NCBI Bookshelf. A service of the National Library of Medicine, National Institutes of Health.
Breman JG, Alilio MS, White NJ, editors. Defining and Defeating the Intolerable Burden of Malaria III: Progress and Perspectives: Supplement to Volume 77(6) of American Journal of Tropical Medicine and Hygiene. Northbrook (IL): American Society of Tropical Medicine and Hygiene; 2007 Dec.
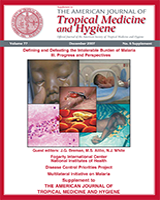
Defining and Defeating the Intolerable Burden of Malaria III: Progress and Perspectives: Supplement to Volume 77(6) of American Journal of Tropical Medicine and Hygiene.
Show detailsArtemisinin-based combination treatments (ACTs) are now generally accepted as the best treatments for uncomplicated falciparum malaria. They are rapidly and reliably effective. Efficacy is determined by the drug partnering the artemisinin derivative and, for artesunate–mefloquine, artemether–lumefantrine, and dihydroartemisinin–piperaquine, this usually exceeds 95%. Artesunate–sulfadoxine–pyrimethamine and artesunate–amodiaquine are effective in some areas, but in other areas resistance to the partner precludes their use. There is still uncertainty over the safety of artemisinin derivatives in the first trimester of pregnancy, when they should not be used unless there are no effective alternatives. Otherwise, except for occasional hypersensitivity reactions, the artemisinin derivatives are safe and remarkably well tolerated. The adverse effect profiles of the artemisinin-based combination treatments are determined by the partner drug. Most malaria endemic countries have now adopted artemisinin-based combination treatments as first-line treatment of falciparum malaria, but in most of these only a minority of the patients that need artemisinin-based combination treatments actually receive them.
Introduction
Falciparum malaria is a mass killer that went out of control. The drug treatments for this potentially lethal infection that have been most widely recommended and provided over the past 50 years (i.e., chloroquine and sulfadoxine–pyrimethamine) no longer work in most tropical countries. Resistance to these drugs emerged in Asia and South America and spread to Africa. As resistance worsened, morbidity and mortality rose as a direct consequence. But this was not appreciated because surveillance was poor, and the clinical and epidemiologic methods used to measure morbidity, mortality, and drug resistance were insensitive. After much procrastination the seriousness of the situation was finally appreciated in the 1990s, although antimalarial policy did not change from ineffective to effective antimalarial drug treatment until the past 3 years in most countries. Replacing the failing chloroquine and sulfadoxine–pyrimethamine with effective drugs required increased donor support because most endemic countries could barely afford the failing medicines, let alone more expensive ones. This was not forthcoming initially. Fortunately things are changing for the better. There is now considerably more funding available for malaria control in endemic countries, particularly from the Global Fund (GFATM). The treatments now recommended by the World Health Organization and supported by the GFATM for uncomplicated falciparum malaria are artemisinin-based combination treatments (ACT); these are combinations of an artemisinin derivative and another structurally unrelated and more slowly eliminated antimalarial. WHO has also “raised the bar” recently in recommending that cure rates should be at least 90% and preferably > 95% assessed at ≥ 28 days.1 Failure rates at 14 days of 25% (which are compatible with true failure rates up to 60%2) are no longer considered acceptable. Provided the partner drug is effective, then ACTs ensure prompt recovery and high cure rates, and they are generally well tolerated. Replacing the older failing or failed monotherapies with effective drugs will reduce morbidity and mortality. In lower transmission settings if coverage is good, then incidence will fall. Policy has now changed in most countries (Figure 1) but the challenge now is to deliver these drugs to the people that need them. Donor support has increased, there are better drugs, and there are better mechanisms for delivery, but there is still an enormous gap between the funds and drugs available and the global need.
Rationale
Malaria is a (eukaryotic) protozoan parasite of red blood cells that may reach burdens as high as 1013 in the blood of the human host—although most symptomatic infections are caused by between 107 and 1012 parasites. The theory underlying combination drug treatment of tuberculosis, leprosy, and HIV infection and many cancers is now well known, and the same general principle is now widely accepted for malaria. If two drugs are used with different modes of action, and therefore different resistance mechanisms, then the per-parasite probability of developing resistance to both drugs at the same cell division is the product of their individual per-parasite probabilities.3–6 This is of particular relevance to malaria because on any one day there are only about 1017 malaria parasites in the entire world.7,8 Most identified mechanisms of antimalarial drug resistance result from genetic mutation. Mutation rates for eukaryotes are of the order of 1 in 106 divisions but viable resistant mutant parasites are selected at much lower frequencies. Gene duplications occur more readily than mutations throughout the parasite genome and may also contribute to drug resistance. Amplification of Pfmdr, which results in increased transcription, and therefore more of this protein “pump” per cell, is the main contributor to mefloquine resistance, but at a fitness cost for the parasite.9,10 The highest frequencies documented for the de novo emergence of mutations conferring drug resistance in acute malaria in humans are for atovaquone and pyrimethamine at around 1 in 1012 parasites.7 So if the per parasite probability of developing resistance to 2 drugs (A and B) are both high at 1 in 1012, then a simultaneously resistant mutant (i.e., resistant to both A and B) will arise spontaneously every 1 in 1024 parasites. But because there is a cumulative total of less than 1020 malaria parasites in existence each year, such a simultaneously resistant parasite would arise spontaneously roughly once every 10,000 years, provided the drugs always confronted the parasites in combination.7 Thus provided the de novo per parasite probability of developing resistance is not much higher than 1 in 1012 cell divisions and both drugs are present together at inhibitory concentrations, then combinations markedly delay the emergence of resistance. But for ACTs, because the artemisinin derivatives are eliminated rapidly, and the partner drugs are eliminated slowly, there is complete protection only for the artemisinin derivative. The combination still provides good protection against the emergence of resistance to the partner drug, but once resistance has developed the residual concentrations of unprotected partner drug do provide a selective filter enhancing the spread of resistance to the partner compound.7,8 Figure 2 shows the pharmacokinetic–pharmacodynamic rationale for ACTs using artesunate–mefloquine as an example.
Although parasite lines with reduced susceptibility can be selected in the laboratory, therapeutically significant resistance to the artemisinin derivatives has not yet been identified. High-grade stable artemisinin resistance cannot be confirmed yet in the laboratory, which suggests that it may be a rare event. The recent report that some parasites from French Guyana with mutations in the gene encoding the putative target PfATPase6 were highly resistant to artemether has raised concerns,11 but these parasites have not been cultured, and this finding must be confirmed. Treatment failure rates are higher and parasite clearance times longer with ACTs in Western Cambodia than elsewhere, the epicentre of drug resistance in Southeast Asia. Even if reduced susceptibility to artemisinin derivatives is not substantiated, and despite the reassuring laboratory studies, it would still be foolish to assume that resistance to these valuable drugs will not happen. If high-level stable resistance to the artemisinin drugs does arise it would be a disaster for the malaria-affected world because they are now the cornerstone of antimalarial treatment. For mutual protection against the emergence of drug resistance, these drugs should be used only in combination with other antimalarials, such that no parasite “sees” the artemisinin component without the other drug being present.
Artemisinin derivatives are particularly effective in combinations because of their high killing rates (parasite reduction ratios RR circa 10,000 fold per cycle),6,7 lack of adverse effects, and absence of significant resistance. In ACTs a 3-day treatment course exposes 2 asexual cycles and so reduces the number of parasites in the body by approximately one hundred million-fold. The gametocytocidal activity of the artemisinin compounds is an important bonus reducing transmissibility and thus further reducing malaria incidence in low-transmission settings.12–14
Artemisinin and its derivatives are the most rapidly eliminated of all antimalarials with half-lives of approximately 1 hour. The “ideal” pharmacokinetic properties for an antimalarial drug have been a subject of much discussion. As described, from a resistance prevention perspective, the combination partners should have similar pharmacokinetic properties to provide optimum mutual protection. Slow elimination of the partner drug allows 3-day regimens to be given, but at the price of providing days or weeks of subtherapeutic blood levels that provide a selective filter for resistant parasites acquired from elsewhere, and thereby encouraging the spread of resistance15–17 (Figure 2). On the other hand these residual “prophylactic” levels18 suppress new infections giving a period of post treatment prophylaxis (PTP) which, in high-transmission settings, may improve clinical and hematological recovery. Rapid elimination ensures that the residual concentrations do not provide a selective filter for resistant parasites, but rapidly eliminated drugs (if used alone) do not provide any PTP, must be given for 7 days, and adherence to 7-day regimens is poor.6 Incomplete treatment encourages resistance. Even 7-day regimens of artemisinin derivatives (as monotherapy) are associated with approximately 10% failure rates.19,20 Thus to be highly effective in a 3-day regimen, the terminal elimination half-life of at least one drug component must exceed 24 hours (longer for less active drugs) such that concentrations in the fourth drug-exposed asexual cycle (7 to 8 days after starting treatment) are still sufficient to suppress multiplication of the most resistant parasites prevalent.6,7 Provided there is not high-level resistance to the partner drug, then ACTs provide complete protection for the artemisinin derivatives from selection of a de novo resistant mutant if adherence is good (i.e., no parasite is exposed to artemisinin during one asexual cycle without the partner being present), but this does leave the partner’s slowly eliminated “tail” unprotected by the artemisinin derivative. However, because artemisinin and its derivatives reduce parasite numbers by approximately 10,000-fold per 2- day asexual cycle, the residual number of parasites exposed to the slowly eliminated partner drug alone, after 2 asexual cycles of artemisinin exposure, is a tiny fraction (< 0.0001%) of those present at the peak of the acute symptomatic infection (Figure 2). Furthermore these residual parasites are exposed to relatively high levels of partner drug and, even if susceptibility was reduced, these levels are usually sufficient to eradicate the infection. But the long elimination phase “tail” of the partner drug does provide a selective filter for resistant parasites acquired from elsewhere, and thereby contributes to the spread of resistance once it has developed. Although the greatest use of antimalarials is in high-transmission areas, historically resistance has emerged and spread most rapidly in low-transmission settings. This illustrates the important role of host immunity in delaying the emergence and spread of resistance.
Deployment
The main obstacles to the success of combination treatment in preventing the emergence of resistance will be inadequate treatment (e.g., substandard drugs, incorrect dosing, unusual pharmacokinetics, poor adherence) and, as for antituberculous drugs, use of one of the combination partners alone. This is why blister packing has been encouraged and fixed dose combinations are now being developed and recommended. Cost is a major obstacle to ensuring adequate treatment because patients may not have enough money to purchase a full course of treatment or, once they feel better, will keep the remaining prescribed drugs for themselves or a family member when they next fall ill. Poor quality drugs are common in tropical areas of the world and counterfeit medicines are a major concern. Antimalarials are available widely in the market place, and often sold at incorrect doses or without correct advice. Even when a correct course is obtained adherence to antimalarial treatment regimens is often incomplete. Resistance to the artemisinins may not have happened yet, but it would be unwise to be complacent. If artemisinin resistance does emerge it will most likely arise in a hyperparasitemic patient who received an inadequate dose of a single antimalarial drug (i.e., not in combination with another suitable antimalarial agent).7 Irrespective of the epidemiologic setting, ensuring that patients with high parasitemias receive a full course of treatment with adequate doses of ACTs would be an effective method of slowing the de novo emergence of antimalarial drug resistance.
Ideally, to ensure the maximum useful therapeutic life, there should be no resistance to the partner drug in an ACT, yet on the Northwestern border of Thailand, an area of low transmission where mefloquine resistance had developed already, systematic deployment of artesunate–mefloquine combination therapy was dramatically effective both in stopping resistance, and also in reducing the incidence of malaria.14,21 In fact mefloquine resistance declined after widespread deployment of artesunate–mefloquine.22 In this setting before ACTs were introduced when mefloquine monotherapy was used, resistant parasites had a survival and transmission advantage—which was negated by the artesunate–mefloquine combination treatment. Mefloquine resistance develops rapidly because gene amplification is a relatively frequent mitotic event in P. falciparum, but it may also go rapidly as deamplification is also frequent, and Pfmdr amplification carries a fitness cost. But for the other drugs and resistance mechanisms involving mutations, deploying an ACT containing a failing drug may not lead to a reversal of resistance, and could eventually leave the artemisinin component inadequately protected as resistance to the partner worsens. Four ACTs are currently recommended by WHO: artesunate–mefloquine, artesunate–sulfadoxine–pyrimethamine (SP), artesunate–amodiaquine, and artemether–lumefantrine. The combinations containing SP and amodiaquine are both threatened by resistance.1
Access
Artemisinin-based combination treatments are highly effective and really could make a major contribution to global malaria control, but despite the recent changes in policy in most malaria endemic countries (Figure 1) only a minority of people who need these drugs actually receive them. Cost and access remain formidable obstacles. Few public health structures reach out far enough or efficiently enough to provide good coverage of effective and affordable drugs to rural communities. The Institute of Medicine reviewed and assessed the various deployment options available.23 The review recognized that the private sector still played a major role in anti-malarial drug delivery in most tropical countries, and concluded that existing frameworks for antimalarial drug delivery would not provide sufficient ACT coverage to make a major impact on malaria, would not provide sufficient protection against the emergence of artemisinin resistance, and would continue to provide incentives to fake or substandard drugs. The IOM committee recognized the potential contribution ACTs could make to global malaria control and recommended subsidizing the cost of ACTs both to the public and to the private sectors such that these new effective treatments would cost no more than chloroquine to the end user. Thus market forces would drive the ICT distribution. They noted that antimalarial drug resistance has usually arisen in Asia and therefore preventing resistance arising in Asia should benefit Africa. There is increasing support for this initiative, but there is much work to do. The current challenge is to raise sufficient funds, to work out how best to operate such a subsidy, and how to put in place all the necessary monitoring and evaluation mechanisms necessary.
Pharmacology
Artemisinin, the parent drug, has now largely given way to the more potent dihydroartemisinin and its derivatives, artemether, artemotil, and artesunate. The 3 derivatives are all converted back in vivo to dihydroartemisinin. Oral absorption is good (bioavailability > 60%) with peak concentrations usually achieved within 4 hours. Dihydroartemisinin has more variable oral bioavailability dependent on the excipients in the oral formulation.24–30 All are eliminated rapidly by metabolic biotransformation with half-lives of 1 hour or less (Table 1). Artesunate is readily hydrolyzed at neutral and acidic pH, and deesterification is accelerated by red cell and plasma esterases. Artemether and artemotil are biotransformed more slowly, being demethylated by metabolic transformation (principally by CYP 3A4).31 These drugs are highly active against all Plasmodium species. The artemisinin derivatives are the most rapidly acting of all antimalarial drugs and produce the fastest clinical responses to treatment. They are noticeably better than other antimalarial drugs. They have a broad spectrum of antimalarial activity acting against the young ring form parasites and preventing their development to the more mature pathogenic stages.6 This explains the rapid therapeutic responses obtained with the artemisinin derivatives. This effect is particularly important in the management of severe malaria, where artesunate has been shown to reduce mortality. Artemether and artemotil have slightly less antimalarial activity than artesunate in vitro but all 3 compounds are readily converted in vivo to dihydroartemisinin, which contributes the majority of antimalarial effect in treatment. Dose regimens of the drugs are similar. In combination with more slowly eliminated antimalarials, the addition of artesunate, artemether, or dihydroartemisinin consistently improves cure rates (unless they were 100% already) and has the advantage of producing a more rapid clinical response to treatment, reduction in gametocyte carriage, and thus malaria transmission, and, when deployed extensively in low-transmission areas, a reduction in malaria incidence.12–14 A 3-day course of the artemisinin derivative in combination with a slowly eliminated partner drug (elimination half life > 1 day) is required for optimum cure rates. Two-day and 1-day courses are insufficient because they expose only one asexual cycle to the artemisinin derivative. If the partner drug is effective then such regimens will still give high cure rates, but they will not provide sufficient protection against the emergence of resistance. There has been some investigation of the optimum dose with in-vivo dose response studies suggesting that at least 2 mg/kg/d for 3 days of artesunate is required for maximal effect.32 Current ACTs regimens contain between 2.5 and 4 mg/kg of artemisinin derivative given daily for 3 days. They are easy to use, and are well tolerated. There are no specific pediatric formulations, although a pediatric formulation of artemether–lumefantrine and fixed dose combinations of artesunate–mefloquine and artesunate–amodiaquine with pediatric tablet sizes have been developed.33
Table 1
Summary of pharmacokinetic properties of antimalarial drugs that have been used in artemisinin combination treatments
Adverse Effects
Artemisinin and its derivatives are safe and remarkably well tolerated. There have been reports of mild gastrointestinal disturbances, dizziness, tinnitus, and bradycardia, although none of these associations are convincing.34 The only potentially serious adverse effect that has been reported with this class of drugs in clinical trials is type 1 hypersensitivity reactions in approximately 1 in 3,000 patients.35 Transient reticulocytopenia, neutropenia, and elevated liver enzyme values have been reported but none have been clinically significant. Electrocardiographic abnormalities, including prolongation of the QT interval, have also been reported although most studies have not found any significant electrocardiographic abnormalities. Indeed the weight of evidence suggests these drugs have no adverse cardiovascular effects at all.34 In severe malaria, as with quinine, blackwater fever has been reported. In all species of animals tested, intramuscular artemether and artemotil cause an unusual selective pattern of neuronal damage to certain brain stem nuclei.36,37 Neurotoxicity in experimental animals is related to the sustained blood concentrations that follow intramuscular administration of the oil-based derivatives artemether and artemotil, because it is much less evident when the same doses are given orally, or with similar doses of water-soluble drugs such as artesunate, despite transiently higher blood concentrations.38–41 With the single exception of one much disputed report,42,43 extensive clinical, neurophysiological, and pathologic studies in humans have not shown similar findings with therapeutic use of these compounds.44–48 Artemisinin and its derivatives inhibit erythropoesis in the early fetus, and cause fetal resorption in all experimental animals tested to date. This can in some circumstances lead to developmental abnormalities in rodents and rabbits.49–51 Because these drugs have not been evaluated extensively in early pregnancy in humans, they should be avoided in patients in the first trimester of pregnancy with uncomplicated malaria until more information is available (see below).1 There is no evidence for adverse effects on the fetus exposed in the second and third trimesters, when these drugs are recommended depending on the safety profile of the partner drug. The safety profile of ACTs is therefore determined by the partner drug. Large-scale safety monitoring or pharmacovigilance is often talked about in the context of antimalarial drugs, but it is difficult, and it is not often done. As deployment increases the ACTs will be used with increasing frequency in individuals. More information on safety with frequent dosing is needed.
The ACTs
Chloroquine resistance is now so widespread that it is not included in currently recommended ACTs.52 Artesunate–SP and artesunate–amodiaquine are used in areas with drug susceptibility to these drugs. In areas where resistance to sulphonamide–pyrimethamine, chloroquine, and amodiaquine is prevalent, then artemisinin combinations with either lumefantrine or mefloquine are the current alternatives. Soon these drugs will be joined by ACTs in fixed dose coformulations containing piperaquine, pyronaridine, or chlorproguanil–dapsone. In general, blister-packed oral formulations of these drugs have shelf-lives in tropical conditions of 2 years. Longer shelf-lives would considerably facilitate deployment.
Artesunate–Sulfadoxine–Pyrimethamine
Sulfadoxine-pyrimethamine is a fixed combination of a long-acting sulfonamide and the antifolate pyrimethamine. These are synergistic against sensitive parasites. Minor adverse effects are unusual. Serious sulfonamide toxicity is unusual with a single-dose treatment of malaria. The anti-folate properties of pyrimethamine rarely produce toxicity. The combination with artesunate is available as separate scored tablets containing 50 mg of artesunate and tablets containing 500 mg of sulfadoxine and 25 mg of pyrimethamine. There are no plans for developing a fixed dose combination. The total recommended treatment is 4 mg/kg BW of artesunate, given once a day for 3 days and a single administration of sulfadoxine–pyrimethamine 1.25/25 mg base/kg BW on admission. This SP dose was developed in adults but in the main target group (children aged 2–5 years) the weight adjusted dose produced blood concentrations of both components that are approximately half those in adults.53 Thus the standard dose may be sub-optimal in younger children. The combination has been evaluated extensively in adults and children with uncomplicated malaria and is sufficiently efficacious in areas where 28-day cure rates with sulfadoxine–pyrimethamine alone exceed 80%.52,54 This ACT is currently being used in parts of South America, the Middle East, and South Asia where SP susceptibility remains high. Because sulfadoxine–pyrimethamine, sulfalene–pyrimethamine, and trimethoprim–sulfamethoxazole (cotrimoxazole) are still widely used as “monotherapies,” resistance is likely to worsen.
Artesunate–Amodiaquine
Amodiaquine, like chloroquine, is a 4-aminoquinoline; it is effective against chloroquine-resistant strains of P. falciparum, although there is some cross-resistance. In recent years resistance has worsened considerably in parts of East and Southern Africa. After oral administration amodiaquine is largely converted to desethylamodiaquine, which contributes the majority of antimalarial activity. Amodiaquine is generally reasonably well tolerated and slightly more palatable than chloroquine, although in some areas it has not been a popular substitute. The serious adverse effects that have been associated with its prophylactic use (agranulocytosis and severe liver toxicity) are considered rare when amodiaquine is used in malaria treatment, although more data are needed to characterize the risks. More data are also required in pregnancy. The combination of amodiaquine with artesunate is currently available in blister packs as separate scored tablets containing 50 mg of artesunate and 153 mg base of amodiaquine, but co-formulated tablets have been developed recently by the Drugs for Neglected Diseases initiative (DNDi). The total recommended treatment is 4 mg/kg BW of artesunate and 10 mg base/kg BW of amodiaquine once a day for 3 days. Artesunate–amodiaquine has proved to be an efficacious combination in areas where 28-day cure rates with amodiaquine monotherapy exceed 80%.55–57
Artemether–Lumefantrine
This was the first fixed dose combination of an artemisinin derivative with a second unrelated antimalarial compound. Lumefantrine (formerly benflumetol) is an aryl amino-alcohol in the same general group as mefloquine and halofantrine. It was discovered and developed in the People’s Republic of China. Lumefantrine is active against all the human malaria parasites, including multi-drug–resistant P. falciparum (although there is some cross-resistance with halofantrine and mefloquine). Artemether–lumefantrine is dispensed as tablets containing 80/480 mg respectively. It was introduced originally as a 4-dose regimen given at 0, 8, 24, and 48 hours. This shorter course proved insufficiently efficacious. Pharmacokinetic–pharmacodynamic (PK–PD) studies indicated that the principal PK determinant of cure was the area under the plasma lumefantrine concentration time curve (AUC), or its surrogate, the day 7 lumefantrine level.58 Lumefantrine absorption (like that of atovaquone and halofantrine) is critically dependent on co-administration with fats and thus plasma concentrations vary markedly between patients.58 In Thailand day 7 levels over 500 ng/mL were associated with > 90% cure rates. With the 4-dose regimen plasma concentrations of lumefantrine during the third and fourth post-treatment cycles (4–8 days) were insufficient to eradicate all infections. To increase the AUC and thus cure rate, a 6-dose regimen (adult dose 80/480 mg at 0, 8, 24, 36, 48, and 60 hours) was then evaluated.59 This has proved highly effective and remarkably well tolerated. Against multi-drug–resistant falciparum malaria the 6-dose regimen of artemether–lumefantrine is generally as effective as and better tolerated than artesunate–mefloquine.60–64 Artemether–lumefantrine is becoming increasingly available in tropical countries. The excellent adverse effects profile and recent price reductions (down to US $1 per adult treatment) make it an increasingly attractive treatment option. New formulations have also been produced but these must demonstrate comparable bioavailability with the original formulation before they can be recommended. However, the complexity of the treatment (2 doses per day) and the required fat co-administration (albeit small amounts; at least 1.2 g/dose) are obstacles.65 Once a day dosing is not an option because absorption is dose limited.66 There is increasing evidence of safety for this combination in the second and third trimesters of pregnancy. But plasma concentrations of artemether, the metabolite dihydroartemisinin, and lumefantrine are all significantly reduced in late pregnancy, suggesting that a longer course of treatment may be needed in this vulnerable patient group.67
Artesunate–Mefloquine
Mefloquine is a quinoline methanol compound related to quinine.68 Several different mefloquine formulations are now available with different oral bioavailability. Employment of mefloquine as monotherapy for the management of malaria has lead to rapid spread of resistance, mediated mainly by an increase in copy number and expression of the P. falciparum multi-drug resistance (MDR) gene (Pfmdr1).69 There is theoretical evidence to suggest that initial deployment of the lower dose of mefloquine encourages resistance, and that initial use of higher doses, preferably in combination with an artemisinin derivative is less likely to lead to resistance.70 Where adherence can be assured the dose should be split at 15 mg/kg initially followed 8–24 hours later by a second 10 mg/kg or as 8.3 mg/kg daily for 3 days (this is approximately the dose in the new fixed dose combination). This improves bioavailability and reduces vomiting.71 There is no formulation of mefloquine for children. Despite earlier restrictions there is no reason to withhold mefloquine from young children. Limited information suggested that mefloquine was probably safe in pregnancy, although the observation in Thailand of an increased stillbirth risk when mefloquine was used in treatment at any stage of pregnancy has cast uncertainty over its use in pregnant women.72 This effect was not seen in large studies conducted in Malawi.73 Mefloquine commonly induces nausea, dysphoria, and dizziness, and in approximately 1:1,000 Asian patients, and up to 1:200 Caucasians or African subjects, mefloquine treatment induces a self-limiting acute neuropsychiatric syndrome manifest by encephalopathy, convulsions, or psychosis.34 Suicide has been reported. The risks of this acute neuropsychiatric syndrome are increased if the patient has a previous history of psychiatric illness or epilepsy. There is a considerable increase in the risk if mefloquine is used after severe malaria. Approximately 1:20 patients given mefloquine after recovery from cerebral malaria will have an acute reaction and therefore mefloquine should not be used in this group.74 Neuropsychiatric reactions are also more common if mefloquine has been used in the previous 2 months, and therefore mefloquine should not be used to treat recrudescent infections occurring within 2 months of treatment. However in practice the principle adverse effect of mefloquine is vomiting. This is more likely in young children, and even if the drug is administered again, low blood levels and an increased risk of treatment failure result. Combining artesunate or artemether (4 mg/kg/day for 3 days) with mefloquine has all the advantages of a combination treatment previously described,75 and the additional benefit that if mefloquine is split as 8.3mg/kg/day for 3 days or not given until the second day of treatment then absorption is increased and gastrointestinal adverse effects are lessened.71,76 A fixed combination of mefloquine and artesunate has recently been developed. This is dispensed as tablets containing 200 mg of artesunate and 400 mg mefloquine (base). Recent trials in Asia indicated that the tolerability of this new regimen (mefloquine dose 8 mg/kg/d for 3 days) is better than that of the standard regimen.76,77 This combination has been evaluated and used mainly in Southeast Asia and South America. More information on tolerability, safety, and efficacy is needed in African children so that its potential utility in Africa can be assessed objectively.
Artesunate–Chlorproguanil–Dapsone
Chlorproguanil–dapsone is an antifol-sulfonamide combination with a similar mode of action and synergistic properties to sulfadoxine–pyrimethamine. Chlorproguanil can be considered as a prodrug for the active antifol chlorcycloguanil to which it is metabolized by the polymorphic CYP450 2C19.78 Activity of this enzyme is reduced in approximately 20% of Orientals and is also reduced by estrogens (e.g., pregnancy, oral contraceptive). The advantages of this combination are good tolerability and rapid elimination79 providing less selective pressure on the spread of resistance and greater activity than SP against moderately resistant Plasmodium falciparum (although both compounds are ineffective against P. falciparum with the Ile164Leu mutation common in Asia and South America, and recently identified in Africa). Disadvantages are resistance selected already by SP, some concerns over the safety of dapsone (hemolytic anemia), and lack of a post-treatment prophylactic effect. Whether this rapidly eliminated drug will be sufficiently effective for patients with high parasite burdens in a 3-day regimen remains to be seen. The ACT is in the late stage development and should be registered in the near future.80
Artesunate–Atovaquone–Proguanil
This fixed dose combination of 2 established drugs has not been developed as a 3-drug fixed dose ACT although it has been evaluated and found to be well tolerated, safe, and effective.81 Atovaquone–proguanil has a different (and synergistic) mode of action to other antimalarials affecting parasite respiration at the level of the cytochrome chain. Proguanil is acting itself in the combination, and not via the antifol triazine metabolite cycloguanil—so it remains effective against antifol-resistant parasites. Mutations in the gene encoding cytochrome b confer high level atovaquone resistance. Atovaquone absorption (like that of lumefantrine and halofantrine) is augmented by co-administration with fats. Its elimination half-life of 1–2 days provides for an effective 3- day treatment regimen. As with many antimalarials, plasma concentrations of both drugs are reduced in pregnancy.82 It is remarkably well tolerated with no serious adverse effects. The main impediment to its use is the high cost of atovaquone manufacture. The drug is essentially unaffordable in malaria endemic areas.
Artesunate–Pyronaridine
Pyronaridine is one of many synthetic antimalarials developed in China and used originally as a monotherapy. It bears closest structural similarity to amodiaquine, although pyronaridine is much more active against resistant parasites. Pyronaridine’s pharmacokinetic properties have not been fully characterized yet but like several other drugs in this general class of antimalarials, it is extensively distributed and eliminated slowly. The mechanism of action of the drug and mechanisms of potential resistance have not been well characterized but are likely to be related to those of the other drugs in this general class. Pyronaridine and the ACT combination are well tolerated and effective. The fixed dose ACT is in late-stage development.
Dihydroartemisinin–Piperaquine
Piperaquine is a bisquinoline compound related to chloroquine and other 4-aminoquinolines. It was discovered in France and developed as an antimalarial by Chinese scientists over 30 years ago.83 Piperaquine replaced chloroquine as the first-line treatment of falciparum malaria in China in 1978. After over 200 metric tons were used, including in mass treatment, resistance to piperaquine developed in P. falciparum in the late 1980s. Piperaquine was not used outside of China. The mechanism of action of the drug and mechanism of resistance have not been well characterized but are likely to be related to those of the other drugs in this general class. Piperaquine has a large apparent volume of distribution of > 500 L/kg and a terminal elimination half-life estimated at 2 to 3 weeks.84 With increasing sensitivity of the assay, the true terminal half-life is probably similar to that of chloroquine; 1–2 months.85 Oral bioavailability increases with co-administration with fat.86 The fixed dose combination formulated in tablets containing dihydroartemisinin (40 mg) and piperaquine (320 mg) is commercially available in many countries in Asia, and also more recently in Africa. Recent clinical trials have shown that the fixed combination given once daily for 3 days was effective and well tolerated.87–93 The most common adverse effects are gastrointestinal (nausea, vomiting, abdominal pain, and diarrhea), but they are usually mild and self limiting. The main determinant of the parasitological efficacy is the slow elimination of piperaquine. This also determines the “post-treatment prophylactic effect,” which is important if the drug is going to be used as Intermittent Preventive Treatment (IPT).18 The simplicity of administration, the excellent efficacy even against multi-drug–resistant strains and the favorable toxicity profile, make the DHA-piperaquine combination one of the more promising of the currently available ACTs.
ACTs in Children
The ACTs seem to be tolerated as well or better in children than in adults. There is no specific age-related toxicity. In younger children vomiting or regurgitation of the administered dose are always a concern but are no more common with ACTs than monotherapies. Recent pharmacokinetic studies indicate that the dose regimen advocated for SP in children for many years is probably too low.53 There are insufficient pharmacokinetic data on amodiaquine94 and more data on the pharmacokinetics of piperaquine in children are needed. Dose regimens for artesunate–mefloquine and artemether–lumefantrine in children are justified by pharmacokinetic studies. Further work is needed to optimize dosing in children based on weight or surface area and, where necessary, to introduce specific pediatric formulations.
ACTs in Pregnancy
Pregnant women are a high-risk group. They are more susceptible to malaria, more likely to develop anemia, and if non-immune are more likely to develop complications. Birth-weight is consistently reduced by malaria. Therefore, pregnant women desperately need effective and safe antimalarial treatments. The main concern surrounding the general deployment of ACTs is their safety in the first trimester of pregnancy.49–51 Work by Chinese scientists in rodents and rabbits conducted in the 1970s indicated that early pregnancy exposure could induce fetal resorption. Recent reproductive toxicity studies have confirmed that this is a class effect of the compounds and is seen in all experimental animal species studied. It results from a specific inhibition of fetal erythropoeisis. Fetal resorption would result in early pregnancy loss. Much more worrying is the potential to cause developmental abnormality. In rodents and rabbits at doses close to human therapeutic doses, artemisinins given in a critical window in the early stage of gestation, may also cause limb deformation. In primates, doses of 12–30 mg/kg daily given continuously between day 20 and day 50 pc (equivalent to 20–56 days in human pregnancy) cause fetal resorption and slight (4–7%) long bone shortening, but no abnormalities. No effects were observed at 4 mg/kg.95
Given these uncertainties the artemisinin containing drugs are not indicated for the management of uncomplicated malaria in the first trimester of pregnancy unless there are no effective alternatives. But it is important to emphasise that artesunate should be used in severe malaria where it is clearly superior in terms of life-saving efficacy to quinine. In the second and third trimesters there is increasing evidence of safety for these drugs, with no evidence of adverse effects in over 1,000 prospectively followed pregnancies.96,97 Questions on dosage remain as concentrations of artesunate, artemether, and dihydroartemisinin, and several of the partner drugs are reduced in late pregnancy compared with non-pregnant adults.67,98,99 In the second and third trimesters, recommendations are determined more by the evidence for the partner drug. However, there is a grave paucity of data on the safety, the efficacy, and the pharmacokinetic properties of the other antimalarials in pregnancy. For example there are no published pharmacokinetic data on amodiaquine, or sulfadoxine–pyrimethamine despite their extensive deployment. A recent review on the use of antimalarials in pregnancy also emphasizes the lack of data on the safety of some drugs that have been used for decades.97 On the other hand, drugs that are considered safe and for which pharmacokinetic data are available to support current dosing recommendations are either ineffective (e.g., chloroquine) or there are safety concerns (e.g., mefloquine, quinine). Mefloquine was associated with an increased risk of stillbirths in Thailand but not in Malawi.72,73 Quinine is associated with a high risk of hypoglycemia in late pregnancy. Clearly more information on the other drugs is needed urgently. The 2006 edition of the WHO Malaria Treatment Guidelines1 recommends that ACTs should be used in the second and third trimesters of pregnancy. These recommendations were the result of careful examination of the evidence available at the time and will be reviewed and modified by new evidence. It is generally accepted that a drug should first be shown to be safe and effective in treatment before it can be introduced in an IPT strategy.
Plasmodium Vivax
The artemisinin derivatives and the ACTs work as well or better against Plasmodium vivax compared with Plasmodium falciparum infections.100 The exception to this is artesunate–SP, which is ineffective in those areas with high-level antifol resistance in P. vivax. These drugs do not affect the hypnozoites, so relapses are not prevented. The slowly eliminated drugs (e.g., chloroquine, mefloquine, piperaquine) suppress the emergence of the first relapse in the frequently relapsing “tropical strains” of P. vivax so the first successful emergence of a relapse (i.e., the second relapse) typically occurs about 6 weeks after treatment.
Effects on Transmission
All effective antimalarials prevent the development of gametocytes in P. vivax, P. malariae, and P. ovale infections and the early-stage gametocytes (stages 1 to 3) of P. falciparum. The artemisinin derivatives inhibit development of more mature P. falciparum gametocytes.12,101 Gametocytemia is greater in recrudescent than primary infections. In low-transmission settings this gametocytocidal effect and the high cure rates obtained with ACTs both contribute to reducing transmission, and thus the incidence of malaria.
Conclusions
The ACTs are now accepted as the best treatments for uncomplicated falciparum malaria, and policy change has taken place in most countries to make these the first-line recommended drugs. The evidence base for efficacy and safety has grown considerably in recent years as these have become the most studied of all antimalarial drugs. Together with improved diagnosis and appropriate vector control measures, the ACTs should have a significant effect in reducing the burden of malaria throughout the tropical world, but to achieve this they will need to become more affordable and more available. This would be best achieved by subsidizing their cost both in the public and the private sectors.23 As deployment of ACTs increases, we will need to invest more in education, health service delivery, monitoring of rare adverse effects, and assessment of resistance to optimize their use, and thereby ensure they have the greatest impact on malaria.
Acknowledgments: FN and NW are both supported by the Wellcome Trust
References
- 1.
- World Health Organisation. Guidelines for the Treatment of Malaria. WHO; Geneva: 2006.
- 2.
- Stepniewska K, Taylor WRJ, Mayxay M, Price R, Smithuis F, Guthmann J-P, Barnes K, Myint H, Adjuik M, Olliaro P, Pukrittayakamee S, Looareesuwan S, Hien TT, Farrar J, Nosten F, Day NPJ, White NJ. The in vivo assessment of antimalarial drug efficacy in falciparum malaria; the duration of follow-up. Antimicrob Agents Chemother. 2004;48:4271–4280. [PMC free article: PMC525402] [PubMed: 15504852]
- 3.
- Peters W. Drug resistance—a perspective. Trans R Soc Trop Med Hyg. 1969;63:25–45. [PubMed: 4892498]
- 4.
- Curtis CF, Otoo LN. A simple model of the build-up of resistance to mixtures of antimalarial drugs. Trans R Soc Trop Med Hyg. 1986;80:889–892. [PubMed: 3603638]
- 5.
- Chawira AN, Warhurst DC, Robinson BL, Peters W. The effect of combinations of qinghaosu (artemisinin) with standard antimalarial drugs in the suppressive treatment of malaria in mice. Trans R Soc Trop Med Hyg. 1987;8:554–558. [PubMed: 3328341]
- 6.
- White NJ. Assessment of the pharmacodynamic properties of the antimalarial drugs in-vivo. Antimicrob Agents Chemother. 1997;41:1413–1422. [PMC free article: PMC163932] [PubMed: 9210658]
- 7.
- White NJ. Antimalarial drug resistance and combination chemotherapy. Philos Trans R Soc Lond B Biol Sci. 1999;354:739–749. [PMC free article: PMC1692562] [PubMed: 10365399]
- 8.
- White NJ. Antimalarial drug resistance. J Clin Invest. 2004;113:1084–1092. [PMC free article: PMC385418] [PubMed: 15085184]
- 9.
- Cowman AF, Galatis D, Thompson JK. Selection for mefloquine resistance in Plasmodium falciparum is linked to amplification of the pfmdr1 gene and cross-resistance to halofantrine and quinine. Proc Natl Acad Sci USA. 1994;91:1143–1147. [PMC free article: PMC521470] [PubMed: 8302844]
- 10.
- Uhlemann AC, McGready R, Ashley EA, Brockman A, Singhasivanon P, Krishna S, White NJ, Nosten F, Price RN. Intrahost selection of Plasmodium falciparum pfmdr1 Alleles after antimalarial treatment on the northwestern border of Thailand. J Infect Dis. 2007;195:134–141. [PMC free article: PMC4337981] [PubMed: 17152017]
- 11.
- Jambou R, Legrand E, Niang M, Khim N, Lim P, Volney B, Ekala MT, Bouchier C, Esterre P, Fandeur T, Mercereau-Puijalon O. Resistance of Plasmodium falciparum field isolates to in-vitro artemether and point mutations of the SERCA-type PfATPase6. Lancet. 2005;366:1960–1963. [PubMed: 16325698]
- 12.
- Price RN, Nosten F, Luxemburger C, ter Kuile F, Paiphun L, Chongsuphajaisiddhi T, White NJ. The effects of artemisinin derivatives on malaria transmissability. Lancet. 1996;347:1654–1658. [PubMed: 8642959]
- 13.
- Barnes KI, Durrheim DN, Little F, Jackson A, Mehta U, Allen E, Dlamini SS, Tsoka J, Bredenkamp B, Mthembu DJ, White NJ, Sharp BL. Effect of artemether-lumefantrine policy and improved vector control on malaria burden in KwaZulu-Natal, South Africa. PLoS Med. 2005;2:e330. [PMC free article: PMC1240068] [PubMed: 16187798]
- 14.
- Carrara VI, Sirilak S, Thonglairuam J, Rojanawatsirivet C, Proux S, Gilbos V, Brockman A, Ashley EA, McGready R, Krudsood S, Leemingsawat S, Looareesuwan S, Singhasivanon P, White N, Nosten F. Deployment of early diagnosis and mefloquine-artesunate treatment of falciparum malaria in Thailand: the Tak Malaria Initiative. PLoS Med. 2006;3:e183. [PMC free article: PMC1470664] [PubMed: 16719547]
- 15.
- Watkins WM, Mosobo M. Treatment of Plasmodium falciparum malaria with pyrimethamine-sulphadoxine: selective pressure for resistance is a function of long elimination half-life. Trans R Soc Trop Med Hyg. 1993;87:75–78. [PubMed: 8465404]
- 16.
- Hastings I, Watkins WM, White NJ. Pharmacokinetic parameters affecting the evolution of drug-resistance in malaria; The role of the terminal elimination half-life. Philos Trans R Soc Lond B Biol Sci. 2002;357:505–519. [PMC free article: PMC1692966] [PubMed: 12028788]
- 17.
- Nzila AM, Nduati E, Mberu EK, Hopkins Sibley C, Monks SA, Winstanley PA, Watkins WM. Molecular evidence of greater selective pressure for drug resistance exerted by the long-acting antifolate Pyrimethamine/Sulfadoxine compared with the shorter-acting chlorproguanil/dapsone on Kenyan Plasmodium falciparum. J Infect Dis. 2000;181:2023–2028. [PubMed: 10837185]
- 18.
- White NJ. Intermittent presumptive treatment for malaria. A better understanding of the pharmacodynamics will guide more rational policymaking. PLoS Medicine. 2005;2:29–33. [PMC free article: PMC545196] [PubMed: 15696210]
- 19.
- McIntosh HM, Olliaro P. Artemisinin derivatives for treating uncomplicated malaria. Cochrane Database Syst Rev. 2000;(2):CD000256. [PMC free article: PMC6532741] [PubMed: 10796519]
- 20.
- Price RN, van Vugt M, Nosten F, Luxemburger C, Brockman A, Phaipun L, Chongsuphajaisiddhi T, White NJ. Artesunate versus artemether for the treatment of recrudescent multidrug-resistant falciparum malaria. Am J Trop Med Hyg. 1998;59:883–888. [PubMed: 9886194]
- 21.
- Nosten F, van Vugt M, Price R, Luxemburger C, Brockman A, McGready R, ter Kuile F, Looareesuwan S, White NJ. Effects of artesunate-mefloquine combination on incidence of Plasmodium falciparum malaria and mefloquine resistance in western Thailand; a prospective study. Lancet. 2000;356:297–302. [PubMed: 11071185]
- 22.
- Brockman A, Price RN, van Vugt M, Heppner DG, Walsh D, Sookto P, Wimonwattrawatee T, Looareesuwan S, White NJ, Nosten F. Plasmodium falciparum antimalarial drug susceptibility on the northwestern border of Thailand during five years of extensive artesunate-mefloquine use. Trans R Soc Trop Med Hyg. 2000;94:537–544. [PMC free article: PMC4340572] [PubMed: 11132385]
- 23.
- Institute of Medicine. Saving Lives, Buying Time; the Economics of Malaria Drugs in an Age of Resistance. National Academies Press; Washington DC: 2004. [PubMed: 25009879]
- 24.
- Newton P, Suputtamongkol Y, Teja-Isavadharm P, Pukrittayakamee S, Navaratnam V, Bates I, White NJ. Antimalarial bioavailability and disposition of artesunate in acute falciparum malaria. Antimicrob Agents Chemother. 2000;44:972–977. [PMC free article: PMC89800] [PubMed: 10722499]
- 25.
- Suputtamongkol Y, Newton P, Angus B, Teja-Isavadharm P, Keeratithakul D, Rasameesoraj M, Pukrittayakamee S, White NJ. A comparison of oral artesunate and artemether antimalarial bioactivities in acute falciparum malaria. Br J Clin Pharmacol. 2001;52:655–661. [PMC free article: PMC2014567] [PubMed: 11736876]
- 26.
- Newton PN, van Vugt M, Teja-Isavadharm P, Siriyanonda D, Rasameesoroj M, Teerapong P, Ruangveerayuth R, Slight T, Nosten F, Suputtamongkol Y, Looareesuwan S, White NJ. Comparison of oral artesunate and dihydroartemisinin antimalarial bioavailabilities in acute falciparum malaria. Antimicrob Agents Chemother. 2002;46:1125–1127. [PMC free article: PMC127101] [PubMed: 11897605]
- 27.
- Na-Bangchang K, Krudsood S, Silachamroon U, Molunto P, Tasanor O, Chalermrut K, Tangpukdee N, Matangkasombut O, Kano S, Looareesuwan S. The pharmacokinetics of oral dihydroartemisinin and artesunate in healthy Thai volunteers. Southeast Asian J Trop Med Public Health. 2004;35:575–582. [PubMed: 15689069]
- 28.
- Teja-Isavadharm P, Watt G, Eamsila C, Jongsakul K, Li Q, Keeratithakul G, Sirisopana N, Luesutthiviboon L, Brewer TG, Kyle DE. Comparative pharmacokinetics and effect kinetics of orally administered artesunate in healthy volunteers and patients with uncomplicated falciparum malaria. Am J Trop Med Hyg. 2001;65:717–721. [PubMed: 11791963]
- 29.
- Binh TQ, Ilett KF, Batty KT, Davis TM, Hung NC, Powell SM, Thu LT, Thien HV, Phuong HL, Phuong VD. Oral bio-availability of dihydroartemisinin in Vietnamese volunteers and in patients with falciparum malaria. Br J Clin Pharmacol. 2001;51:541–546. [PMC free article: PMC2014487] [PubMed: 11422013]
- 30.
- Kongthaisong M, Na-Bangchang K, Mungthin M, Sinchaipanid N, Tan-Ariya P. Comparison of the bioequivalence of three oral formulations of dihydroartemisinin based on ex vivo blood schizontocidal activities against Plasmodium falciparum. Am J Trop Med Hyg. 2004;71:703–710. [PubMed: 15642958]
- 31.
- Li XQ, Bjorkman A, Andersson TB, Gustafsson LL, Masimirembwa CM. Identification of human cytochrome P(450)s that metabolise anti-parasitic drugs and predictions of in vivo drug hepatic clearance from in vitro data. Eur J Clin Pharmacol. 2003;59:429–442. [PubMed: 12920490]
- 32.
- Angus BJ, Thaiaporn I, Chanthapadith K, Suputtamongkol Y, White NJ. Oral artesunate dose response relationship in acute falciparum malaria. Antimicrob Agents Chemother. 2002;46:778–782. [PMC free article: PMC127461] [PubMed: 11850261]
- 33.
- Chanda P, Hawela M, Kango M, Sipilanyambe N. Assessment of the therapeutic efficacy of a paediatric formulation of artemether-lumefantrine (Coartesiane) for the treatment of uncomplicated Plasmodium falciparum in children in Zambia. Malar J. 2006;5:75. [PMC free article: PMC1574335] [PubMed: 16938133]
- 34.
- Taylor WR, White NJ. Antimalarial drug toxicity: a review. Drug Saf. 2004;27:25–61. [PubMed: 14720085]
- 35.
- Leonardi-Nield E, Gilvary G, White NJ, Nosten F. Severe allergic reactions to oral artesunate: a report of two cases. Trans R Soc Trop Med Hyg. 2001;95:182–183. [PubMed: 11355556]
- 36.
- Brewer TG, Grate SJ, Peggins JO, Weina PJ, Petras JM, Levine BS, Heiffer MH, Schuster BG. Fatal neurotoxicity of arteether and artemether. Am J Trop Med Hyg. 1994;51:251–259. [PubMed: 7943542]
- 37.
- Brewer TG, Peggins JO, Grate SJ, Petras JM, Levine BS, Weina PJ, Swearengen J, Heiffer MH, Schuster BG. Neurotoxicity in animals due to arteether and artemether. Trans R Soc Trop Med Hyg. 1994;88(Suppl 1):S33–S36. [PubMed: 8053022]
- 38.
- Petras JM, Kyle DE, Gettatacamin M, Young GD, Bauman RA, Webster HK, Corcoran KD, Peggins JO, Vane MA, Brewer TG. Arteether: risks of two week administration in Macaca mulatta. Am J Trop Med Hyg. 1997;56:390–396. [PubMed: 9158046]
- 39.
- Nontprasert A, Nosten-Bertrand M, Pukrittayakamee S, Vanijanonta S, Angus BJ, White NJ. Assessment of the neurotoxicity of parenteral artemisinin derivatives in mice. Am J Trop Med Hyg. 1998;59:519–522. [PubMed: 9790421]
- 40.
- Nontprasert A, Pukrittayakamee S, Nosten-Bertrand M, Vanijanonta S, White NJ. Studies of the neurotoxicity of oral artemisinin derivatives in mice. Am J Trop Med Hyg. 2000;62:409–412. [PubMed: 11037787]
- 41.
- Genovese RF, Newman DB, Brewer TG. Behavioral and neural toxicity of the artemisinin antimalarial, arteether, but not artesunate and artelinate, in rats. Pharmacol Biochem Behav. 2000;67:37–44. [PubMed: 11113482]
- 42.
- Toovey S, Jamieson A. Audiometric changes associated with the treatment of uncomplicated falciparum malaria with co-artemether. Trans R Soc Trop Med Hyg. 2004;98:261–267. [PubMed: 15109547]
- 43.
- Mehta U, Barnes KI, Kathard H, Vugt M, Durrheim D. Comment on: Audiometric changes associated with the treatment of uncomplicated falciparum malaria with co-artemether. Trans R Soc Trop Med Hyg. 2005;99:313–314. [PubMed: 15708390]
- 44.
- Price RN, van Vugt M, Phaipun L, Luxemburger C, Simpson J, McGready R, ter Kuile F, Kham A, Chongsuphajaisiddhi T, White NJ, Nosten F. Adverse effects in patients with acute falciparum malaria treated with artemisinin derivatives. Am J Trop Med Hyg. 1999;60:547–555. [PubMed: 10348227]
- 45.
- van Vugt M, Angus BM, Price RN, Mann C, Simpson JA, Poletto C, Htoo SE, Looareesuwan S, White NJ, Nosten F. A case-control auditory evaluation of patients treated with artemisinin derivatives for multi-drug resistant Plasmodium falciparum malaria. Am J Trop Med Hyg. 2000;62:65–69. [PubMed: 10761725]
- 46.
- Kissinger E, Hien TT, Hung NT, Nam ND, Tuyen NL, Dinh BV, Mann C, Phu NH, Loc PP, Simpson JA, White NJ, Farrar JJ. Clinical and neurophysiological study of the effects of multiple doses of artemisinin on brain-stem function in Vietnamese patients. Am J Trop Med Hyg. 2000;63:48–55. [PubMed: 11357994]
- 47.
- Hutagalung R, Htoo H, Nwee P, Arunkamomkiri J, Zwang J, Carrara VI, Ashley E, Singhasivanon P, White NJ, Nosten F. A case-control auditory evaluation of patients treated with artemether-lumefantrine. Am J Trop Med Hyg. 2006;74:211–214. [PubMed: 16474072]
- 48.
- Hien TT, Turner GDH, Mai NTH, Phu NH, Bethell D, Blakemore W, Cavanagh JC, Dayan A, Medana I, Weller RO, Day NPJ, White NJ. Neuropathological assessment of artemether treated severe malaria. Lancet. 2003;362:295–296. [PubMed: 12892962]
- 49.
- Longo M, Zanoncelli S, Torre PD, Riflettuto M, Cocco F, Pesenti M, Giusti A, Colombo P, Brughera M, Mazue G, Navaratman V, Gomes M, Olliaro P. In vivo and in vitro investigations of the effects of the antimalarial drug dihydroar-temisinin (DHA) on rat embryos. Reprod Toxicol. 2006;22:797–810. [PubMed: 16959470]
- 50.
- White TE, Bushdid PB, Ritter S, Laffan SB, Clark RL. Artesunate-induced depletion of embryonic erythroblasts precedes embryolethality and teratogenicity in vivo. Birth Defects Res B Dev Reprod Toxicol. 2006;77:413–429. [PubMed: 17066416]
- 51.
- Clark RL, White TE, A Clode S, Gaunt I, Winstanley P, Ward SA. Developmental toxicity of artesunate and an arte-sunate combination in the rat and rabbit. Birth Defects Res B Dev Reprod Toxicol. 2004;71:380–394. [PubMed: 15617018]
- 52.
- Adjuik M, Babiker A, Garner P, Olliaro P, Taylor W, White N. International Artemisinin Study Group. Artesunate combinations for treatment of malaria: meta-analysis. Lancet. 2004;363:9–17. [PubMed: 14723987]
- 53.
- Barnes KI, Little F, Smith PJ, Evans A, Watkins WM, White NJ. Sulfadoxine-pyrimethamine pharmacokinetics in malaria: pediatric dosing implications. Clin Pharmacol Ther. 2006;80:582–596. [PubMed: 17178260]
- 54.
- von Seidlein L, Milligan P, Pinder M, Bojang K, Anyalebechi C, Gosling R, Coleman R, Ude JI, Sadiq A, Duraisingh M, Warhurst D, Alloueche A, Targett G, McAdam K, Greenwood B, Walraven G, Olliaro P, Doherty T. Efficacy of artesunate plus pyrimethamine-sulphadoxine for uncomplicated malaria in Gambian children: a double-blind, randomised, controlled trial. Lancet. 2000;355:352–357. [PubMed: 10665554]
- 55.
- Adjuik M, Agnamey P, Babiker A, Borrmann S, Brasseur P, Cisse M, Cobelens F, Diallo S, Faucher JF, Garner P, Gikunda S, Kremsner PG, Krishna S, Lell B, Loolpapit M, Matsiegui PB, Missinou MA, Mwanza J, Ntoumi F, Olliaro P, Osimbo P, Rezbach P, Some E, Taylor WR. Amodiaquine-artesunate versus amodiaquine for uncomplicated Plasmodium falciparum malaria in African children: a randomised, multi-centre trial. Lancet. 2002;359:1365–1372. [PubMed: 11978332]
- 56.
- Martensson A, Stromberg J, Sisowath C, Msellem MI, Gil JP, Montgomery SM, Olliaro P, Ali AS, Bjorkman A. Efficacy of artesunate plus amodiaquine versus that of artemether-lumefantrine for the treatment of uncomplicated childhood Plasmodium falciparum malaria in Zanzibar, Tanzania. Clin Infect Dis. 2005;41:1079–1086. [PubMed: 16163624]
- 57.
- Durrani N, Leslie T, Rahim S, Graham K, Ahmad F, Rowland M. Efficacy of combination therapy with artesunate plus amodiaquine compared to monotherapy with chloroquine, amodiaquine or sulfadoxine-pyrimethamine for treatment of uncomplicated Plasmodium falciparum in Afghanistan. Trop Med Int Health. 2005;10:521–529. [PubMed: 15941414]
- 58.
- White NJ, van Vugt M, Ezzet F. Clinical pharmacokinetics and pharmacodynamics of artemether-lumefantrine. Clin Pharmacokinet. 1999;37:105–125. [PubMed: 10496300]
- 59.
- van Vugt M, Wilairatana P, Gemperli B, Gathmann I, Phaipun L, Brockman A, Luxemburger C, White NJ, Nosten F, Looareesuwan S. Efficacy of six doses or artemether-benflumetol in the treatment of multi-drug resistant falciparum malaria. Am J Trop Med Hyg. 1999;60:936–942. [PubMed: 10403324]
- 60.
- van Vugt M, Brockman A, Gemperli B, Luxemburger C, Gathman I, Royce C, Slight T, Looareesuwan S, White NJ, Nosten F. Randomised comparison of artemether-benflumetol and artesunate-mefloquine in the treatment of multi-drug resistant falciparum malaria. Antimicrob Agents Chemother. 1998;42:135–139. [PMC free article: PMC105468] [PubMed: 9449273]
- 61.
- Stohrer JM, Dittrich S, Thongpaseuth V, Vanisaveth V, Phetsouvanh R, Phompida S, Monti F, Christophel EM, Lindegardh N, Annerberg A, Jelinek T. Therapeutic efficacy of artemether-lumefantrine and artesunate-mefloquine for treatment of uncomplicated Plasmodium falciparum malaria in Luang Namtha Province, Lao People’s Democratic Republic. Trop Med Int Health. 2004;9:1175–1183. [PubMed: 15548313]
- 62.
- Hutagalung R, Paiphun L, Ashley EA, McGready R, Brockman A, Thwai KL, Singhasivanon P, Jelinek T, White NJ, Nosten FH. A randomized trial of artemether-lumefantrine versus mefloquine-artesunate for the treatment of uncomplicated multi-drug resistant Plasmodium falciparum on the western border of Thailand. Malar J. 2005;4:46. [PMC free article: PMC1261533] [PubMed: 16179089]
- 63.
- Mueller EA, van Vugt M, Kirch W, Andriano K, Hunt P, de Palacios PI. Efficacy and safety of the six-dose regimen of artemether-lumefantrine for treatment of uncomplicated Plasmodium falciparum malaria in adolescents and adults: a pooled analysis of individual patient data from randomized clinical trials. Acta Trop. 2006;100:41–53. [PubMed: 17045558]
- 64.
- Price RN, Uhlemann AC, van Vugt M, Brockman A, Hutagalung R, Nair S, Nash D, Singhasivanon P, Anderson TJ, Krishna S, White NJ, Nosten F. Molecular and pharmacological determinants of the therapeutic response to artemether-lumefantrine in multidrug-resistant Plasmodium falciparum malaria. Clin Infect Dis. 2006;42:1570–1577. [PMC free article: PMC4337983] [PubMed: 16652314]
- 65.
- Ashley EA, Stepniewska K, Lindegårdh N, Annerberg A, Kham Am, Brockman A, Singhivason P, White NJ, Nosten F. How much fat is necessary to optimise lumefantrine oral bioavailability? Trop Med Int Health. 2007;12:195–200. [PubMed: 17300625]
- 66.
- Ashley EA, Stepniewska K, Lindegårdh N, McGready R, Annerberg A, Hutagalung R, Singtoroj T, Hla G, Brockman A, Proux S, Wilahphaingern J, Singhivason P, White NJ, Nosten F. A pharmacokinetic study of artemether-lumefantrine given once daily for the treatment of uncomplicated multidrug resistant falciparum malaria. Trop Med Int Health. 2007;12:201–208. [PubMed: 17300626]
- 67.
- McGready R, Stepniewska K, Lindegardh N, Ashley EA, La Y, Singhasivanon P, White NJ, Nosten F. The pharmacokinetics of artemether and lumefantrine in pregnant women with uncomplicated falciparum malaria. Eur J Clin Pharmacol. 2006;62:1021–1031. [PubMed: 17053895]
- 68.
- Palmer KJ, Holliday SM, Brogden RN. Mefloquine. A review of its antimalarial activity, pharmacokinetic properties and therapeutic efficacy. Drugs. 1993;45:430–475. [PubMed: 7682911]
- 69.
- Price RN, Cassar C, Brockman A, Duraisingh M, van Vugt M, White NJ, Nosten F, Krishna S. The pfmdr1 gene is associated with a multidrug resistance phenotype in Plasmodium falciparum from the western border of Thailand. Antimicrob Agents Chemother. 1999;43:2943–2949. [PMC free article: PMC89592] [PubMed: 10582887]
- 70.
- Simpson JA, Watkins ER, Price RN, Aarons L, Kyle DE, White NJ. Mefloquine pharmacokinetic-pharmacodynamic models: implications for dosing and resistance. Antimicrob Agents Chemother. 2000;44:3414–3424. [PMC free article: PMC90214] [PubMed: 11083649]
- 71.
- Simpson JA, Price RN, ter Kuile FO, Teja-Isvadharm P, Nosten F, Aarons L, White NJ. Population pharmacokinetics of mefloquine in patients with acute falciparum malaria. Clin Pharmac Ther. 1999;66:472–484. [PubMed: 10579474]
- 72.
- Nosten F, Vincenti M, Simpson JA, Yei P, Kyaw Lay Thwai, de Vries A, Chongsuphajaisiddhi T, White NJ. The effects of mefloquine treatment in pregnancy. Clin Infect Dis. 1999;28:808–815. [PubMed: 10825043]
- 73.
- Steketee RW, Wirima JJ, Slutsker L, Khoromana CO, Heymann DL, Breman JG. Malaria treatment and prevention in pregnancy: indications for use and adverse events associated with use of chloroquine or mefloquine. Am J Trop Med Hyg. 1996;55(1 Suppl):50–56. [PubMed: 8702037]
- 74.
- Mai NTH, Day NPJ, Chuong LV, Waller D, Phu NH, Bethell DB, Hien TT, White NJ. Post-malaria neurological syndrome. Lancet. 1996;348:917–921. [PubMed: 8843810]
- 75.
- Nosten F, Luxemburger C, ter Kuile FO, Woodrow C, Pa Eh J, Chongsuphajaisiddhi T, White NJ. Treatment of multi-drug resistant Plasmodium falciparum malaria with 3-day arte-sunatemefloquine combination. J Infect Dis. 1994;170:971–977. [PubMed: 7930743]
- 76.
- Ashley EA, Stepniewska K, Lindegardh N, McGready R, Hutagalung R, Hae R, Singhasivanon P, White NJ, Nosten F. Population pharmacokinetic assessment of a new regimen of mefloquine used in combination treatment of uncomplicated falciparum malaria. Antimicrob Agents Chemother. 2006;50:2281–2285. [PMC free article: PMC1489761] [PubMed: 16801402]
- 77.
- Ashley EA, Lwin KM, McGready R, Simon WH, Phaiphun L, Proux S, Wangseang N, Taylor W, Stepniewska K, Nawamaneerat W, Thwai KL, Barends M, Leowattana W, Olliaro P, Singhasivanon P, White NJ, Nosten F. An open label randomized comparison of mefloquine-artesunate as separate tablets vs. a new co-formulated combination for the treatment of uncomplicated multidrug-resistant falciparum malaria in Thailand. Trop Med Int Health. 2006;11:1653–1660. [PubMed: 17054744]
- 78.
- Winstanley P, Ward S. Malaria chemotherapy. Adv Parasitol. 2006;61:47–76. [PubMed: 16735162]
- 79.
- Simpson JA, Hughes D, Manyando C, Bojang K, Aarons L, Winstanley P, Edwards G, Watkins WA, Ward S. Population pharmacokinetic and pharmacodynamic modelling of the antimalarial chemotherapy chlorproguanil/dapsone. Br J Clin Pharmacol. 2006;61:289–300. [PMC free article: PMC1885012] [PubMed: 16487222]
- 80.
- van Vugt M, Leonardi E, Phaipun L, Slight T, Thway KL, McGready R, Brockman A, Villegas L, Looareesuwan S, White NJ, Nosten F. Treatment of uncomplicated multidrug-resistant falciparum malaria with artesunate-atovaquone-proguanil. Clin Infect Dis. 2002;35:1498–1504. [PubMed: 12471569]
- 81.
- Davis TM, Karunajeewa HA, Ilett KF. Artemisinin-based combination therapies for uncomplicated malaria. Med J Aust. 2005;182:181–185. [PubMed: 15720175]
- 82.
- McGready R, Stepniewska K, Ward SA, Cho T, Gilveray G, Looareesuwan S, White NJ, Nosten F. Pharmacokinetics of dihydroartemisinin following oral artesunate treatment of pregnant women with acute uncomplicated falciparum malaria. Eur J Clin Pharmacol. 2006;62:367–371. [PubMed: 16552504]
- 83.
- Davis TM, Hung TY, Sim IK, Karunajeewa HA, Ilett KF. Piperaquine: a resurgent antimalarial drug. Drugs. 2005;65:75–87. [PubMed: 15610051]
- 84.
- Hung TY, Davis TM, Ilett KF, Karunajeewa H, Hewitt S, Denis MB, Lim C, Socheat D. Population pharmacokinetics of piperaquine in adults and children with uncomplicated falciparum or vivax malaria. Br J Clin Pharmacol. 2004;57:253–262. [PMC free article: PMC1884452] [PubMed: 14998421]
- 85.
- Tarning J, Lindegardh N, Annerberg A, Singtoroj T, Day NP, Ashton M, White NJ. Pitfalls in estimating piperaquine elimination. Antimicrob Agents Chemother. 2005;49:5127–5128. [PMC free article: PMC1315981] [PubMed: 16304183]
- 86.
- Sim IK, Davis TM, Ilett KF. Effects of a high-fat meal on the relative oral bioavailability of piperaquine. Antimicrob Agents Chemother. 2005;49:2407–2411. [PMC free article: PMC1140540] [PubMed: 15917540]
- 87.
- Denis MB, Davis TM, Hewitt S, Incardona S, Nimol K, Fandeur T, Poravuth Y, Lim C, Socheat D. Efficacy and safety of dihydroartemisinin-piperaquine (Artekin) in Cambodian children and adults with uncomplicated falciparum malaria. Clin Infect Dis. 2002;35:1469–1476. [PubMed: 12471565]
- 88.
- Hien TT, Mai PP, Dolecek C, Phuong P, Dung NT, Truong NT, Thanh N, Thai LH, An DTH, Quyen NTH, White NJ, Farrar JJ. Dihydroartemisinin-piperaquine against multidrug resistant falciparum malaria in Viet Nam: randomized clinical trial. Lancet. 2004;363:18–22. [PubMed: 14723988]
- 89.
- Ashley EA, Krudsood S, Phaiphun L, Srivilairit S, McGready R, Leowattana W, Hutagalung R, Wilairatana P, Brockman A, Looareesuwan S, Nosten F, White NJ. Randomized, controlled dose-optimization studies of dihydroartemisinin-piperaquine for the treatment of uncomplicated multidrug-resistant falciparum malaria in Thailand. J Infect Dis. 2004;190:1773–1782. [PubMed: 15499533]
- 90.
- Karunajeewa H, Lim C, Hung TY, Ilett KF, Denis MB, Socheat D, Davis TM. Safety evaluation of fixed combination piperaquine plus dihydroartemisinin (Artekin) in Cambodian children and adults with malaria. Br J Clin Pharmacol. 2004;57:93–99. [PMC free article: PMC1884411] [PubMed: 14678346]
- 91.
- Mayxay M, Thongpraseuth V, Khanthavong M, Lindegardh N, Barends M, Keola S, Pongvongsa T, Phompida S, Phetsouvanh R, Stepniewska K, White NJ, Newton PN. An open, randomized comparison of artesunate plus mefloquine vs. dihydroartemisinin-piperaquine for the treatment of uncomplicated Plasmodium falciparum malaria in the Lao People’s Democratic Republic (Laos). Trop Med Int Health. 2006;11:1157–1165. [PubMed: 16903879]
- 92.
- Smithuis F, Kyaw MK, Phe O, Aye KZ, Htet L, Barends M, Lindegardh N, Singtoroj T, Ashley E, Lwin S, Stepniewska K, White NJ. Efficacy and effectiveness of dihydroartemisinin-piperaquine versus artesunate-mefloquine in falciparum malaria: an open-label randomised comparison. Lancet. 2006;367:2075–2085. [PubMed: 16798391]
- 93.
- Karema C, Fanello CI, van Overmeir C, van Geertruyden JP, van Doren W, Ngamije D, D’Alessandro U. Safety and efficacy of dihydroartemisinin/piperaquine (Artekin) for the treatment of uncomplicated Plasmodium falciparum malaria in Rwandan children. Trans R Soc Trop Med Hyg. 2006;100:1105–1111. [PubMed: 16766006]
- 94.
- Hombhanje FW, Hwaihwanje I, Tsukahara T, Saruwatari J, Nakagawa M, Osawa H, Paniu MM, Takahashi N, Lum JK, Aumora B, Masta A, Sapuri M, Kobayakawa T, Kaneko A, Ishizaki T. The disposition of oral amodiaquine in Papua New Guinean children with falciparum malaria. Br J Clin Pharmacol. 2005;59:298–301. [PMC free article: PMC1884785] [PubMed: 15752375]
- 95.
- Clark RL, Kumemura M, Makori M, Nkata Y, Bernrad F, White TEK, Arima A. Teratology Society abstracts. 2006. Artesunate developmental toxicity in monkeys; p. 30.
- 96.
- McGready R, Cho T, Keo NK, Villegas L, Looareesuwan S, White NJ, Nosten F. Artemisinin antimalarials in pregnancy: a prospective treatment study of 539 multidrug resistant P.falciparum episodes. Clin Infect Dis. 2001;33:2009–2010. [PubMed: 11712093]
- 97.
- Nosten F, McGready R, Mutabingwa T. Case management of malaria in pregnancy. Lancet Infect Dis. 2007;7:118–125. [PubMed: 17251082]
- 98.
- McGready R, Stepniewska K, Edstein MD, Cho T, Gilveray G, Looareesuwan S, White NJ, Nosten F. The pharmacokinetics of atovaquone and proguanil in pregnant women with acute falciparum malaria. Eur J Clin Pharm. 2003;59:545–552. [PubMed: 12955371]
- 99.
- McGready R, Stepniewska K, Ward SA, Cho T, Gilveray G, Looareesuwan S, White NJ, Nosten F. Pharmacokinetics of dihydroartemisinin following oral artesunate treatment of pregnant women with acute uncomplicated falciparum malaria. Eur J Clin Pharmacol. 2006;62:367–371. [PubMed: 16552504]
- 100.
- Pukrittayakamee S, Chantra A, Simpson JA, Vanijanonta S, Clemens R, Looareesuwan S, White NJ. Therapeutic responses to different antimalarial drugs in vivax malaria. Antimicrob Agents Chemother. 2000;44:1680–1685. [PMC free article: PMC89932] [PubMed: 10817728]
- 101.
- Targett G, Drakeley C, Jawara M, von Seidlein L, Coleman R, Deen J, Pinder M, Doherty T, Sutherland C, Walraven G, Milligan P. Artesunate reduces but does not prevent posttreatment transmission of Plasmodium falciparum to Anopheles gambiae. J Infect Dis. 2001;183:1254–1259. [PubMed: 11262208]
Authors’ addresses: Nicholas White, Faculty of Tropical Medicine, Mahidol University, 420/6 Rajvithi Rd., Bangkok 10400, Thailand, Tel: (66) 2 354 9172, Fax: (66) 2 354 9169, E-mail: ca.serdemport@wkcin. François Nosten, Centre for Tropical Medicine and Vaccinology, Churchill Hospital, Oxford, UK, E-mail: ca.serdemport@URMS
- Introduction
- Rationale
- Deployment
- Access
- Pharmacology
- Adverse Effects
- The ACTs
- Artesunate–Sulfadoxine–Pyrimethamine
- Artesunate–Amodiaquine
- Artemether–Lumefantrine
- Artesunate–Mefloquine
- Artesunate–Chlorproguanil–Dapsone
- Artesunate–Atovaquone–Proguanil
- Artesunate–Pyronaridine
- Dihydroartemisinin–Piperaquine
- ACTs in Children
- ACTs in Pregnancy
- Plasmodium Vivax
- Effects on Transmission
- Conclusions
- References
- Artemisinin-Based Combination Treatment of Falciparum Malaria - Defining and Def...Artemisinin-Based Combination Treatment of Falciparum Malaria - Defining and Defeating the Intolerable Burden of Malaria III: Progress and Perspectives
- Malaria Burden among Pregnant Women Living in the Rural District of Boromo, Burk...Malaria Burden among Pregnant Women Living in the Rural District of Boromo, Burkina Faso - Defining and Defeating the Intolerable Burden of Malaria III: Progress and Perspectives
- Drosophilidae NADH dehydrogenase subunit 5 (ND5) gene, partial cds; mitochondria...Drosophilidae NADH dehydrogenase subunit 5 (ND5) gene, partial cds; mitochondrial.PopSet: 168481220PopSet
Your browsing activity is empty.
Activity recording is turned off.
See more...