NCBI Bookshelf. A service of the National Library of Medicine, National Institutes of Health.
IARC Working Group on the Evaluation of Carcinogenic Risks to Humans. Some Chemicals that Cause Tumours of the Kidney or Urinary Bladder in Rodents and Some Other Substances. Lyon (FR): International Agency for Research on Cancer; 1999. (IARC Monographs on the Evaluation of Carcinogenic Risks to Humans, No. 73.)
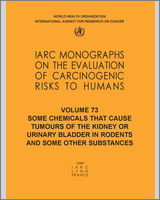
Some Chemicals that Cause Tumours of the Kidney or Urinary Bladder in Rodents and Some Other Substances.
Show detailsThis substance was considered by previous working groups, in 1978 (IARC, 1979) and 1987 (IARC, 1987). Since that time, new data have become available, and these have been incorporated into the monograph and taken into consideration in the present evaluation.
1. Exposure Data
1.1. Chemical and physical data
1.1.1. Nomenclature
- Chem. Abstr. Serv. Reg. No.: 87-68-3
- Chem. Abstr. Name: 1,1,2,3,4,4-Hexachloro-1,3-butadiene
- IUPAC Systematic Name: Hexachlorobutadiene
- Synonyms: HCB; hexachloro-1,3-butadiene; perchloro-1,3-butadiene; perchlorobutadiene
1.1.2. Structural and molecular formulae and relative molecular mass
1.1.3 Chemical and physical properties of the pure substance
- (a) Description: Clear, colourless liquid with mild odour (Lewis, 1993)
- (b) Boiling-point: 215°C (Lide, 1997)
- (c) Melting-point: −21°C (Lide, 1997)
- (d) Density: 1.556 g/cm3 at 25°C (Lide, 1997)
- (e) Solubility: Insoluble in water; soluble in ethanol and diethyl ether (Lide, 1997)
- (f) Volatility: Vapour pressure: 2926 Pa at 100°C (Verschueren, 1996)
- (g) Octanol/water partition coefficient (P): log P, 4.78 (Verschueren, 1996)
- (h) Conversion factor: mg/m3 = 10.66 × ppm
1.2. Production and use
Hexachlorobutadiene is produced chiefly as a by-product in the manufacture of chlorinated solvents and related products, in which it occurs in the heavy fractions (WHO, 1994). Information available in 1994 indicated that hexachlorobutadiene was produced only in Austria (Chemical Information Services, 1995).
Hexachlorobutadiene is used as a solvent for natural rubber, synthetic rubber and other polymers. It is used as a heat-transfer liquid, a transformer liquid, a hydraulic fluid and as a washing liquour for removing hydrocarbons (Verschueren, 1996). It is also used as a pesticide, insecticide, herbicide, algicide and chemical intermediate (National Toxicology Program, 1991).
1.3. Occurrence
1.3.1. Natural occurrence
Hexachlorobutadiene is not known to occur naturally.
1.3.2. Occupational exposure
According to the 1981–83 National Occupational Exposure Survey (National Institute for Occupational Safety and Health, 1998), approximately 1000 workers in the United States were potentially exposed to hexachlorobutadiene. Occupational exposure to hexachlorobutadiene may occur during its production and during its use as a solvent, heat-transfer liquid, transformer liquid, hydraulic fluid, washing liquour, pesticide or chemical intermediate (National Toxicology Program, 1991).
1.3.3. Environmental occurrence
Hexachlorobutadiene has been measured in urban air at concentrations < 0.5 µg/m3; the concentrations in remote areas were < 1 pg/m3. Hexachlorobutadiene is frequently detected in ambient water and has been detected in drinking-water at 2–3 ng/L. In lake and river water in Europe, concentrations up to 2 µg/L have been recorded, but the mean level is usually < 0.1 µg/L. In the Great Lakes area of Canada, much lower concentrations (around 1 ng/L) were measured; however, the concentrations in bottom sediment can be as high as 120 µg/kg of dry weight. Sediment layers from around 1960 contained up to 550 µg/kg of wet weight (Agency for Toxic Substances and Disease Registry, 1994; WHO, 1994).
The concentrations of hexachlorobutadiene detected in aquatic organisms, birds and mammals indicate bioaccumulation but not biomagnification. In several species in polluted waters, concentrations > 1000 µg/kg of wet weight have been measured, and 120 mg/kg (lipid base) were found in one species. The concentrations in organisms distant from industrial outflows are generally < 100 µg/kg of wet weight. Hexachlorobutadiene has been detected in human urine, blood and tissues. Certain food items with a high lipid fraction, such as fish, have been found to contain up to 40 µg/kg and, in one case, over 1000 µg/kg (WHO, 1994).
According to the Environmental Protection Agency Toxic Chemical Release Invetory for 1987, 1600 kg hexachlorobutadiene were released into the air, 86 kg were discharged into water, and 32 kg were disposed of by underground injection from manufacturing and processing facilities in the United States. By 1996, the concentrations were 1100 kg released into air, 120 kg discharged into water and 430 kg disposed of by underground injection (National Library of Medicine, 1998).
1.4. Regulations and guidelines
The American Conference of Governmental Industrial Hygienists (1997) recommended 0.21 mg/m3 as the 8-h time-weighted average threshold limit value, with a skin notation for potential dermal absorption, for exposure to hexachlorobutadiene in workplace air. Values of 0.21–0.25 mg/m3 have been used as standards or guidelines in other countries (International Labour Office, 1991).
WHO (1993) has established an international drinking-water guideline for hexachlorobutadiene of 0.6 µg/L.
2. Studies of Cancer in Humans
No data were available to the Working Group.
3. Studies of Cancer in Experimental Animals
Previous evaluation
Hexachlorobutadiene was tested in one experiment in rats by oral administration, producing renal tubule adenomas and carcinomas in animals of each sex. It was tested inadequately in one experiment in mice by intraperitoneal injection (IARC, 1979).
New studies
3.1. Skin application
Mouse: Groups of 30 female non-inbred Ha:ICR Swiss mice, six to eight weeks of age, each received applications of 2 or 6 mg hexachlorobutadiene (purity confirmed) in 0.2 mL acetone on the dorsal skin three times per week for 440–594 days. Their rate of survival was described as ‘excellent’. The treatment produced no squamous-cell papillomas or carcinomas of the skin (Van Duuren et al., 1979).
3.2. Administration with known carcinogens or modifying factors
Mouse: Groups of 30 female non-inbred Ha:ICR Swiss mice received a single application of 15 mg hexachlorobutadiene (purity ‘confirmed’) in 0.2 mL acetone on the dorsal skin, followed 14 days later by 5 µg phorbol myristate acetate in 0.2 mL acetone three times a week as a promoting agent for 428–576 days. The rate of survival was described as ‘excellent’. The compound did not initiate skin tumours (Van Duuren et al., 1979).
Rat: Groups of 21 male Wistar rats, six weeks of age, were given 0.1% N-nitrosoethylhydroxyethylamine (NEHEA) in the drinking-water for two weeks and then 0.1% hexachlorobutadiene (purity, > 99%) in the diet for 30 weeks. Three other groups received either hexachlorobutadiene or NEHEA according to the same regimen or basal diet for 32 weeks. The rate of survival was 100%. The incidence of renal tubular tumours in the group given NEHEA plus hexachlorobutadiene (15/21) was greater than that in rats given NEHEA alone (5/10), and the incidence of preneoplastic renal tubular hyperplasia was also increased (21/21 versus 4/10) (Nakagawa et al., 1998).
4. Other Data Relevant to an Evaluation of Carcinogenicity and its Mechanisms
4.1. Absorption, distribution, metabolism and excretion
4.1.1. Humans
The sulfoxidation of the mercapturic acid N-acetyl-S-(1,2,3,4,4-pentachlorobuta-1,3-dienyl)-l-cysteine (N-ac-PCBC), a urinary metabolite of the glutathione-dependent bioactivation of hexachlorobutadiene, was studied in liver microsomes from male and female donors with use of reconstituted recombinant cytochrome P450 enzymes (Werner et al., 1995). N-ac-PCBC sulfoxide is a major urinary metabolite of hexachlorobutadiene in male rats dosed orally, and only liver microsomes from male rats catalyse the sulfoxidation of N-ac-PCBC (Birner et al., 1995; see section 4.1.2). In contrast, liver microsomes from both male and female donors are capable of oxidizing N-ac-PCBC to the corresponding sulfoxide diastereomers. The correlation of N-ac-PCBC sulfoxidation with the rates of oxidation of P450 enzyme-specific substrates suggests that only cytochrome P450 3A enzymes oxidize N-ac-PCBC. Moreover, only gestodene and troleandomycin, two selective inhibitors for P450 3A isozymes, significantly reduced the rates of N-ac-PCBC sulfoxidation. No reduction in sulfoxidation rates was observed with inhibitors for other P450 enzymes. Incubation of N-ac-PCBC with purified and reconstituted recombinant P450s 1A2, 2E1, 3A4 and 3A5 resulted in sulfoxide formation only with P450s 3A4 and 3A5. In summary, these results indicate that P450 from the 3A family may sulfoxidize N-ac-PCBC (Werner et al., 1995).
4.1.2. Experimental systems
Hexachlorobutadiene caused a marked reduction in glutathione concentration when incubated with male or female rat hepatic microsomal or cytosolic fractions fortified with glutathione. The depletion of glutathione in the microsomal fraction was not related to the formation of metabolites via cytochrome P450 but appeared to be due to a direct reaction catalysed by a microsomal glutathione S-transferase. Mass spectral analysis of a glutathione adduct of hexachlorobutadiene showed that the structure was S-(pentachlorobutadienyl)glutathione, confirming a direct substitution reaction without prior oxidation. This conjugate was formed faster by the hepatic microsomal fraction than by the cytosolic fraction. Glutathione adducts were also formed by male and female kidney cytosol and microsomal fractions but at a slower rate than in the liver fractions. It was suggested that the glutathione conjugate of hexachlorobutadiene is converted to the cysteine derivative, the structure of which is similar to that of S-dichlorovinyl-l-cysteine, and may therefore be nephrotoxic by a similar mechanism (Wolf et al., 1984).
The hepatic metabolism of hexachlorobutadiene was investigated in isolated rat hepatocytes and after administration in vivo. Exposure of isolated hepatocytes to hexachlorobutadiene resulted in dose-dependent depletion of glutathione, and formation of both a mono- and a bis-substituted glutathione conjugate of hexachlorobutadiene was observed. The identity of the mono- and bis-glutathione conjugates was confirmed by high-performance liquid chromatography in which synthetic standards had identical retention times. The production of total and individual glutathione conjugates was both dose- and time-dependent. The production of total conjugates and the ratio of mono- to bis-conjugate was found to depend on the availability of glutathione. After exposure of isolated hepatocytes to a low concentration of hexachlorobutadiene, the bis-substituted conjugate accounted for more than 20% of the total conjugate produced, while this value decreased at higher concentrations. Analysis of bile collected from rats given hexachlorobutadiene intraportally revealed the presence of both the mono- and bis-substituted glutathione conjugates and additional 14C-containing metabolites (Jones et al., 1985).
The glutathione conjugate of hexachlorobutadiene formed in the liver, S-(pentachlorobutadienyl)glutathione, is secreted into bile (because of its high molecular mass and the presence of an effective transporter at the canalicular membrane of the hepatocyte), reabsorbed in the gut, intact or after hydrolysis to S-(pentachlorobutadienyl)-l-cysteine, and undergoes enterohepatic circulation or translocation to the kidney. Hepatic uptake and metabolism of S-(pentachlorobutadienyl)glutathione and S-(pentachlorobutadienyl)-l-cysteine may thus influence their renal disposition. In isolated perfused rat liver, dose-dependent uptake of both the glutathione and the cysteine conjugate from the perfusion medium was demonstrated, the cysteine conjugate being cleared from the perfusion medium to a much greater extent than the glutathione conjugate. Both conjugates were extensively biotransformed by further conjugation with glutathione and subsequent hydrolysis of the glutathione moieties. Hepatic biosynthesis of N-ac-PCBC from the cysteine conjugate was a minor pathway in both cysteine and glutathione conjugate metabolism in liver. These results indicate that hepatic biosynthesis of mercapturic acids may not contribute to the disposition of S-conjugates formed from hexachlorobutadiene in vivo and that S-(pentachlorobutadienyl)glutathione is, at least in part, delivered intact to the kidney (Koob & Dekant, 1992).
After administration of [14C]-hexachlorobutadiene to male and female Wistar rats at 200 mg/kg bw by gavage, no significant difference was observed between males and females in the disposition or rate of excretion of radiolabel. Of the dose, 16% (± 4.2%) was excreted in the faeces and 3.1% (± 0.7%) in the urine of male rats and 11% (± 3.8%) in the faeces and 4.5% (± 1.5%) in the urine of female rats. The major metabolite excreted by female rats was N-ac-PCBC; small amounts of S-(pentachlorobutadienyl)-l-cysteine were detected. The urine of male rats contained these two compounds and also N-ac-PCBC sulfoxide and unmetabolized hexachlorobutadiene (Birner et al., 1995).
Birner et al. (1995) reported the excretion of unmetabolized hexachlorobutadiene in the urine of male but not female rats. In order to investigate the basis for the excretion of highly lipophilic hexachlorobutadiene in the urine, [14C]hexachlorobutadiene (200 mg/kg bw) was administered orally to male and female rats of the Sprague-Dawley strain and to NCI Black-Reiter (NBR) rats which are deficient in α2u-globulin. No significant difference in the disposition or rate of excretion of radiolabel was observed between male and female animals of either strain. The previously observed sex-specific differences in the formation of urinary metabolites in Wistar rats were confirmed in Sprague-Dawley rats and were also found in NBR rats. In contrast to male Sprague-Dawley rats, however, NBR rats did not excrete unmetabolized hexachlorobutadiene in the urine. [14C]Hexachlorobutadiene (10% of total urinary metabolites) was present only in the urine of male Sprague-Dawley rats and was associated with the α2u-globulin fraction in urine and renal cytosol; the radiolabelled compound was identified as hexachlorobutadiene bound to the protein. The results indicate that male-specific urinary excretion of hexachlorobutadiene is associated with its binding to α2u-globulin (Pähler et al., 1997).
4.2. Toxic effects
4.2.1. Humans
No data were available to the Working Group.
4.2.2. Experimental systems
A single intraperitoneal dose of 100 mg/kg bw hexachlorobutadiene given to male Sprague-Dawley rats caused reductions in body weight and urine osmolality throughout the 96-h observation period. The rate of urine flow increased slightly, and marked increases in urinary protein, glucose and ketones content were observed. Renal slices taken after the same dose showed reduced accumulation of polycyclic aromatic hydrocarbons, whereas the transport of other organic compounds was affected only slightly. After daily administration on four successive days of 25–100 mg/kg bw hexachlorobutadiene, a dose-dependent response was observed for both transport and overall renal function. Glutathione administered in a 2.5-fold molar excess did not prevent the nephrotoxic effects of hexachlorobutadiene (Berndt & Mehendale, 1979).
Intraperitoneal administration of 300 mg/kg bw hexachlorobutadiene to male Sprague-Dawley rats decreased the urine osmolality and glomerular filtration rate and increased urinary excretion of proteins and ketones within 4 h. At 24 h, an elevated blood urea nitrogen concentration was found; however, no definite signs of hepatotoxicity were observed up to 48 h after treatment. Control rats excreted 40% of an intraperitoneal tracer dose (0.1 mg/kg bw) of [14C]hexachlorobutadiene in the faeces and 30% in the urine over 48 h, whereas rats with hexachlorobutadiene-induced nephrotoxicity excreted only 7% in faeces and 6% in urine. Extraction of urine and bile samples with hexane revealed that all of the radiolabel in bile and 87% of that in urine was water-soluble, indicating that the insoluble hexachlorobutadiene was extensively biotransformed into polar metabolites (Davis et al., 1980).
Two-month-old male Wistar rats given a single intraperitoneal injection of 100 mg/kg bw hexachlorobutadiene showed necrosis of the S3 portion of the proximal convoluted tubule 24 h after administration. Significant increases in the activity of glutamine transaminase K (β-lyase) were detected in the urine as early as 10 h after dosing (Trevisan et al., 1998).
The renal and hepatic glutathione concentrations were measured after treatment of male Sprague-Dawley rats with hexachlorobutadiene or citrinin (a nephrotoxic mycotoxin), alone or in combination, and after pretreatment with the glutathione-depleting agent diethylmaleate. Both renal and hepatic glutathione depletion was increased significantly when either citrinin (35 mg/kg bw) or hexachlorobutadiene (300 mg/kg bw) was given after diethylmaleate, while no difference in glutathione concentration was observed in the absence of pretreatment. When hexachlorobutadiene and citrinin were given in combination, the effect on the glutathione concentration was approximately additive. Renal tubular organic ion transport in kidney slices was also compromised significantly when either citrinin or hexachlorobutadiene was given after pretreatment with diethylmaleate. Hexachlorobutadiene depressed tetraethylammonium transport after diethylmaleate treatment; when given alone, hexachlorobutadiene had no effect on tetraethyl-ammonium transport (Baggett & Berndt, 1984).
In isolated rat renal tubular cells, N-ac-PCBC sulfoxide was more cytotoxic than N-ac-PCBC. After identical doses of hexachlorobutadiene, the extent of necrosis of the pars recta of the proximal tubules was greater in male than in female rats. While the livers of female animals appeared normal histologically, those of male rats had slight toxic centrilobular changes. In vitro, only liver microsomes from male rats catalysed the formation of N-ac-PCBC sulfoxide from N-ac-PCBC. The authors concluded that formation of this mercapturic acid sulfoxide from hexachlorobutadiene in male rats might contribute to the sex difference in nephrotoxicity (Birner et al., 1995).
Administration of a single intraperitoneal dose of 50 mg/kg bw hexachlorobutadiene caused necrosis of the renal proximal tubules of male Alderly Park albino mice. The earliest morphological changes observed by light microscopy were seen after 4 h; by 16 h, extensive proximal tubular necrosis was seen throughout the cortex. Active tubular regeneration was apparent by day 5, and substantial recovery had occurred by day 14. Electron microscopy showed mitochondrial swelling in the S1 and S2 segments of the proximal tubule 1 and 2 h after dosing, whereas by 4 and 8 h the major pathological changes were confined to the lower S2 and S3 segments and consisted of mitochondrial swelling and cellular necrosis. The extent of renal injury and regeneration correlated well with measurements of renal function, i.e. plasma urea, renal water and non-protein sulfhydryl content (Ishmael et al., 1984).
Hexachlorobutadiene (50 mg/kg bw intraperitoneally) produced a time- and dose-related increase in hepatic water content in male Alderley Park mice. The increase in liver water was maximal one to two days after a treatment, but the content had returned to normal by day 5. The increased water was accompanied by a parallel increase in Na+ and K+ ion concentrations, with no overall change in the intracellular cation concentration. The liver non-protein sulfhydryl content was not decreased after administration of 50 mg/kg bw hexachlorobutadiene. Histopathological examination of the liver showed fine cytoplasmic vacuolation of periportal hepatocytes, which was more marked after a dose of 100 or 200 mg/kg bw than after 50 mg/kg bw hexachlorobutadiene. Ultrastructural changes were evident 4 h after administration of 50 mg/kg bw and consisted of mitochondrial swelling in periportal hepatocytes; in contrast, the pericentral hepatocytes appeared to be normal. By 16 h, marked mitochondrial swelling and some proliferation of the smooth endoplasmic reticulum were evident in periportal hepatocytes. Male mice of the C57BL/10J and C3H strains appeared to be more sensitive to hexachlorobutadiene-induced hepatic hydropic change than male or female Alderley Park strain or male BALB/c and DBA/2J strains. The authors concluded that hexachlorobutadiene or a metabolite may disrupt mitochondria in periportal hepatocytes, resulting in an influx of water and ions into the cell without compromising the Na+ pump (Lock et al., 1985).
Male and female Alderley Park rats, six to eight weeks of age, were given a single intraperitoneal injection of 0–200 mg/kg bw hexachlorobutadiene or its glutathione conjugate, its cysteine conjugate or its N-acetylcysteine conjugate (0–100 mg/kg bw) and killed 24 h later. Nephrotoxicity was assessed by histological examination and plasma urea concentration. All three glutathione-derived conjugates increased the amount of necrosis in the pars recta of the renal proximal tubules, with corresponding increases in plasma urea, as did hexachlorobutadiene. The S-conjugates were stronger nephrotoxins than hexachlorobutadiene itself. Hexachlorobutadiene was about four times more toxic to female rats than males, and females were also more susceptible to the metabolites (Ishmael & Lock, 1986).
Renal damage was assessed by histopathological examination and urine analysis in male Wistar rats treated with either hexachlorobutadiene (a single intraperitoneal dose of 170 mg/kg bw, which caused proximal tubule necrosis), adriamycin (a single intraperitoneal dose of 5 mg/kg bw, which caused minimal glomerular changes up to 35 days) or hexachlorobutadiene given two weeks after adriamycin; the animals were then compared with age-matched control rats for 21 days. Analysis of the urine of adriamycin-treated rats showed minimal renal changes. Hexachlorobutadiene significantly increased the urine volume (10-fold), protein (5-fold), glucose (175-fold) and brush border enzyme activities (10–600-fold), indicating severe proximal tubular damage, but most of these parameters had returned to pretreatment levels six days after treatment. In adriamycin-pretreated rats subsequently given hexachlorobutadiene, both urinary alkaline phosphatase activity and the ratio of kidney:body weight were significantly higher for longer periods. Histopathological examination showed that the hexachlorobutadiene-induced proximal tubular lesions were confined to the outer stripe of the outer medulla. Advanced regeneration and repair were evident 21 days after treatment. In the adriamycin-pretreated rats, the hexachlorobutadiene-induced lesions were more severe, affected the entire cortex and were characterized by marked tubular epithelial calcification, with little evidence of repair 21 days after treatment. Enzyme histochemistry showed that γ-glutamyl transpeptidase was localized to the proximal tubules. The enzyme staining was lost after hexachlorobutadiene treatment, but returned in parallel with histological recovery up to 21 days. The distribution and intensity of γ-glutamyl transpeptidase was unchanged in adriamycin-treated rats, but the distribution and intensity of this enzyme in kidneys of adriamycin-pretreated rats given hexachlorobutadiene had not returned to normal by day 21. The results indicate that pretreatment with adriamycin increases hexachlorobutadiene-induced nephrotoxic damage and decreases renal cortical repair capacity (Kirby & Bach, 1995).
Light microscopic examination of the kidneys from the study of Pähler et al. (1997) described in section 4.1 revealed the formation of hyaline droplets, indicative of the accumulation of α2u-globulin, in the kidneys of male Sprague-Dawley rats. Haematoxylin- and-eosin staining demonstrated more pronounced necrotic changes in the renal tubules of male Sprague-Dawley rats than in those of females, as previously described for Wistar rats. [The Working Group noted that the changes in α2u-globulin took place against a background of severe renal toxicity.]
The intranephron distribution of two major cysteine S-conjugate β-lyases was determined in order to clarify the role of these enzymes in promoting the nephrotoxicity associated with certain halogenated xenobiotics. Collagenase-treated rat kidneys were micro-dissected in order to isolate various nephron segments: glomeruli, early, middle and terminal portions of the proximal tubules (S1, S2 and S3, respectively), thick ascending limbs, distal tubules and collecting tubules. Each segment was dissected in Hanks’ solution, solubilized with Triton X-100 and applied to a micropolyacrylamide gel constructed with a continuous gradient. The gels were subjected to electrophoresis and then incubated in the dark in a solution containing dichlorovinylcysteine, sodium α-keto-γ-methiolbutyrate, phenazine methosulfate and nitroblue tetrazolium. The positions of the cysteine S-conjugate β-lyase and L-amino acid oxidase activities in the gels were revealed by the presence of blue formazen dye bands, and the relative intensities of the bands were determined by optical scanning with a microdensitometer. Three bands were detected: band I (Mr similar to 330 000), corresponding to a recently described high-molecular-mass cysteine S-conjugate β-lyase; band III (Mr similar to 90 000), corresponding to a lower Mr cysteine S-conjugate β-lyase identical to cytosolic glutamine transaminase K; and band II (Mr similar to 240 000), corresponding to l-amino acid oxidase, a unique activity of the B isoform of rat kidney l-hydroxy acid oxidase (Kim et al., 1997).
4.3. Reproductive and developmental effects
4.3.1. Humans
No data were available to the Working Group.
4.3.2. Experimental systems
No deleterious effects on fertility or on the health of chicks were noted when adult male and female Japanese quail were maintained on a diet that contained up to 30 ppm (320 mg/m3) per day hexachlorobutadiene for 90 days prior to mating (Schwetz et al., 1974).
Groups of six female Wistar-derived rats were fed a diet containing 0, 150 or 1500 ppm hexachlorobutadiene (purity, > 95%; coresponding to 0, 1600 and 16 000 mg/m3) for four weeks prior to mating with untreated males. Exposure continued until the offspring were 18 weeks of age, when they were necropsied. Females at the high dose lost weight progressively during exposure and produced no litters. The pups of dams at the low dose had significantly reduced birth weights and subsequent growth but no grossly observable malformation. At necropsy, the kidneys of pups of dams at the low dose were heavier and displayed hypercellularity of the epithelial lining and hydropic degeneration and necrosis of cells in the straight limbs of the proximal tubules (Harleman & Seinen, 1979).
Hexachlorobutadiene was administered at 0 or 10 mg/kg bw by intraperitoneal injection to groups of 10–15 Sprague Dawley rats on days 1–15 of gestation, and the fetuses were examined for viability, growth and morphology on gestation day 21. Maternal toxicity [nature not specified] and fetal toxicity (delayed development of the heart and urogenital tract) but no teratogenic effects were reported (Hardin et al., 1981). [The Working Group noted the limited reporting of the experimental results.]
Groups of 24 female Sprague Dawley rats were exposed via inhalation to hexachlorobutadiene (purity, 99%) at concentrations of 0, 2, 5 or 15 ppm (corresponding to 0, 21, 53, 110 and 160 mg/m3 ) for 6 h per day on days 6–20 of gestation. Fetuses were examined on gestation day 21 by standard teratological techniques. Maternal weight gain during gestation was significantly reduced at the two highest doses. There was no effect on fetal viability, but the body weights of fetuses of dams at 15 ppm were reduced. No significant effects were observed on fetal morphology (Saillenfait et al., 1989).
4.4. Genetic and related effects
4.4.1. Humans
No data were available to the Working Group.
4.4.2. Experimental systems (see Table 1 for references)
Table 1
Genetic and related effects of hexachlorobutadiene.
Hexachlorobutadiene produced a dose-dependent increase in Arar forward mutation frequency in Salmonella typhimurium that was more marked in the absence than in presence of an exogenous metabolic system. Hexachlorobutadiene was not mutagenic to S. typhimurium strains TA98 and TA100 either in the presence or the absence of the usual metabolic activation systems; however, it was mutagenic to the TA100 strain when a fortified system was used and when glutathione was added during preincubation. Furthermore, the mutagenic effect was enhanced by a longer preincubation time and by the inclusion of rat kidney microsomes. In the presence of metabolic activation, the mutagenic response induced by the mercapturic acid derivative of hexachlorobutadiene was 80 times greater than that of the parent compound (Wild et al., 1986). Perchloro-3-butenoic acid and perchloro-3-butenoic acid chloride, two possible oxidative metabolites of hexachlorobutadiene, were mutagenic in the absence of metabolic activation and exerted a mutagenic effect two- to threefold higher than that of hexachlorobutadiene in the presence of activation (Reichert et al., 1984). Hexachlorobutadiene did not induce sex-linked recessive lethal mutations in Drosophila melanogaster.
Unscheduled DNA synthesis, as measured by liquid scintillation counting, was not induced in rat primary hepatocytes but was elicited by hexachlorobutadiene and its putative metabolite pentachlorobutanoic acid in Syrian hamster embryo fibroblasts, the responses being approximately three times greater in the presence than in the absence of exogenous metabolization. Morphological transformation was induced in Syrian hamster embryo fibroblasts by hexachlorobutadiene and its putative metabolite pentachlorobutenoic acid. Hexachlorobutadiene increased the frequency of sister chromatid exchange in Chinese hamster ovary cells in both the absence and presence of an exogenous metabolic system from liver, but did not induce chromosomal aberrations under the same experimental conditions.
Covalent binding of [14C]hexachlorobutadiene metabolites to rat kidney DNA and to renal and hepatic mitochondrial DNA was detected in mice given a single oral dose of 30 mg/kg bw of the parent compound, binding being 15-fold higher in kidneys. The metabolites bound to a much greater extent to mitochondrial DNA than to nuclear DNA in the kidneys, probably because of greater β-lyase activity in the mitochondrial membrane and the absence of histones (Schrenk & Dekant, 1989).
Further information on the reactive metabolites of hexachlorobutadiene is provided by the results of the following studies. The cysteine and glutathione conjugates of hexachlorobutadiene were mutagenic to S. typhimurium TA100 in the absence and to a greater extent in the presence of a metabolic activation system from rat kidney. The cysteine conjugate was found to be metabolized by rat kidney slices and rat kidney β-lyase to pyruvate, ammonia and an unidentified reactive metabolite, and this effect is consistent with the known renal carcinogenicity of hexachlorobutadiene (Green & Odum, 1985). S-(Pentachlorobutadienyl)-l-cysteine, which is regarded as the penultimate metabolite of hexachlorobutadiene, was mutagenic to S. typhimurium strains TA100, TA2638 and TA98 without the addition of a metabolic activation system. In the presence of a 100 000 × g supernatant from these bacteria, which contain significantly higher concentrations of β-lyase than rat subcellular fractions, the cleavage of S-(pentachlorobutadienyl)-l-cysteine resulted in a time-dependent, linear formation of pyruvate, ammonia and a thiol which is regarded as the ultimate genotoxic metabolite (Dekant et al., 1986). S-(Pentachlorobutadienyl)-l-cysteine was shown to be activated by β-lyase to thioacylating reactive intermediates which bind covalently to DNA of S. typhimurium TA100 and of isolated rat renal proximal tubular cells (Vamvakas et al., 1988b). No DNA single-strand breaks but some evidence of DNA cross-links was observed in isolated rabbit renal tubules after exposure to 1 mmol/L S-(pentachlorobutadienyl)cysteine (Jaffe et al., 1983).
4.5. Mechanistic considerations
The cysteine S-conjugate of hexachlorobutadiene is concentrated in the renal proximal tubules and cleaved by β-lyases, which are very active in this part of the nephron, and the reactive thioketene formed can acylate proteins and DNA. This toxification pathway is responsible for the renal-specific toxicity and carcinogenicity of hexachlorobutadiene in rodents (Anders et al., 1992; Koob & Dekant, 1992). The metabolism of hexachlorobutadiene has not been investigated in humans; however, the demonstrated glutathione pathway of the structurally related haloalkenes tri- and tetrachloroethene in humans indicates that hexachlorobutadiene is metabolized similarly in humans and rodents and has similar renal-specific toxicity.
Hexachlorobutadiene is a selective nephrotoxin in experimental animals, causing damage primarily to the proximal tubules. Renal toxicity is presumed to be due to bioactivation by glutathione S-conjugate formation. Hexachlorobutadiene is conjugated with glutathione to S-(pentachlorobutadienyl)glutathione and further transformed to S-(pentachlorobutadienyl)-l-cysteine, which is N-acetylated in the liver to form N-ac-PCBC. This metabolite accumulates in the kidney, where renal acylases cleave it to S-(pentachlorobutadienyl)-l-cysteine, which is a substrate for renal cysteine conjugate β-lyase and is transformed into a reactive thioketene. Binding of this intermediate to renal macromolecules is probably responsible for the nephrotoxicity of hexachlorobutadiene.
Studies in rats given kidney-specific toxins as single intraperitoneal or subcutaneous injections were conducted to determine the distribution of β-lyase within the nephron. When potassium dichromate was given as a single subcutaneous injection of 25 mg/kg bw, vacuolar degeneration and focal necrosis of the S1–S2 segment of the proximal convoluted tubule were accompanied by significant increases in the activity of β-lyase in the urine. When mercuric chloride was given as a single intraperitoneal dose of 1 mg/kg bw and separate groups of rats were given a single intraperitoneal dose of 100 mg/kg bw hexachlorobutadiene, both chemicals resulted in diffuse necrosis of the S3 portion of the proximal tubule 24 h later. These changes were accompanied by increased urinary β-lyase activity, which also peaked 24 h after administration of either compound. These results show that necrosis in the S1–S2 or S3 portion of the proximal tubules is accompanied by the release of β-lyase into the urine and support the conclusion that β-lyase is distributed throughout the proximal tubules. Although the distribution of necrosis in the rat kidney is more limited than that of β-lyase, the relative contribution of additional factors such as specific uptake systems and the differing concentrations of the second enzyme involved, γ-glutamyl transpeptidase, suggest that the proposed mechanism is consistent with the data (Trevisan et al., 1998).
The activity of γ-glutamyl transpeptidase in human kidney is reported to be approximately 10-fold lower than that in Fischer rat kidney (Lau et al., 1990). In addition, the activity of β-lyase in human kidney has been reported to be a further 10-fold lower than that in rat kidney (Lash et al., 1990). These two factors have important implications with respect to the relative hazard of hexachlorobutadiene to human kidney.
The molecular mechanism of the bioactivation of hexachlorobutadiene that accounts for the kidney-selective tumour induction has been elucidated. The conjugation of hexachlorobutadiene with glutathione, catalysed by hepatic glutathione S-transferase, results in the formation of S-(pentachlorobutadienyl)glutathione, which is eliminated from rat liver with the bile and translocated to the kidney either intact or after metabolism to the corresponding cysteine-S-conjugate. These conjugates are metabolized by renal tubular cysteine conjugate β-lyase to a reactive intermediate, which is most likely a thioacylchloride or a thioketene.
5. Summary of Data Reported and Evaluation
5.1. Exposure data
Exposure to hexachlorobutadiene has occurred principally as a result of its production and release as a by-product from the manufacture of chlorinated solvents and related products. It has been widely detected in ambient air, water, foods and human tissues.
5.2. Human carcinogenicity data
No data were available to the Working Group.
5.3. Animal carcinogenicity data
Hexachlorobutadiene was tested by oral administration in one study in rats, by skin application in mice and inadequately in one experiment in mice by intraperitoneal injection. After oral administration in rats, it produced benign and malignant tumours in the kidneys of animals of each sex. It did not produce skin tumours after repeated application or show initiating activity in a two-stage initiation–promotion study in mice. It enhanced the incidence of renal tubular tumours induced by N-nitrosoethylhydroxyethylamine in a two-stage model of renal carcinogenesis.
5.4. Other relevant data
Hexachlorobutadiene is metabolized exclusively by glutathione conjugation and γ-glutamyl transpeptidase to its corresponding cysteine S-conjugate, S-(1,2,3,4,4-pentachloro-1,3-butadienyl)-l-cysteine, which is concentrated in the renal proximal tubules. This conjugate is partly acetylated to the corresponding mercapturic acid and excreted in urine. It is also a substrate for β-lyases, which have high activity in this part of the nephron and cleave the S-conjugate to produce a reactive thioketene, which can acylate proteins and DNA. This toxification pathway is responsible for the kidney-specific toxicity and carcinogenicity of hexachlorobutadiene in rodents. The metabolism of hexachlorobutadiene has not been investigated in humans; however, the demonstrated formation of mercapturic acids from the structurally related haloalkenes tri- and tetrachloroethylene in humans indicates that hexachlorobutadiene would be metabolized in humans in a manner similar to that in rodents.
In rats exposed during development, fetal growth retardation has been observed, usually at maternally toxic doses. Malformations have not been reported. Negative results were obtained in a short-term assay to screen for teratogenicity in mice exposed by gavage.
No data were available on the genetic and related effects of hexachlorobutadiene in humans or in rodents in vivo. There is weak evidence for its genotoxicity in mammalian cells in vitro. The findings for mutagenicity in bacteria are equivocal.
5.5. Evaluation
There is inadequate evidence in humans for the carcinogenicity of hexachlorobutadiene.
There is limited evidence in experimental animals for the carcinogenicity of hexachlorobutadiene.
Overall evaluation
Hexachlorobutadiene is not classifiable as to its carcinogenicity to humans (Group 3).
6. References
- Agency for Toxic Substances and Disease Registry (1994) ATSDR Toxicological Profiles: Hexachlorobutadiene, Department of Health and Human Services, Public Health Service (CD ROM)
- American Conference of Governmental Industrial Hygienists (1997) 1997 TLVs® and BEIs®, Cincinnati, OH, p. 26.
- Anders M.W., Dekant W., Vamvakas S. Glutathione dependent toxicity. Xenobiotica. 1992;22:1135–1145. [PubMed: 1441604]
- Baggett J.M., Berndt W.O. Renal and hepatic glutathione concentrations in rats after treatment with hexachloro-1,3-butadiene and citrinin. Arch. Toxicol. 1984;56:46–49. [PubMed: 6517712]
- Berndt W.O., Mehendale H.M. Effects of hexachlorobutadiene (HCBD) on renal function and renal organic ion transport in the rat. Toxicology. 1979;14:55–65. [PubMed: 161085]
- Birner G., Werner M., Ott M.M., Dekant W. Sex differences in hexachlorobutadiene biotransformation and nephrotoxicity. Toxicol. appl. Pharmacol. 1995;132:203–212. [PubMed: 7785049]
- Chemical Information Services (1995) Directory of World Chemical Producers 1995/96 Standard Edition, Dallas, TX, p. 556.
- Davis M.E., Berndt W.O., Mehendale H.M. Disposition and nephrotoxicity of hexachloro-1,3-butadiene. Toxicology. 1980;16:179–191. [PubMed: 7423529]
- Dekant W., Vamvakas S., Berthold K., Schmidt S., Wild D., Henschler D. Bacterial β-lyase mediated cleavage and mutagenicity of cysteine conjugates derived from the nephrocarcinogenic alkenes trichloroethylene, tetrachloroethylene and hexachlorobutadiene. Chem.-biol. Interactions. 1986;60:31–45. [PubMed: 3536138]
- Galloway S.M., Armstrong M.J., Reuben C., Colman S., Brown B., Cannon C., Bloom A.D., Nakamura F., Ahmed M., Duk S., Rimpo J., Margolin B.H., Resnick M.A., Anderson B., Zeiger E. Chromosome aberrations and sister chromatid exchanges in Chinese hamster ovary cells: Evaluations of 108 chemicals. Environ. mol. Mutag. 1987;10 Suppl. 10:1–175. [PubMed: 3319609]
- Green T., Odum J. Structure/activity studies of the nephrotoxic and mutagenic action of cysteine conjugates of chloro-and fluoroalkenes. Chem.-biol. Interactions. 1985;54:15–31. [PubMed: 4017103]
- Hardin B.D., Bond G.P., Sikov M.R., Andrew F.D., Beliles R.P., Niemeier R.W. Testing of selected workplace chemicals for teratogenic potential. Scand. J. Work Environ. Health. 1981;7:66–75. [PubMed: 7330632]
- Harleman J.H., Seinen W. Short-term toxicity and reproduction studies in rats with hexachloro-(1,3)-butadiene. Toxicol. appl. Pharmacol. 1979;47:1–14. [PubMed: 425109]
- Haworth S., Lawlor T., Mortelmans K., Speck W., Zeiger E. Salmonella mutagenicity test results for 250 chemicals. Environ. Mutag. 1983;5 Suppl. 1:3–142. [PubMed: 6365529]
- IARC (1979) IARC Monographs on the Evaluation of the Carcinogenic Risk of Chemicals to Humans, Vol. 20, Some Halogenated Hydrocarbons, Lyon, pp. 179–193.
- IARC (1987) IARC Monographs on the Evaluation of Carcinogenic Risks to Humans, Suppl. 7, Overall Evaluations of Carcinogenicity: An Updating of IARC Monographs Volumes 1 to 42, Lyon, p. 64. [PubMed: 3482203]
- International Labour Office (1991) Occupational Exposure Limits for Airborne Toxic Substances, 3rd Ed. (Occupational Safety and Health Series No. 37), Geneva, pp. 216–217.
- Ishmael J., Lock E.A. Nephrotoxicity of hexachlorobutadiene and its glutathionederived conjugates. Toxicol. Pathol. 1986;14:258–262. [PubMed: 3764322]
- Ishmael J., Pratt I., Lock E.A. Hexachloro-1:3-butadiene-induced renal tubular necrosis in the mouse. J. Pathol. 1984;142:195–203. [PubMed: 6707786]
- Jaffe D.R., Hassal C.D., Brendel K., Gandolfi A.J. In vivo and in vitro nephrotoxicity of the cysteine conjugate of hexachlorobutadiene. I. Toxicol. environ. Health. 1983;11:857–867. [PubMed: 6620416]
- Jones T.W., Gerdes R.G., Ormstad K., Orrenius S. The formation of both a mono- and a bis-substituted glutathione conjugate of hexachlorobutadiene by isolated hepatocytes and following in vivo administration to the rat. Chem.-biol. Interactions. 1985;56:251–267. [PubMed: 4075451]
- Kim H.S., Cha S.H., Abraham D.G., Cooper A.J.L., Endou H. Intranephron distribution of cysteine S-conjugate β-lyase activity and its implication for hexachloro 1,3 butadiene induced nephrotoxicity in rats. Arch. Toxicol. 1997;71:131–141. [PubMed: 9049049]
- Kirby G.M., Bach P.H. Enhanced hexachloro-1:3-butadiene nephrotoxicity in rats with a preexisting adriamycin-induced nephrotic syndrome. Toxicol. Pathol. 1995;23:303–312. [PubMed: 7659954]
- Koob M., Dekant W. Biotransformation of the hexachlorobutadiene metabolites 1-(glutathion-S-yl)-pentachlorobutadiene and 1-(cystein-S-yl)-pentachlorobutadiene in the isolated perfused rat liver. Xenobiotica. 1992;22:125–138. [PubMed: 1615703]
- Lash L.H., Nelson R.M., Van Dyke R.A., Anders M.W. Purification and characterization of human kidney cytosolic cysteine conjugate β-lyase activity. Drug Metab. Disposition. 1990;18:50–54. [PubMed: 2139845]
- Lau S.S., Jones T.W., Sioco R., Hill B.A., Pinon R.K., Monks T.J. Species differences in renal γ-glutamyltranspeptidase activity do not correlate with susceptibillity to 2-bromo-(diglutathion-S-yl)-hydroquinone nephrotoxicity. Toxicology. 1990;64:291–311. [PubMed: 1980038]
- Lewis, R.J., Jr (1993) Hawley’s Condensed Chemical Dictionary, 12th Ed., New York, Van Nostrand Reinhold Co., p. 595.
- Lide, D.R., ed. (1997) CRC Handbook of Chemistry and Physics, 78th Ed., Boca Raton, FL, CRC Press, p. 3–88.
- Lock E.A., Pratt I.S., Ishmael J. Hexachloro-1,3-butadiene-induced hydropic change in mouse liver. J. appl. Toxicol. 1985;5:74–79. [PubMed: 3998377]
- Nakagawa Y., Kitahori Y., Cho M., Konishi N., Tsumatani K-I, Ozono S., Okajima E., Hirao Y., Hiasa Y. 1998 Effect of hexachloro-1,3-butadiene on renal carcinogenesis in male rats pretreated with N-ethyl-N-hydroxyethylnitrosamine. Toxicol. Pathol. 26, 361–366. [PubMed: 9608641]
- National Institute for Occupational Safety and Health (1998) National Occupational Exposure Survey (1981–83), Cincinnati, OH.
- National Library of Medicine (1998) Toxic Chemical Release Inventory 1987 and 1996 (TRI87 and TRI96), Bethesda, MD.
- National Toxicology Program (1991) NTP Chemical Repository Data Sheet: Hexachloro-1,3-butadiene, Research Triangle Park, NC.
- Pähler A., Birner G., Ott M.M., Dekant W. Binding of hexachlorobutadiene to α2u-globulin and its role in nephrotoxicity in rats. Toxicol. appl. Pharmacol. 1997;147:372–380. [PubMed: 9439732]
- Rapson W.H., Nazar M.A., Butsky V.V. Mutagenicity produced by aqueous chlorination of organic compounds. Bull. environ. Contam. Toxicol. 1980;24:590–596. [PubMed: 6991030]
- Reichert D., Neudecker T., Spengler U., Henschler D. Mutagenicity of dichloroacetylene and its degradation products trichloroacetyl chloride, trichloroacryloyl chloride and hexachlorobutadiene. Mutat Res. 1983;117:21–29. [PubMed: 6339907]
- Reichert D., Neudecker T., Schütz S. Mutagenicity of hexachlorobutadiene, perchlorobutenoic acid and perchlorobutenoic acid chloride. Mutat. Res. 1984;137:89–93. [PubMed: 6381998]
- Roldán-Arjona T., Garciá-Pedrajas M.D., Luque-Romero F.L., Hera C., Pueyo C. An association between mutagenicity of the Ara test of Salmonella typhimurium and carcinogenicity in rodents for 16 halogenated aliphatic hydrocarbons. Mutagenesis. 1991;6:199–205. [PubMed: 1881351]
- Saillenfait A.M., Bonnet P., Guenier J.P., de Ceaurriz J. Inhalation teratology study on hexachloro-1-3-butadiene in rats. Toxicol. Lett. 1989;47:235–240. [PubMed: 2749768]
- Schiffmann D., Reichert D., Henschler D. Induction of morphological transformation and unscheduled DNA synthesis in Syrian hamster embryo fibroblasts by hexachlorobutadiene and its putative metabolite pentachlorobutenoic acid. Cancer Lett. 1984;23:297–305. [PubMed: 6744254]
- Schrenk D., Dekant W. Covalent binding of hexachlorobutadiene metabolites to renal and hepatic mitochondrial DNA. Carcinogenesis. 1989;10:1139–1141. [PubMed: 2720907]
- Schwetz B.A., Norris J.M., Kociba R.J., Keeler P.A., Cornier R.F., Gehring P.J. Reproduction study in Japanese quail fed hexachlorobutadiene for 90 days. Toxicol. appl. Pharmacol. 1974;30:255–265.
- Stott W.T., Quast J.F., Watanabe P.G. Differentiation of the mechanisms of oncogenicity of 1,4-dioxane and 1,3-hexachlorobutadiene in the rat. Toxicol. appl. Pharmacol. 1981;60:287–300. [PubMed: 7281189]
- Trevisan A., Cristofori P., Fanelli G., Bicciato F., Stocco E. Glutamine transaminase K intranephron localization in rats determined by urinary excretion after treatment with segment specific nephrotoxicants. Arch. Toxicol. 1998;72:531–535. [PubMed: 9765069]
- Vamvakas S., Kordowich F.J., Dekant W., Neudecker T., Henschler D. Mutagenicity of hexachloro-1,3-butadiene and its S-conjugates in the Ames test—role of activation by the mercapturic acid pathway and its nephrocarcinogenicity. Carcinogenesis. 1988a;9:907–910. [PubMed: 3286031]
- Vamvakas S., Müller D.A., Dekant W., Henschler D. DNA-binding of sulfur-containing metabolites from 35S-(pentachlorobutadienyl)-l-cysteine in bacteria and isolated renal tubular cells. Drug Metab. Drug Interactions. 1988b;6:349–358. [PubMed: 3078293]
- Van Duuren B.L., Goldschmidt B.M., Loewengart G., Smith A. C., Melchionne S., Seidman I., Roth D. 1979 Carcinogenicity of halogenated olefinic and aliphatic hydrocarbons in mice. J. natl Cancer Inst. 63, 1433–1439. [PubMed: 292813]
- Verschueren, K. (1996) Handbook of Environmental Data on Organic Chemicals, 3rd Ed., New York, Van Nostrand Reinhold Co., pp. 1070–1072.
- Werner M., Guo Z., Birner G., Dekant W., Guengerich F.P. The sulfoxidation of the hexachlorobutadiene metabolite N-acetyl-S-(1,2,3,4,4-pentachlorobutadienyl)-L-cysteine is catalyzed by human cytochrome P450 3A enzymes. Chem. Res. Toxicol. 1995;8:917–923. [PubMed: 8555406]
- WHO (1993) Guidelines for Drinking Water Quality, 2nd Ed., Vol. 1, Recommendations, Geneva, pp. 73–74.
- WHO (1994) Hexachlorobutadiene (Environmental Health Criteria 156), Geneva, International Programme on Chemical Safety.
- Wild D., Schütz S., Reichert D. Mutagenicity of the mercapturic acid and other S-containing derivates of hexachloro-1,3-butadiene. Carcinogenesis. 1986;7:431–434. [PubMed: 3512118]
- Wolf C.R., Berry P.N., Nash J.A., Green T., Lock E.A. Role of microsomal and cytosolic glutathione S-transferases in the conjugation of hexachloro-1:3-butadiene and its possible relevance to toxicity. J. Pharmacol. exp. Ther. 1984;228:202–208. [PubMed: 6694103]
- Woodruff R.C., Mason J.M., Valencia R., Zimmering S. Chemical mutagenesis testing in Drosophila. V. Results of 53 coded compounds tested for the National Toxicology Program. Environ. Mutag. 1985;7:677–702. [PubMed: 3930237]
- PubMedLinks to PubMed
- Review Chlorothalonil.[IARC Monogr Eval Carcinog Risk...]Review Chlorothalonil.. IARC Monogr Eval Carcinog Risks Hum. 1999; 73:183-93.
- [Clinical hygiene data on working conditions in the use of hexachlorobutadiene on vineyards].[Vrach Delo. 1982][Clinical hygiene data on working conditions in the use of hexachlorobutadiene on vineyards].Burkatskaia EN, Viter VF, Ivanova ZV, Kaskevich LM, Gorskaia NZ. Vrach Delo. 1982 Nov; (11):99-102.
- Review ortho-phenylphenol and its sodium salt.[IARC Monogr Eval Carcinog Risk...]Review ortho-phenylphenol and its sodium salt.. IARC Monogr Eval Carcinog Risks Hum. 1999; 73:451-80.
- [Effect of separate and combined effects of plutonium-239, hexachlorobutadiene and tributyl phosphate on the thymus gland of rats].[Gig Sanit. 1990][Effect of separate and combined effects of plutonium-239, hexachlorobutadiene and tributyl phosphate on the thymus gland of rats].Spirina SS, Chudin VA. Gig Sanit. 1990 Dec; (12):56-8.
- [Long-term consequences of the effects of single inhalation of Pu-239 in association with tributyl phosphate and hexachlorobutadiene in rats].[Radiats Biol Radioecol. 1996][Long-term consequences of the effects of single inhalation of Pu-239 in association with tributyl phosphate and hexachlorobutadiene in rats].Kalmykova ZI, Lemberg VK, Chudin VA. Radiats Biol Radioecol. 1996 Jan-Feb; 36(1):88-93.
- Hexachlorobutadiene - Some Chemicals that Cause Tumours of the Kidney or Urinary...Hexachlorobutadiene - Some Chemicals that Cause Tumours of the Kidney or Urinary Bladder in Rodents and Some Other Substances
- THE MONOGRAPHS - Some Chemicals that Cause Tumours of the Kidney or Urinary Blad...THE MONOGRAPHS - Some Chemicals that Cause Tumours of the Kidney or Urinary Bladder in Rodents and Some Other Substances
- PREAMBLE - Some Chemicals that Cause Tumours of the Kidney or Urinary Bladder in...PREAMBLE - Some Chemicals that Cause Tumours of the Kidney or Urinary Bladder in Rodents and Some Other Substances
- homo sapiens[Organism] AND FOPNL AND (alive[prop]) (4)Gene
Your browsing activity is empty.
Activity recording is turned off.
See more...