NCBI Bookshelf. A service of the National Library of Medicine, National Institutes of Health.
Weichbrod RH, Thompson GAH, Norton JN, editors. Management of Animal Care and Use Programs in Research, Education, and Testing. 2nd edition. Boca Raton (FL): CRC Press/Taylor & Francis; 2018. doi: 10.1201/9781315152189-21
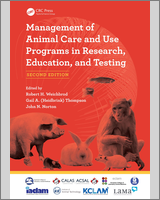
Management of Animal Care and Use Programs in Research, Education, and Testing. 2nd edition.
Show detailsIntroduction
The selection of small animal housing enclosures for laboratory facilities is dictated by a multitude of factors. Design of the actual facility and the configuration of the caging system can significantly affect the health and well-being of the rodents used in research, as well as the research results. In general, the preferred housing environment is comfortable for the animals and allows them to engage in species-specific behaviors, while allowing scientific study. This chapter reviews the primary considerations in the selection of an appropriate housing environment for small animals, predominantly rodents. Additionally, it reviews the importance of selection of appropriate bedding, feed, and environmental enrichments, with considerations for operational efficiencies and animal well-being.
Regulatory Requirements and Considerations
The regulatory requirements for small animal enclosures and housing in the United States focus primarily on animal health and well-being. Regulatory issues for animal housing differ according to species and are intended to account for physical, physiologic, and behavioral needs. Legal requirements for research animal housing are captured in the Animal Welfare Act (AWA) (Public Law 89-544) (APHIS 2002) and the Health Research Extension Act of 1985 (Public Law 99-158) (United States 1985). Additional guidance can be found in the Guide for the Care and Use of Laboratory Animals (Guide) (NRC 2011).
The AWA was established in 1966 and has been amended many times. The AWA confers authority for enforcement of the law to the U.S. Department of Agriculture (USDA) Animal Plant Health Inspection Service (APHIS). These regulations cover care, handling, housing, and transportation for rabbits, guinea pigs, and hamsters, but specifically exclude the commonly used laboratory rats of the genus Rattus and mice of the genus Mus. In general, primary enclosures for covered species are required to be structurally sound, in good repair, and constructed of materials such that animals are safe and dry. Animals housed in solid-bottom cages should be provided with bedding, and for mesh-bottomed cages, construction should protect feet and legs from injury while minimizing animal contact with waste. Space requirements are provided for each species, but all mandate that primary enclosures must allow “each animal to make normal postural adjustments with adequate freedom of movement” with “convenient access to clean food and water” (APHIS 2002).
The Health Research Extension Act requires compliance with the Public Health Service (PHS) Policy on Humane Care and Use of Laboratory Animals in order for institutions to compete for or receive federal funds. The Office of Laboratory Animal Welfare (OLAW) is responsible for ensuring compliance with the PHS Policy, utilizing the Guide as the basis for evaluating animal care programs. The Guide represents the current state of knowledge surrounding best practices for the care and maintenance of laboratory animals. The Guide also acts as the primary reference document for AAALAC International, which provides a voluntary assessment for accreditation of institutional animal care programs. These documents define laboratory animal as any vertebrate animal used in research, teaching, or testing, and therefore including rats and mice. The eighth edition of the Guide reaffirms a dedication to the promotion of animal well-being; however, in relation to the primary enclosure, increased emphasis has been placed on provision of enrichment and increased space to account for an animal’s species-specific needs (NRC 2011).
Internationally, there is a growing focus on the need to enhance laboratory animal well-being. Guidelines and regulations regarding small animal enclosures have been adopted by several countries, including Canada (Canadian Council on Animal Care 2003), Europe (Council of Europe 1986), Australia (National Health and Medical Research Council 2013), and Singapore (Singapore Government National Advisory Committee for Laboratory Animal Research 2004). Cultural and religious differences between countries may impact animal treatment and the definition of animal welfare. A discussion of the international issues and a comparison of trends in findings by AAALAC International provide an interesting perspective on the attitude toward animal environment and housing worldwide (Bayne et al. 2014).
Caging Systems
Rodent caging systems can be quite variable, but most fit a standard design of a simple box with modifications to provide environmental enhancements for the animals, to meet experimental objectives, and to ensure bioprotection or containment of pathogens or allergens. Although the actual space needs for rodents are not well defined (e.g., see Patterson-Kane et al. 2004; Nicholson et al. 2009; Whittaker et al. 2012) and can vary greatly by species and strain and gender, the majority of regulatory agencies provide minimum floor space and height requirements in their guidance documents (Table 21.1).
Table 21.1
Space Requirements for Rodents According to International Sources.
Materials
As the body of knowledge in animal care increases, the selection of materials for the construction of rodent caging has adapted to provide increased bioprotection, balanced with improvements to animal well-being.
Stainless steel is a common cage construction component. Historically, suspended and shoe box–style caging have been manufactured from stainless steel due to their longevity and sturdiness (Figure 21.1). However, these cages were heavy to manipulate and created a cool, dark housing environment for the rodents, potentially interfering with normal physiology. In the modern animal facility, stainless steel is a common component of the feed and water holders for shoe box caging. The vast majority of racks, including those for larger rodents, such as guinea pigs and chinchillas, are also constructed from stainless steel, although generally with plastic molded floors (Figure 21.2). Aluminum is utilized in the construction of some individually ventilated caging (IVC) units to minimize the weight associated with them, but care must be taken with these units because of their increased susceptibility to physical damage.
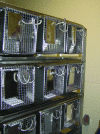
Figure 21.1
(See color insert.) Example of a stainless steel, mesh-bottomed caging for rats. The food bin is located on the inside of the cage. Water bottles would be placed on the outside (where the springs are located). Note the white plastic resting boards on (more...)
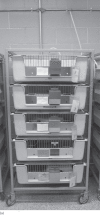
Figure 21.2
Examples of housing for (a) guinea pigs. Note the molded plastic bottom with bedding. (b) chinchillas or rabbits. Note the molded plastic bottom with bedding. Additional enrichment items can be placed, such as the house (c) or the shelter and ball (d). (more...)
Modern caging for small rodents is generally constructed of plastics, with the type of plastic utilized having changed over time. With the addition of ventilation of rodent enclosures, caging has moved from opaque plastics (generally constructed from polypropylene) (Figure 21.3) to translucent plastics (such as polycarbonate or polysulfone) that afford better visibility of the cage inhabitants (Figure 21.4). Cages that allow for easier observation of the animals without removal of the cage from the rack are especially important in IVC systems where the interval between cage changes may be 14 days or more. Additionally, the need for biosecurity and sterility has increased the demand for plastics that can withstand repeated cleaning with laboratory-grade detergents and steam sterilization without deterioration. In general, modern caging plastics can be divided into two broad categories: thermoplastics intended for repeated use and disposable plastics intended for single-use applications. Current thermoplastics include polycarbonate, polysulfonate, polythermide, and polypropylene. Caging manufacturers use injection molding techniques with these thermoplastics to create a caging unit with minimal variability and even strength.
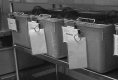
Figure 21.3
Example of rat caging made from polypropylene. Note the opaque nature of the cage and the presence of a wire lid to hold food and water. This caging is open to the room environment. (Photo by Deb Hickman.)
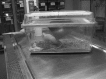
Figure 21.4
Example of mouse caging made from polysulfonate. Note that it is possible to visualize the mouse from outside of the cage. This configuration has added a lid with a filter top for enhanced bioprotection of the mouse within the cage. (Photo by Deb Hickman.) (more...)
One of the first thermoplastics used in the construction of rodent caging was polypropylene (Lipman 1999). This thermoplastic made an opaque cage that hid the animals from view, and as mice and rats are burrowing animals in the wild, it is possible that rodents felt more secure in the opaque caging. However, these cages interfered with the ability of technical staff to perform the mandated daily health checks of animals without disturbing the cage. They also deteriorated when subjected to the high temperatures and pressures associated with autoclaving, so the advent of static microisolation and IVC resulted in decreased use of caging manufactured from this thermoplastic, although these cages can still be purchased commercially.
Polycarbonate was the next commonly used thermoplastic. This plastic is clear, can withstand temperatures of 121°C (250°F), and generally has a life span of approximately 1 year under conditions of normal use. It can be tinted, which could provide additional visual protections for the animals (Dauchy et al. 2013). Rodents do not have good visual acuity associated with wavelengths of light in the infrared end of the spectrum. Therefore, there is speculation that use of caging that has been tinted red or yellow may decrease the intensity of the light perceived by rodents, perhaps even to the extent of appearing opaque. Even if this is not the case, protecting rodents from exposure to bright light decreases general stressors (Dauchy et al. 2013) and physical changes, such as retinal degeneration (LaVail et al. 1987).
Polysulfones have also been used in caging construction. These thermoplastics have the advantage of being able to withstand temperatures of 134°C (273°F), with a prolonged life span of approximately 3 years under conditions of normal use. Naturally colored amber or yellow, these plastics could also provide some visual protection from light intensities for the rodents. Most recently, polyethermide (PEI) has been utilized for the construction of rodent caging. This thermoplastic is able to withstand temperatures of 134°C (274°F) and has a life span of approximately 5 years under conditions of normal use, making it a sound financial investment for the animal facility. These cages are also deep amber to yellow, possibly providing some visual protection to the animals.
Disposable caging, manufactured from polyethylene terephthalate (PET), can also be used for the housing of small rodents. These cages provide superior bioprotection or containment opportunities, as they can be used once and disposed of through either the normal waste stream or recycling, if available. In addition to their value for high-level barrier or biocontainment situations, their use can also increase operational efficiency by circumventing the need for a cage wash space. If this space is not needed, the facility can use the footprint for additional housing, while resulting in a cost savings because there is no purchase of costly equipment with significant ongoing utility costs.
Types
The different types of caging that are currently available provide an interesting look at the developmental progress of the laboratory animal science field and how the priorities of bioprotection and animal well-being have shaped modifications to existing designs.
Conventional housing is defined as housing where the housing environment is not isolated from the animal housing room. Although this style of housing is seen much less commonly than containment caging, such as static microisolation and IVC, in the modern animal facility, it can still be an appropriate housing method for some commercial and experimental needs. There are generally two styles of conventional caging: suspended wire bottom caging (Figure 21.1) and shoe box caging with a wire top feeder (Figure 21.3). The major concern with both of these methods is that there is no bioprotection of the animals or the personnel working with the animals.
Suspended wire bottom caging was the historically preferred method of housing rats and mice used in research, as these caging systems required significantly less maintenance than those used today. However, there are health and well-being concerns associated with the use of this caging for those species. For example, older, heavier rats are predisposed to pododermatitis when housed for prolonged periods of time in these cages (Blair 2013), and neonates quickly become chilled if a secure nesting location is not provided. The use of this type of caging now requires scientific justification and approval of the animal ethics body. When used, modern caging includes modifications such as resting boards and provision of other enrichment devices, such as houses or manipulanda on which to gnaw. Generally, the racks have pans with paper or bedding beneath them (“indirect bedding”), which are changed at least once a week, while the actual animal housing cages are washed less frequently.
The conventional shoe box cage with a wire top feeder is the more common configuration of conventional rodent housing seen in the modern vivarium. The cage is generally made of plastic with a solid bottom, into which contact bedding is placed and directly bedded. Feed (and sometimes water) is provided using a stainless steel wire bar lid that holds the food above the animals (Figure 21.3).
A modification of the conventional shoe box caging system was the addition of a cage lid with filtration capabilities (Figure 21.4). These caging systems allowed the housing environment of the cage to be isolated from the animal housing room”“especially when used in conjunction with laminar flow workstations of biosafety cabinets. These lids are sufficiently porous enough to allow the exchange of gases and moderation of temperature, but prevent the escape of potentially infectious or allergenic compounds. However, because the exchange of gases and heat is not as efficient as a system without the filtration top, these cages often require changes that are much more frequent than the conventional shoe box caging system.
IVC systems were created in response to the accumulation of gases that were noted in the static filter-top systems. In an IVC system, the lidded cages are placed on a rack that forces air through the caging, creating air changes within the housing environment to remove the potentially noxious gases (Figure 21.5). There are many different designs of IVC systems (Figure 21.6). In the original designs, air was brought into the cage from the room, without filtration aside from that provided by the filter-top lid, and was discharged from the cage by exhaust blowers. Now, these units are generally designed so that air is brought in from the animal room, passed through a high-efficiency particulate air (HEPA) filter, delivered to the cage, and then HEPA filtered out, although there are many configurations available from the various vendors (Figure 21.6). High-level biocontainment studies utilize specialized IVC systems where a gasket is present between the lid and the cage to ensure a tight seal with no leakage of any pathogens of concern from the cage. Filtration of both the supply and exhaust air produces superior bioprotection for the animals and decreases particulate contamination of the animal housing room. In addition to providing a controlled housing environment, another significant advantage to the use of IVC systems is that it is possible to house more cages within an existing footprint.
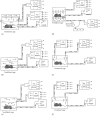
Figure 21.6
Schematic representations of types of commercially available IVC. All systems are shown with automatic watering. Numbers within brackets reflect the three commercially available versions of the intracage supply–intracage exhaust ventilated caging (more...)
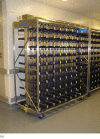
Figure 21.5
(See color insert.) (a) Example of an IVC rack. There are no cages, but the rack can hold up to 160 mouse cages. The metal wall in the center of the rack provides forced air to the cages on the rack. (b) Close-up of IVC with empty bays next to the cages. (more...)
Isolators are specialized caging systems that are used in cases where bioprotection of the animals needs to be very high. Examples include very valuable immunocompromised animals, germ-free animals, and gnotobiotics. In an isolation system, sterile caging with wire top lids are introduced into a sterile environment (although the equipment can be placed prior to sterilization). Rodents are generally introduced after caesarean section to allow for control of the biome. The entire environment is maintained aseptically. Items such as food and bedding are sterilized prior to introduction into the isolator. Caging equipment can be washed within the isolator or removed for cleaning, but then is sterilized prior to reintroduction into the isolator. In the strictest protocols, nothing (aside from waste) is removed from the isolator until the colony is removed, and the unit is broken down and cleaned and resterilized prior to its next use.
Metabolic cages allow the researcher to collect information about the animal by collecting samples from the cages. The most commonly used caging designs house the rat or mouse in a Plexiglas or glass cylinder with a wire mesh floor. All feces and urine are collected over a period of time, generally 24 hours. A funnel system that is incorporated into the floor of the cage allows the collection of urine and feces separately from each other, facilitating in vitro analysis. More recently, metabolic cages have been designed that can measure more of the metabolic output of the rodent than just the urine and feces. In this style of metabolic chamber, the rodent is placed in a sealed cage that is provided with fixed amounts of air. The computerized system is able to measure multiple variables, such as activity, body weight, feeding, drinking, urine collection, sleep detection, body temperature, heart rate, and carbon dioxide production.
Integration
The racks and caging systems that are used in an animal facility drive some of the specialized infrastructure requirements in the facility. Structurally, doors need to be sufficiently large to allow personnel to move racks, with or without their exhaust and supply blowers, in and out of rooms. This is needed as the functions of rooms may change over time, in addition to the regular sanitation requirements.
The facility heating, ventilation, and air-conditioning (HVAC) system plays a significant role in the success of the housing system selected. When using conventional caging systems, high levels of air changes, such as the 10–15 air changes per hour recommended by the Guide, reduce the odors and allergens in the animal rooms (Korpi et al. 2007). The use of IVC systems and isolators may remove the requirement for the high level of air changes for the control of odors and dander, especially if the racks exhaust directly into the building exhaust. However, the motors associated with these units also add a heat load to the room, which often requires that the air changes per hour remain high to prevent the animal housing room from warming.
Control of temperature at the animal housing room level is targeted to be within 1°–2° of the established set point. Recently, more consideration has been given to the effect of the IVC on the housing environment of the cage. As air changes per hour at the cage level can be rather high (upward of 60 air changes per hour in some models), there is a possibility of drafts and chilling of the cage inhabitants. For this reason, it has been recommended that the lowest air changes per hour required to remove gases be utilized and that nesting material be provided to rodents, to allow them to thermoregulate. All this is very dependent on the system that is in use, which is why it behooves the laboratory animal facility personnel to be familiar with how the air flows in and out of the cages and to be observant of how their animals are using the space and enrichments provided.
When working with IVC, isolators, and the modern computerized metabolism systems, emergency power is absolutely critical. Because these systems require forced air, if the power to the fans is disrupted, the levels of ammonia, carbon dioxide, and relative humidity, and the temperature in the housing environment of the cages can rise very quickly, resulting in the death of animals (Huerkamp et al. 2003). Conventional housing does not have this risk, although emergency power is still recommended to ensure that the HVAC and light cycle for the animal housing room are consistent during extended outages.
Sanitation
The Guide is a primary resource for animal facility managers and Institutional Animal Care and Use Committees (IACUCs) when selecting and approving standard operating procedures for maintenance and sanitation of primary enclosures. Housing density, cage change frequency, bedding material, and the use of ventilated caging affect the housing environmental conditions (Reeb-Whitaker et al. 2001; Rosenbaum et al. 2009). The frequency of bedding changes and cleaning and disinfection of the cage is a primary consideration for the maintenance of environmental conditions appropriate for animal health and well-being (NRC 2011). Fluctuations in these parameters can cause alterations in metabolic or physiologic processes, as well as disease susceptibility. The Guide utilizes performance standards such as temperature, humidity, and concentration of gases and particulate matter to support the selected frequency and intensity of cleaning and disinfection. The frequency and intensity of sanitation also depend on specific physiologic and behavioral characteristics of the species being housed, the use and type of bedding material, and the rate and degree of soiling of the enclosure surface.
The accepted practice for sanitation of solid-bottom cages is once per week, with sanitation of equipment and accessories such as cage lids once every 2 weeks (NRC 2011). The use of IVC has increased the sanitation interval for solid-bottom cages to 2 weeks, as these cages receive frequent HEPA-filtered air changes. Increased intracage ventilation removes excess humidity and waste gases from cages, reduces disease transmission, and maximizes the efficient use of space and personnel resources (Reeb et al. 1998; Carty 2008; Rosenbaum et al. 2009). Recommended cage sizes for rodents are primarily based on the weight of the animal and the numbers of animals per cage; however, as cage density increases, cage change frequency must also increase to prevent accumulation of waste gases (Gonder and Laber 2007; Rosenbaum et al. 2009, 2010). Standard practices in many rodent breeding facilities permit less frequent cage changes to prevent disruption of newborn pups; however, studies with C57BL/6NTac mice and NTac:NIH-Whn rats indicate that breeding performance may not be impacted by disruption of neonates during the cage change-out (Sanderson et al. 2010).
In terms of sanitation, bedding provided in rodent cages can act to absorb moisture, minimize bacterial growth, limit animal contact with excreta, and reduce the accumulation of intracage ammonia (NRC 2011). Several bedding materials are available for rodents, including corncob, wood chips, paper products, and grass fiber pellets. Bedding should be evaluated for absorbency, biodegradability, toxicity, dust, palatability, comfort, cost, availability, damage to cage washers, animal preference, and effect on research (Wirth 1983; Ras et al. 2002; Smith et al. 2004; Domer et al. 2012). Soiled bedding should be changed “as often as necessary” to keep ammonia levels low and animals clean and dry. Intracage ammonia concentration is influenced by temperature, humidity, ventilation, urine, and bacteria. Humidity may be influenced by ventilation or bedding material, with highly absorbent materials and increased airflow decreasing intracage humidity levels (Lipman et al. 1992). Intracage ammonia is produced by the combination of urine and urease-producing bacteria found in feces and processed bedding material. The initial bacterial content in bedding can be controlled by autoclaving prior to use (Reeb et al. 1998). Disadvantages of autoclaving bedding include personnel time, autoclave energy consumption and maintenance costs, and loss of absorptive capacity of the bedding material. Although a correlation between cage appearance and animal health has not been documented, human perception of cage cleanliness remains a significant factor in the determination of cage change frequency (Rosenbaum et al. 2009; Domer et al. 2012).
Selection of the appropriate plastic materials is an important consideration for the maintenance and sanitation of cages (Demorotski 2008). Frequency of cage change-out, type of detergents, autoclaving, and water quality and temperature will impact cage longevity. Deterioration of plastic and residue deposition from the sanitation process may cause cages to become opaque. Opaque cages make visualization of the animal difficult and increase the time required for animal health observations. Hard water and calcium buildup on cages may be removed by manual scraping, which may damage the plastic, or by use of chemical rinses, which may damage expensive cage wash equipment (Chipps et al. 2012). Routine examination and treatment or removal of damaged or opaque plastic should be performed to ensure continued effective sanitation and clear observation of the animal. Likewise ventilated caging systems that utilize air nozzles that penetrate the cage should routinely be examined for occlusion by hair, bedding, food particulates, or debris to ensure that appropriate airflow is maintained to the cage. Occluded nozzles may result in increased humidity and accumulation of ammonia within the cage (Creamer et al. 2014), necessitating more frequent cage change-outs.
Water
Animals must have access to potable, uncontaminated drinking water (NRC 2011). Water entering an animal research facility is typically supplied by a local source and meets the standard necessary for human consumption (Hessler 2011). Most groundwater in the United States contains significant levels of calcium, magnesium, or iron, which may cause mineral scale to develop within pipes or sipper tubes. Water treatment with ultrafiltration or reverse osmosis (RO) provides high-quality drinking water and reduces the potential for scale to form on equipment or within pipes. Further treatment of animal drinking water by hyperchlorination (12–15 ppm) or acidification (pH 2.5–3) has been used to limit the growth of opportunistic microorganisms that may have a negative impact on transgenic or immunosuppressed rodents (Hessler and Lehner 2009). The choice of water delivery system is determined by species, facility, and type of water available. Water may be supplied to cages by water bottles, pouches (i.e., Hydropac®), or automatic water systems (Figure 21.5b). A detailed discussion of water treatment and the pros and cons of the different cage delivery systems is provided by Allen et al. in Chapter 28 of this book.
The use of closed-top or specialized caging systems to maintain barrier classifications for rodent housing has implications for water delivery and consumption. Water intake by rodents has been shown to be affected by intracage humidity (Hoyt et al. 2007) and type of caging (Memarzadeh et al. 2004; Rosenbaum et al. 2010). The increased use of ventilated racks for housing rodents has raised concerns about the potential for higher airflow to cause dehydration (Bekkevold et al. 2013). Conversely, higher intracage humidity has been reported to be associated with decreased water consumption by mice housed in closed-top ventilated and static microisolator cages (Memarzadeh et al. 2004; York et al. 2012). Wire bar or smooth plastic cage lids are molded to accommodate water bottles or bags as a separate component of the rodent cage. Cage lids are designed to allow the water bottle or bag to be added either externally, that is, outside the closed cage lid, or internally, that is, completely captured within the bonnet of the cage lid. The definition of barrier status at the level of the cage may dictate the need for a particular style of cage lid.
Automatic water systems are a common mechanism for the delivery of potable water to a variety of small laboratory animal species. Ventilated rodent racks may support automatic water systems with water valves permanently attached to the rack, removable valves attached to the rack, or valves attached permanently to the inside of the cage. Water valves permanently attached to the inside of the cage provide challenges for sanitation, requiring more attention for removal of bedding that may become caught up around the inverted stem. Water valves that remain affixed to the rack provide the opportunity for cross-contamination of cages if cage locations are inadvertently switched during cage change-out or during experimental procedures. Recommendations for sanitation and maintenance of automatic watering systems have been proposed for certain predefined conditions (Gonzalez et al. 2011; Molk et al. 2013); however, unique standard operating procedures should be developed to meet the individual facility and equipment conditions.
Changing Stations and Biological Safety Cabinets
When working with static microisolators or IVC, a changing station is a critical tool to ensure that the cage-level containment is not compromised. Most changing stations are built with a laminar flow design, where HEPA-filtered air is forced across the work surface, providing protection to the animals in the cage, and then captured and exhausted out through an additional HEPA filter to provide allergen protection to the animal housing room and the personnel working with the caging. However, the placement of HEPA filters is not consistent, and the design of the unit should be evaluated to ensure that it is performing in a way that meets the intended need. The design can have the air flowing from the back of the unit, across the work surface, to the front of the unit, or it can have a top-down configuration, where the air is forced from the ceiling of the cabinet down onto the work surface, and collected to be exhausted at the work surface (Figure 21.7). Both of these configurations provide excellent bioprotection for the majority of rodent pathogens and allergens, although they do not provide appropriate guarantees of protection of the worker from possibly infectious zoonotic pathogens or from exposure to chemical contaminants, such as isoflurane.
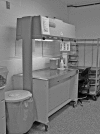
Figure 21.7
Example of a laminar flow workstation. In this configuration, air is HEPA filtered in the top of the unit and forced down over the work surface. Exhaust ports on the edges of the work surface collect the air for additional filtration before the air is (more...)
When working with agents that are listed at biosafety level 2 or higher, a changing station will not provide sufficient personnel protection and a biological safety cabinet (BSC) should be used. Class I biosafety cabinets are generally not advised for use in an animal facility. These units provide personnel and environmental protection, but no product (animal) protection, thus compromising the biocontainment established with the use of static filtration or IVC. Class II cabinets protect the animals, the animal housing room, and the personnel. There are four subtypes of Class II cabinets. Types A1 and A2 blow the air over the work surface from the top of the cabinet. The air is exhausted at the bottom of the cabinet and returned to the top, where 70% of the air recirculates through the system HEPA and 30% is exhausted through the exhaust HEPA. These are normally “thimble” ducted, but they do not have to be ducted to operate properly. The difference between Type A1 and A2 cabinets is in the inflow velocity of the supply air. Type B1 and B2 cabinets must be hard-ducted to an exhaust system (Figure 21.8). In these cabinets, 60% of the air is exhausted and only 40% is recirculated. These cabinets can be used with chemical hazards, in addition to biological hazards, due to their configuration. Class II cabinets are the style that is commonly used in most laboratories. Class III cabinets are generally reserved for maximum containment laboratories.
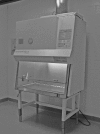
Figure 21.8
Example of a BSC suitable for use with biological hazards. (Photo by Deb Hickman.)
Barrier Housing
The use of barrier housing for rodents has become the standard for housing research and breeding animals (Hessler 2011). Barrier facilities are classified on the basis of operational criteria that are used to protect animals from unwanted or excluded organisms (Committee on Infectious Diseases of Mice and Rats 1991). The need for barrier housing has expanded with the use of immunocompromised, fragile, or extremely valuable transgenic animals. The barrier may exist at the cage, room, or facility level. In addition, specialized caging and equipment, such as ventilated racks, flexible film, or hard-sided isolators, may be utilized to create a barrier within a specific area of an animal facility (Hessler 2011). The criteria for classification of a barrier system consist of a number of factors that have not changed over the years (Committee on Infectious Diseases of Mice and Rats 1991): (1) quality, number, and source of animals; (2) frequency and method of animal introduction; (3) processing of materials into the barrier; (4) entry of personnel into the barrier; (5) environmental systems (HVAC, temperature, and lighting); and (6) monitoring practices.
The effective maintenance of a barrier requires the development of standard operating procedures that are understood and supported by all personnel. Designation of entry order based on the criteria listed above may be applied to the closed microisolator cage, room, or facility access. Establishment of the barrier at the cage level requires specialized handling procedures, sanitized microisolator caging, and sterile water, food, and bedding (Baker et al. 2014). Barrier facilities may be managed at different levels of microbiological control, which in turn dictates the way in which supplies and personnel enter the facility. Movement of materials and personnel should always travel from the “cleanest” to the “dirtiest” areas, with barrier-specific requirements for personnel to shower and/or change clothes prior to reentry from a dirtier to a cleaner barrier area. Materials or supplies that are cleaned or set up outside of the barrier may be wrapped or autoclaved for transport into the barrier. The use of pass-through autoclaves may eliminate the need for wrapping and unwrapping of supplies transported into the barrier (Hessler 2011). Conversely, the utilization of irradiated food and bedding may decrease employee time spent autoclaving supplies. Laminar flow rooms, biosafety cabinets, and change stations allow for animals to be handled outside of the barrier of the cage and still maintain strict barrier status.
The use of personal protective equipment (PPE) is an integral component of the barrier management practices designed to maintain animals free of excluded organisms. Animal care personnel are often required to enter through a vestibule with interlocking doors, where sterile outer garments or uniforms are donned prior to entry into the barrier facility (Hessler 2011). In addition to a sterile lab coat or uniform, typical PPE for entry into a barrier includes hair bonnet, face mask, shoe covers, and gloves (ACLAM 2010). The general consensus within the laboratory animal community is that PPE is important; however, recent discussion regarding PPE has focused on determining what amount of PPE is essential for disease control versus what is worn as part of historical practices (ACLAM 2010). The increased use of microisolator and individually ventilated rodent caging systems has been shown to be very effective at controlling pathogen transmission between cages within the same room, and even on the same rack, for a variety of disease outbreaks (Clough et al. 1995; Hasegawa et al. 2003; Bohr et al. 2006). A shift in the definition of the barrier unit from the facility or room to the cage level may allow for decreased use of some PPE and still maintain barrier status (Hickman-Davis et al. 2012; Baker et al. 2014).
Enrichment
All species evolved in a rich world of experiences, but in order to reduce variability in studies, small rodent housing environments can be quite barren, resulting in aberrant behavior and physiology in some animals (Olsson et al. 2003; NRC 2011; Weary 2013). Although it has been assumed that a standardized environment results in standardized animals, the concerns with this assumption are demonstrated repeatedly in colonies of genetically identical rodents where there are behavioral differences in incidence of maternal cannibalism, fighting, and self-destructive compulsive behaviors. Therefore, a more appropriate animal model would be one raised and living in an enriched environment (Smith and Corrow 2005; Arranz et al. 2010; Konkle et al. 2010). Continual improvements in understanding and technology should be used to reduce and refine animal use (Russell and Burch 1959). Enrichment needs to be a committed part of every institution’s budget and management plan (Patterson-Kane 2011). This is necessary to nurture not only the animals under our care, but also the staff that provide the care. When unsupported by the institution, caring personnel may enrich animals on their own, resulting in a loss of personal resources for staff and the very real possibility of unaccounted for variables for animal studies (Patterson-Kane 2011).
There are many ways to enrich animals because animals have a variety of behaviors to fulfill. Enrichment strategies can be broken down into several general categories: social, bedding, nesting, shelters, oral, exercise, scent, and sound. Unfortunately, there is no one perfect “best enrichment” practice or formula. Allowable and ideal enrichment strategies change depending on facility operation, study design and intent, animal strain, and individual animal preferences (Baumans 2005). When strategizing a complete enrichment plan, many considerations must be taken under advisement. For some institutions, it is preferable to invest in durable devices that can be sanitized and reused; others may find it better to use disposable devices. When reusable items are employed, they should be considered a potential vector, with impacts on cleaning and quality control monitoring (Smith and Hargaden 2001). Understanding of the species and strain needs is also critical. For example, an enrichment device can be a resource to be guarded and result in escalated aggression, especially in some group-housed male mice (Howerton et al. 2008).
Using a positive psychology approach to environmental enrichment, the first step is defining the ideal quality of life for each species (Olsson et al. 2003; Timmins et al. 2007). An international panel of 12 experts determined that welfare usually refers to observable and measurable experiences of an animal, whereas quality of life describes animals’ mental state (Timmins et al. 2007). Often, the picture of quality of life is established using the concept of these animals in the wild or as pets. The goal then should be to continually move toward that standard.
Social
The Guide recommends housing social animals with conspecifics as a default (NRC 2011). It is through play with other young and interactions with adults that animals learn important social skills, such as fighting, appeasement, mating calls, and parenting (Panksepp and Beatty 1980; Varlinskaya et al. 1999; Burgdorf et al. 2008; Viegas 2010; Kulesskaya et al. 2011). A social partner can be a soothing presence during times of stress, a playmate for exercise, and with the rise of IVC, a partner to share body heat. Mice in isolation show higher heart rates and disruptions in sleep patterns than pair-housed mice (Spani et al. 2003). The importance of social housing is illustrated in a study that demonstrated that a mouse will choose to be with a historically aggressive mouse over solitary housing (Van Loo et al. 2003).
Social enrichment may also be in the form of positive interactions between animals and their handlers. Although it is impractical in many facilities due to the large number of animals, gently handling animals does have its rewards. This can be done with young animals, for a few minutes a day, or even with adult animals that will be handled for procedures. Habituation is the simplest form of learning, and it has the benefit of reducing the novelty, and accompanying fear, of human handling (Varty et al. 2000). Handlers should keep in mind that every interaction with an animal is a training session; therefore, calm, gentle, respectful handling should be practiced during every encounter.
Bedding
In general, bedding is a standard addition to cage bottoms for the purpose of absorbing waste. There are many bedding options, including various wood, corn, and paper products. As burrowing creatures in the wild, some rodents prefer deep bedding (Freymann et al. 2014). Unfortunately, this practice is not practical for widespread institutional use, because it would add significant cost and ergonomic risk due to increased weight of the bedded cages.
Nesting
A common mistake is to assume that bedding and nesting in a cage are the same. Bedding can be considered the litter pan of the cage, and serve a similar purpose (unless provided in such volume as to also be able to be used for burrowing). Animals have been shown to give off separate pheromones in relation to these two different purposes (Van Loo et al. 2000). Nesting materials are used by rodents to build areas for warmth, sleep, and relaxation (Van Loo et al. 2004; Winnicker 2012; David et al. 2013; Gaskill et al. 2013b). Nesting material is likely the best single enrichment device for mice, coming also in a variety of options, most commonly facial tissue, paper strips, and compressed cotton (Hutchinson et al. 2005, 2012; Smith and Corrow 2005; Kulesskaya et al. 2011; Narver 2013). Recently, there has been a rise in the popularity of paper strips, as they allow mice to weave complex nests, as seen in the wild (Hess et al. 2008; Gaskill et al. 2012, 2013a). As always, selection of the best nesting material will depend on strain, study, and institution. Nude or hairless mice can get conjunctivitis from fibers of nesting material. Weak mice may lack the constitution required to make use of compressed cotton or even paper strips. Some nesting materials may strangulate or stick to neonates, causing injury or death (Smith and Corrow 2005). Rats tend to use nesting material to form bedded resting areas rather than enclosed nests, but it has been shown to be a valid enrichment option that rats will work to acquire (Manser et al. 1998; Van Loo and Baumans 2004) (Figure 21.9).
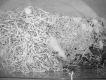
Figure 21.9
Example of nesting material use in rats. In this case, the rat was provided with two styles of nesting material”“cotton batting and shredded paper. Note how the rat has created a shelter, which it is climbing out of, and how the cotton (more...)
Shelters
In-cage shelters provide both mice and rats respite from the drafts of ventilated caging, the ability to withdraw from bright lights, a place of security or escape from cage mates, and a place to hide from perceived predators. Rats will stay in a nest box for the duration of a room’s light cycle, and when a shelter is not available, will retreat to a darkened cage corner (Manser et al. 1998). Although it conceals them from husbandry staff, rats prefer opaque shelters with enclosed corners (Manser et al. 1998) (Figure 21.10).
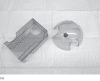
Figure 21.10
Examples of intracage shelters. These come in a variety of styles and are fabricated from a variety of materials, including plastic (a, c) and paper (b). (Photo by Deb Hickman.).
Diets, Treats, and Gnawing
Foraging is a positive time expense for many species; in fact, mice prefer to search for food, even when it is readily available (Baumans 2005; Pritchett-Corning et al. 2013). In the absence of adequate nesting material, mice can compensate for the thermal stress of IVC by food grinding for higher-energy parts of food (Pritchett-Corning et al. 2013).
Rats demonstrate a clear preference for gnawing enrichment (Smith and Corrow 2005). Gnawing is an activity that should be understood to be different from eating. It is reasonable to expect some small pieces of gnawing enrichment to make their way through the gastrointestinal tract; however, these small pieces generally tend to be innocuous (Smith and Hargaden 2001), assuming that the devices have been assessed to ensure they are nontoxic (Figure 21.11).
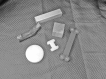
Figure 21.11
Examples of gnawing devices. The top devices are fabricated from wood. The bottom devices are fabricated from a variety of plastics. The variety in firmness can create novel gnawing experiences for the rodents. (Photo by Deb Hickman.)
Exercise
An exercise wheel is the most common tool utilized to stimulate exercise within a cage. The challenge in providing this for lab animals is finding a design that will fit and remain stable within a typical shoe box–style cage. When available, rodents do make use of them with resulting beneficial effects (Leggio et al. 2005; Neuman et al. 2013). Other options for in-cage exercise opportunities can be provided via a trapeze or hook hanging from the hopper. Additionally, expanding the vertical space of cages (Figure 21.12) (Wheeler et al. 2014) or including shelves to increase the environmental complexity has been shown to increase the quality of life of laboratory rodents.
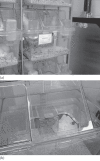
Figure 21.12
Example of a multilevel caging system for rodents. (a) Note the rats utilizing the upper shelf, while (b) provides a detailed view of the shelf that bisects the cage. The shelf and space to stand provide a complex environment for the rodents.
Sound and Scent
Noise stress (such as a fire alarm, motor vibration, and loud in-room human activity) has been demonstrated to have an adverse effect on breeding and stress levels (Castelhano-Carlos and Baumans 2009; Alworth and Buerkle 2013). Although music has been shown to have a beneficial effect on captive animals (Whiteman 2001; Wells et al. 2002; Boone and Quelch 2003; Hutchinson et al. 2005; Menon and Levitin 2005; Ying et al. 2007; Leeds and Wagner 2008; Davila et al. 2011; Kogan et al. 2012; Alworth and Buerkle 2013), it is unclear if this is a direct effect of the music itself. It is possible that it is an indirect effect resulting from the possibilities that the music enrichment serves to mask other unpleasant vivarium noises, has a calming effect on caretakers that extends to the animals, or serves as mental stimulation in the otherwise monotonous cage (Burgdorf et al. 2008; Leeds and Wagner 2008; Alworth and Buerkle 2013). If employed, calming music should be used, at a conversational (or lower) level, and it should be turned off at the end of each workday (NRC 2011). Music after a noise stress or restraint has been shown to reduce stress levels in mice, and rats exposed to music composed by Mozart had a significant reduction in blood pressure as long as 2 hours postexposure (Alworth and Buerkle 2013).
Scent is a critical component of rodent communication. The role of pheromones in the synchronization of the reproductive cycle (Brennan 2010), social facilitation (Stockley et al. 2013; Semple et al. 2014; MacRae et al. 2015), and signaling of distress (Inagaki et al. 2010; Voznessenskaya 2014) has been described, but is still not well understood. As nesting material can contain “appeasement” pheromones, movement of the nest to a new cage at the time of cage change may help to decrease aggression between mice (Whary et al. 2015). Additional studies are ongoing to determine how scents and pheromones can be used to improve the quality of life for laboratory rodents.
Emerging Technologies
Improvements to the operational efficiencies of animal facilities represent a rapidly progressing arena. By the time this book is published, it is a certainty that there will be new technologies available to improve the quality of life of animals and the efficiencies of the animal facilities. This section addresses some currently emerging technologies as examples of the opportunities that are coming.
Automated Census
The accurate collection of census data is critical for the vast majority of animal facilities that rely on per diems to provide the fiscal support for their operation. Bar code scanning has been a significant improvement over manual counting of cages, but it still requires that each cage be scanned individually to be counted. The use of wireless technology and radio frequency identification (RFID) has provided an opportunity for significant improvements in operational efficiencies. These systems allow the cages to essentially self-report their presence, fully automating the census-taking operation.
Alarms
Technology is also providing opportunities to intervene in cases of equipment malfunction at the level of the cage. Automated systems that alert a user when a cage is not engaged properly to IVC can help detect in real time that the cage is receiving appropriate air changes or access to an automatic watering system. Some manufacturers have developed IVC racks that integrate with the facility animal housing room monitoring systems to alert facility staff when there is a concern with a particular rack. Ultimately, technology will allow alerts at the housing environment level as well.
Thermoregulation at the Cage Level
Recognition that the animal housing room is generally kept at temperatures that are comfortable for humans, but could be cool for rodents, has become another area for potential refinement. The potential cooling of rodents is further complicated by the relatively high air changes per hour that are present in an IVC housing environment. Additionally, there are some studies that show that cold stress of the rodent model can negatively affect research results, such as tumor growth (Kokolus et al. 2010, 2014). To ameliorate this issue, provision of nesting material has been recommended, as this allows the mice to behaviorally thermoregulate (Gordon et al. 2014; Maher et al. 2015). To support some tumor growth studies, recommendations have been made to increase the temperature of the animal housing room. Additionally, some caging manufacturers have developed caging systems that will allow the housing environmental temperature of the cage to be elevated without affecting the room temperature.
Research Impact and Considerations
The effect of the housing environment on experimental variables is an area of ongoing active evaluation between scientists and the animal facility staff. The use of filtration tops for rodent caging was driven by the understanding that exposure to pathogens resulted in confounding variables in a wide variety of studies, including studies that are affected by challenges to the immune system, such as tumor growth (Baker 1998). Additionally, scientists who are concerned about the development and functioning of the brain often have concerns about changes in the housing environment that may affect the development or behavior of the animals that they are studying (Toth et al. 2011; Toth 2015). Inappropriate social groups and provision of environmental enrichment that might create distress for the animals are also confounding variables that must be evaluated in consideration of selection of the appropriate housing environment to be provided (Toth et al. 2011). Light contamination can result in alterations of tumor growth (Dauchy et al. 2013), as can changes in the temperature of the macro- and housing environment (Kokolus et al. 2014). Some strains breed well in IVC, while others do better in static filtration or conventional situations. Because there is so much variation in the range of scientific evaluations that are performed on a daily basis, laboratory animal professionals are highly encouraged to work closely with their scientific colleagues when evaluating the options that are available for housing environments.
References
- ACLAM (American College of Laboratory Animal Medicine). ACLAM2010Forum”“PPE questionaire. https://www
.aclam.org /Content/files/files /Public/Active/forum2010 _ppe_surveyresults.pdf. - Alworth, L. C., and S. C.Buerkle. 2013. The effects of music on animal physiology, behavior and welfare. Lab Anim (NY)42 (2):54–61. [PubMed: 23340788]
- APHIS (Animal and Plant Health Inspection Service). 2002. Animal Welfare Act and animal welfare regulations, 2131–5. Washington, DC: U.S. Department of Agriculture, APHIS.
- Arranz, L., N. M.De Castro, I.Baeza, I.Mate, M. P.Viveros, and M.De la Fuente. 2010. Environmental enrichment improves age-related immune system impairment: Long-term exposure since adulthood increases life span in mice. Rejuvenation Res13 (4):415–28. [PubMed: 20707722]
- Baker, D. G.1998. Natural pathogens of laboratory mice, rats, and rabbits and their effects on research. Clin Microbiol Rev11 (2):231–66. [PMC free article: PMC106832] [PubMed: 9564563]
- Baker, S. W., K. A.Prestia, and B.Karolewski. 2014. Using reduced personal protective equipment in an endemically infected mouse colony. J Am Assoc Lab Anim Sci53 (3):273–7. [PMC free article: PMC4128565] [PubMed: 24827569]
- Baumans, V.2005. Environmental enrichment for laboratory rodents and rabbits: Requirements of rodents, rabbits, and research. ILAR J46 (2):162–70. [PubMed: 15775025]
- Bayne, K., A. C. D.Bayvel, and V.Williams. 2014. Laboratory animal welfare: International issues. In Laboratory Animal Welfare, ed. K.Bayne and P.Turner, pp. 77–93. San Diego: Elsevier.
- Bekkevold, C. M., K. L.Robertson, M. K.Reinhard, A. H.Battles, and N. E.Rowland. 2013. Dehydration parameters and standards for laboratory mice. J Am Assoc Lab Anim Sci52 (3):233–9. [PMC free article: PMC3690443] [PubMed: 23849404]
- Blair, J.2013. Bumblefoot: A comparison of clinical presentation and treatment of pododermatitis in rabbits, rodents, and birds. Vet Clin North Am Exot Anim Pract16 (3):715–35. [PubMed: 24018034]
- Bohr, U. R., M.Selgrad, C.Ochmann, S.Backert, W.Konig, A.Fenske, T.Wex, and P.Malfertheiner. 2006. Prevalence and spread of enterohepatic Helicobacter species in mice reared in a specific-pathogen-free animal facility. J Clin Microbiol44 (3):738–42. [PMC free article: PMC1393101] [PubMed: 16517848]
- Boone, A., and V.Quelch. 2003. Effects of harp music therapy on canine patients in the veterinary hospital setting. Harp Ther J8 (2):4–5, 15.
- Brennan, P. A.2010. Pheromones and mammalian behavior. In The Neurobiology of Olfaction, ed. A.Menini, pp. 167–9. Boca Raton, FL: CRC Press. [PubMed: 21882427]
- Burgdorf, J., R. A.Kroes, J. R.Moskal, J. G.Pfaus, S. M.Brudzynski, and J.Panksepp. 2008. Ultrasonic vocalizations of rats (Rattus norvegicus) during mating, play, and aggression: Behavioral concomitants, relationship to reward, and self-administration of playback. J Comp Psychol122 (4):357–67. [PubMed: 19014259]
- Canadian Council on Animal Care. 2003. Laboratory Animal Facilities”“Characteristics, Design and Development. Ottawa, ON: Canadian Council on Animal Care.
- Carty, A. J.2008. Opportunistic infections of mice and rats: Jacoby and Lindsey revisited. ILAR J49 (3):272–6. [PubMed: 18506060]
- Castelhano-Carlos, M. J., and V.Baumans. 2009. The impact of light, noise, cage cleaning and in-house transport on welfare and stress of laboratory rats. Lab Anim43:311–27. [PubMed: 19505937]
- Chipps, J., V. K.Bergdall, N.Hanson, and D.Hadziselimovic. 2012. Increasing the usable lifespan of clouded rodent caging via implementation of a novel cage wash sanitation process. J Am Assoc Lab Anim Sci51:637.
- Clough, G., J.Wallace, M. R.Gamble, E. R.Merryweather, and E.Bailey. 1995. A positive, individually ventilated caging system: A local barrier system to protect both animals and personnel. Lab Anim29 (2):139–51. [PubMed: 7603000]
- Committee on Infectious Diseases of Mice and Rats, Institute of Laboratory Animal Resources, Commission on Life Sciences, National Research Council. 1991. Infectious Diseases of Mice and Rats. Washington, DC: National Academy Press.
- Council of Europe. 1986. European Convention for the Protection of Vertebrate Aimals Used for Experimental and Other Scientific Purposes. Strasbourg, France: Council of Europe. http://conventions
.coe .int/Treaty/en/Treaties/Html/123.htm. - Creamer, M. A., J.Petty, T.Martin, V. K.Bergdall, and J. M.Hickman-Davis. 2014. Implications of natural occlusion of ventilated racks on ammonia and sanitation practices. J Am Assoc Lab Anim Sci53 (2):174–9. [PMC free article: PMC3966274] [PubMed: 24602544]
- Dauchy, R. T., E. M.Dauchy, J. P.Hanifin, S. L.Gauthreaux, L.Mao, V. P.Belancio, T. G.Oomset al.2013. Effects of spectral transmittance through standard laboratory cages on circadian metabolism and physiology in nude rats. J Am Assoc Lab Anim Sci52 (2):146–56. [PMC free article: PMC3624782] [PubMed: 23562097]
- David, J. M., S.Knowles, D. M.Lamkin, and D. B.Stout. 2013. Individually ventilated cages impose cold stress on laboratory mice: A source of systemic experimental variability. J Am Assoc Lab Anim Sci52 (6):738–44. [PMC free article: PMC3838608] [PubMed: 24351762]
- Davila, S. G., J. L.Campo, M. G.Gil, M. T.Prieto, and O.Torres. 2011. Effects of auditory and physical enrichment on 3 measurements of fear and stress (tonic immobility duration, heterophil to lymphocyte ratio, and fluctuating asymmetry) in several breeds of layer chicks. Poult Sci90 (11):2459–66. [PubMed: 22010229]
- Demorotski, D.2008. Selecting plastic animal cages. ALN. http://www.alnmag.com/articles/2008/02 /selecting-plastic-animal-cages.
- Domer, D. A., R. L.Erickson, J. M.Petty, V. K.Bergdall, and J. M.Hickman-Davis. 2012. Processing and treatment of corncob bedding affects cage-change frequency for C57BL/6 mice. J Am Assoc Lab Anim Sci51 (2):162–9. [PMC free article: PMC3314518] [PubMed: 22776115]
- European Union. 2010. Directive 2010/63/EU of the European Parliament and of the council of 22 September 2010 on the protection of animals used for scientific purposes. Off J Eur Union276:33–79.
- Freymann, J., P. P.Tsai, H.Stelzer, and H.Hackbarth. 2014. The amount of cage bedding preferred by female BALB/c and C57BL/6 mice. Lab Anim (NY)44 (1):17–22. [PubMed: 25526055]
- Gaskill, B. N., C. J.Gordon, E. A.Pajor, J. R.Lucas, J. K.Davis, and J. P.Garner. 2012. Heat or insulation: Behavioral titration of mouse preference for warmth or access to a nest. PLoS One7 (3):e32799. [PMC free article: PMC3316552] [PubMed: 22479340]
- Gaskill, B. N., A. Z.Karas, J. P.Garner, and K. R.Pritchett-Corning. 2013a Nest building as an indicator of health and welfare in laboratory mice. J Vis Exp (82):51012. [PMC free article: PMC4108067] [PubMed: 24429701]
- Gaskill, B. N., K. R.Pritchett-Corning, C. J.Gordon, E. A.Pajor, J. R.Lucas, J. K.Davis, and J. P.Garner. 2013b Energy reallocation to breeding performance through improved nest building in laboratory mice. PLoS One8 (9):e74153. [PMC free article: PMC3770541] [PubMed: 24040193]
- Gonder, J. C., and K.Laber. 2007. A renewed look at laboratory rodent housing and management. ILAR J48 (1):29–36. [PubMed: 17170493]
- Gonzalez, D. M., S. J.Graciano, J.Karlstad, M.Leblanc, T.Clark, S.Holmes, and J. D.Reuter. 2011. Failure and life cycle evaluation of watering valves. J Am Assoc Lab Anim Sci50 (5):713–8. [PMC free article: PMC3189677] [PubMed: 22330720]
- Gordon, C. J., C.Aydin, E. A.Repasky, K. M.Kokolus, G.Dheyongera, and A. F.Johnstone. 2014. Behaviorally mediated, warm adaptation: A physiological strategy when mice behaviorally thermoregulate. J Therm Biol44:41–6. [PubMed: 25086972]
- Hasegawa, M., S.Kagiyama, M.Tajima, K.Yoshida, Y.Minami, and T.Kurosawa. 2003. Evaluation of a forced-air-ventilated micro-isolation system for protection of mice againstPasteurella pneumotropica. Exp Anim52 (2):145–51. [PubMed: 12806889]
- Hess, S. E., S.Rohr, B. D.Dufour, B. N.Gaskill, E. A.Pajor, and J. P.Garner. 2008. Home improvement: C57BL/6J mice given more naturalistic nesting materials build better nests. J Am Assoc Lab Anim Sci47 (6):25–31. [PMC free article: PMC2687128] [PubMed: 19049249]
- Hessler, J., and N.Lehner. 2009. Planning and Designing Research Animal Facililties. San Diego: Academic Press.
- Hessler, J. R.2011. Laboratory animal facilities and equipment for conventional, barrier, and containment housing systems. In Handbook of Laboratory Animal Science, ed. S. J.Schapiro and J.Hau, pp. 74–5. Boca Raton, FL: CRC Press.
- Hickman-Davis, J. M., M. L.Nicolaus, J. M.Petty, D. M.Harrison, and V. K.Bergdall. 2012. Effectiveness of shoe covers for bioexclusion within an animal facility. J Am Assoc Lab Anim Sci51 (2):181–8. [PMC free article: PMC3314521] [PubMed: 22776118]
- Howerton, C. L., J. P.Garner, and J. A.Mench. 2008. Effects of a running wheel-igloo enrichment on aggression, hierarchy linearity, and stereotypy in group-housed male CD-1 (ICR) mice. Appl Anim Behav Sci115 (1–2):90–103.
- Hoyt, R., J.Hawkins, M.St. Claire, and M.Kennett. 2007. Mouse physiology. In The Mouse in Biomedical Research, ed. W.Barthold, S.Fox, M. T.Davisson, F.Newcomer, W.Quimby, and A. L.Smith, 23–90. San Diego: Elsevier.
- Huerkamp, M. J., W. D.Thompson, and N. D.Lehner. 2003. Failed air supply to individually ventilated caging system causes acute hypoxia and mortality of rats. Contemp Top Lab Anim Sci42 (3):44–5. [PubMed: 19760835]
- Hutchinson, E., A.Avery, and S.Vandewoude. 2005. Environmental enrichment for laboratory rodents. ILAR J46 (2):148–61. [PubMed: 15775024]
- Hutchinson, E. K., A. C.Avery, and S.Vandewoude. 2012. Environmental enrichment during rearing alters corticosterone levels, thymocyte numbers, and aggression in female BALB/c mice. J Am Assoc Lab Anim Sci51 (1):18–24. [PMC free article: PMC3276961] [PubMed: 22330863]
- Inagaki, H., Y.Kiyokawa, Y.Takeuchi, and Y.Mori. 2010. The alarm pheromone in male rats as a unique anxiety model: Psychopharmacological evidence using anxiolytics. Pharmacol Biochem Behav94 (4):575–9. [PubMed: 19969015]
- Kogan, L. R., R.Schoenfeld-Tacher, and A. A.Simon. 2012. Behavioral effects of auditory stimulation on kenneled dogs. J Vet Behav7 (5):268–75.
- Kokolus, K. M., C. C.Hong, and E. A.Repasky. 2010. Feeling too hot or cold after breast cancer: Is it just a nuisance or a potentially important prognostic factor?Int J Hyperthermia26 (7):662–80. [PMC free article: PMC3012377] [PubMed: 20849261]
- Kokolus, K. M., H. M.Spangler, B. J.Povinelli, M. R.Farren, K. P.Lee, and E. A.Repasky. 2014. Stressful presentations: Mild cold stress in laboratory mice influences phenotype of dendritic cells in naive and tumor-bearing mice. Front Immunol5:23. [PMC free article: PMC3918933] [PubMed: 24575090]
- Konkle, A. T., A. C.Kentner, S. L.Baker, A.Stewart, and C.Bielajew. 2010. Environmental-enrichmentrelated variations in behavioral, biochemical, and physiologic responses of Sprague-Dawley and Long Evans rats. J Am Assoc Lab Anim Sci49 (4):427–36. [PMC free article: PMC2919182] [PubMed: 20819388]
- Korpi, A., S.Lappalainen, E.Kaliste, P.Kalliokoski, K.Reijula, and A. L.Pasanen. 2007. A multi-faceted approach to risk assessment of laboratory animal allergens at two facilities. Am J Ind Med50 (2):127–35. [PubMed: 17238142]
- Kulesskaya, N., H.Rauvala, and V.Voikar. 2011. Evaluation of social and physical enrichment in modulation of behavioural phenotype in C57BL/6J female mice. PLoS One6 (9):e24755. [PMC free article: PMC3169619] [PubMed: 21931844]
- LaVail, M. M., G. M.Gorrin, and M. A.Repaci. 1987. Strain differences in sensitivity to light-induced photoreceptor degeneration in albino mice. Curr Eye Res6 (6):825–34. [PubMed: 3608569]
- Leeds, J., and S.Wagner. 2008. Through a Dog’s Ear. Boulder, CO: Sounds True, Inc.
- Leggio, M. G., L.Mandolesi, F.Federico, F.Spirito, B.Ricci, F.Gelfo, and L.Petrosini. 2005. Environmental enrichment promotes improved spatial abilities and enhanced dendritic growth in the rat. Behav Brain Res163 (1):78–90. [PubMed: 15913801]
- Lipman, N. S.1999. Isolator rodent caging systems (state of the art): A critical view. Contemp Top Lab Anim Sci38 (5):9–17. [PubMed: 12086409]
- Lipman, N. S., B. F.Corning, and M. A.CoiroSr.. 1992. The effects of intracage ventilation on microenvironmental conditions in filter-top cages. Lab Anim26 (3):206–10. [PubMed: 1501435]
- MacRae, M., W. M.Kenkel, and A. C.Kentner. 2015. Social rejection following neonatal inflammation is mediated by olfactory scent cues. Brain Behav Immun49:43–8. [PubMed: 25744105]
- Maher, R. L., S. M.Barbash, D. V.Lynch, and S. J.Swoap. 2015. Group housing and nest building only slightly ameliorate the cold stress of typical housing in female C57BL/6J mice. Am J Physiol Regul Integr Comp Physiol308 (12):R1070–9. [PMC free article: PMC6345206] [PubMed: 25876655]
- Manser, C. E., D. M.Broom, P.Overend, and T. H.Morris. 1998. Investigations into the preferences of laboratory rats for nest-boxes and nesting materials. Lab Anim32 (1):23–35. [PubMed: 9481691]
- Memarzadeh, F., P. C.Harrison, G. L.Riskowski, and T.Henze. 2004. Comparison of environment and mice in static and mechanically ventilated isolator cages with different air velocities and ventilation designs. Contemp Top Lab Anim Sci43 (1):14–20. [PubMed: 14984284]
- Menon, V., and D. J.Levitin. 2005. The rewards of music listening: Response and physiological connectivity of the mesolimbic system. Neuroimage28 (1):175–84. [PubMed: 16023376]
- Molk, D. M., C. L.Karr-May, E. D.Trang, and G. E.Sanders. 2013. Sanitization of an automatic reverseosmosis watering system: Removal of a clinically significant biofilm. J Am Assoc Lab Anim Sci52 (2):197–205. [PMC free article: PMC3624790] [PubMed: 23562105]
- Narver, H. L.2013. Care and monitoring of a mouse model of melanoma. Lab Anim (NY)42 (3):92–8. [PubMed: 23423298]
- National Health and Medical Research Council. 2013. Australian code of practice for the care and use of animals for scientific purposes. Canberra: National Health and Medical Research Council. https://www
.nhmrc.gov .au/guidelines-publications/ea16. - Neuman, J. C., K. A.Albright, and K. L.Schalinske. 2013. Exercise prevents hyperhomocysteinemia in a dietary folate-restricted mouse model. Nutr Res33 (6):487–93. [PubMed: 23746565]
- Nicholson, A., R. D.Malcolm, P. L.Russ, K.Cough, C.Touma, R.Palme, and M. V.Wiles. 2009. The response of C57BL/6J and BALB/cJ mice to increased housing density. J Am Assoc Lab Anim Sci48 (6):740–53. [PMC free article: PMC2786928] [PubMed: 19930822]
- NRC (National Research Council). 2011. Guide for the Care and Use of Laboratory Animals. 8th ed. Washington, DC: National Academies Press. [PubMed: 21595115]
- Olsson, I. A. S., C. M.Nevison, E. G.Patterson-Kane, C. M.Sherwin, H. A.Van de Weerd, and H.Würbel. 2003. Understanding behaviour: The relevance of ethological approaches in laboratory animal science. Appl Anim Behav Sci81 (3):245–64.
- Panksepp, J., and W. W.Beatty. 1980. Social deprivation and play in rats. Behav Neural Biol30 (2):197–206. [PubMed: 7447871]
- Patterson-Kane, E. P.2011. Horizons of enrichment: The history, accomplishments, obstacles and aspirations of environmental enrichment. Enrichment Rec7 (April):12–14.
- Patterson-Kane, E. P., M.Hunt, and D.Harper. 2004. Short communication: Rat’s demand for group size. J Appl Anim Welf Sci7 (4):267–72. [PubMed: 15857812]
- Pritchett-Corning, K. R., R.Keefe, J. P.Garner, and B. N.Gaskill. 2013. Can seeds help mice with the daily grind?Lab Anim47 (4):312–5. [PubMed: 23760566]
- Ras, T., M.van de Ven, E. G.Patterson-Kane, and K.Nelson. 2002. Rats’ preferences for corn versus woodbased bedding and nesting materials. Lab Anim36 (4):420–5. [PubMed: 12396285]
- Reeb, C., R.Jones, D.Bearg, H.Bedigan, D.Myers, and B.Paigen. 1998. Microenvironment in ventilated animal cages with differing ventilation rates, mice populations, and frequency of bedding changes. Contemp Top Lab Anim Sci37 (2):43–49. [PubMed: 12456167]
- Reeb-Whitaker, C. K., B.Paigen, W. G.Beamer, R. T.Bronson, G. A.Churchill, I. B.Schweitzer, and D. D.Myers. 2001. The impact of reduced frequency of cage changes on the health of mice housed in ventilated cages. Lab Anim35 (1):58–73. [PubMed: 11201289]
- Rosenbaum, M. D., S.VandeWoude, and T. E.Johnson. 2009. Effects of cage-change frequency and bedding volume on mice and their microenvironment. J Am Assoc Lab Anim Sci48 (6):763–73. [PMC free article: PMC2786931] [PubMed: 19930825]
- Rosenbaum, M. D., S.VandeWoude, J.Volckens, and T.Johnson. 2010. Disparities in ammonia, temperature, humidity, and airborne particulate matter between the micro- and macroenvironments of mice in individually ventilated caging. J Am Assoc Lab Anim Sci49 (2):177–83. [PMC free article: PMC2846005] [PubMed: 20353692]
- Russell, W. M. S., and R. L.Burch. 1959. The Principles of Humane Experimental Technique. London: Methuen.
- Sanderson, A. E., H. M.Multari, J. J.Lohmiller, and S. R.Boutin. 2010. Effect of cage-change frequency on rodent breeding performance. Lab Anim (NY)39 (6):177–82. [PubMed: 20485357]
- Semple, B. D., L. J.Noble-Haeusslein, Y.Jun Kwon, P. N.Sam, A. M.Gibson, S.Grissom, S.Brownet al.2014. Sociosexual and communication deficits after traumatic injury to the developing murine brain. PLoS One9 (8):e103386. [PMC free article: PMC4126664] [PubMed: 25106033]
- Singapore Government National Advisory Committee for Laboratory Animal Research. 2004. Animals and birds act guidelines on the care and use of animals for scientific purposes. Singapore: Singapore Government National Advisory Committee for Laboratory Animal Research.
- Smith, A. L., and D. J.Corrow. 2005. Modifications to husbandry and housing conditions of laboratory rodents for improved well-being. ILAR J46 (2):140–7. [PubMed: 15775023]
- Smith, E., J. D.Stockwell, I.Schweitzer, S. H.Langley, and A. L.Smith. 2004. Evaluation of cage micro-environment of mice housed on various types of bedding materials. Contemp Top Lab Anim Sci43 (4):12–17. [PubMed: 15264763]
- Smith, M. M., and M.Hargaden. 2001. Developing a rodent enrichment program. Lab Anim (NY)30 (8):36–41. [PubMed: 11910415]
- Spani, D., M.Arras, B.Konig, and T.Rulicke. 2003. Higher heart rate of laboratory mice housed individually vs in pairs. Lab Anim37 (1):54–62. [PubMed: 12626072]
- Stockley, P., L.Bottell, and J. L.Hurst. 2013. Wake up and smell the conflict: Odour signals in female competition. Philos Trans R Soc Lond B Biol Sci368 (1631):20130082. [PMC free article: PMC3826211] [PubMed: 24167312]
- Timmins, R. P., K. D.Cliff, C. T.Day, B. L.Hart, L. A.Hart, R. C.Hubrecht, K. F.Hurleyet al.2007. Enhancing quality of life for dogs and cats in confined situations. Anim Welf16 (2):83–7.
- Toth, L. A.2015. The influence of the cage environment on rodent physiology and behavior: Implications for reproducibility of pre-clinical rodent research. Exp Neurol270:72–7. [PubMed: 25913548]
- Toth, L. A., K.Kregel, L.Leon, and T. I.Musch. 2011. Environmental enrichment of laboratory rodents: The answer depends on the question. Comp Med61 (4):314–21. [PMC free article: PMC3155397] [PubMed: 22330246]
- United States. 1985. Health Research Extension Act of 1985. Public Law 99-158. U.S. Statutes at Large99 (Title IV, Sections 1–12), 99–158. [PubMed: 11686169]
- Van Loo, P. L., and V.Baumans. 2004. The importance of learning young: The use of nesting material in laboratory rats. Lab Anim38 (1):17–24. [PubMed: 14979984]
- Van Loo, P. L. P., C. L. J. J.Kruitwagen, L. F. M.Van Zutphen, J. M.Koolhaas, and V.Baumans. 2000. Modulation of aggression in male mice: Influence of cage cleaning regime and scent marks. Anim Welf9 (3):281–95.
- Van Loo, P. L. P., E.Van der Meer, C. L. J. J.Kruitwagen, J. M.Koolhaas, L. F. M.Van Zutphen, and V.Baumans. 2004. Long-term effects of husbandry procedures on stress-related parameters in male mice of two strains. Lab Anim38 (2):169–77. [PubMed: 15070457]
- Van Loo, P. L. P., L. F. M.Van Zutphen, and V.Baumans. 2003. Male management: Coping with aggression problems in male laboratory mice. Lab Anim37:300–13.
- Varlinskaya, E. I., L. P.Spear, and N. E.Spear. 1999. Social behavior and social motivation in adolescent rats. Physiol Behav67 (4):475–82. [PubMed: 10549884]
- Varty, G. B., M. P.Paulus, D. L.Braff, and M. A.Geyer. 2000. Environmental enrichment and isolation rearing in the rat: Effects on locomotor behavior and startle response plasticity. Biol Psychiatry47 (10):864–73. [PubMed: 10807959]
- Viegas, J.2010. Male mice sing ultrasonic love songs. March5. http://news
.discovery .com/animals/zoo-animals /mouse-courtship-songs-ultrasonic .htm. - Voznessenskaya, V. V.2014. Influence of cat odor on reproductive behavior and physiology in the house mouse: (Mus musculus). In Neurobiology of Chemical Communication, ed. C.Mucignat-Caretta, pp. 403–5. Boca Raton, FL: CRC Press. [PubMed: 24830030]
- Weary, D. M.2013. What is suffering in animals?23. http://awp
.landfood.ubc .ca/files/2013/06/Weary_suffering.pdf. - Wells, D. L., L.Graham, and P. G.Hepper. 2002. The influence of auditory stimulation on the behaviour of dogs housed in a rescue shelter. Anim Welf11 (4):385–93.
- Whary, M. T., N.Baumgarth, J. G.Fox, and S. W.Barthold. 2015. Biology and diseases of mice. In Laboratory Animal Medicine, ed. L. C.Anderson, G.Otto, K. R.Pritchett-Corning, and M. T.Whary. San Diego: Elsevier.
- Wheeler, R. R., M. P.Swan, and D. L.Hickman. 2014. Effect of multilevel laboratory rat caging system on the well-being of the singly-housed Sprague Dawley rat. Lab Anim49 (1). [PubMed: 25117586]
- Whiteman, B.2001. ”Moosic study’ reveals way of increasing milk yields. University of Leicester. http://www
.le.ac.uk/press /press/moosicstudy.html (accessed October 16, 2014). - Whittaker, A. L., G. S.Howarth, and D. L.Hickman. 2012. Effects of space allocation and housing density on measures of wellbeing in laboratory mice: A review. Lab Anim46 (1):3–13. [PubMed: 22114068]
- Winnicker, C.2012. Mice no longer out in the cold. January10. http://www
.criver.com /about-us/eureka/blog /january-2012/mice-no-longer-out-in-the-cold. - Wirth, H.1983. Criteria for the evaluation of laboratory animal bedding. Lab Anim17 (2):81–4. [PubMed: 6865326]
- Ying, H., X.Lan-Wen, Y.Fei and Y.Ping. 2007. The effects of enrichment with music or colorful light on the welfare of restrained mice. Lab Anim Comp Med27 (2):71–6.
- York, J. M., A. W.McDaniel, N. A.Blevins, R. R.Guillet, S. O.Allison, K. A.Cengel, and G. G.Freund. 2012. Individually ventilated cages cause chronic low-grade hypoxia impacting mice hematologically and behaviorally. Brain Behav Immun26 (6):951–8. [PMC free article: PMC3398166] [PubMed: 22561683]
- Review Environmental Factors: Macroenvironment versus Microenvironment.[Management of Animal Care and ...]Review Environmental Factors: Macroenvironment versus Microenvironment.Hogan MC, Norton JN, Reynolds RP. Management of Animal Care and Use Programs in Research, Education, and Testing. 2018
- Review Behavioral Management Programs to Promote Laboratory Animal Welfare.[Management of Animal Care and ...]Review Behavioral Management Programs to Promote Laboratory Animal Welfare.Bloomsmith MA, Perlman JE, Hutchinson E, Sharpless M. Management of Animal Care and Use Programs in Research, Education, and Testing. 2018
- Review Feed and Bedding.[Management of Animal Care and ...]Review Feed and Bedding.Carter RL, Lipman NS. Management of Animal Care and Use Programs in Research, Education, and Testing. 2018
- Review Large (Nonagricultural) Animal Enclosures and Housing.[Management of Animal Care and ...]Review Large (Nonagricultural) Animal Enclosures and Housing.Wyatt JD. Management of Animal Care and Use Programs in Research, Education, and Testing. 2018
- Review Wire-bottom versus solid-bottom rodent caging issues important to scientists and laboratory animal science specialists.[Contemp Top Lab Anim Sci. 2001]Review Wire-bottom versus solid-bottom rodent caging issues important to scientists and laboratory animal science specialists.Stark DM. Contemp Top Lab Anim Sci. 2001 Nov; 40(6):11-4.
- Small Animal Enclosures and Housing - Management of Animal Care and Use Programs...Small Animal Enclosures and Housing - Management of Animal Care and Use Programs in Research, Education, and Testing
Your browsing activity is empty.
Activity recording is turned off.
See more...