NCBI Bookshelf. A service of the National Library of Medicine, National Institutes of Health.
National Academies of Sciences, Engineering, and Medicine; Division on Engineering and Physical Sciences; Space Studies Board; Committee on Extraterrestrial Sample Analysis Facilities. Strategic Investments in Instrumentation and Facilities for Extraterrestrial Sample Curation and Analysis. Washington (DC): National Academies Press (US); 2018 Dec 20.
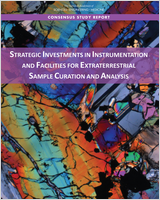
Strategic Investments in Instrumentation and Facilities for Extraterrestrial Sample Curation and Analysis.
Show detailsThis chapter focuses on the following questions posed by the charge to the committee:
- What laboratory analytical capabilities are required to support the NASA Planetary Science Division's (and partners') analysis and curation of existing and future extraterrestrial samples?
- Which of these capabilities currently exist, and where are they located (including international partner facilities)?
4.1. RETRIEVAL, CURATION, AND CHARACTERIZATION OF RETURNED EXTRATERRESTRIAL SAMPLES
To understand the significance of existing infrastructure for sample curation and analysis and the tasks associated with retrieving the samples from the spacecraft, initial characterization and curation are first summarized (see Figure 4.1).1

FIGURE 4.1
Returned sample processing flowchart. NOTE: CAPTEM, Curation and Analysis Planning Team for Extraterrestrial Materials.
- 1.
Recovery and initial triaging: Recovery from the field of sample return landing craft and delivery to an appropriate curatorial facility generally calls for procedures and equipment idiosyncratic to each mission and separate from permanent curation and analysis facilities. However, retrieving sample containers from that craft, initial handling and opening of those containers, and initial inspection of their contents will occur within facilities considered by this report. Key capabilities include environments and handling devices that carry minimal contamination, environments with controlled atmospheres and temperatures, and instruments for nondestructive inspection (e.g., optical microscopes).
- 2.
Sample description: Recovered samples must be described for their form (e.g., solid or gas), material properties (e.g., rock chips or powders), and size (volume and/or mass), and initial interpretations made regarding their general categorization (e.g., petrologic classification). These activities also commonly take place in a curatorial facility and call for various common, nondestructive or minimally destructive (e.g., thin sections of hard samples) observational or measuring tools (e.g., microscopes, spectroscopes, balances).
- 3.
General nondestructive analyses: As sample investigation transitions from initial description and categorization to scientific inquiry, the first detailed observations are generally made with a set of nondestructive (or minimally destructive) methods that are sufficiently general that they are likely to be useful for virtually any material or motivating science question. Examples include optical microscopies and spectroscopies, and scanning electron microscopy (SEM). Laboratories for such measurements are commonly available at the same institutions that fill a curatorial role, but analyses also may be made in other laboratories, as an initial step to more specialized measurements.
- 4.
Subsampling and preparation for specialized analysis: While initial triaging of returned samples calls for some level of sample subdivision to facilitate organization and simple descriptions, preparation for specialized, hypothesis-driven measurements often requires more significant modification, and destruction of samples is often required. This might include sorting by physical properties, crushing, grinding, cutting or polishing, exposure to solvents or other reagents, or manipulation by specialized devices such as microtomes or focused ion beam (FIB) mills. This is another activity that is frequently performed at curatorial facilities, but also often performed in analytical laboratories as part of their sample preparation procedures. These procedures have a high potential for sample contamination or destruction and therefore can be carried out only by highly experienced and skilled staff who strictly follow established protocols.
- 5.
Specialized nondestructive or minimally destructive analyses: Detailed studies aimed at addressing mission science questions may call on techniques that are highly specialized but generally nondestructive or minimally destructive to the sample, once the sample is prepared. These techniques include spectroscopic techniques to assess compositional, structural, and physical properties of materials using a variety of probes, such as light sources (from infrared to X-ray), and electron, neutron, and ion beams. Most facilities for these specialized studies exist outside curatorial institutions.
- 6.
Specialized destructive analyses: Some fraction of returned samples may be sacrificed for destructive analysis, including most methods of mass spectrometric study for molecular identification or isotope ratio determination. Most facilities for these specialized studies exist outside curatorial institutions. These types of analyses are performed to answer questions related to the age and the origin of extraterrestrial sample materials. Cutting-edge technologies that are currently in use and that will remain necessary in the future include those that are capable of measuring the isotopic composition of various elements (e.g., mass spectrometers). Diverse radiogenic isotope systems can be used for geochronology and source tracing purposes for both terrestrial and extraterrestrial materials (e.g., long-lived Re-Os, Rb-Sr, Sm-Nd, Lu-Hf, Ar-Ar, K-Ar, U-Th-Pb). Because of their short half-life, short-lived radiogenic isotope systems are powerful tools to study early solar system processes and chronology (e.g., Al-Mg, Fe-Ni, Mn-Cr, Pd-Ag, Hf-W, I-Xe, Pu-Xe, Sm-Nd), and some of these systems are exclusively used in extraterrestrial studies. Recently, an increasing number of capabilities are also being developed for the study of nontraditional stable isotope systems (e.g., Si, Zn, Cr, Mg, K, Mo, Nd) in order to detect nucleosynthetic anomalies. This diversity of isotope systems and accompanying analytical capabilities are required to study the range of extraterrestrial materials (metal, silicate, liquid, gas). The challenge for the future is to develop capabilities to adapt to all sample sizes (rocks to dust particles) and materials (e.g., ices, gases).
- 7.
Archiving: Data products generated during all stages of sample study and any leftover sample material that was not consumed during subsampling and destructive analysis are archived. In general, long-term archiving of sample materials occurs at a curatorial facility, whereas archiving of observational data is tasked to the laboratories in which those observations were made.
4.2. FACILITIES FOR CURATION, TRIAGING, AND DESCRIPTION OF RETURNED SAMPLES
Curation of returned extraterrestrial samples occurs at several facilities around the world, as described in Chapter 2. Russian Luna samples are mainly curated at the Vernadsky Institute of Geochemistry and Analytical Chemistry, Moscow. The main portion of the Hayabusa sample return is maintained at the Extraterrestrial Sample Curation Center (ESCuC) at the Institute of Space and Astronautical Science, Sagamihara City, Japan. A small fraction (10-15 percent) of returned samples within the United States are stored at the White Sands Test Facility in New Mexico. The vast majority of NASA mission returned samples (Apollo, Genesis, and Stardust), as well as aliquots of Hayabusa and Luna samples, are curated by NASA at the Johnson Space Center (JSC) facility, which is described below.
With nearly 50 years of experience since the return of the Apollo samples, JSC has been a world leader in curatorial management and has developed a range of techniques, materials, and expertise to handle returned samples. The samples curated at JSC are diverse, including lunar samples returned by six crewed Apollo missions, solar wind collectors from the Genesis mission, samples from the coma of comet Wild 2 collected in aerogel by the Stardust mission, a subset of samples collected from the surface of asteroid Itokawa by the Hayabusa mission, cosmic dust collected in the stratosphere, and meteorites collected in Antarctica (which are co-curated with the Smithsonian Institution). Each of these sample types are curated in different facilities that have unique requirements for sample handling and contamination control.
The largest and oldest curatorial facility at JSC is the approximately 300-square-meter Apollo sample suite, which is subdivided into four laboratories. One laboratory securely stores pristine Apollo samples that have not previously been allocated. These are housed in 22 stainless steel nitrogen-purged storage cabinets under International Standards Organization (ISO) Class 6 clean room conditions (Figure 4.2).2 A second ISO Class 6 clean laboratory, the largest of the suite at 186 square meters, is used to process previously unallocated samples and includes a specialized band saw and core processing cabinets. A Return Sample Vault (RSV) securely stores previously allocated and returned Apollo samples in either aluminum or stainless steel under ISO Class 7 conditions. Portions of other collections (Cosmic Dust, Genesis, OSIRIS-REx [Origins, Spectral Interpretation, Resource Identification, Security, Regolith Explorer] contamination knowledge, Mars 2020 contamination knowledge) are stored in the RSV on a semi-permanent basis. If processing is required on samples from these collections, they are returned to their main laboratory for work. Several ISO Class 7 clean rooms are used for secure processing of previously allocated and returned Apollo samples. These are processed either in nitrogen-purged stainless-steel cabinets or within two laminar flow hoods.

FIGURE 4.2
A lunar sample processor prepares to begin work on pristine lunar samples by placing her hands in the gloves attached to a nitrogen-filled glove box. SOURCE: NASA, “Lunar Sample Laboratory Tour,” https://curator.jsc.nasa.gov/lunar/laboratory_tour.cfm, (more...)
The Genesis sample suite consists of three ISO Class 4 clean laboratories (~93 m2 in overall area) that were used to assemble the Genesis Sample return capsule, load the collector arrays, and clean and store the array and concentrator collectors after their return. First is a laboratory where the Genesis collectors are stored in six permanent nitrogen-purged desiccators. This is where the samples are characterized using a spectroscopic ellipsometer, a compound scanning microscope, and a micro-Fourier-transform infrared spectroscope (FTIR) situated on vibration isolation tables. Three digital cameras are used for imaging on each microscope. A second laboratory houses an ultra-pure water cleaning wet bench that is used to clean Genesis samples prior to allocation and study. Sample containers and processing tools are also cleaned here. A liquid particle counter and a total organic carbon unit are used to verify water quality prior to cleaning procedures. The third and final laboratory of the Genesis suite is an anteroom where a clean flow bench, a compound microscope, and a high-efficiency particulate air (HEPA) cabinet are used for return sample processing and storage. An ultraviolet ozone cleaner is also used here for thin-film removal from collector surfaces.
A 65-square-meter ISO Class 5 clean room is used to store and process the Stardust cometary and Stardust interstellar trays (Figure 4.3). It consists of an anteroom with a clean flow bench and two micropipette pullers, which are used to create pulled quartz needles that are used to subdivide the aerogel cells for allocation. The sample storage and processing room is used to store the aerogel samples in four custom nitrogen-purged desiccators. The samples are imaged and processed using specialized microscope systems situated on three vibration isolation tables. Three scanning compound microscopes are used to subdivide and document cometary and interstellar track morphology using MATLAB scripts. Four digital cameras are attached to the microscopes for high-resolution image capture.

FIGURE 4.3
Scientists examine the Stardust aerogel collector upon its arrival in the curatorial facility at NASA Johnson Space Center. SOURCE: NASA Johnson Space Center, “Stardust: Dust from Comets and Interstellar Space,” https://curator.jsc.nasa.gov/stardust/index.cfm, (more...)
The aliquot of Hayabusa sample returned from the Itokawa asteroid by the Japan Aerospace Exploration Agency (JAXA) and provided to NASA is housed in a small, approximately 18-square-meter ISO Class 5 clean laboratory that contains a nitrogen-purged glove box for storage and handling of the samples. A compound scanning microscope with a digital camera is situated on a vibration isolation table with micromanipulators for sample handling.
Other suites within the curatorial facility house Antarctic meteorites, cosmic dust, and the microparticle impact collection (satellites that have recorded microparticle impacts in space). Additional information about curation of lunar, Genesis, and Stardust returned samples is provided in the relevant sections of Chapter 2.
While the present suite of samples curated at JSC is diverse, the samples share the common feature of being primarily rocky samples composed of materials that are largely stable at ambient pressure and temperature conditions (i.e., hard condensed matter, as defined in Box 1.1). As such, capabilities developed during Apollo (e.g., dry nitrogen storage, Class 6-7 clean rooms, etc.) have largely preserved the pristine condition of these samples. More recent sample return missions, such as the cometary dust from Wild 2 captured in aerogel by the Stardust mission, have required corresponding development of new techniques. This development has been facilitated by close partnership between JSC Astromaterials Curation and the mission team. As currently formulated, curation of samples returned by Discovery and New Frontiers class missions will be conducted at JSC, with major development and initial operations costs paid by the respective mission, while long-term curation is supported by NASA through funding of JSC Astromaterials Curation. Planning for potential future sample return missions, such as from Mars, is discussed in Chapter 5 (see Section 5.3).
4.3. SAMPLE DISTRIBUTION
Once returned samples are documented and characterized by the mission team and safely housed in the curatorial facility, aliquots may be sent to laboratories around the world for cutting-edge analyses. As all returned samples are considered national and future heritage resources, strict protocols are in place for requesting, transporting, and securing these samples. All requests for samples curated at JSC are vetted first by the relevant curator, and then by the Curation and Analysis Planning Team for Extra Terrestrial Materials (CAPTEM).3
Apollo sample requests are made to the Apollo Sample Curator at JSC, who reviews them for content, and those deemed to be suitably mature are sent to CAPTEM for further evaluation.4 Investigators must provide evidence that their science, including analytical protocols, have passed peer review. This generally requires funding of a science proposal (from NASA, or other foreign or domestic government or nonprofit funding agency) within the past 3 years to work on the requested samples or submittal of a science proposal to work on the samples, backed up by evidence of peer-reviewed publications that report results using the same methods to be used on the requested samples and thorough documentation of the analytical methodology. CAPTEM then reviews the appropriateness of the sample request and makes a recommendation to the Apollo Sample Curator. Investigators must furthermore adhere to strict protocols for transporting, storing, and documenting sample handling and weight.
Similarly, Genesis mission sample requests are made to the Genesis Solar Wind Sample Curator at JSC, who reviews them for content, and those deemed to be suitably mature are sent to the Genesis Allocation Subcommittee of CAPTEM for further evaluation.5 The proposals must define the science objectives and document the sensitivity, precision, and accuracy of the analytical methods to be employed in the investigation. The proposals must also document that the method exceeds set precision and accuracy goals, or justify why the science can be accomplished without meeting these goals, as well as providing a plan for surface cleaning of the samples appropriate for the particular analyses being proposed. Also, because the Genesis samples are small and easily contaminated, the investigator is encouraged to design a shipping container that will safeguard the integrity of the sample or use a shipping container supplied by the curatorial staff.
Stardust mission sample requests are sent to the Stardust Sample Curator, who evaluates them and then forwards viable proposals to the Stardust Sample Allocation Subcommittee (SSAS) of CAPTEM. This committee evaluates “the scientific content of the proposal, capability of the proposers, availability of requested samples, and the realism of the investigation. SSAS will also weigh the overall merit of the proposal with the required amount of sample and any possible collateral damage to the remainder of the collection.”6 Because of the extremely limited sample size, investigators are encouraged to work in consortia to maximize the science yield from each particle—in particular, coordinating analyses that entail sample destruction.
The committee has not evaluated CAPTEM's processes in detail, but notes that CAPTEM has been an effective means by which to allocate existing Apollo, Stardust, Genesis, and meteorite samples.
Finding: Allocation of returned samples to laboratories around the world requires careful vetting of requests for samples and special handling during shipment and storage at an analytical facility.
4.4. ANALYTICAL EQUIPMENT
4.4.1. Classifications and Overview of Analytical Instrumentation
The scientific goals of sample return missions are broadly defined. For example, one of the OSIRIS-REx mission's goals is to “return and analyze a pristine carbon rich asteroid sample.”7 These analyses are accomplished using a large range of instruments distributed across dozens of institutions. Additionally, the types of materials being analyzed are changing, from the lunar samples composed primarily of silicates, oxides, glasses, and metals, to the more recent and upcoming samples that contain significant amounts of organic materials. Accordingly, the committee has focused on techniques that have been used to analyze both rocky and organic materials, as well as some methods likely to become more relevant in the near future. To facilitate discussion of this complex topic, the discussion is organized by defining how each technology relates to the following four traits or qualities:
- 1.
Types and purposes of methods: Extraterrestrial sample analysis involves diverse technologies and modes of material description and quantification. Tables 4.1 and 4.2 provide a guide to these methods, which are subdivided into several broad categories (microscopy, tomography, etc.), each of which is further divided into subcategories described by brief narrative explanations of their core technologies or procedures and their purposes.
- 2.
Availability and access: A very large number of laboratories have contributed to sample return science or could be used as analytical resources for ongoing or near-future sample return missions. The committee has not attempted to create an exhaustive list of all such facilities (and the committee believes this could not be done without omissions). Instead, each technology is categorized according to a five-tier scheme describing its availability and access. These categories range from commonly available and accessible (category 1), to unique technologies that are available at only one institution and accessible only through a gatekeeping procedure controlled by that institution (category 5). Thus, availability and access generally become more restricted as the numbering level in this scheme increases. (See Table 4.1 for details.)
The instruments and methods described as common (category 1) are in widespread use in tier-1 research universities and other relevant research institutions, and typically have few restrictions on their access and use. They can be assumed to be available for current sample return science (and likely will remain so in the future, provided the instruments are replaced once they reach their operational lifetime). Multiple regional facilities (categories 2 and 3) include instruments that provide “flagship” analytical or experimental capabilities for leading research laboratories; they exist in multiple U.S. and international institutions and are widely recognized in the sample return science community, yet their expense and sophistication is such that only relatively well funded laboratories with highly trained staff can obtain and operate them. For this reason, they may exist as regional centers, used by both members of their home institutions and visitors from other institutions. The categories of unique instruments (categories 4 and 5) include experimental or prototype instruments, generally designed to meet specific analytical or experimental goals that cannot be reached by other technologies, and that exist in only one location and generally require highly specialized skills to use.
Both regional facilities and unique instrumentation can present difficulties with respect to access, depending on the policies of the stakeholders who control them; for this reason, they are subdivided into user facilities and nonuser facilities. User facilities (categories 3 and 5), are open to external access, usually subject to a peer-reviewed proposal review process. Limited access facilities (categories 2 and 4) are generally used only by their directing scientific staff, and occasionally through collaboration with outside users. The distinction here is that user facilities have routine procedures in place to permit access to any outside user, whereas limited access facilities treat outside use on a case-by-case basis.
- 3.
Relevance to sample return missions and materials: Investments in analytical infrastructure for sample return missions are guided by the science goals of missions, as defined by a traceability matrix or equivalent statement of concrete goals. However, the long timescales of mission return science and the complexity and unpredictability of returned materials make it difficult to foresee which technologies might be useful for a given set of mission objectives, or what questions future generations might ask about returned samples. Moreover, much of the analytical infrastructure available to the community of scientists concerned with analysis of returned samples is either heritage technology developed for some previous mission having different goals or was created to meet some different need in the natural or applied sciences. For these reasons, many forms of instrumentation used by the institutions engaged in sample return science have potential value. Nevertheless, limitations to the resources available through the Planetary Science grant programs—Laboratory Analysis of Returned Samples (LARS) and Planetary Major Equipment and Facilities (PMEF)—mean one must discriminate between investments that advance the goals of sample return science and those that have no recognized use for that purpose. For this reason, Table 4.2 also includes a brief description of the ways in which each listed analytical technology has a recognized relevance to the scientific goals of sample return missions.
One challenge faced in making instrument investment decisions in the coming years is the changing nature of science goals driving sample return missions. Prior to the Genesis mission, extraterrestrial sample analysis science focused exclusively on lunar samples returned as part of the Apollo or Luna programs, cosmic dust, or meteoritic materials. A large fraction of this work focused on characterizing the mineralogy and elemental and isotopic compositions of silicates, oxides, glasses, and metals, typically on scales of micrometers or larger. More recent sample return missions, including Stardust, Hayabusa, OSIRIS-REx and Hayabusa2, call for study of the structures and molecular chemistries of organic materials, structurally amorphous organic solids with highly complex molecular structures, and small (submicrometer) objects or domains. This means that technologies that used to be considered highly mission relevant may now have lesser relevance to ongoing or near-future missions. The committee's evaluation of this issue considers continued science on materials returned by the Apollo program, but more strongly emphasizes science goals of the Genesis, Stardust, and Hayabusa missions, the ongoing OSIRIS-REx and Hayabusa2 missions, and planned near-future sample return missions (including possible Mars sample return and cometary sample return).
- 4.
Stakeholders and hosting institutions: Sections 4.2 through 4.4—the main body of this chapter—consist of detailed descriptions of the curatorial and analytical facilities relevant to study of returned extraterrestrial materials in the United States and international partners in sample return missions. This material is organized according to the stakeholders in each institution. A stakeholder in a facility or instrument is the party principally responsible for investing the capital costs and paying for related infrastructure, staffing and continuing costs of operation. Many instruments are initially purchased with multiple stakeholders (e.g., through cost sharing arrangements), so the definition used here considers the full costs of operating an instrument over its useful lifetime.
Stakeholders generally have a high level of access and control over an instrument's uses, condition, staffing, and associated sample holding and preparation facilities. Non-stakeholders may make use of an instrument, and they benefit from lack of responsibility for continuing costs, but in exchange they often must adapt their samples and analytical goals to conform to the laboratory's practices. In the case of the precious and sensitive (possibly hazardous) materials considered by sample return missions, an entity that controls the samples, but is not an analytical facility, may be forced to make difficult judgments as to whether a given instrument out of its control is maintained and operated in a way that meets scientific and safety standards (which may differ from mission to mission, and over time—see Section 4.3 for a description about how sample allocation is determined for present return samples). These issues are particularly important when considering facilities and instruments that are not common or user facilities, yet have high mission relevance (by the definitions used in Table 4.1). In such cases, it is particularly important that a stakeholder in that capability has direct connections with the science teams and funding agencies of sample return missions. Thus, investment decisions will need to balance availability and mission relevance of technologies with some understanding of which institutions need to be stakeholders in those technologies in order for them to be used appropriately for sample return mission science.
Specifically, the committee classifies a given facility or instrument as having NASA, or any other U.S. institution (such as a university or non-NASA national laboratory), or any international institution as the primary stakeholder. Some judgment was required in some such instances—for example, the committee regards the MegaSIMS instrument as having NASA as the stakeholder, because it was funded and operated largely using mission-specific NASA funding, but it is housed at a U.S. university (University of California, Los Angeles [UCLA]) and is therefore listed under U.S. laboratories external to NASA. Finally, there are two types of analytical technologies that raise special issues with regard to investment strategies for sample return missions and associated science: (1) Cutting-edge technologies are instruments and methods that break new ground in fundamental abilities to observe physical and chemical properties of natural materials; recent examples include MegaSIMS (developed for the Genesis mission) and the Chicago Instrument for Laser Ionization (CHILI; built to study samples from Stardust and similarly small, complex, extraterrestrial materials). Such projects present unique risks (as their outcomes cannot be confidently foreseen), but also unique opportunities, where investment can lead to dramatic advances, creating new ways of observing, describing, and analyzing materials and environments. Highly innovative or inventive technologies may have increased “return on investment” because of the new opportunities for scientific advancement that they create, and therefore are an important part of any balanced portfolio of investments in instrumentation. (2) Nontraditional technologies are instruments and methods that may be common in scientific disciplines that have not had significant overlap with the community of researchers performing sample return science, but they have potential to impact the study of samples returned by ongoing or near-future missions. Examples include the atom probe, various forms of advanced molecular mass spectrometry (Fourier-transform mass spectrometry, tandem mass spectrometry), and high-sensitivity molecular surface analysis (soft sputtering ion sources).
TABLE 4.1
Classification of Facilities by Availability and Access (1-5) and by Broad Types of Analysis (A-C).
TABLE 4.2
Examples of Specific Instruments, Methods, Facilities or Facility Types Used in Extraterrestrial Sample Analyses.
A few examples of how to interpret Table 4.2, referencing Table 4.1: Scanning electron microscopy is classified as 1B, 1C in availability and access—that is, used for physical analysis and commonly available and accessible. SEM is classified as Mission Relevance (MR) I and MR II in mission relevance—that is, relevant to the fundamental analysis of all returned samples, as well as some SEM techniques having more specialized applications. On the other hand, synchrotron-based X-ray Raman spectroscopy is classified as 5B and 5C in availability and access—that is, used for structural and chemical analysis and available at unique facilities with user access. It is classified as MR IV in mission relevance—that is, not commonly used for analysis of returned samples but could provide unique data and become more relevant to future missions. Helium ion microscopy (4C, MR II) is used for high-spatial-resolution imaging and compositional analysis and is available at unique facilities with only limited access. It is a specialized tool currently relevant for rock and metal samples, but it is rapidly developing for organic and hybrid structures (i.e., materials that are a mixture of hard and soft condensed matter).
The following sections discuss laboratory instrumentation available at the institutions involved in sample return science, organized according to the dominant stakeholder.
4.4.2. NASA Center Analytical Laboratories and Facilities
4.4.2.1. Johnson Space Center
JSC has over 200 active research and operational scientists, analysts, and technicians who support the missions of NASA, and of these, approximately 75 are involved in analyses of extraterrestrial materials. JSC is involved in developing planetary science mission concepts and providing Earth imagery to the Earth science community. There is a wide range of equipment in the Astromaterials Research and Exploration Science section that is used for the analysis and classification of terrestrial, planetary, and solar materials and space-exposed hardware. See Appendix B for a listing of the analytical equipment available at JSC.
4.4.2.2. NASA Goddard Space Flight Center
The Astrobiology Analytical Laboratory at NASA Goddard Space Flight Center occupies 167 square meters of laboratory space. Staffed with nine principal scientists and technical staff, it provides state-of-the-art analytical capabilities for studies of organic molecules in terrestrial analogs and extraterrestrial materials. The analytical instrumentation primarily focuses on chromatographic systems for molecular separation that employ either gas chromatographs or liquid chromatographs. These include multiple instruments. Molecular detection is accomplished via optical detectors (e.g., linear diode array detectors and fluorescence detectors) and mass spectrometry (e.g., quadrupole mass analyzers, dual quad mass analyzers, ion cyclotron resonance mass spectrometry, time-of-flight mass analyzers, and isotope ratio mass spectrometric analyzers. In addition to this major analytical instrumentation, the Astrobiology Analytical Laboratory is equipped with a wide range of sample preparation facilities, including HEPA-filtered benches, furnaces for off-line pyrolysis, ball mill for sample pulverization, balances, fume hoods, and so on.
4.4.2.3. Jet Propulsion Laboratory
The Jet Propulsion Laboratory (JPL) maintains three research groups concerned with cosmochemistry and astrobiology, two of which focus primarily on chemical analysis relevant to sample return science (the third is a group focused on geobiology and astrobiology but with no history of study of returned extraterrestrial materials; therefore, it is not included in this review). The Planetary Chemistry and Astrobiology Division at JPL includes a laboratory for the analysis of trace metals and their isotopes in extraterrestrial samples, including ultra-clean laboratories for sample handling, digestion, and chemical separation and analytical instrumentation for characterizing metal abundances (using ICP-MS) and isotope ratios (using TIMS). The primary research focus of this group has been early solar system chronology, based on the study of meteorites and samples returned by the Apollo program. This group is being restructured at present, in response to the impending retirement of its long-time director. The analytical capabilities will be merged with the laboratories for geochemistry and cosmochemistry at Caltech. A second laboratory at JPL uses ToF-SIMS techniques to study trace contamination of surfaces of space flight instruments and platforms, with the aim of characterizing and improving planetary protection threats and contamination hazards to sample return missions.
4.4.3. Keck/NASA Reflectance Experiment Laboratory
The Keck/NASA Reflectance Experiment Laboratory (RELAB) is housed at Brown University but supported by NASA as a multiuser spectroscopy facility. Laboratory time is available at no charge to investigators who are in funded NASA research programs. Users can visit the laboratory or send samples to be analyzed. RELAB maintains the spectral database, a reference for spectral reflectance data returned by planetary missions. The facility has two operational spectrometers available to NASA-funded scientists:
- A near-ultraviolet, visible, and near-infrared bidirectional spectrometer; and
- A near- and mid-infrared FTIR spectrometer.
These spectrometers are being used to expand the spectral database, which is a freely accessible online archive.8 The database is becoming the principal reference for remotely sensed spectral reflectance data for planetary science. The RELAB policy is that all data are publicly archived within 3 years of acquisition.
The RELAB has two technical staff positions that aid in the maintenance of the laboratory, the spectrometers, and the spectral database. The technicians also assist users who visit the facility and analyze samples sent for analysis. Technical support is directly funded by NASA, as is instrument upkeep and modernization. User fee models are considered unsustainable and will add volatility to retaining technical staff, as corporate memory and experience is highly valued.
Conclusion: RELAB has been a community resource in producing and compiling spectral databases of rocks, asteroids, and planets. In light of flat or decreased budgets, this type of multiuser facility may be an appropriate future model for other common types of instrumentation used for extraterrestrial analyses.
4.4.4. U.S. Laboratories External to NASA or Other Government-Supported Facilities
The committee requested information from a number of U.S.-based laboratories currently undertaking analyses of extraterrestrial materials (see Appendix B for data compilation) to ascertain the array of analytical capabilities and staffing. These are mainly university-based laboratories, but also include museums (Smithsonian, American Museum of Natural History) and private institutions (Carnegie Institution of Washington). This synopsis does not include large, multiuser facilities such as synchrotrons, or laboratories supported by government agencies other than NASA, which are described separately in following sections. However, the committee recognizes that these demarcations of how facilities are supported are not necessarily clearly defined. Many user facilities benefit from leveraged support among different agencies, including NASA. For example, the upgrade of the X-ray beamline at the Geo Soil Enviro Center for Advanced Radiation Sources (GSECARS) user facility at the Advanced Photon Source (APS) was cost-shared by NASA, the National Science Foundation (NSF), and the Department of Energy (DOE).
A wide variety of analytical equipment is currently in use in non-NASA U.S. laboratories to characterize and study extraterrestrial samples. This instrumentation covers every major category of technology and analytical target that can be addressed by existing technologies, including common, commercially available instruments—i.e., nonprototype versions of microscopes (e.g., SEM, TEM, EPMA), spectroscopies (e.g., FTIR/Raman), commercially available common and more specialized mass spectrometry instrumentation (e.g., (MC)-ICP-MS, TIMS, SIMS, isotope ratio mass spectrometry, and RIMS), as well as specialized sample preparation equipment (e.g., FIB).9 It also includes two unique instruments designed for high-resolution sampling and high-precision in situ isotopic measurements: the CHILI instrument at the University of Chicago, and the MegaSIMS at UCLA. Collectively, these data suggest that, at present, U.S. laboratories are generally well instrumented to carry out extraterrestrial sample analyses.
The data provided by NASA detailing funding of analytical instrumentation through the LARS and PMEF programs over the past 10 years shows that NASA funding generally falls well below the cost of instrument purchases from commercial vendors (see Section 5.2.1).10 Thus, it can be inferred that much of the instrumentation currently used for extraterrestrial sample analysis is funded entirely by or via cost-share arrangement with other funding agencies (e.g., NSF), foundations (e.g., Keck), institutions, or other sources, and is likely also used for analyses of terrestrial samples.
Appendix B shows that staffing of analytical laboratories varies greatly from one institution to another, and from one type of institution to another. Most laboratories undertaking extraterrestrial sample analyses employ one or more highly trained technical staff, as well as postdoctorates and graduate students. Generally, institutional-based funding for technical staff is more readily available at NASA centers, museums, or private research institutions compared to university-based laboratories. This reflects the fact that universities have significantly reduced funding for technical support staff over the past three decades, which has implications for the sustainability of such laboratories, as addressed in Chapter 5.
Lastly, most sample return analyses to date have focused on rocks, minerals, glasses, and metals (hard condensed matter). Future missions, as detailed by the decadal survey, may seek to return gases, ices, and associated organic materials. Whereas the handling and analysis of extraterrestrial organic molecules, both low and high molecular weight (e.g., amino acids to polymeric organic matter) in primitive meteorites and comet samples (comet 81P/Wild 2 via the Stardust Mission) is now very mature in many laboratories (e.g., NASA, universities, and private institutions), the handling and transfer of more fragile samples such as ices and gases presents a new set of challenges for both NASA and sample recipients.
4.4.5. Other U.S. Government-Funded Facilities
The United States is the world leader in materials characterization, not only with a breadth of state-of-the-art characterization techniques but also with a broad portfolio of laboratory governance modalities (single-principal investigator [PI] laboratories, regional multi-PI laboratories, and larger multiuser facilities) and funding modes (governmental, nongovernmental/nonprofit, and commercial entities, as sole-funding entities or part of a consortium). As a result, a healthy ecosystem of non-NASA facilities across the nation can provide standard, routine, or specialized materials handling, characterization, measurements, and related capabilities for extraterrestrial materials and their analysis.
The materials characterization enterprise of the United States consists of a diverse portfolio of laboratories at all scales (from tabletop experiment to multiexperiment particle accelerators) with a variety of scopes (serving specific scientific and or technical niches, or covering a range of science or techniques), with a diversity of paths to access (from access based on a personal relationship with the PI to merit-reviewed, proposal-based open access), and funded by a variety of organizations or a combination of organizations (including government, nongovernmental nonprofit, and commercial organizations).
While an exhaustive summary and review of all U.S. capabilities in materials characterization is beyond the scope of this report, in this section a synopsis is provided of the (mostly governmental) organizations that provide a large part of the funding for materials research centers, with particular focus on examples of multiuser facilities that are most pertinent to present and likely future extraterrestrial sample return.
4.4.5.1. U.S. Department of Energy Major User Facilities
DOE is known for historical stewardship of diverse user facilities for high-energy and radiation-based tools, techniques, and measurements. These facilities and associated infrastructure have been developed and nurtured by specific DOE divisions, programs, and initiatives, but they are typically broadly accessible to users (including international users). The access to these DOE facilities often requires a relatively straightforward proposal process, and the facilities provide technical support (staff, data gathering/analysis, etc.) before, during, and after the experiments, together with computational analysis.
Specifically, DOE has significantly invested in and manages synchrotron X-ray scattering, neutron scattering, and electron-beam-based facilities. DOE also operates nanoscale science research centers, which provide user access to synthesis and fabrication of nanoscale structures and systems that will likely be relevant to the sampling/concentrators, characterization, and measurements of extraterrestrial materials analysis.
Some noteworthy and globally unique user facility examples include the synchrotron radiation source and associated capabilities at the APS at Argonne National Laboratory; the National Synchrotron Light Source-II (NSLS-II) at Brookhaven National Laboratory; the Advanced Light Source at Lawrence Berkeley National Laboratory (LBNL); the Spallation Neutron Source at Oak Ridge National Laboratory (ORNL); among several others partly supported or managed by DOE.
The DOE Office of Science is a useful resource and acts as a “one-stop-shop” for all information related to facilities and infrastructure for instrumentation related to fabrication, characterization and measurements.11
4.4.5.2. U.S. National Science Foundation Facilities and Infrastructure Programs
NSF has invested in the development of state-of-the-art tools for advanced materials research, with direct or indirect applications to extraterrestrial materials characterization through a variety of programs, including within the Directorate for Geosciences, the Division of Materials Research, and in crosscutting programs from the Office of Integrated Activities. These include multiuser distributed instrumentation networks and arrays, accelerators, research vessels, aircraft, telescopes, and simulators, among others. In addition, NSF has also invested in Internet-based and distributed user facilities; some of which may be relevant to extraterrestrial materials analysis, archiving, and data analytics.12
In some cases, NSF-supported programs manage major facilities and infrastructure programs for extended duration—for example, the Laser Interferometer Gravitational Wave Observatory. In other cases, NSF partially or fully manages infrastructure programs and initiatives—for example, the National High Magnetic Field Laboratory (NHMFL)13 at Florida State University, which can be used to make high-performance nuclear magnetic resonance measurements to characterize organic molecular structures, and the National Superconducting Cyclotron Laboratory (NSCL),14 which can be used to generate tracers for tomographic imaging and related radioactive decay measurements. NHMFL and NSCL are unique, unusual, and potentially useful tools and techniques for analysis of returned extraterrestrial samples.
The Directorate of Geoscience (GEO) has several programs and initiatives that support specialized facilities and analytical instrumentation with overlaps with the sample return community. David Lambert, program director of instrumentation and facilities of the GEO provided an overview of these programs to the committee, which include funding of a single PI for instruments costing up to $500,000 through the regular program (e.g., SEMs, quadrupole ICP-MS), or, for more expensive instrumentation (e.g., electron microprobes, large-radius SIMS instruments), through the Major Research Instrumentation program, which provides funding in the range of $100,000 to $4 million. Very expensive instrumentation and facilities costing more than $100 million (e.g., telescopes) can be funded through the Major Research Equipment and Facilities Construction (MREFC) program. NSF-funded user facilities within the GEO directorate include the Consortium for Mineral Physics Research in the Earth Sciences (COMPRES), which funds mineral physics research in the Earth sciences, and GSECARS, which provides support for the synchrotron X-ray user facility for Earth sciences at APS. Many of the instruments currently employed for extraterrestrial sample analyses have been partially funded by NSF.15
Other notable NSF programs and facilities relevant to extraterrestrial materials analysis include the National Nanotechnology Coordinated Infrastructure (NNCI) program,16 which provides regional nodes of excellence in fabrication, and characterization facilities that are accessible to local/regional, national, and international institutions, as well as to corporations. These are geographically widely distributed and each one has some unique or integrated capabilities often needed to solve a particular materials analysis challenge.
4.4.5.3. National Institute for Standards and Technology Facilities
As part of the Department of Commerce, the National Institute for Standards and Technology (NIST) traditionally provides measurements and standards expertise to the scientific, technical, and corporate communities. As extraterrestrial materials handling, analysis, data archiving, and dissemination become more pervasive and globally accessible, NIST may offer unique opportunities for the community to standardize various experimental and computational parameters for more consistent and cross-correlative undertakings.
NIST runs a number of laboratories and operates two key facilities—the NIST Center for Neutron Research (NCNR) and the Center for Nanoscale Science and Technology.17 Several NIST facilities and expertise are available to the broader analytical community and users. For example, the Materials and Measurement Laboratory (MML) serves “as the national reference laboratory for measurements in the chemical, biological and materials sciences,”18 especially related to the certified reference materials, critically evaluated data and analysis, and other programs to ensure and assure the quality of measurements (see Section 2.2.3). MML coordinates the NIST-wide Standard Reference Material and Standard Reference Data programs.
The Precision Measurement Laboratory is involved in the science of “measurement” of diverse kinds. It sets the definitive U.S. standards for nearly every kind of measurement employed in commerce and research, provides NIST-traceable calibrations, and disseminates standards and best practices.
4.4.5.4. U.S. Department of Defense Programs and Facilities
The U.S. Department of Defense (DOD) science and technology (S&T) enterprise provides basic and applied research support and operates directly or indirectly several facilities and infrastructure programs related to materials synthesis, characterization, behavior, and system- or device-level considerations. The end of this section contains relevant Web portals that provide a broad overview and keyword searchable items for facilities and equipment available for research at the DOD S&T enterprise and related capabilities relevant to extraterrestrial materials analysis.
Some of the DOD laboratories are well known for their unique capabilities for materials research and analysis, which have been developed for many decades. Some notable examples include the Naval Research Laboratory (NRL), Wright-Patterson Air Force Base, and Edgewood Chemical Biological Center, which offer advanced technical capabilities and in-house expertise that may be relevant to handling and analysis of future extraterrestrial materials. The NRL has been involved in analyses of Stardust returned samples.19 Other major or unique capabilities include materials, structures, phenomena, systems and their behavior in the context of high-velocity impact, energetic materials and other military-related specialized capabilities and facilities.
DOD laboratories and facilities are typically accessible through collaborative programs or through contact with individual division and section heads, per local protocol and access constraints driven by DOD considerations.20
4.4.5.5. National Institutes of Health Infrastructure and Facilities
The National Institutes of Health (NIH) offer major instrumentation access and capabilities through their intramural program and support to external institutions and consortia. Some of these are called “cores,” which provide centralized and coordinated capabilities for specific biomedical research and development needs. Unlike agencies such as DOE or DOD, NIH does not have or has not invested in widespread physical presence or facilities and infrastructure. However, the cores and facilities affiliated with universities or institutions (at least partly supported by NIH) are spread widely throughout the United States (and outside) and have several modes of user access per constraints of the specific programs or NIH division or institute support. The Association of Biomolecular Resource Facilities (ABRF) provides a searchable database of various “cores” and associated tools, techniques, and capabilities for researchers. Some of these programs, initiatives and core facilities will likely have directly relevant experience for extraterrestrial materials and their analysis, especially as future incoming sample return materials will likely include organic or volatile substances, and thus be susceptible to damage during handling and examination.
In addition to primary NIH support, some of the core facilities and specialized instrumentation infrastructure for biomedical research across the United States are partly or wholly supported by foundations such as the Howard Hughes Medical Institute or the Gates Foundation, among many others. Extraterrestrial materials handling and analysis will likely benefit from the aforementioned capabilities, although these are geographically distributed and often invested for specific NIH institute support with specific mandates and goals.
NIH is now considering infrastructure investment similar to NSF and DOE programs (e.g., the regional facilities model of the NNCI program) in large projects such as Cryo-EM centers, magnetic resonance imaging (MRI), and related imaging initiatives. These facilities will likely operate analogous to DOE facilities and be accessible to communities and researchers for extraterrestrial materials analysis.
There are also many national biomedical and clinical research centers with extensive facilities and instrumentation infrastructure. In fact, each of the 28 institutes supports centers with research facilities. In particular, the National Institute of Biomedical Imaging and Bioengineering (NIBIB) may be a useful resource for extraterrestrial materials handling and characterization, as it also supports, nurtures, and (at least partly) manages research facilities.21 Other facilities include the NIH Core Facilities Support Portal22 and the ABRF portal.23
4.4.5.6. Miscellaneous Government-Funded Facilities/Infrastructure
There was a widespread recognition in policy circles in the United States after World War II that “science won the war.” This recognition was further reinforced during the Cold War and led to several public-private partnerships for research and development on behalf of the U.S. government. These are typically administered through the U.S. Code of Federal Regulations (Title 48, Part 35, Section 35.017) by universities and corporations.
These federally funded research and development centers (FFRDCs) in many cases have unique and useful resources for scientific and technical communities. FFRDCs also and often include major facilities, capabilities, and intellectual resources for materials handling, synthesis, characterization, measurements, and systems—quite possibly relevant for extraterrestrial materials analysis. For example, FFRDCs include the Center for Nuclear Waste Regulatory Analysis, operated by the Southwest Research Institute on behalf of the Nuclear Regulatory Commission, which may become an important resource should there be radioactive extraterrestrial materials returned in future missions. Other such niche examples can be found on FFDRCs Web portal.24
4.5. OVERVIEW OF INTERNATIONAL FACILITIES
The scientific study of extraterrestrial materials, including meteorites, cosmic dust, and returned samples, is a well-established field in more than a dozen countries, with noteworthy centers of excellence in Canada, Denmark, France, Germany, Japan, the United Kingdom, and Switzerland. Space missions aimed at solar system exploration, including sample return, are increasingly structured as international collaborations (e.g., Hayabusa2, OSIRIS-REx), with explicit plans for sharing returned materials between nations and dividing laboratory work to meet mission science goals. For these reasons, it will be advantageous to consider existing and likely future capabilities of prospective international partners when defining future investment in U.S. infrastructure for sample return science.
Appendix C summarizes technical data for more than two dozen international facilities that perform analytical science in support of previous, ongoing, or planned near-future sample return missions. The following list summarizes the committee's findings from review of these data:
- Instrumentation and technology strengths. The collective analytical instrumentation of the international facilities considered relevant to this study covers every major category of technology and analytical target that can be addressed by existing technologies, including common, commercially available instruments—that is, nonprototype versions of microscopes (SEM, TEM, EPMA) and spectroscopies (FTIR/Raman); commercially available common and more specialized mass spectrometry instrumentation—for example, MC-ICP-MS, TIMS, SIMS, and IRMS;25 large-scale user facilities based at national-scale synchrotron X-ray or neutron beam sources; and unique instrumentation specially built for returned sample analyses—for example, the Refrigerator Enhanced Laser Analyzer for Xenon (RELAX) instrument at the University of Manchester.The breadth and depth of the analytical capabilities of international facilities are impressive. Most of these laboratories duplicate widely available capabilities present in U.S. laboratories. However, if gaps emerge in U.S. capabilities in the general category of common, commercially available instruments (e.g., through decommissioning or nonrenewal of existing laboratories), it is notable that similar facilities exist in top laboratories doing return sample analyses elsewhere in the world. More importantly, several of the international facilities possess unique or prototype analytical or experimental equipment that cannot be found in U.S. institutions. Examples include the Argus collision cell in the MC-ICP-MS laboratory in Bristol, UK; the RELAX resonance ionization noble gas isotope instrument in Manchester, UK; the high-velocity particle impact laboratory in Heidelberg, Germany; and the breadboard Orbitrap flight instrument (dubbed the Cosmorbitrap) in Université d'Orléans, France. Each of these capabilities represents years to decades of investment and would be expensive to duplicate or supersede.
- Instrumentation and technology weaknesses. The primary weaknesses of international facilities, as compared to their U.S. peers, from the perspective of this report's charge, are the relatively small number and brief history of technology development projects directly inspired by and connected to the aims of returned sample science. There are several examples of ambitious, impactful instrument development projects conducted in the United States that grew directly out of sample return mission goals (in some cases even directly funded by sample return missions; e.g., Mega-SIMS and CHILI), and these are just the most recent examples of a long history of engagement between sample return missions and analytical laboratory developments (e.g., the Lunatic class of TIMS instruments in the 1970s). The lack of similar long-term engagement in international institutions puts international partners at a significant disadvantage, at least in the near term, when it comes to organizing and executing programs of technical development in support of sample return missions.
- Staffing strengths. The committee's review of international facilities revealed two significant strategic strengths in personnel management: (1) several narrow but scientifically important areas of technical and scientific leadership, particularly in the noble gas geochemistry of extraterrestrial materials (Nancy, France, and Manchester, UK). The decades of excellence demonstrated by these groups could not be readily duplicated elsewhere and are a valuable resource to support collective goals. (2) It is common (although not universal) for international institutions to provide salaried, permanent staff positions to support the construction, maintenance, and use of analytical instruments. This support is strongest in France, Germany, Canada, and Switzerland, where it is common for laboratories to be staffed by highly qualified technical staff whose salaries are paid by the university or government. For example, the Centre National de la Recherche Scientifique (CNRS) in France hired 332 new technical support staff in 2016.26 The stability of funding for these positions means these facilities can develop and maintain well-trained and experienced staff and are able to translate their skills from one generation to the next. This level of continuity is important for maintaining the highest levels of technical readiness, particularly for projects such as sample return missions, where the time span from mission conception to sample analysis can be 10 or more years. This model of staff support has largely disappeared in U.S. institutions over the last approximately 20 years, in response to changing financial models for academic institutions and funding goals and models from federal agencies. International partners provide clear examples of the benefits of their vision for the connection between support for technology and support for long-term development of the staff who make that technology work.
- Staffing weaknesses. While each individual laboratory and facility has its unique sets of strengths and weaknesses with regard to human resources, two trends are typical to foreign laboratories' staffing strategies in conjunction with sample return missions and represent weaknesses in these programs. First, compared with the United States, international laboratories have a relatively short heritage of close connection between the research groups spearheading the sample return missions and the research groups involved in the primary characterization of the returned samples. A second issue is that many European and Japanese academic organizations are strongly hierarchical, with fewer opportunities for promotions. This is an important issue for technical support staff who might also be early-career academics. The culture makes it difficult for junior scientists to advance in their careers over the time scale of a given mission. This serves as a disincentive for early-career scientists to take on the role of scientifically trained technical support staff.
4.6. LABORATORY AND FACILITY CONCLUSIONS
This chapter describes broad classes and thematic categories of analytical instrumentation and capabilities that are used in curation and analysis of existing returned samples and that may be used for future sample returns. This chapter also discusses various national and international facilities and their locations around the world. Specific examples of laboratories in the United States and abroad that house these capabilities are provided in Appendix Tables B.1 and C.1, respectively. Researchers use these instruments and facilities to characterize the physical properties and chemical composition of returned samples. In many cases, the functional behavior or properties associated with the samples can also be determined using complementary analytical techniques, or by using the same instrument in a different manner. For example, the atomic force microscope is often used for “metrology” (i.e., determining the sample size, shape, and related physical measurements). Yet, the same instrument can be used for magnetic force microscopy to map magnetic domain formation in the material, or to help reveal the distribution of magnetic phases across the field of view.
Infrastructure, instrumentation, and facilities are well developed for characterization and analysis of hard sample returns (i.e., minerals, glasses, metals, and rocks). The committee did not identify any techniques or instruments that are highly relevant to current returned samples or missions in flight that are missing or entirely unavailable to U.S. researchers. In some cases, such as synchrotrons with broad mission relevance, facilities are few, but they are generally available to users via peer-reviewed proposals. However, given finite life spans, instrumentation and facilities require continual upkeep and renewal to ensure that the latest technologies and methods are available for characterization and analysis of returned samples.
Samples of soft condensed matter, including organic materials, as well as gas- and ice-based materials, are becoming increasingly important to returned sample analysis. For example, organic materials are important targets for both missions currently in flight to sample primitive asteroids (Hayabusa2 and OSIRIS-REx). In addition, future missions may aim to return ices and gases. Thus, more increased capabilities for the collection, transport, curation, and analysis of such fragile and damage-prone materials and structures will be needed in the future, as outlined in the conclusions below.
Conclusion: The committee's analysis of analytical equipment available at U.S. laboratories indicates that there is a wide range of instrumentation that is currently accessible for returned sample analyses. There are no obvious gaps in instrumentation for analysis of returned rocks, glasses, minerals, and the current inventory of organic materials.
Conclusion: Missions in flight will not return samples for at least 5 years; therefore, some of the current analytical capabilities will be decommissioned before the samples are available.
Conclusion: Future sample return missions are focused on returning and analyzing more challenging materials (e.g., gases, ices, organic compounds) and will require investment in technologies that are not currently widely utilized by the sample return community.
Footnotes
- 1
See Table 4.2 for a listing of various types of instrumentation and Appendix F for definitions of abbreviations used for these instruments.
- 2
ISO Class 6 indicates less than 1,000 particles greater than or equal to 5 microns in size per square foot of air.
- 3
NASA, “CAPTEM Homepage,” https://www
.lpi.usra.edu/captem/, last updated September 26, 2018. - 4
NASA Johnson Space Center, 2012, “Lunar Sample Allocation Guidebook,” JSC-06090 Revision F, Houston, Tex., https://curator
.jsc.nasa .gov/lunar/sampreq /lunarallochndbk-jsc06090_revf_2012 .pdf. - 5
NASA Johnson Space Center, 2012, Genesis Research Sample Investigator's Guidebook, JSC 63358 Revision A, https://curator
.jsc.nasa .gov/genesis/forms /genesisguidebook-jsc63358reva.pdf. - 6
NASA Johnson Space Center, 2006, Stardust Sample Investigator's Guidebook, https://curator
.jsc.nasa .gov/stardust/forms /stardustinvestigatorsguidebook.pdf. - 7
NASA Goddard Space Flight Center, 2017, “NASA's OSIRIS-REx Asteroid Sample Return Mission,” FS-206-4-411-GSFC, http://www
.nasa.gov/sites /default/files/atoms /files/osiris_rex_factsheet5-9.pdf. - 8
Brown University, 2014, “RELAB Spectral Database,” NASA Reflective Laboratory disclaimer, http://www
.planetary .brown.edu/relabdocs/relab_disclaimer .htm. - 9
See Appendix F for abbreviations and Table 4.2 for a more detailed enumeration of capabilities.
- 10
Jeffrey Grossman, NASA, personal communication.
- 11
U.S. Department of Energy, “User Facilities,” https://science
.energy .gov/user-facilities/, last updated July 2, 2018. - 12
National Science Foundation, “Major Multi-User Research Facilities,” https://www
.nsf.gov/about /budget/fy2018/pdf/36_fy2018.pdf, accessed December 7, 2018. - 13
National High Magnetic Field Laboratory, “Homepage,” https:
//nationalmaglab.org, accessed December 7, 2018. - 14
National Superconducting Cyclotron Laboratory, “Homepage,” https://www
.nscl.msu.edu/index.php, accessed December 7, 2018. - 15
D. Lambert, R. Kelz, and K. Johnson, 2017, “Instrumentation & Facilities (IF) Program: Division of Earth Sciences, Directorate for Geosciences,” presentation to the committee, November 20, 2017.
- 16
National Nanotechnology Coordinated Infrastructure, “Homepage,” https://www
.nnci.net/, accessed December 7, 2018. - 17
National Institute for Standards and Technology, “User Facilities,” https://www
.nist.gov /labs-major-programs/user-facilities, last updated October 12, 2018. - 18
Material Measurement Laboratory, “Homepage,” https://www
.nist.gov/mml, accessed December 7, 2018. - 19
B.T. De Gregorio, R.M. Stroud, L.R. Nittler, and A.L.D. Kilcoyne, 2017, Evidence for reduced, carbon-rich regions in the solar nebula from an unusual cometary dust particle, Astrophysical Journal 848(2), doi:10.3847/1538-4357/aa8c07.
- 20
For a detailed list of DOD laboratories, see www
.defenseinnovationmarketplace .mil/laboratories.html. For DOD science and technology listings, see https://www .acq.osd.mil /chieftechnologist/ and https://www .defense.gov /News/Special-Reports /0715_science-tech/. For searchable facilities/laboratories doing basic research at DOD, see http: //basicresearch.defense.gov/. All accessed December 7, 2018. - 21
National Institute of Biomedical Imaging and Bioengineering, “Research Funding NIBIB-Supported Biomedical Technology Resource Centers,” https://www
.nibib.nih .gov/research-funding /featured-programs /biomedical-technology-resource-centers /supported-centers, accessed December 7, 2018. - 22
National Institutes of Health, “Frequently Asked Questions: Core Facilities,” https://grants
.nih.gov /grants/policy/core_facilities_faqs .htm#3626, last updated April 18, 2013. - 23
Federation of American Societies of Experimental Biology, 2018, Instrumentation: Federal Grants and Programs for the Life Sciences, FASEB Shared Research Resources Subcommittee, updated April 25, http://www
.faseb.org /Science-Policy--Advocacy-and-Communications /Science-Policy-and-Research-Issues /Shared-Research-Resources.aspx. - 24
National Science Foundation, “Master Government List of Federally Funded R&D Centers,” https://www
.nsf.gov/statistics /ffrdclist/, last updated March, 2018. - 25
See Appendix F for abbreviations and Table 4.2 for a more detailed enumeration of capabilities.
- 26
Centre National de la Recherche Scientifique 2016, “2016: A Year at CNRS, Excerpts from the 2016 Annual Report,” http://www
.cnrs.fr/en /science-news/docs/RA2016-en.pdf.
- Current Laboratories and Facilities - Strategic Investments in Instrumentation a...Current Laboratories and Facilities - Strategic Investments in Instrumentation and Facilities for Extraterrestrial Sample Curation and Analysis
Your browsing activity is empty.
Activity recording is turned off.
See more...