NCBI Bookshelf. A service of the National Library of Medicine, National Institutes of Health.
National Academies of Sciences, Engineering, and Medicine; Division on Earth and Life Studies; Board on Environmental Studies and Toxicology; Committee on Endocrine-Related Low-Dose Toxicity. Application of Systematic Review Methods in an Overall Strategy for Evaluating Low-Dose Toxicity from Endocrine Active Chemicals. Washington (DC): National Academies Press (US); 2017 Jul 18.

Application of Systematic Review Methods in an Overall Strategy for Evaluating Low-Dose Toxicity from Endocrine Active Chemicals.
Show detailsTo safeguard public health, the US Environmental Protection Agency (EPA) must keep abreast of new scientific information and emerging technologies, so it can apply them to public-health protection and regulatory decision making. In the chemical assessment arena, the agency has dealt for decades with questions about what animal-testing data to use to predict human-health hazards, estimate dose-response relationships, protect susceptible subpopulations, and address uncertainties. As alternatives to traditional toxicity testing have emerged, the agency has faced additional decisions about incorporating nontraditional data into its chemical assessments and whether such tests can replace some traditional testing methods. Calls for more transparency in assessments that influence regulatory decisions have led to the agency's use of better-defined and -documented approaches for evaluating and integrating evidence. More recently, EPA has embraced recommendations that its Integrated Risk Information System (IRIS) program use approaches that are more systematic in their literature-based assessments. Some context about these challenges and how they led to the request for this report are briefly discussed below.
ADVANCES IN TOXICITY TESTING
Toxicity tests evaluate chemicals for their potential to cause cancer, birth defects, and other adverse health effects. Information from toxicity testing serves as an important basis for public-health protection and regulatory decisions concerning chemicals. Traditional test methods were developed incrementally over several decades and are conducted using laboratory animals. Researchers typically test chemicals at high doses in animals to induce toxicity and to identify a dose at which no adverse effect is observed. Uncertainty factors are then used to derive a human exposure level designed to be protective of human health. Assumptions are made that dose-response relationships from these studies can be extrapolated below the tested dose range and that the effects observed in animals are relevant to humans. Examples have shown, however, that these assumptions are not applicable to all chemicals (e.g., Maronpot et al. 2004; NRC 2004; Bracken et al. 2009), and debates often arise about how to account for differences in response between test animals and humans. Research directed at addressing those debates has focused on understanding the mechanisms of how chemicals cause adverse effects, with increasing emphasis being placed on evidence of biological effects (versus overt toxicity) as a predictor of adverse effects.
In the early 2000s, EPA and other federal agencies began to alter their testing strategies to focus on developing in vitro test methods that would allow less expensive and more rapid toxicity screening of large numbers of chemicals. The National Research Council (NRC) report Toxicity Testing in the 21st Century: A Vision and a Strategy (NRC 2007) was influential in stimulating large-scale initiatives to determine how in vitro testing methods could be used to predict human toxicity, most notably the Tox21 program. The Tox21 program is a collaborative effort among EPA's National Center for Computational Toxicology, the National Toxicology Program, the National Institutes of Health National Center for Advancing Translational Sciences, and the US Food and Drug Administration. The program's work focuses on understanding chemically induced biological effects and developing predictive models of human biological response. An important contribution to the Tox21 program is the chemical screening results from EPA's Toxicity Forecaster (ToxCast). The ToxCast program has used hundreds of high-throughput screening methods and computational toxicology approaches to evaluate thousands of chemicals. High-throughput assays evaluate specific types of bioactivity at the molecular or cellular level. Information about a particular biochemical interaction might help predict modulation of specific biological pathways that can lead to an adverse outcome. Thus, the number of biological targets for ascertaining the toxicity of a chemical has grown substantially.
EPA has used computational methods to estimate the human daily oral doses that would produce steady-state in vivo blood concentrations equivalent to chemical concentrations used in ToxCast assays. The estimates were compared with data on human oral exposures to identify chemicals for which the values overlap, and in vivo effects associated with this subset of chemicals (evaluated from the standard toxicological studies required for product registration) were compared with assay end point hits (Rotroff et al. 2010; Wetmore et al. 2012; Judson et al. 2014). One goal of these evaluations was to predict reproductive, developmental, and cancer end points (Judson et al. 2014).
ENDOCRINE ACTIVE CHEMICALS
Endocrine active chemicals (EACs), also referred to as endocrine disruptors, have the ability to modulate hormone function by mimicking, blocking, or otherwise altering activities of endogenous hormones. Because small alterations in hormone concentrations or activities, particularly during sensitive life stages, could have lasting and significant effects, environmental exposures to these types of chemicals are of particular concern. EPA was directed by Congress in the 1996 Food Quality Protection Act and the Safe Drinking Water Act amendments to begin screening pesticides for their potential to produce effects similar to those produced by estrogen in humans. The agency also was given authority to screen other chemicals and to evaluate other endocrine effects. In response to the congressional mandate, EPA created the Endocrine Disruptor Screening and Testing Committee, which developed a two-tiered screening approach. The first tier is used to screen chemicals for their potential to interact with the estrogen, androgen, or thyroid hormone systems, and EPA has worked for many years on developing and validating a battery of assays for these purposes. Because of developments associated with the Tox21 program, an initiative called “EDSP21” was begun in 2012 to validate and incorporate computational or in silico models and in vitro high-throughput screening methods into the program. The second tier of testing involves identifying adverse endocrine-related effects and quantitatively characterizing the dose-response relationship. Despite advancements and expansion of some testing protocols to include endocrine sensitive end points, concerns continue to be raised that traditional toxicity-testing practices might not include evaluation of end points relevant to endocrine disruptors (Vandenberg et al. 2012; EFSA 2013; Gore et al. 2015).
DEFINING LOW DOSE
Early in its deliberations, the committee considered several definitions of low dose that were developed by other National Academies committees, EPA, and the National Toxicology Program (NTP). For example, EPA (2013) adopted NTP's definition of a low-dose effect as “a biological change occurring in the range of typical human exposures or at doses lower than those typically used in standard testing protocols” (NTP 2001). An independent group that evaluated the scientific evidence on low-dose effects and nonmonotonic dose-response (NMDR) relationships for endocrine-disrupting chemicals in mammalian species adopted similar language (Melnick et al. 2002).1
For the purposes of this report, the committee looked for common ground to define low dose. Some definitions of low dose include language similar to that found in the EPA definition that obliquely defines a point of reference: “doses lower than those typically used in standard testing protocols.” Those phrases help define an upper exposure range because the highest dose used in many guideline-driven animal toxicity studies is often determined by the maximum tolerated dose (MTD).2 The lowest dose used in standard guideline-driven toxicity studies is frequently a log order lower than the MTD. The vast majority of relevant human exposures will fall below the levels that are used in regulatory toxicity tests. However, some animal studies use dose ranges that deviate from standard testing protocols, thereby blurring the distinction between doses used in animal studies and human exposures. Therefore, the committee considered the need for additional context when defining a high-dose point of reference based on animal toxicity studies.
The committee also considered elements found in other definitions. For example, some definitions of low dose, including that developed by EPA, contain the clause “a biological change occurring in the range of typical human exposures” without explicitly defining “typical” human exposures. Another issue that was debated at length related to the variety of terms used by the scientific community to describe dose (e.g., applied and internal dose) and exposure (e.g., exposure is an external measure, whereas dose is an internal measure) and how the terms might best be incorporated into the definition of low dose. The NRC report Exposure Science in the 21st Century: A Vision and a Strategy (NRC 2012) also commented on the inconsistent use of the terms dose and exposure and advocated primarily using the term exposure because it is more broadly applicable. Consistent with that recommendation, the committee used exposure rather than dose in its definition of low dose.
The committee also debated whether occupational exposures might represent a “typical” human exposure because they might be orders of magnitude higher than exposures that occur in the general population. Despite the differences inherent between environmental and occupational exposure (Semple 2005), the definition of low dose developed by the committee does not exclude occupational epidemiologic studies from the evidence stream for evaluating low-dose effects. That decision was deemed important because occupational studies could provide critical evidence regarding whether a chemical has endocrine-active effects. Additionally, occupational exposures are not necessarily higher than nonoccupational exposures for certain types of compounds and uses. Therefore, the committee's definition recognizes that a range of exposures from environmental to occupational might be relevant to the investigation of low-dose endocrine effects. However, the committee recognizes that, in trying to define low-dose endocrine effects, information on effects at environmental exposures might take precedence over or have higher priority than information on effects at higher exposures that might occur in occupational settings.
Ultimately, the committee defined low dose as external or internal exposure that falls within the range estimated to occur in humans. Human exposure estimates may be based on environmental or biomarker measurements and/or computational models; ideally, the estimates account for toxicokinetic processes. If no human exposure estimates are available, low dose is defined on a case-by-case basis relative to an explicitly specified exposure in a particular context, such as “below the U.S. EPA Reference Dose (RfD) for chemical X” or “below the point of departure (NOAEL or BMDL) derived from the extended one-generation study for chemical X.” This definition acknowledges that a single definition cannot be used in all contexts, so no generalizations can be made.
The committee recognizes that some readers might not be comfortable with its definition because it lacks a bright line by which low dose can be defined. Defining such a bright line is not strictly based on science but rather encompasses policy decisions that were beyond the purview of the committee. Similarly, although the idea of a threshold or the shape of the dose-response relationship is important to the historical etiology of the concept of low dose (Chateauraynaud et al. 2014), the committee concluded that it was not necessary to address these ideas explicitly in its definition.
LOW-DOSE EFFECTS
Traditional animal testing was designed to identify effect levels by using a dosing regimen that includes a dose that elicits an overt response and at least two lower doses, one of which should be a dose where no effect is observed. In EPA chemical assessments, such studies are often used to identify a no-observed-adverse-effect level (NOAEL) or a lowest-observed-adverse-effect level (LOAEL), which is then used as a starting point for quantifying human reference levels. Concerns have been raised that low-dose effects of EACs are not being identified by traditional toxicity tests because the dosing regimen does not include doses within the range of human exposure or because some end points are not evaluated (e.g., Vandenberg et al. 2012; EFSA 2013; Gore et al. 2015). In some cases, scientists disagree about whether certain effects are adverse. Disagreements include the degree to which a biological change from normal is adverse; whether some effects are adaptive and have little effect on an organism; defining the degree to which functional capacity is so impaired as to be considered adverse; and uncertainties about the reliability and sensitivity of precursor effects to predict adverse outcomes.
NONMONOTONIC DOSE-RESPONSE CURVES
A conventional assumption of toxicology is that the dose-response relationship between a chemical and an adverse health effect will have a monotonic shape: that is, the slope of the curve does not change sign. NMDR curves change sign at one or more inflection points. In the case of chemicals that exhibit a U-shaped curve, effects are more prominent at low and high doses than they are at intermediate doses. Evidence for such curves could be missed if the doses tested are not low enough or not enough doses are tested to reveal such a shape. This situation is also true for identifying inverted U-shaped curves and more complex dose-response curves.
NMDR curves have been reported to be of particular concern for EACs (Vandenberg et al. 2012; Lagarde et al. 2015). Although NMDR relationships are often discussed in conjunction with low-dose effects, they are separate issues. In 2013, EPA issued a draft report, State of the Science Evaluation: Nonmonotonic Dose Responses as They Apply to Estrogen, Androgen, and Thyroid Pathways and EPA Testing and Assessment Procedures, to evaluate the evidence on NMDR curves and to make judgments about EPA's toxicity-testing practices and the implications for its risk-assessment procedures. The draft concluded that exposure to EACs can result in NMDR curves for specific end points and that such curves were found more often in in vitro studies, at high doses, and for exposures of short duration. It also asserted that there was insufficient evidence that NMDR curves for adverse effects occur below traditional thresholds derived from toxicity testing. An NRC committee reviewed that report and found deficiencies in how the agency conducted its evaluation (NRC 2014a). Specifically, the committee noted that different literature-evaluation strategies were used to assess chemicals that affect the estrogen, androgen, and thyroid systems, and these differences weakened the agency's ability to draw firm conclusions. The committee recommended that a more structured and formal process be used to evaluate different evidence streams, in keeping with recommendations of other NRC reports (NRC 2011, 2014b). More recent reviews of the evidence on NMDRs have been conducted by ANSES (the French Agency for Food, Environmental and Occupational Health and Safety) and the European Food Safety Authority (EFSA) (Lagarde et al. 2015; Beausoleil et al. 2016).
Although the committee was not charged with specifically addressing NMDR curves, it notes that important considerations in evaluating such relationships is whether studies have sufficient statistical power or a broad enough dose range to identify whether an NMDR exists. Human epidemiologic data in particular might lack the power to identify an NMDR relationship. That opinion is supported by a recent EFSA report that reviewed the evidence of NMDRs for substances relevant to food safety. Beausoleil et al. (2016) developed screening criteria—an indication of an NMDR was reported in the study, at least three dose groups were used, and the relevant test substance was not tested in the form of a mixture—to evaluate the relevance and reliability of the evidence from in vivo, in vitro, and human epidemiologic studies. Data sets from studies deemed relevant and reliable were later evaluated using statistical software and then ranked on the basis of the strength of the evidence for an NMDR curve. None of the human data sets were considered suitable for NMDR analysis. Challenges with using human data were found in another study that developed qualitative methods to assess published in vivo, in vitro, and human epidemiologic studies for the presence of NMDRs (Lagarde et al. 2015).
SYSTEMATIC REVIEW
Two NRC reports (2011, 2014b) have made recommendations that EPA's IRIS program use more transparent and consistent methods for conducting its toxicological evaluations. Systematic review methods were identified as having the necessary elements to support those types of assessments. A systematic review uses explicit, prespecified methods to identify, select, assess, and summarize findings of similar but separate studies to answer a focused research question. The systematic review process is undertaken to identify all relevant studies on the agent of interest, to evaluate the studies identified, and to provide a qualitative and, where possible, a quantitative synthesis of the identified studies. Methods for conducting systematic reviews of the comparative effectiveness of clinical interventions are well established (e.g., Cochrane Collaboration [Higgins and Green 2011] and IOM [2011]), and the methods have been adapted (e.g., Woodruff and Sutton 2014; NTP 2015) and used to answer environmental health questions. EPA has also begun incorporating systematic review approaches into its chemical evaluations.
STATEMENT OF TASK
Faced with the challenges described above, EPA requested that the National Academies convene an ad hoc committee to develop a strategy for evaluating evidence of low-dose adverse human effects that act through an endocrine-mediated pathway. The verbatim statement of task is provided in Box 1-1. In response to this request, the National Academies convened the Committee on Endocrine-Related Low-Dose Toxicity, which prepared this report. Biographical information on the committee members is presented in Appendix A.
COMMITTEE'S APPROACH
The statement of task had several components that could be interpreted in a variety of ways. The following is the committee's interpretation of the task and how it approached and addressed each of the components. One objective of the task was to develop a strategy for EPA to evaluate evidence of low-dose adverse human health effects, with the aim of using the strategy to evaluate whether its toxicity-testing practices are adequate for identifying low-dose effects. The committee's strategy included the use of the systematic review method as an investigation and data analysis tool. This helped tie the strategy with other major elements of the task, namely the performance of systematic reviews for animal and human evidence streams for two chemicals. The other components of the task were performed in support of the strategy or as part of the systematic review process. Key definitions for terms used throughout the report are provided in Box 1-2.
The requirement that the committee perform systematic reviews of at least two chemicals appeared to be a request for a demonstration of how to perform such reviews and for consideration of how systematic review methods would fit in an overall strategy for evaluating low-dose effects. From a methodologic standpoint, two sets of guidelines for performing systematic reviews for environmental health assessments were considered by the committee: the Navigation Guide (Woodruff and Sutton 2014) and the National Toxicology Program's Office of Health Assessment and Translation (OHAT) method (Rooney et al. 2014; NTP 2015). The guidelines have similar methods, and the committee used both approaches to conduct its systematic reviews.
It was necessary to focus on EACs that have a robust database of human and animal studies because the task specified that the committee demonstrate the integration of animal and human evidence. The committee judged that the strongest evidence would likely be from developmental exposure, so preference was given to developmental outcomes. A number of potential chemicals were considered, including bisphenol A, DDT, genistein, methoxychlor, parabens, perfluorooctanoic acid, phthalates, polybromindate diphenyl ethers (PBDEs), polychlorinated biphenyls (PCBs), 2,3,7,8-tetrachlorodibenzo-p-dioxin (TCDD), and triclosan.
A public workshop was held on February 3, 2016, to assist the committee with selecting the topics for its systematic reviews. The committee decided that three case examples would be the focus of the workshop, with the expectation that the discussions would be relevant to other EACs. The three chemicals (and end points) explored at the workshop were phthalates (male reproductive malformations), TCDD (male reproductive effects), and bisphenol A (female reproductive effects). Experts on these chemicals and other EACs were invited to serve as panelists at the workshop to discuss the available data and issues that are relevant to performing a systematic review. Draft PECO statements3 and a series of questions to consider provided the platform for the workshop discussions. The questions posed to the panelists were aimed at facilitating discussion about what end points had enough data on which to base a systematic review that would ultimately help answer questions about toxicity-testing practices. The PECO statements and the questions posed during the workshop are presented in Appendix B.
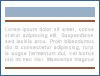
BOX 1-1
Verbatim Statement of Task.
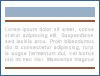
BOX 1-2
Definitions of Terms Used in the Consensus Study Report.
Informed by the deliberations of the February 2016 workshop, the review articles on EACs, and the expertise of the committee members, the committee decided to focus on two chemical classes and specific developmental outcomes: phthalate effects on male reproductive-tract development and PBDE effects on neurodevelopment. During the course of exploring the database on PBDEs, the committee discovered that a systematic review of the association between developmental exposures to PBDEs and human neurodevelopment was under way and soon to be completed (Lam et al. 2015). Because of this, PBDEs were removed from consideration as a case example at the workshop. However, after further consideration, the committee decided to conduct a case study of how an existing systematic review could be used by EPA. In this case example, the existing systematic review on PBDEs and human neurodevelopment was evaluated and updated by the committee and the results integrated with an independent systematic review of the animal evidence on PBDEs and neurodevelopment. Thus, the committee performed three independent systematic reviews and updated one existing systematic review. The approach used by the committee for its independent reviews was the OHAT method, whereas the authors of the existing review followed the Navigation Guide method. Table 1-1 summarizes the approaches used in the different reviews and illustrates that the methods are compatible. It also presents the approaches used to integrate the evidence.
After the systematic reviews were performed, the committee integrated the human and animal evidence using an approach developed by OHAT (NTP 2015). Mechanistic information was considered in this step to make determinations about the biological plausibility of the observed effects, to consider concordance or discordance in the evidence, and to draw conclusions about the potential hazard to humans. The nature and relevance of dose-response relationships in animals and humans were examined for the purposes of making a determination about whether observed effects are associated with low doses. Where possible, the implications of the results for the purpose of evaluating the adequacy of EPA's toxicity-testing practices were examined.
ORGANIZATION OF THE CONSENSUS STUDY REPORT
The committee's report is organized into a summary, five chapters, and six appendixes. Chapter 2 presents the committee's proposed strategy for evaluating evidence of low-dose adverse human effects that act through an endocrine-mediated pathway. Chapters 3 and 4 focus on the systematic reviews performed by the committee. Chapter 3 presents the evaluation of the animal and human evidence on phthalates and male reproductive-tract development and describes how the evidence was integrated to draw conclusions about associations and low-dose effects. Chapter 4 presents the results of the systematic reviews and assessments that were performed on PBDEs and developmental neurotoxicity. The lessons learned from performing the systematic reviews and further reflections on the statement of task are provided in Chapter 5.
Appendix A provides biosketches of the committee members. The other appendixes are provided as a PDF file available at https://www.nap.edu/catalog/24758.
TABLE 1-1
Methods Used in the Systematic Reviews and Evidence Integration Presented in Chapters 3 and 4.
REFERENCES
- Beausoleil C, Beronius A, Bodin L, Bokkers BGH, Boon PE, Burger M, Cao Y, De Wit L, Fischer A, Hanberg A, Leander K, Litens-Karlsson S, Rousselle C, Slob W, Varret C, Wolterink G, Zilliacus J. Review of non-monotonic dose-responses of substances for human risk assessment. EFSA Supporting Publication. 2016;13(5):1027E. [May 3, 2016]; https://www
.efsa.europa .eu/en/supporting/pub/1027e. - Bracken MB. Why animal studies are often poor predictors of human reaction to exposure. J. R. Soc. Med. 2009;102(3):120–122. [PMC free article: PMC2746847] [PubMed: 19297654]
- CDC (Centers for Disease Control and Prevention). Fourth Report on Human Exposure to Environmental Chemicals. Atlanta, GA: U.S. Department of Health and Human Services, Centers for Disease Control and Prevention; 2009. [June 15, 2016]. http://www
.cdc.gov/exposurereport/ - Chateauraynaud F, Debaz J, Fintz M. Chemical substances on the frontiers of health security: Metro-logical controversies over endocrine disruptors and low doses. Soc. Sci. Inform. 2014;53(4):437–452.
- EFSA (European Food Safety Authority) Scientific Committee. Scientific opinion on the hazard assessment of endocrine disruptors: Scientific criteria for identification of endocrine disruptors and appropriateness of existing test methods for assessing effects mediated by these substances on human health and the environment. EFSA J. 2013;11(3):3132. [June 15, 2016]; http:
//onlinelibrary .wiley.com/doi/10.2903/j .efsa.2013.3132/full. - EPA (U.S. Environmental Protection Agency). State of the Science Evaluation: Nonmonotonic Dose Responses as They Apply to Estrogen, Androgen, and Thyroid Pathways and EPA Testing and Assessment Procedures. U.S. Environmental Protection Agency; Jun, 2013. 2013. [May 15, 2016]. https://www
.epa.gov/sites /production/files /2016-01/documents/nmdr.pdf. - Gore AC, Chappell VA, Fenton SE, Flaws JA, Nadal A, Prins GS, Toppari J, Zoeller RT. EDC-2: The Endocrine Society's second scientific statement on endocrine-disrupting chemicals. Endocr. Rev. 2015;36(6):E1–E150. [PMC free article: PMC4702494] [PubMed: 26544531]
- Guyatt GH, Oxman AD, Kunz R, Brozek J, Alonso-Coello P, Rind D, Devereaux PJ, Montori VM, Freyschuss B, Vist G, Jaeschke R, Williams JW Jr., Murad MH, Sinclair D, Falck-Ytter Y, Meerpohl J, Whittington C, Thorlund K, Andrews J, Schunemann HJ. GRADE guidelines 6. Rating the quality of evidence—imprecision. J. Clin. Epidemiol. 2011;64(12):1283–1293. [PubMed: 21839614]
- Higgins JPT, Green S, editors. Cochrane Handbook for Systematic Reviews of Interventions, Version 5.1.0 (updated March 2011). The Cochrane Collaboration; 2011. [March 8, 2017]. www
.handbook.cochrane.org. - IOM (Institute of Medicine). Finding What Works in Health Care: Standards for Systematic Review. Washington, DC: The National Academies Press; 2011. [PubMed: 24983062]
- IPCS (International Programme on Chemical Safety). Part 1: IPCS/OECD Key Generic Terms Used in Chemical Hazard/Risk Assessment. Part 2: IPCS Glossary of Key Exposure Assessment Terminology. Geneva: World Health Organization; 2004. [November 11, 2015]. IPCS Risk Assessment Terminology. http://www
.inchem.org /documents/harmproj/harmproj/harmproj1 .pdf. - Judson R, Houck K, Martin M, Knudsen T, Thomas RS, Sipes N, Shah I, Wambaugh J, Crofton K. In vitro and modelling approaches to risk assessment from the U.S. Environmental Protection Agency ToxCast Programme. Basic Clin. Pharmacol. Toxicol. 2014;115(1):69–76. [PubMed: 24684691]
- Lagarde F, Beausoleil C, Belcher SM, Belzunces LP, Emond C, Guerbet M, Rousselle C. Nonmonotonic dose-response relationships and endocrine disruptors: A qualitative method of assessment. Environ. Health. 2015;14(13) [PMC free article: PMC4429934] [PubMed: 25971433]
- Lam J, Sutton P, McPartland J, Davidson LI, Daniels N, Sen S, Axelrad D, Lanphear B, Bellinger D, Woodruff TJ. Applying the Navigation Guide Systematic Review Methodology, Case Study #5. Association between Developmental Exposures to PBDEs and Human Neurodevelopment: A Systematic Review of the Evidence Protocol. 2015 2015 April; [November 10, 2016]; http://www
.crd.york.ac .uk/PROSPEROFILES/17890 _PROTOCOL_20150322.pdf. - Lam J, Lanphear B, Bellinger D, Axelrad DA, McPartland J, Sutton P, Davidson L, Daniels N, Sen S, Woodruff T. Systematic review and meta-analysis of association between PBDE exposure and IQ or ADHD. 2016. Draft report provided J. Lam (University of California, San Francisco) on July 1, 2016. [PMC free article: PMC5783655] [PubMed: 28799918]
- Maronpot RR, Flake G, Huff J. Relevance of animal carcinogenesis findings to human cancer predictions and prevention. Toxicol. Pathol. 2004;32(Suppl. 1):40–48. [PubMed: 15209402]
- Melnick R, Lucier G, Wolfe M, Hall R, Stancel G, Prins G, Gallo M, Reuhl M, Ho SM, Brown T, Moore J, Leakey J, Haseman J, Kohn M. Summary of the National Toxicology Program's report of the endocrine disruptors low-dose peer review. Environ. Health Perspect. 2002;110(4):427–431. [PMC free article: PMC1240807] [PubMed: 11940462]
- NRC (National Research Council). Intentional Human Dosing Studies for EPA Regulatory Purposes: Scientific and Technical Issues. Washington, DC: The National Academies Press; 2004. Appendix A. Values and limitations of animal toxicity data; pp. 159–167. [PubMed: 25009864]
- NRC. Toxicity Testing in the 21st Century: A Vision and a Strategy. Washington, DC: The National Academies Press; 2007.
- NRC. Review of the Environmental Protection Agency's Draft IRIS Assessment of Formaldehyde. Washington, DC: The National Academies Press; 2011. [PubMed: 25032397]
- NRC. Exposure Science in the 21st Century: A Vision and a Strategy. Washington, DC: The National Academies Press; 2012. [PubMed: 24901193]
- NRC. Review of the Environmental Protection Agency's State-of-the Science Evaluation of Nonmontonic Dose-Response Relationships as They Apply to Endocrine Disruptors. Washington, DC: The National Academies Press; 2014a.
- NRC. Review of EPA's Integrated Risk Information System (IRIS) Process. Washington, DC: The National Academies Press; 2014b. [PubMed: 25101400]
- NTP (National Toxicology Program). National Toxicology Program's Report of the Endocrine Disruptors Low Dose Peer Review. National Toxicology Progrm, U.S. Department of Health and Human Services, National Institute of Environmental Health Sciences, National Institutes of Health; Research Triangle Park, NC: 2001.
- NTP. Handbook for Conducting a Literature-Based Health Assessment Using OHAT Approach for Systematic Review and Evidence Integration. Office of Health Assessment and Translation, Division, National Toxicology Program, National Institute of Environmental Health Sciences; Jan 9, 2015. 2015. [September 21, 2015]. http://ntp
.niehs.nih .gov/ntp/ohat/pubs/handbookjan2015_508 .pdf. - Rooney AA, Boyles AL, Wolfe MS, Bucher JR, Thayer KA. Systematic review and evidence integration for literature-based environmental health science assessments. Environ. Health Perspect. 2014;122(7):711–718. [PMC free article: PMC4080517] [PubMed: 24755067]
- Rotroff DM, Wetmore BA, Dix DJ, Ferguson SS, Clewell HJ, Houck KA, LeCluyse EL, Andersen ME, Judson RS, Smith CM, Sochaski MA, Kavlock RJ, Boellmann F, Martin MT, Reif DM, Wambaugh JF, Thomas RS. Incorporating human dosimetry and exposure into high-throughput in vitro toxicity screening. Toxicol. Sci. 2010;117(2):348–358. [PMC free article: PMC6280841] [PubMed: 20639261]
- Semple S. Assessing occupational and environmental exposure. Occup. Med. 2005;55(6):419–424. [PubMed: 16140834]
- Vandenberg LN, Colborn T, Hayes TB, Heindel JJ, Jacobs DR Jr., Lee EH, Shioda T, Soto AM, vom Saal FS, Welshons WV, Zoeller RT, Myers JP. Hormones and endocrine-disrupting chemicals: Low-dose effects and nonmonotonic dose responses. Endocr. Rev. 2012;33(3):378–455. [PMC free article: PMC3365860] [PubMed: 22419778]
- Wetmore BA, Wambaugh JF, Ferguson SS, Sochaski MA, Rotroff DM, Freeman K, Clewell HJ III, Dix DJ, Andersen ME, Houck KA, Allen B, Judson RS, Singh R, Kavlock RJ, Richard AM, Thomas RS. Integration of dosimetry, exposure, and high-throughput screening data in chemical toxicity assessment. Toxicol. Sci. 2012;125(1):157–174. [PubMed: 21948869]
- Whiting P, Savovic J, Higgins JB, Caldwell DM, Reeves BC, Shea B, Davies P, Kleijnen J, Churchill R. ROBIS: A new tool to assess risk of bias in systematic reviews was developed. J. Clin. Epidemiol. 2016;69:225–234. [PMC free article: PMC4687950] [PubMed: 26092286]
- Woodruff TJ, Sutton P. The Navigation Guide systematic review methodology: A rigorous and transparent method for translating environmental health science into better health outcomes. Environ. Health Perspect. 2014;122(10):1007–1014. [PMC free article: PMC4181919] [PubMed: 24968373]
Footnotes
- 1
Low-dose effects were defined as “biologic changes that occur in the range of human exposures or at doses lower than those typically used in the standard testing paradigm of the US EPA for evaluating reproductive and developmental toxicity” (Melnick et al. 2002, p. 427).
- 2
An MTD is broadly defined as the highest dose of a chemical that can be administered to an animal without causing excessive toxicity.
- 3
A PECO (Population, Exposure, Comparator, and Outcome) statement is a framework to clarify aspects of the review question. It guides the literature search, inclusion and exclusion criteria, the types of data to be considered, and the approach to synthesizing the evidence.
- Introduction - Application of Systematic Review Methods in an Overall Strategy f...Introduction - Application of Systematic Review Methods in an Overall Strategy for Evaluating Low-Dose Toxicity from Endocrine Active Chemicals
- Halosaccion glandiforme voucher KAM0114 ribulose-1,5-bisphosphate carboxylase/ox...Halosaccion glandiforme voucher KAM0114 ribulose-1,5-bisphosphate carboxylase/oxygenase large subunit (rbcL) gene, partial cds; chloroplastgi|2186270433|gnl|uoguelph|ABMMC246 .rbcL|gb|MN992110.1|Nucleotide
- Halosaccion glandiforme voucher 17_Seaweeds_AS0036 cytochrome oxidase subunit 1 ...Halosaccion glandiforme voucher 17_Seaweeds_AS0036 cytochrome oxidase subunit 1 (COI) gene, partial cds; mitochondrialgi|2057579043|gnl|uoguelph|SDP65126 COI-5P|gb|MN456829.1|Nucleotide
- txid644663[Organism:noexp] (1)PMC
Your browsing activity is empty.
Activity recording is turned off.
See more...