NCBI Bookshelf. A service of the National Library of Medicine, National Institutes of Health.
Varki A, Cummings RD, Esko JD, et al., editors. Essentials of Glycobiology. 2nd edition. Cold Spring Harbor (NY): Cold Spring Harbor Laboratory Press; 2009.
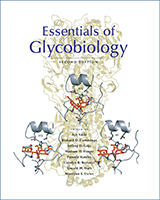
Essentials of Glycobiology. 2nd edition.
Show detailsThis chapter discusses unique aspects of glycans in model organisms that belong to the deuterostome lineage, with particular emphasis on sea urchins, frogs, zebrafish, and mice. These organisms provide models for studying the function of glycans in development and physiology.
EVOLUTIONARY BACKGROUND
Animal evolution split into two major lineages approximately 600 million years ago: the deuterostomes and the protostomes. The superphylum Deuterostomia contains the major phyla of Echinodermata (sea urchins and starfish), Hemichordata (acorn worms), Urochordata (ascidians), Cephalochordata (amphioxus), and Vertebrata (fish, amphibia, reptiles, birds, and mammals). In deuterostomes, cell divisions of the zygote occur by radial cleavage, and cell fates are not precisely determined. Another characteristic feature is that the first opening to form in the blastula becomes the anus and the second opening becomes the mouth. The other superphylum, the Protostomia, contains the major phyla of Porifera (corals and sponges), Cnidara (anemones and hydra), Annelida (segmented worms), Mollusca (clams, oysters, snails, and slugs), and Arthropoda (insects, spiders, and crustacea). In protostomes, unlike deuterostomes, cell division during early development occurs in a highly organized manner and cell fate is precisely determined. Examples of protostome model organisms include the nematode Caenorhabditis elegans (see Chapter 23) and the arthropod Drosophila melanogaster (see Chapter 24).
Much effort has been devoted to studying the deuterostomes because of their relatedness to humans and because they provide excellent model systems for studying vertebrate biology. In particular, sea urchins, frogs, zebrafish, and mice have received the most attention. Each of these systems provides certain advantages for studying the function of glycans (e.g., in fertilization, early development, or adult physiology). In some cases, these models have revealed aspects of glycobiology that were later confirmed in more evolutionarily advanced organisms, and they often serve as models for studying human disease. Each of these models is briefly described below with reference to other sections of the book for additional details.
SEA URCHINS
Sea urchin glycans that are involved in fertilization have been studied extensively. In general, most of what we know about the biochemistry of fertilization was first discovered in this organism (Figure 25.1), and subsequently the information was applied to mammalian sperm–egg interaction. One of the advantages of studying fertilization in sea urchins is that eggs and sperm are easy to obtain in large quantities. Because fertilization occurs outside the adult body, it is easy to manipulate sperm–egg interaction.

FIGURE 25.1
(Left) The purple sea urchin Strongylocentrotus purpuratus. (Reprinted, with permission, courtesy of Charles Hollahan, Santa Barbara Marine Biologicals.) (Right) Sperm binding to a sea urchin egg. (Reprinted, with permission, courtesy of M. Tegner and (more...)
Egg Glycans and Fertilization
Sea urchin eggs are covered by a hydrated jelly coat. About 80% of the weight of egg jelly is a high-molecular-weight linear fucose sulfate polymer (FSP), which has a molecular mass of more than 106 daltons. The FSP binds to receptor proteins on the sperm, triggering the opening of two pharmacologically distinct calcium channels that induce the exocytosis of the sperm’s acrosome vesicle (the “acrosome reaction”). Additionally, exiting H+ raises the internal pH of the sperm from 7.50 to 7.75, activating the polymerization of actin to form a finger-like acrosomal process, which fuses with the egg, uniting the two cells into one cell. The ionic mechanisms that trigger the acrosome reaction are conserved in mammals, but the nature of the sperm surface receptors varies. Blocking the acrosome reaction in mammals has long been a target for nonhormonal contraception.
FSP is a species-selective inducer of the sperm acrosomal reaction. FSP has no associated protein with only one exception (Strongylocentrotus droebachiensis) and consists of linear α1–3-linked fucose polymers. The individual fucose residues are sulfated differently, so that most FSPs are made of tri- or tetrasaccharide repeats. The number of fucose residues per repeat, the linkage, and sulfation patterns all help ensure species selectivity for inducing the acrosome reaction (Figure 25.2). For instance, the α1–3-linked polymer of S. franciscanus is sulfated only at C-2, whereas S. pallidus has an α1–3 tetrafucosyl repeat, with site-specific C-2 and C-4 sulfate groups. Other variations are also known (e.g., the egg jelly of Echinometra lacunter contains a sulfated galactan).

FIGURE 25.2
Fucose sulfate polymers. Structures of sulfated α-fucans and a sulfated α-galactan from sea urchin egg jelly. Shown are eight fully characterized structures of sulfated polysaccharides from the egg jellies of seven species of sea urchins. (more...)
Glycan-binding proteins are important for sea urchin fertilization. FSP binds to at least one sperm surface receptor (REJ1; receptor for egg jelly-1) that is located on the sperm plasma membrane over the acrosome vesicle. REJ1 is a 1450-amino-acid protein with two C-type lectin domains in its amino-terminal region (see Chapter 31).
About 20% of the egg jelly mass is a large glycoprotein containing a unique form of polysialic acid (sialoglycan), which can be purified from crude sea urchin egg jelly by β-elimination. Sialoglycan potentiates the acrosome reaction induced by FSP, possibly by regulating sperm pH. Treating sialoglycan with neuraminidase or mild periodate oxidation completely destroys its biological activity. Studies on two closely related sea urchin species show that the polysialic acid is attached to protein backbones of very different sizes, suggesting that the protein components are encoded by different genes. The sialoglycan has a novel structure—[Neu5Gcα2–5-O-glycolylNeu5Gc]n, but its receptor on the sperm membrane is unknown.
When sea urchin sperm undergo the acrosome reaction, a protein named bindin is released from the acrosomal vesicle that cements the sperm to a large egg surface glycoprotein named EBR1. Bindin can agglutinate mammalian red blood cells much like plant lectins (see Chapter 29), and glycopeptide fragments of unfertilized eggs can block bindin-induced red cell agglutination. Bindin is thought to recognize glycans on EBR1. EBR1 itself has lectin-like domains, but its ligands are not known.
Mammalian fertilization shares many features with the sea urchin system. All mammalian eggs are enclosed within an extracellular matrix called the zona pellucida (ZP). The ZP is made of three heavily glycosylated mucin-like proteins, termed ZP1, ZP2, and ZP3. O-linked glycans of ZP3 are recognized by sperm membrane proteins and bind the sperm loosely to the egg in a lectin-type interaction. Not only are the glycans of ZP3 involved in binding sperm to the ZP, but they also induce the acrosome reaction. After the acrosome reaction, binding of ZP2 to the sperm’s inner acrosomal membrane enhances the binding of the sperm to the ZP in another lectin-like interaction. Two clusters of residues on ZP3 contain O-linked glycans that show species selectivity in sperm–egg binding and induction of the acrosome reaction. The mammalian sperm protein strongly implicated in binding ZP3 is called zonadhesin, and it has several domains that could be involved in lectin-like binding to ZP3 glycans.
Lectins
Sea urchin eggs contain other lectins of unknown function. A 12-kD galactose-binding lectin called SUEL can be purified from eggs and is present in all developmental stages of sea urchin embryos. It is stored in secretory granules in the cortex region of the embryonic cells and is released by exocytosis. Much of SUEL is embedded in the extracellular hyaline layer gel that surrounds the embryo, where it may have a role in cell–cell adhesion or in binding microbes attempting to colonize the embryo. The surface of the unfertilized sea urchin egg also contains a 350-kD heavily O-glycosylated protein that has lectin activity for sialic acid. This lectin also binds sperm gangliosides in detergent-insoluble lipid rafts, suggesting that it might be involved in signal transduction in sperm.
Echinonectin is a 230-kD extracellular matrix glycoprotein also found in the sea urchin embryo hyaline layer (a clear Ca++-requiring gel about 3 μm in diameter that surrounds the embryo). This lectin recognizes galactose and fucoidan and shows differential affinity for different types of embryonic cells. Echinonectin probably functions in cell adhesion during early embryogenesis. Loss of affinity for echinonectin might have a role in the movement of cells into the blastocoel cavity during early gastrulation.
Adult sea urchins have a powerful innate immune system to prevent microbial infection. Sea urchins, like mammals, have a true body cavity filled with phagocytes and other types of defense cells. The body cavity fluid contains lectins that bind to invading microorganisms, causing the foreign cells to aggregate and be more easily bound to and ingested by the phagocytes. Thus, lectins are used in sea urchins and all other invertebrates (whether aquatic or terrestrial) to facilitate capture and destruction of any foreign cell or particle.
Adult sea urchins have hundreds of three-jawed, pincer-like structures called pedicellaria, which are located over their entire outer surface where they are used for defense. Some species have very large pedicellaria associated with a venom gland that can cause painful stings to humans handling these animals. Lectins have been purified from pedicellaria of the species Toxopneustes pileolus and T. gratilla. SUL-1 and -2 are galactose-recognizing lectins from T. pileolus, and TGL-1 is a calcium-dependent, heparin-binding lectin from T. gratilla. One lectin, purified from T. pileolus, binds galactose and d-fucose (but not the more commonly seen l-fucose); it will also block histamine release from rat mast cells.
FROGS
The African clawed frog, Xenopus laevis, is a well-established model organism for studying fertilization and embryonic development (Figure 25.3). Adults are easy to maintain and can be induced to lay eggs up to three times a year by injection of human chorionic gonadotropin. The very large eggs make microinjection experiments easy and allow them to be used as an expression system. The embryos also develop quickly. However, the generation time is long (1–2 years) and the genome is tetraploid, making genetic studies difficult. Nevertheless, interesting insights into glycan signaling have recently emerged from studies of chitin formation in Xenopus and a new class of lectins has been discovered.

FIGURE 25.3
Xenopus laevis. (Left) An adult specimen of the African clawed frog. (Image courtesy of Bruce Blumberg, University of California, Irvine.) (Right) Embryonic development from the neurula stage until just prior to hatching, about 24 hours postfertilization. (more...)
DG42, Hyaluronan, and Chitin Oligosaccharide Synthesis
The DG42 gene has homology with fungal chitin synthase and a bacterial Rhizobium NodC gene, which makes short chitin oligomers (GlcNAcβ1–4GlcNAc)n. These products and some of their modified derivatives are required for Rhizobium to invade plant root hairs and produce a nodule for nitrogen fixation (see Chapter 37). DG42 is expressed in Xenopus endoderm cells briefly during mid-late gastrulation. Transgenic expression of DG42 results in chitin oligomers that are digestible by chitinase, which is taken as evidence that vertebrates, at least vertebrate embryos, can synthesize short chitin oligosaccharides. However, DG42 also has considerable homology with the hasA gene from Streptococcus, which is responsible for hyaluronan synthesis (GlcAβ1–3GlcNAcβ1–4)n (see Chapter 15). Expression of DG42 in several cell lines and in yeast (which does not make hyaluronan) showed that the expressed gene increased the synthesis of hyaluronan in those cells. In fact, all mammalian hyaluronan synthase genes isolated and expressed to date have considerable homology with DG42. Thus, by homology and initial biochemical characterization, there is evidence that DG42 can assemble both chitin and hyaluronan. One idea is that chitin oligosaccharides could act as a primer for the assembly of hyaluronan, but supporting data are lacking.
A New Family of Lectins in Xenopus
X. laevis oocyte cortical granules contain a lectin called XL35, which is released at fertilization and binds to mucin-type O-glycans in the egg jelly. This complex forms a layer on the fertilization envelope and blocks polyspermy. Other species such as lamprey, trout, and sea squirts have related proteins, as do humans (HL-1 and HL-2) and mice (interlectin-1 and inter-lectin-2). Other homologous sequences exist as well. XL35 binds to melibiose (a disaccharide consisting of one galactose and one glucose moiety in an α1–6 linkage) and requires calcium, but it does not have the typical sequence motif for C-type lectins (see Chapter 31). Thus, XL35 and related proteins appear to represent a new family of lectins. Although the specific glycan-binding properties of family members differ, they have high degrees of amino acid sequence homology with a common fibrinogen-like motif that may involve glycan binding. Several studies on this new family of lectins suggest that they are stored in specialized vesicles and released by pathogen infections. Some of these proteins bind to bacterial pathogen glycans, suggesting that they may be used for pathogen surveillance by the innate immune system.
ZEBRAFISH
Zebrafish (Danio rerio) provide an excellent genetic model organism for understanding the function of genes in early vertebrate development (Figure 25.4). Females lay several hundred eggs weekly and the eggs undergo external fertilization. Embryos develop rapidly (in hours), and their translucence allows visualization of early development. Zebrafish are relatively easy to maintain compared to other vertebrate species. Furthermore, they can be mutagenized and outbred to produce mutants, or studied using morpholinos to silence genes transiently during early development (gene “knockdowns”).

FIGURE 25.4
Zebrafish (Danio rerio). (Reprinted, with permission, from Sprague J., Bayraktaroglu L., Clements D., et al. 2006. The Zebrafish Information Network: The zebrafish model organism database. Nucleic Acids Res. 34: D581–D585.)
When the first edition of this book was published in 1999, there was only brief mention of zebrafish and only a handful of papers had been published on glycans in this species. Since then, all of the major glycan classes present in mammals have been described in zebrafish and many of them have been manipulated genetically, yielding fresh insights into glycan function (Table 25.1). Because zebrafish live in a nonterrestrial environment and because widespread gene duplications and diversification occurred during teleost evolution, one can predict that novel glycans, pathways of assembly, and biological activities may exist.
TABLE 25.1
Examples of zebrafish glycans under study
Zebrafish Lectins
In addition to the major families of glycans, various orthologs of mammalian lectins have been described in zebrafish. These include all three major galectin types: proto, chimera, and tandem-repeat (see Chapter 33). Zebrafish galectins exhibit distinct patterns of temporal expression during embryo development, suggesting that they have important roles in different tissues. With regard to Siglecs, the only unambiguous ortholog to mammalian molecules corresponds to Siglec-4 (see Chapter 32). Like its mammalian counterparts, zebrafish Siglec-4 is only present in the nervous system, but its function has not yet been established. Zebrafish also express both hyaluronan and hyaluronan-binding proteins, such as members of the aggrecan family (see Chapter 16). Dermacan is distinct from any other identified lecticans and has a restricted pattern of expression. Silencing of dermacan in zebrafish embryos results in defective craniofacial dermal bone development.
Novel Zebrafish Genes
Zebrafish also allow development of sophisticated screening methods for the identification of novel mutants that affect specific physiological systems. For example, high-throughput screenings have identified groups of genes required for fin development and regeneration, which serve as models for cartilage and bone development in mammals. Mutants altered in vascular development and hemostasis have been identified as well. As the candidate genes are identified and cloned, a number of these will undoubtedly encode glycan-synthesizing enzymes or lectins. The ability to identify novel genes in the system and manipulate glycan expression via silencing methods should make this model organism a focus of future studies in vertebrate glycobiology.
MICE (MUS MUSCULUS)
In recent years, mice (Mus musculus) have become the most studied deuterostome because they are mammals, they reproduce quickly, they have manageable genetics, and their genes can be functionally deleted (gene knockouts). Another advantage is that highly inbred mouse strains exist and spontaneous mutations occur that have generated specific morphological or pathophysiological phenotypes. Examples include brachymorphic mice, which are partially deficient in the synthesis of PAPS, the universal sulfate donor (see Chapter 4), and have shortened limbs because of defective chondroitin sulfate synthesis during endochondral ossification (see Chapter 16). Another example is a mutation that causes a muscular dystrophy that was eventually traced to Largemyd, a putative glycosyltransferase that when mutated in humans causes muscular dystrophy because of abnormal synthesis of O-mannose-linked glycan on α-dystroglycan (see Chapters 12 and 42). The majority of information about mouse glycobiology comes from the use of homologous recombination methods to knock out a specific gene systemically, in specific tissues or at selected times in development. In a few cases, human pathological mutations that represent hypomorphic alleles have been engineered into their conserved murine homologs in an attempt to generate models for disease. Table 6.1 lists a sampling of defects based on the phenotype and the organs or systems most affected by the mutations. Not surprisingly, many of these mutations impact multiple systems.
Mutations That Affect Entire Pathways
Because homologous recombination has been used to inactivate numerous genes involved in glycosylation, only a few mutants are mentioned here. Mutant lines have been created in early genes involved in the assembly of all the major subclasses of vertebrate glycans. In most cases, these mutations prevent initiation of the biosynthetic pathways and show embryonic lethality. These include the single O-GlcNAc transferase (see Chapter 18), which is lethal even at the single-cell stage. Loss of the entire N-linked pathway caused by ablation of phosphomannomutase 2 (which generates mannose-1-P and all mannose-containing glycans) and loss of GlcNAc-1-P transferase (which initiates the lipid-linked oligosaccharide precursor) are lethal within a few days of fertilization. Loss of heparan sulfate biosynthesis due to deletion of Ext1 or Ext2 is also lethal at the E6–7 stage because of a failure to differentiate mesoderm. Systemic deletion of the N-glycan-processing enzyme MgatI is lethal around mid-development (E9–10) showing that early development through gastrulation can proceed in the complete absence of hybrid or complex glycans. Chondroitin sulfate and hyaluronan synthesis are essential as well, although developmental arrest occurs during limb generation and organogenesis. Because there are more than 20 partially redundant polypeptide N-acetylgalactosaminyl transferases in mouse, knocking out this pathway completely has not been possible. However, deletion of the single copy core-1 galactosyltransferase (T-synthase, which generates Galβ1–3GalNAcα-O-Ser/Thr) is lethal by stage E14 because of deranged and distorted angiogenesis. Knocking out the O-fucosyltransferase that modifies epidermal growth factor (EGF) modules in Notch is lethal and causes developmental defects similar to those seen in Notch1 knockout mice. Absence of glycosylphosphatidylinositol anchors or O-mannose-based glycans (α-dystroglycan) also results in embryonic lethality.
Mutations That Affect Specific Extensions or Modifications
Mutations in genes that encode glycosyltransferases used to extend the basic glycan chains usually have less severe phenotypes than those that delete entire pathways. Chapters 8–18 describe the effects of mutations on selected classes of glycans or specific modifications found on multiple types of glycans. For example, Chapters 13 and 14 discuss the consequences of gene knockouts that affect the immune system, including leukocyte rolling, lymphocyte homing, and T-cell homeostasis. Knocking out sequential biosynthetic steps or multiple, seemingly redundant, genes frequently reveals another layer of glycan-dependent functions, novel structures, and unrecognized biosynthetic pathways. Many mouse mutants serve as models of human genetic disorders (see Chapters 41 and 42).
Tissue-specific Mutations
To study the function of glycans in specific tissues, tissue-specific lesions in a gene can be created by crossing strains bearing conditional null alleles to strains that selectively delete the gene only in those tissues of interest. The most common method is to flank a portion of the gene with loxP recombination sites and then mate these mice to a strain that produces bacteriophage Cre-recombinase under the control of a tissue-specific or inducible promoter (Figure 25.5). When activated, the Cre-recombinase recognizes the loxP sites and excises the DNA segment contained between them. These techniques can be combined with an environmental stress, dietary modification, or inflammatory challenge to delete genes in both a tissue-specific and temporally regulated fashion.

FIGURE 25.5
Mus musculus. (Top) An adult mouse. (Bottom) Cre-loxP targeting for making conditional knockouts.
Sometimes, altering a gene can have a highly specific effect on a given tissue without affecting other tissues because of the unforeseen presence of related isozymes. α-ManII was thought to have the dominant role in N-glycan processing (see Chapter 8), but α-ManII knockouts survive and have a dyserythropoietic anemia. These mice produce many unusual glycans from the abnormally high levels of incomplete precursors that accumulate and drive the production of other atypical products. They eventually develop a progressive autoimmune nephropathy and accumulate immune complexes in the kidney tubules, probably in response to the abnormal glycan antigens. Their erythrocytes lack complex N-glycans, but other tissues continue to produce them using a different isozyme called α-ManIIx. α-ManIIx-null mice are mostly normal, except that male mice are sterile because spermatocytes fail to make a glycan required for their binding to Sertoli cells in the testes, causing nonfunctional immature spermatocytes to be released into the epididymis. Knock out of both α-ManII and α-ManIIx prevents synthesis of all complex glycans, and most embryos die between E15 and E18 or shortly after birth from respiratory failure.
Strain Differences and Genetic Modifiers
Determining the effect of a gene knockout is usually done in highly inbred strains that are genetically identical except for the gene in question. Sometimes, the phenotype of a mutation changes according to the genetic background (strain) in which the mutation is expressed. For instance, inactivating the N-glycan-processing gene Mgat2, which encodes GlcNAcT-II, is uniformly lethal after birth in one background, but placing it in a different background generates rare survivors with severe gastrointestinal, hematological, and osteogenic abnormalities. Interestingly, these mutants phenocopy the human disorder, CDG-IIa, in which patients lack the same enzyme (see Chapter 42). As another example, lines deficient in global fucosylation due to mutations in the enzyme that converts GDP-mannose to GDP-fucose (see Chapter 4) die as embryos, but when bred into a different background, they survive until birth and can then be maintained on a fucose-supplemented diet. A third example is the formation of bony outgrowths (exostoses) in the growth plates of endochondral bones. In one background, heterozygous mutations in Ext1 or Ext2, which encode the copolymerase involved in heparan sulfate biosynthesis, cause infrequent exostoses, whereas in another background, the incidence of tumors increases severalfold. These experiments suggest the existence of modifiers that could alter the expression levels of enzymes, assembly of precursors, or salvage of sugars from the diet or glycan turnover.
Environmentally Driven Phenotypes
In some mutants, phenotypes are only evident under environmental challenge. For example, deletion of both enzymes responsible for polysialic acid synthesis produces “no-fear” mice that tend to be more aggressive and ignore normally stressful or anxiety-producing situations. Deletion of syndecan-1, a major heparan sulfate core protein (see Chapter 16), shows no obvious changes under normal laboratory conditions, but the mice are more resistant to bacterial lung infections. Apparently, bacteria exploit the shed syndecan in the lung to enhance their virulence and modulate host defenses.
Mice deficient in the synthesis of ganglioside GM3 appear to be fairly normal except for enhanced insulin sensitivity. These mice shunt glycosphingolipid synthesis toward more complex gangliosides that substitute for GM3 loss (see Chapter 10). On the other hand, mice that synthesize only ganglioside GM3, due to inactivation of both Galgt1 and Siat8a, exhibit tonic-clonic (epileptic) seizures, and 90% die in response to sharp sounds. These examples show the importance of having the correct balance of related glycans. They also show how phenotypes depend to a large extent on other genetic factors, diet, and environmental cues. Thus, many mutations that appear silent may in fact elicit strong phenotypes in the natural environment, rather than the controlled laboratory setting.
FURTHER READING
- Kramer KL, Yost HJ. Heparan sulfate core proteins in cell–cell signaling. Annu Rev Genet. 2003;37:461–484. [PubMed: 14616070]
- Lowe JB, Marth JD. A genetic approach to mammalian glycan function. Annu Rev Biochem. 2003;72:643–691. [PubMed: 12676797]
- Biermann CH, Marks JA, Vilela-Silva AC, Castro MO, Mourao PA. Carbohydrate-based species recognition in sea urchin fertilization: Another avenue for speciation. Evol Dev. 2004;6:353–361. [PubMed: 15330868]
- Lee JK, Baum LG, Moremen K, Pierce M. The X-lectins: A new family with homology to the Xenopus laevis oocyte lectin XL-35. Glycoconj J. 2004;21:443–450. [PubMed: 15750785]
- Neill AT, Vacquier VD. Ligands and receptors mediating signal transduction in sea urchin spermatozoa. Reproduction. 2004;127:141–149. [PubMed: 15056779]
- Guerardel Y, Chang LY, Maes E, Huang CJ, Khoo KH. Glycomic survey mapping of zebrafish identifies unique sialylation pattern. Glycobiology. 2006;16:244–257. [PubMed: 16321922]
- Ohtsubo K, Marth JD. Glycosylation in cellular mechanisms of health and disease. Cell. 2006;126:855–867. [PubMed: 16959566]
- Bishop JR, Schuksz M, Esko JD. Heparan sulphate proteoglycans fine-tune mammalian physiology. Nature. 2007;446:1030–1037. [PubMed: 17460664]
- Mourao PA. A carbohydrate-based mechanism of species recognition in sea urchin fertilization. Braz J Med Biol Res. 2007;40:5–17. [PubMed: 17224991]
- Review Deuterostomes.[Essentials of Glycobiology. 2015]Review Deuterostomes.Pierce M, Stanley P. Essentials of Glycobiology. 2015
- Review Deuterostomes.[Essentials of Glycobiology. 2022]Review Deuterostomes.Pierce M, Wilson IBH, Paschinger K, Stanley P. Essentials of Glycobiology. 2022
- Review Nodal signaling and the evolution of deuterostome gastrulation.[Dev Dyn. 2005]Review Nodal signaling and the evolution of deuterostome gastrulation.Chea HK, Wright CV, Swalla BJ. Dev Dyn. 2005 Oct; 234(2):269-78.
- RTK and TGF-beta signaling pathways genes in the sea urchin genome.[Dev Biol. 2006]RTK and TGF-beta signaling pathways genes in the sea urchin genome.Lapraz F, Röttinger E, Duboc V, Range R, Duloquin L, Walton K, Wu SY, Bradham C, Loza MA, Hibino T, et al. Dev Biol. 2006 Dec 1; 300(1):132-52. Epub 2006 Aug 24.
- The genomic underpinnings of apoptosis in Strongylocentrotus purpuratus.[Dev Biol. 2006]The genomic underpinnings of apoptosis in Strongylocentrotus purpuratus.Robertson AJ, Croce J, Carbonneau S, Voronina E, Miranda E, McClay DR, Coffman JA. Dev Biol. 2006 Dec 1; 300(1):321-34. Epub 2006 Aug 30.
- Deuterostomes - Essentials of GlycobiologyDeuterostomes - Essentials of Glycobiology
- Homo sapiens makorin ring finger protein 3 (MKRN3), mRNAHomo sapiens makorin ring finger protein 3 (MKRN3), mRNAgi|1653962586|ref|NM_005664.4|Nucleotide
- Glycomics - Essentials of GlycobiologyGlycomics - Essentials of Glycobiology
Your browsing activity is empty.
Activity recording is turned off.
See more...