NCBI Bookshelf. A service of the National Library of Medicine, National Institutes of Health.
Chattopadhyay A, editor. Serotonin Receptors in Neurobiology. Boca Raton (FL): CRC Press/Taylor & Francis; 2007.
Abstract
The properties of neuronal plasticity are not only studied after brain injury. Adaptive changes also take place in the neurons of genetically modified animals, which provide a rational for genetic studies in humans suffering from mental diseases. Following a brief summary describing the alterations in human serotonin (5-HT, 5-hydrox-ytryptamine) gene receptors found in one of the most complex of mental functions—motivation related to food or illegal drugs intake—we will describe that the only gene deficit, destroying 5-HT receptor, is not enough to produce apparent maladap-tive behavior in rodents. However, when combined with a novel environmental challenge, modified genetic predisposition induces deviant behavior. Locomotion, feeding, and emotion-like state disorders (anxiety-like behavior, depression-like syndrome) are classically underlined by brain deficits and subsequently linked to adaptive neuronal changes. This review gathered evidence to postulate that following genetic alterations, the brain partly “burns” its adaptive resources over time, and lacks a sufficient flexibility to adapt during adulthood. The limits of adaptive changes in neurons in the face of an unexpected environmental context, may therefore represent an “open door” for mental-like disorders to emerge.
INTRODUCTION
Neuronal plasticity concept (neuroplasticity) refers to neurobiological processes that include adaptive changes in the morphology and function of neuronal systems following environmental modifications and/or brain injury. The brain may then exert an adaptive control of behavior. Neuroplasticity persists during adulthood and confers the ability to perform adapted behavior in the face of environmental changes. In other words, over time during development (from conception to death), a “cerebral maturity” may occur, i.e., a progressive equilibrium in neuronal interactions may allow organisms to adapt behavior under environmental challenges, while taking into account internal factors. Surprisingly, behavioral states may appear adapted even when a neuronal imbalance between neuronal interactions exists (neuroplasticity). Subsequently, adaptive changes in neurons following neuronal lesion may represent a “neurobiological support” of adaptive behavioral strategies. In addition, adaptive changes are not equivalent to compensation. Neurons may effectively adapt but may not compensate all deficits. In other words, limits in neuronal adaptive changes exist.
The serotoninergic system is one of the most flexible neuronal systems [1], and there is evidence for a contribution of this neuromodulator system to synaptic plasticity [2]. Again, in neuroscience history, major advances have taken advantage of studies on the functions of serotonin (5-hydroxytryptamine, 5-HT) in the brain and, in particular, of 5-HT receptors (5-HTR). This article synthesizes results from previous studies and suggests that the neuroplasticity confers to mouse models the ability to adapt in spite of an engineered genetic deficit. In other words, organisms maintained in their usual context (home cage) overcome a genetic deficit, as demonstrated for specific 5-HTR knock-out (KO) mouse [3–12]. In contrast, KO phenotypes are often, but not systematically, best revealed following an environmental or pharmacological challenge.
We have summarized results from previous studies focusing on motivational deficits related to feeding, novelty-induced locomotion, and addictive behavior to illustrate this point. Several studies have reported impairments in novelty-induced locomotion in 5-HTR KO mice: 5-HT1A, [5] 5-HT1B, [6] 5-HT4, [10, 11] and 5-HT5A, [9] whereas these mutant mice did not exhibit any motor impairments in their home cage. These studies reinforce the view that (1) adaptive mechanisms are limited in these mice, and (2) serotoninergic systems modulate stress responsiveness in situations such as reactivity to novel environment. Likewise, locomotion induced by psychoactive stimulants (cocaine, 3,4-N-methylenedioxymethamphetamine or ecstasy) was altered in 5-HT1B, 5-HT2C, [7,8,13] and 5-HT4R KO mice (Compan, unpublished). Along these lines, adult 5-HT1BR and 5-HT4R KO mice displayed maladaptive feeding behavior under unusual context [14, 10], where 5-HT2CR KO mouse spontaneously displayed obesity [15].
This review illustrates that deficits in adaptation to stressors (novel environment, psychostimulants, pharmacological substances) coexist with changes in the serotonergic system themselves in the absence of only one 5-HTR, as so far revealed in 5-HT1AR and 5-HT4R KO mice during adulthood but not in 5-HT1BR-null adult mouse [16–18].
Altered 5-HT neuromodulation may underlie a wide range of mental diseases, including anorexia, addiction to illegal drugs or food (bulimia), major depression (stress factor [19]), or anxiety [20–24]. Seven families of 5-HTRs with eighteen subtypes, without including mRNA editing and splice variant 5-HTR isoforms, are currently identified [25, 26]. As will be summarized in the first section, a goal of many studies has been to find whether deficits in genes encoding 5-HTRs are or are not associated with mental disorders, predominantly with depression and schizophrenia, and less frequently with feeding disorders and addiction. However, as for most mental diseases, a genetic component combined with environmental determinism is likely. Such combinations of interactions between internal and external factors are, however, complex to study and are seldom investigated, though gene KO strategies may contribute to this knowledge. So far, ten 5-HTR KO mice lacking either 5-HT1AR [4, 5], 5-HT1BR [27], 5-HT2A [12], 5-HT2B, [28], 5-HT2C [15], 5-HT3 [29], 5-HT4 [10], 5-HT5A [9], 5-HT6 [15], and 5-HT7 [30, 31] receptors have been generated to study 5-HTR functions.
In this conceptual context, where environmental and biologic factors overlap, it is difficult to believe that only a therapeutic treatment would overcome mental diseases. Identifying effective therapeutic treatments from knowledge of specific 5-HTR functions and other related biological events (transduction systems, other neuronal and glial systems), may be promising but unpredictable. However, this article opens the possibility that a genetic deficit is not an absolute “un-overcome” because of neuronal adaptive changes. Extending the limits of adaptive changes in neurons may open the possibility to circumvent mental disease. It can be expected that the overall view of a “psynogenic puzzle”* will suggest possible combined therapies established on both pharmacological and psychological treatments. However, the potential for affecting biologic predispositions as they interact with environmental factors remains considerably unknown.
HUMAN 5-HT RECEPTOR GENE POLYMORPHISMS AND ADDICTIONS TO FOOD AND ILLEGAL DRUGS
The neuronal processes involved in motivation, particularly for eating, have a high reward component. In humans, eating disorders are classified as mental disorders according to the Diagnostic and Statistical Manual of Mental Disorders (DSM IV-TR, APA, 2003). Bulimia is included in addictive behavior, and binge eating disorder is an excessive consumption of food, also observed in patients suffering from bulimia and sometimes from anorexia, and a few obese patients. The excess or absence of food intake is therefore not only a metabolic and/or endocrinological matter, but also, like in the consumption of drugs of abuse, a mental problem. One of the main principles of neural science is that disturbances of brain function underlie mental disorders. “Miscegenation” of neurobiology and psychology (“being in relation”) should be further studied because stress can influence feeding disorders, which coexist with anxiety and depression [32–34].
If the focus of the following association studies is almost systematically based on maladapted phenotypes of 5-HTR KO mouse, in turn, such analyses provide fundamental interest to both in vitro and in vivo pharmacological studies using animal models. Twelve different genes encode metabotropic 5-HTRs in humans (NCBI, Map Viewer at www.ncbi.nlm.nih.gov/mapview/) (e.g., gene structure in Reference [26]) and, five genes encode the only 5-HTR ligand-gated ion channels, 5-HT3R (HTR3A and HTR3B [35]; HTR3C1-4 [36]; HTR3D, HTR3E [37]). Single nucleotide polymorphisms (SNP) may influence transduction systems (e.g., Reference [38]); Bmax of 5-HT1AR [39], 5-HT1BR [40], and 5-HT2AR [41–43] and even affect 5-HT-stimulated intracel-lular calcium flux in cultured cells [44, 45]. The substitution of cysteine for serine at codon 23 in the human HTR2C gene, though not systematically related to mental disorders (see below I.1 polymorphism within human 5-HTR genes and eating disorders and Table 2 in Reference [46] for review), may alter until the constitutive activity of the corresponding receptor [47]. Such an association may be of great importance as the constitutive activity of 5-HT2CR has recently been reported to influence the release of neuromediator (dopamine) within the striatum of freely moving rodents [48]; see also Reference [49]). Each of these examples opens the obvious possibility that synonymous or nonsynonymous polymorphisms may exert an impact on specific neuronal response, including the efficacy of any therapeutic agents. Few association studies focused on the benefit of therapeutic drugs related to HTR5-HT gene polymorphisms, and did not establish a systematic positive relation. For instance, responses to clozapine, used to treat schizophrenia, failed to be linked to the T25N HTR2A SNP [50].
Polymorphism within Human 5-HT Receptor Genes and Eating Disorders
In humans suffering from eating disorders, the HTR2A gene, encoding 5-HT2AR, has been the most frequently analyzed over the last decade. Both positive and absence of associations have been detected between HTR2A gene polymorphisms and eating disorders [51–57]. The 5-HT2AR gene promoter polymorphism (1438A/G) is described to be associated with both anorexia and bulimia nervosa [51–53] or not [54, 55]. Furthermore, no evidence of HTR2A gene polymorphism (1438A/G) has been related to binge eating disorders, whereas again such an association was revealed in patients suffering from anorexia or bulimia nervosa [56] or obesity [58]. In other words, positive or negative associations between HTR2A/2C genes and eating disorders appear to depend mainly on the studied polymorphism [46] and may likely depend on the complexity of psychiatric diagnosis (anorexia, bulimia, hyperphagia, hypophagia, binge eating disorders: e.g., DSM IV-TR, APA, 2003). Likewise, changes in HTR2C gene, encoding 5-HT2CR, are often but not systematically associated with obesity [59–66]. Indeed, the polymorphism of HTR2C gene at codon 23 (cys23ser) has not been associated with bulimia nervosa, binge eating disorders or obesity [59, 60], and its association with both weight loss and gain is unclear [54,59,67]. The association between the 759C/T polymorphism of HTR2C gene and weight gain (obesity, antipsychotic-induced body weight gain) appears more reproducible, as a positive link has been reported by at least five studies [61–63,65,68]. It has been further highlighted that defects in 5-HT2CR pre-mRNA processing controlled by small nuclear RNA may contributes to the Prader-Willi syndrome, which includes hyperphagia and obesity [66].
Less human studies have examined the association of polymorphism of some other human 5-HT receptor genes and feeding disorders. Hinney et al. (1999) [69] concluded that polymorphisms in the HTR1Dß (phe124cys: T371G) and HTR7 (pro279leu), encoding, respectively, 5-HT1DßR (rodent 5-HT1BR analogue) and 5-HT7R, are unlikely related in the regulation of body weight or anorexia nervosa. In contrast, the G861C polymorphism of the HTR1Dß gene was associated with minimum and maximum lifetime body mass indices in women suffering from bulimia [70] and could be related to their potential predisposition to obsessive–compulsive disorder [71].
To our knowledge, no further studies have been conducted to relate any alteration within HTR1A, HTR3, HTR4, HTR5, HTR6, and HTR7 genes with eating disorders. Based on our previous studies, possible alterations within the HTR4 gene related to eating disorders is discussed in the 5-HTR KO and eating disorders section.
Polymorphism within Human 5-HT Receptor Genes and Addiction to Illegal Drugs
Polymorphisms within human 5-HTR genes and alcoholism have been extensively studied focusing on HTR1Dß and HTR2A/2C gene polymorphisms. Only two studies have reported the absence of any influence of HTR1A gene polymorphism on alcoholism [72, 73].
Positive evidence exists to associate G861C polymorphism of the HTR1Dß gene with alcoholism, although the association resulted from either a high frequency in the HTR1Dß 861C allele [74, 75] or the HTR1Dß 861G allele [76]. An association was also found between a lower frequency of the HTR1Dß 861C gene and alcoholism [77]. In divergence, no association with alcohol abuse has been related to the G861C or other polymorphism of the HTR1Dß gene [78–82]. In addition, the HTR1Dß A-161T polymorphism has been reported to be associated with alcohol dependence [83]. Such discrepancies have also been reported with the T/C 102 polymorphism within the HTR2A gene analyzed in patients suffering from alcoholism, with positive [84] and negative associations described [85, 86]. The 1438 G/A HTR2A gene polymorphism has been reported, so far, to influence alcoholism development [58,87,88]. In sharp contrast, there exists a systematic absence of any impact of the cys23ser HTR2C polymorphism on alcohol dependence [85,89,90] associated or not with, other mental diseases such as attention-deficit hyperactivity disorder [91], panic disorder, generalized anxiety disorder including narcolepsy [76] and, alcohol-withdrawal induced seizure [92].
Again, other possible alterations of human 5-HTR genes have not yet been reported to constitute a risk factor for alcoholism. Only one study has described a possible protective influence of the allele 1180G within HTR1Dß gene from addiction to a drugs “cocktail” including heroin and cocaine [93], whereas this is not systematically the case in subjects prominently addicted to cocaine and bearing the A1180G SNP [78]. Mutations within HTR2B gene further appear to influence abuse of illegal drugs [94], but neither T102C nor G1438G HTR2A SNPs seem to exert an impact on heroin consumption [95].
Such discrepancies between results from association studies are classically thought to come from differences in phenotypes of people suffering from eating disorders, and abuse of alcohol and illegal drugs [82], as well as the number of subjects [96]. Mental disorder diagnosis is not limited to one aspect but is based on a large spectrum of maladaptive behavior; one given patient may suffer from both bulimia and anorexia, which both coexist with an elevated level of anxiety [97]. One must also consider the possibility that control subjects may later develop the disorders being studied, so they may not provide an appropriate control comparison. In addition, from results summarized by the above section, it is important to note that 1438 A/G HTR2A and G861C HTR1Dß, but not the cys23ser HTR2C, polymorphisms have been reported to be associated with both eating disorders and alcoholism. In light of the study of Drysdale et al. [98], it appears of important relevance to further take into account, when possible, the impact of multiple single nucleotide polymorphisms (SNPs) within a haplotype (the set of SNPs within one chromosome) on one given phenotype.
Such a wide spectrum of human complexity may certainly explained why there is sometimes, in a first approximation, no reproducibility between phenotypes-related to HTR5 gene polymorphism and 5-HTR KO mice. However, even though we do not intend to anthropomorphize the mouse, increased body weight gain has been related to the 759C/T polymorphism of the HTR2C gene in humans [61–63,65,68] as well as, in 5-HT2CR knockout mouse [15].
KNOCK-OUT OF 5-HT RECEPTORS CLASSICALLY REQUIRED ENVIRONMENTAL CHALLENGES TO PRODUCE MALADAPTIVE BEHAVIOR
5-HT Receptor Knock-Out Mice and Eating Disorders
In industrialized countries, anorexia nervosa is among the mental diseases having the highest mortality rates [99], but no therapeutic strategy is effective to treat this disorder. There is as yet no animal model of this condition, either. If one defines anorexia as a self-imposed starvation despite an energy demand, a similar behavior can be produced in animals and humans following increase in 5-HT neuromodulation. Among drugs increasing the synaptic levels of 5-HT, fenfluramine inhibits the consumption of food in human and rodent [100–102]. Likewise, drugs of abuse such as amphetamine classically interrupt the physiological drive to eat. In particular, the psychogenic compound Ecstasy, 3,4-N-methylenedioxymethamphetamine (MDMA), diminishes both the consumption of food in humans [103] and rats [104] and reduces starvation-induced eating in mice [105]. Recently, 5-HT1BR agonists and fenfluramine have been found to reduce food intake by reciprocally regulating melacortin neurons at the level of the arcuate nucleus of hypothalamus [106]. Pharmacological studies have shown that 5-HT2CR is also importantly involved in 5-HT-mediated hypophagia [107–109]. The contribution of 5-HT2CR in both fenfluramine and MDMA-induced anorexia-like behavior has been demonstrated [105,110,111]. 5-HT2CR KO mice display a lower sensitivity to fenfluramine [110], as described in 5-HT1BR KO mice (Lucas et al., 1998 [14]). An attenuated function of 5-HT2CR may account for the decreased sensitivity of 5-HT1BR KO mice to fenfluramine [112]. The common downstream target of both 5-HT1BR and 5-HT2CR activation may be the melacortin 4 receptor activation in the hypothalamus [106, 113]. Appetite suppressants remain effective in the absence of 5-HT1BR. Indeed, 5-HT1BR KO mice display a normal response to MDMA-induced anorexia-like behavior [105]. Our yet unpublished findings clearly indicate that an elevated function of 5-HT4R may account for the insensitivity of 5-HT1BR KO mice to the anorectic effect of MDMA. Indeed, the intraperitoneal injection of RS39604, a 5-HT4R antagonist, has clearly suppressed MDMA-induced anorexia in starved 5-HT1BR KO compared to wild-type mice (Compan, unpublished). Along these lines, MDMA-treated 5-HT4R KO mice consume a higher amount of food than wild-type mice (Compan, unpublished).
The obesity associated with overeating in 5-HT2CR KO mice further supports that 5-HT2CR influences feeding behavior [15]. Both 5-HT1AR and 5-HT1BR KO are also heavier when born from homozygote lines [6,105,114–116], while the loss of 5-HT2AR, 5-HT3AR, 5-HT4R, as well as 5-HT5AR function did not induce any change in body weight under basal conditions [9,10,12,117].
In particular, overweight in the absence of 5-HT1AR has been reported in male [116], but not in female mutant mice [114]. To our knowledge, no information on the amount of food consumed over time during development has been revealed in the absence of mHTR1A gene under basal condition. In contrast to all other 5-HTR agonists tested so far, the pharmacological stimulation of 5-HT1AR augments the intake of food [118, 119]. 5-HT1AR agonist-induced hyperphagia is classically associated with their negative influence on serotonergic neurons activity [17,120–126] (cf. section titled “5-HT Receptor Knock-Out Mice and Adaptive Changes in Central Serotoninergic Systems”). In other words, the stimulation of 5-HT1AR induced increases in food intake probably because the activation of 5-HT1AR inhibits the electrophysiological activity of serotonergic neurons. On the contrary, 5-HT1AR KO mice display increased spontaneous firing of DRN 5-HT neurons [126, 127] (cf. section titled “5-HT Receptor Knock-Out Mice and Adaptive Changes in Central Serotoninergic Systems”). It may then be expected that 5-HT1AR KO mice would eat a lesser amount of food than their wild-type congeners and would be underweight. Subsequently, the possible adaptive changes that certainly overcome such hypophagia and low body weight can be suspected to highly counterbalance the loss of 5-HT1AR function.
Two earlier studies have reported that male 5-HT1BR KO mice are overweight and consume a higher amount of food than wild-type mice [6,115,116]. In addition, female 5-HT1BR KO mice are also overweight [116]. However, results became the subject of an interesting debate because animals were the offspring of homozygote breeders, suggesting an incidence of parental care [115]. Homozygote offspring from heterozygote breeding pairs were then used [105] in order to avoid indirect effects of parental care behavioral responses, as discussed by Bouwknecht et al. [115] No differences in either baseline food intake or body weight were detected between wild-type and 5-HT1BR KO mice, even following a 24 h period of food deprivation [105], as previously reported [14,128,129]. Whether animals of identical genotype were housed together or not may also likely influence any state (I. Seif, personal observation), including feeding behavior in mutant mice that may display elevated aggressiveness (5-HT1BR KO [27]), hyperanxiety-like state (5-HT1AR KO [4, 5]), hyposensitivity to stress (5-HT4R KO [10]). Less investigated is the phenomenon of stress-induced hypophagia. Hypothalamic-pituitary-adrenal axis hormones have been, however, suggested to be involved in this stress response, at least partially, via the release of 5-HT in the medial prefrontal cortex, nucleus accumbens, amygdala and dorsal hippocampus [130–132].
One study proposed that 5-HT2A/2CRs could be implicated in this cascade of events, using a pharmacological approach [133]. 5-HT2CR KO mice display opposite feeding responses to mild stress, depending on age, whereas only old mutant mice displayed hypersensitivity to repeated stress, which is associated with increased levels in stress hormones [134].
To date, 5-HT4Rs KO mouse is the only known animal model displaying less sensitivity to stress-induced anorexia [10, 11]. Such a maladaptive feeding response to restraint stress in 5-HT4R KO mouse is likely due to deficiencies in the activity of serotonergic systems (see Reference [18]; cf. section titled “5-HT Receptor KnockOut Mice and Adaptive Changes in Central Serotoninergic Systems”) rather than a direct consequence of the hyperactivity of hypothalamo-pituitary axis [10].
Only a few sparse results related to food intake have been reported so far in other 5-HTR KO mice under stressful context such as the open-field. In the novelty suppressed feeding paradigm, starved mice face a dual conflict between the physiological drive to explore a novel environment, eat, and find safety (see Reference [12] and Reference [135]). The latency to eat in this novel space is interpreted as an index of anxiety-like state, when possible bias in locomotor and exploratory activity. The latency to eat is enhanced in starved 5-HT1AR KO [135], and reduced in 5-HT2AR KO mice [12], which were related to their respective hyper- and hypo-anxiety-like state. Along these lines, feeding disorders in 5-HT4R KO mice might be related to a higher level of anxiety-like behavior following stress [10].
Altogether (Table 8.1), these results suggest the presence of at least two modes of action of 5-HT to regulate feeding behavior. Under baseline conditions, the body weight appears to be mainly regulated via the 5-HT2CR whereas after an unusual and strong stressful event, 5-HT4R may become involved. How other 5-HTRs contribute to the ability of stress to decrease food intake remains unexplored, although the loss of 5-HT1AR function, as well as the loss of 5-HT4R function, appears to be associated with overeating when mice were frightened in the open field. It is the inverse in the absence 5-HT2AR. All in all, these examples, with the exception of 5-HT2CR KO mice, indicate that feeding disorders associated with a genetic deficit are best revealed in a stressful context or following a pharmacological challenge.
TABLE 8.1
Serotonin (5-hydroxytryptamine, 5-HT) Receptor Knock-Out Mice Display Maladapted Behaviors under an Environmental or Pharmacological Challenge
As stressful context appears as a determinant factor in human suffering from anorexia that coexists with anxiety and depression [32],– [34] polymorphisms within HTR4 gene may contribute to eating disorders. We have recently raised the possibility that 5-HT4R may represent a new therapeutic target of depression [18,136,137]. 5-HT4R-gene polymorphism has been associated with the bipolar syndrome (manic depression) in humans [138], and increased 5-HT4R density has recently been described in patients suffering from depression leading to suicide [139]. Along these lines, using a range of molecular, biochemical, and physiological in vivo studies, we have gathered evidence indicating that the intra-accumbal activation of the 5-HT4Rs/cAMP/PKA pathway reduced eating and elevated the mRNA expression of an important satiety factor: cocaine- and amphetamine-regulated transcript (CART) into the nucleus accumbens (Compan, unpublished). Neuronal disturbances in the nucleus accumbens are associated with anxiety [140], abusive consumption of illegal drugs [141], and ecstasy-induced anorexia (Compan, unpublished results). Interestingly, one of the HTR4 introns encodes the adrenaline ß2 receptor, a polymorphism of which is related to obesity. Both 5-HT4R and ß2R lead to their heterodimerization [142]. CART gene polymorphisms have also been identified in obese patients (for review see Reference [143]). As multiple SNPs may account for any given mental disease [98], it is interesting to note that both HTR4 and CART genes are also located on the same human chromosome; htR4 gene: 5q31-33 [144], CART gene: 5q13-14 [144–146].
5-HT Receptor Knock-Out Mice and Novelty-Induced Locomotion
Central serotoninergic systems are widely suspected to modulate stress responsiveness in situations such as reactivity to a novel environment [147]. Several studies have further revealed a relationship between specific 5-HTR and novelty-induced locomotion using KO mice: 5-HT1A [5], 5-HT1B [6], 5-HT2C [8], 5-HT4 [10], and 5-HT5A [9]. In the novel open-field test, rodents face a conflict between the physiological drive to explore a novel environment and safety. In addition, under specific environmental conditions, wild-type mice explored the center part less than the periphery of the open field.
5-HT1AR and 5-HT4R KO mice are less reactive to novel environments, whereas their motor activity was not altered in their home cages [4,5,10]. These results suggest that the absence of 5-HT1AR or 5-HT4R results in an attenuation of the motor responses induced by novel environments. In contrast, it is the inverse for 5-HT1B, 5-HT2C or 5-HT5AR KO mice, which display an elevated horizontal activity in the open field (5-HT1B [6, 148]; not systematically see Reference [27]; 5-HT2CR [27]; 5-HT5AR [9]). In contrast, loss of 5-HT2AR [12], 5-HT3/3AR [117,149,150] or, 5-HT6R [151] function has no effect on novelty-induced locomotion (5-HT2AR [12]; 5-HT3/3AR [117,149,150]; 5-HT6R [151]). As well as for 5-HT1AR or 5-HT4R KO, all other generated 5-HTR KO mice did not exhibit any change in home cage activity (5-HT1BR [6, 148]; 5-HT2AR [12]; 5-HT4R [10]; 5-HT5AR [9]; 5-HT6R [151]), with the exception of 5-HT2CR KO that display hyperactivity [152]. To our knowledge, no studies have reported whether the loss of 5-HT3R or 5-HT7R function may alter home cage activity or not.
These data are summarized in Table 8.1 and suggest that a permanent absence of a gene encoding one of the 5-HTR provokes either a hypo- (5-HT1AR and 5-HT4R), hyper- (5-HT1BR, 5-HT2CR, 5-HT5AR) or control-reactivity (5-HT2AR, 5-HT3R, 5-HT6R) to novelty. As novelty-seeking behavior is often related to abuse of illegal drugs [153, 154], the goal of several studies was to test the effects of illegal drugs in the absence of gene encoding 5-HTR. These results are discussed in the following section.
5-HT Receptor Knock-Out Mice and Illegal Drugs such as Ecstasy and Cocaine
Stress may enhance the effects of illegal drugs such as cocaine, which are associated with changes on dopaminergic systems [155]. It has been further demonstrated that cocaine effects involve 5-HTRs [24]. It is unlikely that one given physiological function does not depend on multiple neuronal systems, even if multiple studies have focused on isolated mechanisms. Subsequently, numerous studies have tested whether 5-HTRs may also contribute to both neuronal and behavioral effects of illegal drugs such as cocaine and MDMA (Ecstasy).
MDMA is a “substrate-type 5-HT releaser” [156] and is an amphetamine-like stimulant. MDMA (10 mg/kg) increases the levels of 5-HT but can also increase dopamine (DA) levels, especially at higher doses [157]. Although using different mechanisms of action, cocaine is also well known to target monoamine (DA, 5-HT, NA) transporters [158].
MDMA is a psychoactive substance, first described as an appetite-suppressant in humans, inducing side effects and even death. As summarized in Table 8.1, we have recently shown that MDMA-induced anorexia involves both 5-HT2CR [105] and 5-HT4R (Compan, unpublished). In contrast, the feeding responses to MDMA treatment were maintained in 5-HT1BR KO mice or in animals treated with the 5-HT1B/1DR antagonist GR127935 [105]. A specific dose of a 5-HT4R antagonist (RS39604) suppressed the hypophagic effect of MDMA in 5-HT1BR KO mice, suggesting that 5-HT4R functions adapt over time during development when mice are 5-HT1BR deprived (Compan, unpublished).
MDMA also elicits a dramatic increase in locomotion [159–161], which in mice involves 5-HT1BR, 5-HT2CR, and 5-HT4R [13, 105] (Compan, unpublished). Conversely, the loss of 5-HT1BR, 5-HT2C [7, 8], as well as 5-HT4R (Compan, unpublished), function has clearly enhanced cocaine-induced locomotion in the novel open field test.
5-HT RECEPTOR KNOCK-OUT MICE AND ADAPTIVE CHANGES IN CENTRAL SEROTONINERGIC SYSTEMS
Over the last two decades, studies have demonstrated that 5-HT1A autoreceptors exert a negative feedback influence on 5-HT neuron activity and 5-HT levels in the dorsal (DRN) and medial (MRN) raphe nuclei [17,120–126]. A similar conclusion has been reached following studies with 5-HT1AR KO mice, which display increased spontaneous firing of DRN 5-HT neurons (Figure 8.1) [126, 127].
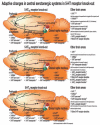
FIGURE 8.1
Adaptive changes in serotonergic neurons in 5-HT receptor knock-out mice. BO: olfactory bulb, CX: cortex, GP: globus pallidus, LC: locus coeruleus, SN: substantia nigra. (From 1. Ase, A.R. et al., J Neurochem 75(6), 2415–26, 2000; 2. Ase, A. R. (more...)
Reduced 5-HT1AR density and sensitivity may also coexist with decreased DRN 5-HT neuron activity. This is observed in monoamine oxidase-A (catabolism enzyme of 5-HT) null mice and interpreted as an overcompensation for the excessive level of 5-HT in these mice [16]. 5-HT neuron firing and the level of 5-HT in DRN are also negatively regulated by 5-HT1B autoreceptors, depending on the species examined [16, 162]. In 5-HT1BR null adult mice, subtle adaptive changes are suspected to overcome the loss-of-function mutation in 5-HT1BR, because 5-HT neuron activity and 5-HT levels are unchanged in the raphe nuclei (Figure 8.1) [16, 17]. Indeed, 5-HT neuron firing and the level of 5-HT in DRN are negatively regulated by 5-HT1B autoreceptors, depending on the species examined [16,162,163]. Although decreased 5-HT neurotransmission may exist in depressed humans [21], there is as yet no genetically modified animal for any G protein-coupled receptor that displays decreased 5-HT neuron activity and 5-HT content in the raphe nuclei. We have recently provided such an animal model, lacking 5-HT4R (Figure 8.1) [18].
CONCLUSION
It is surprising that such dramatic changes in the activity of the serotonergic system as detected in the absence of mHtr1A and mHtr4 genes did not produce more apparent behavioral deficiencies under basal conditions. The neuroplasticity of the brain over the course of development is fascinating and suggests that such adaptive neuronal mechanisms may be able to circumvent genetic deficits.
In contrast, stress-induced adaptive behavior is impaired in genetically modified animals lacking 5-HTR (see Table 8.1), suggesting some limitations on the extent of plasticity in the brain. To an extent, the brain may adapt to underline adapted behavior but appears to “burn” its adaptive resources over time under stress. To date, genetic deficits related to 5-HTR mostly produced less sensitivity to the anorectic effects of stressors, with different changes in reactivity to novelty or illegal drugs and fear states. These responses provide a focus for our efforts to further examine neural mechanisms whereby serotonin systems may take part to a mixed pattern that underline mental disease.
Acknowledgments
I greatly appreciate the assistance of Kerri Holick in editing this text.
REFERENCES
- 1.
- Azmitia EC, Whitaker-Azmitia PM. Awakening the sleeping giant: anatomy and plasticity of the brain serotonergic system. J Clin Psychiatr. 1991;52(Suppl):4–16. [PubMed: 1752858]
- 2.
- Byrne JH, Kandel ER. Presynaptic facilitation revisited: state and time dependence. J Neurosci. 1996;16(2):425–435. [PMC free article: PMC6578640] [PubMed: 8551327]
- 3.
- Heisler LK, Chu HM, Brennan TJ, Danao JA, Bajwa P, Parsons LH, Tecott LH. Elevated anxiety and antidepressant-like responses in serotonin 5-HT1A receptor mutant mice. Proc Natl Acad Sci USA. 1998;95(25):15049–15054. [PMC free article: PMC24573] [PubMed: 9844013]
- 4.
- Parks CL, Robinson PS, Sibille E, Shenk T, Toth M. Increased anxiety of mice lacking the serotonin1A receptor. Proc Natl Acad Sci USA. 1998;95(18):10734–10739. [PMC free article: PMC27964] [PubMed: 9724773]
- 5.
- Ramboz S, Oosting R, Amara DA, Kung HF, Blier P, Mendelsohn M, Mann JJ, Brunner D, Hen R. Serotonin receptor 1A knockout: an animal model of anxiety-related disorder. Proc Natl Acad Sci USA. 1998;95(24):14476–14481. [PMC free article: PMC24398] [PubMed: 9826725]
- 6.
- Brunner D, Buhot MC, Hen R, Hofer M. Anxiety, motor activation, and maternal-infant interactions in 5HT1B knockout mice. Behav Neurosci. 1999;113(3):587–601. [PubMed: 10443785]
- 7.
- Rocha BA, Scearce-Levie K, Lucas JJ, Hiroi N, Castanon N, Crabbe JC, Nestler EJ, Hen R. Increased vulnerability to cocaine in mice lacking the serotonin-1B receptor. Nature. 1998;393(6681):175–178. [PubMed: 9603521]
- 8.
- Rocha BA, Goulding EH, O'Dell LE, Mead AN, Coufal NG, Parsons LH, Tecott LH. Enhanced locomotor, reinforcing, and neurochemical effects of cocaine in serotonin 5-hydroxytryptamine 2C receptor mutant mice. J Neurosci. 2002;22(22):10039–10045. [PMC free article: PMC6757823] [PubMed: 12427861]
- 9.
- Grailhe R, Waeber C, Dulawa SC, Hornung JP, Zhuang X, Brunner D, Geyer MA, Hen R. Increased exploratory activity and altered response to LSD in mice lacking the 5-HT(5A) receptor. Neuron. 1999;22(3):581–591. [PubMed: 10197537]
- 10.
- Compan V, Zhou M, Grailhe R, Gazzara RA, Martin R, Gingrich J, Dumuis A, Brunner D, Bockaert J, Hen R. Attenuated response to stress and novelty and hypersensitivity to seizures in 5-HT4 receptor knock-out mice. J Neurosci. 2004;24(2):412–419. [PMC free article: PMC6729986] [PubMed: 14724239]
- 11.
- Compan V, Charnay Y, Dusticier N, Daszuta A, Hen R, Bockaert J. Feeding disorders in 5-HT4 receptor knockout mice. J Soc Biol. 2004;198(1):37–49. [PubMed: 15146954]
- 12.
- Weisstaub NV, Zhou M, Lira A, Lambe E, Gonzalez-Maeso J, Hornung JP, Sibille E, Underwood M, Itohara S, Dauer WT, Ansorge MS, Morelli E, Mann JJ, Toth M, Aghajanian G, Sealfon SC, Hen R, Gingrich JA. Cortical 5-HT2A receptor signaling modulates anxiety-like behaviors in mice. Science. 2006;313(5786):536–540. [PubMed: 16873667]
- 13.
- Scearce-Levie K, Viswanathan SS, Hen R. Locomotor response to MDMA is attenuated in knockout mice lacking the 5-HT1B receptor. Psychopharmacology (Berl). 1999;141(2):154–161. [PubMed: 9952039]
- 14.
- Lucas JJ, Yamamoto A, Scearce-Levie K, Saudou F, Hen R. Absence of fenfluramine-induced anorexia and reduced c-Fos induction in the hypothalamus and central amygdaloid complex of serotonin 1B receptor knock-out mice. J Neurosci. 1998;18(14):5537–5544. [PMC free article: PMC6793482] [PubMed: 9651234]
- 15.
- Tecott LH, Sun LM, Akana SF, Strack AM, Lowenstein DH, Dallman MF, Julius D. Eating disorder and epilepsy in mice lacking 5-HT2c serotonin receptors. Nature. 1995;374(6522):542–546. [PubMed: 7700379]
- 16.
- Evrard A, Laporte AM, Chastanet M, Hen R, Hamon M, Adrien J. 5-HT1A and 5-HT1B receptors control the firing of serotoninergic neurons in the dorsal raphe nucleus of the mouse: studies in 5-HT1B knock-out mice. Eur J Neurosci. 1999;11(11):3823–3831. [PubMed: 10583471]
- 17.
- Ase AR, Reader TA, Hen R, Riad M, Descarries L. Altered serotonin and dopamine metabolism in the CNS of serotonin 5-HT(1A) or 5-HT(1B) receptor knockout mice. J Neurochem. 2000;75(6):2415–2426. [PubMed: 11080193]
- 18.
- Conductier G, Dusticier N, Lucas G, Cote F, Debonnel G, Daszuta A, Dumuis A, Nieoullon A, Hen R, Bockaert J, Compan V. Adaptive changes in serotonin neurons of the raphe nuclei in 5-HT(4) receptor knock-out mouse. Eur J Neurosci. 2006;24(4):1053–1062. [PubMed: 16930432]
- 19.
- Lopez JF, Akil H, Watson SJ. Neural circuits mediating stress. Biol Psychiatr. 1999;46(11):1461–1471. [PubMed: 10599476]
- 20.
- Chaouloff F. Serotonin, stress and corticoids. J Psychopharmacol. 2000;14(2):139–151. [PubMed: 10890308]
- 21.
- Nemeroff CB, Owens MJ. Treatment of mood disorders. Nat Neurosci. 2002;5(Suppl):1068–1070. [PubMed: 12403988]
- 22.
- Vaswani M, Kalra H. Selective serotonin re-uptake inhibitors in anorexia nervosa. Expert Opin Invest Drugs. 2004;13(4):349–357. [PubMed: 15102585]
- 23.
- Leonardo ED, Hen R. Genetics of affective and anxiety disorders. Annu Rev Psychol. 2006;57:117–137. [PubMed: 16318591]
- 24.
- Muller CP, Huston JP. Determining the region-specific contributions of 5-HT receptors to the psychostimulant effects of cocaine. Trends Pharmacol Sci. 2006;27(2):105–112. [PubMed: 16406129]
- 25.
- Hoyer D, Hannon JP, Martin GR. Molecular, pharmacological and functional diversity of 5-HT receptors. Pharmacol Biochem Behav. 2002;71(4):533–554. [PubMed: 11888546]
- 26.
- Bockaert J, Claeysen S, Becamel C, Dumuis A, Marin P. Neuronal 5-HT metabotropic receptors: fine-tuning of their structure, signaling, and roles in synaptic modulation. Cell Tissue Res. 2006;326:553–572. [PubMed: 16896947]
- 27.
- Saudou F, Amara DA, Dierich A, LeMeur M, Ramboz S, Segu L, Buhot MC, Hen R. Enhanced Aggressive Behavior in Mice Lacking 5-HT1B Receptor. Science. 1994;265(5180):1875–1878. [PubMed: 8091214]
- 28.
- Nebigil CG, Choi DS, Dierich A, Hickel P, Le Meur M, Messaddeq N, Launay JM, Maroteaux L. Serotonin 2B receptor is required for heart development. Proc Natl Acad Sci USA. 2000;97(17):9508–9513. [PMC free article: PMC16895] [PubMed: 10944220]
- 29.
- Zeitz KP, Guy N, Malmberg AB, Dirajlal S, Martin WJ, Sun L, Bonhaus DW, Stucky CL, Julius D, Basbaum AI. The 5-HT3 subtype of serotonin receptor contributes to nociceptive processing via a novel subset of myelinated and unmyelinated nociceptors. J Neurosci. 2002;22(3):1010–1019. [PMC free article: PMC6758503] [PubMed: 11826129]
- 30.
- Guscott MR, Egan E, Cook GP, Stanton JA, Beer MS, Rosahl TW, Hartmann S, Kulagowski J, McAllister G, Fone KC, Hutson PH. The hypothermic effect of 5-CT in mice is mediated through the 5-HT7 receptor. Neuropharmacology. 2003;44(8):1031–1037. [PubMed: 12763096]
- 31.
- Hedlund PB, Danielson PE, Thomas EA, Slanina K, Carson MJ, Sutcliffe JG. No hypothermic response to serotonin in 5-HT7 receptor knockout mice. Proc Natl Acad Sci USA. 2003;100(3):1375–1380. [PMC free article: PMC298780] [PubMed: 12529502]
- 32.
- Donohoe TP. Stress-induced anorexia: implications for anorexia nervosa. Life Sci. 1984;34(3):203–218. [PubMed: 6363858]
- 33.
- Casper RC. Depression and eating disorders. Depression Anxiety. 1998;8(Suppl 1):96–104. [PubMed: 9809221]
- 34.
- Godart NT, Flament MF, Lecrubier Y, Jeammet P. Anxiety disorders in anorexia nervosa and bulimia nervosa: co-morbidity and chronology of appearance. Eur Psychiatr. 2000;15(1):38–45. [PubMed: 10713801]
- 35.
- Miyake A, Mochizuki S, Takemoto Y, Akuzawa S. Molecular cloning of human 5-hydroxytryptamine3 receptor: heterogeneity in distribution and function among species. Mol Pharmacol. 1995;48(3):407–416. [PubMed: 7565620]
- 36.
- Karnovsky AM, Gotow LF, McKinley DD, Piechan JL, Ruble CL, Mills CJ, Schellin KA, Slightom JL, Fitzgerald LR, Benjamin CW, Roberds SL. A cluster of novel serotonin receptor 3-like genes on human chromosome 3. Gene. 2003;319:137–148. [PubMed: 14597179]
- 37.
- Niesler B, Frank B, Kapeller J, Rappold GA. Cloning, physical mapping and expression analysis of the human 5-HT3 serotonin receptor-like genes HTR3C, HTR3D and HTR3E. Gene. 2003;310:101–111. [PubMed: 12801637]
- 38.
- Serretti A, Benedetti F, Zanardi R, Smeraldi E. The influence of serotonin transporter promoter polymorphism (SERTPR) and other polymorphisms of the serotonin pathway on the efficacy of antidepressant treatments. Prog Neuropsychopharmacol Biol Psychiatr. 2005;29(6):1074–1084. [PubMed: 15939518]
- 39.
- Del Tredici AL, Schiffer HH, Burstein ES, Lameh J, Mohell N, Hacksell U, Brann MR, Weiner DM. Pharmacology of polymorphic variants of the human 5-HT1A receptor. Biochem Pharmacol. 2004;67(3):479–490. [PubMed: 15037200]
- 40.
- Huang YY, Grailhe R, Arango V, Hen R, Mann JJ. Relationship of psychopathology to the human serotonin1B genotype and receptor binding kinetics in postmortem brain tissue. Neuropsychopharmacology. 1999;21(2):238–246. [PubMed: 10432472]
- 41.
- Hazelwood LA, Sanders-Bush E. His452Tyr polymorphism in the human 5-HT2A receptor destabilizes the signaling conformation. Mol Pharmacol. 2004;66(5):1293–1300. [PubMed: 15496511]
- 42.
- Khait VD, Huang YY, Zalsman G, Oquendo MA, Brent DA, Harkavy-Fried-man JM, Mann JJ. Association of serotonin 5-HT2A receptor binding and the T102C polymorphism in depressed and healthy Caucasian subjects. Neuropsychopharmacology. 2005;30(1):166–172. [PubMed: 15483560]
- 43.
- Davies MA, Setola V, Strachan RT, Sheffler DJ, Salay E, Hufeisen SJ, Roth BL. Pharmacologic analysis of non-synonymous coding h5-HT2A SNPs reveals alterations in atypical antipsychotic and agonist efficacies. Pharmacogenomics J. 2006;6(1):42–51. [PubMed: 16314884]
- 44.
- Ozaki N, Manji H, Lubierman V, Lu SJ, Lappalainen J, Rosenthal NE, Goldman D. A naturally occurring amino acid substitution of the human serotonin 5-HT2A receptor influences amplitude and timing of intracellular calcium mobilization. J Neurochem. 1997;68(5):2186–2193. [PubMed: 9109547]
- 45.
- Reist C, Mazzanti C, Vu R, Fujimoto K, Goldman D. Inter-relationships of intermediate phenotypes for serotonin function, impulsivity, and a 5-HT2A candidate allele: His452Tyr. Mol Psychiatr. 2004;9(9):871–878. [PubMed: 15037867]
- 46.
- Sanders-Bush E, Fentress H, Hazelwood L. Serotonin 5-ht2 receptors: molecular and genomic diversity. Mol Interv. 2003;3(6):319–330. [PubMed: 14993464]
- 47.
- Okada M, Northup JK, Ozaki N, Russell JT, Linnoila M, Goldman D. Modification of human 5-HT(2C) receptor function by Cys23Ser, an abundant, naturally occurring amino-acid substitution. Mol Psychiatr. 2004;9(1):55–64. [PubMed: 14699441]
- 48.
- De Deurwaerdere P, Navailles S, Berg KA, Clarke WP, Spampinato U. Constitutive activity of the serotonin2C receptor inhibits in vivo dopamine release in the rat striatum and nucleus accumbens. J Neurosci. 2004;24(13):3235–3241. [PMC free article: PMC6730027] [PubMed: 15056702]
- 49.
- Navailles S, De Deurwaerdere P, Spampinato U. Clozapine and haloperidol differentially alter the constitutive activity of central serotonin2C receptors in vivo. Biol Psychiatr. 2006;59(6):568–575. [PubMed: 16182256]
- 50.
- Nothen MM, Rietschel M, Erdmann J, Oberlander H, Moller HJ, Nober D, Propping P. Genetic variation of the 5-HT2A receptor and response to clozapine. Lancet. 1995;346(8979):908–909. [PubMed: 7564704]
- 51.
- Nacmias B, Ricca V, Tedde A, Mezzani B, Rotella CM, Sorbi S. 5-HT2A receptor gene polymorphisms in anorexia nervosa and bulimia nervosa. Neurosci Lett. 1999;277(2):134–136. [PubMed: 10624828]
- 52.
- Ziegler A, Hebebrand J, Gorg T, Rosenkranz K, Fichter M, Herpertz-Dahlmann B, Remschmidt H, Hinney A. Further lack of association between the 5-HT2A gene promoter polymorphism and susceptibility to eating disorders and a meta-analysis pertaining to anorexia nervosa. Mol Psychiatr. 1999;4(5):410–412. [PubMed: 10523809]
- 53.
- Nishiguchi N, Matsushita S, Suzuki K, Murayama M, Shirakawa O, Higuchi S. Association between 5HT2A receptor gene promoter region polymorphism and eating disorders in Japanese patients. Biol Psychiatr. 2001;50(2):123–128. [PubMed: 11526993]
- 54.
- Karwautz A, Rabe-Hesketh S, Hu X, Zhao J, Sham P, Collier DA, Treasure JL. Individual-specific risk factors for anorexia nervosa: a pilot study using a discordant sister-pair design. Psychol Med. 2001;31(2):317–329. [PubMed: 11232918]
- 55.
- Gorwood P, Ades J, Bellodi L, Cellini E, Collier DA, Di Bella D, Di Bernardo M, Estivill X, Fernandez-Aranda F, Gratacos M, Hebebrand J, Hinney A, Hu X, Karwautz A, Kipman A, Mouren-Simeoni MC, Nacmias B, Ribases M, Remschmidt H, Ricca V, Rotella CM, Sorbi S, Treasure J. The 5-HT(2A) -1438G/A polymorphism in anorexia nervosa: a combined analysis of 316 trios from six European centres. Mol Psychiatr. 2002;7(1):90–94. [PubMed: 11803452]
- 56.
- Ricca V, Nacmias B, Cellini E, Di Bernardo M, Rotella CM, Sorbi S. 5-HT2A receptor gene polymorphism and eating disorders. Neurosci Lett. 2002;323(2):105–108. [PubMed: 11950504]
- 57.
- Bruce KR, Steiger H, Joober R, Ng Ying Kin NM, Israel M, Young SN. Association of the promoter polymorphism -1438G/A of the 5-HT2A receptor gene with behavioral impulsiveness and serotonin function in women with bulimia nervosa. Am J Med Genet B Neuropsychiatr Genet. 2005;137(1):40–44. [PubMed: 15999344]
- 58.
- Aubert R, Betoulle D, Herbeth B, Siest G, Fumeron F. 5-HT2A receptor gene polymorphism is associated with food and alcohol intake in obese people. Int J Obes Relat Metab Disord. 2000;24(7):920–924. [PubMed: 10918541]
- 59.
- Lentes KU, Hinney A, Ziegler A, Rosenkranz K, Wurmser H, Barth N, Jacob K, Coners H, Mayer H, Grzeschik KH, Schafer H, Remschmidt H, Pirke KM, Hebebrand J. Evaluation of a Cys23Ser mutation within the human 5-HT2C receptor gene: no evidence for an association of the mutant allele with obesity or underweight in children, adolescents and young adults. Life Sci. 1997;61(1):PL9–16. [PubMed: 9200673]
- 60.
- Burnet PW, Smith KA, Cowen PJ, Fairburn CG, Harrison PJ. Allelic variation of the 5-HT2C receptor (HTR2C) in bulimia nervosa and binge eating disorder. Psychiatr Genet. 1999;9(2):101–104. [PubMed: 10412190]
- 61.
- Yuan X, Yamada K, Ishiyama-Shigemoto S, Koyama W, Nonaka K. Identification of polymorphic loci in the promoter region of the serotonin 5-HT2C receptor gene and their association with obesity and type II diabetes. Diabetologia. 2000;43(3):373–376. [PubMed: 10768099]
- 62.
- Reynolds GP, Zhang ZJ, Zhang XB. Association of antipsychotic drug-induced weight gain with a 5-HT2C receptor gene polymorphism. Lancet. 2002;359(9323):2086–2087. [PubMed: 12086765]
- 63.
- Reynolds GP, Zhang Z, Zhang X. Polymorphism of the promoter region of the serotonin 5-HT(2C) receptor gene and clozapine-induced weight gain. Am J Psychiatr. 2003;160(4):677–679. [PubMed: 12668355]
- 64.
- McCarthy S, Mottagui-Tabar S, Mizuno Y, Sennblad B, Hoffstedt J, Arner P, Wahlestedt C, Andersson B. Complex HTR2C linkage disequilibrium and promoter associations with body mass index and serum leptin. Hum Genet. 2005;117(6):545–557. [PubMed: 16021472]
- 65.
- Pooley EC, Fairburn CG, Cooper Z, Sodhi MS, Cowen PJ, Harrison PJ. A 5-HT2C receptor promoter polymorphism (HTR2C — 759C/T) is associated with obesity in women, and with resistance to weight loss in heterozygotes. Am J Med Genet B Neuropsychiatr Genet. 2004;126(1):124–127. [PubMed: 15048662]
- 66.
- Kishore S, Stamm S. The snoRNA HBII-52 regulates alternative Splicing of the serotonin receptor 2C. Science. 2006;311(5758):230–232. [PubMed: 16357227]
- 67.
- Westberg L, Bah J, Rastam M, Gillberg C, Wentz E, Melke J, Hellstrand M, Eriksson E. Association between a polymorphism of the 5-HT2C receptor and weight loss in teenage girls. Neuropsychopharmacology. 2002;26(6):789–793. [PubMed: 12007749]
- 68.
- Miller del D, Ellingrod VL, Holman TL, Buckley PF, Arndt S. Clozapine-induced weight gain associated with the 5HT2C receptor -759C/T polymorphism. Am J Med Genet B Neuropsychiatr Genet. 2005;133(1):97–100. [PubMed: 15635667]
- 69.
- Hinney A, Herrmann H, Lohr T, Rosenkranz K, Ziegler A, Lehmkuhl G, Poustka F, Schmidt MH, Mayer H, Siegfried W, Remschmidt H, Hebebrand J. No evidence for an involvement of alleles of polymorphisms in the serotonin1Dbeta and 7 receptor genes in obesity, underweight or anorexia nervosa. Int J Obes Relat Metab Disord. 1999;23(7):760–763. [PubMed: 10454111]
- 70.
- Levitan RD, Kaplan AS, Masellis M, Basile VS, Walker ML, Lipson N, Siegel GI, Woodside DB, Macciardi FM, Kennedy SH, Kennedy JL. Polymorphism of the serotonin 5-HT1B receptor gene (HTR1B) associated with minimum lifetime body mass index in women with bulimia nervosa. Biol Psychiatr. 2001;50(8):640–643. [PubMed: 11690602]
- 71.
- Levitan RD, Kaplan AS, Masellis M, Basile VS, Richter MA, Kennedy JL. The serotonin-1Dbeta receptor gene and severity of obsessive-compulsive disorder in women with bulimia nervosa. Eur Neuropsychopharmacol. 2006;16(1):1–6. [PubMed: 15944142]
- 72.
- Hammoumi S, Payen A, Favre JD, Balmes JL, Benard JY, Husson M, Ferrand JP, Martin JP, Daoust M. Does the short variant of the serotonin transporter linked polymorphic region constitute a marker of alcohol dependence? Alcohol. 1999;17(2):107–112. [PubMed: 10064377]
- 73.
- Himei A, Kono Y, Yoneda H, Sakai T, Koh J, Sakai J, Inada Y, Imamichi H. An association study between alcoholism and the serotonergic receptor genes. Alcohol Clin Exp Res. 2000;24(3):341–342. [PubMed: 10776672]
- 74.
- Lappalainen J, Long JC, Eggert M, Ozaki N, Robin RW, Brown GL, Naukkarinen H, Virkkunen M, Linnoila M, Goldman D. Linkage of antisocial alcoholism to the serotonin 5-HT1B receptor gene in 2 populations. Arch Gen Psychiatr. 1998;55(11):989–994. [PubMed: 9819067]
- 75.
- Hasegawa Y, Higuchi S, Matsushita S, Miyaoka H. Association of a polymorphism of the serotonin 1B receptor gene and alcohol dependence with inactive aldehyde dehydrogenase-2. J Neural Transm. 2002;109(4):513–521. [PubMed: 11956970]
- 76.
- Fehr C, Szegedi A, Anghelescu I, Klawe C, Hiemke C, Dahmen N. Sex differences in allelic frequencies of the 5-HT2C Cys23Ser polymorphism in psychiatric patients and healthy volunteers: findings from an association study. Psychiatr Genet. 2000;10(2):59–65. [PubMed: 10994642]
- 77.
- Soyka M, Preuss UW, Koller G, Zill P, Bondy B. Association of 5-HT1B receptor gene and antisocial behavior in alcoholism. J Neural Transm. 2004;111(1):101–109. [PubMed: 14714219]
- 78.
- Cigler T, LaForge KS, McHugh PF, Kapadia SU, Leal SM, Kreek MJ. Novel and previously reported single-nucleotide polymorphisms in the human 5-HT(1B) receptor gene: no association with cocaine or alcohol abuse or dependence. Am J Med Genet. 2001;105(6):489–497. [PMC free article: PMC6148750] [PubMed: 11496363]
- 79.
- Gorwood P, Aissi F, Batel P, Ades J, Cohen-Salmon C, Hamon M, Boni C, Lanfumey L. Reappraisal of the serotonin 5-HT(1B) receptor gene in alcoholism: of mice and men. Brain Res Bull. 2002;57(1):103–107. [PubMed: 11827742]
- 80.
- Kranzler HR, Hernandez-Avila CA, Gelernter J. Polymorphism of the 5-HT1B receptor gene (HTR1B): strong within-locus linkage disequilibrium without association to antisocial substance dependence. Neuropsychopharmacology. 2002;26(1):115–122. [PubMed: 11751038]
- 81.
- Huang YY, Oquendo MA, Friedman JM, Greenhill LL, Brodsky B, Malone KM, Khait V, Mann JJ. Substance abuse disorder and major depression are associated with the human 5-HT1B receptor gene (HTR1B) G861C polymorphism. Neuropsychopharmacology. 2003;28(1):163–169. [PubMed: 12496953]
- 82.
- Sinha R, Cloninger CR, Parsian A. Linkage disequilibrium and haplotype analysis between serotonin receptor 1B gene variations and subtypes of alcoholism. Am J Med Genet B Neuropsychiatr Genet. 2003;121(1):83–88. [PubMed: 12898580]
- 83.
- Sun HF, Chang YT, Fann CS, Chang CJ, Chen YH, Hsu YP, Yu WY, Cheng AT. Association study of novel human serotonin 5-HT(1B) polymorphisms with alcohol dependence in Taiwanese Han. Biol Psychiatr. 2002;51(11):896–901. [PubMed: 12022963]
- 84.
- Hwu HG, Chen CH. Association of 5HT2A receptor gene polymorphism and alcohol abuse with behavior problems. Am J Med Genet. 2000;96(6):797–800. [PubMed: 11121184]
- 85.
- Schuckit MA, Mazzanti C, Smith TL, Ahmed U, Radel M, Iwata N, Goldman D. Selective genotyping for the role of 5-HT2A, 5-HT2C, and GABA alpha 6 receptors and the serotonin transporter in the level of response to alcohol: a pilot study. Biol Psychiatr. 1999;45(5):647–651. [PubMed: 10088053]
- 86.
- Fehr C, Schleicher A, Szegedi A, Anghelescu I, Klawe C, Hiemke C, Dahmen N. Serotonergic polymorphisms in patients suffering from alcoholism, anxiety disorders and narcolepsy. Prog Neuropsychopharmacol Biol Psychiatr. 2001;25(5):965–982. [PubMed: 11444684]
- 87.
- Nakamura T, Matsushita S, Nishiguchi N, Kimura M, Yoshino A, Higuchi S. Association of a polymorphism of the 5HT2A receptor gene promoter region with alcohol dependence. Mol Psychiatr. 1999;4(1):85–88. [PubMed: 10089015]
- 88.
- Galeeva AR, Iur’ev EB, Khusnutdinova EK. Polymorphism of the serotonin 2A receptor gene in populations from the Volga-Ural region. Genetika. 2001;37(11):1581–1584. [PubMed: 11771315]
- 89.
- Lappalainen J, Long JC, Virkkunen M, Ozaki N, Goldman D, Linnoila M. HTR2C Cys23Ser polymorphism in relation to CSF monoamine metabolite concentrations and DSM-III-R psychiatric diagnoses. Biol Psychiatr. 1999;46(6):821–826. [PubMed: 10494451]
- 90.
- Wodarz N, Lange K, Laufkotter R, Johann M. ADHD and alcohol dependence: a common genetic predisposition? Psychiatr Prax. 2004;31(Suppl 1):S111–113. [PubMed: 15570522]
- 91.
- Johann M, Bobbe G, Putzhammer A, Wodarz N. Comorbidity of alcohol dependence with attention-deficit hyperactivity disorder: differences in phenotype with increased severity of the substance disorder, but not in genotype (serotonin transporter and 5-hydroxytryptamine-2c receptor). Alcohol Clin Exp Res. 2003;27(10):1527–1534. [PubMed: 14574222]
- 92.
- Samochowiec J, Smolka M, Winterer G, Rommelspacher H, Schmidt LG, Sander T. Association analysis between a Cys23Ser substitution polymorphism of the human 5-HT2c receptor gene and neuronal hyperexcitability. Am J Med Genet. 1999;88(2):126–130. [PubMed: 10206230]
- 93.
- Proudnikov D, LaForge KS, Hofflich H, Levenstien M, Gordon D, Barral S, Ott J, Kreek MJ. Association analysis of polymorphisms in serotonin 1B receptor (HTR1B) gene with heroin addiction: a comparison of molecular and statistically estimated haplotypes. Pharmacogenet Genomics. 2006;16(1):25–36. [PubMed: 16344719]
- 94.
- Lin Z, Walther D, Yu XY, Drgon T, Uhl GR. The human serotonin receptor 2B: coding region polymorphisms and association with vulnerability to illegal drug abuse. Pharmacogenetics. 2004;14(12):805–811. [PubMed: 15608559]
- 95.
- Li T, Liu X, Zhao J, Hu X, Ball DM, Loh el W, Sham PC, Collier DA. Allelic association analysis of the dopamine D2, D3, 5-HT2A, and GABA(A)gamma2 receptors and serotonin transporter genes with heroin abuse in Chinese subjects. Am J Med Genet. 2002;114(3):329–335. [PubMed: 11920858]
- 96.
- Uhl GR, Liu QR, Walther D, Hess J, Naiman D. Polysubstance abuse-vulnerability genes: genome scans for association, using 1,004 subjects and 1,494 single-nucleotide polymorphisms. Am J Hum Genet. 2001;69(6):1290–1300. [PMC free article: PMC1235541] [PubMed: 11704927]
- 97.
- Jones-Chesters MH, Monsell S, Cooper PJ. The disorder-salient stroop effect as a measure of psychopathology in eating disorders. Int J Eat Disord. 1998;24(1):65–82. [PubMed: 9589312]
- 98.
- Drysdale CM, McGraw DW, Stack CB, Stephens JC, Judson RS, Nandabalan K, Arnold K, Ruano G, Liggett SB. Proc Natl Acad Sci USA. 19. Vol. 97. 2000. Complex promoter and coding region beta 2-adrenergic receptor haplotypes alter receptor expression and predict in vivo responsiveness; pp. 10483–10488. [PMC free article: PMC27050] [PubMed: 10984540]
- 99.
- Sullivan PF. Mortality in anorexia nervosa. Am J Psychiatr. 1995;152(7):1073–1074. [PubMed: 7793446]
- 100.
- Meguid MM, Fetissov SO, Varma M, Sato T, Zhang L, Laviano A, Rossi-Fanelli F. Hypothalamic dopamine and serotonin in the regulation of food intake. Nutrition. 2000;16(10):843–857. [PubMed: 11054589]
- 101.
- Naqvi TZ, Gross SB. Anorexigen-induced cardiac valvulopathy and female gender. Curr Womens Health Rep. 2003;3(2):116–125. [PubMed: 12628081]
- 102.
- O'Connor KA, Roth BL. Finding new tricks for old drugs: an efficient route for public-sector drug discovery. Nat Rev Drug Discov. 2005;4(12):1005–1014. [PubMed: 16341065]
- 103.
- Rochester JA, Kirchner JT. Ecstasy (3,4-methylenedioxymethamphetamine): history, neurochemistry, and toxicology. J Am Board Fam Pract. 1999;12(2):137–142. [PubMed: 10220237]
- 104.
- Frith CH, Chang LW, Lattin DL, Walls RC, Hamm J, Doblin R. Toxicity of methylenedioxymethamphetamine (MDMA) in the dog and the rat. Fundam Appl Toxicol. 1987;9(1):110–119. [PubMed: 2887476]
- 105.
- Conductier G, Crosson C, Hen R, Bockaert J, Compan V. 3,4-N-methlene-dioxymethamphetamine-induced hypophagia is maintained in 5-HT1B receptor knockout mice, but suppressed by the 5-HT2C receptor antagonist RS102221. Neuropsychopharmacology. 2005;30(6):1056–1063. [PubMed: 15668722]
- 106.
- Heisler LK, Jobst EE, Sutton GM, Zhou L, Borok E, Thornton-Jones Z, Liu HY, Zigman JM, Balthasar N, Kishi T, Lee CE, Aschkenasi CJ, Zhang CY, Yu J, Boss O, Mountjoy KG, Clifton PG, Lowell BB, Friedman JM, Horvath T, Butler AA, Elmquist JK, Cowley MA. Serotonin reciprocally regulates melanocortin neurons to modulate food intake. Neuron. 2006;51(2):239–249. [PubMed: 16846858]
- 107.
- Kennett GA, Curzon G. Evidence that hypophagia induced by mCPP and TFMPP requires 5-HT1C and 5-HT1B receptors; hypophagia induced by RU 24969 only requires 5-HT1B receptors. Psychopharmacology (Berl). 1988;96(1):93–100. [PubMed: 2906446]
- 108.
- Klodzinska A, Chojnacka-Wojcik E. Anorexia induced by M-trifluoromethylphenylpiperazine (TFMPP) in rats. Pol J Pharmacol Pharm. 1990;42(1):13–17. [PubMed: 2281017]
- 109.
- Yamada J, Sugimoto Y, Yoshikawa T, Noma T, Horisaka K. The effects of peripheral serotonin2 receptor agonist on food intake of rats. Adv Exp Med Biol. 1996;398:555–557. [PubMed: 8906323]
- 110.
- Vickers SP, Clifton PG, Dourish CT, Tecott LH. Reduced satiating effect of d-fenfluramine in serotonin 5-HT(2C) receptor mutant mice. Psychopharmacology (Berl). 1999;143(3):309–314. [PubMed: 10353435]
- 111.
- Vickers SP, Dourish CT, Kennett GA. Evidence that hypophagia induced by d-fenfluramine and d-norfenfluramine in the rat is mediated by 5-HT2C receptors. Neuropharmacology. 2001;41(2):200–209. [PubMed: 11489456]
- 112.
- Clifton PG, Lee MD, Somerville EM, Kennett GA, Dourish CT. 5-HT1B receptor knockout mice show a compensatory reduction in 5-HT2C receptor function. Eur J Neurosci. 2003;17(1):185–190. [PubMed: 12534984]
- 113.
- Heisler LK, Cowley MA, Tecott LH, Fan W, Low MJ, Smart JL, Rubinstein M, Tatro JB, Marcus JN, Holstege H, Lee CE, Cone RD, Elmquist JK. Activation of central melanocortin pathways by fenfluramine. Science. 2002;297(5581):609–611. [PubMed: 12142539]
- 114.
- Dulawa SC, Gross C, Stark KL, Hen R, Geyer MA. Knockout mice reveal opposite roles for serotonin 1A and 1B receptors in prepulse inhibition. Neuropsychopharmacology. 2000;22(6):650–659. [PubMed: 10788764]
- 115.
- Bouwknecht JA, van der Gugten J, Hijzen TH, Maes RA, Hen R, Olivier B. Male and female 5-HT(1B) receptor knockout mice have higher body weights than wildtypes. Physiol Behav. 2001;74:4–5. 507–516. [PubMed: 11790410]
- 116.
- Dirks A, Pattij T, Bouwknecht JA, Westphal TT, Hijzen TH, Groenink L, van der Gugten J, Oosting RS, Hen R, Geyer MA, Olivier B. 5-HT1B receptor knockout, but not 5-HT1A receptor knockout mice, show reduced startle reactivity and footshock-induced sensitization, as measured with the acoustic startle response. Behav Brain Res. 2001;118(2):169–178. [PubMed: 11164514]
- 117.
- Kelley SP, Bratt AM, Hodge CW. Targeted gene deletion of the 5-HT3A receptor subunit produces an anxiolytic phenotype in mice. Eur J Pharmacol. 2003;461(1):19–25. [PubMed: 12568911]
- 118.
- Aulakh CS, Hill JL, Murphy DL. A comparison of feeding and locomotion responses to serotonin agonists in three rat strains. Pharmacol Biochem Behav. 1988;31(3):567–571. [PubMed: 2977992]
- 119.
- Bendotti C, Samanin R. The role of putative 5-HT1A and 5-HT1B receptors in the control of feeding in rats. Life Sci. 1987;41(5):635–642. [PubMed: 2955189]
- 120.
- Sprouse JS, Aghajanian GK. Electrophysiological responses of serotoninergic dorsal raphe neurons to 5-HT1A and 5-HT1B agonists. Synapse. 1987;1(1):3–9. [PubMed: 3505364]
- 121.
- Haj-Dahmane S, Hamon M, Lanfumey L. K+ channel and 5-hydroxytryptamine1A autoreceptor interactions in the rat dorsal raphe nucleus: an in vitro electrophysiological study. Neuroscience. 1991;41:2–3. 495–505. [PubMed: 1714550]
- 122.
- Le Poul E, Lima L, Laporte AM, Even C, Doucet E, Fattaccini CM, Laaris N, Hamon M, Lanfumey L. Central serotonin receptors and chronic treatment with selective serotonin reuptake inhibitors in the rat: comparative effects of fluoxetine and paroxetine. Encephale. 1995;21(2):123–132. [PubMed: 7781583]
- 123.
- Casanovas JM, Lesourd M, Artigas F. The effect of the selective 5-HT1A agonists alnespirone (S-20499) and 8-OH-DPAT on extracellular 5-hydroxytryptamine in different regions of rat brain. Br J Pharmacol. 1997;122(4):733–741. [PMC free article: PMC1564978] [PubMed: 9375971]
- 124.
- Casanovas JM, Berton O, Celada P, Artigas F. In vivo actions of the selective 5-HT1A receptor agonist BAY x 3702 on serotonergic cell firing and release. Naunyn Schmiedebergs Arch Pharmacol. 2000;362(3):248–254. [PubMed: 10997727]
- 125.
- Celada P, Puig MV, Casanovas JM, Guillazo G, Artigas F. Control of dorsal raphe serotonergic neurons by the medial prefrontal cortex: involvement of serotonin-1A, GABA(A), and glutamate receptors. J Neurosci. 2001;21(24):9917–9929. [PMC free article: PMC6763042] [PubMed: 11739599]
- 126.
- Bortolozzi A, Amargos-Bosch M, Toth M, Artigas F, Adell A. In vivo efflux of serotonin in the dorsal raphe nucleus of 5-HT1A receptor knockout mice. J Neurochem. 2004;88(6):1373–1379. [PubMed: 15009637]
- 127.
- Richer M, Hen R, Blier P. Modification of serotonin neuron properties in mice lacking 5-HT1A receptors. Eur J Pharmacol. 2002;435:2–3. 195–203. [PubMed: 11821026]
- 128.
- Dulawa SC, Hen R, Scearce-Levie K, Geyer MA. Serotonin1B receptor modulation of startle reactivity, habituation, and prepulse inhibition in wild-type and serotonin1B knockout mice. Psychopharmacology (Berl). 1997;132(2):125–134. [PubMed: 9266609]
- 129.
- Boutrel B, Franc B, Hen R, Hamon M, Adrien J. Key role of 5-HT1B receptors in the regulation of paradoxical sleep as evidenced in 5-HT1B knock-out mice. J Neurosci. 1999;19(8):3204–3212. [PMC free article: PMC6782285] [PubMed: 10191333]
- 130.
- Inoue T, Tsuchiya K, Koyama T. Regional changes in dopamine and serotonin activation with various intensity of physical and psychological stress in the rat brain. Pharmacol Biochem Behav. 1994;49(4):911–920. [PubMed: 7886107]
- 131.
- Ge J, Barnes NM, Costall B, Naylor RJ. Effect of aversive stimulation on 5-hydroxytryptamine and dopamine metabolism in the rat brain. Pharmacol Biochem Behav. 1997;58(3):775–783. [PubMed: 9329072]
- 132.
- Konstandi M, Johnson E, Lang MA, Malamas M, Marselos M. Noradrenaline, dopamine, serotonin: different effects of psychological stress on brain biogenic amines in mice and rats. Pharmacol Res. 2000;41(3):341–346. [PubMed: 10675287]
- 133.
- Grignaschi G, Mantelli B, Samanin R. The hypophagic effect of restraint stress in rats can be mediated by 5-HT2 receptors in the paraventricular nucleus of the hypothalamus. Neurosci Lett. 1993;152:1–2. 103–106. [PubMed: 8515859]
- 134.
- Chou-Green JM, Holscher TD, Dallman MF, Akana SF. Repeated stress in young and old 5-HT(2C) receptor knockout mice. Physiol Behav. 2003;79(2):217–226. [PubMed: 12834793]
- 135.
- Gross C, Zhuang X, Stark K, Ramboz S, Oosting R, Kirby L, Santarelli L, Beck S, Hen R. Serotonin1A receptor acts during development to establish normal anxiety-like behaviour in the adult. Nature. 2002;416(6879):396–400. [PubMed: 11919622]
- 136.
- Lucas G, Compan V, Charnay Y, Neve RL, Nestler EJ, Bockaert J, Barrot M, Debonnel G. Frontocortical 5-HT4 receptors exert positive feedback on serotonergic activity: viral transfections, subacute and chronic treatments with 5-HT4 agonists. Biol Psychiatr. 2005;57(8):918–925. [PubMed: 15820713]
- 137.
- Holick KA, Dulawa S, Compan V, Hen R. Abstract viewer/Itinerary Planner. Washington, D.C., : 2005. The contribution of 5-HT1A and 5-HT4 receptors to the effects of fluoxetine in the mouse, Society for Neuroscience Online. (Program N°567.14.)
- 138.
- Ohtsuki T, Ishiguro H, Detera-Wadleigh SD, Toyota T, Shimizu H, Yamada K, Yoshitsugu K, Hattori E, Yoshikawa T, Arinami T. Association between serotonin 4 receptor gene polymorphisms and bipolar disorder in Japanese case-control samples and the NIMH genetics initiative bipolar pedigrees. Mol Psychiatr. 2002;7(9):954–961. [PubMed: 12399948]
- 139.
- Rosel P, Arranz B, Urretavizcaya M, Oros M, San L, Navarro MA. Altered 5-HT2A and 5-HT4 postsynaptic receptors and their intracellular signalling systems IP3 and cAMP in brains from depressed violent suicide victims. Neuropsychobiology. 2004;49(4):189–195. [PubMed: 15118356]
- 140.
- Barrot M, Wallace DL, Bolanos CA, Graham DL, Perrotti LI, Neve RL, Chambliss H, Yin JC, Nestler EJ. Regulation of anxiety and initiation of sexual behavior by CREB in the nucleus accumbens. Proc Natl Acad Sci USA. 2005;102(23):8357–8362. [PMC free article: PMC1149417] [PubMed: 15923261]
- 141.
- Carlezon WA Jr, Duman RS, Nestler EJ. The many faces of CREB. Trends Neurosci. 2005;28(8):436–445. [PubMed: 15982754]
- 142.
- Berthouze M, Ayoub M, Russo O, Rivail L, Sicsic S, Fischmeister R, Berque-Bestel I, Jockers R, Lezoualc'h F. Constitutive dimerization of human serotonin 5-HT4 receptors in living cells. FEBS Lett. 2005;579(14):2973–2980. [PubMed: 15896782]
- 143.
- Hunter RG, Philpot K, Vicentic A, Dominguez G, Hubert GW, Kuhar MJ. CART in feeding and obesity. Trends Endocrinol Metab. 2004;15(9):454–459. [PubMed: 15519893]
- 144.
- Cichon S, Kesper K, Propping P, Nothen MM. Assignment of the human serotonin 4 receptor gene (HTR4) to the long arm of chromosome 5 (5q31–q33). Mol Membr Biol. 1998;15(2):75–78. [PubMed: 9724925]
- 145.
- Echwald SM, Sorensen TI, Andersen T, Hansen C, Tommerup N, Peder-sen O. Sequence variants in the human cocaine and amphetamine-regulated transcript (CART) gene in subjects with early onset obesity. Obes Res. 1999;7(6):532–536. [PubMed: 10574510]
- 146.
- Douglass J, Daoud S. Characterization of the human cDNA and genomic DNA encoding CART: a cocaine- and amphetamine-regulated transcript. Gene. 1996;169(2):241–245. [PubMed: 8647455]
- 147.
- Lowry CA, Moore FL. Regulation of behavioral responses by corticotropin-releasing factor. Gen Comp Endocrinol. 2006;146(1):19–27. [PubMed: 16426606]
- 148.
- Zhuang X, Gross C, Santarelli L, Compan V, Trillat AC, Hen R. Altered emotional states in knockout mice lacking 5-HT1A or 5-HT1B receptors. Neuropsychopharmacology. 1999;21(2 Suppl):52S–60S. [PubMed: 10432489]
- 149.
- Hodge CW, Kelley SP, Bratt AM, Iller K, Schroeder JP, Besheer J. 5-HT(3A) receptor subunit is required for 5-HT3 antagonist-induced reductions in alcohol drinking. Neuropsychopharmacology. 2004;29(10):1807–1813. [PubMed: 15162158]
- 150.
- Bhatnagar S, Nowak N, Babich L, Bok L. Deletion of the 5-HT3 receptor differentially affects behavior of males and females in the Porsolt forced swim and defensive withdrawal tests. Behav Brain Res. 2004;153(2):527–535. [PubMed: 15265651]
- 151.
- Bonasera SJ, Chu HM, Brennan TJ, Tecott LH. A null mutation of the serotonin 6 receptor alters acute responses to ethanol. Neuropsychopharmacology. 2006;31(8):1801–1813. [PubMed: 16452990]
- 152.
- Nonogaki K, Abdallah L, Goulding EH, Bonasera SJ, Tecott LH. Hyper-activity and reduced energy cost of physical activity in serotonin 5-HT(2C) receptor mutant mice. Diabetes. 2003;52(2):315–320. [PubMed: 12540602]
- 153.
- Bardo MT, Donohew RL, Harrington NG. Psychobiology of novelty seeking and drug seeking behavior. Behav Brain Res. 1996;77:1–2. 23–43. [PubMed: 8762157]
- 154.
- Bowirrat A, Oscar-Berman M. Relationship between dopaminergic neurotransmission, alcoholism, and reward deficiency syndrome. Am J Med Genet B Neuropsychiatr Genet. 2005;132(1):29–37. [PubMed: 15457501]
- 155.
- Piazza PV, Le Moal M. The role of stress in drug self-administration. Trends Pharmacol Sci. 1998;19(2):67–74. [PubMed: 9550944]
- 156.
- Rothman RB, Baumann MH. Therapeutic and adverse actions of serotonin transporter substrates. Pharmacol Ther. 2002;95(1):73–88. [PubMed: 12163129]
- 157.
- Colado MI, O’Shea E, Green AR. Acute and long-term effects of MDMA on cerebral dopamine biochemistry and function. Psychopharmacology (Berl). 2004;173(3-4):249–263. [PubMed: 15083264]
- 158.
- Ritz MC, Cone EJ, Kuhar MJ. Cocaine inhibition of ligand binding at dopamine, norepinephrine and serotonin transporters: a structure-activity study. Life Sci. 1990;46(9):635–645. [PubMed: 2308472]
- 159.
- Geyer MA, Callaway CW. Behavioral pharmacology of ring-substituted amphetamine analogs. In: Cho AR, Segal OS, editors. Amphetamine and Its Analogs. Academic Press; New York: 1994. pp. 177–201.
- 160.
- McNamara MG, Kelly JP, Leonard BE. Some behavioural and neurochemical aspects of subacute (+/−)3,4-methylenedioxymethamphetamine administration in rats. Pharmacol Biochem Behav. 1995;52(3):479–484. [PubMed: 8545462]
- 161.
- Ball KT, Budreau D, Rebec GV. Context-dependent behavioural and neuronal sensitization in striatum to MDMA (ecstasy) administration in rats. Eur J Neurosci. 2006;24(1):217–228. [PubMed: 16882018]
- 162.
- Adell A, Celada P, Artigas F. The role of 5-HT1B receptors in the regulation of serotonin cell firing and release in the rat brain. J Neurochem. 2001;79(1):172–182. [PubMed: 11595769]
- 163.
- Hamon M. The main features of central 5-HT1A receptors. Serotoninergic Neurons and 5-HT Receptors in the CNS. In: Baumgarten HG, Göthert M, editors. Handbook of Experimental Pharmacology. Vol. 129. 1997. pp. 239–268.
Footnotes
- *
Psynogeny® groups neurobiology, psychology, and psychiatry (V. Compan, 2003).
- Abstract
- INTRODUCTION
- HUMAN 5-HT RECEPTOR GENE POLYMORPHISMS AND ADDICTIONS TO FOOD AND ILLEGAL DRUGS
- KNOCK-OUT OF 5-HT RECEPTORS CLASSICALLY REQUIRED ENVIRONMENTAL CHALLENGES TO PRODUCE MALADAPTIVE BEHAVIOR
- 5-HT RECEPTOR KNOCK-OUT MICE AND ADAPTIVE CHANGES IN CENTRAL SEROTONINERGIC SYSTEMS
- CONCLUSION
- Acknowledgments
- REFERENCES
- Review Safety and nutritional assessment of GM plants and derived food and feed: the role of animal feeding trials.[Food Chem Toxicol. 2008]Review Safety and nutritional assessment of GM plants and derived food and feed: the role of animal feeding trials.EFSA GMO Panel Working Group on Animal Feeding Trials. Food Chem Toxicol. 2008 Mar; 46 Suppl 1:S2-70. Epub 2008 Feb 13.
- Review Prenatal cocaine produces biochemical and functional changes in brain serotonin systems in rat progeny.[NIDA Res Monogr. 1995]Review Prenatal cocaine produces biochemical and functional changes in brain serotonin systems in rat progeny.Battaglia G, Cabrera TM, Van de Kar LD. NIDA Res Monogr. 1995; 158:115-48.
- Receptor-genes cross-talk: effect of chronic 5-HT(1A) agonist 8-hydroxy-2-(di-n-propylamino) tetralin treatment on the expression of key genes in brain serotonin system and on behavior.[Neuroscience. 2010]Receptor-genes cross-talk: effect of chronic 5-HT(1A) agonist 8-hydroxy-2-(di-n-propylamino) tetralin treatment on the expression of key genes in brain serotonin system and on behavior.Popova NK, Naumenko VS, Cybko AS, Bazovkina DV. Neuroscience. 2010 Aug 11; 169(1):229-35. Epub 2010 Apr 25.
- Adaptive changes in serotonin metabolism preserve normal behavior in mice with reduced TPH2 activity.[Neuropharmacology. 2014]Adaptive changes in serotonin metabolism preserve normal behavior in mice with reduced TPH2 activity.Mosienko V, Matthes S, Hirth N, Beis D, Flinders M, Bader M, Hansson AC, Alenina N. Neuropharmacology. 2014 Oct; 85:73-80. Epub 2014 May 24.
- Review [Posttraumatic stress disorder (PTSD) as a consequence of the interaction between an individual genetic susceptibility, a traumatogenic event and a social context].[Encephale. 2012]Review [Posttraumatic stress disorder (PTSD) as a consequence of the interaction between an individual genetic susceptibility, a traumatogenic event and a social context].Auxéméry Y. Encephale. 2012 Oct; 38(5):373-80. Epub 2012 Jan 24.
- Do Limits of Neuronal Plasticity Represent an Opportunity for Mental Diseases, S...Do Limits of Neuronal Plasticity Represent an Opportunity for Mental Diseases, Such as Addiction to Food and Illegal Drugs? Use and Utilities of Serotonin Receptor Knock-Out Mice - Serotonin Receptors in Neurobiology
Your browsing activity is empty.
Activity recording is turned off.
See more...