearance of Cretaceous species in Zone NP1. These patterns of gradual decline of Cretaceous taxa in Tertiary sediments in sections spanning from low to high latitudes are very similar to those observed in planktic foraminifera and strongly suggest survivorship of at least some Cretaceous taxa.

Figure 4.5
Abundance of dominant calcareous nannofossils (percent) across the K/T boundary in a Brazos River section (modified from Jiang and Gartner, 1986).

Figure 4.6
Abundance of dominant calcareous nannoplankton (percent) across the K/T boundary at ODP Site 690, Weddel Sea, Antarctic Ocean (data from Pospichal and Wise, 1990).

Figure 4.7
Relative abundance of Cretaceous calcareous nannofossils in basal Tertiary sediments at El Kef. Data based on counts of Cretaceous specimens encountered during a 10-min examination per sample with an optical microscope at 1000x.
As among planktic foraminifera, the vanishing Cretaceous species in lower Tertiary sediments are essentially the same group of species that are common in terminal Cretaceous sediments: Arkhangelskiella cymbiformis, Cretarhabdus crenulatus, Eiffellithus turriseiffelii, Micula decussata, Prediscosphaera cretacea, Nephaolithus frequens, Kamptnerious magnificus, and Watznaueria barnesae. An exception is Prediscosphaera quadripunctata (P. stoveri of other authors), which becomes rare above the boundary. Species of Braarudosphaera and the calcareous dinoflagellate genus Thoracosphaera are the only persistent species that become considerably more common above the boundary in the T. imperforata subzone.
Measurements of the size distribution of several Maastrichtian species (Arkhangelskiella cymbiformis, Eiffellithus turriseiffelii, Micula decussata, Prediscosphaera cretacea s. ampl.) from samples just below and just above the boundary in El Kef indicate no significant differences in the size of the specimens, but stable isotope values of δ13C and δ18O from fine fraction carbonate show dramatic changes across the boundary (Perch-Nielsen et al., 1982). Since the fine fraction carbonate consists primarily of Maastrichtian calcareous nannofossils (100% below the boundary and >90% above the boundary), it is difficult to explain how reworked Maastrichtian nannofossils could carry a Tertiary isotope signature. This originally led to the suggestion that most Maastrichtian nannoplankton species survived the K/T boundary crisis (Perch-Nielsen, 1981; Perch-Nielsen et al., 1982). More studies of this nature will be necessary to differentiate Cretaceous survivors from reworked populations in Tertiary sediments.
Population Response to K/T Disturbance
Relative abundance changes in dominant species groups of both planktic foraminifera and nannofossils yield a more reliable measure of environmental disturbance than systematic diversity. For instance, at the K/T boundary, virtually all planktic foraminiferal species that became extinct were numerically rare (<1%) in the latest Cretaceous ocean, and even relatively minor disturbances of the ecosystem may have caused their demise. In contrast, survivor species dominated the faunal assemblages. In this group, disturbance of the ecosystem is reflected in relative abundance changes and particularly the terminal abundance decline. In each of the sections examined (except Site 528), there are strong similarities in the timing of faunal change among planktic foraminifera, although faunal compositions vary from one region to another.
Among calcareous nannofossils the most commonly preserved taxa in the uppermost Maastrichtian are also still the most common in basal Tertiary deposits as also observed in planktic foraminifers (Figures 4.5 and 4.6). However, since these taxa did not give rise to the new Tertiary species and their presence in Tertiary deposits may be due to reworking, Jiang and Gartner (1986) labeled them ''Cretaceous species" (Figure 4.5). Taxa they considered "survivors" or persistent species are very rare in the Maastrichtian and become dominant only in early Tertiary sediments of middle to high latitudes in the Atlantic (Denmark, Biarritz, Zumaya, DSDP Site 524) and Antarctic Oceans (Figure 4.6). These taxa include relatively large extant and new species of the genera Biscutum, Cyclagelosphaera, Markalius, Neocrepidolithus, Zygodiscus, and Placozygus (Figures 4.5 and 4.6). In contrast, such survivors are rare in lower latitudes of the Tethyan Sea (Caravaca and El Kef), where very small new species appear and soon dominate the assemblages. In addition to these floral changes there are increases in the genera Thoracosphaera and Braarudosphaera in both realms, although blooms of the latter are not observed in all sections. The faunal and floral turnovers at El Kef, Caravaca, Brazos, DSDP Site 528, and ODP Site 738 are discussed below.
El Kef, Tunisia
Figure 4.8 shows the relative abundances of survivor species of planktic foraminifera at El Kef along with changes in δ13C values for planktic and benthic foraminifera (data from Keller, 1988; Keller and Lindinger, 1989). The K/T boundary, defined by the first appearance of Tertiary planktic foraminifera and nannofossils, coincides with an iridium anomaly and spinels (Kuslys and Krahenbuhl, 1983; Robin et al., 1991), a lithological shift from tan colored marls to black clay with a thin basal red layer, a shift of -3.0%o in δ13C values, and elimination of the surface-to deep δ13C gradient, which suggests a drop in surface productivity (Zachos and Arthur, 1986; Keller and Lindinger, 1989). At El Kef the terminal decline of survivor species begins about 10 cm (or about 8000 to 10,000 yr) after the K/T boundary and leads the to near disappearance (<1%) of this group within a few thousand years.

Figure 4.8
Abundance of Cretaceous planktic foraminiferal species (percent) across the K/T boundary at El Kef in relation to δ13C values of benthic foraminifera and sediment fine fraction. Note the terminal decline of Cretaceous taxa (except for G. cretacea) (more...)
The decline of the Cretaceous species and drop in δ13C values are accompanied by the rise and dominance of Guembelitria cretacea (a Cretaceous survivor) in Zone P0 and the evolution of Tertiary species. Figure 4.9 illustrates a sequence of rapidly evolving and changing dominant faunal components in the earliest Tertiary Zones P0 and P1a that seem to be related to low surface productivity as indicated by the very low δ13C values, also observed in numerous low latitude sections (Zachos and Arthur, 1986; Barrera and Keller, 1990). Increased surface productivity (higher δ13C values) at the top of Zone P1a (4 m above K/T) coincides with the disappearance of dominant earliest Tertiary species (G. conusa, P. eugubina), evolution of new species, and increased diversity (Keller, 1988, 1989b; Keller and Lindinger, 1989). This initial recovery of the ecosystem occurred about 250,000 to 350,000 yr after the K/T boundary (Keller, 1988, 1993).

Figure 4.9
Abundance of dominant planktic foraminiferal taxa (percent) in the early Danian at El Kef in relation to benthic (A. acuta) and planktic (<25 mm sediment fraction) δ13C values. Note the increase in species diversity coincident with (more...)
Among calcareous nannofossils, semiquantitative data from Perch-Nielsen (1981) indicate that the first group to bloom in the basal Tertiary are species of Thoracosphaera followed by Neobiscutum romeinii and N. parvulum. The interval with common N. parvulum also contains common Lanternithus minutus, Braarudosphaera bigelowii, Chiastozygus ultimus, Cruciplacolithus primus, and Placozygus sigmoides. This increased floral diversification corresponds to the initial increase in surface productivity and first major increase in foraminiferal diversity (top of P1a) that marks the initial recovery of the ecosystem at El Kef (Figure 4.9). The N. parvulum abundance peak is followed by abundance peaks in Futyania petalosa (Toweius petalosus), Praeprinsius of P. dimorphesus, and Octolithus multiplus (Perch-Nielsen, 1981).
Caravaca, Spain
The planktic foraminiferal assemblages that dominate during the latest Maastrichtian at Caravaca (as well as Agost) are similar to those at El Kef, although species abundance varies significantly (Figure 4.10; Canudo et al., 1991). The K/T boundary is characterized by the same species datums as well as lithological and geochemical markers (Robin et al., 1991), but the boundary clay is much thinner (7 cm) than at El Kef (50 cm). Species abundances are variable across the boundary. Heterohelix navarroensis, H. glabrans, and H. globulosa decline in abundance 5 to 10 cm below the boundary, whereas H. globulosa, Pseudotextularia costulata, P. kempensis, Globigerinelloides aspera, and G. yaucoensis remain the same or increase in the boundary clay. All Cretaceous species, except G. cretacea, decline to <2% immediately above the clay layer. As indicated earlier, this sharp faunal change marks a short hiatus (Figure 4.3), and part of the anomalous abundance increase in P0 may be due to reworking of Cretaceous taxa. Nevertheless, these relative abundance changes suggest ecological disturbances beginning prior to the K/T boundary and continuing into the earliest Tertiary (7-cm clay layer).

Figure 4.10
Percentage CaCO3, δ13C values, and abundance of dominant planktic foraminiferal species (percent) across the K/T boundary at Caravaca, SE Spain. Note the presence of abundant Cretaceous specimens in the boundary clay (Zone P0).
At Caravaca and El Kef, similar sequences of rapidly evolving and changing dominant planktic foraminiferal components are present in the earliest Tertiary, including the maximum abundances of P. longiapertura, P. eugubina, and Woodringina (Canudo et al., 1991). However, because the earliest Tertiary at Caravaca is more condensed and contains two short hiatuses (Figure 4.3), the ranges are truncated. Similar to El Kef, δ13C values at Caravaca also show low surface productivity through Zone P0 and the lower part of Zone P1a, with initial recovery in the upper part of this zone (Figure 4.10).
Calcareous nannofossil distribution at Caravaca is also similar to El Kef, with a succession of dominant Thoracosphaera, B. bigelowii, and N. parvulum parallel with the major decrease of Cretaceous nannofossils in the basal Tertiary (Romein, 1979; Romein and Smit, 1981). Differences in floral successions include the possible absence of a bloom of N. romeinii, which has not yet been studied, and the occurrence of common Octolithus multiplus preceding an abundance peak of F.petalosa.
Brazos, Texas
Brazos River sections do not have a well-defined boundary clay but contain a 2-mm thin red-brown layer that exhibits the iridium anomaly (Beeson et al., in preparation). The first appearance of Tertiary foraminifera and nannofossils places the K/T boundary at this thin red-brown layer and Ir anomaly (Jiang and Gartner, 1986; Keller, 1989a) and at the onset of the δ13C shift in the Brazos Core section (Barrera and Keller, 1990) (Figure 4.11). The same group of Cretaceous survivors dominates foraminiferal assemblages, although because of the shallow neritic water depth, H. globulosa is most abundant. There is no major faunal change apparent below or at the K/T boundary. Above the boundary, Cretaceous species thrive well into Zone P1a (1 m above the K/T) similar to Nye Klov, Denmark (Keller et al., 1993), in contrast to Caravaca and El Kef where Cretaceous survivors become rare within or immediately above Zone P0. However at Brazos, H. globulosa populations also begin their terminal decline in Zone P0, parallel with a gradual decline in δ13C values of H. globulosa and the benthic species Lenticulina (Figure 4.11; Barrera and Keller, 1990). This is the first time that the δ13C shift has been observed as a gradual rather than a sudden change (Hsu et al., 1982; Zachos et al., 1986) and implies deteriorating environmental changes beginning within a few thousand years of the K/T boundary.

Figure 4.11
Abundnace of planktic foraminifera (percent) and δ13 C values of benthic (solid diamond = Lenticulina sp.; open diamond = analysis of single specimens) and planktic (triangles = H. globulosa) foraminifera across the K/T boundary in the Brazos (more...)
Above the K/T boundary the Cretaceous survivors G. cretacea and G. trifolia dominate (50 to 60%) through Zones P1a-P1b (Figure 4.11). Such prolonged dominance of this group has not been observed in other sections and seems to be the result of local ecological conditions. However, the evolutionary sequence of early Tertiary planktic foraminiferal species is similar to that of Nye Klov, ODP Site 738C, El Kef, and Caravaca, although P. eugubina is rare, presumably because of the shallow neritic environment. The δ13C curve is similar to El Kef and Caravaca in that low surface productivity prevails during most of Zone P1a, and increasing but fluctuating values mark the top of Zone P1a (Figure 4.11; Keller, 1989a; Barrera and Keller, 1990).
Calcareous nannofossil abundance changes in the nearby Brazos-1 section show a similar pattern of rapid decline beginning near the K/T boundary and ending about 1 m above (Jiang and Gartner, 1986; Figure 4.5). The decline of the Cretaceous species is accompanied by increased abundance of Thoracosphaera spp. followed by Braarudosphaera and N. romeinii. As at El Kef and Caravaca there are some differences in the succession of N. parvulum and F. petalosa abundance distributions. At Brazos, abundance peaks of these taxa overlap with the maximum abundance of C. primus, whereas at Caravaca and El Kef the C. primus and F. petalosa peaks follow after the abundance peak of N. parvulum. It is possible that these differences are due primarily to differing taxonomic concepts among nannofossil workers especially for N. parvulum, a very small species that is difficult to identify with an optical microscope.
DSDP Site 528, South Atlantic
Relative abundances of planktic foraminiferal species are illustrated in Figure 4.12 for Site 528. Although the K/T boundary is located between two cores, a laminated boundary transition was recovered in the core catcher (D'Hondt and Keller, 1991). As noted earlier, Site 528 has a hiatus or interval of nondeposition at the K/T boundary and Zone P0, and the lower part of Zone P1a is missing (Figure 4.3). Moreover, nannofossil assemblages lack the characteristic basal Tertiary taxa N. romeinii, N. parvulum, and F. petalosa (Manivit, 1984; Manivit and Feinberg, 1984). Instead, a typical high latitude Zone NP1 assemblage with common Thoracosphaera and Braarudosphaera is present immediately above the K/T boundary. Thus, it is because of a hiatus, rather than catastrophic extinctions, that all planktic foraminiferal species truncate at the K/T boundary and Tertiary species suddenly appear. Because of this hiatus, which is present in virtually all deep-sea sections (Worsley, 1974; MacLeod and Keller, 1991a,b), the ecological response to the K/T boundary disturbance cannot be evaluated from such deep-sea sections. However, it is interesting to note that as in continental shelf sections, a similar species assemblage consisting of small cosmopolitan heterohelicids, globigerinellids, and hedbergellids dominates the late Cretaceous at Site 528, and there seems to be a trend toward decreased abundance in the heterohelicids, globotruncanids, and globotruncanellids in the terminal Cretaceous, indicating a changing environment.

Figure 4.12
Abundance of dominant planktic foraminiferal species (percent) across the K/T boundary in DSDP Site 528 (data from D'Hondt and Keller, 1991). Note the sudden faunal change at the K/T boundary is due to a hiatus that encompasses Zone P0.
ODP Site 738C, Indian Antarctic Ocean
Relative abundances of planktic foraminifera for Site 738C are illustrated in Figure 4.13. In this southern high latitude section the K/T transition is preserved within a 15-cm-thick laminated layer (which has been analyzed at 1-cm intervals), with the K/T boundary and Ir anomaly 2 cm above the base of the laminated layer (Schmitz et al., 1991; Thierstein et al., 1991; Keller, 1993). Planktic foraminiferal changes are dramatic across the K/T transition, but do not coincide with the Ir anomaly and K/T boundary. No significant species extinctions coincide directly with the K/T boundary; instead, there is a gradual elimination of more specialized and generally rare taxa below the K/T boundary. Moreover, all dominant species survive well into the Tertiary and die out as the diversity of new Tertiary species increases (Zones P1b and P1c)

Figure 4.13
Planktic foraminiferal turnover at the centimeter-scale in the laminated interval that spans the K/T boundary in the Antarctic Indian Ocean ODP Site 738C. Note the absence of significant species extinctions at the K/T boundary, and the survivorship of (more...)
The major faunal turnover, however, begins 5 cm below the K/T boundary with a sharp decline in abundances of H. globulosa from 40 to 4% by K/T boundary time, the decline and disappearance of H. complanata, and gradual declines in G. aspera and G. subcarinatus and hedbergellids. The most dramatic change is seen in Chiloguembelina waiparaensis, which increased from 15 to 90% in the 4 cm below the K/T boundary as triserial taxa (guembelitrids) increased in Zone P0 and new Tertiary species evolved. All Cretaceous taxa are dwarfed beginning 4 cm below the K/T boundary and through Zone P0, and reach maturity at less than half their usual size.
Indian Antarctic Ocean Site 738C thus illustrates a record of long-term environmental deterioration beginning well before and continuing well after the K/T boundary. It also shows that the demise of the cosmopolitan latest Maastrichtian assemblage cannot have been caused by a K/T boundary bolide impact, but was related to the environmental changes that resulted in a change of sediment deposition from calcareous ooze to laminated clay. Clearly, there was no sudden and catastrophic mass extinction at Site 738C (or in the northern-high latitude Nye Klov section; Keller et al., 1993). In fact, it seems impossible to differentiate the effects of the proposed bolide impact from the continued environmental changes in progress. At most, the bolide impact may have hastened the demise of an already declining latest Cretaceous cosmopolitan fauna.
Stable isotope measurements of planktic and benthic foraminifera indicate that there is no negative δ13C shift across the K/T boundary. In fact, δ13C values remain stable or increase slightly (0.2%o, Barrera and Keller, 1994). This contrasts with the 3.0%o negative shift observed in low latitudes and supports the interpretation that the effects of the K/T boundary event may have been limited to low latitudes.
Magnitude of K/T Disturbance
Planktic Foraminifera
The magnitude of the K/T disturbance can be estimated from faunal and floral abundance changes. In Figure 4.14, planktic foraminiferal abundance data are summarized for all four sections. Species and their relative numerical abundances are grouped into (a) species extinct at or before the K/T boundary, (b) survivor species except Guembelitria, (c) Guembelitria, and (d) evolving Tertiary species. The most dramatic change among the five sections is seen in the relative abundances of all species extinct at or before the K/T boundary. For instance, in the Brazos Core (deposited in a middle to inner neritic environment), species extinctions account for less than 5% of the individuals in the total fauna, although one-third of the species disappear. At El Kef, Caravaca, and Site 528, which were deposited in outer neritic to upper bathyal and bathyal depths, respectively, only between 10 and 30% of the individuals are affected, whereas two-thirds of the species disappear. Moreover, Figure 4.14 shows that at El Kef and Site 528 there is a gradual decline in numerical abundance of the disappearing fauna beginning below the K/T boundary. In contrast to these low- and middle-latitude sites, almost all species survive the K/T boundary in the Indian Antarctic Ocean Site 738C (Keller, 1993). These data illustrate that a taxonomic census alone significantly overestimates the environmental effects of the K/T boundary crisis. However, these data also imply that the effect on faunal populations was variable between shallow nearshore and open marine environments, and between low or middle and high latitudes.

Figure 4.14
Faunal turnover across the K/T boundary in planktic foraminifera between midneritic and bathyal environments based on percentage of the population eliminated (number of individuals in all taxa that disappear at the K/T boundary) and percentage of the (more...)
The numerical abundance of Cretaceous survivors (excluding G. cretacea) shows a similar pattern between El Kef and Caravaca, with a relatively rapid decline above the K/T boundary over about 10,000 yr and a more gradual decline over about 30,000 to 40,000 yr at Brazos. No data are available for Site 528 because of a hiatus. At Site 738C the decline of Cretaceous survivors is masked by the concurrent increase in C. waiparaensis. The Cretaceous survivor Guembelitria generally thrived after the K/T boundary as illustrated at El Kef, but is not present in great abundance at Caravaca in Zones P0 and basal P1a. This absence may be due, however, to a short hiatus at this interval at Caravaca (Figure 4.3). A peak in Guembelitria abundance within Zone P1a is present, however, in both Caravaca and Site 528 (Figure 4.13), as well as El Kef (Figure 4.9), but not at high latitude Site 738C. At Brazos, Guembelitria is common throughout the Maastrichtian and increases in abundance in the early Tertiary. Thus, Figure 4.14 illustrates unequivocally that only a small group of the planktic foraminiferal population became extinct at the K/T boundary, that the dominant group gradually declined and became extinct well after the boundary, and that the adverse effects of the K/T boundary event diminished toward high latitudes. The magnitude of the K/T boundary disturbance is thus significantly overestimated if based on taxic diversity alone or if low- to high latitude differences are disregarded.
Calcareous Nannoplankton
Quantitative data to estimate the magnitude of the effect of the K/T boundary disturbances are available for El Kef, Brazos River (Perch-Nielsen et al., 1982; Jiang and Gartner, 1986), and ODP Sites 690, 738, and 761 (Pospichal and Wise, 1991; Wei and Pospichal, 1991; Pospichal and Bralower, 1992). However, as noted earlier, their interpretation is not unambiguous and depends on whether the so-called vanishing Cretaceous taxa are assumed to have survived at least partly into the basal Tertiary, or whether they are considered to be reworked above the K/T boundary.
If we assume that the vanishing Cretaceous species survived into the early Tertiary, then their abundance rapidly decreases to less than 10% by the N. romeinii subzone in the Brazos section. This decline in Cretaceous nannofossils parallels that of Cretaceous planktic foraminifers in the basal 1 m of the Tertiary at Brazos. A similar rapid decline in vanishing Cretaceous species is observed at El Kef. In higher-latitude sections (including DSDP Site 524, ODP Sites 690C, 738, and 761, Biarritz (France) and the Danish sections), vanishing Cretaceous species seem to remain relatively common (10 to 30%) into the upper part of NP1 (Perch-Nielsen, 1979a,b; Pospichal and Wise, 1991; Wei and Pospichal, 1991; Pospichal and Bralower, 1992). Their decrease is accompanied by a rapid increase in Thoracosphaera and, in high latitudes, also by an increase in persistent species.
If we assume that all vanishing Cretaceous species became extinct at the K/T boundary and their presence in Tertiary sediments is due to reworking, then the extinction rate exceeds 100 species per year, compared to an average of 1.5 species per million years (m.y.) and 5% per m.y. estimated by Perch-Nielsen (1986) and Roth (1987), respectively, for the Late Cretaceous. Given the uncertainties in this assumption, this estimate is not a satisfactory approximation of the magnitude of the K/T boundary event on the species level. Extinctions clearly occurred at a rate at least one order of magnitude higher than during the Late Cretaceous.
On the genus level about 18 of some 50 to 60 Cretaceous genera survived into the Tertiary (Perch-Nielsen, 1985, 1986). Among these, 4 genera are still extant; the remainder disappeared during the Paleocene (7 genera) and Eocene (7 genera) at an average rate of about 0.5 genus/m.y. This rate of extinction is similar to that of Paleocene to Middle Eocene floras in general. Because of the present ambiguity in determining whether Cretaceous species in basal Tertiary deposits are reworked or in situ, an accurate estimate of the effect of the K/T crisis based on nannoplankton cannot be obtained.
Are Specific Habitats Selectively Destroyed?
Most planktic foraminifera live in the upper 200 to 400 m of the water column and within this interval can be grouped into surface, intermediate, or deep dwellers based on their oxygen and carbon isotope ranking and the assumption that they grew their shells in isotopic equilibrium with the sea water in which they lived (see Table 4.1; Douglas and Savin, 1978; Boersma and Shackleton, 1981; Stott and Kennett, 1989; Lu and Keller, 1993). Species living in warm surface waters have the lightest δ18O and heaviest δ13C values, whereas species living in deeper, cooler waters have successively heavier δ18O and lighter δ13C values. Variations in the distribution of these groups may indicate environmental changes related to climate, ocean circulation, and water chemistry.
TABLE 4.1
Oxygen Isotope Ranking of Cretaceous Species.
Because stable isotope ranking of Cretaceous species is still preliminary and awaiting further analyses of individual species, Table 4.1 groups by genera. However, future isotope analyses may show that not all species within a genus inhabited the same depth environment, or that some species calcified their shells in disequilibrium with the seawater in which they grew. Heterohelix globulosa is one example of a species that calcified in isotopic disequilibrium or changed its habitat over time. In the Cretaceous, this species has been reported with relatively heavy δ18O values in the open ocean (although other heterohelicid species have light values), indicating that it lived at thermocline depths (Boersma and Shackleton, 1981). However, in the shallow water (<100 m) Brazos sections (where this species is dominant throughout the Late Cretaceous and into the early Tertiary (Keller, 1989a), it apparently adapted to living in a shallower environment. The same pattern has been observed in the shallow water Stevns Klint and Nye Klov sections (Schmitz et al., 1992; Keller et al., 1993). In this study H. globulosa has therefore been grouped with surface dwellers.
In Figure 4.15, the Cretaceous fauna has been grouped into surface, intermediate, and deep dwellers based on two faunal parameters, the percentage of species in each group and the percentage of individuals in each group. The latter is a more sensitive parameter of environmental change because it weighs species according to their relative abundance in the total fauna, whereas the former gives each species the same significance regardless of whether it is represented by 1% or 80% in the faunal assemblage. These data are illustrated in Figure 4.15 along with the stable isotope record for each of the four sections, which are arranged from shallow (Brazos) to deep (Site 528).

Figure 4.15
Cretaceous planktic foraminiferal species grouped into surface, intermediate, and deep water dwellers based on stable isotope ranking. Data are presented in terms of percentage of species and fauna (individuals) in each category and are arranged from (more...)
Both faunal parameters in the four sections show a strong trend toward decreasing deep and intermediate dwellers and increasing surface dwellers. About 15 to 20% of the species are deep dwellers at Site 528 (midbathyal) and Caravaca (upper bathyal), and a decrease to 8 and 12%, respectively, occurs in the latest Maastrichtian (Figure 4.15). At El Kef (outer neritic) an average of 10% of the species are deep dwellers, and at Brazos 0% (midneritic). The relative abundance of individuals in this group shows the same trend, but they comprise a significantly smaller part of the population except at Site 528. Abundance of deep dwelling individuals decreases from 15 to 20% at Site 528, to 5 to 10% at Caravaca and El Kef, and 0% at Brazos. All deep dwellers disappear at the K/T boundary.
Intermediate dwellers show a similar trend. At Site 528, 5 to 10% of the species are in this group; at Caravaca 15 to 20%; at El Kef 10 to 15%; and at Brazos between 10 and 20% decreasing up-section and disappearing well below the K/T boundary (Figure 4.15). The relative abundance of individuals in this group is smaller. At Site 528, maximum abundance is 10%, decreasing to 2% well below the K/T boundary; at Caravaca maximum 5% decreasing up-section to <2%; at El Kef <2%; and at Brazos <1%. Like the deep water dwellers all intermediate dwellers disappear at the K/T boundary.
Surface dwellers are the largest component of the late Maastrichtian fauna. Their abundance increases from 75 to 85% in the open marine Site 528, to 85 to 90% at Caravaca and El Kef, and 99 to 100% at Brazos. In all sections the dominant surface dwellers are cosmopolitan taxa and survive the K/T boundary event; no data are available for Site 528 because the earliest Tertiary is missing. The relative abundance of dominant survivor species rapidly declines in the boundary clay at Caravaca and El Kef, but at Brazos, Cretaceous survivors thrive well into Zone P1a. Rapid expansion of the evolving Tertiary fauna accompanies the decline of Cretaceous survivors.
Figure 4.15 thus illustrates environmental conditions in four different geographic localities and at four different water depths. Some differences between these sections are due to changing water depths and some are due to other global environmental changes. For instance, the variations in the faunal assemblages among the four sites are due primarily to changing water depth and its effect on the thermocline and vertical stratification of species. Global sea-level changes and their effect on water mass stratification are indicated by the decrease and disappearance of deep and intermediate groups at each locality, especially in the middle to upper bathyal and outer neritic environments of Site 528, Caravaca, and El Kef. These gradual faunal changes indicate a changing environment beginning 100,000 to 300,000 yr before the end of the Maastrichtian (Keller and Barrera, 1990). The terminal disappearance of all deep and intermediate dwellers near the K/T boundary, however, indicates accelerated environmental changes that favored the survival of cosmopolitan surface dwellers. The effect of these changes may have included global warming, disruption of the vertical water mass stratification and thermocline, and rapid reduction of marine primary productivity. Stable isotope data indicate that both warming and a drop in marine productivity beginning at the K/T boundary were restricted to low latitudes. However, through the latest Maastrichtian, stable isotope data indicate high marine productivity. The gradual faunal changes prior to the K/T disturbance imply Earthderived causes and may be related to the latest Maastrichtian sea-level regression followed by a transgression across the K/T boundary clay (Schmitz et al., 1992; Keller et al., 1993), but large-scale volcanism and mantle plume activity may also have been contributing factors. However, the considerably smaller mass extinction in planktic foraminifera than generally assumed suggests that the effects of a bolide impact on marine plankton would have been significantly less catastrophic than proposed by current theory. Moreover, recent studies of high latitude sections show that the effects of the K/T boundary event were negligible in high latitudes.
Discussion And Summary
We have examined the effect of global change on calcareous marine microplankton across the K/T boundary with respect to four critical topics: (1) the temporal completeness of boundary sequences; (2) the nature of species extinctions; (3) the effect on dominant species populations; and (4) the specific habitats affected. Our investigation has not uncovered any simple answers. The negative effects of global environmental changes across the K/T transition appear complex, prolonged, and unlikely to result from a single cause. We summarize the results below.
1. MacLeod and Keller (1991a,b) have demonstrated that nearly all K/T boundary sequences are temporally incomplete, but that the hiatus patterns may vary between deep-sea and neritic environments. In deep-sea sections a short hiatus or interval of nondeposition that includes the basal Tertiary Zone P0 and part of Zone P1a is virtually always present. In continental shelf sequences, sediment deposition continued at this time, albeit at a reduced rate, and short hiatuses are usually present at the P0/P1a Zone boundaries. This hiatus pattern seems to be linked to the sea-level regression maximum just below the K/T boundary, followed by a rapid transgression across the K/T boundary and into Zone P0 (Schmitz et al., 1992; Keller et al., 1993), which trapped terrigenous sediment and organic carbon on continental shelves and temporarily deprived deep ocean basins of an inorganic sediment source and enhanced carbonate dissolution (Loutit and Kennett, 1981; Haq et al., 1987; Keller, 1988, 1989a, 1993; Keller et al., 1993). The two short hiatuses coincide with sea-level lowstands. In view of this information, the sudden, near-complete mass extinction observed in deep-sea sections reflects artificial truncation of species ranges due to a hiatus as illustrated here for DSDP Site 528. Moreover, the abrupt changes in geochemical tracers (iridium, shocked quartz, soot, δ13C, CaCO3, total organic carbon) observed in many deep-sea sections also need to be reevaluated, and the possible effects of elemental concentrations during a time of no carbonate or terrigenous sediment deposition must be considered (see Donovan et al., 1988).
2. The record of species extinctions and evolution in the low latitude El Kef section, the temporally most complete K/T boundary sequence known to date, indicates that Cretaceous foraminiferal species extinctions occurred over an extended time period beginning well before and ending well after the K/T boundary. About one-third of the species disappeared before the boundary, nearly one-third disappeared at the boundary, and more than one-third survived and gradually disappeared during the early Tertiary. Moreover species extinctions appear systematic, affecting tropical complex, large, and highly ornamented morphologies first and favoring the survival of more cosmopolitan, smaller, and less ornamented morphologies. Furthermore, Cretaceous survivor species in Tertiary deposits are generally dwarfed. Evolution of Tertiary species begins immediately after the K/T boundary with the appearance of very small unornamented and primitive morphologies. Their increasing diversification and abundance seem to have contributed to the demise of the Cretaceous survivors. This species extinction pattern is representative of depositional sequences that are temporally complete across the K/T boundary in low latitudes (including Caravaca, Agost, Brazos) and illustrates the absence of a near-complete mass extinction as commonly reported from the deep-sea sections that contain a hiatus. Moreover, this gradual species extinction pattern cannot be reconciled with a single geologically instantaneous cause.
The species extinction pattern in high latitudes (Site 738C, Nye Klov) is quite different from that in low latitudes. In contrast to low latitudes, no significant species extinctions occur at the K/T boundary and nearly all species survive well into the Tertiary. Yet, as in low latitudes, extinctions occur over an extended time, beginning well below and extending well above the K/T boundary. From low to high latitudes, Cretaceous survivor taxa are primarily small cosmopolitan heterohelicids, hedbergellids, guembelitrids, and globigerinellids. These taxa were able to tolerate changing environmental conditions, whereas the more specialized tropical and subtropical taxa went extinct.
The emerging global pattern of species extinctions among planktic foraminifera suggests that the K/T boundary event may have been most severe in low latitudes but that its effect diminished into high latitudes. This interpretation is supported by the -3.0%o shift of δ13C in low latitudes and absence in high latitudes (Keller et al., 1993; Barrera and Keller, 1994).
3. Changes in the numerical abundances of species are an important factor in evaluating the magnitude of global change. For instance, the numerical abundance of all planktic foraminiferal species extinct at or before the K/T boundary is relatively small, less than 5% in midneritic environments (Brazos) and between 10 and 30% in outer neritic and bathyal depths (El Kef, Caravaca, and Site 528), and this group declined gradually during the late Maastrichtian. Cretaceous survivors dominate during the late Maastrichtian and decline rapidly in the Tertiary over about 10,000 to 40,000 yr in low latitudes when maximum negative conditions are reached accompanied by low primary marine productivity. Thus, only a small group of the planktic foraminiferal population became extinct at or before the K/T boundary. The major adverse effect of the global environmental change is seen in the rapid but gradual post-K/T faunal, floral, and δ13C changes in low latitudes.
4. Changes in the marine environment are inferred from δ18O and δ13 C depth stratification of Cretaceous planktic foraminiferal species. We demonstrate that faunal differences between neritic and bathyal depths are due primarily to changes in paleodepth and their effect on the thermocline and vertical stratification of species. Most surprising, however, is the total disappearance of deep and intermediate water dwellers (as well as some surface dwellers) at or before the K/T boundary in all sections. Only surface water dwellers survived the K/T boundary. This implies that the global environmental change preferentially eliminated deeper habitats first, favoring survival in surface waters, or that subsurface dwellers were less able to adapt to changing environmental conditions. Environmental changes, however, seem to have been gradual beginning 100,000 to 300,000 yr before the end of the Cretaceous; accelerating at the boundary; and reaching a negative maximum between about 10,000 and 40,000 yr after the boundary, coincident with maximum low primary marine surface productivity in low latitudes. Return to a more stable ecosystem and increased marine productivity does not occur until about 250,000 to 350,000 yr after the K/T boundary.
There is no single cause that can account for this prolonged environmental change. Hallam (1989) has consistently advocated sea-level changes as a major cause of faunal turnover. The latest Maastrichtian sea-level regression followed by a rapid transgression across the K/T boundary and into the early Tertiary (Brinkhuis and Zachariasse, 1988 Donovan et al., 1988, Schmitz et al., 1992; Keller et al., 1993), however, has largely been ignored as an important factor in the K/T faunal transition. The data presented here in terms of the differential nature of hiatus patterns in the deep-sea and neritic environment, the selective nature of pre-K/T species extinctions, and the elimination of all deep- and intermediate-dwelling planktic foraminifera at the K/T boundary all point toward sea-level change as a major contributor, if not causal factor. The late Maastrichtian sea-level regression reached a maximum just before the K/T boundary, followed by a rapid transgression into the earliest Tertiary (Figure 4.3). Elimination of deeper-dwelling species could have occurred as a result of the breakdown in the water mass structure, a change in thermocline, expansion of the oxygen minimum zone, and decreased productivity. However, many sea-level changes of equal or greater magnitude have occurred during the past 100 m.y., and none has resulted in a complete change of marine plankton over a few 100,000 yr. Thus, sea-level change is probably only one, although perhaps the major, contributing factor to the K/T faunal transition. Other environmental changes (volcanism, bolide impact) may have accelerated the demise of a Cretaceous fauna already on the decline.
Acknowledgments
We thank the reviewers H. Thierstein and W. Berger for their critical and helpful comments, and we gratefully acknowledge contributions from N. MacLeod, I. Canudo, S. D'Hondt, S. Gartner, and J. Pospichal. This research was supported by NSF Grants OCE 90-21338 and EAR 91-15044 to G.K.
References
- Alvarez, W., L. W. Alvarez, F. Asaro, and H. V. Michel (1980). Extraterrestrial cause for the Cretaceous-Tertiary extinction, Science 208 , 1095-1108. [PubMed: 17783054]
- Barrera, E., and B. T. Huber (1990). Evolution of Antarctic Water during the Maastrichtian: Foraminifer oxygen and carbon isotope ratios, Leg 113, in Proceedings of the Ocean Drilling Program, Scientific Results 113 , P. S. Barker, editor; , J. P. Kennett, editor. et al., eds., Ocean Drilling Program, College Station, Texas, pp.813-827.
- Barrera, E., and G. Keller (1990). Foraminiferal stable isotope evidence for gradual decrease of marine productivity and Cretaceous species survivorship in the earliest Danian, Paleoceanography , 5 , 867-890.
- Barrera, E., and G. Keller (1994). Productivity across the Cretaceous-Tertiary boundary in high latitudes, Geological Society of America Bulletin , in press.
- Beeson, D., S. Gartner, G. Keller, N. MacLeod, J. Médus, and R. Rocchia (in preparation). Multidisciplinary stratigraphy and depositional environment across the Cretaceous-Tertiary boundary at the Brazos River, Falls County, Texas, Palaios .
- Berger, W. H. (1970). Biogenous deep-sea sediments: Fractionation by deep-sea circulation, Geological Society of America Bulletin 81 , 1385-1402.
- Berger, W. H., and E. L. Winterer (1974). Plate stratigraphy and fluctuating carbonate line, International Association of Sedimentology Special Publication 1 , 11-48.
- Berggren, W. A. (1962). Some planktonic foraminifera from the Maastrichtian and type Danian stages of southern Scandinavia, Stockholm Contributions in Geology 9(1), 1-102.
- Boersma, A., and N. J. Shackleton (1981). Oxygen and carbon isotope variations and planktonic foraminiferal depth habitats: Late Cretaceous to Paleocene, central Pacific, DSDP Sites 463 and 465, Leg 65, in Initial Reports of the Deep-Sea Drilling Project 65 , U.S. Government Printing Office, Washington, D.C., pp.513-526.
- Brinkhuis, W., and W. J. Zachariasse (1988). Dinoflagellate cysts, sea-level changes and planktonic foraminifers across the Cretaceous-Tertiary boundary at El Haria, northwest Tunisia, Marine Micropaleontology 13 , 153-191.
- Canudo, I. J., G. Keller, and E. Molina (1991). K/T boundary extinction pattern and faunal turnover at Agost and Caravaca, SE Spain, Marine Micropaleontology 17 , 319-341.
- D'Hondt, S., and G. Keller (1991). Some patterns of planktic foraminiferal assemblage turnover at the Cretaceous-Tertiary boundary, Marine Micropaleontology 17 , 77-118.
- Donovan, A. D., G. R. Baum, G. L. Blechschmidt, T. S. Loutit, C. E. Pflum, and P. R. Vail (1988). Sequence stratigraphic setting of the Cretaceous-Tertiary boundary in central Alabama, Society of Economic Paleontologists and Mineralogists Special Publication No. 42 , 300-307.
- Douglas, R. G., and S. M. Savin (1978). Oxygen isotope evidence for depth stratification of Tertiary and Cretaceous planktic foraminifera, Marine Micropaleontology 3 , 175-196.
- Hallam, A. (1989). The case for sea-level change as a dominant causal factor in mass extinction of marine invertebrates, Philosophical Transactions of the Royal Society of London, Series B 325 , 437-455.
- Haq, B. U., J. Hardenbol, and P. R. Vail (1987). Chronology of fluctuating sea-levels since the Triassic, Science 235 , 1156-1166. [PubMed: 17818978]
- Herbert, T. D., and S. D'Hondt (1990). Environmental dynamics across the Cretaceous-Tertiary extinction horizon measured 21 thousand year climate cycles in sediments, Earth and Planetary Science Letters 99 , 263-275.
- Hofker, J. (1960). The foraminifera of the lower boundary of the Danish Danian, Meddr. Dansk Geol. Forening 14 , 212-242.
- Huber, B. T. (1991). Maastrichtian planktonic foraminifer biostratigraphy and the Cretaceous-Tertiary boundary at ODP Hole 738C (Kerquelen Plateau, Southern Indian Ocean), in Proceedings of the Ocean Drilling Program, Scientific Results 119 , Ocean Drilling Program, College Station, Texas, pp.451-466.
- Jiang, M. J., and S. Gartner (1986). Calcareous nannofossil succession across the Cretaceous-Tertiary boundary in east-central Texas, Micropaleontology 32 , 232-255.
- Kauffman, E. G. (1984). The fabric of Cretaceous marine extinctions, in Catastrophes and Earth History , W. A. Berggren, editor; and J. A. Van Couvering, editor. , eds., Princeton University Press, Princeton, N.J., pp.151-237.
- Keller, G. (1988). Extinction, survivorship and evolution of planktic foraminifers across the Cretaceous-Tertiary boundary at El Kef, Tunisia, Marine Micropaleontology 13 , 239-263.
- Keller, G. (1989. a). Extended Cretaceous/Tertiary boundary extinctions and delayed population change in planktonic foraminiferal faunas from Brazos River, Texas, Paleoceanography 4, 287-332.
- Keller, G. (1989. b). Extended period of extinctions across the Cretaceous/Tertiary boundary in planktonic foraminifera of continental shelf sections: Implications for impact and volcanism theories, Geological Society of America Bulletin 101 , 1408-1419.
- Keller, G., (1993). The Cretaceous Tertiary boundary transition in the Antarctic Ocean and its global implications, Marine Micropaleontology .
- Keller, G., and E. Barrera, E. (1990). The Cretaceous-Tertiary boundary impact hypothesis and the Paleontological record, Geological Society of America Special Paper 247 , 556-575.
- Keller, G., and C. Benjamini (1991). Paleoenvironment of the eastern Tethys in the Early Paleocene, Paleogeography, Paleoclimatology, Paleoecology 6 , 439-464.
- Keller, G., and M. Lindinger (1989). Stable isotope, TOC and CaCO3 record across the Cretaceous-Tertiary boundary at El Kef, Tunisia, Palaeogeography, Palaeoclimatology, Palaeoecology 67 , 243-265.
- Keller, G., C. Benjamini, M. Magaritz, and S. Moshkovitz (1990). Faunal, erosional and CaCO3 events in the early Tertiary eastern Tethys, Geological Society of America Special Paper 247 , 471-480.
- Keller, G., E. Barrera, B. Schmitz, and E. Mattson (1993). Gradual mass extinction, species survivorship and long-term environmental changes across the Cretaceous-Tertiary boundary in high latitudes, Geological Society of America Bulletin 105 , 979-997.
- Kuslys, M., and U. Krahenbuhl (1983). Noble metals in Cretaceous/Tertiary sediments from El Kef, Radiochimica Acta 34 , 139-141.
- Lindinger, M. (1988). The Cretaceous/Tertiary boundaries of El Kef and Caravaca: Sedimentological, geochemical and clay mineralogical aspects, Ph.D. thesis, Swiss Federal Institute of Technology, Zurich, 254 pp.
- Loper, D. E., and K. McCartney (1986). Mantle plumes and the periodicity of magnetic field reversals, Geophysical Research Letters 13 , 1525-1528.
- Loper, D. E., and K. McCartney (1988). Shocked quartz found at the K/T boundary: A possible endogenous mechanism, EOS 69, 971-972.
- Loutit, T. S., and J. P. Kennett (1981). Australasian Cenozoic sedimentary cycles, global sea-level changes and the deep-sea sedimentary record, Oceanologia Acta , 46-63.
- MacLeod, N. and G. Keller (1991. a). Hiatus distribution and mass extinctions at the Cretaceous/Tertiary boundary, Geology 19 , 497-501.
- MacLeod, N., and G. Keller (1991. b). How complete are K/T boundary sections? A chronostratigraphic estimate based on graphic correlation, Geological Society of America Bulletin 103 , 1439-1457.
- Manivit, H. (1984). Paleogene and upper Cretaceous calcareous nannofossils from Deep-Sea Drilling Project Leg 73, in Initial Reports of the Deep-Sea Drilling Project 74 , U.S. Government Printing Office, Washington, D.C., pp.475-500.
- Manivit, H., and H. Feinberg (1984). Correlation of magnetostratigraphy and nannofossil biostratigraphy in upper Cretaceous and lower Paleocene sediments of the Walvis Ridge Area, in Initial Reports of the Deep-Sea Drilling Project 74 , U.S. Government Printing Office, Washington, D.C., pp.469-474.
- McLean, D. M. (1985). Mantle degassing induced dead ocean, in The Cretaceous-Tertiary Transition , Geophysical Monograph 32, American Geophysical Union, Washington, D.C., pp.493-503.
- Officer, C. B., and C. Drake (1985). Terminal Cretaceous environmental events, Science 227 , 1161-1167. [PubMed: 17757851]
- Olsson, R. K. (1960). Foraminifera of latest Cretaceous and earliest Tertiary age in the New Jersey coastal plain, Journal of Paleontology 34(1) , 1-58.
- Olsson, R. K., and C. Liu (1993). Controversies on the placement of the Cretaceaus-Paleogene boundary and the K/T mass extinction of planktic foraminifera, Palaios 8 , 127-139.
- Perch-Nielsen, K. (1979. a). Calcareous nanofossils at the K/T boundary in Tunisia, in KT Boundary Events , W. A. Christensen, editor; and R. G. Bromley, editor. , eds., University of Copenhagen, Denmark, pp.238-243.
- Perch-Nielsen, K. (1979. b). Calcareous nannofossils from the Cretaceous between the North Sea and the Mediterranean, International Union of Geological Sciences, Ser. A 6 , 223-272.
- Perch-Nielsen, K. (1981). Nouvelles observations sur les nannofossiles calcaires á la limite Crétacé/Tertiaire prés de El Kef, Tunisie, Cah. Micropaléontol. 3 , 25-36.
- Perch-Nielsen, K. (1982).Maastrichtian coccoliths in the Danian survivors or reworked ''dead bodies," Abstract IAS .
- Perch-Nielsen, K. (1985). Cenozoic calcareous nannofossils. Mesozoic calcareous nannofossils, in Plankton Stratigraphy , H. M. Bolli, editor; , J. B. Saunders, editor; , and K. Perch-Nielsen, editor. , eds., Cambridge University Press, Cambridge, pp.329-554.
- Perch-Nielsen, K. (1986). Geologic events and the distribution of calcareous nannofossils—Some speculations, Bull. Centres Rech. Explor. Prod. Eif-Aquitaine 102 , 421-432.
- Perch-Nielsen, K., J. McKenzie, and Q. He (1982). Biostratigraphy and isotope stratigraphy and the catastrophic extinction of calcareous nannoplankton at the Cretaceous/Tertiary boundary, Geological Society of America Special Paper 190 , 353-371.
- Percival, S. F., Jr. (1984). Late Cretaceous to Pleistocene calcareous nannofossils from the South Atlantic, Deep-Sea Drilling Project Leg 73, in Initial Reports of the Deep-Sea Drilling Project 73 , U.S. Government Printing Office, Washington D.C., pp.391-424.
- Percival, S. F., and A. G. Fischer (1977). Changes in calcareous nannoplankton in the Cretaceous-Tertiary biotic crisis at Zumaya, Spain, Evolutionary Theory 2 , 1-35.
- Pospichal, J. J., and T. J. Bralower (1992). Calcareous nannofossils across the Creaceous/Tertiary boundary, Site 761, Northwest Australian margin, in Proceedings of the Ocean Drilling Program, Scientific Results 122 , Ocean Drilling Program, College Station, Texas, pp.735-751.
- Pospichal, J. J., and W. Wise, Jr. (1990). Calcareous nannofossils across the K/T boundary, ODP Hole 690C, Maud Rise, Weddell Sea, in Proceedings of the Ocean Drilling Program, Scientific Results 113 , Ocean Drilling Program, College Station, Texas, pp.515-532.
- Robin, E., D. Boclet, D. Benté, L. Froget, C. Jéhanno, and R. Rocchia (1991). The stratigraphic distribution of Ni-rich spinels in Cretaceous-Tertiary boundary rocks at El Kef (Tunisia), Caravaca (Spain) and Hole 761 (Leg 122), Earth and Planetary Science Letters 107 , 715-721.
- Romein, A. J. T. (1979). Lineages in early Paleogene calcareous nannoplankton, Utrecht Micropaleontological Bulletins 22, 1-231.
- Romein, A. J. T., and J. Smit (1981). The Cretaceous/Tertiary boundary: Calcareous nannofossils and stable isotopes, Koninkliijke Nederlandse Akademie van Wetenschapen Proceedings, Series B 84(3) , 295-314.
- Roth, P. (1987). Mesozoic calcareous nannofossil evolution: Relation to paleoceanographic events, Paleoceanography 2 , 601-611.
- Schmitz, B., G. Keller, and O. Stenvall (1992). Stable isotope and foraminiferal changes across the Cretaceous/Tertiary boundary at Stevns Klint, Denmark: Arguments for long-term oceanic instability before and after bolide impact, Palaeogeography, Palaeoclimatology, Palaeoecology 96 , 233-260.
- Shaw, A. B. (1964). Time in Stratigraphy , McGraw-Hill, New York, 365 pp.
- Sloan, R. E., J. K. Rigby, L. M. Van Valen, and D. Gabriel (1986). Gradual dinosaur extinction and simultaneous ungulate radiation in the Hell Creek Formation, Science 232 , 629-633. [PubMed: 17781415]
- Smit, J. (1982). Extinction and evolution of planktonic foraminifera after a major impact at the Cretaceous/Tertiary boundary, Geological Society of America Special Paper 190 , 329-352.
- Smit, J. (1990). Meteorite impact, extinctions and the Cretaceous-Tertiary boundary, Geologie en Mijnbouw 69 , 187-204.
- Smit, J., and A. J. T. Romein (1985). A sequence of events across the Cretaceous-Tertiary boundary, Earth and Planetary Science Letters 74 , 155-170.
- Stott, L. D., and J. P. Kennett (1989). New constraints on early Tertiary paleoproductivity from carbon isotopes in foraminifera, Nature 342 , 526-529.
- Thierstein, H. R. (1982). Terminal Cretaceous plankton extinctions: A critical assessment, Geological Society of America Special Paper 190 , pp.385-399.
- Thierstein, H. R., F. Asaro, W. U. Ehrman, B. Huber, H. V. Michel, H. Sakai, and B. Schmitz (1991). The Cretaceous/Tertiary boundary at Site 738, South Kerguelen Plateau, in Proceedings of the Ocean Drilling Program, Scientific Results 119 , Ocean Drilling Program, College Station, Texas, pp.849-868.
- Wei, W., and J. J. Pospichal (1991). Danian calcareous nannofossil succession at Site 738 in the Southern Indian Ocean, in Proceedings of the Ocean Drilling Program, Scientific Results 119 , Ocean Drilling Program, College Station, Texas, pp.495-512.
- Worsley, J. (1974). The Cretaceous-Tertiary boundary event in the ocean, in Studies in Paleo-oceanography , W. W. Hay, editor. , ed., Society of Economic Paleontologists and Mineralogists Special Publication 20, pp.94-125.
- Zachos, J. C., and M. A. Arthur (1986). Paleoceanography of the Cretaceous/Tertiary boundary event: Inferences from stable isotopic and other data, Paleoceanography 1 , 5-26.
- PubMedLinks to PubMed
- Cretaceous-Tertiary (K/T) Mass Extinction: Effect of Global Change on Calcareous...Cretaceous-Tertiary (K/T) Mass Extinction: Effect of Global Change on Calcareous Microplankton - Effects of Past Global Change on Life
- Homo sapiens nuclear prelamin A recognition factor (NARF), transcript variant 2,...Homo sapiens nuclear prelamin A recognition factor (NARF), transcript variant 2, mRNAgi|134284353|ref|NM_031968.2|Nucleotide
- nitrate- and nitrite sensing domain-containing protein [Streptomyces resistomyci...nitrate- and nitrite sensing domain-containing protein [Streptomyces resistomycificus]gi|921247467|ref|WP_053191548.1|Protein
- Homo sapiens POTE ankyrin domain family member G (POTEG), transcript variant 1, ...Homo sapiens POTE ankyrin domain family member G (POTEG), transcript variant 1, mRNAgi|1653961221|ref|NM_001005356.3|Nucleotide
Your browsing activity is empty.
Activity recording is turned off.
See more...