NCBI Bookshelf. A service of the National Library of Medicine, National Institutes of Health.
Varki A, Cummings R, Esko J, et al., editors. Essentials of Glycobiology. Cold Spring Harbor (NY): Cold Spring Harbor Laboratory Press; 1999.
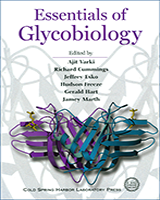
Essentials of Glycobiology.
Show detailsPrimary contributions to this chapter were made by R.D. Cummings (University of Oklahoma Health Sciences Center) and J.B. Lowe (HHMI, University of Michigan Medical School).
THIS CHAPTER SUMMARIZES THE CURRENT KNOWLEDGE concerning the structure, function, and expression of the family of C-type lectins termed selectins and the corresponding glycan structures that decorate the ligands for these molecules and are required for ligand activity. The three members of this family, termed L-selectin, E-selectin, and P-selectin, have been given the cluster designations CD62L, CD62E, and CD62P. These molecules share overall structure and primary sequences and exhibit similarities in the cognate glycans that contribute to their adhesion function, and each participates in mediating interactions among blood cells and endothelial cells. In most cases, these interactions are characterized by relatively rapid on/off kinetics of binding and are most relevant in the context of vascular shear flow.
Background (1–13)
The discovery of the selectins arose from efforts to define the molecules and mechanisms that facilitate the recirculation of lymphoid cells from the intravascular compartment to the secondary lymphoid organs, including lymph nodes and Peyer's patches, from which these cells then return to the circulation through the lymphatic system. This recirculation process provides lymphocytes the opportunity to encounter foreign antigens displayed by antigen-presenting cells within secondary lymphoid organs. Early studies indicated that blood lymphocytes enter lymph nodes in postcapillary venules within these organs. These postcapillary venules are lined with specialized endothelium and are termed high endothelial venules, or HEVs. These endothelial cells are rather cuboidal in shape and their surfaces are decorated with certain glycoproteins required for adhesion of lymphocytes to the HEVs, leading to subsequent transmigration.
Early studies of this adhesion process involved the generation of a monoclonal antibody called MEL-14 that blocked both in vitro adhesion of lymphocytes to HEVs and in vivo homing of lymphocytes to peripheral lymph nodes. Contemporaneous studies provided indirect evidence that oligosaccharide components of the HEV glycocalyx contributed to lymphocyte-HEV adhesive events. For instance, formaldehyde fixation of HEVs exposed on frozen sections did not disrupt lymphocyte HEV adhesion (the Stamper-Woodruff assay), implying that the fixation-resistant glycan components of the HEV surface are responsible for adhesion. Furthermore, assay of lymphocyte-HEV interactions using this approach showed that various specific mono- and polysaccharides could inhibit lymphocyte-HEV interactions. The most potent polysaccharides were fucoidan (a sulfated high-molecular-weight polysaccharide from brown seaweed containing α2- and α3-linked fucose residues) and PPME (a yeast-derived mannan-based polysaccharide containing an abundance of Man-6-P groups). Both inhibited lymphocyte adhesion at concentrations in the low nanomolar range. Moreover, treatment of lymphocytes with PPME inhibited their adhesion to HEVs, whereas pretreatment of the HEV did not inhibit adhesion. These observations and others showing direct binding of PPME to lymphocytes implied that PPME was recognized by a carbohydrate-binding protein or lectin expressed on the surface of lymphocytes and that it was involved in adhesion to HEVs. Subsequent studies demonstrated that PPME interacts with the lymphocyte cell surface protein recognized by the MEL-14 antibody. These observations implied that the MEL-14 antigen corresponded to a carbohydrate-binding protein, now known as L-selectin (CD62L), that mediates lymphocyte adhesion to HEV through recognition of specific carbohydrate-based ligands on this endothelium.
This hypothesis was further substantiated by studies demonstrating a requirement for sialic acids in this interaction. Specifically, sialidase treatment of paraformaldehyde-fixed HEVs or intravascular injection of bacterial sialidases yielded, respectively, decreased lymphocyte adhesion to HEV in vitro and diminished short-term homing of lymphocytes to peripheral lymph nodes in vivo. These observations indicated that sialic acid is an important contributor to the carbohydrate-based molecules on the HEVs that mediated lymphocyte trafficking.
Meanwhile, the molecule now known as E-selectin (CD62E) was identified as endothelial leukocyte adhesion molecule I (ELAM-1). This arose from studies that sought to identify leukocyte adhesion molecules expressed by vascular endothelium in the context of inflammation. Monoclonal antibodies generated against endothelial cell surface molecules induced following treatment with IL1-β or TNF-α were screened for their ability to block adhesion of neutrophils to this activated endothelium. These antibodies were later used to clone cDNAs encoding E-selectin.
In parallel with this work, P-selectin was discovered as an antigen expressed on the surfaces of activated platelets; it was originally termed granule membrane protein 140 (GMP-140) or platelet activation-dependent granule to external membrane (PADGEM) protein, because of its localization to platelet α granules and its release following platelet activation. These terms are no longer in general use. However, P-selectin (CD62P) is also expressed in megakaryocytes, and in the Weibel-Palade bodies of vascular endothelial cells. Within minutes following activation of either platelets or endothelial cells, P-selectin is expressed on the cell surface as a result of fusion of the intracellular storage compartments with the plasma membrane. Storage granule mobilization and fusion are observed following treatment of endothelial cells with histamine, protein kinase C activators, fragments of complement, and thrombin, for example.
In 1989, the nearly simultaneous cloning of these three proteins resulted in the realization that they belonged to a single unique family. The presence of the amino-terminal C-type lectin domain in all three cDNAs and the previous evidence for carbohydrate involvement in L-selectin binding triggered work in many laboratories that eventually led to the recognition of their carbohydrate ligands and their functions in vivo.
L-Selectin Structure and Expression (3,4,8,13)
Molecular cloning studies subsequently isolated cDNAs corresponding to the MEL-14 antigen and its human homolog. This protein is termed L-selectin and has been previously referred to as LEC-CAM, Ly-22, Leu8, TQ1, DREG-56, and LAM-1 by different groups, although these terms are no longer in general use. The polypeptide sequence derived from the cloned cDNAs predicted a molecule corresponding to a type I transmembrane glycoprotein with a major amino-terminal extracellular segment (Figure. 26.1). Analysis of this polypeptide sequence defined a series of discrete domains, beginning with an amino-terminal signal peptide sequence. The amino-terminal segment of the mature protein shows primary sequence similarity to other members of the C-type (Ca++-dependent) lectin family (see Chapter 25). The amino-terminal 116 residues of mature L-selectin protein share approximately 25–30% amino acid sequence identity with other C-type lectins, such as the CRDs of the mammalian hepatocyte asialoglycoprotein receptor, the Kupffer cell fucose-binding protein, and several mannose-binding proteins. Four cysteine residues conserved among the C-type lectins are similarly maintained in L-selectin. Mouse and human L-selectin carbohydrate recognition domains share 83% amino acid sequence identity, which is consistent with observations implying that mouse and human L-selectin share ligand recognition properties.
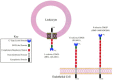
Figure 26.1
Domain structures of L-selectin, E-selectin, and P-selectin.
The CRD in L-selectin is followed by a domain with primary sequence similarity to the EGF precursor. The EGF-like domain corresponds to a protein sequence motif found in many proteins, including the Notch protein in Drosophila, coagulation factor X, and the LDL receptor. The carboxyl terminus in the EGF-like domain is adjacent to a tandemly repeated pair of 62-amino-acid-long domains. Each of these domains shares primary sequence similarity to a sequence motif in decay accelerating factor, complement receptor I, and the receptor for the complement component C3d, among others. This motif is called the CR repeat (complement-regulatory or consensus repeat) because of its frequent occurrence in proteins that regulate the complement pathway. The CRD, EGF-like, and CR domains of L-selectin are in turn attached to a transmembrane segment linked in turn to a short cytoplasmic domain. Analyses of the genomic structures of the human and mouse L-selectin loci indicate that the signal peptide sequence, the CRD, the EGF domain, each CR repeat, and the transmembrane segment are encoded by discrete exons. The cytosolic domain is encoded by two exons. The human L-selectin locus resides on chromosome 1 between bands q22 and q25, at a substantial distance from the genes encoding several other CR repeat-containing proteins that localize to band 1q32. As noted below, the E-selectin and P-selectin loci are organized similarly to the L-selectin locus and are closely linked to this locus.
The functional contributions of each domain within L-selectin have been examined using antibody blocking approaches and recombinant L-selectin chimeras. In general, these studies confirm that the CRD in L-selectin is directly involved in adhesion interactions with glycan-based ligands, including especially amino acids within the carboxy-terminal portion of the CRD. Such studies also indicate that the EGF-like domain plays an important part in helping to maintain the CRD in its proper configuration for ligand recognition. The two CR repeat segments within L-selectin are required to maintain optimal recognition of its ligands.
L-selectin is expressed on blood monocytes, blood neutrophils, subsets of natural killer cells, and T and B lymphocytes, including virtually all lymphocytes of the “naive” phenotype, both in blood and in lymphoid organs. L-selectin is generally not expressed by lymphocytes that exhibit the “memory” phenotype. L-selectin is also expressed in both early and mature hematopoietic cells in the bone marrow, except that cells of the B-lymphocyte lineage seem to express this molecule only near the latter stages of the B-lineage developmental program.
L-selectin is a glycoprotein that varies in size according to the type of leukocyte in which it is produced. These observations imply that lineage-specific glycosylation processes regulate glycan structures associated with L-selectin. L-selectin is shed from the surface of leukocytes when they are activated, and this shedding involves proteolytic cleavage immediately adjacent to the outer surface of the cell membrane, between residues 283 and 284 of the mature protein. L-selectin shedding is catalyzed by one or more metalloproteinases (secretases), including the tumor necrosis-α converting enzyme. There is evidence that calmodulin regulates the protease-dependent shedding process. It has been proposed that L-selectin shedding serves to facilitate release of adhesion of cells from the endothelium during the transmigration process, but evidence also exists to the contrary, leaving this issue unresolved. The soluble form of L-selectin ocurs in serum from normal and diseased individuals. The functional significance of the circulating form of this molecule is not clear.
Adhesion mediated by L-selectin is characterized by interactions of apparently low affinity (0.1 mm range), exhibiting relatively rapid “on-rate” and “off-rate” characteristics. These adhesive interactions operate predominantly under conditions of vascular shear flow. Similar considerations apply to the cell adhesion processes that involve E-selectin and P-selectin, although L-selectin exhibits an interesting “shear-dependent” cell adhesion. Selectin-dependent cell adhesion in vivo is characterized by “rolling” of leukocytes along the surface of the endothelial cell monolayer. This process is mediated by torque exerted on the leukocyte as a consequence of a force differential between the more rapidly flowing stream near the center of the vessel (and on the luminal surface of the rolling leukocyte) and the relatively slow flow near the endothelial cell surface (and the adjacent abluminal side of the rolling leukocyte). These forces, together with the relatively rapid on and off rates of selectin-selectin ligand adhesive interactions occurring at the leukocyte-endothelial cell interface, cause the leukocyte to roll along the endothelial lining of the vessel.
L-selectin-dependent lymphocyte rolling on HEVs and the corresponding process that leads to lymphocyte homing in vivo require localization of L-selectin to the tips of the microvilli characteristic of the surfaces of lymphocytes and other leukocytes. Microvillus localization seems especially important for L-selectin-dependent tethering events involved in the initiation of rolling. L-selectin-dependent rolling activity is also dependent on an association between the cytosolic domain of L-selectin and the actin cytoskeleton, although this is apparently not related to ligand recognition or to microvillus-sorting processes. The cytosolic domain of L-selectin is also apparently involved in signal transduction processes, since cross-linking of L-selectin, or ligation of L-selectin by multivalent ligand analogs, can lead to intracellular phosphorylation events implicated in signal transduction processes in other systems. The relevance of these observations to leukocyte trafficking and effector cell function remains to be discovered.
L-Selectin Ligands on High Endothelial Venules (13–31)
Glycan-based, HEV-borne molecules recognized by lymphocyte L-selectin have been characterized using a variety of biochemical and cell biological approaches, including purification of such ligand molecules using L-selectin immunoglobulin chimeras, followed by characterization of their polypeptide components through molecular cloning, and analysis of their glycan components through oligosaccharide structural analyses and monoclonal-antibody-staining approaches. Early efforts demonstrated that the molecules elaborated by HEVs, and precipitable by L-selectin-Ig chimeras, represented glycoproteins whose component glycan moieties were most likely serine/threonine-linked and were modified by sulfate, fucose, and sialic acid. Several apparently distinct, L-selectin-reactive polypeptides were seen in these studies, implying the existence of multiple glycoprotein ligands for L-selectin.
Biochemical and molecular cloning approaches have now defined four distinct, HEV-borne glycoproteins that are recognized by L-selectin. The first of these to be isolated, termed GlyCAM-1, for glycosylation-dependent cell adhesion molecule, is a protein of 132 amino acids containing numerous serine and threonine residues. The predicated molecular mass of this protein, 14,154 daltons, is significantly less than the size observed with electrophoretic methods with the glycoprotein (50 kD). GlyCAM-1 corresponds to the L-selectin-Ig-precipitable molecule termed Sgp50. GlyCAM-1 is elaborated as a soluble molecule by HEVs in organ cultures, and immunoelectron microscopy studies do not identify significant amounts of GlyCAM-1 at the HEV cell surface. These observations implied that O-glycans contribute substantially to the apparent molecular mass of GlyCAM-1, and further imply that the molecule is a mucin-type glycoprotein. These observations are consistent with the fact that the sequence of GlyCAM-1 does not predict a transmembrane segment, suggesting that any association between GlyCAM-1 and the HEV cell surface must be mediated by virtue of a 21-residue amphipathic helix at its carboxyl terminus, or more likely by interactions with one or more other membrane-tethered molecules. GlyCAM-1 is detectable in serum and has been proposed to regulate the adhesive and/or activation state of L-selectin-bearing leukocytes through interactions with L-selectin on such cells.
A second L-selectin ligand expressed by HEV is CD34, which corresponds to the L-selectin-Ig-precipitable molecule termed Sgp90. CD34 is a sialomucin containing several mucin-like domains with numerous serine and threonine residues predicted to be heavily O-glycosylated. Since CD34 is a transmembrane glycoprotein, this L-selectin ligand is positioned to contribute substantially to the process of tethering and adhesion of circulating lymphocytes to lymph node HEVs. However, CD34 is clearly not the sole contributor of L-selectin ligand activity in HEVs, since CD34-null mice maintain virtually normal L-selectin-dependent lymphocyte homing activity. CD34 is expressed elsewhere, including on vascular endothelial cells, but does not function as an L-selectin ligand at these locations because it is not appropriately posttranslationally modified.
A third molecule identified as an L-selectin ligand is termed MAdCAM-1, for mucosal addressin cell adhesion molecule. MAdCAM-1 had been studied previously as the ligand for the integrin-type lymphocyte homing receptor α4β7, a molecule responsible for the bulk of lymphocyte homing to Peyer's patches. Biochemical experiments indicate that MAdCAM-1 expressed in mesenteric lymph nodes is decorated with O-glycans that can support L-selectin-dependent leukocyte adhesion. MAdCAM-1 is therefore predicted to maintain ligand activity for both L-selectin and the α4β7 integrin.
A fourth HEV-expressed L-selectin ligand is the transmembrane sialomucin podocalyxin-like protein or PCLP. Like CD34, PCLP is expressed on some vascular endothelia, but it is also expressed on the foot processes of glomerular podocytes. HEV-derived PCLP interacts with recombinant L-selectin-Ig chimera and supports L-selectin-dependent lymphocyte adhesion under physiological conditions.
As noted above, early biochemical studies provided indirect evidence that L-selectin ligands required sulfate and sialic acid moieties for proper function. For instance, broad-spectrum sialidases inactivate L-selectin ligand activity in a variety of in vitro and in vivo assays, and the use of a sialidase specific for α2–3-linked sialic acid residues (Newcastle disease virus neuraminidase) inactivates ligand activity displayed by GlyCAM-1. These studies implied that sialic acid in α2–3 linkage is required for L-selectin ligand activity. A requirement for sulfation was inferred by studies in which chlorate was used to inhibit sulfation in organ cultures of peripheral lymph nodes, from which GlyCAM-1 was then purified. The resulting nonsulfated GlyCAM-1 was not bound by an L-selectin-Ig chimera. (Chlorate competitively blocks synthesis of PAPS, the donor for all known sulfotransferase reactions in cells.)
Direct biochemical analysis of the O-glycans on GlyCAM-1 yielded a series of candidate O-glycan “capping” structures implicated in L-selectin recognition. The proposed structures correspond to Core-2-type O-glycans with the terminal structures 6-sulfosialyl Lex, 6′-sulfosialyl Lex, and 6,6′-bis sulfosialyl Lex (Figure 26.2).
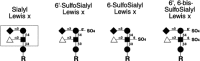
Figure 26.2
Candidate “capping” structures for the glycans of HEV-borne L-selectin ligands.
Evidence supporting the hypothesis that one or more of these molecules are indeed essential, or at least important, contributors to L-selectin ligand activity comes from studies involving inhibition of L-selectin-dependent adhesion using these structures or their variants. Supporting evidence also derives from studies using monoclonal antibodies that recognize these structures and mice lacking specific glycosyltransferases (and see below). In vitro studies that assess the relative ability of these structures, and related ones, to block L-selectin-dependent recognition of its ligand(s) have yielded conflicting results over the relative inhibitory “potencies” of 6′-sulfosialyl Lex, 6-sulfosialyl Lex, and some structural analogs of the 6′,6-sulfosialyl Lex moiety. However, studies using monoclonal antibodies directed against these structures, and their isomers, indicate that 6-sulfosialyl Lex is abundantly expressed on human peripheral node HEVs and imply that the 6′-sulfosialyl Lex and the 6′,6-sulfosialyl Lex structures are not necessarily present in substantial amounts on HEVs.
Biochemical analyses and genetic studies have provided evidence for an ordered biosynthetic scheme in the elaboration of these sulfated, sialylated, fucosylated structures (Figure 26.3). Some of the glycosyltransferases participating in this biosynthesis have been defined, but most others remain to be identified (and see further below). This scheme begins with Core 2 O-glycan branch formation, followed by lactosamine unit addition. There is evidence that 6-O sulfation of the subterminal GlcNAc residue precedes addition of β1–4-linked galactose. Addition of 6-O-linked sulfate to the terminal galactose residue and addition of α2–3-linked sialic acid to this galactose moiety are presumed to occur subsequently, but the relative order of their addition is not known. It is generally accepted that the addition of fucose in α1–3 linkage represents a terminal step in the pathway. The relative contributions of each of these three proposed structures to physiological L-selectin-dependent adhesion events in HEVs are not yet clear. Since the HEV-borne L-selectin ligands each displays multiple O-glycans, it is possible that each of these three structures exists on all four ligands and may substitute the component glycans at multiple sites, possibly at positions that are idiosyncratic to the individual structure, with the attendant possibility for functional importance. Detailed structural analyses and corresponding functional correlations are needed to clarify these issues.
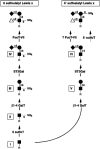
Figure 26.3
Proposed biosynthetic scheme for “capping” structures shown in Figure 26.2. A Core-2-type O-glycan (R), containing a terminal N-acetylglucosamine residue (I), serves as the synthetic precursor for both capping structures. The pathway leading (more...)
L-Selectin-dependent Leukocyte Adhesion to Microvascular Endothelium (32–42)
L-selectin also has a role in adhesion of neutrophils, eosinophils, and monocytes to nonlymphoid vascular endothelium (Figure 26.4). Much of the evidence for this process stems from in vitro and in vivo observations showing that anti-L-selectin antibodies can block flow-dependent rolling of leukocytes on activated vascular endothelium. At least three different mechanisms have been proposed to account for this process. The first mechanism proposes that neutrophil-borne L-selectin displays glycan ligands for E-selectin, which is expressed by the activated, pro-adhesive endothelial cell. As discussed later in this chapter, E-selectin mediates adhesion of neutrophils to activated vascular endothelium by recognition of sialylated, fucosylated glycoconjugates expressed by neutrophils. L-selectin expressed by human neutrophils is decorated with sialylated, fucosylated glycans within the family of such molecules implicated in E-selectin ligand activity. These considerations imply that a component of the L-selectin-dependent rolling processes observed in vitro, and in vivo, may be accounted for by “presentation” of E-selectin ligands by neutrophil L-selectin.
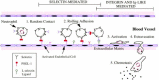
Figure 26.4
Rolling adhesion of circulating leukocytes to activated endothelium via interactions between selectins and their ligands. The example shows a neutrophil expressing PSGL-1 and L-selectin on its microvilli and interacting by random contact with membrane (more...)
Evidence also exists that the endothelial cell displays molecules that directly mediate L-selectin-dependent adhesion. Biochemical analyses disclose that a substantial proportion of such L-selectin ligand activity is Ca++dependent and is contributed by heparan sulfate proteoglycans. These molecules may contribute to L-selectin-dependent adhesion of monocytes and neutrophils, and possibly other leukocytes. The structure of such molecules and the regulation of their expression are not yet well defined.
The third mechanism through which L-selectin can mediate adhesion of neutrophils and other leukocytes to nonlymphoid vascular endothelium involves leukocyte-leukocyte interactions. Observations using an in vitro flow chamber disclosed that adherent neutrophils can support L-selectin-dependent rolling of other leukocytes. These initial studies implied that the adherent leukocytes represent a stationary surface that displays L-selectin ligand activity. Such activity was sialidase-sensitive, temporally transient, and operative in a unidirectional manner characterized by adhesion between L-selectin on the rolling leukocyte and the ligand on the adherent leukocyte. These observations implied that neutrophils display both L-selectin and L-selectin ligands and would seem to predict L-selectin-dependent leukocyte aggregation in vivo. However, L-selectin-dependent adhesion is only evident under certain conditions of shear flow, accounting for the general absence of L-selectin-dependent leukocyte agglutination in vivo. The apparently unidirectional nature of the L-selectin-dependent leukocyte-leukocyte rolling phenomenon noted in the initial in vitro observations may be accounted for by the relative absence of L-selectin on the adherent neutrophils, consequent to activation-dependent shedding of L-selectin from those cells. However, it is now clear that L-selectin, displayed as a chimeric molecule or by adherent cell monolayers, can initiate tethering and capture of incoming leukocytes under shear, indicating that L-selectin-dependent tethering and capture of neutrophils by other neutrophils can be a bidirectional process. An important component of neutrophil ligands for L-selectin is the molecule PSGL-1 (P-selectin glycoprotein ligand-1), a mucin-type sialylated, fucosylated glycoprotein that is discussed subsequently in this chapter. There is also evidence that leukocytes express other mucin-type ligands for L-selectin that are distinct from PSGL-1. Although it is clear that sialic acid residues are clearly important for such L-selectin ligand activities, important contributions by other glycan components may also exist, but they have not yet been defined.
Functions for L-selectin in vivo have been explored through the generation and analysis of mice that are homozygous for null alleles at the L-selectin locus. These mice have a modest defect in neutrophil trafficking through extravascular sites in the context of inflammation, which is consistent with roles proposed for neutrophil L-selectin in adhesion to vascular endothelium and to adherent neutrophils. These mice are also deficient in the homing of naive, L-selectin-positive T lymphocytes to peripheral and mesenteric lymph nodes. The lymph nodes in these mice are consequently small and relatively deficient in naive T lymphocytes. This deficit leads to an inability of these mice to mount a delayed contact hypersensitivity response.
E-Selectin Structure and Regulation (5,8,13,43–44)
The sequence of the E-selectin cDNA predicts a type-1 transmembrane glycoprotein with a CRD, EGF-like domain, and four CR repeat domains (see Figure 26.1). A recombinant fragment of E-selectin composed of the CRD and EGF-like domain has been crystallized and its structure solved. The E-selectin CRD superimposes substantially on the CRD of the mannose-binding protein but differs from the latter molecule in that the E-selectin CRD coordinates a single calcium ion, whereas the mannose-binding protein and other crystallized C-type lectins coordinate two calcium ions. Furthermore, the segment of the E-selectin CRD that coordinates calcium differs from that found in the mannose-binding protein. Mutagenesis of the E-selectin CRD and the CRDs of L-selectin and P-selectin localizes shared and distinct amino acids associated with binding of small carbohydrate ligands. These residues typically map within the vicinity of the calcium-coordinating site of the protein. Positively charged residues invariant among the three selectins have also been disclosed by such mutagenesis analyses and are proposed to interact with sialic acid or fucose, which make essential contributions to selectin ligand affinity, as discussed below.
As noted above for L-selectin, the E-selectin CRD, EGF-like, CR repeat, and transmembrane domains are each encoded by separate exons, whereas the E-selectin cytosolic domain derives from two exons. The E-selectin locus flanks the L-selectin locus on chromosome 1.
In primates and rodents, there is little, if any, constitutive expression of E-selectin by the vascular endothelium. Cytokine-dependent transcriptional processes lead to an inducible expression of E-selectin at the surface of the endothelium. Inducible transcription of the E-selectin locus is mediated by TNF-α, IL1-β, and lipopolysaccharide, for example, and is mediated at least in part through NF-κB-dependent events. Cytokine-dependent regulation of the E-selectin locus yields E-selectin expression beginning approximately 2 hours after cytokine treatment, with maximal expression at approximately 4 hours. In vitro, E-selectin expression declines to initial levels within 12 hours, but it may be expressed chronically at sites of inflammation in vivo. Decline of E-selectin expression is associated with decreased transcription of the E-selectin locus, degradation of the E-selectin transcripts, and internalization and degradation of E-selectin molecules by the endothelium. Soluble forms of E-selectin are detectable in the serum, and increased levels of circulating E-selectin have been associated with acute and chronic inflammatory states. The functional relevance of soluble E-selectin in the blood is unknown. Acute and chronic inflammatory conditions associated with E-selectin expression include, for example, sepsis, kidney inflammation, rheumatoid arthritis, and organ transplantation.
E-Selectin Ligands (43–46)
E-selectin ligands are expressed by neutrophils, monocytes, eosinophils, memory-effector T-like lymphocytes, and natural killer cells. Each of these cell types is found in acute and chronic inflammatory sites in association with expression of E-selectin, thus implicating E-selectin in the recruitment of these cells to such inflammatory sites. Efforts to assign a causal relationship to this association have included the generation and analysis of mice homozygous for null alleles at the E-selectin locus. These mice maintain virtually normal leukocyte recruitment in a peritoneal exudate model and in a delayed-type hypersensitivity model, both of which measure recruitment of neutrophils and other leukocytes in the context of acute inflammation. However, a leukocyte-trafficking defect in these mice is unmasked by administration of a blocking monoclonal antibody against P-selectin, a treatment that does not yield a leukocyte-trafficking defect in wild-type animals. These observations imply that both E- and P-selectin maintain an apparent functional redundancy, at least in mice, in the limited circumstances where inflammation has been carefully examined. Nevertheless, other animal studies using blocking anti-E-selectin antibodies have shown that E-selectin can provide an essential, nonredundant contribution to leukocyte trafficking in acute inflammation.
The presence of a CRD in E-selectin suggested that it recognizes glycoconjugate-based leukocyte ligands. This possibility was supported by the close structural similarity between E-selectin and L-selectin and by studies implying that L-selectin recognizes carbohydrate-dependent ligands. Clues to the identity of E-selectin ligands could be found among the glycans expressed by the types of leukocytes recognized by E-selectin (e.g., neutrophils), but not on blood cells that do not interact with E-selectin (e.g., red blood cells). Glycan structural analyses on myeloid lineage cells and erythroid lineage cells had previously identified a group of fucosylated, sialylated oligosaccharides absent from erythroid cells, but abundant on myeloid cells. These glycans could be isolated from the glycoprotein and glycolipid components of the myeloid cell membranes and included polylactosamine chains modified by terminal or subterminal substitution with α2–3-linked or α2–6-linked sialic acid residues. Myeloid lineage specificity on such structures was conferred by the presence of α1–3-linked fucose residues absent from red cell glycans. The prototype for these myeloid-specific structures is the sialyl Lex tetrasaccharide (Figure 26.5). This tetrasaccharide is representative of a family of α2–3-sialylated α1–3-fucosylated glycans that exist on N-glycans, on lipid-linked glycoconjugates, and on O-glycans. The latter set includes Core-2-based lactosamine and polylactosamine chains. The polylactosamine chains representative of these molecules may be fucosylated variably, at one or more GlcNAc residues in the polymer.
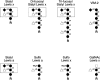
Figure 26.5
Structures of all fucosylated, sialylated oligosaccharides that have been shown to mediate E-selectin-dependent cell adhesion. These include sLex, difucosylated sLex, sLea, 3′sulfo Lex, 3′sulfo Lea, “GalNAc-Lex (also termed the (more...)
Evidence supporting a role for such molecules in E-selectin-dependent leukocyte adhesion is derived from studies in which soluble forms were tested in vitro for their ability to inhibit such adhesion. Similarly, gene transfer approaches have been used to force the expression of these glycan structures on various cultured cell lines that point to the sialyl Lex structure and its variants as important components of E-selectin ligands. Considered together, this rather large body of data indicates that the sialyl Lex and sialyl Lea structure and some (but not all) sulfated forms of these molecules can function in vitro as E-selectin ligands (Figure 26.5). These studies also indicate that the unsialylated Lex or Lea structures do not support E-selectin-dependent cell adhesion, nor does the Lex structure artificially substituted with α2–6-linked sialic acid. Type-2 lactosamine chains with a terminal α2–3-linked sialic acid residue singly substituted with α1–3-linked fucose on an internal GlcNAc residue (VIM-2; Figure 26.5) do support the E-selectin-dependent cell adhesion under some in vitro circumstances.
Assignment of the sialyl Lex tetrasaccharide and/or its structural variants as “ligands” for E-selectin is not as straightforward as originally envisioned. Specifically, consideration must be given to discriminating between structures that can support E-selectin-dependent cell adhesion in vitro and those that actually do support this process in vivo. Clearly, this issue depends also on the nature of the in vitro assays used to characterize putative E-selectin ligands. In this regard, it is important to point out that the sialyl Lea and sulfated forms of sialyl Lex and sialyl Lea are not known to be found on leukocytes, implying that they do not participate in E-selectin-dependent leukocyte adhesion events, even though they can clearly support E-selectin binding in the laboratory. It is also important to note that although monoclonal antibodies directed against such structures have played an important part in establishing correlations between structures and E-selectin ligand “activity,” such antibodies generally only identify surrogate markers for E-selectin ligand activity. For example, the monoclonal antibodies CSLEX-1 and HECA-452 recognize epitopes on leukocytes that are strongly correlated with E-selectin activity. However, HECA-452 clearly reacts with the sialyl Lea structure, whereas CSLEX-1 does not. Similarly, skin-homing memory T cells that are known to express E-selectin ligands do not react with the CSLEX-1 antibody, but they do bind the HECA-452 antibody. Thus, it is important to try to purify physiological E-selectin ligands from leukocytes, to characterize the composition and structure of their glycan components, and to evaluate the contribution of each of the glycan components to E-selectin adhesion activity.
Such studies have assigned E-selectin ligand function to at least three different glycoproteins. The first of these, PSGL-1, which was discussed above as an L-selectin ligand and is clearly also a ligand for P-selectin, may serve as a “scaffold” to present α2–3-sialylated, α1–3-fucosylated glycans to E-selectin. Importantly, PSGL-1 will not support E-selectin-dependent binding interactions if it is not modified by α2–3 sialylation or α1–3 fucosylation. Furthermore, PSGL-1 is clearly not required for E-selectin ligand activity in some leukocyte cell lines, although it is possible that this mucin-like molecule may contribute to E-selectin ligand activity in leukocytes in vivo.
A second candidate E-selectin ligand is a glycoprotein purified from bovine γ/δ T lymphocytes. This protein has an unreduced molecular mass of 250 kD, and may also function as a ligand for P-selectin. Although this molecule is apparently not the bovine homolog of PSGL-1, its structure and other functional properties remain to be explored.
A third E-selectin ligand candidate, termed ESL-1, has been purified from murine myeloid lineage cells. ESL-1 corresponds to a splice variant of a murine homolog of a previously studied chicken FGF-binding protein. ESL-1 displays N-glycans with E-selectin ligand activity, and O-glycans are not involved. This molecule has been found both in the Golgi and on microvilli of the cell surface.
Glycolipids have also been assigned E-selectin ligand activity. Experiments in which myeloid cell glycolipids have been purified and fractionated identify α2–3-sialylated α1–3-fucosylated gangliosides that support E-selectin-dependent cell adhesion. These structures are characterized by α1–3 fucosylation at internal GlcNAc residues, in the absence of α1–3 fucosylation of the most terminal GlcNAc residue, i.e., VIM-2 structures. In contrast, glycolipid molecules whose terminal GlcNAc residue is α1–3-fucosylated do not exhibit E-selectin ligand activity. As with the studies involving candidate glycoprotein ligands for E-selectin, it is important to note that assay of these glycolipid structures for E-selectin ligand activity involves in vitro biochemical procedures that do not necessarily reflect physiological circumstances. In vivo studies that support a role for these glycolipids and selectin ligand activity are not yet available.
P-Selectin Structure and Expression (6–8,47–55)
Molecular cloning studies have defined the amino acid sequence of P-selectin and the genomic organization of the P-selectin locus. P-selectin shares the domain structure described for E-selectin and L-selectin, consisting of a single peptide, a CRD, a single EGF-like motif, nine CR repeats in human P-selectin, a single transmembrane segment, and a short cytosolic domain (see Figure 26.1). Each of these domains corresponds to a single exon at the P-selectin locus, excepting the cytosolic domain, which is derived from two different exons. This organization mirrors those defined for the E-selectin and L-selectin molecules. The P-selectin locus is adjacent to the L-selectin locus on human chromosome 1.
P-selectin is found within the Weibel-Palade bodies of endothelial cells and in α-granules of platelets. Sequences within the cytoplasmic domain of P-selectin apparently mediate sorting to the granule, through interactions with molecules that direct this process. Cell surface expression of P-selectin is generally transient and is a consequence of rapid endocytotic events mediated through the protein's cytoplasmic tail. Splice variants of P-selectin transcripts yield forms of P-selectin that are without a transmembrane domain, accounting for the fact that some P-selectin is released as a soluble form.
The cytosolic tail of P-selectin undergoes phosphorylation at specific threonine, tyrosine, and histidine residues. The P-selectin cytosolic tail is also myristoylated at a single cysteine in this domain. The functional relevance of these posttranslational modifications has not yet been defined. P-selectin expression is also under transcriptional control, with induction by TNF-α, IL1-β, or LPS. The time course of expression of P-selectin in these contexts is similar to that observed for E-selectin.
P-selectin contributes to leukocyte recruitment in a variety of acute and chronic inflammatory contexts. Its role in leukocyte recruitment and inflammation is a function of both acute and chronic expression of P-selectin by endothelium and by platelet activation-dependent P-selectin expression. The role of this molecule in leukocyte recruitment in the context of acute inflammation is well illustrated by analysis of mice that are homozygous for null P-selectin alleles. These mice exhibit a substantial delay in neutrophil recruitment in the context of acute inflammation, relative to wild-type mice, indicating that P-selectin plays an important part in the early phases of leukocyte recruitment. P-selectin can also contribute to chronic inflammation, presumably through transcriptional up-regulation of its expression during the latter stages of an inflammatory response. For instance, P-selectin-deficient mice exhibit delayed trafficking of monocytes to an inflamed peritoneal space during the most acute phase of this inflammatory model. These observations imply that transcription-dependent expression of P-selectin at later times normally contributes to monocyte recruitment. Similarly, T lymphocytes exhibit a requirement for P-selectin when trafficking to skin in the context of chronic inflammation.
Platelet-derived P-selectin also contributes to leukocyte trafficking, as well as to wound healing and blood clotting. For example, activated platelets adhere to neutrophils, monocytes, natural killer cells, and some subsets of T lymphocytes. This adhesion is mediated through P-selectin, in part, and represents a mechanism to augment the recruitment of leukocytes and platelets to sites of vascular compromise. Platelet-derived P-selectin may also contribute to hemostatic processes by its ability to stimulate monocytes to express tissue factor and by facilitating fibrin deposition during clot formation. The inferred role for platelet-derived P-selectin in hemostatic processes is not completely consistent with the generally mild hemostatic defect observed in P-selectin null mice, and this issue remains to be explored further.
P-Selectin Ligands (56–86)
P-selectin binding to cells is inhibited by many different anionic compounds, including sulfated glycoconjugates (e.g., heparin and sulfatide) and phosphorylated compounds (e.g., Man-1-P). These early observations led to the belief that P-selectin might be highly undiscriminating in the types of ligands it recognizes. Remarkably, leukocytes express only one glycoprotein that binds with high affinity to P-selectin, and that is PSGL-1, which is a relatively minor mucin on leukocyte surfaces (Figure 26.6A). The identification and characterization of a single specific receptor for P-selectin were especially surprising, given the view up to recent times that most lectins only recognize carbohydrate determinants (or modifications of the carbohydrate) and the concept that typical carbohydrate determinants in a cell are expressed on a multitude of glycoproteins.
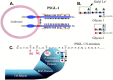
Figure 26.6
(A) Predicted disulfide-bonded dimeric form of PSGL-1 in leukocytes. The multiple O-glycan and three potential N-glycans on the molecule are indicated. (B) Two major fucose-containing Core 2 O-glycans identified in PSGL-1. The sialyl Lex determinant in (more...)
The binding of P-selectin to neutrophils, however, is abolished upon sialidase treatment, which suggested originally that sialic acid may be a critical determinant required for P-selectin recognition. The possible importance of the sialyl Lex moiety to P-selectin recognition was derived from experiments showing that (1) P-selectin binds to a mutant CHO cell line expressing the sialyl Lex moiety, but not to cells lacking the epitope; (2) antibodies to the sialyl Lex epitope, but not the Lex epitope, can block binding of cells to P-selectin; and (3) glycans containing the sialyl Lex moiety inhibit P-selectin-mediated adhesion, and P-selectin binds directly to immobilized glycans containing the sialyl Lex epitope. However, the binding of P-selectin to neutrophils displays much higher affinity interactions than to nonmyeloid cells, even those expressing sialyl Lex, originally suggesting that myeloid cells possess a unique ligand(s) for P-selectin. Using 125I-labeled P-selectin blotting and affinity chromatography on immobilized human P-selectin, a glycoprotein ligand for P-selectin was identified and purified, starting with total membrane glycoproteins extracted from human neutrophils and the human promyelocytic cell line HL60. The purified ligand, now known as PSGL-1, behaves as a disulfide-bonded approximately 250-kD protein in nonreducing SDS-PAGE and approximately 120-kD in reducing SDS-PAGE. In contrast to other selectin ligands, PSGL-1 binding to P-selectin is characterized by high-affinity binding (Kd in the range of 100 nm) and very fast association and dissociation rates.
The cDNA encoding PSGL-1 was expression-cloned in COS cells transfected to coexpress an α1–3(4)-fucosyltransferase (human FucT-III), which allows both sialyl Lex and sialyl Lea synthesis in these cells. The sequence of PSGL-1 predicts a protein of 412 amino acids with an 18-amino-acid signal sequence and a tetrapeptide consensus cleavage site for a paired basic amino-acid-converting enzyme in leukocytes at residues 38–41 (-R-D-R-R). Thus, the amino terminus of the mature protein begins at residue 42. There are 16 decapeptide repeating units with the consensus sequence -A-T/M-E-A-Q-T-T-X-P/L-A/T- spanning residues 118–277 in the long form of the protein, and the short form is missing the residues 132–141. A single cysteine residue occurs at position 320 in the extracellular domain preceding the predicted single transmembrane-domain-spanning residues 321–341 and the cytoplasmic domain of residues 342–412. The coding region for human PSGL-1 is contained entirely in exon 2 of the gene, which maps to chromosome 12q24. The cDNA for the murine PSGL-1 encodes a predicted 397-amino-acid protein that has recognizable homology with the human sequence. The murine protein contains a predicted signal sequence and propeptide identical in size to that of human PSGL-1; the mature murine PSGL-1 is also predicted to begin at residue 42. However, the mouse homolog has only ten decameric repeats with the consensus sequence -E-T-S-Q/K-P-A-P-T/M-E-A- that are obviously different in sequence from that of the human PSGL-1. The highest homology between the human and murine PSGL-1 occurs in the transmembrane (83%) and cytoplasmic domains (76%).
Each subunit of human PSGL-1 contains 70 serine and threonine residues in the extracellular domain that are potential sites for O-glycosylation and three potential sites for N-glycosylation. The murine PSGL-1 also contains numerous extracellular serine and threonine residues and two potential sites for N-glycosylation. The murine PSGL-1 also contains a single unpaired extracellular cysteine at residue 307 that preceeds the predicted transmembrane domain. Interestingly, human PSGL-1 contains three predicted tyrosine sulfation sites at residues 46, 48, and 51 that fall in the consensus sequence in which tyrosine residues are flanked by acidic residues. The murine PSGL-1 contains two predicted tyrosine sulfation sites at residues 54 and 56.
The large size and extensive glycosylation of PSGL-1 present a daunting challenge to understanding how it is specifically recognized by P-selectin. It was anticipated at first that PSGL-1 might be a high-affinity and unique ligand for P-selectin by virtue of its mucin-like nature and the presumption that the ligand contained large amounts of the sialyl Lex antigen, thereby enhancing its avidity for P-selectin. However, as discussed below, this prediction was not correct.
Treatment of purified PSGL-1 with sialidase abolishes its binding to P-selectin, but treatment of neutrophil-derived PSGL-1 with peptide N-glycosidase F, which removes most, if not all, N-glycans on the molecule, does not affect its recognition by P-selectin. Such results suggested that sialylated O-glycans, rather than N-glycans, are important determinants for P-selectin recognition. The involvement of O-glycans was supported by the observation that treatment of either neutrophils or purified PSGL-1 with the O-sialoglycoprotease from Pasteurella hemolytica, an enzyme that degrades sialylated mucins, blocks binding of the cells or ligand, respectively, to P-selectin. In addition, when HL60 cells are treated with benzyl-α-GalNAc, which inhibits extension of O-glycans, the cells bind less to P-selectin. Other studies demonstrated that treatment of isolated PSGL-1 with endo-β-galactosidase, a bacterial endoglycosidase capable of degrading type-2 polylactosamine repeats [-3Galβ1–4GlcNAcβ1-]n, significantly reduces binding to P-selectin, thus indicating that polylactosamine, presumably on O-glycans, may also be important for binding.
The structures of the O-glycans of native PSGL-1 purified from human HL60 cells reveal that most contain a simple Core 2 structure with one or two sialic acid residues and generally lack fucose residues. Fucose is present in only two relatively minor O-glycans, termed glycan 1 and glycan 2 (Figure 26.6B). Both glycans 1 and 2 contain the sialyl Lex antigen; however, glycan 1, but not glycan 2, contains a polylactosamine on the Core 2 structure with multiple fucose residues. The possible synthesis of glycan 1 is discussed below (Figure 26.7). Thus, the O-glycans of PSGL-1 generally lack fucose and only a few O-glycans display the sialyl Lex antigen, presumed to be important in P-selectin binding. The fact that glycan 2 is predicted to occur in substoichiometric quantities led to the expectation that glycan 1 may be the more important O-glycan for P-selectin recognition, but this is not yet clear. The glycosyltransferases participating in P-selectin ligand formation are discussed in the next section.
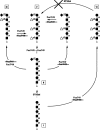
Figure 26.7
Synthetic scheme for synthesis of sialyl Lex, difucosylated sialyl Lex, Lex, and VIM-2. A polylactosamine chain (I) displayed by O-linked, N-linked, or lipid-linked glycoconjugates (R) serves as the precursor for the pathways. One important example of (more...)
Although proper glycosylation of PSGL-1 is required for its binding to P-selectin, other studies suggested the sulfation is also important. Treatment of purified PSGL-1 with a bacterial aryl sulfatase, which removes sulfate from tyrosine residues in PSGL-1, abrogates binding of the molecule to P-selectin. Furthermore, recombinant forms of PSGL-1 in which the three tyrosine residues, which occur in a putative tyrosine sulfate motif at the extreme amino terminus of the molecule, have been changed to phenylalanine also fail to bind P-selectin. In addition, treatment of cells with sodium chlorate also blocks expression of a functional PSGL-1 molecule.
The critical binding domain appears to reside in the extreme amino terminus of PSGL-1. Several different lines of evidence are consistent with this possibility: (1) A blocking monoclonal antibody (PL1) was developed that recognized a peptide epitope overlapping the tyrosine sulfate consensus sites, whereas nonblocking monoclonal antibodies (e.g., PL2) mapped to epitopes outside this region. The PL1 monoclonal antibody prevents neutrophil, monocyte, eosinophil, and lymphocyte adhesion and rolling on P- and L-selectin. (2) Chimeric glycoproteins containing the extreme amino-terminal domain of PSGL-1, when produced in cells expressing appropriate glycosyltransferases, are recognized by P-selectin. (3) Treatment of neutrophils with mocarhagin, a cobra venom metalloproteinase, removes the extreme amino-terminal ten amino acid residues from PSGL-1 and abrogates its binding to P-selectin. Such data point to a model in which the combination of tyrosine sulfate residues and oligosaccharides on the protein are required for high-affinity binding to P-selectin (Figure 26.6C).
Another approach to explore the fine structure of the PSGL-1 amino-terminal domain required for P-selectin recognition is selective mutagenesis of amino acids in that domain and assessment of the binding of recombinant PSGL-1 to P-selectin. Replacement of all three amino-terminal tyrosine residues with phenylalanine abolishes binding of the recombinant PSGL-1 to P-selectin, but not E-selectin. Interestingly, only one of the three potential tyrosine sulfate residues is absolutely necessary for binding to P-selectin. Even more surprising is the finding that replacement of threonine at position 57 with alanine blocks binding of the recombinant molecule to P-selectin. These data support the model in Figure 26.6C, where the C-type lectin domain of PSGL-1 may contact tyrosine sulfate residues and the sialyl Lex structure on a Core 2 O-glycan at Thr-57. However, it must be stressed that this model is only a prediction, and there is no direct evidence that such an O-glycan, as depicted in Figure 26.6C, even occurs at Thr-57 in PSGL-1, nor is there yet any direct proof that there is coordinate binding of such an O-glycan and one or more tyrosine sulfates.
The specific in vivo functions of PSGL-1 have been explored using blocking antibodies to the protein and recombinant forms. A blocking monoclonal antibody to PSGL-1 (PL1) and its F(ab) fragments dramatically reduced rolling of human polymorphonuclear neutrophils and HL60 cells in venules of acutely exteriorized rat mesentery, indicating that PSGL-1 is important in vivo for rolling of myeloic cells in mesenteric venules at physiologic shear stress. Another approach to study PSGL-1 function in vivo during inflammation is to explore its role in ischemia/reperfusion injury models, in which blood flow is blocked, thereby stimulating P-selectin expression by endothelial cells. In a rat model of ischemia/reperfusion injury, treatment of animals with recombinant PSGL-1 significantly enhanced rat survival and liver function and recovery. PSGL-1 may also be important for lymphocyte recruitment to sites of inflammation in vivo, since blocking antibodies to mouse PSGL-1 blocks entry of T-helper-1 cells into inflamed areas of the skin during a cutaneous delayed-type hypersensitivity reaction model. There is also evidence that P- and possibly E-selectin are important contributing factors to development of atherosclerotic lesions. Although such studies are beginning to define a role for PSGL-1 in leukocyte functions in vivo, many more studies are needed to understand the involvement of PSGL-1, as opposed to or in concert with other selectin ligands, in the overall response to inflammation.
Glycosyltransferases Involved in Selectin Ligand Biosynthesis (87–100)
Much remains to be learned concerning the structure of the physiological leukocyte ligands for the selectin, but the nature of the genetic loci responsible for synthesis of these structures has become more apparent in recent years. For instance, the identities of the leukocyte α1–3 fucosyltransferases that decorate leukocyte selectin ligands and contribute to selectin ligand activity have been identified from analysis of cells transfected with candidate fucosyltransferase genes and from an analysis of animals in which these genes have been deleted. Similar approaches have identified the role of a Core 2 β1–6 N-acteylglucosaminyltransferase in selectin ligand biosynthesis (see below).
The human genome maintains at least five distinct α1–3 fucosyltransferase loci. Three of these, FucT-III, FucT-V, and FucT-VI, are not apparently expressed in leukocytes or their progenitors, and there is no obvious defect in selectin ligand synthesis in individuals who are homozygous for null alleles at the FucT-III locus or at the FucT-VI locus. In contrast, the α1–3 fucosyltransferases FucT-IV and FucT-VII are expressed in the leukocyte lineages and have represented strong candidates for participation in the fucosylation of E-selectin ligands. Published evidence for a role for FucT-IV in this process is conflicting, since this gene is able to determine expression of E-selectin ligand activity, i.e., sialyl Lex, can in some culture cell lines, but not others. Biochemical analyses indicate that FucT-IV can generate the Lex structure and can generate internally fucosylated products using α2–3-sialylated polylactosamine precursors (Figure 26.7). However, this enzyme is rather ineffective in the formation of the sialyl Lex tetrasaccharide from α2–3-sialylated lactosamine chain precursors, except in the context of its expression in some cultured cell lines.
FucT-IV and FucT-VII may make mutually exclusive contributions to fucosylation of the α2–3-sialylated lactosamine precursors to glycans with selectin ligand activity. These observations further suggest that such distinct fucosylation events may make correspondingly distinct contributions to selectin ligand activity. These ideas have yet to be confirmed in experiments in which selectin ligands bearing glycan structures determined by FucT-IV, or by FucT-VII, or by the two operating together, have been studied with respect to their relative selectin ligand “activity.” From the possible biosynthetic pathway shown in Figure 26.7, FucT-VII is predicted to act cooperatively with FucT-IV to generate a high-affinity selectin ligand, particularly with regard to PSGL-1.
Evidence supporting a central role for the fucose residue in providing L-selectin ligand structures comes from an analysis of mice made deficient in the α1–3 fucosyltransferase, FucT-VII, which is expressed in peripheral node HEVs. Mice homozygous for a FucT-VII null allele are deficient in L-selectin ligand formation in the HEVs. They also exhibit a marked leukocytosis i.e., elevated leukocyte levels, with a deficiency in lymphocyte colonization of the lymph nodes. Moreover, mice lacking FucT-VII are impaired in experimentally induced inflammatory responses by assessing neutrophil recruitment to inflamed peritoneum. HEV-borne L-selectin ligand activity is reduced more than 90% when assessed by immunohistochemical procedures or by in vivo lymphocyte-homing studies, confirming an essential role for α3-linked fucose residues in L-selectin ligand activity. In vitro, FucT-VII effectively utilizes the nonfucosylated 6-sulfated, sialylated precursor to form the 6-sulfosialyl Lex structure. In contrast, the 6′-sulfated, sialylated precursor is not utilized by FucT-VII to form the 6′-sulfosialyl Lex structure. No information is yet available about the ability of FucT-VII to use the bis-sulfated precursor.
There is similarly strong evidence for an essential role for FucT-VII in E- and P-selectin ligand biosynthesis. For example, FucT-VII consistently generates α1–3-fucosylated, α2–3-sialylated epitopes recognized by the CSLEX-1 and HECA-452 antibodies, e.g., when expressed in cultured cell lines with glycosylation phenotypes capable of supporting this synthetic pathway. FucT-VII maintains a rather limited acceptor substrate specificity, in comparison to the other α1–3 fucosyltransferases, since it is unable to utilize neutral precursors to form the Lex antigen, nor is it able to utilize type I precursors to form the Lea or sialyl Lea structure. There is also biochemical evidence that FucT-VII operates exclusively on N-acetylglucosamine residues at the terminus of an α2–3-sialylated polylactosamines, to the exclusion of activity on N-acetylglucosamine residues at more proximal positions.
The recombinant form of PSGL-1 when expressed in CHO cells fails to bind P-selectin. However, if the cells are transfected to coexpress α1–3 fucosyltransferases, which can support sialyl Lex synthesis, and the Core 2 GlcNAcT participating in Core 2 O-glycan formation, the recombinant PSGL-1 efficiently binds to P-selectin. Such results suggested that expression of both the sialyl Lex antigen and Core 2 GlcNAcT is required for PSGL-1 binding to P-selectin. The vast majority of leukocyte binding to P-selectin is lost in mice rendered genetically deficient in FucT-VII.
The relevance of the Core 2 O-glycan structure to selectin ligand formation has been explored in mice that are homozygous for null alleles at the Core 2 GlcNAcT locus. The L-selectin ligand activity of the peripheral lymph node HEVs in these mice is only partially reduced as assessed by immunohistochemical procedures but not functionally affected as judged by normal lymphocyte abundance in the nodes, and in vivo lymphocyte homing assays. The apparent absence of a physiologic contribution to L-selectin ligand activity by the Core 2 GlcNAc linkage may be explained by compensatory processes in the mice and/or by N-linked or lipid-linked (O-sialyloglycoproteinase-resistant) L-selectin ligands that have been described on peripheral lymph node HEVs.
In contrast, neutrophils from Core 2 GlcNAcT-null mice bind poorly to P-selectin, and especially to E-selectin and L-selectin. Although the detailed structures of O- and N-glycans on murine leukocytes have not yet been defined, these studies strongly implicate the Core 2 O-glycans containing the sialyl Lex antigen, as observed on human PSGL-1, as critical determinants for selectin recognition. The inflammation response in Core 2 GlcNAcT-null mice is severely affected, similar to the response deficit in FucT-VII-null mice. A moderate leukocytosis occurs in mice lacking Core 2 GlcNAcT, which is almost completely due to increased levels of neutrophils. The myeloid specificity and nature of the phenotype indicate that selectin ligand function, in either lymphocyte homing or myeloid inflammation, appears segregated by Core 2 O-glycan biosynthesis.
Much remains to be understood regarding the biosynthesis of physiologic selectin ligands. A glycan structural correlate for loss of selectin binding with FucT-VII deficiency in leukocytes of null mice is not yet available. The cDNAs encoding two human tyrosylprotein sulfotransferases capable of sulfating Tyr residues in PSGL-1 have been identified, and a cDNA encoding a GlcNAc 6-sulfotransferase capable of synthesizing 6-sulfosialyl Lex has been identified. However, the nature of other sulfotransferases relevant to selectin ligand biosynthesis is under intense study. Interestingly, the precise nature of the α2–3 sialyltransferase(s) relevant to the biosynthetic scheme in HEVs has not been defined as of this writing.
Future Directions
The identification of selectins as carbohydrate-binding proteins important in regulating leukocyte trafficking and adhesion marked a milestone in the development of the field of glycobiology. Selectins serve as a premier example of the structure-function relationships between glycosyltransferases and their cognate carbohydrate structural products as recognition molecules. In addition, a better understanding of selectin biology is leading to the development of specific pharmaceuticals capable of blocking selectin-mediated adhesive events and represents a major effort in the budding field of glycobiotechnology. It is anticipated that further understanding of the regulated expression of selectins and their ligands and the detailed molecular nature of their interactions will provide new paradigms into how carbohydrate-binding proteins interact with specific glycans to regulate adhesive interactions among blood cells.
References
- 1.
- Stamper H B Jr, Woodruff J J. An in vitro model of lymphocyte homing. I. Characterization of the interaction between thoracic duct lymphocytes and specialized high-endothelial venules of lymph nodes. J. Immunol. 1977;119:772–780. [PubMed: 407305]
- 2.
- Yednock T A, Stoolman L M, Rosen S D. Phosphomannosyl-derivatized beads detect a receptor involved in lymphocyte homing. J. Cell Biol. 1987;104:713–723. [PMC free article: PMC2114559] [PubMed: 2950121]
- 3.
- Lasky L A, Singer M S, Yednock T A, Dowbenko D, Fennie C, Rodriguez H, Nguyen T, Stachel S, Rosen S D. Cloning of a lymphocyte homing receptor reveals a lectin domain. Cell. 1989;56:1045–1055. [PubMed: 2647302]
- 4.
- Siegelman M H, van de Rijn M, Weissman I L. Mouse lymph node homing receptor cDNA clone encodes a glycoprotein revealing tandem interaction domains. Science. 1989;243:1165–1172. [PubMed: 2646713]
- 5.
- Bevilacqua M P, Stengelin S, Gimbrone M A, Seed B. Endothelial leukocyte adhesion molecule 1: An inducible receptor for neutrophils related to complement regulatory proteins and lectins. Science. 1989;243:1160–1165. [PubMed: 2466335]
- 6.
- Johnston G I, Cook R G, McEver R P. Cloning of GMP-140, a granule membrane protein of platelets and endothelium: Sequence similarity to proteins involved in cell adhesion and inflammation. Cell. 1989;56:1033–1044. [PubMed: 2466574]
- 7.
- Larsen E, Celi A, Gilbert G E, Furie B C, Erban J K, Bonfanti R, Wagner D D, Furie B. Padgem Protein: a Receptor that Mediates the Interaction of Activated Platelets with Neutrophils and Monocytes. Cell. 1989;59:305–312. [PubMed: 2478294]
- 8.
- Stoolman L M. Adhesion molecules controlling lymphocyte migration. Cell. 1989;56:907–910. [PubMed: 2647304]
- 9.
- Bevilacqua M, Butcher E, Furie B, Gallatin M, Gimbrone M, Harlan J, Kishimoto K, Lasky L, McEver R, Paulson J, Rosen S, Seed B, Siegelman M, Springer T, Stoolman L, Tedder T, Varki A, Wagner D, Weissman I, Zimmerman G. Selectins: A family of adhesion receptors. Cell. 1991;67:233. [PubMed: 1717161]
- 10.
- Imai Y, True D D, Singer M S, Rosen S D. Direct demonstration of the lectin activity of gp90MEL, a lymphocyte homing receptor. J. Cell Biol. 1990;111:1225–1232. [PMC free article: PMC2116278] [PubMed: 2202735]
- 11.
- Imai Y, Singer M S, Fennie C, Lasky L A, Rosen S D. Identification of a carbohydrate-based endothelial ligand for a lymphocyte homing receptor. J. Cell Biol. 1991;113:1213–1221. [PMC free article: PMC2289002] [PubMed: 2040648]
- 12.
- Rosen S D, Chi S I, True D D, Singer M S, Yednock T A. Intravenously injected sialidase inactivates attachment sites for lymphocytes on high endothelial venules. J. Immunol. 1989;142:1895–1902. [PubMed: 2921520]
- 13.
- Kansas G S. Selectins and their ligands: Current concepts and controversies. Blood. 1996;88:3259–3287. [PubMed: 8896391]
- 14.
- Lasky L A, Singer M S, Dowbenko D, Imai Y, Henzel W J, Grimley C, Fennie C, Gillett N, Watson S R, Rosen S D. An endothelial ligand for L-selectin is a novel mucin-like molecule. Cell. 1992;69:927–938. [PubMed: 1376638]
- 15.
- Foxall C, Watson S R, Dowbenko D, Fennie C, Lasky L A, Kiso M, Hasegawa A, Asa D, Brandley B K. The three members of the selectin receptor family recognize a common carbohydrate epitope, the sialyl Lewis(x) oligosaccharide. J. Cell Biol. 1992;117:895–902. [PMC free article: PMC2289454] [PubMed: 1374413]
- 16.
- Imai Y, Lasky L A, Rosen S D. Sulphation requirement for GlyCAM-1, an endothelial ligand for L-selectin. Nature. 1993;361:555–557. [PubMed: 7679207]
- 17.
- Hemmerich S, Rosen S D. 6′-sulfated sialyl Lewis x is a major capping group of GlyCAM-1. Biochemistry. 1994;33:4830–4835. [PubMed: 8161542]
- 18.
- Hemmerich S, Bertozzi C R, Leffler H, Rosen S D. Identification of the sulfated monosaccharides of GlyCAM-1, an endothelial-derived ligand for L-selectin. Biochemistry. 1994;33:4820–4829. [PubMed: 7512827]
- 19.
- Hemmerich S, Leffler H, Rosen S D. Structure of the O-glycans in GlyCAM-1, an endothelial-derived ligand for L-selectin. J. Biol. Chem. 1995;270:12035–12047. [PubMed: 7538131]
- 20.
- Chandrasekaran E V, Jain R K, Larsen R D, Wlasichuk K, Matta K L. Selectin ligands and tumor-associated carbohydrate structures: Specificities of α 2,3-sialyltransferases in the assembly of 3′-sialyl-6-sialyl/sulfo Lewis a and x, 3′-sialyl-6′-sulfo Lewis x, and 3′-sialyl-6-sialyl/sulfo blood group T-hapten. Biochemistry. 1995;34:2925–2936. [PubMed: 7534477]
- 21.
- Fuhlbrigge R C, Alon R, Puri K D, Lowe J B, Springer T A. Sialylated, fucosylated ligands for L-selectin expressed on leukocytes mediate tethering and rolling adhesions in physiologic flow conditions. J. Cell Biol. 1996;135:837–848. [PMC free article: PMC2121069] [PubMed: 8909555]
- 22.
- Hwang S T, Singer M S, Giblin P A, Yednock T A, Bacon K B, Simon S I, Rosen S D. GlyCAM-1, a physiologic ligand for L-selectin, activates 2 integrins on naive peripheral lymphocytes. J. Exp. Med. 1996;184:1343–1348. [PMC free article: PMC2192819] [PubMed: 8879206]
- 23.
- Rosen S D, Hwang S T, Giblin P A, Singer M S. High-endothelial-venule ligands for L-selectin: Identification and functions. Biochem. Soc. Trans. 1997;25:428–433. [PubMed: 9191130]
- 24.
- Springer T A. Traffic signals on endothelium for lymphocyte recirculation and leukocyte emigration. Annu. Rev. Physiol. 1995;57:827–872. [PubMed: 7778885]
- 25.
- Varki A. Selectin ligands: Will the real ones please stand up? J. Clin. Invest. 1997;99:158–162. [PMC free article: PMC507781] [PubMed: 9005982]
- 26.
- Vestweber D. Ligand-specificity of the selectins. J. Cell. Biochem. 1996;61:585–591. [PubMed: 8806082]
- 27.
- Sanders W J, Katsumoto T R, Bertozzi C R, Rosen S D, Kiessling L L. L-selectin-carbohydrate interactions: Relevant modifications of the Lewis x trisaccharide. Biochemistry. 1996;35:14862–14867. [PubMed: 8942649]
- 28.
- Smith P L, Gersten K M, Petryniak B, Kelly R J, Rogers C, Natsuka Y, Alford J A, Scheidegger E P, Natsuka S, Lowe J B. Expression of the α(1,3)fucosyltransferase Fuc-TVII in lymphoid aggregate high endothelial venules correlates with expression of L-selectin ligands. J. Biol. Chem. 1996;271:8250–8259. [PubMed: 8626519]
- 29.
- Crockett-Torabi E. Selectins and mechanisms of signal transduction. J. Leukoc. Biol. 1998;63:1–14. [PubMed: 9469467]
- 30.
- Bowman K G, Hemmerich S, Bhakta S, Singer M S, Bistrup A, Rosen S D, Bertozzi C R. Identification of an N-acetylglucosamine-6-O-sulfotransferase activity specific to lymphoid tissue: An enzyme with a possible role in lymphocyte homing. Chem. Biol. 1998;5:447–460. [PubMed: 9710564]
- 31.
- Mitsuoka C, Sawada-Kasugai M, Ando-Furui K, Izawa M, Nakanishi H, Nakamura S, Ishida H, Kiso M, Kannagi R. Identification of a major carbohydrate capping group of the L-selectin ligand on high endothelial venules in human lymph nodes as 6-sulfo sialyl Lewis X. J. Biol. Chem. 1998;273:11225–11233. [PubMed: 9556613]
- 32.
- Bargatze R F, Kurk S, Butcher E C, Jutila M A. Neutrophils roll on adherent neutrophils bound to cytokine-induced endothelial cells via L-selectin on the rolling cells. J. Exp. Med. 1994;180:1785–1792. [PMC free article: PMC2191733] [PubMed: 7525838]
- 33.
- Alon R, Fuhlbrigge R C, Finger E B, Springer T A. Interactions Through L-selectin Between Leukocytes and Adherent Leukocytes Nucleate Rolling Adhesions on Selectins and Vcam-1 in Shear Flow. J. Cell Biol. 1996;135:849–865. [PMC free article: PMC2121073] [PubMed: 8909556]
- 34.
- McEver R P, Moore K L, Cummings R D. Leukocyte trafficking mediated by selectin-carbohydrate interactions. J. Biol. Chem. 1995;270:11025–11028. [PubMed: 7538108]
- 35.
- Norgard-Sumnicht K E, Varki N M, Varki A. Calcium-dependent heparin-like ligands for L-selectin in nonlymphoid endothelial cells. Science. 1993;261:480–483. [PubMed: 7687382]
- 36.
- Koenig A, Norgard-Sumnicht K, Linhardt R, Varki A. Differential interactions of heparin and heparan sulfate glycosaminoglycans with the selectins—Implications for the use of unfractionated and low molecular weight heparins as therapeutic agents. J. Clin. Invest. 1998;101:877–889. [PMC free article: PMC508636] [PubMed: 9466983]
- 37.
- Walcheck B, Moore K L, McEver R P, Kishimoto T K. Neutrophil-neutrophil Interactions Under Hydrodynamic Shear Stress Involve L-selectin and Psgl-1: a Mechanism that Amplifies Initial Leukocyte Accumulation on P-selectin in Vitro. J. Clin. Invest. 1996;98:1081–1087. [PMC free article: PMC507527] [PubMed: 8787668]
- 38.
- Zimmerman G A, McIntyre T M, Prescott S M. Adhesion and signaling in vascular cell-cell interactions. J. Clin. Invest. 1996;98:1699–1702. [PMC free article: PMC507606] [PubMed: 8878418]
- 39.
- Frenette P S, Wagner D D. Insights into selectin function from knockout mice. Thromb. Haemost. 1997;78:60–64. [PubMed: 9198128]
- 40.
- Konstantopoulos K, McIntire L V. Effects of fluid dynamic forces on vascular cell adhesion. J. Clin. Invest. 1997;98:2661–2665. [PMC free article: PMC507727] [PubMed: 8981908]
- 41.
- Rainger G E, Buckley C, Simmons D L, Nash G B. Cross-talk between cell adhesion molecules regulates the migration velocity of neutrophils. Curr. Biol. 1997;7:316–325. [PubMed: 9115397]
- 42.
- Rossiter H, Alon R, Kupper T S. Selectins, T-cell rolling and inflammation. Mol. Med. Today. 1997;3:214–222. [PubMed: 9176884]
- 43.
- Diacovo T G, Roth S J, Morita C T, Rosat J P, Brenner M B, Springer T A. Interactions of human α/β and γ/δ T lymphocyte subsets in shear flow with E-selectin and P-selectin. J. Exp. Med. 1996;183:1193–1203. [PMC free article: PMC2192327] [PubMed: 8642261]
- 44.
- Lowe J B, Ward P A. Therapeutic inhibition of carbohydrate-protein interactions in vivo. J. Clin. Invest. 1997;99:822–826. [PMC free article: PMC507887] [PubMed: 9062337]
- 45.
- Steegmaier M, Levinovitz A, Lesmann S, Borges E, Lenter M, Kocher H P, Kleuser B, Vestweber D. The E-selectin ligand ESL-1 is a variant of a receptor for fibroblast growth factor. Nature. 1995;373:615–620. [PubMed: 7531823]
- 46.
- Steegmaier M, Borges E, Berger J, Schwarz H, Vestweber D. The E-selectin-ligand ESL-1 is located in the Golgi as well as on microvilli on the cell surface. J. Cell. Sci. 1997;110:687–694. [PubMed: 9099943]
- 47.
- McEver R P, Martin M N. A monoclonal antibody to a membrane glycoprotein binds only to activated platelets. J Biol. Chem. 1984;259:9799–9804. [PubMed: 6746667]
- 48.
- Stenberg P E, McEver R P, Shuman M A, Jacques Y V, Bainton D F. A platelet alpha-granule membrane protein (GMP-140) is expressed on the plasma membrane after activation. J. Cell Biol. 1985;101:880–886. [PMC free article: PMC2113718] [PubMed: 2411738]
- 49.
- Berman C L, Yeo E L, Wencel-Drake J D, Furie B C, Ginsberg M H, Furie B. A platelet alpha granule membrane protein that is associated with the plasma membrane after activation. Characterization and subcellular localization of platelet activation-dependent granule-external membrane protein. J Clin Invest. 1985;78:130–137. [PMC free article: PMC329541] [PubMed: 2941452]
- 50.
- Moore K L, Thompson L F. P-selectin (CD62) binds to subpopulations of human memory T lymphocytes and natural killer cells. Biochem. Biophys. Res. Commun. 1992;186:173–181. [PubMed: 1378721]
- 51.
- Ushiyama S, Laue T M, Moore K L, Erickson H P, McEver R P. Structural and functional characterization of monomeric soluble P-selectin and comparison with membrane P-selectin. J. Biol. Chem. 1993;268:15229–15237. [PubMed: 7686912]
- 52.
- Weyrich A S, McIntyre T M, McEver R P, Prescott S M, Zimmerman G A. Monocyte tethering by P-selectin regulates monocyte chemotactic protein-1 and tumor necrosis factor- secretion. J. Clin. Invest. 1995;95:2297–2303. [PMC free article: PMC295843] [PubMed: 7537762]
- 53.
- Zannettino A C W, Berndt M C, Butcher C, Butcher E C, Vadas M A, Simmons P J. Primitive human hematopoietic progenitors adhere to P-selectin (CD62P). Blood. 1995;85:3466–3477. [PubMed: 7540063]
- 54.
- Weyrich A S, Elstad M R, McEver R P, McIntyre T M, Moore K L, Morrissey J H, Prescott S M, Zimmerman G A. Activated platelets signal chemokine synthesis by human monocytes. J. Clin. Invest. 1996;97:1525–1534. [PMC free article: PMC507213] [PubMed: 8617886]
- 55.
- Patel K D, Nollert M U, McEver R P. P-selectin must extend a sufficient length from the plasma membrane to mediate rolling of neutrophils. J. Cell Biol. 1995;131:1893–1902. [PMC free article: PMC2120654] [PubMed: 8557755]
- 56.
- Zhou Q, Moore K L, Smith D F, Varki A, McEver R P, Cummings R D. The selectin GMP-140 binds to sialylated, fucosylated lactosaminoglycans on both myeloid and nonmyeloid cells. J. Cell Biol. 1991;115:557–564. [PMC free article: PMC2289144] [PubMed: 1717488]
- 57.
- Moore K L, Stults N L, Diaz S, Smith D L, Cummings R D, Varki A, McEver R P. Identification of a specific glycoprotein ligand for P-selectin (CD62) on myeloid cells. J. Cell Biol. 1992;118:445–456. [PMC free article: PMC2290037] [PubMed: 1378449]
- 58.
- Sako D, Chang X -J, Barone K M, Vachino G, White H M, Shaw G, Veldman G M, Bean K M, Ahern T J, Furie B. et al. Expression cloning of a functional glycoprotein ligand for P-selectin. Cell. 1993;75:1179–1186. [PubMed: 7505206]
- 59.
- Moore K L, Eaton S F, Lyons D E, Lichenstein H S, Cummings R D, McEver R P. The P-selectin glycoprotein ligand from human neutrophils displays sialylated, fucosylated, O-linked poly-N-acetyllactosamine. J. Biol. Chem. 1994;269:23318–23327. [PubMed: 7521878]
- 60.
- Moore K L, Patel K D, Bruehl R E, Fugang L, Johnson D A, Lichenstein H S, Cummings R D, Bainton D F, McEver R P. P-selectin glycoprotein ligand-1 mediates rolling of human neutrophils on P-selectin. J. Cell Biol. 1995;128:661–671. [PMC free article: PMC2199883] [PubMed: 7532174]
- 61.
- Li F, Erickson H P, James J A, Moore K L, Cummings R D, McEver R P. Visualization of P-selectin glycoprotein ligand-1 as a highly extended molecule and mapping of protein epitopes for monoclonal antibodies. J. Biol. Chem. 1996;271:6342–6348. [PubMed: 8626430]
- 62.
- Wilkins P P, Moore K L, McEver R P, Cummings R D. Tyrosine sulfation of P-selectin glycoprotein ligand-1 is required for high affinity binding to P-selectin. J. Biol. Chem. 1995;270:22677–22680. [PubMed: 7559387]
- 63.
- Pouyani T, Seed B. Psgl-1 Recognition of P-selectin is Controlled by a Tyrosine Sulfation Consensus at the Psgl-1 Amino Terminus. Cell. (1995);83:333–343. [PubMed: 7585950]
- 64.
- Sako D, Comess K M, Barone K M, Camphausen R T, Cumming D A, Shaw G D. A Sulfated Peptide Segment at the Amino Terminus of Psgl-1 is Critical for P-selectin Binding. Cell. 1995;83:323–331. [PubMed: 7585949]
- 65.
- Norman K E, Moore K L, McEver R P, Ley K. Leukocyte rolling in vivo is mediated by P-selectin glycoprotein ligand-1. Blood. 1995;86:4417–4421. [PubMed: 8541529]
- 66.
- Norgard K E, Moore K L, Diaz S, Stults N L, Ushiyama S, McEver R P, Cummings R D, Varki A. Characterization of a specific ligand for P-selectin on myeloid cells. A minor glycoprotein with sialylated O-linked oligosaccharides. J. Biol. Chem. 1993;268:12764–12774. [PubMed: 7685350]
- 67.
- Furie B, Furie B C. The Molecular Basis of Platelet and Endothelial Cell Interaction with Neutrophils and Monocytes: Role of P-selectin and the P-selectin Ligand, Psgl-1. Thromb. Haemost. 1995;74:224–227. [PubMed: 8578462]
- 68.
- Laszik Z, Jansen P J, Cummings R D, Tedder T F, McEver R P, Moore K L. P-selectin glycoprotein ligand-1 is broadly expressed in cells of myeloid, lymphoid, and dendritic lineage and in some nonhematopoietic cells. Blood. 1996;88:3010–3021. [PubMed: 8874199]
- 69.
- De Luca M, Dunlop L C, Andrews R K, Flannery J V Jr, Ettling R, Cumming D A, Veldman G M, Berndt M C. A Novel Cobra Venom Metalloproteinase, Mocarhagin, Cleaves a 10-amino Acid Peptide from the Mature N-terminus of P-selectin Glycoprotein Ligand Receptor, Psgl-1, and Abolishes P-selectin Binding. J. Biol. Chem. 1995;270:26734–26737. [PubMed: 7592904]
- 70.
- Borges E, Eytner R, Moll T, Steegmaier M, Campbell M A, Ley K, Mossmann H, Vestweber D. The P-selectin glycoprotein ligand-1 is important for recruitment of neutrophils into inflamed mouse peritoneum. Blood. 1997;90:1934–1942. [PubMed: 9292527]
- 71.
- Guyer D A, Moore K L, Lynam E, Schammel C M G, Rogelj S, McEver R P, Sklar L. P-selectin glycoprotein ligand-1 (PSGL-1) is a ligand for L-selectin in neutrophil aggregation. Blood. 1996;88:2415–2421. [PubMed: 8839831]
- 72.
- Wilkins P P, McEver R P, Cummings R D. Structures of the O-glycans on P-selectin glycoprotein ligand-1 from HL-60 cells. J. Biol. Chem. 1996;271:18732–18742. [PubMed: 8702529]
- 73.
- Veldman G M, Bean K M, Cumming D A, Eddy R L, Sait S N J, Shows T B. Genomic organization and chromosomal localization of the gene encoding human P-selectin glycoprotein ligand. J. Biol. Chem. 1995;270:16470–16475. [PubMed: 7541799]
- 74.
- Vachino G, Chang X -J, Veldman G M, Kumar R, Sako D, Fouser L A, Berndt M C, Cumming D A. P-selectin glycoprotein ligand-1 is the major counter-receptor for P-selectin on stimulated T cells and is widely distributed in non-functional form on many lymphocytic cells. J. Biol. Chem. 1995;270:21966–21974. [PubMed: 7545173]
- 75.
- Spertini O, Cordey A -S, Monai N, Giuffre L, Schapira M. P-selectin glycoprotein ligand-1 (PSGL-1) is a ligand for L-selectin on neutrophils, monocytes and CD34+ hematopoietic progenitor cells. J. Cell Biol. 1996;135:523–531. [PMC free article: PMC2121046] [PubMed: 8896607]
- 76.
- Li F, Wilkins P P, Crawley S, Weinstein J, Cummings R D, McEver R P. Post-translational modifications of recombinant P-selectin glycoprotein ligand-1 required for binding to P- and E-selectin. J. Biol. Chem. 1996;271:3255–3264. [PubMed: 8621728]
- 77.
- McEver R, Cummings R D. Role of Psgl-1 Binding to Selectins in Leukocyte Recruitment. J. Clin. Invest. 1997;100:485–914. [PubMed: 9413410]
- 78.
- Hidari K I, Weyrich A S, Zimmerman G A, McEver R P. Engagement of P-selectin glycoprotein ligand-1 enhances tyrosine phosphorylation and activates mitogen-activated protein kinases in human neutrophils. J. Biol. Chem. 1997;272:28750–28756. [PubMed: 9353345]
- 79.
- Takada M, Nadeau K C, Shaw G D, Marquette K A, Tilney N L. The cytokine-adhesion molecule cascade in ischemia/reperfusion injury of the rat kidney. Inhibition by a soluble P-selectin ligand. J. Clin. Invest. 1997;99:2682–2690. [PMC free article: PMC508114] [PubMed: 9169498]
- 80.
- Dong Z M, Chapman S M, Brown A A, Frenette P S, Hynes R O, Wagner D D. The combined role of P- and E-selectins in atherosclerosis. J. Clin. Invest. 1998;102:145–152. [PMC free article: PMC509076] [PubMed: 9649568]
- 81.
- Mehta P, Cummings R D, McEver R P. Affinity and kinetic analysis of P-selectin binding to P-selectin glycoprotein ligand-1. J. Biol. Chem. 1998;273:32506–32513. [PubMed: 9829984]
- 82.
- Liu W, Ramachandran V, Kang J, Kishimoto T K, Cummings R D, McEver R P. Identification of N-terminal residues on P-selectin glycoprotein ligand-1 required for binding to P-selectin. J. Biol. Chem. 1998;273:7078–7087. [PubMed: 9507018]
- 83.
- Yang J, Galipeau J, Kozak C A, Furie B C, Furie B. Mouse P-selectin glycoprotein ligand-1: Molecular cloning, chromosomal localization, and expression of a functional P-selectin receptor. Blood. 1996;87:4176–4186. [PubMed: 8639776]
- 84.
- Evangelista V, Manarini S, Sideri R, Rotondo S, Martelli N, Piccoli A, Totani L, Piccardoni P, Vestweber D, de Gaetano G, Cerletti C. Platelet/polymorphonuclear Leukocyte Interaction: P-selectin Triggers Protein-tyrosine Phosphorylation-dependent Cd11b/cd18 Adhesion: Role of Psgl-1 as a Signaling Molecule. Blood. 1999;93:876–885. [PubMed: 9920836]
- 85.
- Dulkanchainun T S, Goss J A, Imagawa D K, Shaw G D, Anselmo D M, Kaldas F, Wang T, Zhao D, Busuttil A A, Kato H, Murray N G, Kupiec-Weglinski J W, Busuttil R W. Reduction of hepatic ischemia/reperfusion injury by a soluble P-selectin glycoprotein ligand-1. Ann. Surg. 1998;227:832–840. [PMC free article: PMC1191386] [PubMed: 9637546]
- 86.
- Scalia R, Hayward R, Armstead V E, Minchenko A G, Lefer A M. Effect of recombinant soluble P-selectin glycoprotein ligand-1 on leukocyte-endothelium interaction in vivo: Role in rat traumatic shock. Circ. Res. 1999;84:93–102. [PubMed: 9915778]
- 87.
- Lowe J B, Stoolman L M, Nair R P, Larsen R D, Berhend T L, Marks R M. Elam-1-dependent Cell Adhesion to Vascular Endothelium Determined by a Transfected Human Fucosyltransferase Cdna. Cell. 1990;63:475– 484. [PubMed: 1699667]
- 88.
- Ellies L G, Tsuboi S, Petryniak B, Lowe J B, Fukuda M, Marth J D. Core 2 oligosaccharide biosynthesis distinguishes between selectin ligands essential for leukocyte homing and inflammation. Immunity. 1998;9:881–890. [PubMed: 9881978]
- 89.
- Goelz S E, Hession C, Goff D, Griffiths B, Tizard R, Newman B, Chi-Rosso G, Lobb R. Elft: a Gene that Directs the Expression of An Elam-1 Ligand. Cell. 1990;63:1349–1356. [PubMed: 1702034]
- 90.
- Kumar R, Potvin B, Muller W A, Stanley P. Cloning of a human α(1,3)-fucosyltransferase gene that encodes ELFT but does not confer ELAM-1 recognition on Chinese hamster ovary cell transfectants. J. Biol. Chem. 1991;266:21777–21783. [PubMed: 1718983]
- 91.
- Goelz S, Kumar R, Potvin B, Sundaram S, Brickelmaier M, Stanley P. Differential expression of an E-selectin ligand (SLex) by two Chinese hamster ovary cell lines transfected with the same α(1,3)-fucosyltransferase gene (ELFT). J. Biol. Chem. 1994;269:1033–1040. [PubMed: 7507103]
- 92.
- Lowe J B, Kukowska-Latallo J F, Nair R P, Larsen R D, Marks R M, Macher B A, Kelly R J, Ernst L K. Molecular Cloning of a Human Fucosyltransferase Gene that Determines Expression of the Lewis X and Vim-2 Epitopes But Not Elam-1-dependent Cell Adhesion. J. Biol. Chem. 1991;266:17467–17477. [PubMed: 1716630]
- 93.
- Picker L J, Warnock R A, Burns A R, Doerschuk C M, Berg E L, Butcher E C. The Neutrophil Selectin Lecam-1 Presents Carbohydrate Ligands to the Vascular Selectins Elam-1 and Gmp-140. Cell. 1991;66:921–933. [PubMed: 1716182]
- 94.
- Polley M J, Phillips M L, Wayner E, Nudelman E, Singhal A K, Hakomori S, Paulson J C. CD62 and endothelial cell-leukocyte adhesion molecule 1 (ELAM-1) recognize the same carbohydrate ligand, sialyl-Lewis x. Proc. Natl. Acad. Sci. 1991;88:6224–6228. [PMC free article: PMC52055] [PubMed: 1712483]
- 95.
- Natsuka S, Gersten K M, Zenitas K, Kannagi R, Lowe J B. Molecular cloning of a cDNA encoding a novel human leukocyte α1,3-fucosyltransferase capable of synthesizing the sialyl Lewis x determinant. J. Biol. Chem. 1994;269:16789–16794. [PubMed: 8207002]
- 96.
- Asa D, Raycroft L, Ma L, Aeed P A, Kaytes P S, Elhammer Å P ,, Geng J -G. The P-selectin glycoprotein ligand functions as a common human leukocyte ligand for P- and E-selectins. J. Biol. Chem. 1995;270:11662–11670. [PubMed: 7538120]
- 97.
- Lowe J B. Selectin ligands, leukocyte trafficking, and fucosyltransferase genes. Kidney Int. 1997;51:1418–1426. [PubMed: 9150453]
- 98.
- Lenter M, Levinovitz A, Isenmann S, Vestweber D. Monospecific and common glycoprotein ligands for E- and P-selectin on myeloid cells. J. Cell Biol. 1994;125:471–481. [PMC free article: PMC2120038] [PubMed: 7512971]
- 99.
- Maly P, Thall A D, Petryniak B, Rogers G E, Smith P L, Marks R M, Kelly R J, Gersten K M, Cheng G Y, Saunders T L. et al. The α(1,3)fucosyltransferase Fuc-TVII controls leukocyte trafficking through an essential role in L-, E-, and P-selectin ligand biosynthesis. Cell. 1996;86:643–653. [PubMed: 8752218]
- 100.
- Niemela R, Natunen J, Majuri M L, Maaheimo H, Helin J, Lowe J B, Renkonen O, Renkonen R. Complementary Acceptor and Site Specificities of Fuc-tiv and Fuc-tvii Allow Effective Biosynthesis of Sialyl-trilex and related polylactosamines present on glycoprotein counterreceptors of selectins. J. Biol. Chem. 1998;273:4021–4026. [PubMed: 9461592]
- Background
- L-Selectin Structure and Expression
- L-Selectin Ligands on High Endothelial Venules
- L-Selectin-dependent Leukocyte Adhesion to Microvascular Endothelium
- E-Selectin Structure and Regulation
- E-Selectin Ligands
- P-Selectin Structure and Expression
- P-Selectin Ligands
- Glycosyltransferases Involved in Selectin Ligand Biosynthesis
- Future Directions
- References
- Selectins - Essentials of GlycobiologySelectins - Essentials of Glycobiology
- Exploring the Biological Roles of Glycans - Essentials of GlycobiologyExploring the Biological Roles of Glycans - Essentials of Glycobiology
Your browsing activity is empty.
Activity recording is turned off.
See more...