NCBI Bookshelf. A service of the National Library of Medicine, National Institutes of Health.
Institute of Medicine (US) Committee on Assessing Genetic Risks; Andrews LB, Fullarton JE, Holtzman NA, et al., editors. Assessing Genetic Risks: Implications for Health and Social Policy. Washington (DC): National Academies Press (US); 1994.
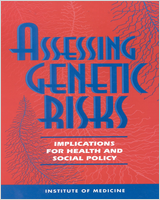
Assessing Genetic Risks: Implications for Health and Social Policy.
Show detailsThe mapping of the human genome will lead to the identification of virtually all disease-causing genes and the development of tests to detect at least some of the mutations that are responsible for single-gene disorders and for susceptibility to many other common disorders. This proliferation of genetic tests will benefit those at risk of genetic and chromosomal disorders by permitting early treatment or, when treatment is not available, by providing prospective parents with options for avoiding the conception or birth of children affected with serious disorders. Consequently, errors in test performance and interpretation will detract from the benefit of genetic testing. Laboratory errors do occur. Errors have been documented in newborn screening (Holtzman et al., 1986; Hannon and Adam, 1991; Adam and Hannon, 1992), in biochemical genetic testing (Hommes et al., 1990), in karyotyping in cytogenetics* (Vockley et al., 1991), in linkage analysis, and in interpretation of results (P.M. Conneally, personal communication, February 1992).
Such errors in performance are likely to continue for the following reasons:
- many clinical laboratories are unfamiliar with the recombinant DNA techniques used in most new genetic tests;
- the use of very small samples with polymerase chain reaction (PCR) increases the chance of contamination with foreign DNA;
- the large volume of tests increases the chance of unintentional switching of samples;
- the vast majority of results will be in the normal range, a tendency that reduces the vigilance of those performing the test;
- the nature of genetic disorders increases the chance of errors in interpretation;
- tests at the DNA level may not detect all disease-causing or susceptibility-conferring mutations, resulting in false negative results; and
- a positive test result cannot always predict disease severity and, in some instances, may falsely predict the future occurrence of disease particularly in tests for predispositions to multifactorial disorders and disorders of variable expressivity.
This chapter reviews current programs and regulations for assessing the quality of laboratories providing genetic tests, including the interpretation of test results, and current provisions for assessing the safety and effectiveness of genetic test kits and their critical components. For purposes of the committee's definition, genetic tests are those that are used primarily for predicting the risk of genetic or gene-influenced disease either in the person being tested or in his or her descendants. Tests that are used primarily for other purposes, but may contribute to diagnosing a genetic disease (e.g., blood smears, certain serum chemistries), would not be covered by this definition.
Because the field of genetic testing is developing very rapidly, few systematic data are available on how many genetic tests are being done, who is doing them, or how well they are being done (Meaney, 1992). Some data are available on newborn screening (Holtzman et al., 1986; Hannon and Adam, 1991; Adam and Hannon, 1992; CORN, 1992). Similarly, few published data on genetic laboratory quality are available (Holtzman et al., 1986; Hommes et al., 1990; Hannon and Adam, 1991; Meaney, 1992). To assess the nature and extent of special laboratory issues in human genetics, therefore, the committee held an expert workshop to review existing voluntary, professional, and governmental regulatory efforts to ensure the quality of genetic laboratory testing (participants are listed in Appendix A of this report). Committee members also met with representatives of the Food and Drug Administration (FDA), the Health Care Financing Administration (HCFA), and the Centers for Disease Control and Prevention (CDC) to learn the agencies' current policies and plans for assessing the safety and effectiveness of genetic tests.
Identifying problems of the quality of genetic tests is complicated because of the ways in which they are provided. A few are sold as kits, primarily to clinical laboratories; these have to undergo scrutiny by the FDA. An increasing number of tests are being marketed as laboratory services by commercial laboratories and a few academic laboratories. Many more are being provided for clinical decisionmaking purposes by research laboratories in academic medical centers. Although the critical reagents (which usually are not part of FDA-approved kits) used for testing in commercial and research laboratories, and the laboratories themselves, are subject to new federal regulations, many laboratories and investigators are unaware of them. Part of the reason is the federal government's failure to apply current regulations to genetic tests on a systematic and thorough basis. Were it not for the availability of tests through research laboratories, many people at risk for rare diseases could be deprived of an opportunity to use these tests; they are of such limited commercial value that manufacturers of in vitro diagnostics have little interest in them. One regulatory challenge is to ensure quality without diminishing the availability of these tests. The committee is concerned that the regulatory burden not impede further development of tests or the provision of genetic testing services by laboratories that currently provide them.
PROGRAMS AND REGULATIONS FOR ASSESSING THE QUALITY OF LABORATORIES PROVIDING GENETIC TESTS
Assessment of the quality of laboratories providing genetic tests is currently conducted by a few states and by voluntary participation in the proficiency testing programs of private organizations. At present, only 10 states have some form of specific requirements for the licensing of clinical laboratories providing genetic tests (M. Watson, personal communication to Congressman Ted Weiss, 1992). Only New York State has specific legislation and regulations dealing with laboratories providing the full range of genetic tests, including DNA tests.
State Assessments of Laboratories Providing Genetic Tests
New York State began mandatory regulation of genetic testing when it established the first program assessment for cytogenetics laboratories in the United States in 1972, with cytogenetics proficiency testing beginning in 1974 (Willey, 1992). Since 1990, New York has been the only state that has specific mandatory standards and permits for DNA genetics laboratories performing tests on its citizens. It has a list of DNA tests approved for testing, including tests for sickle cell anemia, cystic fibrosis (CF), Duchenne and Becker muscular dystrophy, Tay-Sachs, and the thalassemias (A. Willey, Director, Laboratory of Human Genetics, New York State Department of Health, personal communication, January 1993). To be included on this list of approved DNA tests, the disorders must meet identified criteria (e.g., no form of the disorder that is undetectable because a mutation at another locus is known to exist; the method must identify 90 to 95 percent of cases; reagents and processes must be generally available in the laboratory community—or must be made available; if linkage analysis is required, the markers must be a specified map distance from the disease gene). To be approved to perform DNA tests from this list, the laboratories must demonstrate that they successfully perform the accepted test methodologies. New York developed a special training program for all inspectors who survey laboratories that perform genetic testing. New York has also developed standards for proficiency testing for DNA tests, but these standards have not yet been implemented (Murphy, 1992a). The reach of the New York State program extends beyond the geographic borders of the state, since any laboratory wishing to perform a genetic test on a citizen of New York must be certified just as if it were located in New York; thus, most large commercial genetic testing laboratories who accept specimens from around the United States are licensed to meet New York DNA laboratory standards. Currently, no other state has developed as rigorous a program for the quality control of genetic testing as New York.
BOX 3-1Congressional Oversight Hearing
To focus attention on the special issues in genetic laboratory testing, congressional hearings were held in July 1992 to highlight unresolved laboratory issues in human genetics (House Subcommittee on Human Resources and Intergovernmental Relations, 1992). The hearing included testimony from two patients who had had significant problems with genetic testing. One woman experienced long delays (more than seven months), high cost, and problems with her referring physician—all without receiving any results from the first physician and laboratory, when she sought presymptomatic diagnosis for Huntington disease. A pregnant woman testified about the anxiety caused by two positive reports from maternal serum alpha-fetoprotein (MSAFP) screening tests indicating a high risk for Down syndrome; only weeks later was it determined by amniocentesis that the fetus she carried did not have Down syndrome (Hoyt, 1992). Anxiety of this type is observed frequently while women are awaiting MSAFP results but usually abates when a negative result is reported (Holtzman et al., 1991).
The expert witnesses each presented cases of laboratory problems in various kinds of genetic diagnosis, testing, and screening. In one prominent medical center, the coordinator of the prenatal alpha-fetoprotein screening laboratory identified four significant problems over a two-month period, three involving commercial laboratories that provide MSAFP, and one problem of interpretation by a physician (Holtzman, 1992):
- 1.
In one case, a laboratory reporting an abnormally low MSAFP result, failed to recognize that the woman's age of 36 (considered "advanced maternal age" for purposes of prenatal diagnosis) warranted proceeding directly to a definitive cytogenetic test. Waiting for the results of the tests it recommended could have delayed diagnosis and reduced the woman's options had the confirmatory test been positive.
- 2.
In a second case, the laboratory director was uncertain whether the result had been adjusted for the patient's race and weight, key factors in interpreting test results.
- 3.
In a third case, there was not enough information supplied on the request form to determine that the woman was further into her pregnancy than thought; the laboratory reported that her result was abnormal when, in fact, it was normal for her true stage of pregnancy.
- 4.
In interpreting a woman's results, her physician correctly noted that she was not at increased risk for having a child with Down syndrome, but he failed to observe that she had an elevated MSAFP level and was, therefore, at increased risk for having a child with a neural tube defect.
____________
NOTE: Witnesses at the July 23, 1992, hearing included three panels: (1) Heidi Hoyt, Washington, D.C., and "Kim" of Illinois (both patients reporting their experiences with genetic testing); (2) specialized genetics personnel: Elizabeth Gettig, M.S., Director of Genetic Counseling, West Penn Hospital, Pittsburgh, Pa.; Paul Billings, M.D., Chief, Division of Genetic Medicine, California Pacific Medical Center, Assistant Clinical Professor of Medicine, University of California, San Francisco, Calif.; Neil A. Holtzman, Professor of Pediatrics, Johns Hopkins School of Medicine, Baltimore, Md.; Patricia Murphy, Ph.D., Genica Pharmaceutical Corporation, Worcester, Mass.; and (3) representatives of U.S. Department of Health and Human Services agencies: William Toby, Acting Administrator of the Health Care Financing Administration (HCFA); Barbara Gagle, Acting Director, Health Standards and Quality Bureau, HCFA; Edward Baker, M.D., Director, Public Health Practice Program Office, Centers for Disease Control; Alan Anderson, M.D., Acting Director, Center for Devices and Radiological Health, Food and Drug Administration.
The California Department of Health Services contracts with private laboratories to provide newborn screening and maternal serum alpha-fetoprotein (MSAFP) testing. As part of the process it assesses the quality of the laboratories with whom it contracts. Since 1980, California has limited newborn screening to eight state-monitored laboratories, with centralized computer data collection, quality control, blind proficiency testing, and case follow-up. Fees for newborn screening are collected from the birth hospital or birth attendant. California also has centralized laboratory testing, quality control, and blind proficiency testing for its MSAFP screening program, which must be offered to every pregnant woman in California. California has developed draft regulations on DNA, cytogenetics, and microbiological testing, and for prenatal diagnosis centers and clinical centers; these draft regulations are awaiting public comment and public hearing (Cunningham, 1992).
Maryland has regulations requiring genetic testing and screening laboratories to demonstrate "continuing satisfactory performance" in external proficiency testing programs where they exist. The only approved laboratory for newborn screening in Maryland is the state laboratory, which participates in the U.S. Centers for Disease Control proficiency testing program for newborn screening (see below). Fourteen laboratories (including the state laboratory) are approved for MSAFP screening. Maryland regulations require laboratories doing Tay-Sachs testing to participate successfully in the proficiency testing program of the International Tay-Sachs Disease Quality Control Reference Standards and Data Collection Center (M. Kaback, personal communication, November 1992).
New Jersey requires its laboratories to comply with the New York system for genetic tests, and it now also recognizes College of American Pathology (CAP) proficiency testing for cytogenetics. Florida licenses cytogenetics laboratories using CAP guidelines and proficiency testing, but its legislation is now expiring. Iowa requires that MSAFP testing be done centrally in the state laboratory at the University of Iowa.
In addition, states in the Pacific Northwest Region (PacNoRGG) of the Council of Regional Networks for Genetic Services (CORN), including Washington, Oregon, Idaho, and Alaska, have adopted PacNoRGG proficiency testing standards as a condition for state laboratory license as a cytogenetics laboratory. The PacNoRGG cytogenetics proficiency test involves the provision of blind samples and requires the interpretation of clinical information on individuals and families.
Each round of proficiency testing gets more difficult as the proficiency of the participating laboratories improves.
Voluntary Quality Assurance and Proficiency Testing in Genetics
The Council of Regional Networks of Genetic Services, the College of American Pathologists, the Centers for Disease Control and Prevention, and several other organizations have developed specific genetic tests or tests for specific disorders. These include maternal serum alpha-fetoprotein, Tay-Sachs disease, and Huntington disease, for which the organizations have established voluntary proficiency tests (see Box 3-2). Other organizations interested in the quality of genetic tests include the Organization for Clinical Laboratory Genetics, the American Society for Histocompatibility and Immunogenetics, the American Association of Blood Banks, the Technical Working Group on DNA Analysis Methods (TWGDAM), the National Reference System for the Clinical Laboratory, the National Committee for Clinical Laboratory Standards (NCCLS), and the new American College of Medical Genetics (ACMG). Many of the voluntary quality assurance programs grew from the efforts of the American Society of Human Genetics (ASHG) (Punnett, 1992).
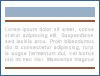
BOX 3-2
Voluntary Quality Assurance and Proficiency Testing Programs.
CORN was established in 1985 as a coordinating body for state genetic services programs organized in 10 regions. CORN is funded by the Genetic Services Program of the Maternal and Child Health Program, Health Resources and Services Administration (HRSA), U.S. Department of Health and Human Services (DHHS). Laboratory quality assurance quickly became and remains a high priority for CORN. CORN has a Quality Assurance Committee (for laboratory services), as well as other committees on quality assurance and proficiency testing, and education. CORN's national proficiency testing programs include alpha-fetoprotein, biochemical genetics, hemoglobinopathies and newborn screening, and most recently, DNA-based tests.
The College of American Pathologists developed guidelines, criteria, and methods for quality control and standards for clinical laboratories. CAP played a key role in the implementation of the Clinical Laboratory Improvement Act of 1967 (see below) when its quality assurance and proficiency testing standards and activities were recognized (''deemed") by the Secretary of Health and Human Services to fulfill the requirements of the law. Since 1967, CAP has worked to maintain itself and M.D.-pathologists as the appropriate professional group to judge the quality of clinical laboratories. CAP has voluntary proficiency testing programs for cytogenetics and MSAFP screening that are recognized by some states. With input from ASHG, CAP has spent two years developing guidelines for what it calls "molecular pathology." The ASHG role in setting standards for laboratory genetics will be assumed by the ACMG in 1993. ACMG laboratory standards for genetics are now under final revision and will be very important in quality assurance in genetic testing.
Although the Centers for Disease Control and Prevention attempted to establish its own cytogenetics proficiency testing program, it never progressed beyond a pilot program (Murphy, 1992a). CDC does run a voluntary proficiency testing program for newborn screening tests discussed below.
Costs, Benefits, and Limitations of State and Voluntary Quality Assurance Programs
In most state-run and voluntary programs, most of the costs of the quality control programs are paid by the participating laboratories. These costs could deter a research laboratory, or other small laboratory without cash reserves, from participating in the program. The costs of participation could be passed on to the consumer through higher laboratory prices, although the increases would not be a very large percentage of the current prices of most genetic tests. California now charges $40 for newborn screening and $55 for the MSAFP screening test, both of which include the cost of quality control and proficiency testing.
Rigorous state licensing provides protection to the citizens of that state, but continued reliance on the states will not afford equal protection to citizens of all states. Separate programs in each state could result in duplication of effort or in competing and, in some instances, conflicting laboratory standards. Many diagnostic laboratories do not now participate in voluntary quality assurance and proficiency testing in genetics. One incentive for such participation would be to publish the results of voluntary quality evaluations by laboratory name, as is now done by the National Tay-Sachs Disease and Allied Disorders Association, Inc., which publishes results of the quality assessment conducted by the International Tay-Sachs Disease Quality Control Reference Standards and Data Collection Center.
Federal Regulation of Clinical Laboratories
History
The Clinical Laboratory Improvement Act of 1967 (CLIA67) established federal control of laboratories providing more than 100 tests per year in interstate commerce for a profit. Only about 12,000 laboratories reimbursed by Medicare and Medicaid were covered. CLIA67 was originally administered by CDC, but authority was transferred to the Health Care Finance Administration in 1978. For laboratories under its purview, CLIA67 required the establishment of standards for laboratory directors and other laboratory personnel (CRS, 1990). The College of American Pathologists was recognized to set laboratory and personnel standards, and to develop a laboratory inspection system nationwide.
The Clinical Laboratory Improvement Amendments of 1988 (CLIA88) were enacted in response to rising concern over media reports of serious errors and variability in laboratory results, and inadequate training and supervision of personnel performing clinical laboratory tests. In particular, so-called Pap mills were found to have serious deficiencies in their cytology analysis of Papanicolaou tests, intended to detect cervical cancer. Public concern had also intensified about the quality of the increasing amount of unregulated laboratory services provided in physicians' office laboratories and other laboratories reimbursed by Medicare and Medicaid.
Laboratories Covered by CLIA88
With few exceptions, laboratories performing an "examination of materials derived from the human body for the purpose of providing information for the diagnosis, prevention, or treatment of any disease or impairment of, or the assessment of the health of, human beings" must obtain certification from HCFA under CLIA88 (Federal Register, 1992a). The exceptions are laboratories performing tests only for forensic purposes, research laboratories that do not report patient specific results for the purposes defined above, and laboratories of federal agencies to the extent excepted by the Secretary of Health and Human Services (Federal Register, 1992a). How a laboratory derives its revenues, or even whether it charges for tests, is no longer a determinant of coverage under CLIA88. Thus, any laboratory that provided a genetic test result on which a clinical decision was based is subject to regulation.
More than 200,000 laboratories are estimated to be covered by CLIA88, over 100,000 of them in physicians' offices. Compliance with CLIA88, including CLIA certification is required for Medicare reimbursement of laboratory services, and many other third-party payers use Medicare certification as one criterion for reimbursement. However, HCFA may not know about laboratories that do not obtain certificates unless there is a complaint.
CLIA88 Regulations
In implementing CLIA88 the government sets standards for laboratories according to whether the tests they perform are of "moderate" or "high" complexity. The distinction between moderate and high complexity depends on a number of factors including knowledge needed to perform the test, characteristics of operational steps, judgment required, and interpretation of results. Thus far, CDC has classified 10,000 tests, approximately 25 percent as high complexity and 75 percent as moderate complexity (as of January 1993).
Laboratories performing tests of moderate or high complexity must conform to general quality control standards as well as those of the "specialty" under which the tests they perform are classified. (Some specialties are microbiology, chemistry, pathology, and hematology.) Laboratories must participate in proficiency testing programs for each specialty in which they perform tests and for which proficiency programs have been established. By 1995, a laboratory that fails a proficiency test two consecutive times or two out of three times will be subject to sanctions and may not continue to perform that test under its CLIA certificate. Nor will it be eligible for Medicare reimbursement for that test (CDC, 1992). HCFA had approved 12 of the 19 proficiency testing programs that had applied as of December 1992. None of these is in genetics (see below).
The absence of a complexity rating for a test does not exempt a laboratory performing only unrated tests from quality control. A laboratory test whose complexity has not been categorized "is considered to be a test of high complexity until PHS (Public Health Service), upon request, reviews the matter and notifies the applicant of its decision" (Federal Register, 1992b). In the meantime, "the laboratory must have a system for verifying the accuracy and reliability of its test results at least twice a year" (Federal Register, 1992c). Moreover, all laboratories filing a certificate of registration with HCFA will be inspected every two years, and the laboratory's quality control and internal proficiency test system will be assessed. HCFA has a training program for laboratory inspectors—many of whom are sent by states—provided by the Department of Laboratory Medicine of the Johns Hopkins University School of Medicine. HCFA may soon deem other organizations (CAP, Joint Committee on Accreditation of Health Organizations, specific states) as capable of conducting its surveys and accrediting laboratories. State accreditation can supplant HCFA accreditation when a state's program is deemed equivalent or more stringent than the federal program.
Quality control under CLIA88 is funded by fees charged to laboratories. Compliance fees vary substantially from hundreds to thousands of dollars depending on how many different types of tests the laboratory performs, their complexity, and the volume of testing.
Genetic Tests Under CLIA88
Very few genetic tests are on the list of tests whose complexity has been defined under CLIA88. Those that are listed have been classified as of moderate complexity including sweat chloride for CF, creatine kinase, and alpha-fetoprotein (AFP) for tumor marker (Federal Register, 1992d). A test subspecialty called "clinical cytogenetics" has been established, but there is no other genetic test subspecialty under CLIA88. Moreover, no proficiency testing for cytogenetic laboratories is required, although well-established programs (e.g., New York State and CAP) have been operating for years. Proficiency tests are not required for any other genetic tests, either.
Few laboratories performing genetic tests as their sole or principal activity are yet complying with the CLIA88 regulations. Based on the committee's workshops and other information, it appears that few genetics laboratories have applied for certification from HCFA even though they provide genetic test information for clinical use. Committee staff also surveyed the directors of 12 genetics laboratories in academic centers to ask if their laboratory had applied for certification, and only I laboratory indicated that it had.
Research Laboratories and Tests for Rare Disorders
Research laboratories are covered under CLIA88 if they also provide tests on which clinical decisions are based. Some of these laboratories provide genetic tests as clinical services that are not directly related to the research they perform, and they may have limited expertise in performing or interpreting such tests. In many large academic hospitals, the central laboratory is not even aware of all the laboratories that provide services. This situation could be rectified either by having these laboratories obtain their own certificates from HCFA or by having them (and the tests they perform) listed under the central laboratory's certificate. Although this would bring them under the purview of HCFA inspectors, it might not ensure quality. It is doubtful that external proficiency tests could be provided economically or efficiently for tests performed only occasionally by many laboratories or that occasional performance of a test can ensure high quality.
To ensure high quality in tests for rare disorders that are seldom performed, the genetics community, under CORN and the American College of Medical Genetics, could take the lead in fostering centralization of these tests. Once centralization occurs and the volume of specific tests performed by the central laboratory increases, a stronger argument can be made that external proficiency testing is economical and efficient. With the establishment of central laboratories, HCFA could consider setting a volume-of-test requirement necessary to ensure quality (as FDA has proposed for MSAFP testing). Laboratories not meeting that requirement would not pass inspection for that particular test. If challenged in court, however, such an arrangement might be considered a restraint of trade. Commercial laboratories, which are often subsidiaries of biotechnology companies, are expanding their range of services and contracting with hospitals, including some academic centers, to take over genetic testing functions.
Interpretation of Laboratory Test Results
For the reasons given earlier in this chapter, the misinterpretation of test results is more likely in genetic tests than in many other areas of clinical testing, and misinterpretation may have more serious consequences particularly in fetal diagnosis. The need for adequate interpretation is even greater when the health care providers ordering tests (1) are not expert in interpreting probabilistic clinical data (Holtzman, 1991); (2) are not expert in genetics; and (3) may not be aware of the implications of both positive and negative test results in genetics. As discussed further in Chapter 6, an increasing proportion of genetic tests will be ordered by primary care physicians, many of whom have limited knowledge of genetics (Hoffman, 1991; Holtzman et al., 1991; Hofman et al., 1993). Special concerns about interpretation also arise when tests may be provided by employers as part of fitness programs or by other organizations not skilled in genetics.
Interpretation can be very complex and can depend on the results of other family members. One way of ensuring that physicians who order tests understand the implications and limitations of results is to require that the laboratory performing the test provide adequate interpretation of the result in its report (Hommes, 1992), although all genetic testing laboratories may not be prepared to provide this service. With few exceptions the interpretation of a positive genetic test result should include the chance of future disease or the chance of having an affected child. In the latter case, the interpretation should note whether the chance is dependent on the carrier status of the mate of the person tested. If misattributed paternity might alter the interpretation, this should also be disclosed. The interpretation of test results should also indicate any confirmatory tests that are available and whether repeat or other follow-up testing is recommended. It might summarize the possible interventions. Interpretation of a negative test result should include the revised estimate of the chance of occurrence and make clear that the test result pertains only to a specific disorder, and within that disorder only to disease-causing mutations detectable by the test, which may not include all such mutations.
No matter how extensive or clear the laboratory's written interpretation, many individuals and couples with positive test results will benefit from genetic counseling as well. Some laboratories employ, or are affiliated with, genetic counselors or other personnel trained in genetics who can discuss test interpretation with referring physicians.
ENSURING THE SAFETY AND EFFECTIVENESS OF NEW GENETIC TESTS
FDA regulates medical devices under the Medical Devices Act of 1976 and the Safe Medical Device Amendments of 1990. This legislation gives FDA the authority to regulate an "in vitro reagent, or any other similar or related article, including any component, part or accessory, which is: intended for use in the diagnosis of disease or other conditions in man" (21 CFR 201(h)). Through classification of such "devices," FDA implements the legislation's intent to provide a ''level of regulation necessary to afford reasonable assurance of safety and effectiveness of the device" (Tsakeris and Yoder, 1992, p. 2). Safety cannot be considered entirely separately from effectiveness. If a test cannot properly distinguish those at high risk of genetic disease from those at low risk, individuals who are mislabeled will suffer harm. False negatives will not be treated, while false positives may suffer from both unnecessary intervention and anxiety.
Only a small proportion of genetic tests in widespread use have been reviewed by FDA; these include tests for hypothyroidism, phenylketonuria (PKU), and MSAFP tests. The technologies used for the detection of most disease-related genotypes have not been submitted to FDA; these include biochemical reagents and DNA probes used in tests for CF, Huntington disease, muscular dystrophy, fragile X syndrome, and other disorders.
Premarket Approval of Medical Devices
Before a medical device can be legally marketed for in vitro diagnostic use, its sponsor (manufacturer, university, or individual scientist) must obtain premarket approval (PMA) or clearance (Tsakeris and Yoder, 1992). On receiving premarket notification from a sponsor, FDA will determine whether the device is "substantially equivalent" to a legally marketed "predicate" device. "Substantial equivalence means the new device must have the same intended use as the predicate device, the same technological characteristics (or, if different,) strong comparability of intended use and performance characteristics, and [not raise] new questions of safety and effectiveness" (Tsakeris and Yoder, 1992, p. 3). For the FDA to make a determination of substantial equivalence, the manufacturer has to demonstrate equivalent performance characteristics (as good as other legally marketed devices). If so, FDA will give the device either Class I or II designation, depending on the class of the predicate device. The safety and efficacy of Class I devices can be reasonably ensured if the manufacturer adheres to general controls that include good manufacturing practices in their production. Class II devices must meet not only general controls, but also special controls, in order to reasonably ensure safety and efficacy.
If FDA fails to find the device substantially equivalent, it will require full premarket approval, designating it a Class III device. This requires the manufacturer to present evidence of the device's safety and effectiveness. Genetic tests and devices placed in Class II include MSAFP kits (MSAFP is not approved by the FDA for use as a screening test for Down syndrome, although it is widely used for that purpose) and kits for the Philadelphia chromosome, tumor markers, and gene rearrangements. Class II tests include the sweat chloride test for CF, creatine kinase test for Duchenne muscular dystrophy, phenylalanine test for PKU, tests for coagulation defects (including detection of carriers of coagulation defects), and tests for sickle cell trait or disease. The copper test for Wilson disease is Class I (Tsakeris and Yoder, 1992).
The committee reviewed FDA's current definition of "substantial equivalence." Although the current definition (Tsakeris and Yoder, 1992) is reassuringly comprehensive, the FDA could interpret substantial equivalence too broadly, permitting a manufacturer to avoid the premarket approval process by claiming, for instance, that a DNA test marketed to detect sickle cell carriers was substantially equivalent to electrophoretic tests already on the market. Once marketed to detect carriers, the DNA test, unlike the electrophoretic test, could also be used for prenatal diagnosis. This would constitute an unapproved or "off-label" use of the device. Similarly, a DNA-based test for some CF mutations might be approved for the diagnosis of CF on the basis of substantial equivalence to the sweat chloride test, although only the DNA test could be used for carrier detection or prenatal diagnosis.
Despite the FDA's current definition of substantial equivalence, off-label use once a test is legally marketed remains an area of concern. Off-label use often goes undetected or unreported, although if manufacturers are aware of off-label use of a device, they must either withhold the product from the user (laboratory) or file a premarket notification of the new use. To prevent the problem of offlabel use of genetic tests, FDA should require the manufacturer to indicate both intended and potential uses (which would include carrier screening, prenatal diagnosis, or presymptomatic testing, etc.). Increasing FDA's capability for postmarket surveillance would also reduce off-label use.
Collection of Data for Test Validation
The general principle that premarket data on test validity should be collected on subjects drawn from the same population as the intended targets of the test, after it is marketed, is often difficult to adhere to in the case of genetic tests. An underlying problem is that no confirmatory ("gold standard") test will be available. This will be the case for many direct tests that search for underlying diseasecausing mutations. Only the appearance of the disease itself provides confirmation. With tests for rare diseases and diseases with a long time lag between the time of the test and the appearance of disease, it may not be feasible to determine sensitivity on subjects of the type in whom the test is intended to be used (e.g., asymptomatic, healthy subjects). Subjects with overt disease can be used to test sensitivity, and nonaffected subjects beyond the age at which the disease usually becomes manifest can be used to assess specificity. Because the age of such subjects will often be considerably older than those in whom such tests can be of value, the findings of such "shortcuts" may not be accurate predictors of sensitivity and specificity under conditions of intended use. Long-term follow-up is, therefore, a better means of assessing the validity of such tests. The assessment of the validity of tests for genetic predispositions will also require long, careful follow-up.
With tests intended for prenatal diagnosis in which only the fetal test result indicates the presence or absence of disease (i.e., there is no independent test or histopathological evidence to confirm the finding), validation will depend on test results in living subjects. In addition, when the parents elect to continue the pregnancy after a positive prenatal diagnostic test, the infant must be followed to determine whether the disease appears. Infants delivered after a prenatal diagnostic test was negative should also be followed, with a similar objective.
For tests for which a new treatment is being assessed in conjunction with test validation, a plan to withhold treatment from some subjects with positive test results is needed (e.g., randomized controlled trials). Otherwise it will be impossible to distinguish a person with a false positive result, who would never get sick, from a true positive in which the treatment was efficacious. When the false positive rate of a test is unknown, treating all people with positive results with an effective therapy will make it impossible to determine the specificity of the test unless the response to the therapy can distinguish true from false positives.
Humanitarian Exemptions
The Safe Medical Device Amendments of 1990 contains a "humanitarian device exemption" for conditions affecting less than 4,000 individuals in the United States. The safety of medical devices must be demonstrated prior to premarket approval, but demonstration of effectiveness can be postponed for 18 months. To qualify, no comparable devices to treat or diagnose the condition can be available and the probable benefit to health must outweigh the risk of illness or injury from its use. Such exempted devices may not be sold for more than the cost of research, development, fabrication, and distribution, and may only be used in institutions with local institutional review boards (IRBs), which approve the clinical use of the device and supervise its clinical testing. Proposed regulations were issued for this provision on December 21, 1992 (Federal Register, 1992e).
Although such an approach recognizes the difficulty of establishing the effectiveness of devices that will only be used occasionally (e.g., in diagnosing rare disorders), the 18-month delay will be insufficient to establish the effectiveness of many genetic tests for the reasons already noted. More importantly, manufacturers may be unwilling to go through premarket approval of tests for rare diseases because of their limited marketability. The Orphan Drug Act does not provide the incentives (including exclusivity for patents and tax credits) for development of medical devices that it does for drugs for orphan diseases. It does, however, offer grants for the development of devices, as well as for medical foods for rare diseases; $10 million is allocated for this purpose in fiscal year 1993 for grants of $100,000 to $200,000 per year for a period of two to three years for clinical tests of such products for rare diseases.
Investigational Use of New Devices
The collection of data to support PMA applications—that is, to determine safety and effectiveness—is the responsibility of the sponsor. To obtain these data the sponsor is allowed to use the device (including DNA probes and reagents) only for investigational purposes. Under FDA regulations, the device must be labeled for "investigational use only" and must be used in accord with a protocol (including specified period of study, number of sites, investigators, and patient samples) and must be approved by an IRB. Informed consent of participants in such studies may be required. This would always be the case if the participant was to be notified of the result or if a clinical decision was based on the result. Under "investigational use" exemptions, manufacturing costs can be recovered by charging for the device, but no profit can be made.
Under current FDA rules, an investigational "device and/or its results will not be used for diagnostic purposes without confirmation of the diagnosis by another, medically established diagnostic device or procedure" (R. Johnson, FDA, letter to device manufacturers, October 17, 1991, p. 3), unless an investigational device exemption has been approved by the FDA. The committee notes that, for many genetic tests involving direct determination of disease-causing mutations, the only confirmation that is possible is the clinical appearance of the disease, which may not happen until many years after the test is performed. In reply to the committee's inquiries, FDA wrote that obtaining an "approved Investigational Device Exemption (IDE) may be required for certain genetic products (e.g., when there is no confirmatory test) to assure the safe use of the device in collecting data to establish the performance characteristics of the device [emphasis added]" (Tsakeris and Yoder, 1992, p. 12).
Institutional Review Boards and Genetic Tests
The submission of protocols to IRBs for investigations covering such devices may overwhelm some IRBs. At some large academic centers, IRBs are appointing subcommittees to deal with new genetic investigations. Most IRBs will not have experts in genetics or individuals knowledgeable about ethical problems in genetic testing. The National Human Genome Research Center at the University of Iowa has created a unit to advise IRBs and other groups on ethical, legal, and social issues arising in genetics research.
Inappropriate Use of Investigational Devices
At some point in their development, genetic tests emerging from research laboratories in academic medical centers are offered as a clinical service. Research laboratories sometimes perform these tests at their convenience, with delays of months in reporting results (Hoffman, 1991; Klinger, 1992). If no manufacturers are interested in commercializing the tests as in vitro diagnostic devices, the research laboratory continues to provide them as a service. In many instances, academic research laboratories do not comply with investigational-use device requirements of FDA, despite the fact that performance of the test for clinical purposes requires them to do so.
FDA is aware of the problem of the use of medical devices that it has not reviewed for marketing and that are not in compliance with its regulations regarding investigational devices. One example is the use of the MSAFP test (which has FDA approval for detection of increased risk of fetal neural tube defects) for detection of increased risk of Down syndrome in the fetus (for which the test is not approved). FDA has proposed to deal with such problems by compiling an "accommodation list" of such tests that it believes to be essential to clinical care. Manufacturers or other sponsors of the tests on this list would be allowed an additional 30 months to collect data for premarket approval applications. Tests not on the list can no longer be legally provided unless they immediately comply with FDA requirements (FDA, 1992). ACMG has pointed out to FDA that the number of genetic tests in this category is very large and that the elimination of tests that are not listed will cause serious problems to patients with, or at risk for, genetic disorders (M. Watson and M. Cohen, letter to F. Yoder, FDA, August 18, 1992). Because many new genetic tests will be for rare diseases, the collection of adequate data to establish safety and effectiveness will take a long time, probably longer than the 30-month time limit given by FDA for devices on an accommodation list. For tests performed only occasionally, "provisional premarket approval" should be given (see below). Once granted, this will permit laboratories to charge a fair price for the test. This should serve as an incentive to accelerate data collection. Data collection can also be speeded by enabling investigators to collaborate, pooling their data. For very rare diseases, commercial sponsors of such collaboration are unlikely to be forthcoming. Support for collaborative studies will greatly speed the process of making these tests available to the public, and long-term support may be needed to conduct some of these studies.
NEWBORN AND OTHER GENETIC SCREENING PROGRAMS
An estimated 4 million screening blood specimens from heel-sticks of newborns are tested annually in the United States for at least one genetic disorder. Many of these tests are performed in state laboratories; some state laboratories subcontract work to commercial laboratories (Table 3-1). CDC provides the only proficiency testing program for newborn screening in the United States. For 39 state and territorial and 28 private laboratories participating in proficiency testing for newborn screening, CDC absorbs the cost of the testing program.
TABLE 3-1
Number and Types of Laboratories Providing Newborn Screening Tests.
Participation in the program is voluntary; 115 organizations participate, including manufacturers and international laboratories, 39 state and territorial laboratories, and 28 private laboratories. Data on the quality of programs participating in CDC's voluntary program show substantial error rates, in excess of 5 percent false negatives (Holtzman et al., 1986; Adam and Hannon, 1992). CDC has consulted HCFA concerning approval of the CDC newborn screening proficiency testing program as a provider of proficiency testing under CLIA88; at present, CLIA88 regulations cover only one of the ten newborn screening tests, hypothyroidism (using thyroxine and thyroid stimulating hormone) (B. Adam, CDC, personal communication, January 1993).
Errors in screening for genetic disorders may also be greater than for other tests because it entails the testing of many people who will not have the condition being screened for. This is true in carrier and prenatal as well as newborn testing. When tests of high sensitivity and specificity are used in populations in which the condition being screened is of low prevalence, the predictive value of the result may be low. If, in addition, laboratory error adds substantially to the number of false negatives and false positives, the safety and benefit of screening come into question. This concern is all the more important because unlike many other medical procedures, it is usually not the patient who initiates the process but the health care provider or the state (in the case of newborn screening). This increases the possibility that the person being tested could misunderstand the objectives of testing and misinterpret the results. Errors in screening may also be greater than in other medical tests because many specimens are handled simultaneously and may be analyzed at a site far removed from where they were obtained. Thus the entire screening process, from informing the patient (or parent) through final disposition (giving the patient or parent the result or placing it on the patient's record), should be subject to quality control.
The regulation of laboratories under CLIA88 can only ensure that performance of the tests is as good as the intrinsic validity of the test. It does not address the inherent safety and effectiveness of the screening tests themselves. None of the current newborn screening tests has gone through the FDA's full premarket approval process. Tests for PKU and congenital hypothyroidism (for which all 50 states screen), sickle cell anemia (for which 42 states screen and 11 other states have limited screening or pilot studies), and galactosemia (for which 39 states screen) have been classified as Class II by FDA. It seems probable that the FDA has not even been notified of many of the "in vitro diagnostic devices" used in state laboratories for screening because they are not marketed outside that laboratory. It is also doubtful that in developing these devices, manufacturers (companies or individuals) have followed FDA requirements for investigational device exemption and use. Recently, mandatory newborn screening for CF has been undertaken in Colorado and Wyoming (Hammond et al., 1991). As far as the committee could determine, the immunoreactive trypsin assay used for CF screening by these states has not been submitted to FDA for premarket review for use in CF screening. An interesting policy dilemma arises if the organization performing the pilot study (e.g., a state laboratory) has no plans to market the test. It would then have no need to apply to FDA for premarket approval and could continue to handle the device as investigational even after screening becomes routine.
FINDINGS AND RECOMMENDATIONS
Ensuring the Quality of Laboratories
The safety and effectiveness of genetic tests must be established before these tests are used routinely and, once that comes to pass, great care must be taken in performance of the tests and interpretation of the results. Laboratory quality control falls into three areas:
- The training and experience of the laboratory personnel: The nature of genetic tests and the implications of their interpretation suggest the need for special requirements for supervisory personnel (laboratory directors and technical supervisors) in laboratories involved in genetic testing.
- The structure and function of the laboratory itself: This requires inspectors with special training in assessing such laboratories.
- Proficiency testing: The most rigorous type should be conducted whenever possible, that is, external, blind proficiency testing in which an outside agency sends specimens to the laboratory under a fictitious patient's name. The laboratory has no way of knowing that the specimen is for assessing laboratory quality. Other types of proficiency testing involve the sending of coded samples from a central source. Proficiency testing should extend to all genetic laboratory tests and to the interpretation provided by the laboratory to referring physicians.
Voluntary quality control programs have helped to establish criteria and standards for genetics laboratories and laboratory personnel. The committee finds the current state of voluntary laboratory quality control programs in human genetics to be beneficial, but generally inadequate to address the special issues posed by genetic testing, because these programs lack essential enforcement authority. The impact of these voluntary programs should be strengthened by the publication of the names of laboratories that have satisfied the proficiency and other requirements. Before names are withheld from a published list of "quality" laboratories, any laboratory not satisfying these requirements should be given an opportunity to rectify its deficiencies.
The clinical implications of commonly performed prenatal tests, particularly the abortion of presumably affected fetuses, warrant that laboratories performing them participate in proficiency testing programs.
The performance standard for genetic testing should be as close tozero error as possible. Laboratories with any error in proficiency testing should be placed on probation, with proficiency testing repeated using blinded methods. Unless the laboratory can attain the required standard in performing and interpreting any genetic test, its certification to perform that test should be removed.
The existing CLIA88 regulations could ensure the quality of genetic laboratory testing were they to be fully implemented and applied to genetic testing. Action by DHHS would help to ensure the quality of the most frequently performed genetic tests by (1) establishing genetics as a subspecialty under the CLIA88 regulations; (2) rating specific genetic tests for complexity; and (3) requiring proficiency testing for genetic tests.
The first step would be to require all laboratories providing any genetic test to obtain a certificate from HCFA. Next, would be development of a system for verifying the accuracy and reliability of the tests they perform. Third, laboratories performing genetic tests would be subject to inspection every two years. To make this approach meaningful, the laboratory inspectors would have to be well versed in the unique aspects of genetic tests, including the interpretation included in the report of results. A specific training unit on genetic testing should be included in HCFA's educational training program for inspectors, and the same training should be required of inspectors in agencies deemed by HCFA capable of performing inspections. In addition to these steps, HCFA could determine that existing proficiency programs for genetic tests satisfy its standards; HCFA could deem these proficiency testing programs to be required by laboratories providing those tests until such time as other programs are developed (see previous section on voluntary programs and Box 3-2 in this chapter). A laboratory that failed proficiency testing in a "deemed" program would be subject to HCFA sanctions even if that program was voluntary and did not itself impose sanctions.
It is doubtful that adequate quality control can be ensured with voluntary proficiency testing. This holds for newborn and other types of screening as well. As with any genetic testing, participation in proficiency testing programs should be required of laboratories providing newborn screening tests. This could be accomplished by designating genetic screening tests as moderate or high complexity under CLIA88. HCFA should examine the proficiency testing programs for genetic screening tests described earlier in this chapter and, if they meet its criteria, deem them acceptable.
The committee recommends that HCFA create a new specialty of clinical genetics into which it can incorporate the existing subspecialty of clinical cytogenetics, and also create new subspecialties of biochemical and molecular genetics. MSAFP testing and other methods of prenatal testing for birth defects should be incorporated into one of these three subspecialties. Most genetic tests should be classified as high complexity under CLIA88, primarily to ensure that supervisory personnel have adequate training in genetics. Although genetic tests might someday be simple enough to be feasible for home use, the difficulties of interpreting results would still render them of high complexity.
Within its existing authorities, HCFA can take steps to enhance the quality of tests for rare disorders, for which specific requirements may never be established. According to a HCFA representative, requirements for proficiency testing are unlikely to be established for low-volume tests unless their clinical or public health implications are high (J. Yost, HCFA, personal communication, January 1992). The establishment of such requirements could be costly both to HCFA and to laboratories that must participate.
The committee strongly recommends that the genetics community, under the leadership of its professional societies, designate a small number of laboratories as centralized facilities for tests for rare disorders . These organizations should establish and publicize a register of the tests performed by these central laboratories and encourage referral of specimens to them. The register should be easily accessible to a wide range of health care providers. It could also be included in the data bases of the National Library of Medicine. An external proficiency testing program should be established for the central laboratories. The genetics community should also study the possibility of setting a minimum volume of a genetic test that a laboratory must perform annually in order to obtain certification for that test and ensure the quality of test performance. With its informatics and data base capabilities, the National Library of Medicine should maintain a data base of centralized laboratories performing tests for rare disorders, genetic counseling centers, and support groups, which should be available to laboratories and providers at no charge.
HCFA and CDC should give high priority to requiring established proficiency programs for genetic tests. The New York State program can be considered a model for many genetic tests, although high priority should also be extended to setting specific requirements for other frequently performed prenatal tests. HCFA should incorporate standards and procedures for assessing genetic tests in its training programs for current and new laboratory inspectors.
Laboratories in academic health centers and elsewhere that conduct research, but that also perform genetic tests as a service (providing the results to referring laboratories or physicians, or directly to patients), should be subject to the same criteria, standards, and regulation as commercial genetic testing laboratories since they fall under the purview of CLIA88. The committee recommends that HCFA inform all hospitals of their legal responsibilities to register with HCFA every laboratory that provides results used in clinical decisions to physicians or patients.
The committee recommends that genetics laboratories provide reports in an easily understandable form for referring physicians who are not genetic specialists. These reports, including interpretation of the results, should be reviewed by HCFA as part of its inspection of laboratories performing genetic tests.
To ensure that physicians and patients receive consistent and accurate information, ''package inserts" (information and instructions both for physicians and patients) should be provided by the manufacturer of genetic test kits through the laboratory from which the physician orders the test.
Ensuring the Safety of New Tests
Because genetic tests seldom will have perfect sensitivity or specificity, particularly when used for predictive purposes, because of the novelty of some genetic testing technologies, and because of the possibilities of misinterpretation of test results, full premarket approval is needed for all new genetic tests; that is, genetic tests should be in Class III. The concerns of the committee will be addressed only if FDA uses criteria for review that address these issues.
In its recent reply to the committee's inquiries, FDA indicated (Tsakeris and Yoder, 1992, p. 8) that a sponsor may be asked to provide information that includes when appropriate
- analytic sensitivity/limits of detection;
- analytic specificity/cross-reactivity/interference studies;
- accuracy studies;
- precision studies;
- reportable range;
- clinical sensitivity and specificity; and
- stability data.
The committee believes that data on all of these areas should be provided in premarket approval submissions for genetic tests. Specifically, sponsors should present evidence of sensitivity in terms not only of the ability of the test to detect specific mutations (analytic sensitivity) but of the proportion of people with clinically significant disease that are detected by the test (i.e., who have the specific mutation detected by the test; clinical sensitivity). One area of concern, for example, is the possibility of FDA approval of a (hypothetical) test kit for CF carriers that detects six mutations and might have 100 percent analytical sensitivity, but only 85 percent clinical sensitivity. The determination of an acceptable clinical sensitivity should be on a test-by-test basis, by taking into consideration not only the benefits of making correct predictions but the risks of making wrong ones.
The FDA should develop guidance to manufacturers for preparing premarket applications for genetic test devices. The application should include
- the intended and potential use(s) of the test (e.g., presymptomatic diagnosis or prediction, carrier screening, prenatal diagnosis);
- for each intended use, data on the sensitivity and specificity of the test, with clinical manifestations serving as an end point in the absence of a "gold standard" test;
- procedures to be used by clinical laboratories to demonstrate their reliability (precision and accuracy) and proficiency in performance of the test; and
- description to be given to health care providers and to patients regarding the objectives of the test and the interpretations of negative or positive findings.
For very rare diseases, it may take a long time to collect sufficient data on specificity and sensitivity. For diseases of late onset, a long lag will occur between the time of the test and the appearance of disease, and it will be difficult for applicants to provide adequate data on safety and effectiveness for subjects of the type in whom the test would be applied (e.g., presymptomatic individuals). It may also be impossible to assess the sensitivity and specificity of prenatal tests by independent tests or histopathological examination of aborted fetuses. In all such cases, the committee recommends that data of the type described in the section "Collection of Data for Test Validation" be required. If these preliminary data suggest that the test is safe and effective for its intended use, the FDA should grant the applicant "provisional premarket approval" in order not to unduly delay submission for PMA. Under this category, the test could be made more widely available, but the manufacturer would be responsible for obtaining and submitting additional periodic postmarket data of an adequate sample of subjects, until sufficient data are available to warrant full premarket approval. These data would be collected from patients' physicians either directly by the manufacturer or by the laboratories to whom it sells the device. The protocol used in the investigational phase (before provisional approval) would still apply, and informed consent would still be needed.
Once provisional premarket approval has been granted, however, the laboratories performing the test could charge a fair market price for the device or test kit. The committee recommends that people receiving the test during this provisional period be informed that the safety and effectiveness of the test have not been fully determined. If the manufacturer is to contact physicians directly, testees must be informed if the manufacturer will have their names. Once provisional premarket approval is granted, manufacturers should be allowed to charge a market price for the test. This process may require new legislation.
Provisional premarket approval, with periodic postmarket study for which the sponsor would be responsible, could also be used to cover the development of tests for rare conditions (which is the intent of the humanitarian device exemption). The committee therefore recommends that Congress consider the need for legislation in the spirit of the Orphan Drug Act that would give manufacturers the incentive to develop diagnostic medical devices for genetic tests of limited marketability.
The appearance of clinical disease is the only possible confirmation of many genetic tests, and that may not occur until many years after testing. Investigators should be permitted to convey the results of investigational tests to subjects who are aware of the investigational nature of the test. Thus, clinical decisions could be made on the basis of the results as long as the investigator has an approved investigational device exemption. FDA should make it clear that in such cases, with an approved IDE, results could be communicated to the patient or to his or her physician so that interventions can be instituted accordingly.
Since many IRBs are not experienced in the review of investigational genetic testing protocols, the committee recommends that the National Institutes of Health (NIH) Office for Protection from Research Risks (OPRR) and the National Center for Human Genome Research Ethical, Legal, and Social Implications (ELSI) Program coordinate efforts to assist IRBs in coping with this responsibility, and ELSI should consider supporting efforts to assist IRBs in this task. Other federal agencies (such as the FDA) and professional groups should also consider developing guidelines to help IRBs cope with this added responsibility (such as the informed consent guidelines for research involving genetic testing developed by the Alliance of Genetic Support Groups and the ASHG). The formation of a national advisory body on genetic testing could also be helpful in educating IRBs concerning research involving genetic testing (see Chapter 9). IRBs used by commercial organizations should also have a broad, unbiased membership.
Compliance with FDA requirements for premarket approval and approved investigational device exemption is essential to ensuring safe and effective use of a genetic test, just as compliance with CLIA88 is essential for any laboratory performing genetic tests for clinical purposes. The committee recommends that the FDA publicize widely to potential sponsors, including academic centers, that DNA probes and other reagents essential to the performance of genetic tests are medical devices. Consequently, whenever they are used for clinical purposes, genetic test devices (i.e., test kits, reagents, probes, etc.) either must be labeled "for investigational use only" (and must comply with FDA requirements for such use) or must have been approved or or cleared for marketing by FDA. When genetic test devices are used investigationally for clinical purposes, manufacturers—including commercial or academically based laboratories preparing their own devices—should apply for FDA approval of an investigational device exemption, including an IRB approved protocol, and periodic reports on the results of their investigations.
To speed the widespread availability of investigational devices of limited marketability, FDA should grant provisional premarket approval, as described earlier, when adequate preliminary evidence of safety and effectiveness has been collected. In addition, the NIH and private funding agencies should support meritorious studies designed to assess the safety and effectiveness of investigational genetic testing devices. To speed the collection of data on tests of limited marketability, national collaborative studies should be encouraged. Funding agencies should also support long-term studies on safety and effectiveness of genetic test devices (through the phase of provisional premarket approval) for diseases in which a long lag will occur between the time of the test and the clinical appearance of the disease.
The committee recommends that all genetic tests should either be designated as investigational devices—subject to IRB approval and FDA regulation—or be submitted to the FDA for premarket approval. FDA should clarify that when an approved IDE is obtained for a test for which no independent confirmatory test is available, the results may be given to the patient's health care provider or to the patient. When a device with an approved IDE is used to provide clinical information, the laboratory performing the test should be allowed to charge for the costs of testing, record keeping, and complying with reporting requirements. Because the investigational phase of new genetic tests may be prolonged, the laboratories performing these tests should be subject to external quality assessment. The committee recommends that HCFA inform every organization (e.g., academic health center or other hospital) that all laboratories in which investigational devices are being used for genetic testing are covered by CLIA88 and must register with HCFA to obtain CLIA88 certificates and be inspected.
The FDA has taken important first steps to increase the pool of advisors expert in genetics by inviting applications for service as FDA advisors ("special government employees") under its Clinical Chemistry and Toxicology Devices Panel of Experts. The agency should also consider developing workshops on critical aspects of genetic testing technology for manufacturers and clinical laboratories.
The committee is concerned that screening tests may become routine standard of care without adequate studies of their safety, effectiveness, or clinical utility. To ensure that adequate studies are conducted, the committee recommends that any new screening test should comply with FDA rules regarding investigational devices, including a protocol reviewed by an IRB. The committee recommends that the decision to move from "pilot" or "investigational" use to rou tine practice involve review of data collected in the pilot study and elsewhere by both FDA and a policy-making body, usually at the state level, that is independent of the organization directly responsible for conducting the pilot study. A national oversight body (see Chapter 9 ) could facilitate collection and dissemination of data from pilot "investigational" studies. An adequately conducted pilot study in one or a few states need not be repeated in others as long as the other state(s) can maintain the same standards of the pilot study in routine operation. The committee also recommends that some mechanism be found to resolve the dilemma posed by the need to demonstrate that the device is safe and effective for its intended use, whether or not it will be commercially marketed.
The preceding sections, as well as other chapters in this report, indicate that genetic tests for screening and other purposes differ in many respects from other laboratory tests. Some federal agencies, particularly FDA, have recognized this by planning special guidance for manufacturers of genetic tests and by inviting geneticists to participate on advisory groups. The committee welcomes such activity and encourages other agencies to do likewise. In particular, the committee recommends a Genetic Device Advisory Panel to provide FDA with continuing and timely access to expert advice. In addition, the Clinical Laboratory Improvement Advisory Council should appoint a subcommittee on genetics to make recommendations on improving the quality of laboratories performing genetic tests under CLIA88.
REFERENCES
- Adam, B., and Hannon, W.1992 (published in 1994). The Centers for Disease Control's infant screening quality assurance program: Overview, accomplishments, and initiatives. In Fullarton, J. (ed.) Proceedings of the Committee on Assessing Genetic Risks. Washington, D.C.: National Academy Press.
- Benn, P., et al. 1992. A rapid (but wrong) prenatal diagnosis. New England Journal of Medicine326(24): 1638-1639. [PubMed: 1584278]
- Centers for Disease Control (CDC). 1992. Morbidity and Mortality Weekly Report 41 (RR-2), February 28. [PubMed: 1538689]
- Congressional Research Service (CRS). 1990. Clinical Laboratory Improvement Amendments of 1988. Washington, D.C.
- Collaborative Research Group for Huntington's Disease. 1993. A novelgene containing a trinucleotide repeat that is expanded and unstable on Huntington's disease chromosomes. Cell72:971983. [PubMed: 8458085]
- Council of Regional Networks for Genetic Services (CORN). 1992. Newborn Screening Report: 1990 (Final report, February 1992).
- Cunningham, G.1992 (published in 1994). California newborn screening program. In Fullarton, J. (ed.) Proceedings of the Committee on Assessing Genetic Risks. Washington, D.C.: National Academy Press.
- Federal Register. 1992a. 57 (40), February 28, 1992, Sec. 493.2, p. 7139; Sec. 493.3, p. 7140.
- Federal Register. 1992b. 57 (40), February 28, 1992, Sec. 493.17{C}{4}, p. 7141.
- Federal Register. 1992c. 57 (40), February 28, 1992, Sec. 493.1709, p. 7184).
- Federal Register. 1992d. 57 (131), July 8, 1992.
- Federal Register. 1992e. 57 (60491); 21 CFR 812, Docket 91N0404.
- Food and Drug Administration (FDA). 1992. Draft "accommodation list" for device tests essential to clinical practice, but not approved by FDA for those uses. Washington, D.C. (personal communication of draft for comment, May 3, 1992).
- Haddow J., and McKnight, G.1992 (published in 1994). Quality of genetic laboratory procedures in the United States. In Fullarton, J. (ed.) Proceedings of the Committee on Assessing Genetic Risks. Washington, D.C.: National Academy Press.
- Hammond, K., et al. 1991. Efficacy of statewide neonatal screening for cystic fibrosis by assay of trypsinogen concentrations. New EnglandJournal of Medicine325:769-774. [PubMed: 1870650]
- Hannon, W., and Adam, B.1991. Identified problems found in the voluntary newborn screening proficiency testing program conducted by the Centers for Disease Control. In An overview of the national infant screening quality assurance program: Update and future directions. In Proceedings of the Eighth Annual Neonatal Screening Symposium, Saratoga Springs, N.Y., April.
- Hoffman, E.1991. Presentation at the Conference on Biotechnology and the Diagnosis of Genetic Disease: Forum on the Technical, Regulatory and Societal Issues. Program on Technology and Health Care, Department of Community and Family Medicine, Georgetown University Medical Center. Washington, D.C., April.
- Hofman, K., et al. 1993. Physicians' Knowledge of Genetics and Genetic Tests. Academic Medicine68(8):625-631. [PubMed: 8352875]
- Holtzman, C., et al. 1986. PKU newborn screening in descriptive epidemiology of missed cases of phenylketonuria and congenital hypothyroidism. Pediatrics78(4):553-558. [PubMed: 3763262]
- Holtzman, N.1991. The interpretation of laboratory results: The paradoxical effect of medical training. Journal of Clinical Ethics2(4): 1-2. [PubMed: 1804393]
- Holtzman, N.1992. Testimony on genetic testing before the Subcommittee on Human Resources and Intergovernmental Relations, Committee on Government Operations, U.S. House of Representatives, July 23.
- Holtzman, N., et al. 1991. Effect of education on physicians' knowledgeof a new technology: The case of alpha-fetoprotein screening for neural tube defects. Journal of Clinical Ethics2(4): 1-5.
- Hommes, F.1992 (published in 1994). Laboratory quality assurance efforts in biochemical genetics. In Fullarton, J. (ed.) Proceedings of the Committee on Assessing Genetic Risks. Washington, D.C.: National Academy Press.
- Hommes, F., et al. 1990. Documented errors and improvements in biochemical genetic testing. In Proficiency testing for biochemical genetics laboratories: The first ten rounds of testing. American Journal of Human Genetics46:1001-1004. [PMC free article: PMC1683598] [PubMed: 2339686]
- House Subcommittee on Human Resources and Intergovernmental Relations. 1992. Committee on Government Operations, U.S. House of Representatives, hearing on genetic testing, July 23.
- Hoyt, H.1992. Testimony on genetic testing before the Subcommittee on Human Resources and Intergovernmental Relations, Committee on Government Operations, U.S. House of Representatives, July 23.
- Kaback, M., et al. 1977. Tay-Sachs disease heterozygote detection: A quality control study. TaySachs disease: Screening and prevention. In Kaback, M. (ed.)Progress in Clinical and Biological Research18:267-277. [PubMed: 601080]
- Klinger, K. 1992 (published in 1994). New developments in genetic testing. In Fullarton, J. (ed.) Proceedings of the Committee on Assessing Genetic Risks. Washington, D.C.: National Academy Press.
- Meaney, J.1992 (published in 1994). Council of Regional Networksfor Genetic Services data on laboratory procedures . In Fullarton, J. (ed.) Proceedings of the Committee on Assessing Genetic Risks. Washington, D.C.: National Academy Press.
- Murphy, P.1992a (published in 1994). New York City DNA Laboratory Quality Assurance. In Fullarton, J. (ed.) Proceedings of the Committee on Assessing Genetic Risks. Washington, D.C.: National Academy Press.
- Murphy, P.1992b. Testimony on genetic testing before House Subcommittee on Human Resources and Intergovernmental Relations, Committee on Government Operations, U.S. House of Representatives, July 23.
- Punnett, H.1992 (published in 1994). Quality assurance efforts of the American Society of Human Genetics. In Fullarton, J. (ed.) Proceedings of the Committee on Assessing Genetic Risks. Washington, D.C.: National Academy Press.
- Tsakeris, T., and Yoder, F.1992 (published in 1994). FDA response to Institute of Medicine questions on regulation of human genetic devices. In Fullarton, J. (ed.)Proceedings of the Committee on Assessing Genetic Risks. Washington, D.C.: National Academy Press.
- Vockley, J., et al. 1991. "Pseudomosaicism" for 4p- in amniotic fluid cell culture proven to be true mosaicism after birth. American Journal of Medical Genetics39:81-83. [PubMed: 1714238]
- Ward, B., et al. 1992. Response. New England Journal of Medicine326(24):1639-1640.
- Willey, A.1992 (published in 1994). New York State Genetic Quality Assurance Efforts. In Fullarton, J. (ed.) Proceedings of the Committee on Assessing Genetic Risks. Washington, D.C.: National Academy Press.
Footnotes
- *
Martinez v. Long Island Jewish Hillside Center, 512 N.E.2d. 535, New York; 1987.
- Laboratory Issues in Human Genetics - Assessing Genetic RisksLaboratory Issues in Human Genetics - Assessing Genetic Risks
Your browsing activity is empty.
Activity recording is turned off.
See more...