NCBI Bookshelf. A service of the National Library of Medicine, National Institutes of Health.
Varki A, Cummings R, Esko J, et al., editors. Essentials of Glycobiology. Cold Spring Harbor (NY): Cold Spring Harbor Laboratory Press; 1999.
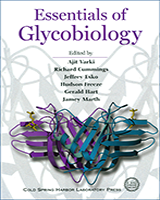
Essentials of Glycobiology.
Show detailsPrimary contributions to this chapter were made by R.D. Cummings (University of Oklahoma Health Science Center).
MANY PROTOZOAL AND HELMINTHIC PARASITES synthesize unusual carbohydrate structures, which are often antigenic and required for invasion of their hosts. Parasites also synthesize carbohydrate-binding proteins for attachment and invasion of host cells. The major protozoal parasites include Plasmodium species (causing malaria), Entamoeba histolytica (causing amebiasis), Leishmania species (causing leishmaniasis), and Trypanosoma species (causing sleeping sickness and Chagas′ disease). The major helminthic parasites include nematodes, such as Ascaris lumbricoides; trematodes, such as Schistosoma mansoni (causing schistosomiasis); and cestodes or tapeworms, such as Taenia solium (causing taeniiasis). This chapter focuses on what is known about the structures of the glycoconjugates and lectins synthesized by these parasites and the roles of glycoconjugates in host-parasite interactions.
Background on Parasite Glycobiology (1)
Parasitism may be defined as a condition in which one organism (the parasite) either harms its host or survives in some way at the expense of the host. Parasites affect millions of people worldwide and cause tremendous suffering and death, especially in less developed countries—some sobering statistics are listed in Table 36.1—and thus the importance of research into parasite glycobiology and biochemistry. In addition, the study of such organisms that have evolved with great success to deceive and compromise the immune systems of infected animals may provide important new insights into molecular pathology. However, parasite glycobiology can be frustrating because of the difficulty in both obtaining sufficient amounts of material for study and carrying out in vitro experimentation. In addition, many parasites have specific primary and intermediate hosts, making it difficult to study all stages of the life cycle.
Table 36.1
Worldwide distribution of some major parasitic diseases.
Despite these obstacles, exciting new results demonstrate that glycoconjugates are very important in the life cycles and pathology of most major parasites. It is becoming clear that some of the protozoal and helminthic parasites rely on carbohydrate-binding proteins in the host to promote their parasitism (also see Chapter 28), and they have elaborated intriguing strategies to defeat the anticarbohydrate immunity of the host. Some of the major parasitic diseases infecting humans and animals are listed as two categories: the protozoal (single-celled) parasites (Table 36.2) and helminths (Table 36.3).
Table 36.2
Some of the major protozoal parasites of humans.
Table 36.3
Some of the major helminthic parasites of mammals.
Malaria (2–10)
Malaria is caused by Plasmodium species, and several major species infect humans, with P. falciparum being the most virulent. The life cycle of P. falciparum is complex (Table 36.4), and there are sexual and asexual stages in the human and insect hosts. Cell-cell interactions between parasite and host are critical for the successful completion of each step in the life cycle. The interaction of sporozoites with hepatocytes requires a protein expressed by the malarial circumsporozoites that recognizes hepatocyte heparan sulfate, possibly in association with the low-density lipoprotein receptor-related protein (LRP) (Table 36.5; also see Chapter 28). Sulfated glycoconjugates can inhibit sporozoite interactions with cells in vitro, and malarial sporozoites and the circumsporozoite protein do not bind to Chinese hamster cell mutants defective in glycosaminoglycan synthesis (see Chapter 31).
Table 36.4
Life cycle of Plasmodium falciparum that causes malaria.
Table 36.5
Some major parasites and their carbohydrate-binding proteins.
In addition, sialic acid on the erythrocytes is critical for binding and invasion of those cells by the merozoite stage. Desialylation of erythrocytes blocks interactions, and individuals lacking glycophorin A (Ena-) or glycophorin B (MkMk) are resistant to invasion. The glycophorins are the major sialic-acid-containing glycoproteins on erythrocytes. Merozoites probably contain several sialic-acid-binding proteins; two have been identified so far and named EBA-175 and MSA-1, which are immunologically distinct and may have different functions. Hapten inhibition experiments indicate that both of these proteins bind sialic acid specifically and that the sialic acid linkage is important.
Glycoconjugates synthesized by the parasite may also be important in the invasion process. Plasmodium synthesizes both free and protein-bound GPI lipids, which are structurally different and whose expression is developmentally regulated (see Chapter 10). The free GPI lipids from Plasmodium are bioactive and can elevate expression of host adhesion molecules, such as I-CAM-1, V-CAM-1, and E-selectin in human umbilical vein endothelial cells. This activity occurs via a tyrosine phosphorylation cascade that is blocked by a specific antagonist of tyrosine kinases, but the specific mechanism of action is unclear.
Trypanosomiasis (11–18)
Trypanosoma cruzi is the cause of Chagas′ disease, a debilitating disease in South America affecting multiple organ systems; T. brucei gambiense and T.b. rhodesiense are responsible for African sleeping sickness in humans. Still another subspecies, T.b. brucei, does not infect humans, but it causes a disease called nagana in native antelopes and other African ruminants. T. brucei lives free in the host blood and lymph, where it is constantly exposed to host immunity, whereas T. cruzi is sequestered in host cells, where it can partly evade the immune system. The complex life cycle of T. cruzi is shown in Figure 36.1. To evade host immunity, T. brucei expresses high levels of a GPI-anchored variable surface glycoprotein on its plasma membrane (~10 million copies per cell) (see Chapter 10). The primary structure of the VSG changes periodically during infection due to expression of a different VSG gene. The organism can express up to 1000 different VSGs, but only one is expressed at a time. The new variants are not recognized by preexisting antibodies, thus allowing a temporary evasion of immunity. When immunity arises to these variants resulting in their destruction, the numbers of other variants that are not recognized by the immune system expand. This cycle continues until the hosts dies. Other trypanosomes also express GPI anchors, like all eukaryotes, but the core structures differ in terms of their modifications (Figure 36.2). GPI anchors in T. cruzi are required for effective parasitism. Induction of a GPI deficiency in T. cruzi by the heterologous expression of T. brucei GPI-phospholipase C depresses surface glycoprotein expression and decreases virulence in infected animals.

Figure 36.1
Life cycle of Trypanosoma cruzi. (Adapted, with permission, from [11] Adam et al. 1971.)

Figure 36.2
GPI anchors of Trypanosoma sp. and Leishmania sp. In T. brucei VSG: R1, R2, and R24 = OH, R3 = αGal0–5, and the lipid is a diacyl-glycerol. In T. cruzi 1G7: R1 = αMan, R2, R3, and R4 = OH, and the lipid is undefined. In L. major (more...)
As discussed for Plasmodium, sialic acid residues are also critical for succcessful attachment of circulating T. cruzi trypomastigotes to host cells. The interesting adhesion molecule for this attachment was identified as a GPI-anchored trans-sialidase on the surface of the protozoa. The enzyme can act as a conventional sialidase, but it also is capable of removing sialic acid from host glycoconjugates and transferring it to its own glycoconjugates. These observations explain the anomaly that the parasites are sialylated but lack a conventional sialyltransferase. This activity is specific for sialic acid linkage (α2,3) and for attachment to terminal galactose residues. The sialic acids appear to protect the organism from complement activation and antibody formation. Antibodies induced to the trans-sialidase by immunization with cDNA for the enzyme provide protective immunity to challenged animals. Trypomastigotes also contain a surface heparin-binding protein of approximately 60 kD termed penetrin that interacts with heparan sulfate molecules of host cells. Mannose-containing glycoproteins on amastigotes may also be ligands for human macrophage mannose-binding proteins (see Chapter 28).
In addition to the obvious value of studying trypanosomes for insights into their biology, studies of N-glycan biosynthesis in trypanosomes have been particularly fruitful in defining the general functions of glycoconjugates in glycoprotein biosynthesis. Trypanosomes are unable to synthesize Dol-P-Glc and therefore synthesize a lipid-linked oligosaccharide donor that is Man6,7,or9GlcNAc2-P-P-Dol, depending on the species. The oligosaccharyltransferase of trypanosomes, unlike the mammalian enzyme, efficiently transfers these nonglucosylated donor oligosaccharides to asparagine residues in newly synthesized glycoproteins. However, trypanosomes have a glucosidase-II-like activity, which was shown to be required for deglucosylation of N-glycans glucosylated by the UDP-Glc:glycoprotein glucosyltransferase, an activity that was first detected in those parasites. Glucosylation of newly synthesized glycoproteins is probably an important aspect of chaperone-assisted (calnexin/calreticulin) glycoprotein folding and exit from the ER in all eukaryotes (see Chapter 7).
Leishmaniasis (19–24)
Leishmania donovani is a protozoal parasite that causes visceral leishmaniasis; other species cause mucocutaneous leishmaniasis (see Figure 36.3). The surface of the promastigote is covered with a macromolecular glycoconjugate, a phosphoglycan, containing high amounts of mannose. In L. donovani, this is present in the lipophosphoglycan, shown in Figure 36.4, that is not attached to protein. In contrast, the phosphoglycan of L. mexicana is linked to serine residues in proteins, such as secreted acid phosphatase (sAP); in L. major, the phosphoglycan is serine linked to a proteophosphoglycan. The LPG of L. donovani is synthesized by sequential addition of Man-1-P from GDP-Man and galactose from UDP-Gal. The LPG of L. donovani is a critical ligand for recognition by the macrophage mannose receptor and entry of the parasite into macrophages. In addition, the L. donovani LPG can inhibit phagosome-endosome fusion and prevent lysis of the endocytosed parasite. The LPG of L. donovani is generally required for the parasite's survival; mutagenized Leishmania promastigotes that cannot synthesize LPG grow well in vitro, but they cannot grow within the sand fly nor establish infections in macrophages. Although the bioactivity of the LPG is unclear, it inhibits protein kinase C in macrophages, which is normally required for oxidative burst in these cells. Thus, the phosphoglycans may all function to allow survival and reproduction of parasites within vacuoles of infected cells. One of the mutations in the Leishmania affecting mannose incorporation into LPG was identified to be within a gene encoding a protein that has predicted homology with membrane transporters and is predicted to encode a GDP-Man transporter (see Chapter 6).

Figure 36.3
Life cycle of Leishmania donovani. (Adapted from [57] Zaman and Keong 1989.)
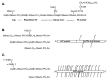
Figure 36.4
Phosphoglycans of Leishmania sp. (A) L. donovani LPG. The procyclic LPG contains approximately 15 repeating units; (B) L. mexicana secreted acid phosphatase (sAP); (C) L. major proteophosphoglycan (PPG). The phosphoglycans, represented by the wavy lines, (more...)
The LPG of L. donovani also has other interesting biological activities. For example, it can activate HIV-1 replication in monocytoid cells and directly up-regulate HIV-1 transcription in T cells, via activation of transcription factors that recognize NF-κB-binding sites. These results support the possibility that L. donovani may be a putative cofactor in HIV-1 pathogenesis. Altogether, the phosphoglycans expressed by Leishmania sp. may be among the most potent weapons of defense presented by protozoal parasites, since these organisms are able to infect and thrive in host macrophages.
Schistosomiasis (25–45)
Schistosomiasis is caused by a parasitic trematode, and three major species exist that infect humans worldwide (Figure 36.5). The parasite is unique in that the male/female pair live in the blood vessels of its host and lay eggs that adhere to the endothelium. The eggs may become lodged in the host tissues; many eventually pass into the stool and continue the cycle through intermediate snail hosts, which differ for each Schistosoma species.
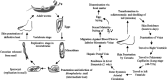
Figure 36.5
Life cycle of Schistosoma mansoni and its movement within its vertebrate host.
Schistosomes generate huge quantities of membrane-bound and circulating glycoproteins containing fucosylated antigens. Three of the notable antigenic carbohydrate structures found in schistosome glycans include the Lewis X antigen, LDN, LDNF, and several others (Figure 36.6). Overall, fucosylation is a common theme for most schistosome glycoconjugates. Interestingly, other helminths, such as Echinococcus granulosus, Dirofilaria immitis, and Haemonchus contortus, also synthesize glycoproteins containing LDN and LDNF, in addition to other fucosylated and xylosylated glycans (Figure 36.7). In general, schistosomes appear to be especially rich in carbohydrate structures and contain an impressive array of glycosphingolipids and O- and N-glycans on a multitude of glycoproteins. However, among all helminths tested so far, only schistosomes appear to synthesize the Lewis X antigen.
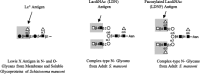
Figure 36.6
Some fucosylated schistosome glycoconjugates.

Figure 36.7
Other types of glycoconjugates from schistosomes and Haemonchus contortus.
Another theme in schistosomes and other helminths studied so far is the absence of sialic acid in glycoconjugates synthesized by the worms and the absence of sialyltransferase activities. Schistosomes synthesize many interesting glycoconjugates in their so-called cercarial glycocalyx and in eggs. Glycoproteins derived from the tegument, gut, and eggs of the parasite are highly antigenic and occur in the circulation of the infected animal. The expression of many of these glycan structures is developmentally regulated and stage-specific, but their fundamental roles in parasite development and host pathogenesis are not clear. It is also likely that the different schistosome species differ in several ways in terms of their glycoconjugate structures. For example, the S. mansoni glycosphingolipids have an extended difucosylated oligosaccharide, but the terminal difucosylated GalNAc is absent from glycosphingolipids of S. japonicum (Figure 36.7).
Individuals infected with Schistosoma species develop an autoimmunity to the Lewis X antigen. This is surprising in many ways, since the Lewis X antigen is a common mammalian leukocyte marker (CD15) (see Chapter 16). The adult worms have a rough tegumental syncytial membrane that is highly regenerative, even in response to complement attack. This is an interesting phenomenon, because schistosomiasis is characterized by intense humoral immunity to carbohydrate antigens, but immune attack is ineffective in destroying the mature worms, except when the curative drug praziquantel is administerd, which requires humoral immunity for effectiveness. Evidence now exists that expression of the Lewis X antigen by the parasite may be important in compromising host cellular immunity. During chronic schistosome infection, Th2 immune responses (promoting humoral immunity) predominate over Th1 responses (promoting cellular immunity). In response to glycans containing the Lewis X antigen, murine B-1 cells secrete in vitro large amounts of IL-10. Because IL-10 can depress Th1 responses in animals, the Lewis X antigen may partly contribute to Th2 dominance in early stages of schistomiasis.
Glycans synthesized by schistosome eggs may also be involved in adhesion to the endothelium and may be ligands for selectins, such as soluble forms of L-selectin, a member of the C-type family of carbohydrate-binding proteins expressed in the vascular bed (see Chapter 26). Surprisingly, the schistosomes themselves are reported to express selectin-like molecules that interact with host cells expressing fucosylated glycans. The identification of many different antigenic glycoconjugates from schistosomes is helping design new diagnostic procedures for schistosomiasis. In addition, characterization of the glycosyltransferases in schistosomes and responsible for antigenic glycan biosynthesis and of enzymes in their intermediate snail hosts may help identify new drug targets and develop carbohydrate-based vaccines.
Glycobiology of Other Parasites (46–57)
Glycoconjugates and carbohydrate-binding proteins, as discussed above, play an impressive part in host infection by many different parasites. Several additional examples are shown in Table 36.4. Many parasitic protozoans appear to use carbohydrate-binding proteins as a major mechanism for host-cell attachment and invasion (see Chapter 28). For example, one of the major glycoproteins expressed by Entamoeba histolytica, a major cause of amoebic dysentery, is a lectin that recognizes Gal/GalNAc residues, resulting in adherence of trophozoites to host cells. Adhesion is followed by contact-dependent cytolysis of host cells. Acanthamoeba keratitis, which causes severe eye infections involving the corneal epithelium, also adheres via a lectin interaction. This lectin-mediated adhesion of A. keratitis to host cells is a prerequisite for the amoeba-induced cytolysis of target cells. The adhesion occurs via a mannose-binding protein that is highly inhibited by Manα1–3Man disaccharides. Interestingly, mannose and Man-6-P can inhibit adhesion of Giardia lamblia trophozoites. One of the proteins from the parasite that may bind mannose and Man-6-P is termed taglin. The cDNAs of several galectin family members, identified initially as antigenic proteins, have been cloned from parasitic nematodes, such as Teladorsagia circumcincta. Sporozoites from Cryptosporidium parvum, an opportunistic protozoan infecting individuals with compromised immunity, have hemagglutinating activity, and a lectin on the parasite surface may have a crucial role in host-cell attachment.
In addition to these ongoing studies on carbohydrate-binding proteins in parasites, the carbohydrate antigens of many parasites are being characterized in the hope that the information may lead to the development of vaccines and new diagnostics for these diseases. For example, the major antigenic glycoconjugates synthesized by larvae of the parasitic nematodes Toxocara canis and T. cati are O-methylated trisaccharides containing 2-O-methyl fucosyl and galactosyl residues. The intestinal nematode Trichinella spiralis synthesizes several highly immunogenic glycoproteins that contain the unusual sugar tyvelose (3,6-dideoxy-d-arabino-hexose). Tyvelose is found in complex-type N-glycans of larvae from the T. spiralis and is a critical antigenic determinant recognized by antibodies in infected animals. Strong immunity to these glycans provide protective immunity, which causes expulsion of the invading larvae from the intestine. Protective immunity is also provided by antibodies to uncharacterized carbohydrate antigens from Haemonchus contortus, an intestinal parasitic nematode of ruminants.
Future Directions
Although much more work remains to identify and fully characterize the roles that parasite-derived lectins and glycans have in parasitic infections, the information gathered so far suggests that glycoconjugates are immensely important to many types of protozoal and helminthic parasites. Future studies in this area will probably aim at developing new drugs that block unique parasite glycoconjugate biosynthesis or interfere with parasite recognition by host receptors. Information about the important carbohydrate-binding proteins of the parasites and the unique glycoconjugates expressed by the parasites might promote development of new types of diagnostic assays. In addition, because many parasites express specific lectins required for cell adhesion, vaccines based on these lectins could be particularly effective in limiting infection. Vaccines could also be developed against specific parasite glycoconjugates identified as being uniquely antigenic and important for parasite invasion.
References
- 1.
- Schmidt G.D. and Roberts L.S. 1996. Foundations of parasitology, 5th edition, p. 2, Wm. C. Brown, New York.
- 2.
- Hoessli D C, Davidson E A, Schwarz R T, Nasir-ud-Din Glycobiology of Plasmodium falciparum: An emerging area of research. Glycoconj. J. 1996;13:1–3. [PubMed: 8785480]
- 3.
- Camus D, Hadley T J. A Plasmodium falciparum antigen that binds to host erythrocytes and merozoites. Science. 1985;230:553–556. [PubMed: 3901257]
- 4.
- Shakibaei M, Frevert U. Dual interaction of the malaria circumsporozoite protein with the low density lipoprotein receptor-related protein (LRP) and heparan sulfate proteoglycans. J. Exp. Med. 1996;184:1699–1711. [PMC free article: PMC2192891] [PubMed: 8920859]
- 5.
- Pancake S J, Holt G D, Mellouk S, Hoffman S L. Malaria sporozoites and circumsporozoite proteins bind specifically to sulfated glycoconjugates. J. Cell Biol. 1992;117:1351–1357. [PMC free article: PMC2289501] [PubMed: 1607393]
- 6.
- Schofield L, Novakovic S, Gerold P, Schwarz R T, McConville M J, Tachado S D. Glycosylphosphatidylinositol toxin of Plasmodium up-regulates intercellular adhesion molecule-1, vascular cell adhesion molecule-1, and E-selectin expression in vascular endothelial cells and increases leukocyte and parasite cytoadherence via tyrosine kinase-dependent signal transduction. J. Immunol. 1996;156:1886–1896. [PubMed: 8596041]
- 7.
- Clark D L, Su S, Davidson E A. Saccharide anions as inhibitors of the malaria parasite. Glycoconj. J. 1997;14:473–479. [PubMed: 9249145]
- 8.
- Schmidt A, Schwarz R T, Gerold P. Plasmodium falciparum: Asexual erythrocytic stages synthesize two structurally distinct free and protein-bound glycosylphosphatidylinositols in a maturation-dependent manner. Exp. Parasitol. 1998;88:95–102. [PubMed: 9538863]
- 9.
- el Moudni B, Philippe M, Monsigny M, Schrevel J. N-acetylglucosamine-binding proteins on Plasmodium falciparum merozoite surface. Glycobiology. 1993;3:305–312. [PubMed: 8400546]
- 10.
- Ward H.D. 1997. Glycobiology of parasites: Role of carbohydrate-binding proteins and their ligands in the host-parasite interaction. In Glycosciences: Status and perspectives (ed. Gabius H.J. and Gabius S.), pp. 399–413. Chapman and Hall, Weinheim, Germany.
- 11.
- Adam K.M.G., Paul J., and Zaman V. 1971. Medical and veterinary protozoology: An illustrated guide. Churchill Livingston, Edinburgh, United Kingdom.
- 12.
- Schenkman S, Eichinger D, Pereira M E A, Nussenzweig V. Structural and functional properties of Trypanosoma cruzi trans-sialidase. Annu. Rev. Microbiol. 1994;48:499–523. [PubMed: 7826016]
- 13.
- Kahn S J, Wleklinski M, Ezekowitz R A, Coder D, Aruffo A, Farr A. The major surface glycoprotein of Trypanosoma cruzi amastigotes are ligands of the human serum mannose-binding protein. Infect. Immun. 1996;64:2649–2656. [PMC free article: PMC174122] [PubMed: 8698491]
- 14.
- Costa F, Franchin G, Pereira-Chioccola V L, Ribeirao M, Schenkman S, Rodrigues M M. Immunization with a plasmid DNA containing the gene of trans-sialidase reduces Trypanosoma cruzi infection in mice. Vaccine. 1998;16:768–74. [PubMed: 9627933]
- 15.
- Mehlert A, Zitzmann N, Richardson J M, Treumann A, Ferguson M A. The glycosylation of the variant surface glycoproteins and procyclic acidic repetitive proteins of Trypanosoma brucei. Mol. Biochem. Parasitol. 1998;91:145–152. [PubMed: 9574932]
- 16.
- Parodi A J. N-glycosylation in trypanosomatid protozoa. Glycobiology. 1993;3:193–199. [PubMed: 8358146]
- 17.
- Ribeirao M, Pereira-Chioccola V L, Eichinger D, Rodrigues M M, Schenkman S. Temperature differences for trans-glycosylation and hydrolysis reaction reveal an acceptor binding site in the catalytic mechanism of Trypanosoma cruzi trans-sialidase. Glycobiology. 1997;7:1237–1246. [PubMed: 9455925]
- 18.
- Garg N, Postan M, Mensa-Wilmot K, Tarleton R L. Glycosylphosphatidylinositols are required for the development of Trypanosoma cruzi amastigotes. Infect. Immun. 1997;65:4055–4060. [PMC free article: PMC175583] [PubMed: 9317007]
- 19.
- Mengeling B J, Beverley S M, Turco S J. Designing glycoconjugate biosynthesis for an insidious intent: Phosphoglycan assembly in Leishmania parasites. Glycobiology. 1997;7:873–880. [PubMed: 9363429]
- 20.
- Ma D, Russell D G, Beverley S M, Turco S J. Golgi GDP-mannose uptake requires Leishmania LPG2. A member of a eukaryotic family of putative nucleotide-sugar transporters. J. Biol. Chem. 1997;272:3799–3805. [PubMed: 9013638]
- 21.
- Bernier R, Barbeau B, Tremblay M J, Olivier M. The lipophosphoglycan of Leishmania donovani up-regulates HIV-1 transcription in T cells through the nuclear factor-κB elements. J. Immunol. 1998;160:2881–2888. [PubMed: 9510191]
- 22.
- Mengeling B J, Turco S J. Microbial glycoconjugates. Curr. Opin. Struct. Biol. 1998;8:572–577. [PubMed: 9818260]
- 23.
- Smith D F, Rangarajan D. Cell surface components of Leishmania: Identification of a novel parasite lectin? Glycobiology. 1995;5:161–166. [PubMed: 7780190]
- 24.
- Opat A, Ng K, Currie G, Handman E, Bacic A. Characterization of lipophosphoglycan from a ricin-resistant mutant of Leishmania major. Glycobiology. 1996;6:387–397. [PubMed: 8842702]
- 25.
- Cummings R D, Nyame A K. Glycobiology of schistosomiasis. FASEB J. 1996;10:838–848. [PubMed: 8666160]
- 26.
- Ko A I, Drager U C, Harn D A. A Schistosoma mansoni epitope recognized by a protective monoclonal antibody is identical to the stage-specific embryonic antigen 1. Proc. Natl. Acad. Sci. 1990;87:4159–4163. [PMC free article: PMC54067] [PubMed: 1971946]
- 27.
- Velupillai P, Harn D A. Oligosaccharide-specific induction in interleukin 10 production by B22+ cells from schistosome-infected mice: A mechanism for regulation of CD4+ T-cell subsets. Proc. Natl. Acad. Sci. 1994;91:18–22. [PMC free article: PMC42877] [PubMed: 7904066]
- 28.
- Khoo K H, Chatterjee D, Caulfield J P, Morris H R, Dell A. Structural characterization of glycophingolipids from the eggs of Schistosoma mansoni and Schistosoma japonicum. Glycobiology. 1997;7:653–661. [PubMed: 9254047]
- 29.
- Khoo K H, Nieto A, Morris H R, Dell A. Structural characterization of the N-glycans from Echinococcus granulosus hydatid cyst membrane and protoscoleces. Mol. Biochem. Parasitol. 1997;86:237–248. [PubMed: 9200129]
- 30.
- Halkes K M, Vermeer H J, Slaghek T M, van Hooft P A, Loof A, Kamerling J P, Vliegenthart J F. Preparation of spacer-containing di-, tri-, and tetrasaccharide fragments of the circulating anodic antigen of Schistosoma mansoni for diagnostic purposes. Carbohydr. Res. 1998;309:175–188. [PubMed: 9741076]
- 31.
- van den Eijnden D H, Neeleman A P, Bakker H, van Die I. Novel pathways in complex-type oligosaccharide synthesis. New vistas opened by studies in invertebrates. Adv. Exp. Med. Biol. 1998;435:3–7. [PubMed: 9498060]
- 32.
- DeBose-Boyd R, Nyame A K, Cummings R D. Schistosoma mansoni: Characterization of an α1–3 fucosyltransferase in adult parasites. Exp. Parasitol. 1996;82:1–10. [PubMed: 8617325]
- 33.
- Marques E T Jr, Weiss J B, Strand M. Molecular characterization of a fucosyltransferase encoded by Schistosoma mansoni. Mol. Biochem. Parasitol. 1998;93:237–250. [PubMed: 9662708]
- 34.
- Hokke C H, Neeleman A P, Koeleman C A, van den Eijnden D H. Identification of an α3-fucosyltransferase and a novel α2-fucosyltransferase activity in cercariae of the schistosome Trichobilharzia ocellata: Biosynthesis of the Fucα1–2Fucα1–3[Gal(NAc)β1–4]GlcNAc sequence. Glycobiology. 1998;8:393–406. [PubMed: 9499387]
- 35.
- Srivatsan J, Smith D F, Cummings R D. Schistosoma mansoni synthesizes novel biantennary Asn-linked oligosaccharides containing terminal β-linked N-acetylgalactosamine. Glycobiology. 1992;2:445–452. [PubMed: 1457973]
- 36.
- Neeleman A P, van der Knaap W P, van den Eijnden D H. Identification and characterization of a UDP-GalNAc:GlcNAc β-R β1→4-N-acetylgalactosaminyltransferase from cercariae of the schistosome Trichobilharzia ocellata. Catalysis of a key step in the synthesis of N,N′-diacetyllactosediamino (lacdiNAc)-type glycans. Glycobiology. 1994;4:641–651. [PubMed: 7881179]
- 37.
- Trottein F, Nutten S, Papin J P, Leportier C, Poulain-Godefroy O, Capron A, Capron M. Role of adhesion molecules of the selectin-carbohydrate families in antibody-dependent cell-mediated cytoxicity to schistosome targets. J. Immunol. 1997;159:804–811. [PubMed: 9218598]
- 38.
- Velupillai P, Secor W E, Horauf A M, Harn D A. B-1 cell (CD5+B220+) outgrowth in murine schistosomiasis is genetically restricted and is largely due to activation by polylactosamine sugars. J. Immunol. 1997;158:338–344. [PubMed: 8977208]
- 39.
- van Lieshout L, Polderman A M, Visser L G, Verwey J J, Deelder A M. Detection of the circulating antigens CAA and CCA in a group of Dutch travellers with acute schistosomiasis. Trop. Med. Int. Health. 1997;2:551–557. [PubMed: 9236822]
- 40.
- van Dam G J, Bergwerff A A, Thomas-Oates J E, Rotmans J P, Kamerling J P, Vliegenthart J F G, Deelder A M. The immunologically reactive O-linked polysaccharide chains derived from circulating cathodic antigen isolated from the human blood fluke Schistosoma mansoni have Lewis x as repeating unit. Eur. J. Biochem. 1994;225:467–482. [PubMed: 7925469]
- 41.
- El Ridi R, Velupillai P, Harn D A. Regulation of schistosome egg granuloma formation: Host-soluble L-selectin enters tissue-trapped eggs and binds to carbohydrate antigens on surface membranes of miracidia. Infect. Immun. 1996;64:4700–4705. [PMC free article: PMC174434] [PubMed: 8890228]
- 42.
- Bergwerff A A, van Dam G J, Rotmans J P, Deelder A M, Kamerling J P, Vliegenthart J F G. The immunologically reactive part of immunopurified circulating anodic antigen from Schistosoma mansoni is a threonine-linked polysaccharide consisting of →6)-β-d-GlcpA-(1→3))-β-d-GalpNAc-(1→ repeating units. J. Biol. Chem. 1994;269:31510–31517. [PubMed: 7989318]
- 43.
- Haslam S M, Coles G C, Reason A J, Morris H R, Dell A. The novel core fucosylation of Haemonchus contortus N-glycans is stage specific. Mol. Biochem. Parasitol. 1998;93:143–147. [PubMed: 9662037]
- 44.
- Haslam S M, Coles G C, Munn E A, Smith T S, Smith H F, Morris H R, Dell A. Haemonchus contortus glycoproteins contain N-linked oligosaccharides with novel highly fucosylated core structures. J. Biol. Chem. 1996;271:30561–30570. [PubMed: 8940027]
- 45.
- Nyame A K, Debose-Boyd R, Long T D, Tsang V C, Cummings R D. Expression of Lex antigen in Schistosoma japonicum and S. haematobium and immune responses to Lex in infected animals: Lack of Lex expression in other trematodes and nematodes. Glycobiology. 1998;8:615–624. [PubMed: 9592128]
- 46.
- Reason A J, Ellis L A, Appleton J A, Wisnewski N, Grieve R B, McNeil M, Wassom D L, Morris H R, Dell A. Novel tyvelose-containing tri- and tetra-antennary N-glycans in the immunodominant antigens of the intracellular parasite Trichinella spiralis. Glycobiology. 1994;4:593–603. [PubMed: 7881173]
- 47.
- Petri W Jr, Mann B J. Molecular mechanisms of invasion by Entamoeba histolytica. Semin. Cell Biol. 1993;4:305–313. [PubMed: 7504959]
- 48.
- Farhing J J, Pereira M E, Keusch G T. Description and characterization of a surface lectin from Giardia lamblia. Infect. Immun. 1986;51:661–667. [PMC free article: PMC262400] [PubMed: 3943906]
- 49.
- Cao Z, Jefferson D M, Panjwani N. Role of carbohydrate-mediated adherence in cytopathogenic mechanisms of Acanthamoeba. J. Biol. Chem. 1998;273:15838–15845. [PubMed: 9624184]
- 50.
- Joe A, Verdon R, Tzipori S, Keusch G T, Ward H D. Attachment of Cryptosporidium parvum sporozoites to human intestinal epithelial cells. Infect. Immun. 1998;66:3429–3432. [PMC free article: PMC108364] [PubMed: 9632617]
- 51.
- Ward H D, Feusch G T, Pereira M A E. Induction of a phosphomannosyl binding activity in Giardia. BioEssays. 1990;12:211–215. [PubMed: 2196051]
- 52.
- Katelaris P H, Naeem A, Farthing M J. Attachment of Giardia lamblia trophozoites to a cultured human intestinal cell line. Gut. 1995;37:512–518. [PMC free article: PMC1382903] [PubMed: 7489938]
- 53.
- Thea D M, Pereira M E, Kotler D, Sterling C R, Keusch G T. Identification and partial purification of a lectin on the surface of the sporozoite of Cryptosporidium parvum. J. Parasitol. 1992;78:886–893. [PubMed: 1403433]
- 54.
- Yi D, Lee R T, Longo P, Boger E T, Lee Y C, Petri W A Jr, Schnaar R L. Substructural specificity and polyvalent carbohydrate recognition by the Entamoeba histolytica and rat hepatic N-acetylgalactosamine/galactose lectins. Glycobiology. 1998;8:1037–1043. [PubMed: 9719685]
- 55.
- Khoo K H, Maizels R M, Page A P, Taylor G W, Rendell N B, Dell A. Characterization of nematode glycoproteins: The major O-glycans of Toxocara excretory-secretory antigens are O-methylated trisaccharides. Glycobiology. 1991;1:163–171. [PubMed: 1823159]
- 56.
- Jasmer D P, Perryman L E, Conder G A, Crow S, McGuire T. Protective immunity to Haemonchus contortus induced by immunoaffinity isolated antigens that share a phylogenetically conserved carbohydrate gut surface epitope. J. Immunol. 1993;151:5450–5460. [PubMed: 7693812]
- 57.
- Newton S E, Monti J R, Greenhalgh C J, Ashman K, Meeusen E N. cDNA cloning of galectins from third stage larvae of the parasitic nematode Teladorsagia circumcincta. Mol. Biochem. Parasitol. 1997;86:143–153. [PubMed: 9200121]
- 58.
- Zaman V. and Keong L.A. 1989. Handbook of medical parisitology, 2nd edition, p. 35. K.C. Ang Publishing Pte Ltd., Singapore.
- Glycobiology of Protozoal and Helminthic Parasites - Essentials of GlycobiologyGlycobiology of Protozoal and Helminthic Parasites - Essentials of Glycobiology
Your browsing activity is empty.
Activity recording is turned off.
See more...