NCBI Bookshelf. A service of the National Library of Medicine, National Institutes of Health.
Committee on State of Molybdenum-99 Production and Utilization and Progress Toward Eliminating Use of Highly Enriched Uranium; Nuclear and Radiation Studies Board; Division on Earth and Life Studies; National Academies of Sciences, Engineering, and Medicine. Molybdenum-99 for Medical Imaging. Washington (DC): National Academies Press (US); 2016 Oct 28.
This chapter addresses the third charge of the statement of task for this study (see Sidebar 1.3 in Chapter 1):
An assessment of progress made in the previous 5 years toward establishing domestic production of molybdenum-99 for medical use, including the extent to which other medical isotopes that have been produced with molybdenum-99, such as iodine-131 and xenon-133, are being used for medical purposes.
As noted in Chapter 1, the committee interprets “the previous 5 years” to encompass the time since completion of the 2009 Academies report on medical isotope production (NRC, 2009), that, is since January 2009.
Since 2009, the Department of Energy's National Nuclear Security Administration (DOE-NNSA) has signed cooperative agreements with five private-sector companies to assist their efforts to develop domestic (i.e., U.S.-based) capabilities to supply molybdenum-99 (Mo-99) without the use of highly enriched uranium (HEU) targets. Section 4.1 describes the progress by these partners in establishing domestic production as of June 2016.1
Several U.S. national laboratories are also being funded by NNSA to support the development of domestic production of Mo-99 without HEU. The involved national laboratories include Argonne National Laboratory (ANL), Oak Ridge National Laboratory (ORNL), Los Alamos National Laboratory (LANL), Pacific Northwest National Laboratory (PNNL), and Savannah River National Laboratory (SRNL). The Y-12 National Security Complex, an NNSA facility located in Oak Ridge, Tennessee, is also providing technical support. These development activities, which are public domain and nonproprietary, are summarized in Table 4.1.
TABLE 4.1
National Laboratory Support to Non-HEU Mo-99 Production Technologies.
In addition to the activities funded directly by NNSA, some national laboratories have also established cooperative research and development agreements or strategic partnership projects (formerly known as work for others agreements) directly with companies seeking to develop Mo-99 production technologies. These agreements constitute contracts between companies and national laboratories to carry out company-specified research and development (R&D) work. The companies pay the labs directly for this work, and the results of this work are provided directly to the companies and are not made public.
At least seven other private-sector companies are working independently of NNSA to develop domestic Mo-99 production or related capabilities. These efforts are described in Section 4.2. Efforts to develop capabilities for domestic production of iodine-131 (I-131) and xenon-133 (Xe-133) are described in Section 4.3. The committee's analysis of progress toward establishing domestic production of Mo-99 and other medical isotopes is provided in Section 4.4.
4.1. DOE-NNSA COOPERATIVE AGREEMENT PROJECTS
DOE-NNSA supports the establishment of U.S. domestic production of Mo-99 under its HEU minimization mission. As noted previously, NNSA has signed cooperative agreements with five companies to assist their efforts to develop domestic capabilities to produce Mo-99:
- Babcock & Wilcox (B&W) (now BWX Technologies)
- General Atomics (GA)
- General Electric-Hitachi (GEH)
- NorthStar Medical Radioisotopes
- SHINE Medical Technologies
These cooperative agreements provide for 50-50 cost sharing between NNSA and these companies; NNSA provides up to $25M in cost sharing per project for work that contributes directly to the establishment of a domestic Mo-99 production capability.
The first NNSA cooperative agreements were awarded to B&W and GEH in 2009 through a noncompetitive process. The companies suspended their projects in 2012 and 2011, respectively, after completing their planned first phases of work but before developing any commercial Mo-99 production capabilities. NNSA awarded $9.1M to B&W2 and $2.25M to GEH in cost-shared funding before the companies suspended work.
A competitive process was put into place by NNSA in 2010 to select the remaining three cooperative agreement partners.3 The criteria for evaluation of projects by NNSA included project significance, technical feasibility, ability to demonstrate the capability to produce 3,000 6-day curies (Ci) per week by the end of calendar year 2013, and business-case viability. NNSA informed the committee that it established this production demonstration goal for three reasons4:
- 1.
To provide the team of independent technical experts (which was assembled by NNSA to review the proposed projects) with an objective criterion by which to judge the viability of the proposed projects.
- 2.
To give the commercial partners, selected under the NNSA's Funding Opportunity Announcement (FOA), an aggressive schedule goal to work toward in executing their projects.
- 3.
To encourage commercial entities in the United States to secure a domestic supply of Mo-99 before Canada stops production of Mo-99 after October 2016 (see Chapters 3 and 7).
The 3,000 6-day Ci per week production demonstration goal proved to be overly optimistic: None of the cooperative agreement partners met this goal by the end of 2013; in fact, this goal still had not been met when the present report was being finalized for publication.
NNSA currently evaluates proposals for additional funding from current cooperative agreement partners using 2016 as the evaluation criterion for schedule. NNSA uses other criteria in addition to schedule for evaluating these proposals.
The cooperative agreement projects are depicted graphically in Figure 4.1 and described in the following subsections. These descriptions are based on information provided to the committee by representatives of the companies that are involved in these projects and from the committee's independent research. The schedule information provided below is current as of the end of July 2016.

FIGURE 4.1
Domestic Mo-99 production projects supported by NNSA cooperative agreements.
4.1.1. NorthStar Medical Radioisotopes
NorthStar Medical Radioisotopes LLC5 (NorthStar) has entered into cooperative agreements with NNSA to develop domestic Mo-99 production using two different processes:
- 1.
Photon-induced transmutation of molybdenum-100 (100Mo(γ,n)99Mo) using photons produced with linear accelerators. The cooperative agreement for this project was awarded in September 2010 (Staples, 2011).
- 2.
Neutron capture of molybdenum-98 (98Mo(n,γ)99Mo) using neutrons produced in a research reactor. The cooperative agreement for this project was awarded in November 2013 (NNSA, 2013).
These production processes are described in Section 2.3.2 in Chapter 2. To date, NNSA has given NorthStar roughly $6M for its photon-induced transmutation project and $25M for its neutron capture project.
The Mo-99 produced by these two processes has a low specific activity (1-10 curies (Ci)/gram), too low to be used in the conventional technetium generators described in Chapter 2. NorthStar has developed a new technetium generator system, the RadioGenix Tc-99m Generating System, to utilize low-specific-activity Mo-99. This system is described in Section 4.1.1.3 in this chapter.
4.1.1.1. Photon-Induced Transmutation
NorthStar plans to establish the capacity to produce >3,000 6-day Ci of Mo-99 per week at its Beloit, Wisconsin, facility by irradiating Mo-100 targets with electrons produced by linear accelerators. This approach heavily leveraged work at the Idaho National Laboratory in the mid-1990s (Bennett et al., 1999) and the late 2000s (Nelson et al., 2007; TRIUMF, 2008). Several accelerators and target stations will be used, each capable of producing roughly 500 6-day Ci per week of low-specific-activity Mo-99 (see Figure 4.2). The target stations will contain many separated disks of Mo-100 that will be cooled with high-pressure gaseous helium (He) during irradiation. The targets will be irradiated with a total of 240 kilowatts (kW) of 40 megaelectron volt (MeV) electrons (120 kW would be directed at each end of the target assembly). Two different types of electron accelerators are being considered to generate the electron beams.

FIGURE 4.2
Schematic layout of an accelerator vault for NorthStar's photon-induced transmutation production project. The vault accommodates two linear accelerators and two target irradiation stations. SOURCE: Gregory E. Dale, Los Alamos National Laboratory.
NorthStar is working with ANL and LANL to develop their technical approach for Mo-99 production using photon-induced transmutation (see Table 4.1). Four critical subsystems have been tested at ANL using an electron linear accelerator having 10-20 kW power: (1) the target, (2) He cooling, (3) accelerator diagnostics, and (4) Mo-100 recovery. In addition, ANL is helping to design the production facility beamline and shielding. LANL is designing the production target and remote handling assembly.
The targets tested at ANL consist of up to 25 molybdenum disks, each 12 mm in diameter, 1-mm thick, and separated by 1 mm for He cooling. The target being designed for NorthStar would consist of 82 molybdenum disks, 29 mm in diameter, 0.5-mm thick and separated by 0.25 mm for He cooling. The ANL tests have also studied the He-cooling system and accelerator diagnostics (diagnostics are challenging because of high radiation in the target region). Full-power tests of these systems have not been completed.
One of the challenges with this production method is the availability and cost of Mo-100 target material. Enriched targets are required for this production method to be effective (natural molybdenum contains only about 10 percent Mo-100). The cost of the targets will be significant.6 Only a small fraction of the Mo-100 is converted into Mo-99 during irradiation; consequently, cost-effective recycling schemes will be needed to recover unused Mo-100. Approximately 95 percent efficiency of recycling of Mo-100 has been demonstrated at ANL (Tkac, 2015).
NorthStar plans to install and start up the accelerators at its Beloit, Wisconsin, facility in 2018 and to establish the capability to produce 3,000 6-day Ci of Mo-99 by the end of 2019. The feasibility of Mo-100 production using accelerators has been demonstrated at low power, but tests of high-power systems have not yet been completed.
4.1.1.2. Neutron Capture Production
NorthStar will use the University of Missouri Research Reactor Center7 (MURR) reactor to irradiate Mo-98 targets to produce Mo-99 by neutron capture. The targets will contain either natural (24 percent Mo-98) or enriched (>90 percent Mo-98) molybdenum. The company expects to be able to produce 750 6-day Ci of Mo-99 per week using natural molybdenum targets and at least 3,000 6-day Ci of Mo-99 per week using enriched Mo-98 targets.
A few kilograms of enriched Mo-98 will be required each year to produce these quantities of Mo-99,8 assuming that Mo-98 from irradiated targets is recovered and reused. Recycling of enriched targets has been demonstrated at ANL with about a 95 percent recovery rate (Tkac and Vandegrift, 2015).
A proprietary system has been constructed at MURR to dissolve natural and enriched molybdenum targets and extract Mo-99; two of the hot cells used for Mo-99 extraction are illustrated in Figure 4.3. Additionally, the first of two fill lines has been installed at MURR to prepare Mo-99 for shipment to nuclear pharmacies that will be equipped with NorthStar's RadioGenix Tc-99m Generating System. A second fill line is planned for installation at MURR in fall 2016. Spent generator solutions will be returned to NorthStar, where they will be held to decay before recycling or disposal. Recycling of enriched targets and spent solutions will take place in the company's Beloit facility.

FIGURE 4.3
Top: Hot cells at MURR for processing of Mo-98 targets for NorthStar's neutron capture production process. Bottom: Dissolution apparatus in the hot cell. SOURCE: Courtesy of University of Missouri Research Reactor Center.
NorthStar has completed its first Mo-99 production-scale test run9 and has received approval from MURR10 to begin routine production of 200 6-day Ci of Mo-99 per week. The next significant step in the regulatory approval process will be Food and Drug Administration (FDA) approval of a final amendment to NorthStar's New Drug Application (NDA) (see Appendix 4A). NorthStar expects to have the capacity to produce up to 3,000 6-day Ci of Mo-99 per week on a routine basis in 2017.
4.1.1.3. RadioGenix Tc-99m Generating System
The RadioGenix Generator System utilizes a Tc-99m-selective resin column (ABEC-2000) to recover sodium pertechnetate (NaTcO4) containing Tc-99m from an alkaline solution of Mo-99 (see Figure 4.4).11 The generator can utilize either high- or low-specific-activity Mo-99 but was designed specifically for use with the low-specific-activity Mo-99 produced by NorthStar. The column is charged with a solution of low-specific-activity Mo-99 in 5 molar (M) KOH. The column is sequentially washed with a 5 M KOH solution to remove any Mo-99 that was adsorbed onto the column and then with a pH 8 buffer solution.

FIGURE 4.4
NorthStar's RadioGenix Tc-99m Generating System. The system is 54” (1.4 m) wide × 28” (0.7 m) deep × 75” (1.9 m) tall, including the monitor. SOURCE: Jim Harvey, NorthStar Medical Radioisotopes.
Tc-99m is eluted from the generator as sodium pertechnetate using 0.9 percent NaCl solution. The eluate is passed through an alumina (Al2O3) guard column to remove residual Mo-99 impurities. The Tc-99m eluate is also passed through a 0.22-μm membrane filter (located inside the system) for microbe removal prior to preparation of radiopharmaceuticals or direct administration to patients.
The RadioGenix system is largely automated. After 40 minutes of initial setup, the system will generate an elution of sodium pertechnetate in a few tens of minutes without further operator intervention (the total cycle time is 60-70 minutes). The system includes shielded waste containers and an ozonation system to control bio-contamination. The generator can be eluted multiple times a day just like conventional technetium generators. Tc-99m separation efficiencies of >90 percent have been obtained for several consecutive days of elution with no detectable Mo-99 breakthrough.
The RadioGenix system is currently undergoing FDA review. An NDA (see Appendix 4A) was submitted in 2013 and modifications were made in response to a Complete Response Letter. The current (fifth generation) version of the RadioGenix system is being used to generate data to support a resubmission of the NDA for FDA review. NorthStar plans to introduce the generator system to the market once this NDA is approved by the FDA.
NorthStar has signed a letter of intent with GE Healthcare to supply Mo-99 to produce Tc-99m for compounding and distribution (NorthStar, 2014a) once its generator system has been approved by the FDA and is commercially available. NorthStar also recently signed a similar agreement with the nuclear pharmacy chain Triad Isotopes, Inc. (NorthStar, 2014b).
4.1.2. SHINE Medical Technologies
Morgridge Institute for Research,12 in cooperation with SHINE Medical Technologies,13 entered into a cooperative agreement with NNSA in September 2010 (Sereno, 2012) and has so far received $15M in funding from NNSA (NNSA, 2015a). The company is developing an accelerator-driven subcritical low enriched uranium (LEU) assembly to produce Mo-99 by neutron fission (235U(n,f)99Mo). The general concept is illustrated in Figure 4.5. SHINE plans to build multiple accelerator–LEU target assemblies to produce 4,000 6-day Ci per week of Mo-99 for domestic and overseas sale.

FIGURE 4.5
Top: Schematic depiction of SHINE's deuterium-tritium fusion-driven subcritical reactor concept. Bottom: Image of the system. SOURCE: Courtesy of SHINE Medical Technologies, Inc.
A linear accelerator is used to inject a 300 kV, 60 mA beam of deuterium ions (see Figure 4.6) into a tritium gas target. The deuterium fuses with tritium to produce helium and approximately 5 × 1013 free 14 MeV neutrons per second over a 100 cm length. The neutrons are multiplied as they pass through a natural uranium layer. These neutrons are used to irradiate an aqueous solution of LEU uranyl sulfate to produce Mo-99, I-131, Xe-133, and other fission products. The LEU solution is passed through a titanium dioxide column after about 5.5 days of irradiation to extract Mo-99.

FIGURE 4.6
Photo of a beam of deuterium ions after acceleration in the neutron driver produced for SHINE by Phoenix Nuclear Labs. SOURCE: Courtesy of Phoenix Nuclear Labs.
ANL has performed the first several in a series of “mini-SHINE” experiments (ANL/CSE-14/2; see Table 4.1) in which they demonstrated the overall integrated process, which includes target solution preparation from uranium metal, target solution irradiation, extraction, and purification. The main difference between these experiments and the planned SHINE facility is that an electron accelerator was used in the experiments to generate neutrons. ANL has conducted the first two of these irradiations, successfully producing Mo-99 to British Pharmacopeia14–level specifications at curie levels. ANL plans to scale up production to tens of curies in fiscal year 2017. Additionally, LANL has demonstrated that the target solution can be recycled, allowing for multiple irradiations of a target solution preparation without affecting molybdenum extraction.
The electrostatic accelerator-based neutron driver is produced for SHINE by Phoenix Nuclear Labs (PNL). The accelerator generates deuterium ions, accelerates them, and focuses them into the gaseous tritium target chamber. PNL has built three generations of this neutron generator, and the lab has demonstrated stable operation with 99 percent uptime at 50 percent of the planned beam output for the facility version. PNL has also successfully demonstrated the required ion extraction and focusing behavior, target gas operation and pumping, and the expected fusion reaction yields in the target chamber. PNL has delivered a fully functioning, high-reliability accelerator to Picatinny Arsenal for the U.S. Army, a system that shares many design aspects with the accelerator design for the SHINE facility. PNL has also delivered a high-output neutron generator to Ultra Electronics in the United Kingdom.
The tritium inventory in the accelerator and pumping systems is <100 mg (<1,000 Ci). The tritium recovery and purification system used by SHINE is based on a process from the SRNL known as the Thermal Cycling Absorption Process (TCAP). This process has been used at SRNL for decades on a significantly larger scale than is required for SHINE. SRNL has built a smaller prototype TCAP system that can handle recovery demands from three operating accelerators. The TCAP prototype acceptance testing was conducted at SRNL in December 2013.
The SHINE assembly does not reach criticality15 like an aqueous reactor does; however, the nuclear physics principles governing the operations of subcritical assemblies and reactors are similar. These principles have been validated through a number of national laboratory experiments, including in KEWB-Core A, KEWB-Core B, LOPO, HYPO, SUPO, and SILENE aqueous reactors. These reactors have been shown to operate in a predictable and stable manner. Working in support of SHINE, LANL has shown excellent agreement between the modeling codes and the measurements from these reactors for steady-state and transient behavior. SHINE has also validated its computer models against benchmark data to ensure that the predictions of the codes are accurate.
Irradiation of the uranyl sulfate target solution produces hydrogen and oxygen through radiolysis. These gases bubble out of the target solution and accumulate in the target solution vessel (TSV) headspace. Without proper mitigation, the hydrogen in this headspace would quickly reach potentially explosive concentrations. SHINE uses a gas recirculation and treatment system called the TSV Off-Gas System (TOGS) to mitigate this risk and maintain hydrogen concentrations well below lower flammability limits. TOGS is designed to remove hydrogen from the TSV atmosphere using catalytic recombination, a recognized and proven technology. Reduced-scale demonstration systems have been built at LANL and ANL, and a full-scale test system has been built at SHINE. Testing and experimentation on these systems has demonstrated that the established technologies implemented in TOGS are capable of safely and reliably mitigating hydrogen accumulation risk in the TSV. Additionally, hydrogen recombination using catalytic recombiners is a common process used at nuclear power facilities throughout the United States.
After irradiation and cool-down, the LEU target solution is transferred to a processing hot cell and loaded onto a proprietary titanium oxide chromatography column for separation of molybdenum from uranium and most other fission products. This process has been demonstrated a number of times at both LANL (May et al., 2013, 2014) and ANL (Stepinski et al., 2013; Youker et al., 2014). Additionally, multiple irradiations have been performed on target solutions without affecting the ability to extract molybdenum, allowing for multiple recycles of the target solution.16 The recycling of target material allows for higher uranium-235 utilization compared to conventional reactor-based Mo-99 production.
After separation, the molybdenum is further purified for medical use. This purification consists of several well-established chemical processing steps that have been demonstrated multiple times at ANL.17 A similar purification process was in commercial use at Cintichem (formerly Union Carbide in Tuxedo, New York) and is currently in commercial use in Indonesia at Badan Tenaga Atom Nasional, where LEU is used to produce Mo-99. GE Healthcare announced in November 2015 that it had successfully obtained pharmaceutical-grade Tc-99m from their Drytec generators using Mo-99 made from the SHINE process.
The licensing process for the SHINE project is in progress. An Environmental Impact Statement and Safety Evaluation Report were published in October 2015, and the Construction Permit for the facility in Janesville, Wisconsin, was issued in February 2016.
SHINE has signed agreements to supply Mo-99 to GE Healthcare,18 Lantheus,19 and China's HTA Co., Ltd.20 SHINE plans to begin commercial sales of Mo-99 in 2019.
4.1.3. Babcock & Wilcox
B&W, now known as BWX Technologies, was awarded a cooperative agreement by NNSA on September 30, 2009, to develop a 200-kW homogeneous solution reactor, referred to as the Medical Isotope Production System (MIPS), to produce Mo-99 (Reynolds, 2008). B&W suspended work on this project in 2012 after determining that the time and cost involved with the project would be greater than anticipated (SNMMI, 2012).
The MIPS is a compact cylindrical solution reactor that is surrounded by a neutron reflector and contains control rods and cooling coils. The reactor fuel is a solution containing an LEU salt, such as uranyl nitrate [UO2(NO3)2], dissolved in water and acid. This fuel is also the target material for Mo-99 production. The reactor is designed to operate at about 80°C and ambient atmospheric pressure. The reactor would be operated for approximately 5 days, during which time Mo-99 builds up in the fuel solution as a result of uranium fission reactions. The reactor would then be shut down for two days, during which time the Mo-99 will be recovered and purified (DOE, 2014; IAEA, 2013). A three-reactor system could supply about 50 percent (~2,500 6-day Ci) of U.S. demand for Mo-99.
B&W has also investigated uranyl sulfate fuel solutions. The main advantage of this fuel is that its radiolytic decomposition products are H2 and O2 only. Radiolytic decomposition of a uranyl nitrate solution is far more complex, because nitrate is reduced to form nitrite, nitrogen, and nitrogen oxide (NOx) gases. Ammonium ions are also generated (IAEA, 2013). The difficulty of using the uranyl nitrate solution was a major contributor to the discontinuation of this project.
The MIPS reactor is conceptually similar in design to the Argus Reactor at the Kurchatov Institute in Russia. This reactor has already been used to demonstrate the production of Mo-99 from uranyl sulfate (Ball, 1999).
R&D was under way to address several technical issues when the project was suspended (e.g., Chemerisov et al., 2008; Gelis et al., 2008; Vandegrift et al., 2008; Ziegler et al., 2008). The Argentine company INVAP was performing R&D under a contract with B&W on reactor design and Mo-99 sorbent efficiency. ANL was carrying out analyses of the chemistry of salt solutions in operating solution reactors and the recovery of Mo-99. The laboratory was researching the potential for formation of uranium precipitates in the nitrate salt solution, radiation effects on oxidation of molybdenum, and the effects of nitrate decomposition on the treatment of gases produced by radiolysis in the reactor. Argonne researchers reported that there was a “high potential for the successful implementation of this technology” (Vandegrift et al., 2008).
B&W had hoped to construct MIPS facilities to supply Mo-99 to U.S. and international markets. The company estimated that their facilities would be operating by 2014-2015 provided that they had identified a radiopharmaceutical partner and had obtained full funding for the project. This schedule assumed the successful completion of the R&D programs and the resolution of several legal and regulatory issues, including MIPS licensing, waste disposal, and LEU availability.
4.1.4. General Electric-Hitachi
GEH was awarded a cooperative agreement by NNSA on September 30, 2009, to pursue the use of power reactors as the source of neutrons to produce Mo-99 via neutron capture (98Mo(n,γ)99Mo), the same process being used in one of the NorthStar projects (see Section 4.1.1). GEH and Exelon announced in September 2011 that they were jointly studying the feasibility of producing Mo-99 at the Clinton Power Station, a boiling water reactor located in central Illinois. Clinton is already being used to produce cobalt-60 for medical use. GEH also signed memoranda of understanding with NuView Life Sciences (see Section 4.2.6 in this chapter) and NorthStar Medical Radioisotopes to process irradiated targets and sell the recovered Mo-99 (GEH, 2011). GEH suspended the project in February 2012 because of an adverse market outlook (Forrest, 2012).
4.1.5. General Atomics
NNSA selected a project proposed by GA21 for cooperative agreement funding through the 2010 FOA. However, GA did not sign the agreement with NNSA until October 2015. The project has received $9.7M in NNSA funding to date (NNSA, 2015b).
GA is partnering22 with Nordion and MURR to develop, demonstrate, and commercialize a GA-patented technology, selective gaseous extraction (SGE), for production of Mo-99 with LEU targets. GA is developing the LEU targets and gas extraction systems; MURR will install these systems in its reactor and operate them to produce Mo-99 for Nordion; and Nordion is providing the necessary capital to develop and implement the technology and has exclusively licensed it to MURR for a 20-year period.
The original concept for this project involved the in situ extraction of Mo-99 from two LEU targets installed in the graphite reflector in the MURR reactor (see Figure 4.7) to produce up to 4,200 6-day Ci of Mo-99 per week. The targets would be irradiated continuously while the reactor was operating. Mo-99 would be extracted periodically from each target by flowing a gas mixture containing chlorine and oxygen through the target material. (Molybdenum would be extracted as gaseous molybdenum oxychloride [MoO2Cl2]). The gas mixture containing extracted Mo-99 would be piped to a hot cell for subsequent processing.

FIGURE 4.7
Schematic illustration of the major components of the reactor at MURR. The targets for selective gaseous extraction will be installed in the graphite reflector. SOURCE: Modified from a graphic provided by Ralph Butler, MURR.
This technical approach was modified after preliminary testing of some design components. Mo-99 will now be produced using single-use targets consisting of UO2 pellets contained in zirconium rods.23 The rods will be placed into two wedge-shaped housings (see Figure 4.8) installed in the graphite reflector in the MURR reactor. The targets will be irradiated continuously for 1-3 weeks depending on customer demand and then removed for processing during planned reactor shutdowns.

FIGURE 4.8
GA's target assembly for Mo-99 production. The target material is contained in the vertical tubes shown in green; the large tube shown in gray at the top of the target housing is for water cooling. SOURCE: Kathy Murray, General Atomics (GA).
The rods will be transferred to a bank of hot cells at MURR and processed using SGE to recover Mo-99. Noble gases will be co-extracted with Mo-99 and captured in a cryogenic trap. Some other fission products will also be separated.24 The extraction waste will consist of UO2 powder. The separated Mo-99 will be transported to Nordion for purification and packaging for commercial sale. Nordion estimates that up to 3,200 6-day Ci of Mo-99 per week can be produced using this modified SGE process.
SGE has been demonstrated in the laboratory with single-use targets doped with Mo-99 and with irradiated target pellets. According to Nordion, both tests demonstrated high Mo-99 yields.
Work is under way to obtain regulatory approvals for this modified process. MURR is proposing a license amendment to its regulator (NRC) to implement this technology. Once regulatory approval is received, prototype targets will be installed in the MURR reactor. (This action is planned for the summer of 2017.) The prototypes will be irradiated over a period of 3 months to gather the data needed for filing a Drug Master File (DMF; see Appendix 4A) with the FDA. Once the DMF is completed, Nordion will install additional targets in the MURR reactor for producing Mo-99 for commercial sale. Nordion expects to install these targets and begin producing Mo-99 in the first half of 2018.
4.2. OTHER PROJECTS FOR DOMESTIC PRODUCTION OF Mo-99
Several private companies are working toward establishing domestic production of Mo-99 or related processes without NNSA funding. The committee obtained information on development efforts by seven companies25 (NNSA, 2014):
- Coquí RadioPharmaceuticals
- Eden Radioisotopes
- Flibe Energy
- Niowave
- Northwest Medical Isotopes (NWMI)
- NuView Life Sciences
- PermaFix Medical
The first five of these projects are depicted graphically in Figure 4.9, and all seven of these projects are described briefly in the following subsections.

FIGURE 4.9
Five private-sector domestic Mo-99 production projects.
4.2.1. Coquí RadioPharmaceuticals
Coquí RadioPharmaceuticals, a Puerto Rico–based company, plans to produce up to 7,000 6-day Ci of Mo-99 per week for the U.S. and export markets by irradiating LEU targets in two 10 megawatt (MW) LEU-fueled research reactors (Coquí RadioPharmarmaceuticals, 2015a). The company plans to build these reactors and associated Mo-99 production facility on the 170 acre “Duct Island” site at Oak Ridge, Tennessee. The land was granted by DOE and the Community Reuse Organization of East Tennessee in June 2016.26 Coquí has contracted with the Argentinian nuclear engineering firm INVAP to design and construct the facility, which will include two open-pool reactors similar in design to Australia's INVAP-designed OPAL reactor. The facility will also include a radioisotope processing plant, a waste conditioning plant, a radiopharmaceutical production plant, nuclear and medical support services, and administrative offices (Coquí RadioPharmaceuticals, 2015b).
A schematic and concept design for the facility was completed in April 2015 (Coquí RadioPharmaceuticals, 2015c), and the company has announced its intention to submit a license application to the NRC to construct the facility. The application had not been submitted when the present report was being finalized for publication.
4.2.2. Eden Radioisotopes
Eden Radioisotopes, established in 2013, has licensed Sandia National Laboratories' design for a dedicated Mo-99 production reactor (Salem, 2014). This open-pool reactor has a compact (less than 0.5 m in diameter and height), low-power (<2 MW) core consisting of LEU fuel that also serves as targets for Mo-99 production. The individual fuel elements would be removed and chemically processed using a modified Cintichem process to recover Mo-99, I-131, and Xe-133. The company claims that it could supply U.S. needs for Mo-99 by operating the reactor at 670 kW and removing the fuel elements for chemical processing every 7 days. The company also claims that it could supply current world needs for Mo-99 by operating the reactor at 1.5 MW.27 The company has notified the NRC that it intends to file a license application to construct a reactor and processing facility near Hobbs, New Mexico. The company is in the process of raising funds to complete and submit the license application. The company must also qualify the fuel and targets to be used in the reactor.
4.2.3. Flibe Energy
Flibe Energy, located in Huntsville, Alabama, but registered in Delaware, is a nuclear startup company that aims to develop a liquid fluoride thorium reactor (LFTR) for nuclear power production.28 The company submitted a notice of intent (Flibe Energy, 2013) to the NRC in May 2013 to submit a license application for an LFTR and Mo-99 production facility. The site for the reactor and production facility has not been selected. The notice indicated that the reactor would have a thermal power of less than 2 MW and would be fueled with fluoride salt containing thorium.
During operation of the reactor, thorium in the reactor fuel captures a neutron and is transmuted to uranium-233 (U-233), which is fissile. The fission of U-233 with neutrons produces a spectrum of fission products including Mo-99. The Mo-99 produced in the reactor would be extracted on-line.
The notice of intent indicated that the company was raising private funding to support the conceptual design, construction, licensing, and operation of the reactor, and also that the company tentatively anticipated the submission of a research reactor license application in the fall of 2015. This application had not been submitted by the time the present report was being finalized for publication.
4.2.4. Niowave
Niowave, a Michigan-based company, plans to build a Mo-99 medical isotope production facility in Lansing, Michigan.29 The facility would produce Mo-99 using a neutron source that drives a subcritical LEU assembly to create fission Mo-99, similar to the approach being developed by SHINE (see Section 4.1.2 in this chapter).
The Niowave accelerator could produce 1-2 × 1014 neutrons per second using a 100 kW, 40 MeV electron beam on a lead-bismuth eutectic target, or >1015 neutrons per second with a 400 kW, 80 MeV electron beam. The 100 kW electron beam is expected to be sufficient to create roughly 400 6-day Ci of Mo-99 per week with an upgrade path to a 400 kW electron beam that would produce roughly 1,500 6-day Ci of Mo-99 per week.30
The company produces a line of turnkey commercial superconducting electron linear accelerators that could be used to produce a 100 kW, 40 MeV electron beam. The company indicated that this accelerator could be upgraded to 400 kW electron beam power.
Niowave has constructed a facility adjacent to the Lansing airport that will house the superconducting accelerator and associated infrastructure, and it has installed the necessary cryogenics plant for a 100 kW accelerator. This accelerator facility is licensed by the State of Michigan. The liquid lead-bismuth target for neutron production has been demonstrated at 2 kW beam power and was used to irradiate a small sample of uranium. Niowave announced the production of small quantities of Mo-99 from a LEU sample in late 2015. Further testing is under way, but a schedule for completing the testing and commencing of Mo-99 production has not yet been announced.
4.2.5. Northwest Medical Isotopes
NWMI, an Oregon-based company, plans to produce 3,500 6-day Ci of Mo-99 per week by irradiating LEU targets in the MURR reactor and a research reactor at Oregon State University. The targets would be processed to recover Mo-99 in a radioisotope production facility to be constructed by the company in Columbia, Missouri.31 The production facility would also be used to recover uranium from the dissolved LEU solution and recycle it back into new LEU target material. The LEU material production process would utilize a proprietary technology developed by Oregon State University.
A construction permit application for the processing facility was submitted to the NRC in 2014. The company expects to start construction of the facility in early 2017.
4.2.6. NuView Life Sciences
As noted in Section 4.1.4 of this chapter, NuView Life Sciences, located in Denton, Texas, signed a memorandum of understanding with GEH in 201132 to process irradiated targets from GEH's neuron capture project. GEH announced in February 2012 that it was suspending its Mo-99 production project (Forrest, 2012).
4.2.7. Perma-Fix Medical
Perma-Fix Medical, a subsidiary of Perma-Fix Environmental Services in Atlanta, Georgia, was formed to develop and commercialize a new class of radiation-resistant resin (Micro-Porous Composite Material) that allows for high loading of molybdenum and efficient elution of Tc-99m. This material could be especially useful in technetium generators containing low-specific-activity Mo-99.
In March 2014, Perma-Fix Medical announced the successful validation of the radiation resistance of this resin and its high elution efficiency for Tc-99m based on testing at POLATOM in Warsaw, Poland, and MURR (Perma-Fix Environmental Services, Inc, 2014). The company has filed patent applications for this resin and has signed a letter of intent to form a strategic partnership with Digirad Corporation, a major national provider of in-office nuclear cardiology imaging services (Digirad, 2015). Perma-Fix has no plans to produce Mo-99 or Tc-99m.
4.3. DOMESTIC PRODUCTION OF I-131 AND Xe-133
At present, there is no production of I-131 or Xe-133 in the United States. Nordion (Canada) currently supplies most of these isotopes. Institut National des Radioéléments (IRE) (Belgium) began supplying Xe-133 to the United States under an arrangement with Lantheus Medical Imaging (see Chapter 3).
A number of U.S.-based organizations may produce these isotopes domestically in the future:
- MURR has received a license amendment from the NRC to produce I-131 by irradiating tellurium targets (the production process is described in Chapter 2). MURR is performing qualification runs to develop the information needed for a DMF, which is planned to be filed with the FDA in the second half of 2016. MURR has signed an I-131 supply agreement with one customer (the identity of this customer has not yet been made public) and is in negotiations with another potential customer.
- SHINE is planning to recover Xe-133 and/or I-131 and possibly other isotopes from its Mo-99 production process.33 The decision on which isotopes to recover will be made at a later date and will likely depend on market conditions.
- The contract between Nordion and GA for the SGE technology to be implemented at MURR allows for the recovery of other fission isotopes besides Mo-99.34 However, Nordion has not made any public statements regarding its plans to recover I-131 or Xe-133.
- Eden Radioisotopes has also indicated that it plans to recover both I-131 and Xe-133 as part of its Mo-99 production processes.
4.4. PROGRESS TOWARD ESTABLISHING DOMESTIC PRODUCTION OF Mo-99
This section provides the results of the committee's readiness assessment for each of the Mo-99 production projects described in Sections 4.1 and 4.2 of this chapter. This assessment provides the committee's collective technical judgment about the progress being made by each of these projects to demonstrate the establishment of a domestic capability to produce Mo-99.
The committee used the following criteria to assess project readiness (see Table 4.2):
TABLE 4.2
Criteria Developed by the Committee to Assess Readiness of Domestic Mo-99 Production Projects.
- Has the Mo-99 production technology been demonstrated?
- Are production facilities available?
- Are the target materials needed for production available?
- Is the product (Mo-99 or Tc-99m) compatible in the existing supply chain?
- Have the necessary regulatory approvals for the facilities used for production been obtained?
- Have the necessary regulatory approvals for the production products (Mo-99, Tc-99m) been obtained?
- What is the estimated timescale for bringing these production products to the marketplace?
The committee selected these criteria because (1) they address the wide range of technical and regulatory hurdles that will have to be overcome to demonstrate the establishment of a domestic capability to produce Mo-99; and (2) they can be assessed using publicly available information.
These committee-selected criteria are important for demonstrating the establishment of a domestic production capability, but they are by no means sufficient. Potential domestic suppliers also have to be able to successfully construct and operate Mo-99 production facilities and establish commercial relationships with customers. Their success in this regard will depend on a number of factors, including the availability of funding, previous experience in the medical isotope business, market timing, as well as the other economic considerations described in Section 4.5 of this chapter. These factors cannot be readily assessed with publicly available information.
For example, none of the projects described in Sections 4.1 or 4.2 of this chapter have previous experience in the medical isotope business. However, these projects can probably obtain the necessary experience through consulting or other types of contractual arrangements. The information needed to assess the efficacy of such arrangements is likely to be proprietary.
Project readiness for each of the projects described in Sections 4.1 and 4.2 is assessed by assigning a letter score (A-D) for each criterion, with higher scores indicating higher readiness levels. The scoring system is described in Table 4.2. A summary of the committee's readiness assessment for the NNSA-supported projects (see Section 4.1) is shown in Table 4.3; the assessment for the other projects (see Section 4.2) is shown in Table 4.4. Brief explanations for key readiness scores for these projects are provided below.
TABLE 4.3
Project Readiness for NNSA-Supported Domestic Mo-99 Production Projects.
TABLE 4.4
Project Readiness for Privately Funded Domestic Mo-99 Production Projects.
4.4.1. NorthStar Medical Radioisotopes
NorthStar's neutron capture project has the highest readiness level of all of the NNSA-supported projects. The technology has been demonstrated at commercial scale, production facilities are available, and regulatory approvals have been obtained or are in progress. The project can likely bring Mo-99 to market sometime after Canada stops production of Mo-99 at the end of October 2016 but before NRU shuts down permanently in March 2018. However, large-scale supply of Mo-99 to the market may not occur until after NRU shuts down permanently at the end of March 2018.
This project received lower readiness scores for the availability of enriched Mo-98 target material and the compatibility of the product in the supply chain because
- Enriched Mo-98 is available only from a single supplier in the Russian Federation, although two sources of supply may become available in the future.
- A special technetium generator system (RadioGenix Generating System) is required to use the low-specific-activity Mo-99 produced by neutron capture. The generator system occupies the same floor space as about four conventional Tc-99m generators. Most U.S. nuclear pharmacies have limited floor space, so they may have to reduce or eliminate their conventional generators to accommodate a RadioGenix system. Nuclear pharmacies might be reluctant to adopt a single new generator system that lacks an operational record. Additionally, the long elution time for the RadioGenix generator system (60-70 minute cycle time) compared to conventional technetium generators (about 5 minutes) may be a barrier to use by some nuclear pharmacies.
NorthStar's photon-induced transmutation project received lower readiness scores than the company's neutron capture project because the technology has not been demonstrated at commercial scales and production facilities are not yet available or licensed. NorthStar's photon-induced transmutation project is not likely to bring Mo-99 to market until after March 2018.
4.4.2. SHINE Medical Technologies
SHINE's accelerator-driven subcritical LEU assembly Mo-99 production project received lower readiness scores because the technology has not been demonstrated at commercial scales as an integrated system, production facilities are not yet available, and some, but not all, regulatory approvals have been obtained. This project is not likely to bring Mo-99 to market until 2019, long after NRU shutdown.
SHINE plans to purchase LEU from DOE and will have two options for doing so: (1) it can purchase LEU directly, or (2) it can lease LEU through DOE's Uranium Lease and Take-Back (ULTB) Program (see Sidebar 4.1). Leasing would allow SHINE to pay for the LEU as it is consumed, rather than paying for the entire cost of LEU up front. Additionally, DOE would retain title to the LEU and would dispose of SHINE's LEU-bearing wastes if there was no available commercial disposal pathway.
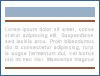
SIDEBAR 4.1
Uranium Lease and Take-Back Program.
4.4.3. General Atomics
GA's SGE process for recovering Mo-99 from irradiated targets has not been demonstrated at commercial scales. The facilities and target materials35 for production are available, but no regulatory approvals have been obtained. This project is not likely to bring Mo-99 to market until after March 2018.
4.4.4. Babcock & Wilcox and General Electric-Hitachi
Readiness scores for both of these projects have been included in Table 4.2 for completeness. As noted previously, however, both projects have been suspended, so they are unlikely to result in any new domestic Mo-99 production.
4.4.5. Other Potential Mo-99 Suppliers
All of the privately supported projects described in Table 4.4 are at a low state of readiness. Some of the production technologies have been demonstrated at commercial scales, but almost all of the projects lack the necessary facilities and regulatory approvals. It is unlikely that any of the projects will bring Mo-99 to market before March 2018, and many of these projects may never be completed.
Four of the projects (Coquí, Eden, Flibe, and NWMI) propose to produce Mo-99 by fission of LEU targets36 in reactors, a process that has been well demonstrated at commercial scale and is currently being used to produce Mo-99 in Australia and South Africa. LEU is available for sale or lease from DOE (see Sidebar 4.1). Even under the best of circumstances, however, it will take at least 5-7 years to design, construct, license, and commission the reactor and target processing facilities and commence Mo-99 production. Note particularly that
- The Coquí project has been working to construct a reactor and target processing facility for about 7 years (since 2009), but license and construction applications have not yet been submitted to the regulator.
- The Eden project proposes to use a new reactor design that has never been licensed. Reactor license and construction applications have not been submitted to the regulator.
- The Flibe project proposes to use a new reactor design with a liquid-fluoride thorium fuel system that, to the committee's knowledge, has not been technically demonstrated. Reactor license and construction applications have not been submitted to the regulator.
- The NWMI project will use a network of existing university reactors, but a new processing facility for Mo-99 recovery and purification and LEU recovery and recycle will need to be constructed and commissioned. In conjunction with the new processing facility, NWMI will have to construct and commission a facility for LEU target fabrication. Additional time will be required to develop, demonstrate, and license the process for recycling LEU from irradiated targets and fabricating new targets. Recovered LEU will need to be held for several months prior to being reused to allow sufficient time for medium-lived isotopes to decay. The company will also need to demonstrate that the Mo-99 produced from recycled targets can meet the FDA's requirements for commercial sale.
- The Niowave project to produce Mo-99 using a subcritical assembly faces many of the same challenges as the SHINE project.
4.5. ECONOMIC CONSIDERATIONS
Some of the domestic Mo-99 production projects described in Sections 4.1 and 4.2 employ technologies that may have significantly different cost structures than those for conventional fission-based Mo-99 production methods. In fact, some of these projects may be able to produce Mo-99 at lower costs than current global suppliers for several reasons, including the following:
- All domestic production will be non-HEU sourced; consequently, domestic Mo-99 suppliers do not have to bear the costs for eliminating HEU from production, unlike most existing global Mo-99 suppliers. (However, some of the costs of eliminating HEU from current production are being covered by NNSA; see Chapter 5.)
- Some domestic production development costs are being offset by NNSA through cooperative agreement funding. National laboratories are also providing NNSA-funded technical support to some projects, and regulators are providing fast-track regulatory approvals.
- Supply lines from potential domestic Mo-99 suppliers to domestic users are shorter than for existing global suppliers. This lowers domestic suppliers' costs because more Mo-99 can be delivered to users for the same unit of production.
- Domestic production in new facilities may be inherently less expensive than production by global suppliers in existing (and aging) reactor and target processing facilities.
The advantage of non-HEU sourcing will be short-lived, however. Some existing global suppliers already supply Mo-99 produced with LEU targets, and others are planning to do so starting in 2017 (see Chapter 3). Marginal production costs will rise for Mo-99 made from LEU targets, but some of the costs of conversion are one-time investments,37 which should have less effect on prices. The lower costs of production and shorter supply lines will continue to be advantageous for domestic suppliers long after conversion is completed.
Domestic suppliers will face many of the same economic and market barriers as global suppliers who plan to enter (e.g., Russia) or expand (e.g., ANSTO, Mallinckrodt, and NTP) their supplies of Mo-99 to the United States (see Chapter 3). At present, the main barrier to entry into the Mo-99 supply market is financial: the present low market prices for Mo-99 make it difficult to demonstrate the kind of return on investment that is needed to attract private financing to develop new domestic supplies. Current Mo-99 prices are low for several reasons:
- Historic government subsidies for Mo-99 production: most of the reactors and many of the facilities being used to produce Mo-99 were constructed with government funding and/or receive ongoing government support for operations.
- Mo-99 production capacity and marginal costs: Global suppliers currently have excess Mo-99 production capacity (see Chapter 3), and their marginal production (both irradiation and processing) costs within that excess are low.
- Oligopsony power38: Global Mo-99 suppliers and technetium generator suppliers are able to buy “products” (irradiation services and Mo-99, respectively) from multiple sellers. Competition among these buyers is limited—the top five Mo-99 suppliers and top four generator suppliers each supply 95 percent of their global market—by highly concentrated markets, so buyers can demand low prices as long as adequate product supplies are available. The technical and economic barriers to entry into these markets are formidable (see Chapter 3 and this chapter).
- Long-term purchasing agreement contracts: Mo-99, technetium generators, and Tc-99m radiopharmaceuticals are usually purchased under long-term proprietary contracts. These contracts usually limit the ability of sellers to increase prices.
- Pricing strategies: Technetium generator suppliers may use their generators as “loss leaders” to sell more profitable proprietary cold kits.
- Medical reimbursement policies: Reimbursement levels for medical procedures are set by government and private-payer insurance, usually based on past claim experience. The time lag between price changes and reimbursement levels can limit the ability of Mo-99/Tc-99m supply chain participants to raise prices over the short term.
The High Level Group on the Security of Supply of Medical Radioisotopes39 preference to allow market forces to govern Mo-99 supplies and prices also contributes to low Mo-99 prices because it reinforces many of the factors described above.
Under full-cost recovery (see Sidebar 2.4 in Chapter 2), governments would end their subsidies to reactors, forcing them to raise their prices for irradiation services. In a less price-constrained market, these irradiation-service price increases would cascade through the Mo-99/Tc-99m supply chain. However, other factors described above inhibit such cascading.
Even if new domestic suppliers are successful in entering the Mo-99 supply market, low Mo-99 prices may make it difficult for them to stay in business. Later domestic supplier entrants may have more difficulty succeeding because they will have to compete against these first entrants as well as then-existing global suppliers. Three existing global suppliers (ANSTO, Mallinckrodt, NTP) are expanding their capacities to supply Mo-99 in anticipation of Canada's exit from the market after October 2016 (see Chapter 3). Expanding supplies of Mo-99 will put further downward pressures on prices absent increased demand, likely making it difficult for new suppliers to gain a foothold in the market.
National governments, including the U.S. government, can provide incentives to push the market toward greater reliability and sustainability. Some actions are already being taken as described in Chapter 5.
4.6. FINDINGS AND RECOMMENDATIONS
FINDING 3A: The American Medical Isotopes Production Act of 2012 and financial support from the Department of Energy's National Nuclear Security Administration have stimulated private-sector efforts to establish U.S. domestic production of molybdenum-99 for medical use. However, no domestic commercial production will be established before Canada stops producing molybdenum-99 after October 2016. Potential domestic molybdenum-99 suppliers face technical, financial, regulatory, and market penetration challenges. The market challenges will likely increase after current global molybdenum-99 suppliers expand production.
As noted in Section 4.1, DOE-NNSA has entered into cooperative agreements with five U.S.-based companies to develop and demonstrate technologies for domestic production of Mo-99. Two of these companies (B&W and GEH) have suspended work on these agreements. Work by the other three companies (GA, NorthStar, and SHINE) to develop domestic production continues to progress toward commercial production.
NNSA initially set an aggressive goal for its cooperative agreement partners to demonstrate a capability to produce 3,000 6-day Ci of Mo-99 per week by the end of calendar year 2013. None of the partners were able to meet this goal. None of these companies will be producing any Mo-99 for commercial sale before Canada stops producing Mo-99 after October 2016; it is also unlikely any of these companies will be producing 3,000 6-day curies of Mo-99 per week on a routine basis before Canada permanently shuts down the NRU reactor in March 2018. One partner (NorthStar) may be able to initiate commercial production of Mo-99 by the end of 2016, but the quantities produced will likely be limited to a few hundred 6-day curies per week, at least initially. In addition, the Mo-99 produced by neutron capture is of low specific activity and cannot be used in conventional Tc-99m generators. NorthStar has developed a new technetium generator system, the RadioGenix Tc-99m Generating System, to utilize low-specific-activity Mo-99.
The potential domestic Mo-99 suppliers described in Sections 4.1 and 4.2 face a variety of technical, financial, regulatory, and market challenges to establishing domestic Mo-99 production. These challenges are described in Section 4.4. The first domestic Mo-99 suppliers to enter the market are likely to have the best chance of survival. Subsequent domestic supplier entrants will have to compete against these first entrants as well as then-existing global suppliers, three of whom are expanding their production capacities. The expanding supply of Mo-99 to the market will put a further downward pressure on prices absent increased demand.
Three of the potential domestic projects (NorthStar, GA, and Northwest) will utilize a reactor that is fueled with HEU (MURR reactor) to produce Mo-99. The University of Missouri has committed to converting its reactor to LEU fuel when such fuel becomes available.40 But the reactor will continue to operate with HEU fuel until suitable LEU fuel is available.
FINDING 3B: There is currently no domestic production of iodine-131 or xenon-133, but U.S. organizations are developing the capability to produce one or both of these isotopes.
At present, Canada supplies most of the I-131 and Xe-133 used in the United States, and IRE recently began supplying Xe-133 to the United States under an arrangement with Lantheus. Plans by domestic organizations to produce I-131 and Xe-133 are described in Section 4.3. MURR has already received a license amendment from the NRC to produce I-131 by irradiating tellurium targets and is currently testing its process. Other potential domestic suppliers may recover I-131 and Xe-133 as part of their Mo-99 production processes.
APPENDIX 4A. FDA Regulatory Submission Process
This appendix describes the steps in obtaining regulatory approval from the Food and Drug Administration (FDA) for the commercial sale of Mo-99 and Tc-99m intended for medical use. These regulatory approval steps are illustrated schematically in Figure 4A.1.

FIGURE 4A.1
FDA approval pathways for Mo-99 and Tc-99m. The flow sheet shows three different approval pathways and the required information and application type for each pathway. FIGURE 4A.1 Notes
4A.1. DRUG MASTER FILE (DMF)
A DMF is a submission to FDA that provides confidential information about facilities, processes, or articles used in the manufacturing, processing, packaging, and storing of drugs administered to humans (Niazi, 2009). The submission of a DMF is made at the discretion of the application holder; it is not required by law or FDA regulation. Although a DMF is not a regulatory requirement, it can facilitate the regulatory approval process for an Investigational New Drug (IND) Application, a New Drug Application (NDA), and an Abbreviated New Drug Application (ANDA), if it provides information in support of the quality and safety of the drug. A DMF may be filed for how Mo-99 is produced. A DMF may be amended when this information changes: for example, when converting target material from HEU to LEU. The DMF can be reviewed by FDA to support new drug applications.41
4A.2. NEW DRUG APPLICATION (NDA)
An NDA is a submission to the FDA that allows drug sponsors to formally propose that the agency approve a new drug for sale and marketing in the United States. The NDA includes data gathered during animal studies and human clinical trials of an IND application (see Section 4A.3 for a description).42
There are three FDA drug approval pathways described in different sections of the Federal Food, Drug, and Cosmetic Act of 1984:
- 1.
The 505(b)(1) approval pathway is for a new drug and includes human subject research to demonstrate safety and efficacy. Tc-99m pertechnetate is an approved drug, so this pathway would likely not be used for a new drug involving Tc-99m use.
- 2.
The 505(b)(2) approval pathway is for a previously approved drug for which safety and efficacy have been approved by the FDA. For Tc-99m pertechnetate, the manufacturing process is often different. This is the likely pathway for NDAs involving Tc-99m use.
- 3.
The 505(j) pathway is for a drug that must be equivalent to the original reference listed drug (RLD), including ingredients, manufacturing, and dosing.
The provisions of 505(b)(2) were created, in part, to help avoid unnecessary duplication of studies already performed on a previously approved drug—an RLD. As a result, the 505(b)(2) pathway can result in a more economical and faster route to approval compared to a traditional development pathway [such as 505(b)(1)].
4A.2.1. Supplemental NDA (sNDA)
A sNDA is the vehicle for companies to change a label, market a new dosage or strength of a drug, or change the way they manufacture a drug. There are four reporting categories of sNDA (FDA, 2004):
- 1.
Major change: Prior Approval Supplement
- 2.
Moderate change: Supplement–Changes Being Effected in 30 Days
- 3.
Moderate change: Supplement–Changes Being Effected
- 4.
Minor change: to be noted in the next annual report
4A2.2. Abbreviated NDA (ANDA)
An ANDA is a submission to the FDA for approval of a generic drug that performs in the same way as a previously approved drug (an RLD).43 The submission is termed abbreviated because it generally does not require the inclusion of animal studies and human clinical trial data to demonstrate the drug's safety and effectiveness.
A generic drug product is one that is comparable to its RLD in active ingredient(s), dosage form, strength, route of administration, and (with certain exceptions) condition of use. The inactive ingredients for generic drug products are allowed to differ from those of the RLD only in preservative, buffer, and/or antioxidant. Additional differences are generally not permitted.44
As of August 12, 2015, no ANDA has been submitted to the FDA for Tc-99m generator (for the production of sodium pertechnetate Tc-99m injection) (Duffy, 2015).
4A.3. INVESTIGATIONAL NEW DRUG (IND)
An IND application is submitted to FDA to seek permission to test a new drug (or biologic) in humans. This route is not typically required for sodium pertechnetate Tc-99m injection obtained from Tc-99m generator with Mo-99 produced from either HEU or LEU processes unless a new clinical indication for sodium pertechnetate Tc-99m injection is required to be studied.
4A.4. 2016 USER FEES
The Prescription Drug User Fee Act was enacted in 1992 and is renewed every 5 years. It authorizes FDA to collect fees from companies that submit applications or supporting information to applications for production of human drug and biological products. These fees have played an important role in expediting the drug approval process.45 The 2016 user fees are shown below.
Submission Fees | |
NDA full fee (clinical data) | $2,374,200 |
NDA ½ fee (no clinical data) | $1,187,100 |
ANDA | $76 030 |
CMC Supplements (NDA) | No Fee |
CMC Supplements (ANDA) | $29,370 |
Supplement (with clinical data) | $1,187,100 |
DMF (supporting NDA) | No fee |
DMF (supporting ANDA) | $42,170 |
Establish and Product Fees | |
Establishments (NDA product) | $585,200 |
Products (NDA product) | $110,370 |
Establishment (ANDA domestic) | $243,903 |
Establishment (ANDA foreign) | $258,905 |
Establishment (DMF domestic) | $40,867 |
Establishment (DMF foreign) | $55,867 |
User fees can be waived or reduced in the circumstances described in section 736(d) of the Federal Food, Drug, and Cosmetic Act:
- A waiver or reduction in fees is necessary to protect public health.
- The fees present a significant barrier to innovation due to limited resources or other circumstances.
- The fees exceed the anticipated costs of FDA's review of the new drug applications.
For a small business,46 FDA will waive the application fee for its first human drug application. However, there are no small business waivers for product or establishment fees.47
Footnotes
- 1
When the present report was being finalized for publication.
- 2
The B&W Phase 1 cooperative agreement was awarded for a total NNSA contribution of $9.1M. Due to a reduction in B&W's scope, the resulting total of the NNSA contribution to the Phase 1 cooperative agreement was $6.1M.
- 3
NNSA published a Funding Opportunity Announcement (FOA) in March 2010 to solicit proposals from private-sector companies. It was implemented under 10 CFR 600 Subpart H, Assistance Regulations. The FOA closed in June 2010.
- 4
Rilla Hamilton, DOE-NNSA, written communication, June 8, 2016.
- 5
NorthStar was founded in 2006 and is based in Madison, Wisconsin.
- 6
At present, the cost of enriched Mo-100 is a few hundred to a thousand U.S. dollars per gram in kilogram quantities. The primary supplier is Isoflex, a U.S-owned and operated company that sole-sources Russian-separated isotopes.
- 7
The reactor at MURR is a 10 megawatt (MW), HEU-fueled, pool-type, light-water-cooled reactor. The reactor is described in NRC (2009). NorthStar has an agreement to use the MURR reactor through 2019.
- 8
NorthStar is purchasing enriched Mo-98 from the chemical plant at Krasnoyarsk, Russian Federation, and is in discussion with two additional potential suppliers.
- 9
The operating license for the reactor at MURR already allows for the irradiation of Mo-98 targets to produce Mo-99.
- 10
NorthStar works under MURR's U.S. Nuclear Regulatory Commission (NRC) license.
- 11
The ABEC resin was developed in the 1990s and became the basis for the Automated Radionuclide Separation (ARS) system developed in the early 2000s. NorthStar bought the technology in 2005 and has continued to develop the system based on feedback from regulators and focus groups. See Le (2014).
- 12
The original cooperative agreement holder for this project was Morgridge Institute for Research. SHINE is now the primary cooperative agreement holder.
- 13
The company was founded in 2010 and is based in Monona, Wisconsin, a suburb of Madison.
- 14
- 15
That is, uranium-fission reactions in the assembly do not become self-sustaining.
- 16
Information about the quantities and concentrations of target solutions is proprietary.
- 17
See Proceedings of the 22nd International Meeting on Reduced Enrichment for Research and Test Reactors, Budapest, Hungary, October 3−8, 1999.
- 18
- 19
- 20
- 21
GA is located in San Diego, California. It has more than 50 years of experience in reactor and fuel target design.
- 22
The partnership was announced in February 2015. See General Atomics (2015).
- 23
The designs of the target rods and housings and supporting performance analyses are proprietary.
- 24
Nordion did not reveal the intended use for these other fission products.
- 25
Additional companies may be developing capabilities to produce Mo-99 and associated medical isotopes with or without publicly announcing their plans.
- 26
Coquí's original plan was to build one reactor at a 25-acre plot in Alachua, Florida. All announcements by the company made in 2015 referred to facilities at that location.
- 27
A company representative characterized these estimates as “conservative and fully account[ing] for cooling, processing, and shipping times to deliver desired quantities of 6-day curies to the customer” (Richard Coats, Eden Radioisotopes LLC, written communication, July 30, 2016).
- 28
- 29
- 30
A similar approach has been proposed by IBA and AMIC using lower energy electron beams. See Stevenson and Schenter (2010) and Stichelbaut and Jongen (2011).
- 31
- 32
- 33
Greg Piefer, SHINE, written communication, March 7, 2016.
- 34
Phil Larabie, Nordion, written communication, March 14, 2016.
- 35
GA would purchase or lease LEU from DOE.
- 36
These companies would also purchase or lease LEU from DOE.
- 37
As noted previously, some of these costs are being covered by NNSA.
- 38
A type of market in which buyers of a product hold pricing power because they are small in number compared to the number of sellers of that product.
- 39
This group operates under the Organisation for Economic Co-operation and Development's Nuclear Energy Agency (OECD-NEA). See Chapter 5.
- 40
The U.S. government is working to develop LEU fuel for use in the MURR reactor and other high-performance research reactors in the United States. However, it will be at least a decade before such fuel is available. See NASEM (2016).
- 43
- 44
- 45
- 41
- 42
See the Federal Food, Drug, and Cosmetic Act of 1984 here: http://www
.fda.gov/RegulatoryInformation /Legislation/FederalFoodDrugandCosmeticActFDCAct /FDCActChapterVDrugsandDevices/. - 46
The Act defines a small business as a business that has fewer than 500 employees, including employees of affiliates.
- 47
Product and establishment fees are defined here: http://www
.fda.gov/Drugs /DevelopmentApprovalProcess /SmallBusinessAssistance /ucm069943.htm#P62_3931.
- Progress Toward Establishing Domestic Production of Molybdenum-99 and Associated...Progress Toward Establishing Domestic Production of Molybdenum-99 and Associated Medical Isotopes - Molybdenum-99 for Medical Imaging
Your browsing activity is empty.
Activity recording is turned off.
See more...