NCBI Bookshelf. A service of the National Library of Medicine, National Institutes of Health.
Committee on Communicating Chemistry in Informal Settings; Board on Chemical Sciences and Technology; Division on Earth and Life Studies; Board on Science Education; Division of Behavioral and Social Sciences and Education; National Academies of Sciences, Engineering, and Medicine. Effective Chemistry Communication in Informal Environments. Washington (DC): National Academies Press (US); 2016 Aug 19.

Effective Chemistry Communication in Informal Environments.
Show details
Michael Faraday's 1827 lecture series on the chemical history of a candle, intended for nonscientist participants, is an early example of a chemist's desire to bring an understanding of chemistry to the general public.1 The 2011 observance of an International Year of Chemistry, which was planned to excite young people about chemistry and raise awareness of the field's vital role in many issues, is a more recent example. Both examples reflect the goals for communication with the public that are discussed in this report.2
A growing body of evidence indicates that people do learn about science outside of school, in a wide range of informal, free-choice settings, and that these experiences are an increasingly important way for the public to learn (Falk and Dierking, 2010; NRC, 2009). However, these educational opportunities vary so much in terms of who provides them, venue, learning goals, content, and approach that assessing them and their effects has been difficult. The committee researched this sort of education, hoping to identify goals to improve it.
The committee explored several areas: The literature on learning science in informal settings includes findings from the science of learning and relevant research on classroom teaching and learning. Research on communications encompasses several fields. The commissioned landscape study provided data specific to chemistry. A thorough review of the relevant research was beyond the project's scope, but the report summarizes highlights from education and communications research, discusses particular challenges for chemistry, and identifies ideas for improving communication in chemistry.
INSIGHTS FROM RESEARCH ON INFORMAL SCIENCE LEARNING
A 2009 National Research Council (NRC) report, Learning Science in Informal Environments, surveyed the research on informal science education and is an invaluable starting point for insights into communicating chemistry in informal settings. The report gathers research from the fields of developmental and cognitive science, science education, museum research and evaluation, social science, and the science disciplines. It provides insight relevant to the venues in which people learn science outside of school, the nature of this learning, and ways it might be improved (NRC, 2009). Also invaluable is the follow-up report, Surrounded by Science: Learning Science in Informal Environments (NRC, 2010), which translates the findings in the 2009 report into concrete examples of effective approaches for practitioners in informal science settings, such as media, libraries, after-school programs, museums, aquariums, and zoos.
The 2009 NRC report characterizes informal science learning as predominantly “learner-motivated, guided by learner interests, voluntary, personal, ongoing, contextually relevant, collaborative, nonlinear, and open-ended” (NRC, 2009, p. 11). This description encompasses a wide range of activities that may take place at home or in public spaces of many kinds. The report notes that the research relevant to this kind of learning includes the study of institutions and the history of public learning (history and sociology); the study of how learning takes place and what promotes it (neuropsychology, psychology, education, and anthropology); and the study of design and other factors that affect visitors' experiences in informal learning settings, such as museums, zoos, and libraries. It also includes the study of audience responses to media stories about science. A significant base of knowledge about informal learning has developed through nonacademic research, such as the analysis of visitors' behavior and opinions or other market research approaches. Front-end, formative, and summative evaluation (see Chapter 5) have been used for years in exhibit development in museums and also inform this report.
More recently, there have been increased efforts to measure the results of informal education, to support decisions about the resources they require. At the same time, new technologies continue to expand the possibilities for informal learning, but also for the dissemination of misinformation, which increases the challenge to consumers seeking to understand the science. It has not been easy for researchers of informal learning to keep up with fast-paced developments or to track the relevant research from other social science disicplines.
Because there are many types of research relevant to informal science learning and many types of such learning, the 2009 report explores several ways to frame the landscape. These frameworks complement one another, and they are summarized in the following because they encompass the findings that are most relevant for the committee's task to develop a framework for communicating chemistry and to identify key areas for future research.
An Ecological Framework for Understanding Learning
The 2009 NRC report describes “An Ecological Framework for Learning Across Places and Pursuits” (NRC, 2009, pp. 31-41). The framework is based on insights from three fields: cognitive science, behavioral psychology, and sociocultural research on the factors that influence development and learning. Drawing on these fields, the report's authors stress the importance of attending to “three cross-cutting aspects of learning that are evident in all learning processes: people, places, and cultures” (NRC, 2009, pp. 31-32).
Ecologic, as defined in that report, refers to the relationships between individuals and their physical and social environments with particular attention to relationships that support learning. Just as biological systems can be understood as complex interactions between multiple natural forces, so can learning be understood as the complex interactions of multifaceted sociocultural contexts. In addition, the authors emphasize that learning about science and the practice of science happens naturally throughout the life span, and that this sort of learning is “inherently cultural” (NRC, 2009, p. 42). Thus, scientists who engage in informal learning activities should think about what people already know and their interests, concerns, and motivations for participating in an informal science learning event.
The 2009 NRC report also notes that for participants to identify with the scientists, participants must gain an understanding of the ways scientists approach their work and of the reality that scientific knowledge “is continually being extended, refined, and revised by the community of scientists” (p. 42). Scientists must understand the cultural norms and modes of expression of science in general and of their particular disciplines. The experiences and perspectives that individuals bring to science will shape their inquiry and their understanding. The ecological approach is useful in considering how to develop informal learning opportunities to meet the needs of participants by incorporating cultural experiences and everyday language, as well as by engaging participants in development, implementation, and other roles.
Goals for Informal Learning
Another model of the landscape of informal learning considers the learning goals that are pursued, which overlap with but also differ from goals for school-based science learning. For example, informal learners are motivated by their work and leisure activities to seek out new skills and information throughout their lives, and they respond to opportunities they encounter in social and public settings. The authors identify six interrelated strands, or dimensions of learning, that characterize informal learning, noting that learning can occur across individual or multiple strands. All six need not be engaged for learning to occur. The strands are presented here along with guidance on how to develop events that promote learning across them.
The authors propose that learners who engage successfully with science in informal environments may do the following (NRC, 2009, p. 43):
- Strand 1: Experience excitement, interest, and motivation to learn about phenomena in the natural and physical world.
- Strand 2: Come to generate, understand, remember, and use concepts, explanations, arguments, models, and facts related to science.
- Strand 3: Manipulate, test, explore, predict, question, observe, and make sense of the natural and physical world.
- Strand 4: Reflect on science as a way of knowing; on processes, concepts, and institutions of science; and on their own process of learning about phenomena.
- Strand 5: Participate in scientific activities and learning practices with others, using scientific language and tools.
- Strand 6: Think about themselves as science learners and develop an identity as someone who knows about, uses, and sometimes contributes to science.
The six strands provide a structure for understanding how people of different ages build on their experiences to learn science. The strands are not unique to informal environments but might also occur in formal education environments. However, informal environments, the report notes, are designed to encourage learners to explore and to satisfy their curiosity without performance expectations. In such settings, learners are likely to experience positive emotions such as excitement, wonder, and surprise, and also to pursue learning that is meaningful to them—both factors that are associated with learning and with retaining what is learned (NRC, 2009).
Informal learning offers an opportunity to ignite enthusiasm about chemistry, and the 2010 NRC report identifies strategies that take advantage of the way emotional responses can stimulate learning (NRC, 2010). For example, one model developed for the design of museum exhibits suggests that they should have six elements (NRC, 2010, p. 83):
- Curiosity—the visitor is surprised and intrigued,
- Confidence—the visitor has a sense of competence,
- Challenge—the visitor perceives that there is something to work toward,
- Control—the visitor has a sense of self-determination and control,
- Play—the visitor experiences sensory enjoyment and playfulness, and
- Communication—the visitor engages in meaningful social interaction.
The 2010 report provides detailed descriptions of activities that engage informal learners in many ways, highlighting the value of interactivity, opportunities for long-term relationships with science activities, and connecting scientific work to everyday life or to environmental and other social issues.
Strand 2, for chemistry, would include fundamentals such as the structure of atoms and properties of matter, as well as areas of application, such as cycles of matter and energy transfer in ecosystems. Research suggests that many of these concepts can be difficult for nonspecialist participants, an issue we discuss in greater detail below. Hands-on informal learning activities, such as making slime or playing with magic sand,3 are often used to introduce young children to chemistry and Strand 3, to manipulate, test, and in other ways explore the natural and physical world. Strand 4, reflecting on science as a way of knowing, may be more effective for reaching adult participants and would encompass learners' awareness of their own process for learning. It would also include their experiences with the chemistry of everyday life, as observed in food and cooking, health and medicine, gardening and agriculture, and use of household products such as cosmetics or cleaners. Strands 3 and 4 both reflect the importance of considering what is relevant and accessible to the participants. For both children and adults, learning about ways of knowing in chemistry would encompass not only how chemists do their work but also how engineering relates to the work of chemistry.4
One way to address Strand 5 is through public participation in scientific research, sometimes called citizen science. This participation may be long term, such as at summer camp or after-school programs, or in cases when children or adults contribute to actual research over time. Such events foster knowledge of the process of science, improve understanding of the relevance of science to everyday life and social issues, and develop relationships between scientists and the public (Grunwald Associates and Education Development Center, 2013). Scientific events in which the public can be involved include (Bonney et al., 2009, p. 11)
- “choosing or defining questions for study;
- gathering information and resources;
- developing explanations (hypotheses) about possible answers to questions;
- designing data collection methodologies (both experimental and observational);
- collecting or analyzing data;
- interpreting data and drawing conclusions;
- disseminating conclusions; and
- discussing results and asking new questions.”
Such events contribute to development along Strand 6 as well. Encouraging individuals to develop a sense of identity as a scientist or chemist is important not only for workforce development but also for helping all learners engage in critical, science-based thinking about their activities and world.
At present, there may not be adequate opportunities for public involvement in chemistry activities that are rich and varied enough to support the development described in the six strands. In two polls conducted by Carlton Research Company for Research!America in May 2010 and March 2011, a substantial percentage of respondents said that they could not name a living scientist. A 1990 survey of science museums and science centers found that fewer than 30 percent had chemistry exhibits (Zare, 1996), and, more recently, research showed that chemistry continues to be one of the least represented disciplines in science museums (Silberman et al., 2004).
Environments for Informal Learning
The 2009 NRC report identified the three primary settings in which informal science learning takes place as a way to consider the characteristics that make it effective in different circumstances.
- Everyday and family life: People learn throughout their lives while participating in the activities of daily life, such as hobbies, outdoor excursions, technology use, or meal planning and preparation. People naturally develop expertise in areas that are important to them. These experiences cannot substitute for more structured opportunities but can be valuable in sparking interest in science and complementing learning that takes place elsewhere.
- Designed environments: Sites that are designed to enhance learning include science museums, zoos, nature centers, and visitor centers. These sites are designed to serve visitors who are there by choice and to allow them to pursue their own interests. Most of the learning is expected to be short in duration, though some long-term effects may occur. Growing attention is on ways to build on such short experiences. Different designed environments, such as museums and online media, may be linked if they share science learning goals, and this can facilitate learning along more of the strands for participants.
- Programs: Programs designed for children and adults include after-school programs, adult learning and continuing education programs, programs in retirement communities or nursing homes, and learning vacations. Like events offered in designed spaces, such programs primarily serve people who choose to participate, and many are intended to benefit people in need of support, such as disadvantaged children. These programs have not been as thoroughly researched as other areas of informal learning but hold potential, particularly for out-of-school programming for young people and for serving an aging population.
The 2009 NRC report also identified “media” as a crosscutting theme. Media activities occur in all venues, with newspapers, magazines, websites, television shows, radio shows, podcasts, and more being used both casually and purposefully at home, in designed spaces, and in youth and adult programming. These media activities include journalism focused on “the news” and entertainment-focused media like Breaking Bad, Mythbusters, and video games, among others.
Assessing Outcomes
The diverse universe of informal science learning yields diverse outcomes, and thus there are multiple ways to assess them. Learning Science in Informal Environments (NRC, 2009) notes that learning outcomes include not only gains in content knowledge and conceptual understanding but also changes in the learners' perceptions about and attitudes toward science and science learning. Some informal learning is very short term, but some can have lasting impact. Learning may occur on a group or even a community level. For example, a science class may improve its capacity to collaborate in solving a science problem as a result of a field trip, or a community might build its capacity to pursue an environmental goal, such as recycling, as a result of educational programming.
Valid assessment of results, the authors note, needs to reflect the goals of a learning opportunity and must not undermine those goals, for example by imposing stressful performance expectations on an experience that is designed to be fun. Accurately identifying the skills, knowledge, and concepts that event participants could develop is complicated, in part because the participants bring diverse qualities to the event—qualities generally unknown by the event's planners. Informal science learning is intended to be responsive to a learner's interests and capabilities, and thus the outcomes may be unexpected and surprising.
Insights from Research on Communication
Many social science disciplines contribute to understanding communication, including psychology, sociology, political science, risk communication, media studies, public relations, decision science, social marketing research, and the science of communication itself. A comprehensive review of the relevant literature from these diverse fields is beyond the scope of this report, but much valuable work has been done to apply communications research to science communication, education, and policy.5 The committee benefited from work on the science of science communication, which has begun to synthesize insights from various fields. Two of the Sackler Colloquia of the National Academy of Sciences provided a valuable overview of research and perspectives on the science of science communication (NAS, 2013; NRC, 2014b). The committee also explored data on public perceptions of science, scientists, and science institutions for insights to help bridge gaps between professional science and everyday communication.
Goals for Communication
The academic field of communication has not identified a set of formalized strands for practitioners. Rather, science communication scholarship acknowledges the wide range of goals of practitioners, from science learning to providing science information to addressing societal challenges to building a stronger science-public interface. For example, the goal of a chemistry communication event could be to build trust with participants, to stimulate a discussion about the chemistry of cooking and learn from participants what interests them, or to build media attention about an area of advancing research.
Baruch Fischoff, a professor of social and decision sciences, describes four tasks for those communicating about science (see Box 4-1). Fischoff emphasizes that communication events that do not reflect the needs, interests, and values of the participants will be unlikely to engage them as intended, if at all. In addition, communication events should be evaluated to avoid assumptions about why an event was unsuccessful, and to avoid perpetuating myths such as that the public does not understand science (Fischoff, 2013).
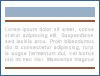
BOX 4-1
Four Communication Tasks.
Public Engagement
For successful public engagement, a chemist needs more than the content to be shared and an event plan. In designing an activity, a communicator must be explicit about what will be addressed, particularly at the conceptual level, as this will frame the activity and aid in the creation of appropriate materials to support the experience (Hmelo-Silver et al., 2007; Lidar et al., 2006; Nathan et al., 2007; Rappoport and Ashkenazi, 2008; Stieff et al., 2013). However, relying on intuition about which messages or engagement mechanisms will be most effective is not likely to succeed (NRC, 2014a). Science communicators must understand what participants hope to gain from the experience and what they bring to it. Participants might, for example, be filling a gap in their understanding of the science topic, might have misconceptions about it, or might have a need for specific information (Fischoff, 2013; NRC, 2009, 2014a).
Science communicators should understand the ideas, beliefs, and perspectives that participants bring, especially with a topic that raises social or political issues about which people disagree (Fischoff, 2013; NRC, 2009, 2014a). In informal settings, where scientists may not know the participants or have an opportunity to build a relationship, understanding what participants bring may be more difficult than in a formal classroom (NRC, 2009). There are ways to elicit a sense of participants' understanding, though strategies for classroom teachers have been studied more than strategies for informal settings (e.g., Ambrose et al., 2010; Fisher, 2004). Research does show that giving participants the opportunity to exercise control over the goals of a particular experience helps them learn (Bandura, 1986, 1997).
Environments for Communication
The scientific community (individuals and their institutions) primarily communicates with colleagues about its work through peer-reviewed journal publications (Harley, 2013). Many scientists also share their research with journalists, who publish news articles about it (Peters, 2013). However, research publications and news articles do not enable scientists to engage with the public. In addition, the range of goals of communicating science with the public, from sharing excitement about science to building trust to informing policy decisions, necessitates a range of communication tools and strategies. There are many communication technologies through which science content can be accessed—science programming on television, newspaper and magazine articles, science fiction writing, and the Internet (e.g., Wikipedia), to name a few—and interactive technology is now omnipresent in designed spaces.
The Internet, in particular, is creating more opportunity for scientists to interact with participants. There are an increasing number of online communities devoted to science interests (Peters et al., 2014). For example, Reddit6 is a popular moderated online forum in which a chemist can participate in an Ask Me Anything—scheduled forum time to answer questions and interact with people interested in science. Another example is #scistuchat, an informal but themed monthly Twitter discussion, created by a high school science teacher so that students can interact with scientists outside of school.7 Another popular online venue is ResearchBlogging.org, a website where scientists post information about peer-reviewed work for discussion both with peers and members of the public (Shema et al., 2012). Evidence suggests that social media may amplify a researcher's scientific impact (Liang et al., 2014). However, very little research has been published on scientists' attitudes toward and use of online and social media, or the effectiveness of public engagement on science topics through social media (SCIMEP, 2015).
LINKING INFORMAL SCIENCE LEARNING AND COMMUNICATION
A Communication Model for Informal Learning Settings
There are many models that describe objectives for communicators, many of which share features. The committee invited Dr. Katherine Rowan to summarize key implications of communications research for informal science education, and she presented one such model, called CAUSE, to organize these implications. This model is described here as an example of the objectives often called for in the communications literature. The acronym CAUSE represents five objectives for communicators, who need to
- earn the Confidence of those with whom they are communicating;
- create Awareness of benefits or dangers;
- deepen the Understanding;
- help participants gain Satisfaction with possible solutions; and
- support the Enactment of solutions.
Dr. Rowan explained that to earn someone's confidence, research indicates that it is important to listen to their views and concerns, show them respect, and create conditions that encourage them to ask questions. To deepen people's understanding, communications experts find it necessary to explain ideas that are often misunderstood, which may include terms (e.g., polymer), complexities that are difficult to envision (e.g., how carbon dioxide traps heat), or counterintuitive concepts (e.g., how a naturally occurring gas such as carbon dioxide can be harmful). When explaining the meanings of key terms, communicators endeavor to convey what the terms do not mean as well as what they do mean—by pointing out, for example, that “chemical” does not mean artificial or human made. It is also useful to provide a range of examples: for example, polymers are giant molecules that can be natural (rubber, starch, or DNA) or made by scientists (nylon or polyethylene).
Another strategy to deepen a participant's understanding is to use analogies or visual representations. A familiar analogy used to explain how carbon dioxide (CO2) traps heat on Earth, for example, is the greenhouse: CO2 allows light energy from the sun to enter Earth's atmosphere, and, much as a greenhouse does, it traps some of the heat that subsequently returns from Earth. When presenting to the committee, Dr. Rowan used Figure 4-1 as an illustration of this concept.

FIGURE 4-1
A visual representation of the greenhouse effect. SOURCE: State of Washington, Department of Ecology: http://www.ecy.wa.gov/climatechange/FAQ.htm [accessed June 12, 2015].
It is also important for communicators to consider “lay theories”—possibly erroneous understandings that people have about familiar aspects of life, such as weather or disease. The communicator can acknowledge the apparent reasonableness of a lay theory, noting, for example, that “most people have not seen molecules so it is understandable if they wonder whether they exist.” The next step is to generate dissatisfaction with the lay theory and then explain the current scientific consensus.
Public Perceptions of Science and Scientists
The challenge of conveying science in informal settings may be complicated by public perceptions of scientists and their research institutions. A 2012 survey conducted by the National Science Board (NSB) showed that the American public has a high regard for scientists in general. However, less than half of Americans reported that they have an excellent or good understanding of how scientists investigate and analyze phenomena (NSB, 2014; see also results from the Pew Research Center, 2013). For example, respondents to the 2012 NSB survey displayed limited understanding of scientific experimentation and the concept of controlling for variables. Data from the General Social Survey (GSS)8 on public confidence in institutions demonstrates that public confidence in the scientific community has remained stable since 1973 and is consistently greater than American confidence in 10 of the other 12 institutions included in the survey, including the Supreme Court, education, and organized religion (Smith and Son, 2013). However, on average the percentage of the public with “only some” or “hardly any” confidence in the scientific community is greater than 50 percent.
Institutional affiliations may influence how publics perceive science and scientists. For example, a public opinion study in Australia found that members of the public are less supportive of stem cell research if it is conducted within a private company than if the research is conducted by a publicly funded (e.g., academic) institution (Critchley, 2008). Similarly, university scientists are the most trusted source of information on the risks and benefits of nanotechnology, compared with industry scientists, regulatory agencies, environmental organizations, and other groups that communicate about science (Scheufele et al., 2009). That finding is particularly important for chemists who work in industry; participant reactions to industry-affiliated chemists may be due to their perceptions of chemical companies rather than the field of chemistry.
Social identity (e.g., culture, political ideology, education level, and religious beliefs) influences public perceptions of science and scientists, perceptions which can be dynamic, and the influence of social identity can vary from topic to topic (Kahan et al., 2011). For example, highly religious and conservative Americans are the most concerned about the risks of synthetic biology (Kahan et al., 2009) yet the least concerned about the risks of global warming (Kahan et al., 2012). More than half of the respondents to a 2009 survey conducted by the Pew Research Center believe that science and religion are often in conflict (Pew Research Center, 2009). Social identity plays a role in perceptions of a wide range of topics such as hydraulic fracturing (Boudet et al., 2014), nanotechnology (Scheufele et al., 2009), and stem cell research (Nisbet and Markowitz, 2014), among others.
Research also suggests that responses to science information and ideas about which authorities to trust depend in part on confirmation bias, the cognitive phenomenon that people are predisposed to accept information that accords with what they already believe and to be more skeptical of information or sources that challenge their beliefs, and motivated reasoning, an unconscious tendency to process information in a way that fits a preconceived outcome or goal (Haidt, 2013; Kahan et al., 2011; Kunda, 1990; Scheufele, 2006).
Because of the breadth and complexity of factors that influence people's perceptions of science, building trust between chemists and participants is critical for science communication and learning in informal settings. Although there is no universal definition of trust, public relations and communications scholars generally agree that trust involves people's confidence in and willingness to open themselves to one another (Chryssochoidis et al., 2009; Hon and Grunig, 1999; Resnick, 2011). In the Guidelines for Measuring Relationships in Public Relations, trust is described as having three primary dimensions: confidence, the belief that an entity has the ability to do what they say they will do; integrity, the belief that an entity is fair and just; and dependability, the belief that an entity will do what they say (Hon and Grunig, 1999). In addition, whether or not someone has trust in someone or something is influenced by perception of the information, of the information source (e.g., scientists or science institutions), and of risk and by the person's own personality and sociocultural characteristics (Chryssochoidis et al., 2009).
Regarding the information source, research on social relationships demonstrates that people are most likely to trust those they believe are competent (perception of capability) and warm (perception of intent; Fiske and Dupree, 2014), two characteristics that reflect the three dimensions of trust described above. People often have preconceptions about how competent or warm different groups are. Scientists, researchers, and engineers are viewed consistently as highly competent but in the middle range for warmth. They fall just within a clustered group that elicits mixed emotions of admiration and resentment, and so their intentions may not always be trusted. For example, survey respondents recruited through Amazon's Mechanical Turk system were asked about climate scientists and answered that “scientists might lie about statistics, complicate simple stories, feel themselves superior to nonscientists, pursue a liberal agenda, or provoke and hurt big corporations,” or that they might slant their research to obtain funding (NRC, 2014b, p. 10). Hence, building trust requires not just warmth but attention to research integrity, openness about political, financial, institutional or other affiliations, and transparency about the motivations for communicating about chemistry.
Regarding information and risk, scientists are respected for their role in education and protecting the environment and may be most trusted when they communicate about scientific information, as opposed to policy proposals (NRC, 2014b). The types of science that are most likely to seem relevant and interesting to participants, however, are likely to relate to challenging policy questions. And, scientists face the challenge of accurately and clearly communicating the degree of uncertainty associated with results (NRC, 2014b). The nature and degree of uncertainty might be important when considering policy options, but complete understanding might require a sophisticated understanding of the scientific methods in question, and science communicators are sometimes cautious, for fear of unintentionally creating misunderstanding.
Web-Based Communication
A significant amount of informal science learning takes place through broadcast, print, and digital media, and the possibilities in this area are evolving quickly. Unfortunately, research on science communication in informal environments has not kept pace with the rapid rise of online and social media as a means of communication and learning about science, including chemistry. Educational television has been the most studied of these media and has been found effective in supporting learning (NRC, 2009). However, the Internet is now the primary source of information about science and technology for most Americans (NSB, 2014). Because Web-based platforms have become such a prevalent means of informal science communication, the committee asked Ayelet Baram-Tsabari, a professor of biology education and science communication at Technion–Israel Institute of Technology, to summarize the recent research. Baram-Tsabari explained that in Western countries, the Internet has become the primary source of science-related information, with 60 percent of the U.S. public citing it as their main source for information about science issues. The majority of Americans who use the Internet rely on nontraditional online sources—only 12 percent use web sources developed by traditional print newspapers and magazines (Baram-Tsabari and Lewenstein, 2013).
The Internet is widely accessible but has disadvantages. Research has indicated that reading on digital screens is inferior to reading paper materials; readers prefer paper and have improved metacognitive performance when they read on paper (Ackerman and Goldsmith, 2011). The divide between those who do and do not have access to the Internet affects access to science information. And, among those who have access, there is significant variability in the skills needed to locate information (Segev, 2010) and to evaluate the quality of the information and the source (Wiley et al., 2009). On the other hand, frequent Internet users report more positive attitudes toward science than people who are offline (Brossard and Scheufele, 2013), and Internet use can compensate for educational disparities (Cacciatore et al., 2014).
Researchers have used data mining to explore the interests and information needs that Internet users demonstrate through their search queries (e.g., Anderson et al., 2010; Choi and Varian, 2009; Ginsberg et al., 2009; Segev and Baram-Tsabari, 2012). This research reveals patterns, such as increased interest in a particular science and technology topic in the wake of events covered in the media that concern the topic. Such interest tends to wane over time, however. Baram-Tsabari suggests that the effectiveness of a teachable moment presented by a public event depends on how well the science community responds to and takes advantage of the opportunity (Baram-Tsabari and Osborne, 2015).
Web-based resources have become increasingly interactive: wikis, blogs, video sharing, massive open online courses, and social networking sites, for example, are all highly interactive resources. Such resources allow nontechnical users to participate in science in new ways. Comment forums can enable scientists and policy makers to learn about the concerns, sources of information, and general knowledge participants have about science topics with social and political ramifications, such as nanotechnology or climate change. Some sites allow participants to ask scientists questions in real time. However, Baram-Tsabari reported that scientists prefer other forms of communication, such as writing articles, to Web-based forms such as managing websites or participating in social media (Baram-Tsabari and Lewenstein, 2013). The attitudes of scientists toward engagement online, how they perceive the public who participate in online interactions, and other critical factors that will frame online science communication are still largely unstudied (Besley, 2013).
CHALLENGES FOR CHEMISTRY
As stated previously, it is important to present science in a context relevant to participants. Finding this relevance for chemistry, however, is challenging because not much research targets public perceptions of chemists and chemistry or the effectiveness of chemistry communication events in informal settings. In a 2000 telephone survey commissioned by the American Chemical Society, approximately one-third of those surveyed had an unfavorable view of the chemical industry, which received the least favorable ratings of 10 science-related industries (NSB, 2002). Reasons included the potential negative environmental and health impacts of chemicals and a view of the industry as one that pollutes the environment and does not communicate with consumers. However, the majority of those surveyed had positive views of chemistry as a profession, particularly in basic chemical research, and 60 percent said that chemicals make everyday life better. This suggests that professional affiliations (e.g., the chemical industry) have a strong influence on public perception of chemists.
Recently, a survey by the Royal Society of Chemistry (RSC) provided positive news regarding public perception of chemistry in the United Kingdom and highlighted differences between the expectations of chemists (who believed public perception would be mostly negative) and the actual public perception (generally positive; RSC, 2015). Comparing such data across surveys and countries is challenging because of cultural, political, and other differences that influence perception (Besley, 2013). Nevertheless, the RSC report is a reminder to chemists considering public communication to consider one's own assumptions about participants before designing an event.
As previously described, trust is a critical factor for learning and public engagement. Research integrity, the credibility of the scientist and content, and institutional or other affiliations affect trust. Thus, the quality of the research being shared, the warmth and competence of the scientist, and transparency about affiliations and motivations for communicating should be considered when planning any event.
Insights from Chemistry Education
Research on student experiences with chemistry in the classroom informs the discussion of participant perception, which often originates in the classroom. Although formal education environments are different than informal ones, there is little research on chemistry in informal environments. However, relevant lessons from formal chemistry education can be used to aid chemists interested in designing public activities to communicate chemistry.
Many students struggle with chemistry. Research suggests that the abstract nature of molecular interactions, difficulty relating submicroscopic structures and macroscopic effects, and a lack of fluency with the tools of representation and symbolism in the field are key reasons. These difficulties can form fundamental conceptual barriers to understanding chemistry's real-world applications that carry forward from early classroom experiences to later life and result in lifelong attitudes about chemistry.
The capacity to think in abstract terms about chemical phenomena is not intuitive—it is developed through experience and training (Erduran et al., 2006). Proficiency in chemistry requires scientists to be fluent in the relationships between chemical phenomena at both visible and submicroscopic levels. Chemists rely on visualizations to understand the underlying processes, solve problems, and communicate ideas, and there is ample evidence that many students find this kind of thinking difficult (Ealy, 1999, 2004; Gilbert, 2005; Hinze et al., 2013; Kozma and Russell, 1997; Mathewson, 1999; Stieff et al., 2011; Wu and Shah, 2004).
These visualizations represent the spatial relationships in atomic and molecular structure through which chemists explain chemical and physical properties that can be observed. While imperfect, they represent chemists' understanding of reality in a way that allows them to communicate easily (within the chemical community) and to advance their work. Chemists are usually aware of the limitations of their models but rarely articulate these issues. For example, although all molecules are three-dimensional, chemists usually represent them with two-dimensional diagrams. Whereas chemists perceive how these diagrams imply three-dimensional relationships, the uninitiated can mistakenly infer that molecules themselves are two-dimensional. Chemists themselves sometimes overlook the limitations of visual representations (Haidar, 1997).
Research examines how people develop mental models of information they know, even if the information is incomplete, suggesting it is relatively easy for a novice to be led astray by a seemingly simple representation (NRC, 2014b). Learners also have difficulty with abstract concepts that are presented without context, such as relevance to everyday life, that helps the learners relate a concept to knowledge they already have (Tobias, 1990). Chemists' reliance on representing abstract concepts in a visual language that is unfamiliar to nonexperts adds to the challenge of communicating chemistry to nonexpert publics and points again to the value of establishing the relationship between teaching objectives and real-world applications.
Because chemistry knowledge is represented on macroscopic, submicroscopic, and symbolic levels, full understanding requires coordination across these levels, linking the symbols to the submicroscopic interactions and to the macroscopic outcomes. Learning environments in which all three levels are presented simultaneously and are explicitly related to each other are likely to support learners in accomplishing this coordination (Johnstone, 1993; Kozma and Russell, 1997, 2005; Kozma et al., 1996; Stieff, 2005; Stieff and McCombs, 2006; Stieff and Wilensky, 2003; Stieff et al., 2011). Thus, the use of multiple, linked representations to communicate a single concept may be more likely to help informal learners grasp it.
Chemistry is frequently concerned with the transformation of spatial objects over time (e.g., molecular vibrations in three dimensions), which is not directly perceptible. Understanding spatiotemporal dynamics and other complex processes can be easier when they are represented both visually and verbally (Clark and Paivio, 1991; Mayer, 2001; Newcombe and Stieff, 2012; Paivio, 1986). Animations and simulations can represent dynamic chemistry concepts, but experts evaluate these visual representations differently than novices do. Novices may not recognize which features they should attend to because they lack familiarity with the underlying conceptual framework (Kombartzky et al., 2010; Mason et al., 2013; Plass et al., 2009, 2010; Stieff et al., 2011). Visual representations are likely to be most useful to novice learners when they use a minimum of information to communicate an idea (Kozhevnikov et al., 2006; Kozma and Russell, 1997; Ruetenik et al., 2011; Stieff and McCombs, 2006). Animations and simulations are useful in informal environments so long as they display limited amounts of information, promote interactive engagement, and are used together with activities that guide learner attention (Stieff and Ryan, 2014).
Evidence of the challenge that chemistry presents is apparent in both secondary school and undergraduate chemistry classrooms (Stieff and Wilensky, 2003) and can be observed even among advanced undergraduate students. Research has shown, for example, that individuals have trouble recognizing that equilibrium is a dynamic process despite years of formal schooling in chemistry. In addition, communicators may encounter conceptual challenges. For example, a fundamental concept in chemistry is the assumption that if two substances are identical in chemical composition and structure on the submicroscopic level, they will have the same properties on the macroscopic level. The converse is not true: chemists do not assume that two macroscopically identical substances will share the same chemical composition and structure. Nonexperts, however, are prone to make this error, thinking, for example, that “copper atoms are shiny because copper wire is shiny” or “two beakers of transparent liquid both contain water molecules.”
Unfortunately, many students' school experiences contribute to misconceptions of chemistry concepts. The Grunwald Associates study suggests that chemistry is not well represented in K-12 education and that it is not taught in ways that promote broad understanding and recognition of real-world applications (Grunwald Associates and Education Development Center, 2013). Traditional instructional methods often focus on molecular-level interactions and treat everyday observations as enrichment activities, though students at all academic levels tend to be more interested in topics with direct relevance to their experiences (Glynn et al., 2007). In addition, negative interactions with science instructors and poor performance discourage many students and may contribute to a perception that science belongs to an “elite” group of individuals (Chiarelott, 1987; Udo et al., 2004). Topics such as the chemistry of health or the environment and those that appear in the news, on television, or in movies (crime investigation is a prime example) help to engage students in the explanatory power of chemistry (Glynn et al., 2007).
Other fields have similar challenges. Biologists have difficulty explaining the effects of bacteria on human health—a problem similar to that of chemists explaining phenomena that are not directly observable—yet the public has come to understand that bacteria impact human health. Also, chemistry is often embedded in topics that are seen as belonging in other fields, and some observers have noted that “there is no single idea that unites the field or common story about what it is and what it means” (Grunwald Associates and Education Development Center, 2013, p. 11). Because chemistry concepts that arise in informal learning settings are often presented in the context of topics in physics or biology, these opportunities do not necessarily bolster understanding of why chemistry is important.
PRINCIPLES FOR CHEMISTRY COMMUNICATION IN INFORMAL ENVIRONMENTS
A few ideas manifested themselves repeatedly in the work we examined and form the basis of a set of principles that can guide the development of effective informal chemistry communication opportunities. These principles provide the basis on which the practical framework in Part B is built.
Use knowledge of the participants to identify clear and specific goals and target outcomes for the chemistry communication experience.
Informal communication about chemistry is most likely to be effective if it meets the needs of the populations it is to serve. The chemistry communicator must have some knowledge of the likely participants to tailor the content and set goals. This knowledge must be integrated into a logical plan that suits the time and resources available. Science communicators should seek to understand the ideas, beliefs, and perspectives that participants bring and should take into account their values and level of knowledge. Engagement around social issues that people have strong opinions about can be a valuable opportunity to develop participants' awareness of what science contributes to understanding and potential solutions.
Use understanding of participants to make the experience engaging and positive.
Chemistry communication should be an opportunity for participants to become excited about phenomena that scientists explore and about scientists' methods. Activities that are fun, and that allow participants to engage and interact with the material, can enable them to see themselves as science learners. Learning about science happens naturally throughout life, and well-planned informal communication opportunities help people connect that learning with science concepts, retain the knowledge, and feel encouraged to seek more knowledge. Topics that link science with environmental and other social issues can provide an incentive to engage. To promote this kind of learning, communicators must incorporate the cultural experiences and everyday language that will be most familiar to participants.
Use the nature of the experience to engage participants.
Specific characteristics of an experience are likely to encourage participants to explore and satisfy their curiosity. Informal settings are easier to explore in, in part because performance expectations are not usually part of the experience but instead may be one outcome. Rather than being tested, participants should be encouraged to do things that scientists do: ask questions, observe phenomena, make models, develop and test hypotheses, examine data and evidence, draw conclusions, imagine and design practical implications, test and revise prototypes, discuss both potential positive and negative impacts of an innovation, and the like. Settings and program designs that encourage participants to think, play, and interact with one another, with the science communicator, and with the materials and content tend to generate the excitement, wonder, and surprise that make the science event meaningful. When such activities become long-term relationships with science (for example, when participants contribute to data collection or other citizen science efforts), the relationships build understanding of or appreciation for the scientific process and help people make connections between science and everyday life. Often, scientists gain from these relationships as well, for example, by learning from interactions with participants or by benefiting from the data participants collect.
Use strategies that are valuable for informal chemistry communication.
For many participants, the abstract nature of molecular interactions, difficulty with the relationship between submicroscopic structures and macroscopic effects, and a lack of fluency with the tools of representation and symbolism in the field can be obstacles to understanding and to an appreciation of chemistry's real-world applications. The use of multiple, linked representations to communicate a single concept may help participants grasp it. Analogies and visual representations, such as animations and simulations, are especially useful in helping novices understand abstract ideas and phenomena that cannot be directly observed. The fact that people learn throughout their lives while participating in the activities of daily life, such as hobbies, outdoor excursions, or cooking, is especially useful with chemistry, because informal learning opportunities can build on the expertise that people naturally develop in areas import to them.
REFERENCES
- Ackerman R, Goldsmith M. Metacognitive regulation of text learning: On screen versus on paper. Journal of Experimental Psychology: Applied. 2011;17(1):18–32. [PubMed: 21443378]
- Ambrose SA, Bridges MW, DiPietro M, Lovett MC, Norman MK. How learning works: Seven research-based principles for smart teaching. San Francisco, CA: Jossey-Bass; 2010. [December 11, 2014]. http://c4ed
.lib.kmutt .ac.th/sites/default /files/HowLearningWorks-Ambrose.pdf. - Anderson AA, Brossard D, Scheufele DA. The changing information environment for nanotechnology: Online audiences and content. Journal of Nanoparticle Research. 2010;12(4):1083–1094. [PMC free article: PMC2988218] [PubMed: 21170132]
- Bandura A. Social foundations of thought and action: A social cognitive theory. Englewood Cliffs, NJ: Prentice-Hall; 1986.
- Bandura A. Self-efficacy: The exercise of control. New York: Freeman; 1997.
- Baram-Tsabari A, Lewenstein B. An instrument for assessing scientists' written skills in public communication of science. Science Communication. 2013;35(1):1–30.
- Baram-Tsabari A, Osbourne J. Bridging science education and science communication research. Journal of Research in Science Teaching. 2015;52(2):135–144.
- Bauer MW, Howard S. Public Understanding of Science—A peer-review journal for turbulent times. Public Understanding of Science. 2012;21(3):258–267.
- Bauer MW, Allum N, Miller S. What can we learn from 25 years of PUS survey research? Liberating and expanding the agenda. Public Understanding of Science. 2007;16(1):79–95.
- Besley J. The state of public opinion research on attitudes and understanding of science and technology. Bulletin of Science, Technology & Society. 2013;33(1-2):12–20. [CrossRef]
- Bonney R, Ballard H, Jordan R, McCallie E, Phillips T, Shirk J, Wilderman C. Public Participation in Scientific Research: Defining the Field and Assessing Its Potential for Informal Science Education. Washington, DC: Center for Advancement of Informal Science Education (CAISE); 2009. [December 11, 2014]. (A CAISE Inquiry Group Report). http:
//informalscience .org/images/research /PublicParticipationinScientificResearch.pdf. - Boudet H, Clarke C, Bugden D, Maibach E, Roser-Renough C, Leiserowitz A. “Fracking” controversy and communication: Using national survey data to understand public perceptions of hydraulic fracturing. Energy Policy. 2014;65:57–67.
- Brossard D, Scheufele DA. Science, new media, and the public. Science. 2013;339(6115):40–41. [PubMed: 23288529]
- Bucchi M, Trench B, editors. Handbook of Public Communication of Science and Technology: Second Edition. New York: Routledge; 2014.
- Cacciatore MA, Scheufele DA, Corley EA. Another (methodological) look at knowledge gaps and the Internet's potential for closing them. Public Understanding of Science. 2014;23(4):376–394. [PubMed: 23825272]
- Chiarelott L. Science anxiety: Implications for science curriculum and teaching. The Clearing House. 1987;60(5):202–205.
- Choi H, Varian H. Predicting the Present with Google Trends. Google Inc.; Apr 10, 2009. 2009. [December 11, 2014]. Draft. http://www
.google.com /googleblogs/pdfs/google _predicting_the_present.pdf. - Chryssochoidis G, Strada A, Krystallis A. Public trust in institutions and information sources regarding risk management and communication: Towards integrating extant knowledge. Journal of Risk Research. 2009;12(2):137–185.
- Clark JM, Paivio A. Dual coding theory and education. Educational Psychology Review. 1991;3(3):149–210.
- Critchley CR. Public opinion and trust in scientists: the role of the research context, and the perceived motivation of stem cell researchers. Public Understanding of Science. 2008;17(3):309–327. [PubMed: 19069082]
- Ealy JB. A student evaluation of molecular modeling in first year college chemistry. Journal of Science Education and Technology. 1999;8(4):309–321.
- Ealy JB. Students' understanding is enhanced through molecular modeling. Journal of Science Education and Technology. 2004;13(4):461–471.
- Erduran S, Ardac D, Yakmaci-Guzel B. Learning to teach argumentation: Case studies of preservice secondary science teachers. Eurasia Journal of Mathematics, Science and Technology Education. 2006;2(2)
- Falk JH, Dierking LD. The 95 percent solution: School is not where most Americans learn most of their science. American Scientist. 2010;98(6):486–493.
- Fischoff B. The sciences of science communication. Proceedings of the National Academy of Sciences of the United States of America. 2013;110(3):14033–14039. [PMC free article: PMC3752164] [PubMed: 23942125]
- Fisher KM. Reform in undergraduate science teaching for the 21st century. Sunal DW, Wright EL, Bland J, editors. Charlotte, NC: Information Age Publishing; 2004. pp. 69–84. (The importance of prior knowledge in college science instruction).
- Fiske S, Dupree C. Emerging trends in the social and behavioral sciences. Scott RA, Kosslyn SM, editors. New York: Wiley; 2015. [June 2014]. (Cognition processes involved in stereotyping). http://www
.fiskelab.org /storage/publications /Sage%20Emerging %20Trends%20Scott%20Kosslyn %20Fiske%20Dupree %20CogStereotyping%20final.pdf. - Gilbert JK. Visualization in science education. Dordrecht: Springer; 2005.
- Ginsberg J, Mohebbi MH, Patel RS, Brammer L, Smolinski MS, Brilliant L. Detecting influenza epidemics using search engine query data. Nature. 2009;457(7232):1012–1014. [PubMed: 19020500]
- Glynn SM, Taasoobshirazi G, Brickman P. Nonscience majors learning science: A theoretical model of motivation. Journal of Research in Science Teaching. 2007;44(8):1088–1107.
- Grunwald Associates and Education Development Center. Communicating Chemistry Landscape Study. May 8, 2013.
- Haidar AH. Prospective chemistry teachers' conceptions of the conservation of matter and related concepts. Journal of Research in Science Teaching. 1997;34(2):181–197.
- Haidt J. The righteous mind: Why good people are divided by politics and religion. New York: Vintage Books; 2013.
- Halsall P, editor. Internet Modern History Sourcebook: Michael Faraday (1791-1867): The Chemical History of a Candle, 1860. Fordham University; 1998. [December 19, 2014]. http://legacy
.fordham .edu/halsall/mod/1860Faraday-candle.asp. - Harley D. Scholarly communication: Cultural contexts, evolving models. Science. 2013;342(6154):80–82. [PubMed: 24092736]
- Hinze SR, Rapp DN, Williamson VM, Shultz MJ, Deslongchamps G, Williamson KC. Beyond the ball-and-stick: Students' processing of novel STEM visualizations. Learning and Instruction. 2013;26:12–21.
- Hmelo-Silver CE, Marathe S, Liu L. Fish swim, rocks sit, and lungs breathe: Expert-novice understanding of complex systems. Journal of the Learning Sciences. 2007;16(3):307–331.
- Hon LC, Grunig JE. Guidelines for Measuring Relationships in Public Relations. Institute for Public Relationships; 1999. [July 13, 2015]. http://www
.shape.nato .int/resources/9/conference%202011 /guidelines _measuring_relationships[1].pdf. - Johnstone AH. The development of chemistry teaching: A changing response to changing demand. Journal of Chemical Education. 1993;70(9):701–705.
- Kahan DM, Braman D, Mandel GN. Risk and Culture: Is Synthetic Biology Different? Yale Law School; 2009. [July 13, 2015]. Harvard Law School Program on Risk Regulation Research Paper No. 09-2. (Public Law Working Paper No 190). http://ssrn
.com/abstract=1347165. [CrossRef] - Kahan DM, Jenkins-Smith H, Braman D. Cultural cognition of scientific consensus. Journal of Risk Research. 2011;14:147–174.
- Kahan DM, Peters E, Wittline M, Slovic P, Ouellett LL, Braman D, Mandel G. The polarizing impact of science literacy and numeracy on perceived climate change risks. Nature Climate Change. 2012;2:732–735. [CrossRef]
- Kombartzky U, Ploetzner R, Schlag S, Metz B. Developing and evaluating a strategy for learning from animations. Learning and Instruction. 2010;20:424–433.
- Kozhevnikov M, Motes M, Rasch B, Blajenkova O. Perspective-taking vs. mental rotation transformations and how they predict spatial navigation performance. Applied Cognitive Psychology. 2006;20:397–417.
- Kozma RB, Russell J. Multimedia and understanding: Expert and novice responses to different representations of chemical phenomena. Journal of Research in Science Teaching. 1997;34(9):949–968.
- Kozma R, Russell J. Visualization in science education. Gilbert J, editor. London: Kluwer; 2005. pp. 121–146. (Students becoming chemists: Developing representational competence).
- Kozma R, Russell J, Jones T, Marx N, Davis J. International perspectives on the psychological foundations of technology-based learning environments. Vosniadou S, Glaser R, De Corte E, Mandl H, editors. Hillsdale, NJ: Lawrence Erlbaum Associates; 1996. pp. 41–60. (The use of multiple, linked representations to facilitate science understanding).
- Kunda Z. The case for motivated reasoning. Psychological Bulletin. 1990;108(3):480–498. [PubMed: 2270237]
- Liang X, Su LY, Scheufele DA, Brossard D, Xenos M, Nealey P, Corley EA. Building buzz: (Scientists) communicating science in new media environments. Journalism & Mass Communication Quarterly. 2014;91(4):772–791. [CrossRef]
- Lidar M, Lundzvist E, Ostman L. Teaching and learning in the science classroom: The interplay between teachers' epistemological moves and students' practical epistemology. Science Education. 2006;90:148–163.
- Mason GS, Shuman TR, Cook KE. Comparing the effectiveness of an inverted classroom to a traditional classroom in an upper-division engineering course. IEEE Transactions on Education. 2013;56(4):430–435. [CrossRef]
- Mathewson JH. Visual-spatial thinking: An aspect of science overlooked by educators. Science Education. 1999;83:33–54.
- Mayer RE. Multimedia Learning. New York: Cambridge University Press; 2001.
- NAE and NRC (National Academy of Engineering and National Research Council). Technically speaking: Why all Americans need to know more about technology. Washington, DC: National Academy Press; 2002.
- NAS (National Academy of Sciences). The science of science communication. Proceedings of the National Academy of Sciences of the United States of America. 2013;10(Suppl 3):14031–14032. [PMC free article: PMC3752170] [PubMed: 23942127]
- Nathan MJ, Eilam B, Kim S. To disagree, we must also agree: How intersubjectivity structures and perpetuates discourse in a mathematics classroom. Journal of the Learning Sciences. 2007;16(4):523–563.
- Newcombe NS, Stieff M. Six myths about spatial reasoning. Interntional Journal of Science Education. 2012;34(6):955–971.
- Nisbet M, Markowitz EM. Understanding public opinion in debates over biomedical research: Looking beyond political partisanship to focus on beliefs about science and society. PLoS One. 2014 February 18; [July 13, 2015]; http:
//journalistsresource .org/studies/society /publichealth/public-opinion-biomedical-research-partisanship#sthash .Heq8i6xx.dpuf. [PMC free article: PMC3928253] [PubMed: 24558393] [CrossRef] - NRC (National Research Council). Learning science in informal environments: People, places, and pursuits. Bell P, Lewenstein B, Shouse AW, Feder MA, editors. Washington, DC: The National Academies Press; 2009.
- NRC. Surrounded by science: Learning science in informal environments. Washington, DC: The National Academies Press; 2010.
- NRC. Climate change education: Engaging family private forest owners on issues related to climate change: A workshop summary. Washington, DC: The National Academies Press; 2014a.
- NRC. The science of science communication II: Summary of a colloquium. Washington, DC: The National Academies Press; 2014b.
- NSB (National Science Board). Science and Engineering Indicators. Arlington, VA: National Science Foundation; 2002. [June 2014]. (NSB 02-1). http://www
.nsf.gov/statistics /seind02/pdfstart.htm. - NSB. Science and Engineering Indicators. Arlington, VA: National Science Foundation; 2014. [December 17, 2014]. (NSB 14-01). http://www
.nsf.gov/statistics/seind14/ - Paivio A. Mental representations: A dual coding approach. Oxford: Oxford University Press; 1986.
- Peters HP. Gap between science and media revisited: Scientists as public communicators. Proceedings of the National Academy of Sciences of the United States of America. 2013;110(Suppl 3):14102–14109. [PMC free article: PMC3752168] [PubMed: 23940312]
- Peters HP, Dunwoody S, Allgaier J, Lo Y, Brossard D. Public communication of science 2.0. EMBO Reports. 2014;15(7):749–753. [PMC free article: PMC4196977] [PubMed: 24920610] [CrossRef]
- Pew Research Center. Public Praises Science; Scientists Fault Public, Media. 2009. [June 2014]. http://www
.people-press .org/2009/07/09/public-praises-science-scientists-fault-public-media/ - Pew Research Center. Public Esteem for Military Still High. 2013. [June 2014]. http://www
.pewforum.org /2013/07/11/public-esteem-for-military-still-high /http://www .pewforum.org/2013 /07/11/publicesteem-for-military-still-high/ - Plass JL, Homer BD, Hayward E. Design factors for educationally effective animations and simulations. Journal of Computing in Higher Education. 2009;21:31–61. [CrossRef]
- Plass JL, Moreno R, Brünken R. Cognitive load theory. New York: Cambridge University Press; 2010.
- Rappoport LT, Ashkenazi G. Connecting levels of representation: Emergent versus submergent perspective. International Journal of Science Education. 2008;30(12):1585–1603.
- Resnik DB. Scientific Research and the Public Trust. Science and Engineering Ethics. 2011;17(3):399–409. [PMC free article: PMC3151305] [PubMed: 20803259]
- RSC (Royal Society of Chemistry). Public Attitudes to Chemistry. 2015. [January 24, 2016]. (Research Report). http://www
.rsc.org/globalassets /04-campaigning-outreach /campaigning /public-attitudes-to-chemistry /publicattitudes-to-chemistry-research-report.pdf?id=8495. - Ruetenik G, Steffke C, Thomas SR, Libarkin J. Analyzing visual representations of climate change. Geological Society of America Abstracts with Programs. 2011;43(5):571.
- Scheufele DA. Engaging science: Thoughts, deeds, analysis and action. Tourney J, editor. London: The Wellcome Trust; 2006. pp. 20–25. (Messages and heuristics. How audiences form attitudes about emerging technologies).
- Scheufele DA, Corley EA, Shih TJ, Dalrymple KR, Ho SS. Religious beliefs and public attitudes to nanotechnology in Europe and the US. Nature Nanotechnology. 2009;4(2):91–94. [PubMed: 19197309]
- Science, Media, and the Public Research Group (SCIMEP); University of Wisconsin–Madison. Scientists and Social Media. Madison, WI: Department of Life Sciences Communication; 2015. [January 2015]. http://scimep
.wisc.edu/projects/reports/ - Segev E. Google and the digital divide: The bias of online knowledge. Cambridge: Woodhead Publishing; 2010.
- Segev E, Baram-Tsabari A. Seeking science information online: Data mining Google to better understand the roles of the media and the education system. Public Understanding of Science. 2012;21(7):813–829. [PubMed: 23832560]
- Shema H, Bar-Ilan H, Thelwall M. Research blogs and the discussion of scholarly information. PLoS One. 2012;7(5):e35869. [PMC free article: PMC3350512] [PubMed: 22606239] [CrossRef]
- Silberman RG, Trautmann C, Merkel SM. Chemistry at a science museum. Journal of Chemical Education. 2004;8(1):51–53.
- Smith TW, Son J. Trends in public attitudes about confidence in institutions. Chicago: NORC; 2013. (General Social Survey 2012 final report).
- Stieff M. Connected chemistry: A novel modeling environment for the chemistry classroom. Journal of Chemical Education. 2005;82(3):489–493.
- Stieff M, McCombs M. Barab S, Hay KE, Hickey DT, editors. Proceedings of the Seventh International Conference of the Learning Sciences (ICLS). Mahwah, NJ: Erlbaum; 2006. pp. 730–736. (Increasing representational fluency with visualization tools).
- Stieff M, Ryan S. Pedagogic roles of animations and simulations in chemistry courses. Washington, DC: American Chemical Society; 2014. Explanatory models for the research & development of chemistry visualizations; pp. 15–41. (ACS Symposium Series, Vol 1142).
- Stieff M, Wilensky U. Connected chemistry—Incorporating interactive simulations into the chemistry classroom. Journal of Science Education and Technology. 2003;12(3):285–302.
- Stieff M, Hegarty M, Deslongchamps G. Identifying representational competence with multi-representational displays. Cognition and Instruction. 2011;29(1):123–145.
- Stieff M, Yip J, Ryu M. Speaking across levels-generating and addressing level confusion in discourse. Chemistry Education Research and Practice. 2013;14(4):376–389.
- Suerdem A, Bauer MW, Howard S, Ruby L. PUS in turbulent times II—A shifting vocabulary that brokers inter-disciplinary knowledge. Public Understanding of Science. 2013;22:2–15. [PubMed: 23832881] [CrossRef]
- Tobias S. They're not dumb, they're different: Stalking the second tier. Tucson, AZ: Research Corporation; 1990.
- Udo MK, Ramsey GP, Mallow JV. Science anxiety and gender in students taking general education science courses. Journal of Science Education and Technology. 2004;13(4):435–446.
- Wiley J, Goldman SR, Graesser AC, Sanchez CA, Ash IK, Hemmerich JA. Source evaluation, comprehension, and learning in Internet science inquiry task. American Educational Research Journal. 2009;46(4):1060–1106.
- Wu HK, Shah P. Exploring visuospatial thinking in chemistry learning. Science Education. 2004;88(3):465–492.
- Zare RN. Where's the chemistry in science museums? Journal of Chemical Education. 1996;73(9):A198–A199.
Footnotes
- 1
For a brief discussion of Faraday and the lectures, see Halsall (1998).
- 2
See http://www
.chemistry2011.org [accessed May 2014] for more information. - 3
For examples, see the National Science Digital Library's informal educational resource database at http://www
.howtosmile .org/content/teaching-chemistry-ideas [accessed May 2014], which provides demonstrations, exhibits, lab activities, games, lesson plans, models, and simulations with learning times ranging from under 5 minutes to 4 weeks. - 4
The National Academy of Engineering and NRC report titled Technically Speaking (NAE and NRC, 2002) notes that most people view technology as referring primarily to artifacts, such as computers and software or vehicles, and do not recognize technology and engineering as fields separate from the science disciplines. For the public, the term “chemistry” suggests not only the academic discipline but also all of its technological applications, such as the development of pesticides or other products.
- 5
For overviews of the field of public communication in science see Bauer and Howard (2012), Bauer et al. (2007), Bucchi and Trench (2014), and Suerdem et al. (2013).
- 6
See https://www
.reddit.com/r/science [accessed February 2016] for more information. - 7
See http://www
.scistuchat.com [accessed February 2016] for more information. - 8
A social science survey of American attitudes, behaviors, and attributes, the GSS is conducted by the independent research organization NORC at the University of Chicago, and has been monitoring American society since 1972.
- Evidence-Based Research on Learning and Communication - Effective Chemistry Comm...Evidence-Based Research on Learning and Communication - Effective Chemistry Communication in Informal Environments
- The Current State of Chemistry Communication - Effective Chemistry Communication...The Current State of Chemistry Communication - Effective Chemistry Communication in Informal Environments
- SRX5180730 (1)SRA
Your browsing activity is empty.
Activity recording is turned off.
See more...