NCBI Bookshelf. A service of the National Library of Medicine, National Institutes of Health.
Varki A, Cummings RD, Esko JD, et al., editors. Essentials of Glycobiology. 2nd edition. Cold Spring Harbor (NY): Cold Spring Harbor Laboratory Press; 2009.
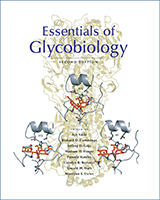
Essentials of Glycobiology. 2nd edition.
Show detailsThe R-type lectins are members of a superfamily of proteins, all of which contain a carbohydrate-recognition domain (CRD) that is structurally similar to the CRD in ricin. Ricin was the first lectin discovered and it is the prototypical lectin in this category. R-type lectins are present in plants, animals, and bacteria, and the plant lectins often contain a separate subunit that is a potent toxin. The structure–function relationships of this group of proteins are discussed in this chapter.
BACKGROUND
In 1888, Peter Hermann Stillmark reported that seed extracts of the poisonous plant Ricinus communis (castor bean) contain a toxin that can agglutinate erythrocytes. He named this agglutinin “ricin.” Other agglutinins were soon discovered in the seed extracts of other species of poisonous plants, and by the middle of the 20th century, these proteins had become important members of the general class of glycan-binding proteins known as “lectins.” As structural studies on ricin proceeded, it was found that the CRD of this lectin is closely related in sequence and three-dimensional structure to the CRDs of a number of other plant lectins. Some of these structural similarities were also noted in a variety of animal and bacterial glycan-binding proteins discussed in this chapter, causing all proteins containing this ricin-type CRD to be classified as R-type lectins (Figure 28.1).

FIGURE 28.1
The R-type lectin superfamily. Different groups within the family are indicated with the domain structures shown.
RICINUS COMMUNIS LECTINS
General Properties
Two different lectins have been purified from R. communis seeds, and in the original nomenclature they were termed RCA-I and RCA-II. RCA-I is an agglutinin but a very weak toxin. RCA-II is commonly called ricin, and it is both an agglutinin and a very potent toxin. The designation RCA-II has now been dropped, but the original name for the agglutinin RCA-I has been retained. The molecular mass of RCA-I is approximately 120 kD and that of ricin is approximately 60 kD.
Ricin is synthesized as a single prepropolypeptide with a 35-amino-acid amino-terminal signal sequence, followed by the A-chain domain, a 12-amino-acid linker region, and the B chain. Proteolysis results in cleavage between the A and B chains. Mature ricin contains four intrachain disulfide bonds and a single disulfide bond links the A chain to the B chain. The A chain contains the catalytic activity responsible for the toxicity and the B chain has the glycan-binding activity. The mature A chain has 267 amino acids and the B chain has 262 amino acids. Each B subunit is a product of gene duplications and has two CRDs, each of which is composed of an ancient 40-amino-acid galactose-binding polypeptide region. RCA-I is a tetramer with two ricin heterodimer-like proteins that are noncovalently associated. Each heterodimer in RCA-I contains an A-chain disulfide linked to the galactose-binding B chain. The sequences of the A chain of ricin and the A chain of RCA-I differ in 18 of 267 residues and are 93% identical, whereas the B chains differ in 41 of 262 residues and are 84% identical. All of the subunits are N-glycosylated and usually express oligomannose-type N-glycans. Because ricin is a multimeric glycoprotein, recombinant forms produced in Escherichia coli (such as ricin B chain) are usually poorly active, and eukaryotic expression systems must be used. The genome of R. communis also encodes several other proteins that have high homology with ricin, and some of these lectins have been designated ricin-A, -B, -C, -D, and -E.
The B chain of ricin has two binding sites for sugars, which are about 35 Å apart. Ricin binds to β-linked galactose and N-acetylgalactosamine, whereas RCA-I prefers β-linked galactose. Indeed, these lectins are often separated from one another by differential elution from galactose-based affinity resins in which ricin is eluted first with N-acetylgalactosamine and then RCA-I is eluted with galactose. The affinities of these lectins for monosaccharides are quite low (Kd in the range 10–3 to 10–4 M). In contrast, the binding to cells is of much higher affinity (Kd in the range 10–7 to 10–8 M), owing to both increased avidity from the multivalency and enhanced binding to glycans terminating in the sequence Galβ1–4GlcNAc-R. Such sequences represent higher-affinity determinants for lectin binding. In general, ricin and RCA-I both preferentially bind to glycans containing nonreducing terminal Galβ1–4GlcNAc-R or GalNAcβ1–4GlcNAc-R, although they will bind weakly to Galβ1–3GlcNAc-R. Neither ricin nor RCA-I binds well to glycoconjugates containing nonreducing terminal α-linked Gal residues. RCA-I is commonly used for glycan isolation and characterization because it is safer than ricin and has higher avidity because of its tetrameric nature. Ricin is often used as a toxin in cell selection for glycosylation mutants, and the A chain of ricin is often used in chimeric proteins as a toxin for specific-cell killing.
R-type Domain
The R-type domain contained in these proteins is the CRD; it is also termed a carbohydrate-binding module (CBM) and has been placed in the CBM13 family in the CAZy database (carbohydrate-active enzymes database) (see Chapter 7). The R-type domain is an ancient type of protein fold that is found in many glycosyltransferases as well as in bacterial and fungal hydrolases. Interestingly, the R-type CRD is the only one conserved between animal and bacterial lectins.
The crystal structure of ricin (Figure 28.2) shows that the A chain has eight α-helices and eight β-strands and it is the catalytic subunit, as discussed above. The B chain, which contains R-type lectin domains, has two tandem CRDs that are about 35 Å apart and have a shape resembling a barbell, with one binding domain at each end. Each R-type domain has a three-lobed organization that is a β-trefoil structure (from the Latin trifolium meaning “three-leaved plant”). The β-trefoil structure probably arose evolutionarily through gene fusion events linking a 42-amino-acid peptide subdomain that has galactose-binding activity. The three lobes are termed α, β, and γ and are arranged around a threefold axis. Conceivably, each lobe could be an independent binding site, but in most R-type lectins only one or two of these lobes retain the conserved amino acids required for sugar binding. Sugar binding is relatively shallow in these loops and arises from aromatic amino acid stacking against the Gal/GalNAc residues and from hydrogen bonding between amino acids and hydroxyl groups of the sugar ligands. A characteristic feature of these loops in the R-type domain in ricin is the presence of (QxW)3 repeats (where x is any amino acid), which are found in many, but not all, R-type family members.

FIGURE 28.2
Ricin and abrin. (a) Photographs showing the leaves and seeds of Ricinus communis and the seeds of Abrus precatorius. R. communis is commonly called the castor-oil plant and the seeds are castor beans. The A. precatorius seeds are sometimes called rosary (more...)
Toxicity of Ricin
Because of its incredible potency as a toxin and its ease of production, ricin has been in the news over the years as a possible lethal agent. The toxicity of ingested ricin is severe and symptoms are initiated after a 2–24-hour latent period following a fatal ingestion. The lethal dose (LD50) of ricin may be as low as 3–5 μg/kg per kilogram body weight, depending on the mode of exposure. Ricin is a type II ribosome-inactivating protein (RIP-II). Although one might predict that RCA-I also would be highly toxic, it has weak activity compared to ricin because it lacks a separate A chain. In contrast, type I ribosome-inactivating proteins (RIP-I), which lack a B subunit, are not lectins and are much less toxic than ricin because of poor ability to enter cells. Examples of RIP-I proteins are saporin, gelonin, and momordin-I.
Ricin binds to cells via interactions with β-linked Gal/GalNAc-containing glycans on the cell surface and is imported into endosomes (Figure 28.3). From there, the protein moves by retrograde trafficking to the endoplasmic reticulum (ER) via the trans-Golgi network and Golgi apparatus. In the ER, the A and B chains separate after reduction of the disulfide bond, perhaps through the action of the ER enzyme, protein disulfide isomerase. Some fraction of the free A chain, which may be partly denatured in the ER, may then escape from the ER by retrotranslocation through the Sec61 translocon and thereby enter the cytoplasm. This retrotranslocation may be through the quality control system in the ER known as ER-associated degradation (ERAD), which is involved in removing misfolded proteins from the ER (see Chapter 36). Some of the A chain may be ubiquitinated and degraded in the proteasome, but the A chain may not be an efficient substrate for ubiquitination because it has few lysine residues.

FIGURE 28.3
Pathway of ricin uptake by cells and the mechanism by which the toxic activity of the A chain in the cytoplasm results in cell death. Symbol Key:
In the cytoplasm, just a few molecules of ricin A chain appear to be sufficient to kill cells. The A chain is an enzyme, RNA N-glycosidase, that cleaves one adenine residue (A4324) in the exposed GAGA tetraloop of the 28S RNA in the eukaryotic 60S ribosomal subunit. This deletion results in a loss of binding of elongation factor-2 and the inability of the ribosome to promote protein synthesis.
OTHER R-TYPE PLANT LECTINS
The toxins abrin, modeccin from Adenia digitata, Viscum album agglutinin (VAA or mistletoe lectin), and volkensin also have R-type domains, belong to the RIP-II class, and kill cells in a manner similar to ricin. There are other R-type plant lectins in the RIP-II class that are not toxic, and these include several proteins from the genus Sambucus (elderberry), such as nigrin-b, sieboldin-b, ebulin-f, and ebulin-r. All of the B subunits of these proteins appear to bind Gal/GalNAc, but they may have some differences in affinity and may recognize different Gal/GalNAc-containing glycoconjugates. Cell lines selected for resistance to killing by modeccin are not resistant to abrin and ricin, and vice versa. The glycan-binding specificity of these lectins should be explored more fully in the future using glycan microarrays and related screening approaches.
In addition to RCA-I and ricin, other plant lectins with R-type domains include the ricin homolog from Abrus precatorius and the bark lectins from the elderberry plant, Sambucus sieboldiana lectin (SSA) and Sambucus nigra agglutinin (SNA). SSA and SNA are unusual in that they are the only R-type lectins that bind well to α2–6-linked sialic acid–containing ligands and they do not bind to α2–3-linked sialylated ligands. SSA and SNA are heterotetramers (~140 kD) composed of two heterodimers each containing an A chain (which resembles ricin A chain) disulfide-bonded to a B chain (which binds glycans and is an R-type lectin). The A chain in these proteins has very weak RIP-II activity in vitro. SSA and SNA may have the same overall organization as RCA-I (see Figure 28.1). Other plant lectins with the R-type domain include lectins from the seeds of Trichosanthes dioica and T. anguina, which also appear to bind β-linked galactose.
Two other plant lectins that can bind to sialylated glycans are from the leguminous tree Maackia amurensis. Although these lectins are discussed here, they do not show a classical R-type domain but instead have an L-type lectin domain (see Chapter 29). M. amurensis leukoagglutinin (MAL) (also referred to commercially as MAA-II) recognizes Siaα2–3Galβ1–4GlcNAcβ-Man-R and does not bind to isomers containing sialic acid in α2–6-linkage. MAL can also bind to sulfated (rather than sialylated) glycans with the sequence sulfate-3Galβ1–4GlcNAcβ-Man-R. Interestingly, the specific sialylated sequences bound by MAL are lacking on human erythrocytes, and hence MAL is a very poor hemagglutinin. However, these sequences are abundant on leukocytes and so the lectin is a strong leukoagglutinin. M. amurensis also contains another lectin termed M. amurensis hemagglutinin (MAH) (also referred to commercially as MAA-I), which shows less specificity than MAL and probably generally binds α2–3-sialylated glycans.
R-TYPE LECTINS IN ANIMALS
The R-type lectin domain is found in several animal lectins, including the mannose receptor (MR) family, discussed in detail below, and in some invertebrate lectins. EW29 is a galactose-binding lectin from the annelid (earthworm) Lumbricus terrestris. EW29 has two homologous domains, an amino-terminal domain and a carboxy-terminal domain, both of which are R-type domains with the three α, β, and γ lobes and both contain the triple-repeated QxW motif. The R-type domain is also found in pierisin-1, which is a cytotoxic protein from the cabbage butterfly Pieris rapae, and in the homologous protein pierisin-2, from Pieris brassicae. Pierisin-1 is a 98-kD protein (comprising 850 amino acids) that exhibits extreme toxicity to animal cells in culture. It has an amino-terminal domain with ADP-ribosylation activity, as found in some bacterial toxins such as cholera and pertussis toxins, and a carboxy-terminal region with four R-type domains and QxW repeats. Through this R-type domain, pierisin-1 binds to glycosphingolipids, including the major ligands globotriaosylce-ramide (Gb3) and globotetraosylceramide (Gb4). It is interesting that both the catalytic domain and the lectin domains are in the same polypeptide. The mechanism by which the catalytic domain enters the cytoplasm is not well understood, but it may involve proteolysis to separate the domains. Tandem R-type motifs are found in some other R-type family members, including ricin, but the presence of four such motifs is unique, thus far, to pierisin-1.
Some proteins with the R-type lectin domain are also enzymes and these are found in both animals and microbes. For example, Limulus horseshoe crab coagulation factor G has a central R-type lectin domain, which is flanked at the amino terminus by a xylanase Z-like domain and at the carboxyl terminus by a glucanase-like domain. This protein also has a subunit that is a serine protease. Some examples of microbial proteins with R-type domains include Streptomyces lividans xylanase 10A, which is discussed in more detail below, and the mosquitocidal toxin (MTX) from Bacillus sphaericus. MTX is a 97-kD ADP-ribosyltransferase, which is activated by proteolytic cleavage releasing the active 27-kD enzyme and a 70-kD carboxy-terminal fragment that contains the R-type domain.
The Mannose Receptor Family
There are four known members of the MR family in humans, all of which contain an R-type lectin domain, and on the basis of a survey of the human genome, no other family members are predicted. The MR family includes the MR, the phospholipase A2 (PLA2) receptor, DEC-205/MR6-gp200, and Endo180/urokinase plasminogen activator receptor-associated protein (Figure 28.4). All of these proteins are large type I transmembrane glycoproteins and they contain a single fibronectin type II domain, multiple C-type lectin domains (CTLDs), and an amino-terminal cysteine-rich domain. Some of the CTLDs in the MR and Endo180 bind glycans, but other CTLDs in these proteins do not bind glycans and have other functions. The CTLDs in DEC-205 have not been shown to bind glycans. Each protein is a recycling plasma membrane receptor with a cytoplasmic domain that mediates clathrin-dependent endocytosis and uptake of extracellular glycan-containing ligands (see Chapter 31). All of the MR family members except DEC-205 recycle back to the cell surface from early endosomes, but DEC-205 recycles from late endosomes. However, each receptor has evolved to have distinct functions and distributions. These receptors are unusual among animal lectins in that they can bind ligands in either a “cis” or “trans” fashion, which means they can bind to cell-surface glycoconjugates on the same cell or to those on other cells and to soluble ligands.

FIGURE 28.4
Members of the mannose receptor family of R-type lectins. These proteins are also in the C-type lectin family and represent a unique group of lectins with more than one type of lectin domain. However, although the mannose receptor and Endo180 can bind (more...)
The Mannose Receptor
The MR (CD206) has important roles in the innate and adaptive immune systems. It is expressed at high levels on hepatic endothelial cells and Kupffer cells as well as on many other endothelial and epithelial cells, macrophages, and immature dendritic cells. The MR is part of the innate immune system and it facilitates the phagocytosis of mannose-rich pathogens. It also assists leukocytes in responding appropriately to antigens by promoting trafficking to the germinal center and is also involved in antigen presentation. The receptor was originally discovered in the 1980s in rabbit alveolar macrophages as a large membrane protein (175 kD) that bound mannose-containing ligands as well as pituitary hormones such as lutropin and thyrotropin, which have 4-O-sulfated N-acetylgalactosamine residues on N-glycans. However, although the MR is a C-type lectin with multiple C-type lectin domains (see Chapter 31), these domains were not required for binding to the sulfated hormones. A 139-residue amino-terminal domain in the mature protein was originally recognized as a cysteine-rich domain, but its importance in binding glycans was not realized until the late 1990s when the sulfated pituitary hormones were found to be cleared from the circulation by binding to the cysteine-rich region. When the cysteine-rich domain was crystallized in a complex with 4-O-sulfated GalNAc, this domain was found to have a β-trefoil structure similar to that reported for ricin B, soybean trypsin inhibitor, fibroblast growth factors, and interleukin-1 (Figure 28.5). Interestingly, the MR and other members of the MR family are among the few mammalian glycan-binding proteins that have two separate lectin motifs (C-type and R-type) in the same molecule. This group is also unusual in that it is the only known lectin group in mammals with more than two C-type lectin domains in the same molecule. Only CTLDs 4 and 5 of the MR have been shown to bind glycans in a Ca++-dependent manner and to bind mannose, N-acetylglucosamine, and fucose.

FIGURE 28.5
Structures of the β-trefoil R-type domains in different proteins. (Top) Cysteine-rich R-type domain of the mannose receptor (MR) in complex with 4-O-sulfated GalNAc; ricin B chain in complex with galactose; and acidic fibroblast growth factor (more...)
The cysteine-rich R-type domain of the MR binds other sulfated glycans and also N-glycans on pituitary glycoprotein hormones containing 4-SO4-GalNAcβ1–4GlcNAcβ1–2Manα1-R. Other ligands for the MR include chondroitin-4-sulfate proteoglycans on leukocytes that also contain 4-SO4-GalNAc β1-R residues and perhaps sulfated glycans,such as those containing 3-O-sulfated galactose, 3-O-sulfated Lex, and 3-O-sulfated Lea. The cysteine-rich R-type domain in the MR binds with relatively low affinity to sulfated monosaccharides (Kd in the range of 10–3–10–4 M), but oligomeric forms of the protein probably display much higher avidity for glycoproteins with multiple sulfated glycans. Interestingly, although the cysteine-rich R-type domain binds sulfated glycans, the C-type lectin domains bind unsulfated glycans and may also play a role in glycoprotein homeostasis and clearance.
The MR is also unusual in that it is the only member of the MR family that can function both in clathrin-dependent endocytosis and in the phagocytosis of nonopsinized microbes and large ligands. The MR can bind many different microorganisms, including Candida albicans, Pneumocystis carinii, Leishmania donovani, Mycobacterium tuberculosis, and Klebsiella pneumoniae. The MR may distinguish self from nonself glycans via its multivalent attachment to the mannose-rich glycoconjugates on these organisms, which are presented in unique clusters. The MR also functions in adaptive immunity through its ability to deliver antigens to major histocompatibility (MHC) class II compartments and through its cleavage and release as a soluble protein into blood. Interestingly, it is speculated that the membrane-bound MR may bind antigens through its C-type lectin domains and then, following proteolytic cleavage, the soluble MR bound to its cargo may move to germinal centers where it may bind via its R-type domain to macrophages and dendritic cells expressing ligands such as sialoadhesion or CD45 that may contain sulfated glycans. The function of the R-type domain in the MR may be unique because DEC-205 does not bind lutropin and sequence comparisons predict that neither the phospholipase A2 (PLA2) receptor nor Endo180 will bind sulfated glycans.
The Phospholipase A2 Receptor
The PLA2 receptor was discovered as a receptor for phospholipase A2 neurotoxins in snake venoms and was referred to as the M-type PLA2 receptor to distinguish it from the neuronal or N-type PLA2 receptor. The cDNA encoding this 180-kD glycoprotein has homology with the MR (with 29% identity). It can occur as both a long form that is a type I trans-membrane glycoprotein with a domain structure like the MR and a shorter form that is secreted. The membrane-bound form can function as an endocytic receptor to internalize phospholipase A2 ligands. In animal cells, secreted phospholipase A2s represent a large family of enzymes that are important in the degradation of phospholipids and the release of arachidonic acid, which is the precursor for prostaglandins, leukotrienes, thromboxanes, and prostacyclins. The murine PLA2 receptor binds to sPLA2-X enzyme, but the human PLA2 receptor may bind to several different phospholipase A2 isozymes. Although early studies suggested that the PLA2 receptor might be involved in clearance of phospholipase A2s, murine knockouts for the PLA2 receptor had the unusual phenotype of resistance to endotoxic shock. This suggested that the PLA2 receptor might be important in regulating production of proinflammatory cytokines by soluble phospholipase A2s. Thus, the PLA2 receptor might function in signal transduction mediated by phospholipase A2 binding. Some of the C-type lectin domains in the PLA2 receptor function by binding phospholipase A2 ligands, rather than binding directly to glycan ligands. The fibronectin type II domain binds to collagen, which is a feature shared by this domain in other MR family members, except for possibly DEC-205.
DEC-205
DEC-205 is a 205-kD member of the MR family that is expressed by dermal dendritic cells and, at a lower level, by epidermal Langerhans cells. It is now designated as CD205. It is also expressed on some epithelial cells, on bone marrow stroma, and by endothelial cells. It was identified originally in the 1980s by a monoclonal antibody NLDC-145, which recognized a surface antigen on Langerhans cells. Subsequent cloning of the gene revealed its relationship to the MR and its unique characteristic of having ten tandem C-type lectin domains, rather than the eight found in other MR family members. DEC-205 is important in the recognition and internalization of antigens for presentation to T cells. Upon endocytosis, DEC-205 internalizes to late endosomes/lysosomes and recycles to the surface. Expression of DEC-205 is enhanced in both types of cells upon cell maturation induced by inflammatory stimuli. None of the ten CTLDs in DEC-205 have the conserved amino acids known to be important in carbohydrate binding, and thus far there is no evidence that these domains bind glycans. There is also no evidence that the cysteine-rich R-type domain at the amino terminus binds to glycan ligands.
Endo180
The protein now known as Endo180 was discovered independently by several groups. It was found to be part of a trimolecular cell-surface complex with urokinase plasminogen activator (uPA) and its receptor (uPAR) and was termed the UPAR-associated protein or UPARAP. It was also discovered as a novel antigen on macrophages and human fibroblasts. Like the MR, Endo180 is expressed on macrophages, but Endo180 is also expressed on fibroblasts and chondrocytes, some endothelial cells, and tissues undergoing ossification. The cysteine-rich R-type domain at the amino terminus of Endo180 has been shown to be unable to bind sulfated glycans. The function of this domain is unknown. Endo180 is predicted to be important in remodeling of the extracellular matrix. It binds to the carboxy-terminal region of type I collagen, and collagens type II, IV, and V, through its FN II domain, and binds to uPA and uPAR, but whether glycan recognition is important for these interactions is not known. Endo180 (CD280) binds in a Ca++-dependent manner to N-acetylglucosamine, mannose, and fucose through CTLD2, and this is the only domain that contains all of the conserved amino acids for binding both Ca++ and sugar. Endo180 is an endocytic receptor for these soluble ligands and may be important in matrix turnover. In addition, cells expressing Endo180 demonstrate enhanced adhesion to matrixes, suggesting that Endo180 may also be important in cell adhesion.
UDP-GalNAc:Polypeptide α-N-Acetylgalactosaminyltransferases
Mucin-type O-glycans have the common core structure of GalNAcα1-Ser/Thr (Figure 28.6), which may be further modified by addition of galactose or N-acetylglucosamine residues (see Chapters 9 and 13). The biosynthesis of the core structure is dependent on a family of UDP-GalNAc:polypeptide α-N-acetylgalactosaminyltransferases (ppGalNAcTs) that function in the Golgi apparatus. Such enzymes are found in all animals. More than a dozen such enzymes have been characterized, and animal genomes encode many potential ppGalNAcTs, including 24 potential ppGalNAcTs in humans and 14 in Drosophila (see Chapter 9). These ppGalNAcTs may be separated into two general classes: those that can transfer N-acetyl-galactosamine from UDP-GalNAc to unmodified polypeptide acceptors, and those that prefer acceptor glycopeptides containing GalNAc-Ser/Thr (i.e., a set predicted to have a CRD). Cloning of the genes encoding these enzymes revealed that they are large proteins and have a unique multidomain structure. The enzymes are all type II transmembrane proteins and have a carboxy-terminal R-type domain of about 130 amino acid residues and an amino-terminal catalytic domain. The R-type domain has the QxW repeat, and recent evidence shows that these domains in the ppGalNAcTs function as lectins during the catalytic process.

FIGURE 28.6
Structure and function of UDP-GalNAc:polypeptide α-N-acetylgalactosaminyltransferases (ppGalNAcTs). (a) The activity of the ppGalNAcT is shown using an acceptor peptide with Ser and Thr residues and UDP-GalNAc as the donor. Some of the ppGalNAcTs (more...)
The first crystal structure for this family was determined for the murine ppGalNAcT, termed mT1. This enzyme does not require the R-type domain for catalysis with either glycopeptide (GalNAc-peptide) or peptide acceptors. In contrast, in human hT2, the lectin domain is important in catalysis with GalNAc-peptide acceptors, but not peptide acceptors, and the lectin domains of both hT2 and hT4 can directly bind N-acetylgalactosamine. In mT1, the R-type domain has the β-trefoil structure and the three-lobe repeating α, β, and γ loops. Mutations in the repeats can abolish binding to GalNAc-peptide acceptors. The ppGalNAcTs are the only known glycosyltransferases that have a lectin and catalytic domain conjoined. The available models suggest that each of the ppGalNAcTs has a somewhat different peptide or glycopeptide acceptor specificity, allowing the assortment of enzymes to be highly efficient at adding N-acetylgalactosamine to a tremendous variety of polypeptide substrates, including long mucin polypeptides of thousands of amino acids, and to specific single serine or threonine residues on membrane and secreted glycoproteins. The presence of the two domains in these enzymes and the multiple family members may promote an efficient processive activity and association of these enzymes with acceptor proteins.
MICROBIAL R-TYPE PROTEINS: STREPTOMYCES LIVIDANS ENDO-β1–4XYLANASE 10A
A feature of many microbial glycosidases is the presence of both a catalytic domain and a CBM. S. lividans endo-β1–4xylanase 10A (Xyn10A) is a good example of such an enzyme. Xyn10A catalyzes the cleavage of β1–4xylans and can bind to xylan and a variety of small soluble sugars, including galactose, lactose, and xylo- and arabino-oligosaccharides. The catalytic domain is at the amino terminus and the carboxyl terminus has an R-type β-trefoil motif. As mentioned above, the R-type domain CBM represents the CBM13 family in the CAZy database. In Xyn10A, all of the original β-trefoil sugar-binding motifs are retained, along with the conserved disulfide bridges, and evidence suggests that each of the three potential sugar-binding sites in the β-trefoil structure interact with sugars and each site may span up to four xylose residues. The binding to monosaccharides is very weak (Kdi n the range of 10–2 to 10–3 M), but multivalent binding to polysaccharides can be of very high affinity.
FURTHER READING
- Stirpe F, Barbieri L, Battelli MG, Soria M, Lappi DA. Ribosome-inactivating proteins from plants: Present status and future prospects. Biotechnology. 1992;10:405–412. [PubMed: 1368484]
- Lord JM, Roberts LM, Robertus JD. Ricin: Structure, mode of action, and some current applications. FASEB J. 1994;8:201–208. [PubMed: 8119491]
- Clausen H, Bennett EP. A family of UDP-GalNAc: Polypeptide N-acetylgalactosaminyl-transferases control the initiation of mucin-type O-linked glycosylation. Glycobiology. 1996;6:635–646. [PubMed: 8922959]
- Hazes B. The (QxW)3 domain: A flexible lectin scaffold. Protein Sci. 1996;5:1490–1501. [PMC free article: PMC2143495] [PubMed: 8844840]
- Gabius HJ. Animal lectins. Eur J Biochem. 1997;243:543–576. [PubMed: 9057819]
- Amado M, Almeida R, Schwientek T, Clausen H. Identification and characterization of large galactosyltransferase gene families: Galactosyltransferases for all functions. Biochim. Biophys. Acta. 1999;1473:35–53. [PubMed: 10580128]
- Dodd RB, Drickamer K. Lectin-like proteins in model organisms: Implications for evolution of carbohydrate-binding activity. Glycobiology. 2001;11:71R–79R. [PubMed: 11425795]
- Rudiger H, Gabius HJ. Plant lectins: Occurrence, biochemistry, functions and applications. Glycoconj J. 2001;18:589–613. [PubMed: 12376725]
- Woodworth A, Baenziger JU. The Man/GalNAc-4-SO4-receptor has multiple specificities and functions. Results Probl Cell Differ. 2001;33:123–138. [PubMed: 11190671]
- East L, Rushton S, Taylor ME, Isacke CM. Characterization of sugar binding by the mannose receptor family member, Endo180. J Biol Chem. 2002;277:50469–50475. [PubMed: 12399458]
- Ten Hagen KG, Fritz TA, Tabak LA. All in the family: The UDP-GalNAc:polypeptide N-acetylgalactosaminyltransferases. Glycobiology. 2003;13:1R–16R. [PubMed: 12634319]
- Sharon N, Lis H. History of lectins: From hemagglutinins to biological recognition molecules. Glycobiology. 2004;14:53R–62R. [PubMed: 15229195]
- Llorca O. Extended and bent conformations of the mannose receptor family. Cell Mol Life Sci. 2008;65:1302–1310. [PubMed: 18193159]
- Review R-Type Lectins.[Essentials of Glycobiology. 2015]Review R-Type Lectins.Cummings RD, L. Schnaar R. Essentials of Glycobiology. 2015
- Review R-Type Lectins.[Essentials of Glycobiology. 2022]Review R-Type Lectins.Cummings RD, Schnaar RL, Ozeki Y. Essentials of Glycobiology. 2022
- Carbohydrate recognition factors of the lectin domains present in the Ricinus communis toxic protein (ricin).[Biochimie. 2006]Carbohydrate recognition factors of the lectin domains present in the Ricinus communis toxic protein (ricin).Wu JH, Singh T, Herp A, Wu AM. Biochimie. 2006 Feb; 88(2):201-17. Epub 2005 Aug 18.
- [Chimeric toxin of the A-subunit of viscumin and the B-subunit of ricin].[Mol Biol (Mosk). 1994][Chimeric toxin of the A-subunit of viscumin and the B-subunit of ricin].Tonevitskiĭ AG, Toptygin AIu, Agapov II, Shamshiev AT, Pfuller U, Ershova GV, Prokof'ev SA, Rakhmanova VA. Mol Biol (Mosk). 1994 May-Jun; 28(3):574-9.
- Structure-function inferences based on molecular modeling, sequence-based methods and biological data analysis of snake venom lectins.[Toxicon. 2006]Structure-function inferences based on molecular modeling, sequence-based methods and biological data analysis of snake venom lectins.Abreu PA, Albuquerque MG, Rodrigues CR, Castro HC. Toxicon. 2006 Nov; 48(6):690-701. Epub 2006 Aug 17.
- R-type Lectins - Essentials of GlycobiologyR-type Lectins - Essentials of Glycobiology
Your browsing activity is empty.
Activity recording is turned off.
See more...