NCBI Bookshelf. A service of the National Library of Medicine, National Institutes of Health.
IARC Working Group on the Evaluation of Carcinogenic Risks to Humans. Some Chemicals that Cause Tumours of the Kidney or Urinary Bladder in Rodents and Some Other Substances. Lyon (FR): International Agency for Research on Cancer; 1999. (IARC Monographs on the Evaluation of Carcinogenic Risks to Humans, No. 73.)
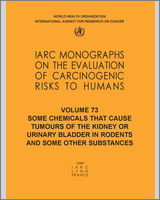
Some Chemicals that Cause Tumours of the Kidney or Urinary Bladder in Rodents and Some Other Substances.
Show detailsThis substance was considered by a previous working group, in 1992 (IARC, 1993). Since that time, new data have become available, and these have been incorporated into the monograph and taken into consideration in the present evaluation.
1. Exposure Data
1.1. Chemical and physical data
1.1.1. Nomenclature
- Chem. Abstr. Serv. Reg. No.: 5989-27-5
- Chem. Abstr. Name: (R)-1-Methyl-4-(1-methylethenyl)cyclohexene
- IUPAC Systematic Name: (R)-(+)-para-Mentha-1,8-diene
- Synonyms: (+)-Dipentene; (R)-4-isopropenyl-1-methyl-1-cyclohexene; d-limonene; d-(+)-limonene; d-(+)-limonene; (+)-limonene; (+)-α-limonene; (+)-(R)-limonene; (+)-(4R)-limonene; (R)-limonene; (R)-(+)-limonene; (4R)-(+)-limonene; (+)-para-mentha-1,8-diene; (R)-p-mentha-1,8-diene; (R)-(+)-para-mentha-1,8-diene
1.1.3. Chemical and physical properties of the pure substance
- (a) Description: Colourless liquid with characteristic citrus odour (National Toxicology Program, 1991)
- (b) Boiling-point: 175.5–176°C (Budavari, 1996)
- (c) Melting-point: −96.9°C (National Toxicology Program, 1991); −74.3°C has also been reported (Lide, 1991)
- (d) Density: 0.8411 g/cm3 at 20°C (National Toxicology Program, 1991)
- (e) Solubility: Slightly soluble in water (13.8 mg/mL at 25°C); soluble in acetone, dimethyl sulfoxide and ethanol (National Toxicology Program, 1991; National Library of Medicine, 1998)
- (f) Volatility: Vapour pressure, 133 Pa at 14°C and 665 Pa at 40.4°C; relative vapour density (air = 1), 4.69 (National Toxicology Program, 1991)
- (g) Conversion factor: mg/m3 = 5.57 × ppm
1.2. Production and use
Information available in 1995 indicated that d-limonene was produced in Australia, Brazil, Germany, Japan and the United States (Chemical Information Services, 1995).
d-Limonene has been used for many years as a flavour and fragrance additive in foods, beverages and consumer products. It is increasingly used as a solvent. It is also used in the manufacture of resins, as a wetting and dispersing agent and in insect control (National Toxicology Program, 1991; IARC, 1993; Budavari, 1996).
d-Limonene and its metabolite perillyl alcohol are currently undergoing clinical trials for use in treatment of breast cancer and other tumours, and chemoprevention trials are under consideration (Gould, 1995; O’Shaugnessy, 1996; Vigushin et al., 1998).
1.3. Occurrence
1.3.1. Natural occurrence
d-Limonene is one of the most common terpenes in nature, occurring in citrus and a wide variety of other plant species. It is a major constituent of oil of citrus rind, dill oil, oil of cumin, neroli, bergamot and caraway (National Toxicology Program, 1991) [see IARC (1993) for a detailed discussion of its occurrence].
1.3.2. Occupational exposure
According to the 1981–83 National Occupational Exposure Survey (National Institute for Occupational Safety and Health, 1998), approximately 138 300 workers in the United States were potentially exposed to d-limonene. Occupational exposure to d-limonene may occur during its production and use, notably as an industrial solvent.
1.3.3. Environmental occurrence
The average daily dietary intake of d-limonene has been estimated to be about 0.3 mg/kg bw (Flavor and Extract Manufacturers’ Association, 1991).
d-Limonene has been detected in indoor and outdoor air in various locations (IARC, 1993; National Library of Medicine, 1998).
1.4. Regulations and guidelines
The 8-h time-weighted exposure limit for d-limonene in Sweden is 150 mg/m3 and the short-term (15 min) exposure limit is 300 mg/m3 (National Board of Occupational Safety and Health, 1996).
No international guidelines for d-limonene in drinking-water have been established (WHO, 1993).
2. Studies of Cancer in Humans
No data were available to the Working Group.
3. Studies of Cancer in Experimental Animals
Previous evaluation
d-Limonene was tested for carcinogenicity by oral gavage in one study in mice and one study in rats. In mice and female rats, there were no treatment-related tumours, but male rats had renal tubular hyperplasia and a significantly increased combined incidence of renal tubular adenomas and carcinomas. In a two-stage experiment, oral treatment with d-limonene after administration of N-nitrosoethylhydroxyethylamine enhanced the development of renal tubular hyperplasia and renal tubular adenomas in male Fischer rats, which synthesize α2u -globulin in the liver in large quantities, but not in male NBR rats, which do not (IARC, 1993). [The present Working Group reconfirmed the adequacy of the bioassays of d-limonene in male and female mice and rats conducted by the National Toxicology Program as cited in IARC (1993), noting the convincing renal tumour response in male rats and the absence of renal tumours in female rats.]
New studies
3.1. Administration with known carcinogens
Mouse: In a model of lung carcinogenesis, groups of 25 female A/J mice, six to seven weeks of age, were fed d-limonene in the diet at a concentration of 0.63% for 17 weeks. One week after commencement of treatment, the mice received an intraperitoneal injection of 10 µmol 4-(methylnitrosamino)-1-(3 pyridyl)-1-butanone (NNK) in 0.1 mL saline. d-Limonene treatment significantly reduced the number of lung tumours per mouse, from 8.1 in mice given NNK alone to 2.4 (p < 0.05), although the percentage of mice with lung tumours was not significantly different between those given NNK (100%) and those given d-limonene (96%) (El-Bayoumy et al., 1996).
Rat: In a model of putative colonic preneoplasia, groups of 12 male Fischer 344 rats, five weeks of age, were given 0.5% d-limonene in the drinking-water (equivalent to 0.67% in the diet) for five weeks. One week after the start of treatment, the rats received subcutaneous injections of 15 mg/kg bw azoxymethane once a week for three weeks and were killed at 10 weeks of age. In rats given azoxymethane and d-limonene, the frequencies of aberrant crypt foci and the numbers of aberrant crypts per focus and of aberrant crypts per colon were significantly decreased (p < 0.01 to p < 0.001) when compared with the group given azoxymethane alone (Kawamori et al., 1996).
In a multi-organ model of carcinogenesis, groups of 20 Fischer 344 male rats aged five weeks were treated sequentially with N-nitrosodiethylamine (100 mg/kg bw by intraperitoneal injection as a single dose at the beginning of the study), N-methyl-N-nitrosourea (20 mg/kg bw by intraperitoneal injection four times during weeks 1 and 2), N-nitrosobutyl-N-(4-hydroxybutyl)amine (0.05% in drinking-water during weeks 1 and 2), 1,2-dimethylhydrazine (40 mg/kg bw by subcutaneous injection four times during weeks 3 and 4) and N-nitrosodihydroxydipropylamine (0.1% in drinking-water during weeks 3 and 4). From the end of week 4, d-limonene at doses of 0.5, 1 or 2% was administered in the diet for 24 weeks, when surviving rats were sacrificed (i.e. 28 weeks after the start of the study). A group receiving 2% d-limonene but no initiating carcinogen schedule was also included. The number of rats with renal tubular adenomas was statistically significantly increased (p < 0.01) at the high dose of d-limonene: 13/19 with the carcinogen schedule plus 2% d-limonene and 4/19 with the carcinogen schedule alone. This increase in renal tumour incidence was accompanied by an increased number of atypical tubules (a preneoplastic lesion) per rat. None of the rats fed 2% d-limonene alone had a renal tumour. No modification of carcinogenesis was observed in any other organ (Kimura et al., 1996).
Hamster: In a model of pancreatic carcinogenesis, groups of 25 Syrian golden hamsters, five weeks of age, were given subcutaneous injections of 15 mg/kg bw N-nitrosobis(2-oxopropyl)amine in 0.9% saline once a week for five weeks and simultaneously fed chow pellets containing 0, 1 or 2% d-limonene for up to 26 weeks, when the animals were killed. The high dose of d-limonene significantly decreased the number of pancreatic carcinomas, from 0.74 ± 0.15 in the animals given the carcinogen alone to 0.25 ± 0.12 in the animals given carcinogen plus 2% d-limonene (p < 0.05) (Nakaizumi et al., 1997).
4. Other Data Relevant to an Evaluation of Carcinogenicity and its Mechanisms
4.1. Absorption, distribution, metabolism and excretion
4.1.1. Humans
d-Limonene is absorbed from the gastrointestinal tract. Two male volunteers given 1.6 g [14C]d-limonene orally excreted 52–83% of the dose in their urine within 48 h. The major urinary metabolite isolated was 8-hydroxy-para-menth-1-en-9-yl-β-d-glucopyranosiduronic acid (Kodama et al., 1976).
The toxicokinetics of d-limonene were studied in volunteers exposed by inhalation for 2 h to 10, 225 or 450 mg/m3 d-limonene and to a workload of 50 W to simulate light physical activity; 10 mg/m3 was considered to be the control concentration. The relative pulmonary uptake was approximately 70% of the amount supplied. Three linear phases of elimination could be distinguished in the time studied: a terminal phase of slope γ for slow elimination (320–1300 min after exposure), an intermediate phase of slope β for rapid elimination (16–319 min after exposure) and an initial phase of slope α (0–15 min after exposure). The plasma half-life of d-limonene was approximately 2.6 min for the α phase, 32 min for the β phase and 75 min for the γ phase. The lung clearance rate in 4 h was 1.1 L/kg per h for 225 mg/m3 and 1.4 L/kg per h for 450 mg/m3, and the lung clearance rate in 21 h was 1.1 L/kg per h for 450 mg/m3 (Falk-Filipsson et al., 1993).
A pilot study was conducted in healthy volunteers (five women, two men) to investigate the metabolism and the toxicity of pharmacologically (supradietary) administered d-limonene. After the subjects had ingested 100 mg/kg d-limonene in a custard, their blood was drawn at 0 and 24 h for blood chemistry and at 0, 4 and 24 h for analysis of metabolites. Gas chromatography–mass spectrometry indicated the presence of five d-limonene metabolites in plasma: two major peaks were identified as dihydroperillic acid and perillic acid and a third major peak was limonene-1,2-diol; limonene itself was only a minor component. Two minor peaks were found to be the respective methyl esters of the acids. In all subjects, the metabolite concentrations were higher at 4 h than at 24 h, but a half-life value was not determined (Crowell et al., 1994).
The toxicokinetics of d-limonene were studied in two women with breast cancer and one man with colorectal cancer. The patients received 0.5–12 g/m2 body surface area per day orally for 21 days, and plasma and urine samples were collected on days 1 and 21. The metabolites were characterized and their structures elucidated by liquid chromatography–mass spectrometry and nuclear magnetic resonance spectrometry. Five major metabolites were detected in plasma: limonene-1,2-diol, limonene-8,9-diol, perillic acid, an isomer of perillic acid and dihydroperillic acid. The urinary metabolites comprised the glucuronides of the two isomers of perrilic acid, limonene-8,9-diol and a monohydroxylated limonene. The results are consistent with those of previously published studies in humans and in animals, but this study was the first in which limonene-8,9-diol and an additional isomer of perillic acid were identified (Poon et al., 1996).
In a phase I clinical trial of orally administered d-limonene, 17 women and 15 men aged 35–78 (median, 57), with advanced metastatic solid tumours received an average of three treatment cycles of 21 days (one dose on day 1, then three daily doses on days 4–21) at doses ranging from 0.5 to 12 g/m2 body surface area. d-Limonene was slowly absorbed, the maximal plasma concentration being attained at 1–6 h. The mean peak plasma concentrations of d-limonene were 11–20 µmol/L, and the predominant metabolites were perillic acid (21–71 µmol/L), dihydroperillic acid (17–28 µmol/L), limonene-1,2-diol (10–21 µmol/L), uroterpinol (14–45 µmol/L) and an isomer of perillic acid. After reaching these peaks, the plasma concentrations decreased according to first-order kinetics. The values for the integrated area under the curve for time–concentration showed little variation with administered dose. There was no accumulation of the parent or metabolites after a treatment cycle (Vigushin et al., 1998).
4.1.2. Experimental systems
[14C]d-Limonene was absorbed rapidly after administration by gavage of 800 mg/kg bw (4.15 µCi/animal) to male Wistar rats. The radiolabel concentration in blood was maximal after 2 h, and large amounts of radiolabel were also observed in the liver (maximal after 1 h) and kidneys (maximal after 2 h). Negligible concentrations were found in blood and organs after 48 h (Igimi et al., 1974).
Urinary recovery of [14C]d-limonene was 77–96% within three days in rats, guinea-pigs, hamsters and dogs. Faecal recovery was 2–9% within three days (Kodama et al., 1976). Bile-duct-cannulated rats given d-limonene orally excreted 25% of the dose in the bile within 24 h (Igimi et al., 1974).
After oral administration of d-limonene to rabbits, the urinary metabolites isolated were para-mentha-1,8-dien-10-ol (M-I on Figure 1), para-menth-1-ene-8,9-diol (M-II), perillic acid (M-III), perillic acid-8,9-diol (M-IV), para-mentha-1,8-dien-10-yl-β-d-glucopyranosiduronic acid (M-V) and 8-hydroxy-para-menth-1-en-9-yl-β-d-glucopyranosiduronic acid (M-VI) (Kodama et al., 1974). After oral administration of d-limonene to dogs and rats, five other urinary metabolites were isolated: 2-hydroxy-para-menth-8-en-7-oic acid (M-VII), perillylglycine (M-VIII), perillyl-β-d-glucopyranosiduronic acid (MIX), para-mentha-1,8-dien-6-ol (M-X) and probably para-menth-1-ene-6,8,9-triol (M-XI). The major urinary metabolite was M-IV in rats and rabbits, M-IX in Syrian hamsters, M-II in dogs and M-VI in guinea-pigs (Kodama et al., 1976). The possible metabolic pathways of d-limonene are shown in Figure 1.

Figure 1
Possible metabolic pathways of d-limonene
Under alkaline extraction conditions, d-limonene was metabolized by rat liver microsomes in vitro to the glycols d-limonene 8,9-diol and d-limonene 1,2-diol via the 8,9- and 1,2-epoxides (Watabe et al., 1980, 1981). Under neutral extraction conditions, no hydrolysis of d-limonene-1,2-epoxide to its corresponding diol was observed (Lehman-McKeeman et al., 1989). In rat liver microsomes, expoxidation of d-limonene at the 1,2 double bond occurs only in the cis orientation, whereas in mouse liver microsomes both cis and trans isomers of this epoxide are formed (Lehman-McKeeman & Caudill, 1992a).
When [14C]d-limonene was administered orally to male and female Sprague-Dawley rats at a dose of 409 mg/kg bw, the renal concentration of d-limonene equivalents was about 2.5 times higher in males than females, and approximately 40% of the radiolabel in male rat kidneys was bound reversibly to renal proteins. The major metabolite bound to the renal protein fraction was identified as d-limonene-1,2-epoxide (> 80%), parent d-limonene and the 1,2-diol representing minor components of the protein-bound moieties. The renal protein to which these metabolites bound was identified as α2u-globulin by high-performance liquid chromatography (Lehman-McKeeman et al., 1989).
4.2. Toxic effects
4.2.1. Humans
Five healthy adult male volunteers who received a single oral dose of 20 g d-limonene all developed transient proteinuria, non-bloody diarrhoea and tenesmus. The results of functional tests of the liver, kidney and pancreas were normal (Igimi et al., 1976).
In the study of volunteers treated by inhalation, described in section 4.1.1, no irritating or central nervous system-related symptoms were found. A 2% decrease in lung vital capacity was found after exposure to 450 mg/m3 when compared with 10 mg/m3; this difference was statistically but not clinically significant (Falk-Filipsson et al., 1993).
In the phase-I clinical trial of orally administered d-limonene described in section 4.1.1, toxicity was limited to gastrointestinal symptoms (irritation, nausea, diarrhoea) and was dose-related over the range 6.5–12 g/m2 body surface area per day (Vigushin et al., 1998).
4.2.2. Experimental systems
The LD50 values for d-limonene in male and female mice were reported to be 5.6 and 6.6 (oral), 1.3 and 1.3 (intraperitoneal) and > 42 and > 42 (subcutaneous) g/kg bw, respectively; those in male and female rats were reported to be 4.4 and 5.1 (oral), 3.6 and 4.5 (intraperitoneal), > 20 and > 20 (subcutaneous) and 0.12 and 0.11 (intravenous) g/kg bw, respectively (Tsuji et al., 1975a). The acute oral LD50 in rats and the acute dermal LD50 in rabbits were reported to exceed 5 g/kg bw (Opdyke, 1975).
After daily administration of d-limonene at 277–2770 mg/kg bw to male and female Sprague-Dawley rats for one month, the highest dose was found to have caused a slight decrease in body weight and food consumption. On histological examination (staining with hematoxylin and eosin), granular casts were observed in the kidney of males, but no significant change was found in the other organs (Tsuji et al., 1975a).
A dose-related increase in relative liver and kidney weights was observed in young adult male Fischer 344 rats given 75, 150 or 300 mg/kg bw d-limonene daily by gavage on five days per week and killed on study days 6 or 27. With Mallory-Heidenhain staining, a dose-related formation of hyaline droplets was observed histologically in the kidneys. Hyaline droplet nephropathy was associated with increased concentrations of α2u-globulin in renal cortical homogenates separated by two-dimensional gel electrophoresis. The concentrations of other renal proteins were not increased by d-limonene treatment. Alterations considered to be sequelae of the hyaline droplet response, including granular casts in the outer zone of the medulla and multiple cortical changes collectively classified as chronic nephrosis, were observed in the kidneys of all rats killed on day 27 (Kanerva et al., 1987).
Oral administration of 75 or 150 mg d-limonene to male Fischer 344/N rats on five days per week for two years was associated with dose-related alterations to the kidney, such as increased incidences of mineralization and epithelial hyperplasia and increased severity of chronic progressive nephropathy. In the same study, no signs of toxicity, including renal hyaline droplet formation, were observed in female Fischer 344/N rats dosed at 300 or 600 mg/kg bw (National Toxicology Program, 1990).
Male and female B6C3F1 mice were treated orally on five days a week for two years with doses of 250 or 500 and 500 or 1000 mg/kg bw, respectively. The mean body weights of females at the high dose were 5–15% lower than those of controls after week 28, but no compound-related toxicity was observed in animals of either sex (National Toxicology Progam, 1990).
In beagle dogs, oral doses of more than 340 mg/kg bw (bitches) and 1000 mg/kg bw (dogs) per day for six months resulted in protein casts in the renal tubules. Daily doses of more than 1000 mg/kg bw (bitches) and 3024 mg/kg bw (dogs) resulted in slight weight loss due to frequent vomiting in some animals (Tsuji et al., 1975b).
In another study in adult beagle dogs, d-limonene at 100 or 1000 mg/kg bw (maximal tolerated dose for emesis) per day by gavage twice daily for six months increased kidney weights but induced no histopathological changes, hyaline droplet accumulation or nephropathy (Webb et al., 1990).
d-Limonene given orally for four days at 1650 mg/kg bw per day caused no renal toxicity in male NCI Black Reiter rats, which do not synthesize the α2u-globulin that is normally present in hyaline droplets found in male Fischer 344 rats with d-limonene-induced nephrotoxicity (Dietrich & Swenberg, 1991a).
The ability of d-limonene to cause hyaline droplet nephropathy was evaluated in C57BL/6-derived transgenic mice engineered to express α2u-globulin. These mice excreted approximately 30% less α2u-globulin than male rats. α2u-Globulin was detected in the kidney by immunoblotting; after d-limonene treatment at 150 mg/kg bw for three days, the concentration of α2u-globulin was increased threefold relative to untreated controls. Spontaneous hyaline droplet formation was not seen in control transgenic mice, but small droplets were observed after d-limonene treatment (Lehman-McKeeman & Caudill, 1994).
d-Limonene at 150 mg/kg bw increased renal-cell proliferation in male Fischer 344 rats, particularly in the P2 segment of the renal proximal tubular epithelium, after 4 or 31 weeks of exposure. Cell proliferation, determined by bromodeoxyuridine labelling, was increased approximately fivefold over that in control rats. No increase in renal-cell proliferation was observed in male NBR rats treated similarly in the same experiment (Dietrich & Swenberg, 1991b).
d-Limonene increased renal-cell proliferation in response to hyaline droplet exacerbation in Fischer 344 rats dosed orally for 91 days at 0, 5, 30, 75 or 150 mg/kg bw. No exacerbation of hyaline droplets was noted at the lowest dose, and there was no increase in proliferating cell nuclear antigen-labelled renal proximal tubular cells. At doses of 30 mg/kg bw d-limonene and higher, both hyaline droplet formation and the percentage of labelled cells were increased. At the highest dose, the percentage of antigen-labelled cells was increased by about six times over that in controls, and the cells were localized to the P2 segment of the proximal tubule (Lehman-McKeeman, 1995).
mRNA for α2u-globulin was markedly reduced or was undectable in the livers of male rats [strain not specified] fed diets containing the peroxisome proliferator and lipid-lowering agent ciprofibrate (0.025% wt/wt) for seven weeks. This finding was confirmed by immunoblot analysis with antibodies against α2u-globulin, although immunohistochemical staining and in-situ hybridization showed the presence of a few cells that contained α2u-globulin protein and its m-RNA. The α2u-globulin m-RNA reappeared in the liver two weeks after cessation of ciprofibrate treatment. Feeding of ciprofibrate for two weeks, followed by simultaneous feeding of ciprofibrate and d-limonene (150 mg/kg bw per day, six days a week), showed that ciprofibrate prevented α2u-globulin accumulation and the nephrotoxicity associated with binding of d-limonene to this protein (Alvares et al., 1996).
4.3. Reproductive and developmental effects
4.3.1. Humans
No data were available to the Working Group.
4.3.2. Experimental systems
Studies in mice, rats and rabbits exposed by oral administration during organogenesis showed impaired weight gains in the dams and a transient reduction in the growth of the offspring. Anomalies of the ribs were observed in offspring of mice and rats (IARC, 1993).
It was reported in an abstract that d-limonene was not teratogenic to frog embryos (Xenopus laevis) although a teratogenic effect was reported at the lowest concentration (0.00114 mg/L) (Holck et al., 1991).
4.4. Genetic and related effects
4.4.1. Humans
No data were available to the Working Group.
4.4.2. Experimental systems (see Table 1 for references)
Table 1
Genetic and related effects of d-limonene and its metabolites.
d-Limonene was not mutagenic to Salmonella typhimurium. In single studies, it did not induce sister chromatid exchange, chromosomal aberrations, trifluorothymidine resistance or transformation of rodent cells in vitro. d-Limonene gave negative results in the mammalian spot test even at toxic doses.
d-Limonene has been found to inhibit gap-junctional intercellular communication in mouse primary keratinocytes and derived cell lines.
The metabolite d-limonene-1,2-oxide gave negative results in the SOS chromotest. It was not mutagenic to S. typhimurium and did not induce unscheduled DNA synthesis in rat hepatocytes in vitro. Essential oils containing d-limonene did not induce differential toxicity in Bacillus subtilis, nor did they induce reverse mutation in S. typhimurium.
d-Limonene did not inhibit the transformation of rat tracheal epithelial cells by benzo[a]pyrene (Steele et al., 1990).
4.5. Mechanistic considerations
4.5.1. Renal tumours in male rats
The criteria for establishing that an agent causes renal tumours in male rats through a response associated with α2u-globulin (Capen et al., 1999) are as follows:
- lack of genotoxic activity (the agent and/or a metabolite) on the basis of an overall evaluation of results obtained in vitro and in vivo;
- nephropathy and renal tumorigenicity seen only in male rats;
- induction in shorter studies of the characteristic sequence of histopathological changes, of which protein droplet accumulation is obligatory;
- identification of the protein that accumulates in tubular cells as α2u-globulin;
- reversible binding of the chemical or metabolite to α2u-globulin;
- induction of sustained increased cell proliferation in the renal cortex; and
- similarities in dose–response relationship of the tumour outcome with those for histopathological end-points (protein droplets, α2u-globulin accumulation, cell proliferation).
These criteria are met by d-limonene, which produces hyaline droplet nephropathy and causes renal tubular tumours in male rats through an α2u-globulin-associated response.
d-Limonene causes a renal syndrome that occurs exclusively in male rats. Male rats are unique in that they exhibit a background of spontaneous protein droplets in the proximal tubule, particularly within the cells of the P2 segment. d-Limonene exacerbates the formation of these droplets, and it was shown by two-dimensional gel electrophoresis that the only protein accumulating was α2u-globulin (Alden et al., 1984; Kanerva et al., 1987).
α2u-Globulin is synthesized in the liver, secreted into the general circulation and reabsorbed by renal proximal tubule cells. Hepatic synthesis of α2u-globulin occurs only in adult male rats (Roy et al., 1966). The critical role of α2u-globulin in the renal effects of d-limonene is demonstrated by the absence of histopathological changes in female rats and in species that do not synthesize α2u-globulin. Thus, d-limonene shows no renal toxicity in female rats, in male NBR rats or in other species studied, including male and female mice and dogs (Chatterjee et al., 1989; Dietrich & Swenberg, 1991a). d-Limonene also has no renal toxicity in ciprofibrate-treated male rats in which synthesis of α2u-globulin is repressed (Alvares et al., 1996). Whereas mice do not develop hyaline droplet nephropathy, transgenic mice that synthesize α2u-globulin develop these lesions (Lehman-McKeeman & Caudill, 1994).
The mechanism by which d-limonene causes α2u-globulin accumulation in the male rat kidney has been elucidated. The prerequisite step in the development of the nephropathy is the binding to α2u-globulin of an agent, which in the case of d-limonene is the 1,2-epoxide (Lehman-McKeeman et al., 1989). This binding is specific for α2u-globulin and reversible, with a binding affinity (Kd) of approximately 5.6 × 10−7 mol/L (Borghoff et al., 1991; Lehman-McKeeman & Caudill, 1992a). Binding of this ligand to α2u-globulin reduces the rate of its lysosomal degradation relative to that of native protein, thereby causing it to accumulate. Lysosomal cathepsin activity towards other protein substrates is not altered (Lehman-McKeeman et al., 1990).
Whereas accumulation of α2u-globulin can be observed after a single oral dose of d-limonene, continued treatment results in additional histological changes in the kidney. Phagolysosomes become enlarged, engorged with protein and show polyangular crystalloid inclusions. After three to four weeks of dosing, progressive renal injury, characterized by single-cell degeneration and necrosis in the P2 segment of the renal proximal tubule, is noted. Dead cells are sloughed into the lumen of the nephron, contributing to the development of granular casts at the cortico-medullary junction (Swenberg & Lehman-McKeeman, 1999). Renal functional perturbations, including reduced uptake of organic anions, cations and amino acids and mild proteinuria resulting from a large increase in the amount of α2u-globulin excreted in urine, are observed. These functional changes occur only in male rats and only at doses that exacerbate the protein droplet formation (Swenberg & Lehman-McKeeman, 1999). In response to the cell death and functional changes, there is a compensatory increase in cell proliferation in the kidney, most notably in the P2 segment of the proximal tubules, the site of protein accumulation (Dietrich & Swenberg, 1991b; Swenberg & Lehman-McKeeman, 1999). With continued treatment, the cell proliferation persists, but it does not restore renal function. The increase in cell proliferation is linked to the development of renal tubular tumours (Dietrich & Swenberg, 1991b). α2u-Globulin nephropathy and renal-cell proliferation occur at doses consistent with those that produce renal tubular tumours.
The link between α2u-globulin nephropathy and renal-cell proliferation was established for d-limonene in comparative studies of male NBR and Fischer 344 rats. Cell proliferation in renal tubules was increased by d-limonene in Fischer 344 rats after 4 or 31 weeks of exposure to d-limonene but not in NBR rats which do not develop α2u-globulin nephropathy. Thus, the increase in cell proliferation was shown to be totally dependent on the presence of α2u-globulin. Furthermore, when evaluated in an initiation–promotion study with N-nitrosoethyl-N-hydroxyethylamine, d-limonene treatment increased the incidence of renal adenomas in Fischer 344 rats, whereas no renal tumours occurred in NBR rats (Dietrich & Swenberg, 1991b).
The available data indicate that renal tubular tumours in male rats develop by a secondary, non-DNA reactive mechanism. Histopathological, biochemical and cell proliferation investigations provide compelling evidence that d-limonene produces a syndrome that begins acutely as accumulation of α2u-globulin but represents a continuum of changes that progress ultimately to renal tubular tumours (Swenberg et al., 1989; Flamm & Lehman-McKeeman, 1991; Hard et al., 1993; Hard & Whysner, 1994; Swenberg & Lehman-McKeeman, 1999).
Relevance to humans
The requisite step in the development of α2u-globulin nephropathy is binding of d-limonene, and particularly the 1,2-epoxide, to α2u-globulin. α2u-Globulin is a member of a superfamily of proteins that bind and transport a variety of ligands. Many of these proteins are synthesized in mammalian species, including humans. Therefore, the question of whether a similar mechanism occurs in humans can be addressed by determining whether these structurally homologous proteins function in humans in a manner analogous to α2u-globulin. This question has been answered both qualitatively and quantitatively.
The protein content of human urine is very different from that of rat urine, as humans excrete very little protein (about 1% of the concentration found in urine of male rats). Human urinary protein is also predominantly a species of high molecular mass, and there is no protein in human plasma or urine identical to α2u-globulin (Olson et al., 1990). No α2u-globulin-like protein has been detected in human kidney tissue (Borghoff & Lagarde, 1993). Saturable binding of d-limonene-1,2-epoxide to α2u-globulin can be shown in vitro, but other superfamily proteins, particularly those synthesized by humans, do not bind this agent (Lehman-McKeeman & Caudill, 1992b).
The unique specificity of the syndrome of renal toxicity in male rats due to α2u-globulin is demonstrated by the lack of toxicity and of renal tumours in mice. Mice synthesize mouse urinary protein, which shares nearly 90% sequence identity to α2u-globulin; however, d-limonene-1,2-epoxide does not bind to the mouse protein and it does not produce a similar syndrome in mice. Additionally, the lack of a response in female rats, which synthesize many other proteins of the superfamily, demonstrates that these proteins are unlikely to contribute to renal toxicity.
The X-ray crystal structure of α2u-globulin has been derived and indicates that although α2u-globulin may share amino acid sequence with many other proteins it has a unique ligand-binding pocket. Other superfamily proteins are characterized by flattened, elongated binding pockets, whereas the ligand-binding site in α2u-globulin is distinguished by a spherical, non-restrictive shape. The elongated, flattened binding pockets in mouse urinary protein and in superfamily proteins synthesized by humans preclude the binding of d-limonene-1,2-epoxide to these proteins (Lehman-McKeeman, 1997).
From a quantitative perspective, adult male rat kidneys reabsorb about 35 mg α2u-globulin per day. Female rats synthesize less than 1% of the amount of α2u-globulin reabsorbed by male rats, but no α2u-globulin is detected in female rat kidney and female rats do not develop nephropathy. The most abundant α2u-globulin superfamily protein in human kidney and plasma is α1-acid glycoprotein, and this protein does not bind to agents that induce α2u-globulin nephropathy in rats.
Taken together, there is no evidence that any human protein can contribute to a renal syndrome similar to α2u-globulin nephropathy, and thus no evidence that d-limonene is carcinogenic in humans by a mechanism similar to α2u-globulin nephropathy.
The induction of renal-cell tumours in male rats by agents that act through an α2u-globulin-associated response is not predictive of carcinogenic hazard to humans (Capen et al., 1999). This conclusion is based on extensive evidence that the presence of α2u-globulin is an absolute requirement for the carcinogenic activity and that neither α2u-globulin nor any protein that can function like α2u-globulin is synthesized by humans (Swenberg & Lehman-McKeeman, 1999).
d-Limonene has no carcinogenic activity at any other site in male rats. Consequently, all of the mechanistic data support the conclusion that the renal tumours in male rats produced by d-limonene are not relevant to humans.
5. Summary of Data Reported and Evaluation
5.1. Exposure data
d-Limonene is a terpene which occurs naturally in citrus and a variety of other plants. Exposure occurs from its presence in foods and its use as a solvent. It is being evaluated in clinical trials for use as a cancer chemotherapeutic agent.
5.2. Human carcinogenicity data
No data were available to the Working Group.
5.3. Animal carcinogenicity data
d-Limonene was tested for carcinogenicity by oral gavage in mice and rats and in several two-stage experiments with multi-organ carcinogens. It significantly increased the incidence of renal tubular tumours (adenomas and carcinomas) and induced atypical renal tubular hyperplasia in male rats, which normally synthesize α2u-globulin in the liver, but not in female rats or in mice of either sex. It consistently enhanced the incidences of renal tubular tumours and atypical renal tubular hyperplasia initiated by carcinogens in two-stage carcinogenesis assays in male rats of a strain conventionally used in bioassays, but not in a strain that lacks hepatic synthesis of α2u-globulin.
d-Limonene was tested as a cancer-preventive agent in other experimental models with known carcinogens. It inhibited lung carcinogenesis in mice, preneoplastic stages of colon carcinogenesis in rats and pancreatic carcinogenesis in hamsters.
5.4. Other relevant data
d-Limonene is metabolized in humans and experimental animals to a variety of metabolites, including perillic acid and d-limonene-1,2-diol. d-Limonene causes a male rat-specific nephrotoxicity resulting from accumulation of the male rat-specific protein α2u-globulin. d-Limonene-1,2-epoxide binds reversibly to α2u-globulin. d-Limonene causes sustained cell proliferation in renal proximal tubular cells, and the dose–response relationships for tumour outcome, enhanced cell proliferation and other histopathological end-points typical of α2u-globulin nephropathy are similar. Female rats, male rats of strains that do not express this protein and other species are not susceptible to the nephrotoxic action of d-limonene.
Developmental toxicity in the form of delayed prenatal growth has been observed in mice, rats and rabbits exposed to d-limonene during gestation. Skeletal anomalies have also been observed in the fetuses of exposed mice and rabbits.
The few available data indicate that d-limonene and its 1,2-epoxide metabolite are not genotoxic.
5.5. Evaluation
There is inadequate evidence in humans for the carcinogenicity of d-limonene.
There is sufficient evidence in experimental animals for the carcinogenicity of d-limonene.
Overall evaluation
In making its overall evaluation of the carcinogenicity to humans of d-limonene, the Working Group concluded that d-limonene produces renal tubular tumours in male rats by a non-DNA-reactive mechanism, through an α2u-globulin-associated response. Therefore, the mechanism by which d-limonene increases the incidence of renal tubular tumours in male rats is not relevant to humans.
d-Limonene is not classifiable as to its carcinogenicity to humans (Group 3).
6. References
- Alden, C.L., Kanerva, R.L., Ridder, G. & Stone, L.C. (1984) The pathogenesis of the nephrotoxicity of volatile hydrocarbons in the male. In: Mehlman, M.A., Hemstreet, C.P., Thorpe, J.J. & Weaver, N.K., eds, Advances in Modern Environmental Toxicology, Vol. 7, Renal Effects of Petroleum Hydrocarbons, Princeton, NJ, Princeton Scientific Publishers, pp. 107–120.
- Alvares K., Subbarao V., Rao M.S., Reddy J.K. Ciprofibrate represses alpha(2u)-globulin expression in liver and inhibits d-limonene nephrotoxicity. Carcinogenesis. 1996;17:311–316. [PubMed: 8625456]
- American Industrial Hygiene Association (1993) Workplace Environmental Exposure Level Guides: d-Limonene, Fairfax, VA.
- Borghoff S.J., Lagarde W.H. Assessment of binding of 2,4,4-trimethyl-2-pentanol to low molecular weight proteins isolated from kidneys of male rats and humans. Toxicol. appl. Pharmacol. 1993;119:228–235. [PubMed: 7683150]
- Borghoff S.J., Miller A.B., Bowen J.P., Swenberg J.A. Characteristics of chemical binding to α2u-globulin in vitro—Evaluating structure activity relationships. Toxicol. appl. Pharmacol. 1991;107:228–238. [PubMed: 1704644]
- Budavari, S., ed. (1996) The Merck Index, 12th Ed., Whitehouse Station, NJ, Merck & Co., p. 938.
- Capen, C.C., Dybing, E., Rice, J.M. & Wilbourn, J.D., eds (1999) Species Differences in Thyroid, Kidney and Urinary Bladder Carcinogenesis (IARC Scientific Publications No. 147), Lyon, IARC.
- Chatterjee B., Demyan W.F., Spong C.S., Garg B.D., Roy A.K. Loss of androgenic induction of α2u-globulin gene family in the liver of NIH Black rats. Endocrinology. 1989;125:1385–1388. [PubMed: 2474439]
- Chemical Information Services (1995) Directory of World Chemical Producers 1995/96 Standard Edition, Dallas, TX, p. 452.
- Crowell P.L., Elson C.E., Bailey H.H., Elegbede A., Haag J.D., Gould M.N. Human metabolism of the experimental cancer therapeutic agent d-limonene. Cancer Chemother. Pharmacol. 1994;35:31–37. [PubMed: 7987974]
- Dietrich D.R., Swenberg J.A. NCI-Black-Reiter (NBR) male rats fail to develop renal disease following exposure to agents that induce α-2u-globulin nephropathy. Fundam. appl. Toxicol. 1991a;16:719–762. [PubMed: 1715830]
- Dietrich D.R., Swenberg J.A. The presence of α2u-globulin is necessary for d-limonene promotion of male rat kidney tumors. Cancer Res. 1991b;51:3512–3521. [PubMed: 1711412]
- El-Bayoumy K., Upadhyaya P., Desai D.H., Amin S., Hoffmann D., Wynder E. L. 1996 Effects of 1,4-phenylenebis(methylene)selenocyanate, phenethyl isothiocyanate, indole-3-carbinol, and d-limonene individually and in combination on the tumorigenicity of the tobacco-specific nitrosamine 4-(methylnitrosamino)-1-(3-pyridyl)-1-butanone in A/J mouse lung. Anticancer Res. 16, 2709–2712. [PubMed: 8917375]
- Fahrig R. Effects of food additives in the mammalian spot test. Prog. clin. biol. Res. 1982;109:334–348. [PubMed: 7167550]
- Falk-Filipsson A., Löf A., Hagberg M., Hjelm E.W., Wang Z. d-Limonene exposure to humans by inhalation: Uptake, distribution, elimination, and effects on the pulmonary function. J. Toxicol. environ. Health. 1993;38:77–88. [PubMed: 8421324]
- Flamm W.G., Lehman-McKeeman L.D. The human relevance of the renal tumor-inducing potential of d-limonene in male rats: Implications for risk assessment. Regul. Toxicol. Pharmacol. 1991;13:70–86. [PubMed: 2024047]
- Flavor and Extract Manufacturers’ Association (1991) d-Limonene Monograph, Washington DC, pp. 1–4.
- Gould M.N. Prevention and therapy of mammary cancer by monoterpenes. J. cell. Biochem. 1995;22 Suppl.:139–144. [PubMed: 8538191]
- Hard G.C., Whysner J. Risk assessment of d-limonene: An example of male rat-specific renal tumorigens. Crit. Rev. Toxicol. 1994;24:231–254. [PubMed: 7945892]
- Hard G.C., Rodgers L.S., Baetcke K.P., Richards W.L., McGaughy R.E., Valcovic L.R. Hazard evaluation of chemicals that cause α2u-globulin, hyaline droplet nephropathy, and tubule neoplasia in the kidneys of male rats. Environ. Health Perspectives. 1993;99:313–349. [PMC free article: PMC1567071] [PubMed: 7686485]
- Haworth S., Lawlor T., Mortelmans K., Speck W., Zeiger E. Salmonella mutagenicity test results for 250 chemials. Environ. Mutag. 1983;Suppl. 1:3–142. [PubMed: 6365529]
- Holck A.R., Estep J.E., Hemeyer R.D. Teratogenicity of d-limonene to Xenopus embryos (Abstract no. P20). J. Am. Coll. Toxicol. 1991;10:624.
- von der Hude W., Seelbach A., Basler A. Epoxides: Comparison of the induction of SOS repair in Escherichia coli PQ37 and the bacterial mutagenicity in the Ames test. Mutat. Res. 1990a;231:205–218. [PubMed: 2200956]
- von der Hude W., Mateblowski R., Basler A. Induction of DNA-repair synthesis in primary rat hepatocytes by epoxides. Mutat. Res. 1990b;245:145–150. [PubMed: 2233836]
- IARC (1993) IARC Monographs on the Evaluation of Carcinogenic Risks to Humans, Vol. 56, Some Naturally Occurring Substances: Food Items and Constituents, Heterocyclic Aromatic Amines and Mycotoxins, Lyon, pp. 135–162.
- Igimi H., Nishimura M., Kodama R., Ide H. Studies on the metabolism of d-limonene (p-mentha-1,8-diene). I. The absorption, distribution and excretion of d-limonene in rats. Xenobiotica. 1974;4:77–84. [PubMed: 4828799]
- Igimi H., Hisatsugu T., Nishimura M. The use of d-limonene preparation as a dissolving agent of gallstones. Dig. Dis. 1976;21:926–939. [PubMed: 790944]
- Jansen L.A.M., Jongen W.M.F. The use of initiated cells as a test system for the detection of inhibitors of gap junctional intercellular communication. Carcinogenesis. 1996;17:333–339. [PubMed: 8625460]
- Kanerva R.L., Ridder G.M., Lefever F.R., Alden C.L. Comparison of short-term renal effects due to oral administration of decalin or d-limonene in young adult male Fischer-344 rats. Food chem. Toxicol. 1987;25:345–353. [PubMed: 3609973]
- Kawamori T., Tanaka T., Hirose Y., Ohnishi M., Mori H. 1996 Inhibitory effects of d-limonene on the development of colonic aberrant crypt foci induced by azoxymethane in F344 rats. Carcinogenesis 17, 369–372. [PubMed: 8625465]
- Kimura J., Takahashi S., Ogiso T., Yoshida Y., Akagi K., Hasegawa R., Kurata M., Hirose M., Shirai T. 1996 Lack of chemoprevention effects of the monoterpene d-limonene in a rat multi-organ carcinogenesis model. Jpn. J. Cancer Res. 87, 589–594. [PMC free article: PMC5921134] [PubMed: 8766522]
- Kodama R., Noda K., Ide H. Studies on the metabolism of d-limonene (p-mentha-1,8-diene). II. The metabolic fate of d-limonene in rabbits. Xenobiotica. 1974;4:85–95.
- Kodama R., Yano T., Furukawa K., Noda K., Ide H. Studies on the metabolism of d-limonene (p-mentha-1,8-diene). IV. Isolation and characterization of new metabolites and species differences in metabolism. Xenobiotica. 1976;6:377–389. [PubMed: 969565]
- Lehman-McKeeman, L.D. (1997) α2u-Globulin nephropathy. In: Sipes, I.G., McQueen, C.A. & Gandolfi, A.J., eds, Comprehensive Toxicology, Vol. 7, Renal Toxicology, New York, Pergamon Press, pp. 677–692.
- Lehman-McKeeman L.D., Caudill D. Biochemical basis for mouse resistance to hyaline droplet nephropathy: Lack of relevance of the α2u-globulin protein superfamily in this male rat-specific syndrome. Toxicol. appl. Pharmacol. 1992a;112:214–221. [PubMed: 1371614]
- Lehman-McKeeman L.D., Caudill D. α2u-Globulin is the only member of the lipocalin protein superfamily that binds to hyaline droplet inducing agents. Toxicol. appl. Pharmacol. 1992b;116:170–176. [PubMed: 1384170]
- Lehman-McKeeman L.D., Caudill D. d-Limonene induced hyaline droplet nephropathy in alpha 2u-globulin transgenic mice. Fundam. appl. Toxicol. 1994;23:562–568. [PubMed: 7532604]
- Lehman-McKeeman L.D., Rodriguez P.A., Takigiku R., Caudill D., Fey M.L. d-Limonene-induced male rat-specific nephrotoxicity: Evaluation of the association between d-limonene and α2u-globulin. Toxicol. appl. Pharmacol. 1989;99:250–259. [PubMed: 2472019]
- Lehman-McKeeman L.D., Rivera-Torres M.I., Caudill D. Lysosomal degradation of α2u-globulin and α2u-globulin–xenobiotic conjugates. Toxicol. appl. Pharmacol. 1990;103:539–548. [PubMed: 1692643]
- Lide, D.R., ed. (1991) CRC Handbook of Chemistry and Physics, 72nd Ed., Boca Raton, FL, CRC Press, p. 3–308.
- Nakaizumi A., Baba M., Uehara H., Iishi H., Tatsuta M. 1997 d-Limonene inhibits N-nitrosobis(2-oxopropyl)amine induced hamster pancreatic carcinogenesis. Cancer Lett. 117, 99–103. [PubMed: 9233838]
- National Board of Occupational Safety and Health (1996) Occupational Exposure Limit Values, Solna.
- National Institute for Occupational Safety and Health (1998) National Occupational Exposure Survey (1981–1983), Cincinnati, OH.
- National Library of Medicine (1998) Hazardous Substances Data Bank (HSDB), Bethesda, MD [Record No. 4186]
- National Toxicology Program (1990) NTP Technical Report on the Toxicology and Carcinogenesis Studies of d-Limonene (CAS No. 5989-27-5) in F344/N Rats and B6C3F1 Mice (Gavage Studies) (NTP TR 347), Research Triangle Park, NC, National Institutes of Health, pp. 145–151. [PubMed: 12704437]
- National Toxicology Program (1991) NTP Chemical Repository Data Sheet: d-Limonene. Research Triangle Park, NC.
- O’Shaughnessy J.A. Chemoprevention of breast cancer. J. Am. med. Assoc. 1996;275:1349–1353. [PubMed: 8614122]
- Olson M.J., Johnson J.T., Reidy C.A. A comparison of male rat and human urinary proteins: Implications for human resistance to hyaline droplet nephropathy. Toxicol. appl. Pharmacol. 1990;102:524–536. [PubMed: 1690459]
- Opdyke D.L.J. d-Limonene. Food Cosmet. Toxicol. 1975;13 Suppl.:825–826. [PubMed: 1225772]
- Oshiro Y., Balwierz P.S., Eurell T.E., Morris D.L., Alden C.L. Exploration of the transformation potential of a unique male rat protein alpha2u-globulin using hamster embryonic cells. Toxicol. Pathol. 1998;26:381–387. [PubMed: 9608644]
- Poon G.K., Vigushin D., Griggs L.J., Rowlands M.G., Coombes R.C., Jarman M. Identification and characterization of limonene metabolites in patients with advanced cancer by liquid chromatography/mass spectrometry. Drug Metab. Disposition. 1996;24:565–571. [PubMed: 8723738]
- Roy A.K., Neuhaus O.W., Harmison C.R. Preparation and characterization of a sex-dependent rat urinary protein. Biochim. biophys. Acta. 1966;127:72–81. [PubMed: 4165834]
- Saito K., Uwagawa S., Kaneko H., Shiba K., Tomigahara Y., Nakatsuka I. alpha(2u)-Globulins in the urine of male rats: A reliable indicator for alpha(2u)-globulin accumulation in the kidney. Toxicology. 1996;106:149–157. [PubMed: 8571386]
- Steele V.E., Kelloff G.J., Wilkinson B.P., Arnold J.T. Inhibition of transformation in cultured rat tracheal epithelial cells by potential chemopreventive agents. Cancer Res. 1990;50:2068–2074. [PubMed: 2138505]
- Swenberg, J.A. & Lehman-McKeeman, L.D. (1999) α2u-Urinary globulin-associated nephropathy as a mechanism of renal tubular cell carcinogenesis in male rats. In: Capen, C.C., Dybing, E., Rice, J.M. & Wilbourn, J.D., eds, Species Differences in Thyroid, Kidney and Urinary Bladder Carcinogenesis (IARC Scientific Publications No. 147), Lyon, IARC, pp. 95–118.
- Swenberg J.A., Short B.G., Borghoff S.J., Strasser J., Charbonneau M. The comparative pathobiology of α2u-globulin nephropathy. Toxicol. appl. Pharmacol. 1989;97:35–46. [PubMed: 2464861]
- Tsuji M., Fujisaki Y., Arikawa Y., Masuda S., Kinoshita S., Okubo A., Noda K., Ide H., Iwanage Y. [Studies on d-limonene, as gallstone solubilizer. II. Acute and subacute toxicities.] Oyo Yakuri. 1975a;9:387–401. (in Japanese)
- Tsuji M., Fujisaki Y., Arikawa Y., Masuda S., Tanaka T., Sato K., Noda K., Ide H., Kikuchi M. [Studies on d-limonene, as gallstone solubilizer. IV. Chronic toxicity in dogs.] Oyo Yakuri. 1975b;9:775–808. (in Japanese)
- Vigushin D.M., Poon G.K., Boddy A., English J., Halbert G.W., Pagonis C., Jarman M., Coombes R.C. Phase I and pharmacokinetic study of d-limonene in patients with advanced cancer. Cancer Chemother. Pharmacol. 1998;42:111–117. [PubMed: 9654110]
- Watabe T., Hiratsuka A., Isobe M., Ozawa N. Metabolism of d-limonene by hepatic microsomes to non-mutagenic epoxides toward Salmonella typhimurium. Biochem. Pharmacol. 1980;29:1068–1071. [PubMed: 6992785]
- Watabe T., Hiratsuka A., Ozawa N., Isobe M. A comparative study of the metabolism of d-limonene and 4-vinylcyclohex-1-ene by hepatic microsomes. Xenobiotica. 1981;11:333–344. [PubMed: 7293224]
- Webb D.R., Kanerva R.L., Hysell D.K., Alden C.L., Lehman-McKeeman L.D. Assessment of the chronic oral toxicity of d-limonene in dogs. Food chem. Toxicol. 1990;28:669–675. [PubMed: 2276695]
- WHO (1993) Guidelines for Drinking Water Quality, 2nd Ed., Vol. 1, Recommendations, Geneva.
- Zani F., Massimo G., Benvenuti S., Bianchi A., Albasini A., Meleari M., Vampa G., Beilotti A., Mazza P. Studies on the genotoxic properties of essential oils with Bacillus subtilis rec-assay and Salmonella/microsome reversion assay. Planta med. 1991;57:237–241. [PubMed: 1910178]
- Lack of chemoprevention effects of the monoterpene d-limonene in a rat multi-organ carcinogenesis model.[Jpn J Cancer Res. 1996]Lack of chemoprevention effects of the monoterpene d-limonene in a rat multi-organ carcinogenesis model.Kimura J, Takahashi S, Ogiso T, Yoshida Y, Akagi K, Hasegawa R, Kurata M, Hirose M, Shirai T. Jpn J Cancer Res. 1996 Jun; 87(6):589-94.
- The presence of alpha 2u-globulin is necessary for d-limonene promotion of male rat kidney tumors.[Cancer Res. 1991]The presence of alpha 2u-globulin is necessary for d-limonene promotion of male rat kidney tumors.Dietrich DR, Swenberg JA. Cancer Res. 1991 Jul 1; 51(13):3512-21.
- Review d-limonene mechanistic data and risk assessment: absolute species-specific cytotoxicity, enhanced cell proliferation, and tumor promotion.[Pharmacol Ther. 1996]Review d-limonene mechanistic data and risk assessment: absolute species-specific cytotoxicity, enhanced cell proliferation, and tumor promotion.Whysner J, Williams GM. Pharmacol Ther. 1996; 71(1-2):127-36.
- Review Quercetin.[IARC Monogr Eval Carcinog Risk...]Review Quercetin.. IARC Monogr Eval Carcinog Risks Hum. 1999; 73:497-515.
- Review d-Limonene.[IARC Monogr Eval Carcinog Risk...]Review d-Limonene.. IARC Monogr Eval Carcinog Risks Hum. 1993; 56:135-62.
- d-Limonene - Some Chemicals that Cause Tumours of the Kidney or Urinary Bladder ...d-Limonene - Some Chemicals that Cause Tumours of the Kidney or Urinary Bladder in Rodents and Some Other Substances
- Hexachlorobutadiene - Some Chemicals that Cause Tumours of the Kidney or Urinary...Hexachlorobutadiene - Some Chemicals that Cause Tumours of the Kidney or Urinary Bladder in Rodents and Some Other Substances
- THE MONOGRAPHS - Some Chemicals that Cause Tumours of the Kidney or Urinary Blad...THE MONOGRAPHS - Some Chemicals that Cause Tumours of the Kidney or Urinary Bladder in Rodents and Some Other Substances
- PREAMBLE - Some Chemicals that Cause Tumours of the Kidney or Urinary Bladder in...PREAMBLE - Some Chemicals that Cause Tumours of the Kidney or Urinary Bladder in Rodents and Some Other Substances
- homo sapiens[Organism] AND FOPNL AND (alive[prop]) (4)Gene
Your browsing activity is empty.
Activity recording is turned off.
See more...