NCBI Bookshelf. A service of the National Library of Medicine, National Institutes of Health.
Mucignat-Caretta C, editor. Neurobiology of Chemical Communication. Boca Raton (FL): CRC Press/Taylor & Francis; 2014.
3.1. INTRODUCTION
The inhabitants of cities and large towns are largely unaware of the constant battle that rural communities have to wage against flies. In rural areas, on the other hand, inhabitants do not always appreciate the crucial role that dung beetles play in controlling dung-breeding fly populations. Not only do dung beetles play an important role in the destruction of the habitat of many dung-breeding flies (Heinrich and Bartholomew 1979), but by burying and dispersing dung, the coprophagous fauna associated with mammals is also responsible for returning a large proportion of plant nutrients to the soil (e.g., Bornemissza and Williams 1970).
Serious problems have been experienced in the cattle farming areas of Australia where cattle were introduced without the associated insect fauna. The dung pats left by cattle become the habitat of dung-breeding flies, some of which are blood-feeding pests and carriers of serious diseases (Hughes 1970). This resulted in the uncontrolled increase of fly populations and the deterioration of pastures in parts of that continent. It has been estimated that cow pats are responsible for the reduction of pasture, albeit only temporarily, by about 20% per animal per year (Waterhouse 1974). During the 1950s, George Bornemissza hypothesized that the introduction of foreign dung beetle species that are able to remove and bury cattle dung would aid not only Australia’s soil fertility by recycling the dung nutrients back into the ground, but would also reduce the number of pestilent flies and parasitic worms that use the dung pats as a breeding resource. In 1965, this idea culminated in the establishment of the Australian Dung Beetle Project, and eventually 43 dung beetle species were imported from Africa and other continents in order to combat the fly problem (Wikipedia August 2012). The introduction of exotic dung beetles and their subsequent establishment in Australia was a highly successful venture and largely solved the fly problem in many parts of the continent.
The emergence of problems similar to those in Australia is now also being observed in other countries. The destruction of the habitat of large mammals by urbanization and modern farming practices invariably results in dwindling numbers of dung beetles and an increase in numbers of flies during the warm summer months. The importance of this process is vividly illustrated in rural areas in Africa where visitors to game reserves with normal herbivore populations are rarely bothered by flies during the summer when dung beetle activity reaches its peak, whereas in small settlements a few kilometers outside these reserves the indigenous people are plagued by swarms of flies.
Extensive research has been devoted to the ecology, ethology, and evolution of dung beetles, notably by Bornemissza, Halffter, and their coworkers. Recently, Simmons and Ridsdill-Smith (2011) edited a wide-ranging review of the literature on the ecology and evolution of dung beetles. Although it is estimated that there are more than 4000 dung beetle species in Africa alone, the olfactory ecology of dung beetles remains a largely unexplored yet potentially very fertile research field. Little research has been carried out on the chemical aspects of the ecology of dung beetles, and practically no information was available on the existence, the modes of operation, and the chemical structures of sex attractants of dung beetles before the work discussed in this chapter commenced in 1978.
On the basis of their nesting behavior, dung beetles of the subfamily Scarabaeinae can be divided into three groups: The paracoprids construct their nests under a dung pat by excavating tunnels in which the dung is packed; the endocoprids excavate a chamber in the dung pat itself, forming brood balls within this chamber; and the telecoprids detach a portion of dung from the pat, rolling it some distance from the dung source before burying it. The majority of dung beetles species in southern Africa are mainly crepuscular paracoprids (87%) (Halffter and Matthews 1966). The diurnal telecoprids are also numerous, but are represented by fewer species (12%) (Ferreira 1972).
Larvae and adults of the majority of species of the subfamily Scarabaeinae are coprophagous, and are morphologically adapted to feed on vertebrate excrement (Halffter and Matthews 1966). Adult dung beetles ingest only the liquid or colloidal constituents of the dung by squeezing portions of the moist dung between highly specialized membranous mandibles and ingesting the expressed juice. There are differences in the courtship, mating, and brooding behavior of the Scarabaeinae species. Telecoprids prepare a brood ball from fresh dung, roll the ball to an apparently carefully selected spot at a distance from the dung pat or dung midden, where the female deposits an egg in a chamber constructed at the top of the dung ball. The larva feeds on whole dung particles with the aid of its chewing mouthparts until eventually it pupates (Waterhouse 1974). The next generation, as well as the adult beetles that have overwintered in the soil, emerge from the soil after the first summer rains when temperatures are high enough for habitation and when the soil is moist enough for the beetles to break free from the brood chamber or from the hard soil.
Dung beetles are attracted to dung on which they feed, and after a feeding period of a few weeks the beetles that have overwintered in the soil and those that breed in their first season are ready to start breeding. Dung beetles utilize volatile compounds emitted by dung to locate a fresh source of a species’ preferred dung type, although dung beetles are apparently not linked exclusively to one type of dung (Dormont et al. 2004, 2007).
In areas with intact ecology, dung beetles of many species arrive in the thousands at a dung pat or rhinoceros midden. There is fierce competition for fresh dung between the beetles. Even in the case of the large volumes of dung voided by, for example, rhinoceros, dung beetles manage to dehydrate the dung or to bury or disperse it within a few hours. A photograph of rhinoceros dung voided during the night in the Mkuzi Game Reserve, South Africa, is shown in Figure 3.1a. At about 10:00 the following morning during a sampling period of 20 minutes, 720 dung beetles were recorded arriving at this resource. The entire dung heap appeared to be in constant movement from the activity of thousands of beetles, resulting in the formation of an almost uniform mixture of dung and dung beetles. By that stage, it was already too late for the larger species to gather enough of the already half-dried-out dung for the formation of a brood ball. In the foreground in Figure 3.1a, the last beetles that had managed to form a dung balls can be seen rolling them away. By 15:00 in the afternoon most of the dung was buried underneath the dung or next to it, or rolled away, and only relatively dry plant material was left at this spot.
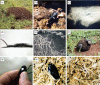
FIGURE 3.1
(a) Dung beetles on and in rhinoceros dung that has been voided during the night. (Photograph courtesy of B. V. Burger.) (b) A K. lamarcki male that has lost his left hind leg in the typical secretion-producing headstand position. (Photograph courtesy (more...)
3.2. CHEMICAL ECOLOGY OF THE GENUS KHEPER
The ecology and ethology of some of the ball-rolling dung beetles of southern Africa were investigated in great detail by Tribe (1976). His observation that the male dung beetles of the genus Kheper produce a visible sex-attracting abdominal secretion created a unique opportunity to investigate an example of the secretion of a pheromone in nonvolatile carrier material. Visible secretions are produced by several insects, for example, by the butterfly larvae Epipyrops anomala (Marshall 1974), Papilio demodocus (Burger et al. 1978), and Cryptoglossa verrucosa (Hadley 1979), and the azalea lace bug, Stehanitis pyrioides (Nair and Braman 2012). However, these secretions are quite different in terms of function from those of dung beetles of the genus Kheper.
The genus Kheper is confined mainly to a belt stretching from the northern part of the Eastern Cape Province of South Africa, through KwaZulu-Natal, Botswana, Zimbabwe, northern Namibia, and into southern Angola. Kheper bonelii is an exception to this distribution; it occurs as an isolated population in the light sandy soil along the coast of the Western Cape Province. Only four of the six Kheper species are found in South Africa (Tribe 1976).
Tribe (1976) describes how, after arriving at a dung pat, the male Kheper lamarcki M’Leay carves out and then molds a dung ball or assumes the highest position on the ball or the dung pat. Supporting his body on the front two pairs of legs, the male raises his abdomen and lowers his head so that his body is at a relatively steep angle of about 45° to the dung (Figure 3.1b). A white flocculent substance resembling fine cotton wool (Figure 3.1c) is extruded from a sieve consisting of several hundred tiny openings in a depression on either side of the first abdominal sternite. The secretion is supplied by a large gland complex immediately underlying the depression in which the sieve is located. The hind legs are retracted simultaneously inward towards the sides of the body, and are then simultaneously rapidly extended. This movement results in the hardened secretion being broken up into tiny particles between brushes on the tibiae (Figure 3.1d) and comblike structures on the abdomen of the male (Figure 3.1e). Small puffs of white powder are dispersed into the air above the abdomen. After a short interval of 20–30 seconds, the legs are again withdrawn inward against the sides of the body, and again extended rapidly. The brushes on the tibiae are usually rudimentary in the females. As will be discussed below, these particles contain a complex mixture of more than 150 organic compounds, of which only a limited number are common to all Kheper species. This behavior continues until a female arrives, by which time both the hind legs and sides of the abdomen have become coated in the white powder.
According to Tribe (1976), normally, the male relinquishes his headstand position as soon as another beetle of the same species appears within range and then challenges the intruder with his forelegs raised. If the intruder is a male, he will respond in a corresponding way by raising his forelegs to meet the challenge. A fight then ensues, but invariably the beetle that retains the highest position on the pat, usually the one releasing the pheromone, is able to forcefully flip the other beetle off the pat with his forelegs and clypeus. An attracted female is challenged by the male; when her presence is detected the male raises his forelegs and advances on the female. Her reaction is a submissive lowering of the forelegs, followed by antennal contact and acceptance by the male. The male then approaches her, and after antennal contact, possibly involving the detection of a pheromone, the female is allowed to cling to the brood ball that the male then rolls rapidly away from the dung pat. If the male has not already made a dung ball before the female arrived, he then detaches a portion of dung from the pat with or without assistance by the female. The dung fragment is rounded by the female and the male proceeds to roll both the ball, and the female clinging to it, away from the dung pat until a suitable site has been found to bury the ball (Figure 3.1f). While the female clings to the dung ball the male removes the soil from below the ball, which results in the ball sinking gradually deeper into the soil. Once the ball is buried, copulation occurs below the soil. The male returns to the surface after four days while the female remains below to lay an egg in the ball and to brood the developing larva.
Although it has not been observed that male K. subaeneus produce a secretion from the top of a dung ball, this species follows basically the same breeding routine. On arrival at a fresh dung source, the male generally vanishes into the dung, only to appear again after a while and start producing the abdominal secretion. After the male has accepted an arriving female or a female has accepted the male, as the case may be, the pair normally disappears into the dung and they mold a dung ball below the surface of the dung. Eventually the dung is broken free from the surrounding dung and is rolled away from the dung pat or midden. Such a smooth and uninterrupted procedure is only possible when the male arrives at the dung pat before a situation such as depicted in Figure 3.1a has developed, with thousands of dung beetles arriving at the dung pat, or when another male attracted by the abdominal secretion of the first male has not taken over the ball-molding process.
In contrast, K. nigroaeneus males first bury a brood ball before assuming the typical secretion-producing stance at the entrance to the burrow in which the ball has been buried. This also happens even when a male is not in possession of a dung ball (Edwards and Aschenborn 1988).
3.3. COLLECTION OF THE ABDOMINAL SECRETION
Permission was granted to us annually by the KwaZulu-Natal Parks Board for the collection of 250 male and 125 female Kheper dung beetles of each of the three species that are found in northern KwaZulu-Natal. Only a few rhinoceros dung middens were easily and safely accessible from the dirt roads in the Mkuzi Game Reserve and these species were therefore mostly trapped in 10 pitfall traps (Tribe 1976) spaced at 100-m intervals along the so-called Beacon Road and baited with fresh horse dung during the first week of November each year. Only 17 male K. lamarcki were collected for research during the first season of the project. Although these dung beetles can easily fly at speeds exceeding 30 km/h and can therefore cover 100 m in a few seconds, traps at specific positions were always less productive than others along that road. Increasingly, fewer dung beetles were trapped in traps positioned towards the southernmost part of this stretch of road where there were often only a few dung beetles per trap, whereas traps along the northern part of the road were often filled to capacity with dung beetles of many different species within a few hours. All collections were carried out in the morning between about 09:00 and 12:00. There was little large herbivore activity in this part of the reserve and little variation in the nature and density of the vegetation along this road. The difference in trap yields could therefore not be ascribed to the competition between the relatively small volumes of horse dung with which the traps were baited and the availability of large quantities of dung in the vicinity of the less productive traps. The largest numbers of dung beetles were always trapped in spots where the soil was somewhat sandier. Apparently dung beetles prefer, and have the ability to detect or otherwise select, places where it would be easier to bury the dung balls.
K. subaeneus is not found in Mkuzi Game Reserve; beetles of this species were collected in the Hluhluwe Game Reserve, which is situated about 50 km south of the Mkuzi Game Reserve. Here, practically no dung beetles of any species were caught in pitfall traps during the early years of the research project, possibly because the dung beetles were drawn to the large numbers of nearby dung middens in the accessible parts of the reserve. It is also possible that here the dung beetle population was depleted in accessible areas because large numbers of dung beetles were removed from those areas in the reserve for the Australian Dung Beetle Project. Fortunately, a few K. subaeneus could be collected from the scores of rhinoceros dung middens on the roads of the reserve. In later years, it was often possible to collect the allotted number of dung beetles during a single 3-km drive from the Monumental Entrance Gate of the reserve (coordinates 28:04:12.26S, 32:08:25:88E).
The collected beetles were transported in aluminum boxes containing moist soil by car from the game reserves and by air as hand luggage in the passenger cabin of a commercial airline from Durban to Cape Town. Once in Stellenbosch, the male and female beetles were kept together at subtropical temperatures in greenhouses (4 m × 3 m), the floors of which were covered with about 150 mm of moist sandy soil. Early on week days, the beetles were supplied with fresh horse dung. Within a few hours the dung beetles managed to spread out the dung in the greenhouses and after a week or two the soil was totally covered by a thick homogeneous layer of dry fibrous material. As soon as the first signs of any fungal growth appeared, the dried out material was removed and replaced with some fresh dung.
In an exploratory attempt at isolating and identifying the constituents of the abdominal secretion of male Kheper beetles, the abdominal glands of a male K. lamarcki were extracted with dichloromethane and the extracts concentrated for gas chromatographic-mass spectrometric (GC-MS) analysis. Such an isolation of trace quantities of a semiochemical mixture from a relatively large volume of glandular tissue is a time-consuming and often futile undertaking. In contrast, the chemical characterization of semiochemicals secreted in a carrier material or a matrix could be a quite simple exercise. In ideal cases it might even be possible to enrich volatile semiochemicals on sorptive traps during production of the secretion by the organism under investigation. Tribe (1976) has found that loss of a hind leg did not deter the male insect from producing secretion. If one of the hind legs of a calling beetle is amputated, the beetle normally disappears underground for a while, after which it reemerges and resumes its secretion-producing stance. The secretion that accumulates as a band on the incapacitated side of the beetle (Figure 3.1b) can then be collected with ophthalmic forceps.
The removal of a hind leg is the only possible way to prevent the dispersal of the secretion, which, if it is not brushed off, collects at that side of the abdomen as a tuft of cotton-wool-like material (Figure 3.1c). This behavior was also observed in captive Kheper males, but they generally did not reappear the same day, and very little secretion was eventually collected using this approach. Therefore, in the following and subsequent seasons, one hind leg was removed from all the males on arrival in Stellenbosch. About 5% died within the first week, but thereafter the surviving beetles appeared to behave normally and quickly learned how to roll dung balls with only one hind leg. In captivity, the production of the sex attractant was sporadic, and contrary to observations in nature, captive beetles sometimes produced secretion even when not in possession of fresh dung. They therefore had to be kept under constant observation. According to Tribe (1976), the beetles are at their most productive at temperatures around 29°C. In captivity, the beetles frequently went through the initial stages of the production of secretion, but they mostly stopped before a visible quantity of material had accumulated. In rare cases, the production of secretion continued for periods of up to 2 hours, resulting in the accumulation of substantial quantities of material. When disturbed in any way, for example by another beetle of the same or another species, male dung beetles immediately stopped secreting the attractant to investigate, and they even disappeared underground, sometimes together with a female. Males observed to assume the attractant-secreting posture were therefore fenced off from other beetles with aluminum strips. If after having secreted some material a beetle lowered its abdomen and prepared to go underground, it was taken firmly between the fingers (Figure 3.1g), and the secretion transferred to a vial with forceps or a spatula. Preferably, however, the secretion was removed periodically from the incapacitated side of the beetle with ophthalmic forceps to prevent losing the material or contaminating it with dust particles, and to avoid evaporation of the volatiles from the carrier material. Meanwhile, the vial with collected material was kept cool in an ice box.
In captivity, the secretion-producing behavior of the Kheper males differed from their behavior in the field. In the Hluhluwe Game Reserve, several K. subaeneus males were often observed simultaneously producing the secretion on rhinoceros dung middens. In captivity, the males only occasionally exhibited calling behavior. Males of this species produce their secretion as a relatively thick band about 5 mm wide. However, over a period of 5 years, only a few milligrams of the secretion was collected from about 700 males. It was also remarkable that in the wild, large numbers of K. subaeneus were often found in or on certain dung middens, while less than 5 m away not a single K. subaeneus could be found in or on a dung midden that contained equally fresh dung. As in many other insect species, the male-produced sex attractant of K. subaeneus apparently also acts as an efficient aggregation pheromone.
As has been mentioned, in nature male K. nigroaeneus first bury a brood ball before assuming the typical secretion-producing stance at the entrance to the burrow in which the ball has been buried (Edwards and Aschenborn 1988). The same behavior was observed in the captive insects. The male beetles never called from atop dung balls or when they were merely in possession of fresh dung. In captivity, often 10 or more beetles simultaneously exhibited calling behavior in one of the greenhouses. Unfortunately, the secretion of this species is extruded in an almost transparently thin and very narrow band, less than 1 mm wide, so that very little material accumulated even when a male continued producing the secretion for 30 min or longer.
K. lamarcki males produced secretion in bands of approximately the same width and thickness as K. subaeneus. In the wild and in captivity they produced secretion less frequently than K. nigroaeneus, but in captivity more frequently than K. subaeneus. The three Kheper species that were kept in greenhouses adapted quite readily to captivity. After initially trying to escape (for a few days), only to repeatedly hit the roof or sides of the greenhouse, they soon gave up trying to fly away. Surprisingly, the beetles that survived their first year in captivity appeared to have permanently lost their urge or ability to fly the next season.
Adult K. bonellii dung beetles were collected from cow dung pats in the vicinity of Elandsbaai on the southwest Cape coast during September each year, transported to Stellenbosch, and kept in greenhouses on horse dung. In captivity, male K. bonellii hardly ever produced secretion, and therefore the constituents of the sex attractant had to be extracted from the glandular material of the males.
3.4. SAMPLE PREPARATION AND ANALYSIS
Although Tribe (1976) describes the abdominal secretion as a “paraffin carrier” (i.e., a hydrocarbon wax), it does not dissolve in any of the common organic solvents. After preliminary small-scale experiments established that a complex mixture of volatile organic compounds (VOCs) could be extracted from the secretion with different solvents, such as pentane, dichloromethane and acetone, the following procedure was followed to isolate the volatile organic fraction of the secretion for GC-MS analysis and to purify the insoluble carrier material for further investigation.
The collected secretion was examined under a microscope for the presence of dust and dung particles that could have been picked up from the abdomen by the emerging secretion. Without spending too much time on the process and risking the loss of any highly volatile components present in the secretion, any visible impurities were carefully removed with ophthalmic forceps. The collected secretion (e.g., 450 µg collected from four K. lamarcki males) was stirred in a 1-ml Reacti-Vial with 100 µl of pentane using a winged magnetic follower to yield a suspension of the white insoluble carrier material in the solvent. To precipitate the carrier material together with any other insoluble matter, the suspension was centrifuged in the Reacti-Vial at 3000 rpm for 30 minutes. Without disturbing the precipitated carrier material, the supernatant pentane solution was carefully removed with a syringe. This extraction was repeated twice. Combination of the pentane extracts followed by slow evaporation of the solvent yielded a concentrate of the soluble VOCs for qualitative and quantitative GC-MS analyses. If only the VOCs had to be analyzed, the impurities visible under a microscope were removed as described, and the soluble organic material simply extracted with the more polar solvent dichloromethane containing just enough pentane to ensure precipitation of the carrier under centrifugation.
To purify the carrier material for further analysis, the differences in density of mixtures of pentane and dichloromethane were employed to separate the abdominal secretion from lighter dung fiber and from heavier sand and dust particles. The secretion was suspended in a mixture containing a relatively high percentage of pentane in which the dung particles remained suspended, while the carrier material and sand particles were precipitated by centrifugation at 3000 rpm. The solvent with the suspended impurities was removed with a syringe or pipette. The process was then repeated with the precipitated material using pentane/dichloromethane mixtures with increasing densities until finally the carrier material remained in suspension and the solvent plus carrier material could be removed with a pipette from the heavier dust particles. The carrier material was precipitated from the suspension by dilution with pentane and centrifuging at 3000 rpm. After removal of the solvent with a syringe and drying of the residue under reduced pressure at room temperature, the highly purified carrier material (400 µg, approximately 90% of the collected secretion) was obtained for further analysis.
As mentioned above, adult male K. bonellii in captivity hardly ever produced secretion. The VOCs were therefore extracted from the excised secretion-producing glands. The glands underlying the abdominal pores on both sides of the first abdominal sternite were carefully removed after the gut of the insect had been removed, the glandular tissue was extracted with dichloromethane of the best purity available (residue analysis grade or pesticide analysis grade) and the extract stored at –20°C until used for analysis.
The first four constituents isolated from the volatile organic fraction of the male abdominal secretion of K. lamarcki, hexadecanoic acid, 2,6-dimethyl-5-heptenoic acid, (E)-nerolidol and 3-methylindole (skatole), were relatively easily identified. However, exploratory GC and GC-MS analyses revealed that the secretions of K. lamarcki, K. nigroaeneus, and K. subaeneus are complex mixtures of at least 150 constituents (Figure 3.2); secretions contained hydrocarbons, alcohols, straight- and branched-chain fatty acids, and methyl and ethyl esters of these and other fatty acids with various carbon chain lengths.
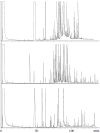
FIGURE 3.2
Gas chromatograms of extracts of the male abdominal secretions of K. lamarcki (top), K. nigroaeneus (middle), and K. subaeneus (bottom). (From Munro, Z. M. 1988. Semiochemiese kommunikasie: Ontwikkeling en toepassing van analitiese tegnieke vir die bepaling (more...)
As shown in Table 3.1, the volatile compounds present in the secretions of these three species are similar, but only some of them are common to two or to all three of the species. Furthermore, these compounds include several chiral long-chain compounds, the structures of which cannot be readily determined even with modern technology.
TABLE 3.1
Some of the Constituents Identified in Extracts of the Male Abdominal Secretions of Dung Beetles of the Genus Kheper
The envisaged complete chemical characterization of all the volatile constituents of the secretions was therefore suspended in favor of the identification of the constituents that could be detected by electroantennographic detection (EAD). However, the development of instrumentation for GC with flame ionization (FID) and electroantennographic detection in parallel (GC-FID/EAD) using the lamellate, clublike antennae of dung beetles proved very difficult due to the exceedingly poor signal-to-noise ratios of the EAD traces that were obtained. The excised antennae of these beetles that were often used in experiments for more than 12 hours appeared to be much more sensitive to interferences such as air turbulence, heat radiation, and fluctuations in air temperature and humidity than those of the Lepidoptera. It was hypothesized that, in addition to the detection of semiochemicals, dung beetle antennae are probably also used for navigation, the determination of air speed, meteorological conditions, and the detection of moisture. The influence of these parameters on EAD recordings was not investigated in any detail, but could be an interesting topic for future research by scientists interested in dung beetle ethology.
3.5. OPTIMIZATION OF GC-FID/EAD INSTRUMENTATION
In addition to the conventional instrumentation and operating parameters normally employed for GC-FID/EAD analyses, extraordinary precautions have to be considered to achieve acceptable results when dung beetle antennae are used as sensing elements.
- 1. Quality, selectivity, and capacity of the GC column. Overloading an apolar GC column with a polar compound, such as a carboxylic acid, results in the polar analyte eluting as a so-called fronting peak, which can be seen as a reversed tailing peak. In EAD analysis, fronting is probably a more serious problem than tailing because the sensilla on the antenna could become saturated by a fronting-EAD active compound before the detection threshold of the system is reached. The analytes must reach the preparation (antenna) as sharp peaks or pulses—the sharper the pulses, the better the resulting signal-to-noise ratios. Highly volatile apolar compounds are therefore mostly eluted from apolar columns as very sharp peaks with high signal-to-noise ratios. Carboxylic acids are eluted with satisfactory peak shapes from columns coated with so-called free fatty acid phase (FFAP) or an equivalent phase. If a sample contains both apolar and polar compounds with medium to long carbon chains then a column coated with the intermediate polar phase OV-1701 would be a good choice. In general, columns with thin stationary film coatings produce sharper peaks, but such columns are easily overloaded and produce broad and/or fronting peaks. Thus, if a semiochemical secretion contains analytes with widely different polarities then a column with a somewhat higher film thickness could be considered. Preferably, capillary columns coated with stationary phases of different polarities should first be tested for the best results. Sharper peaks can also be produced by employing higher temperature programming rates, provided the column’s separation efficiency is not compromised.
- 2. Elimination of the condensation of analytes in the column tip. An analyte that condenses in the tip of the column at the point where the hot effluent is introduced into the cool humidified stream of air will slowly evaporate from that point, resulting in the analyte reaching the preparation as a broad band instead of a sharply eluted peak or pulse. Condensation of the analytes, especially high-boiling compounds, in the tip of the capillary column is avoided by heating the column right up to its very tip. This can best be achieved by routing the column through a heated interface consisting of an aluminum block with a sharp conical tip barely touching the glass tube conducting the humidified air to the antenna (Figure 3.3) (Burger and Petersen 1991). The tip of the column should not extend beyond the tip of the interface. In this way analyte pulses can be delivered into the air duct with a minimum of heat being conducted to the glass tube and without condensation in the tip of the capillary column. Even better results can be obtained by introducing the effluent into the humidified air duct via a retention gap connected to the column tip with a press-fit connector (Rohwer et al. 1986). A retention gap, developed by Grob and Müller (1982), consists of a length of uncoated but properly deactivated fused silica capillary that has zero retention for the analytes eluting from the column at the temperature of the interface.
- 3. Elimination of condensation of high-boiling analytes in the humidified air duct. The column effluent must be introduced into the humidified air duct at a point not too far removed from the antenna in order to avoid condensation of the heavier analytes on the inside surface of the humidified air duct, but sufficiently far enough upstream to ensure proper mixing with the air. The contamination of the air duct can be elegantly eliminated by making provision for replacing a section of glass tubing with a clean section from just after the point of effluent introduction to just before the antenna. In long analyses of complex mixtures, glass sections could be exchanged several times at appropriate intervals during the experiment. To facilitate the exchange, sections of glass tubing have to be cut with a glass-cutting circular saw to exactly the same length. This part of the air duct has to be supported in a V-shaped gutter to facilitate alignment when replacing a section. For easy manipulation, each of the sections has to be fitted with a small handle. Such a setup can also be conveniently converted into an arrangement for electroantennographic (EAG) experiments by using a T-section with a B-10 ground-glass side socket for the connection of a tube containing a test sample on filter paper (Figure 3.3).
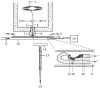
FIGURE 3.3
Schematic view of a gas chromatograph fitted with a column effluent splitter for flame ionization detection (FID) and electroantennographic detection (EAD) in parallel: 1, capillary column; 2, makeup gas at 15 ml/min; 3, FID; 4, fused silica capillary; (more...)
- 4. Elimination of turbulence in the humidified air flowing over the antenna. Turbulence in the humidified air duct just before it reaches the preparation and turbulent mixing of the humidified air with drier, and probably warmer, laboratory air on the surface of the antenna are probably the major causes of an unstable EAD signal and low signal-to-noise ratios. To avoid turbulence in the humidified airstream, there should not be any sharp bends in the glass tube immediately upstream from the preparation. Although it is impossible to totally eliminate turbulence, especially on the surface of the antenna, the best solution is to prepare the antenna in such a manner that it can be inserted “lengthwise” into the air duct by using electrodes that have been bent in an appropriate fashion. Dung beetles are too strong to be tethered and antennae have to be excised for EAD experiments. Fortunately, the insects’ antennae can be used for periods exceeding 12 hours. The antenna can be fastened to one of the electrodes and the two electrodes can be tied together before the preparation is inserted into the air duct (Figure 3.3).
- 5. Elimination of condensed water droplets in the humidified air duct or on the surface of the antenna. The effective lifetime of an antenna in FID/EAD analyses can be extended by cooling the humidified air to a slightly lower than ambient temperature. This is, however, not necessary in analyses with dung beetle antennae. It is also risky because it could lead to condensation of water droplets in the air duct and/or on the surface of the antenna.
- 6. Elimination of pressure pulses in the humidified airsteam. Humidifying the air by using a wide-bore tube to bubble the air through water could add to the turbulence in the air flowing over the preparation. A proper gas-washing bottle with an inverted sintered glass frit should be used instead.
- 7. Elimination of electrostatic interference. It is standard practice to operate the EAD setup in an earthed Faraday cage, which should preferably not be earthed to an outlet of the building’s electrical supply. The cage should be connected with a thick insulated copper cable to a copper mesh mat buried outside the laboratory building.
- 8. Elimination of heat radiation. Finally, a further incremental improvement could possibly be achieved by shielding the preparation and the humidified air duct against heat radiation from the hot interface or other hot parts of the gas chromatograph by installing a water-cooled heat shield as shown in Figure 3.3.
- 9. Recording the FID and EAD chromatograms. Recording the chromatograms on a dual-channel integrator or on a computer using appropriate commercially available software allows for time scale manipulation in order to ensure as far as possible that FID and EAD responses that appear to be coinciding are indeed resulting from the same analyte. If two strip chart recorders are used, they should be accurately matched (synchronized). A relatively high chart speed and constant monitoring of any fine structure in the responses are also essential in order to distinguish between background noise and authentic FID and AEG responses.
3.6. CHEMICAL CHARACTERIZATION OF ABDOMINAL SECRETIONS OF THE MALE KHEPER SPECIES
Ideally, the EAD-active constituents of the abdominal sex attracting secretions of the male Kheper beetles were identified by GC-MS analysis, enantioselective GC analysis, and retention time comparison with authentic synthetic analogs of the natural compounds.
3.6.1. K. lamarcki
Initially, only four of the VOCs of the male abdominal sex attractant secretion of K. lamarcki, hexadecanoic acid (57%), 2,6-dimethyl-5-heptenoic acid (33%), (E)-nerolidol (9%) and skatole (1%), were positively identified in the extract of the secretion (Burger et al. 1983). Synthetic analogs of these compounds were used to formulate an attractant for field tests. Due to the small quantities of these compounds isolated from the secretion, and because GC methods to determine the absolute configuration of chiral compounds had not yet been developed, racemic 2,6-dimethyl-5-heptenoic acid and (E)-nerolidol were used in the synthetic attractant. Purified French chalk was used as inert carrier material. Confusing results were obtained. Not a single dung beetle of any species was caught in pitfall traps baited with the attractant alone, whereas large numbers of dung beetles of a variety of species were trapped in control traps baited with fresh horse dung. Combining the synthetic attractant with horse dung even seemed to reduce the attractiveness of the dung for K. lamarcki as well as for the other dung beetle species.
Females of the genus Kheper produce only one egg per year or perhaps two during a long summer, and after mating they remain underground to care for their brood. Because the tests were carried out late in the active season of K. lamarcki, the majority of the females had probably already gone underground, and the fact that only male K. lamarcki were trapped is probably not significant. Raw data obtained in bioassays carried out earlier in the next season suggested that more females were attracted to pitfall traps baited with horse dung plus the synthetic attractant than to the traps baited with the dung alone. However, rigorous statistical evaluation of the results again showed that the apparent differences in the numbers of male and females were insignificant.
The different yields of the control traps baited with dung confirmed a problem that is probably inherent in field tests with dung beetles; namely, their territorial behavior appears to be also determined by cues other than sex attractants, such as the nature of the soil—the type, texture, and moisture content. To conclude, no evidence was found that the above four VOCs are indeed components of the purported sex attractant of K. lamarcki.
An example of a GC-FID/EAD analysis of the VOCs extracted from the male abdominal secretion of K. lamarcki that was typically obtained during the early years of this project is depicted in Figure 3.4. Obviously, this analysis did not produce any useful information. Despite the incremental improvement of the GC-EAG setup over time, later GC-FID/EAD analyses of extracts of the male abdominal secretion of K. lamarcki did not produce appreciably better results. It is possible that in the case of this species the signals generated by the antennae were simply too weak to be detected by the then-available instrumentation.
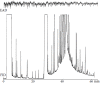
FIGURE 3.4
FID/EAD analysis of an extract of the male abdominal secretion of K. lamarcki using a female antenna as sensing element. (From Petersen, W. G. B. 1997. Semiochemiese kommunikasie by miskruiers van die genus Kheper (Scarabaeinae). PhD dissertation, University (more...)
The injection of an enormous sample of an extract of the male abdominal secretion of K. lamacki in a GC-FID/EAD analysis gave the set of chromatograms depicted in Figure 3.5. The width of the peaks of 2,6-dimethyl-5-heptenoic acid 1 and (E)-nerolidol 3 is an indication of the massive overloading of the column with respect to the major constituents of the secretion. Under these conditions the antenna responded only to the leading edge of the 2,6-dimethyl-5-heptenoic acid peak. The antenna also responded to the elution of skatole 2. Twelve constituents of the secretion eluting with retention times ranging from about 10 to 35 minutes gave weak EAD responses. Only five of these compounds were identified as ethyl butanoate, 2-hexanone, 3-hexen-2-one, 2-methylpropyl propanoate, and 3-methylbutyl propanoate. Although indications of EAD activity were found in the low retention time range of a few subsequent analyses, the responses were always quite weak and not reproducible. No further attention was given to these constituents and the possibility that they could be constituents of a male-secreted pheromone was not seriously considered. The significance of these almost forgotten results was only realized many years later in quite a different context, as will be shown in Section 3.9.1.
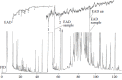
FIGURE 3.5
FID/EAD analysis of an extract of the male abdominal secretion of K. lamarcki using a male antenna as sensing element. Coinciding peaks and responses are indicated by black dots. (From Munro, Z. M. 1988. Semiochemiese kommunikasie: Ontwikkeling en toepassing (more...)
3.6.2. K. nigroaeneus
Better results were obtained in GC-FID/EAD analyses of the VOCs extracted from the abdominal secretion of K. nigroaeneus males (Burger and Petersen 2002). Figure 3.6 depicts a set of chromatograms obtained in a GC analysis of the extract with FID/EAD detection in parallel using a temperature programming rate of 4°C/min. Here a female antenna was used as an EAD sensing element. Male and female antennae mostly gave reproducible and qualitatively similar results, which is not unexpected for a sex attractant secreted by a male insect (Leal 1998).
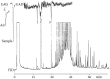
FIGURE 3.6
First part of a GC-FID/EAD analysis of an extract of the male abdominal secretion of K. nigroaeneus using a female antenna as a sensing element. Corresponding peaks in the FID and EAD traces are indicated by dots. The structures of the EAD active compounds (more...)
Compound 1 gave reproducible EAD responses, but despite the relatively large samples that were used, it was not visible in the corresponding FID gas chromatograms. The low concentration in which this compound is present in the secretion and the fact that it was impossible to collect unlimited quantities of abdominal secretion precluded its identification. However, in retrospect, it might have been possible to identify this compound by using the strategy that was used to identify an approximately equally low concentration of a constituent of the abdominal secretion of K. subaeneus (see below).
Compound 2 was identified as 3-methylheptanoic acid by GC-MS analysis in conjunction with 1H NMR analysis of a sample of the compound isolated by preparative GC. The two enantiomers of the synthetic acid are separable on a capillary column coated with a mixture of the stationary phase OV-1701-OH and heptakis(2,3,6-tri-O-methyl)-β-cyclodextrin; the S enantiomer eluting first from this column. Using this column, enantioselective GC analyses with FID and EAD detection in parallel confirmed the EAD activity of the second-eluting R enantiomer. (R)-(+)-3-Methylheptanoic acid was synthesized in high enantiomeric purity from (R)-(+)-3,7-dimethyl-6-octen-1-ol [(R)-(+)-β-citronellol] as starting material (Burger and Petersen 2002). The third EAD active compound, 3 in Figure 3.6, was identified as skatole, which was also identified as a constituent of the abdominal secretions of the other dung beetles of the genus Kheper that were investigated.
3.6.3. K. subaeneus
On a few occasions the male abdominal secretion of captive male K. subaeneus was collected in small quantities—a total of only a few milligrams over a period of 5 years from about 700 males. GC-FID-EAD analyses of extracts of the collected material gave diverse results. In some analyses several of the constituents of the extracts elicited EAD responses, but mostly different constituents produced EAD responses with varying intensities, as illustrated in Figures 3.7 and 3.8.
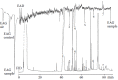
FIGURE 3.7
FID/EAD analysis of an extract of the male abdominal secretion of K. subaeneus using a male antenna as a sensing element. Coinciding peaks and responses are indicated by black dots. 7-Vinyldec-8-en-1-ol was used as control. The structures of the EAD active (more...)
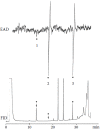
FIGURE 3.8
First part of an analysis of an extract of the male abdominal secretion of K. subaeneus with EAD and FID detection in parallel using a female antenna as a sensing element. The structures of the EAD-active compounds are given in the text. (From Petersen, (more...)
The major constituent of the secretion of this species, constituent 1 in the set of FID and EAD chromatograms depicted in Figure 3.7, elicited a strong EAD response in the male antenna that was used as sensing element. This compound was identified as 2,6-dimethyl-5-heptenoic acid, which had previously been identified in K. lamarcki (Burger et al. 1983). Racemic 2,6-dimethyl-5-heptenoic acid was resolved by GC on a stationary phase mixture of OV-1701-OH and heptakis(2,3,6-tri-O-methyl)-β-cyclodextrin, mentioned previously. The natural compound was identified as (S)-2,6-dimethyl-5-heptenoic acid, which coeluted with the natural compound from the enantioselective column. The synthetic R enantiomer did not elicit EAD responses from male or female antennae, but neither did the synthetic S enantiomer in a large number of male and female antennae that were used in FID/EAD measurements. In view of the observation that the natural compound also did not reproducibly elicit EAD responses, this was not a totally unexpected result.
Constituents 2 and 3 were tentatively identified as 4-dodeken-2-ol and 4-trideken-2-ol, respectively. Constituent 4 was identified as skatole. Constituent 5 remained unidentified and constituent 6 was tentatively characterized as a branched-chain methyl ester.
Two compounds elicited exceptionally strong responses in the first part of the GC-FID/EAD analysis shown in Figure 3.8 (Burger et al. 2002). A female antenna was used as sensing element in this analysis.
Constituent 1 in the set of FID and EAD chromatograms depicted in Figure 3.8 was identified as butanoic acid (n-butyric acid) based on its mass spectrum and coelution with an authentic synthetic analog of the natural compound. Although the EAD response was weak, it was detected in several of the GC-EAD traces. 2,6-Dimethyl-5-heptenoic acid, the major constituent of the secretion, eluted at about 22.2 minutes, but did not elicit an EAD response in this analysis. Constituent 3 was identified as skatole.
Although barely detectable in the FID gas chromatogram, constituent 2 gave a strong and reproducible EAD response in the same antenna and also in other K. subaeneus antennae used as sensing elements. About 10% of the material collected during the previous five summer seasons was used for GC-FID/EAD experiments. The remaining material was used in one final attempt at obtaining some mass spectral information on the structure of this constituent. The resulting mass spectrum (Figure 3.9) contained several ions typically found in the mass spectra of monoterpenoids. GC-MS retention time comparisons showed that constituent 2 could not be a cyclic, an acyclic monoterpene, or a primary monoterpene alcohol, but that it could possibly be a tertiary terpene alcohol other than linalool. Employing retention time data and mass spectral comparison of the unknown constituent with reference compounds synthesized in the laboratory, it was eventually identified as the new natural tertiary terpenoid alcohol (E)-2,6-dimethyl-6-octen-2-ol, for which the name (E)-subaeneol was proposed (Burger et al. 2002).
![FIGURE 3.9. Mass spectrum of the EAD active constituent [(E)-2,6-dimethyl-6-octen-2-ol] of the male abdominal secretion of Kheper subaeneus eluting at 17.](/books/NBK200987/bin/ch3_f09.gif)
FIGURE 3.9
Mass spectrum of the EAD active constituent [(E)-2,6-dimethyl-6-octen-2-ol] of the male abdominal secretion of Kheper subaeneus eluting at 17.75 minutes in the gas chromatogram shown in Figure 3.8. (From Petersen, W. G. B. 1997. Semiochemiese kommunikasie (more...)
3.6.4. K. bonellii
K. bonellii is the last of the four Kheper species of which the male abdominal secretions were investigated. This species is found in the coastal area of the Western Cape, about 200 km from Stellenbosch.
GC analyses of the extract of the abdominal glands of male K. bonellii with FID and EAD recording in parallel gave reproducible results; male and female antennae responded to the same constituents of the extract. An example of a typical analysis is given in Figure 3.10 (Burger et al. 2008). Constituent 1 was identified as 2-butanone, a solvent impurity. Compounds 2, 3, 5, and 6 were identified as propanoic acid, butanoic acid, indole, and skatole, respectively.
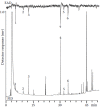
FIGURE 3.10
GC-FID/EAD analysis of an extract of the abdominal sex pheromone glands of the dung beetle K. bonellii using a male antenna as a sensing element. The peaks are numbered to indicate coinciding FID and EAD responses. (From Petersen, W. G. B. 1997. Semiochemiese (more...)
Although the presence of a reasonably abundant ion at m/z 74 in the mass spectrum of constituent 4 suggested the presence of a methyl ester moiety, the ions at m/z 87, 129, and 143 in the mass spectra of saturated methyl esters are present in uncharacteristically low abundances in this spectrum. Fortunately, extracts of the glandular abdominal tissue contained relatively high concentrations of this constituent. It was therefore possible to isolate sufficient material from about 200 beetles by preparative GC on an open-tubular wide bore capillary column for 1H and 13C NMR, APT, and COSY spectral analyses. This constituent was identified as methyl cis-2-(2-hexylcyclopropyl)acetate. Wilson and Prodan (1976) have identified the trans isomer of this ester in the essential oil of Croton eluteria (Euphorbiaceae), and proposed the names cis- and trans-cascarillate for the two isomers. The absolute configuration of the enantiomer present in the male abdominal secretion of K. bonellii was established by stereoselective synthesis, which afforded methyl (R,R)-2-(2-hexylcyclopropyl) acetate [methyl (R,R)-cascarillate] in an enantiomeric excess of 69%. Enantioselective GC-FID/EAD, using a capillary column coated with OV-1701-OH containing 10% heptakis(2,3-di-O-methyl-6-O-tert-butyldimethylsilyl)-β-cyclodextrin as chiral selector, showed that methyl (R,R)-cascarillate was the only enantiomer of racemic methyl cis-cascarillate that coeluted with the natural compound, and that it elicited EAD responses in the antennae of male and female K. bonellii (Figure 3.11) (Burger et al. 2008).

FIGURE 3.11
Enantioselective GC analysis. (a) Racemic methyl cis-cascarillate. (b) Synthetic racemic methyl cis-cascarillate spiked with the natural ester that was isolated by preparative GC from an extract of the male abdominal glands of K. bonellii. (From Petersen, (more...)
Far better GC-FID/EAD analysis results were obtained when the antennae of K. bonellii were used as sensing elements compared to analyses of the VOCs the abdominal secretions of the other Kheper species, probably because the antennae of K. bonellii could be used within a few hours after the insect had been captured. Analyses of the VOCs of the other species were invariably carried out late in the season and often using the antennae from insects that had spent several months in captivity under less than optimal climatic conditions.
The constituents identified in the male abdominal secretions of the four Kheper species are summarized in Table 3.2.
TABLE 3.2
EAD Active Compounds Identified in the Male Abdominal Secretions of Kheper Species
From the research carried out with dung beetles over many years it is clear that semiochemical communication in dung beetles is extraordinarily complex. As far as the inconsistency of antennal responses in EAD analyses with the antennae of this and other Kheper species is concerned, there are various factors that must be considered. It is possible, for example, that there could be some natural variation in the population in terms of antennal responses. Because the research described was carried out with captured insects, it was not possible to determine the age, physiological state, and so forth of the insects that were used in these experiments—factors that could have influenced the EAD results.
The possibility that the response of an antenna to the volatile constituents of the secretion could depend on the concentration of the components flowing over the antenna was investigated. However, results do not seem to explain the inconsistent results obtained with (S)-2,6-dimethyl-5-heptenoic acid and some of the other minor constituents of the secretion. In retrospect, and bearing in mind that this compound is a carboxylic acid, the inconsistent results could possibly have resulted from insufficient attention having been paid to one or more of the extraordinary precautions mentioned earlier that have to be taken to ensure successful GC-FID/EAD analyses.
3.7. LONG-CHAIN CONSTITUENTS OF THE ABDOMINAL SECRETIONS
As far as the reproducible EAD responses are concerned, qualitatively similar results were obtained in GC-FID/EAD analyses with male and female antennae as sensing elements. However, on several occasions, examples were encountered of male and female antennae responding reproducibly to different long-chain compounds on different occasions. However, these results could not be reproduced with other antennae and the identification of these compounds could thus not be verified by EAD analyses of the synthetic compounds. These compounds were always long-chain fatty acids such as hexadecanoic acid, or the methyl or ethyl esters of long-chain acids. It was thought that the large numbers of long-chain compounds present in the abdominal secretions of Kheper males could contribute to the controlled release of the sex attractants of these insects. Unfortunately, no concerted attempts have yet been undertaken to confirm or refute this hypothesis. In retrospect, and in the light of remarks above concerning the importance of stringently applying appropriate GC-EAD techniques, the question arises as to whether such erratic results could perhaps be ascribed to the unfavorable elution profiles of such high molecular mass compounds, their nonoptimal introduction into the humidified air flowing over the antenna, or even to partial condensation on the walls of the air duct. The use of large sample sizes in efforts to detect small quantities of the more volatile EAD-active constituents probably even exacerbated the problem. To the best of the author’s knowledge, long-chain compounds such as those present in the male abdominal secretions of the Kheper beetles have to date not been subjected to GC-EAD analysis. Future investigations into the function of these constituents of the male abdominal secretions of Kheper beetles could require the development of appropriate instrumentation for EAD analyses of these compounds.
The problems associated with analytes being eluted as fronting peaks could be solved by carrying out GC-FID/EAD analyses on a comprehensive two-dimensional gas chromatograph (GC2 or GC × GC) in which a cryogenic modulator (Beens et al. 2001) focuses small portions of the effluent from the so-called first-dimension column into narrow concentrated bands of analytes, which are delivered into the second-dimension column for separation on a different stationary phase. Analytes are eluted from the second column of a GC2 instrument as extremely sharp peaks. Depending on the stationary phases used in the instrument, different compound classes are grouped in different areas of the resulting comprehensive two-dimensional gas chromatogram. Thus, in addition to delivering sharp analyte pulses to the preparation, excellent separation of the constituents of complex mixtures can be achieved, and some information on the chemical properties of EAD-active compounds can even be obtained. If less complex mixtures have to be analyzed, the instrument can be operated in the one-dimensional mode by replacing the second-dimension column with a retention gap of the same internal diameter. Alternatively, a standard GC can be adapted by installing a device for cyclic cooling and heating a short section of a retention gap connected to the capillary column. Construction of an appropriate device for this purpose might require assistance from an experienced chromatographer.
3.8. COMPOSITION OF THE PHEROMONE-DISSEMINATING CARRIER MATERIAL
The minute quantities of VOCs present in the abdominal secretion of the male Kheper beetles are soluble in the common organic solvents and were extracted with, for example, dichloromethane, which left the insoluble white carrier material intact. The carrier material was found to be soluble in concentrated sulfuric acid and in aqueous solutions of other strong acids. When a speck of the secretion was heated in a small flame the typical odor of burning hair was detected, and it was concluded that the carrier material could be, or could contain, a polypeptide. Although the use of a synthetic polypeptide as support material in field tests of the synthetic analogs of the semiochemicals identified was not envisaged, it was evident that more specific information was required for the selection of a chemically similar and readily available protein for this purpose. As far as the general appearance and brittleness of the fibers are concerned, the males of the Kheper species produce a similar material, although that of K. nigroaeneus appears to consist of finer fibers, and that of K. subaeneus softer and slightly sticky fibers.
SDS polyacrylamide gel electrophoresis (SDS-PAGE) of the carrier material of three Kheper species (Figure 3.12) revealed the presence in each secretion of proteins, with molecular masses of approximately 17 kDa and approximately 31 kDa, with the 17-kDa protein as the major fraction.
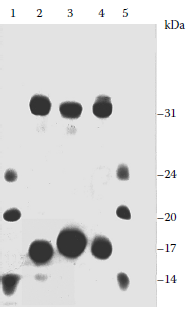
FIGURE 3.12
SDS-PAGE of the pheromone disseminating secretion of K. lamarcki (lane 2), K. nigroaeneus (lane 3), K. subaeneus (lane 4), and mass marker proteins (lanes 1 and 5): trypsinogen, 24 kDa; soybean trypsin inhibitor, 20.1 kDa; α-lactalbumin, 14.2 (more...)
In the absence of the reducing agent, β-mercaptoethanol, the apparent molecular mass of the 17-kDa protein decreased to 15 kDa, indicating that the naturally occurring protein exists as a compactly folded protein stabilized by intramolecular disulphide linkages. On breaking these linkages with β-mercaptoethanol, the molecule unfolds to a more extended structure with lower mobility. Minor differences in the electrophoretic mobility of the proteins secreted by the three species may reflect minor differences in their overall structures. It is possible that the 31-kDa proteins are differently linked dimers of the smaller proteins. Complete amino acid sequencing was not attempted, but partial sequence analysis revealed a single sequence to be present in the 15-kDa protein isolated from the K. nigroaeneus carrier material, whereas two sequences appear to be present in the major constituent from the K. lamarcki carrier material. The molecular mass of the 15-kDa protein of the pheromone-disseminating carrier material of the two species was verified by 252Cf plasma desorption MS. Average molecular masses of 15451±10 Da and 15477±10 Da were calculated for the 15-kDa proteins from the K. lamarcki and K. nigroaeneus carrier materials, respectively (Burger et al. 1990).
Due to the presence of relatively large amounts of glutamic acid and aspartic acid and smaller amounts of the basic amino acids, the proteinaceous carrier materials were expected to have low isoelectric points. The proteins also contain large proportions of hydrophobic amino acids. As most of the VOCs present in the abdominal secretions of these insects are long-chain fatty acids, these properties are not unexpected. The amino acid composition of these proteins is similar to that of serum albumin, which is known to bind a large number of hydrophobic compounds, including dodecyl sulphate and fatty acids (e.g., Noverr and Huffnagle 2004). This suggests that these proteins might have a high affinity for compounds such as the fatty acids that were identified as EAD-active constituents of the volatile organic fraction of the abdominal secretions of these insects. This supports the purported controlled-release pheromone disseminating function of the carrier material. On the other hand, in the dry state, these secretions have large surface areas, which might accelerate the evaporation of the adsorbed volatile constituents. In view of the disappointing results of the bioassays carried out with a few of the organic compounds isolated from the male abdominal secretion of K. lamarcki, no further investigation into the structures of these proteins was undertaken.
This could potentially be an interesting field of research for entomologists and chemists interested in applying the powerful analytical techniques that are now available for the investigation of the role of proteins in the dissemination of semiochemicals.
A number of experiments were carried out to compare the affinity of the carrier protein of K. lamarcki and proteins such as albumin and trypsin for the compound 2,6-dimethyl-5-heptenoic acid, which elicited EAG responses in the antennae of this insect. In these experiments, the compound was allowed to evaporate from a dispensing glass-fiber sleeve or wick, and was taken up in four receiving sleeves spaced equidistant around the dispensing wick. The wicks were coated with the carrier protein of K. lamarcki, bovine albumin, and bovine pancreas trypsin, respectively. The fourth glass fiber wick was left untreated, as a control. The device that was designed for this experiment is depicted in Figure 3.13. Thermal desorption and quantitative GC analysis of the 2,6-dimethyl-5-heptenoic acid retained by the receiving sleeves showed that albumin and the carrier protein retained the acid approximately equally effectively, while trypsin retained the acid about three times less effectively (Burger et al. 1990). These experiments probably constitute the earliest reported attempts at demonstrating that proteins could be involved in pheromone dissemination.
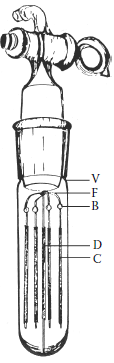
FIGURE 3.13
Device designed for the determination of the affinity of various proteins for 2,6-dimethyl-5-heptenoic acid. V, glass vial; F, five-pronged glass framework constructed from 1.0-mm glass rod and supported on the central prong; D, dispensing glass-fiber (more...)
The strong retention of the acid by the carrier protein suggests that controlled release in order to retain the integrity of the message rather than rapid release of the pheromone might be the primary function of the carrier protein. This unique pheromone disseminating mechanism might be more effective under adverse climatic conditions than other release mechanisms normally employed by insects. Where related species occupy the same habitat, the integrity of their chemical signals may be of vital importance to their survival, especially when, as in the case of these Kheper beetles, a pair of dung beetles normally produces only one or two offspring per year. Using a solid carrier material impregnated with the semiochemicals has the advantage that a chemical message transmitted in a protein particle will retain its integrity over longer distances. As the volatile fraction of the secretion contains compounds with widely different polarities, the polypeptide carrier could also have a marked influence on the ratio in which these compounds are released into the atmosphere. This could be another interesting field of research for interested scientists.
3.9. INTERGENERIC AND INTERSPECIFIC CHEMICAL SIGNALING IN DUNG BEETLES
3.9.1. Intergeneric Communication
Instead of using an antenna of K. lamarcki in a GC-FID/EAD analysis of an extract of the male abdominal secretion of this species, an antenna of another dung beetle, Pachylomerus femoralis, was on occasion inadvertently used as sensing element. The latter species is slightly larger than K. lamarcki. The distribution of these two dung beetles overlaps in areas with sandy soil, which appears to be a prerequisite for colonization by P. femoralis. A series of highly volatile constituents of the K. lamarcki secretion elicited strong responses in the antenna of P. femoralis. Remarkably sharp and strong responses were reproducibly obtained in subsequent analyses carried out under the same experimental conditions. A typical set of gas chromatograms obtained in a GC-FID/EAD analysis of an extract of the abdominal secretion of male K. lamarcki is depicted in Figure 3.14. The majority of the EAD-active constituents identified by GC-MS analysis and by MS and retention time comparison with authentic synthetic compounds are listed in Table 3.3 (Burger et al. 1995a).
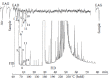
FIGURE 3.14
GC-FID/EAD analysis of the male abdominal secretion of K. lamarcki using an antenna of a female P. femoralis. Corresponding peaks in the FID and EAD traces are numbered consecutively in order of elution. For peak identities, see Table 3.3. (From Petersen, (more...)
TABLE 3.3
Constituents of the Male Abdominal Secretion of K. lamarcki that Elicited Antennal Responses in the Excised Antennae of P. femoralis
Whereas K. lamarcki is an industrious producer of dung balls, P. femoralis has never yet been observed constructing dung balls from dung taken from dung pats or middens. However, it has been found rolling dung fragments that mostly had irregular shapes. A relatively strong EAD response was observed at a retention time between those of ethyl butanoate 7 and methyl 4-pentenoate 9 in Figure 3.14. No indication of the presence of this compound could be found in either the FID trace or the total ion current (TIC) trace obtained in a GC-MS analysis of the extract. Constituents 1, 2, 4, and 8 remained unidentified. Although not present in the sex attractant secretion of K. lamarcki, FID/EAD analyses were also carried out with the methyl and ethyl esters of the E and Z isomers of both 2-pentenoic acid and 3-pentenoic acid. It was found that all of these esters elicited EAD responses that were of similar strength as those elicited by ethyl and methyl 4-pentanoate in P. femoralis antennae.
The possibility that the identified compounds could have been adsorbed from the horse dung on which the dung beetles were fed was investigated by carrying out headspace gas analyses on samples of horse dung using solid-phase microextraction (Arthur and Pawliszyn 1990) as concentration technique in conjunction with GC-FID/EAD analysis. No indication was found that any of the EAD-active esters were present in the horse dung. In a control experiment, the dung was spiked with small amounts of methyl and ethyl pentanoate. These two esters were easily detected in the spiked samples (Burger et al. 1995a).
In field tests, approximately equal numbers of P. femoralis were attracted to pitfall traps baited with horse dung and traps baited with a mixture of the compounds listed in Table 3.3. In the absence of dung, methyl and ethyl (Z)-and (E)-2-butenoic acid (crotonic acid) did not attract any P. femoralis to pitfall traps, whereas the individual saturated esters listed in Table 3.3 attracted small numbers of this species.
Taking the results of the almost forgotten GC-FID/EAD analysis depicted in Figure 3.5 into consideration, it was realized that some or even all of the esters that elicited responses in antennae of P. femoralis could be constituents of the male secreted sex attractant of K. lamarcki or of some other pheromone of this species—unfortunately only after our research on dung beetles had largely been completed.
In the absence of sufficient information, any explanation of the response in P. femoralis to the presence of the short-chain esters in the male abdominal secretion of K. lamarcki must necessarily be of a speculative nature. However, it is possible that P. femoralis, not being capable of forming dung balls, employs the volatile compounds identified in the abdominal secretion of K. lamarcki, as a kairomone to find dung of a suitable shape, such as a dung ball, for transportation to its burrow. The calling behavior of K. lamarcki thus exposes it to being robbed by the aggressive P. femoralis. In contrast, male K. nigroaeneus produce abdominal secretion only after having safely buried their dung balls. In captivity in the greenhouses in Stellenbosch, secreting K. lamarcki males were often disturbed by P. femoralis, while K. nigroaeneus males did not seem to elicit similar interest from P. femoralis. Further interesting aspects of the ethology and chemical ecology of P. femoralis are discussed in Section 3.10.
3.9.2. Interspecific Communication
The discovery of the response elicited in the antennae of P. femoralis by certain constituents in the abdominal secretion of K. lamarcki led to an investigation of the possibility that, for some reason and under certain conditions, coexisting dung beetle species might be semiochemically aware of the presence of other species in their home range. Only two of the four Kheper species under investigation coexist in the Mkuzi Game Reserve and were used in an attempt to test this hypothesis. In GC-FID/EAD analyses, antennae of male as well as female K. nigroaeneus responded reproducibly to 3-methyl-2-butanone, skatole, and (E)-nerolidol, and nonreproducibly to 2,6-dimethyl-5-heptenoic acid (compounds that were identified in extracts of the male abdominal secretion of K. lamarcki). As in other analyses in which antennae of K. lamarcki were used as sensing elements, the VOCs of the abdominal secretion of K. nigroaeneus did not elicit responses in the antennae of the former species with the exception of skatole (Petersen 1990). At this stage there is no plausible explanation for these results. It is possible that many additional compounds could be involved in this type of interspecific semiochemical interaction. It is an interesting phenomenon that could lead to the discovery of interesting information, but it would require the development of an absolutely reliable EAD setup and experimental protocol.
3.10. PECULIAR BEHAVIOR IN P. FEMORALIS
Due to their production of a visible sex-attracting secretion, research was mainly focused on the four species of the genus Kheper that are found in South Africa. However, large numbers of P. femoralis were often found together with K. lamarcki in pitfall traps and sometimes on a few dung middens in the Mkuzi Game Reserve, and it was thus possible to observe the interesting nesting behavior of the latter insect. Both species of the African dung beetle genus Pachylomerus are found in southern Africa, but they do not overlap in distribution. P. femoralis inhabits wetter areas stretching from the northern parts of KwaZulu-Natal through the northern parts of South Africa and into northeastern Botswana. P. opaca is smaller than P. femoralis and inhabits the drier northwestern Cape and southern Namibia (Tribe 1976). P. femoralis is a large dung beetle (approximately 40 mm × 25 mm) with a highly developed prothorax and forelegs.
According to Tribe, the typical behavior of P. femoralis is to construct an unbranched tunnel within about 300 mm of a dung pat by digging with the foretibiae and clypeus and then turning around in the burrow and pushing out the loosened soil or sand, using the prothorax as a shovel. The excavated soil is used to build a ramp that leads to the dung pat. The burrow is provisioned with dung by the beetle making several trips to and from the dung. Irregular pieces of dung are then butted with the head or rolled to the burrow entrance and pushed inside with the head. P. femoralis is very aggressive, defending both a large section of the dung pat and the ramp against any intruder.
Although we never observed the construction of dung balls from dung taken from a dung pat or midden by P. femoralis, this species has on a few occasions been found rolling dung fragments along the roads of the reserve. The dung fragments had irregular shapes, not having been formed into balls, and were relatively small compared to the enormous dung balls that the slightly smaller K. lamarcki usually constructs. Another peculiarity is that, on the few occasions that this otherwise rare rolling behavior was observed, many P. femoralis were observed rolling dung fragments along the roads of the reserve during the late afternoon—and always when a thunderstorm was expected. This behavior was not repeated the following day in the same area after the rain. It is possible that this ball-rolling behavior could be induced by a drop in atmospheric pressure or other climatological conditions.
During field trials with synthetic analogs of the constituents of the male abdominal secretion of K. lamarcki in November 1989, we observed a P. femoralis rolling one of the seeds of a spineless monkey orange tree, Strychnos madagascariensis Poiret. Subsequent work revealed this apparently aberrant behavior to be quite common in this insect, at least in the Mkuzi Game Reserve. This phenomenon has not been described before, despite the intensive research that has been carried out on dung beetles in the Mkuzi and Hluhluwe Game Reserves during the Australian Dung Beetle Project.
The spineless monkey orange tree, also known as the yellow or black monkey orange tree in certain parts of South Africa, is found in large numbers in those parts of the Mkuzi Game Reserve with light sandy soil. It bears fruit with the shape, size, and color of an orange, but with an extremely hard shell. The fruit usually starts ripening towards the middle of November in the northern parts of KwaZulu-Natal. Each fruit contains 10 or more hard seeds to which a thin layer of soft flesh adheres. The seeds have irregular shapes, an average diameter of about 20–30 mm, and are interspaced with thin layers of fleshy material that has a strong pleasant flavor—almost like that of the mango. The fleshy material is consumed by animals that have strong enough jaws to fracture the shell of the fruit. Because the edible parts of the fruit form a relatively small proportion of the fruit, it has not been commercially exploited for human consumption. The dung beetle rolling the seed of this fruit must have found one that had been left intact with its fruit flesh by one of these animals. In a first test carried out immediately after this peculiar behavior had first been observed, two of 12 seeds simply put out on the ground were removed by P. femoralis within a few hours. In another experiment carried out in the same area, 24 P. femoralis beetles were caught in two pitfall traps baited with seeds of the fruit, whereas only one P. femoralis, in addition to several dung beetles from other species, was caught in nine traps baited with horse dung. No dung beetles were caught in traps baited with banana and pawpaw, the only fruit available commercially in the Mkuzi area early in November.
Qualitative analyses were carried out by trapping the VOCs responsible for the flavor of the fruit on an open tubular trap (Grob and Habich 1985) coated with polydimethylsiloxane (film thickness 15 µm). The trapped volatiles were desorbed from the trap and analyzed by GC-MS. The compounds that could possibly be responsible for the attraction of P. femoralis to the fruit flesh of the spineless monkey orange were distinguished from other inactive volatiles using FID and EAD detection in parallel. A typical set of FID/EAG gas chromatograms of the headspace volatiles from the fruit flesh of the spineless monkey orange is depicted in Figure 3.15 (Burger and Petersen 1991).
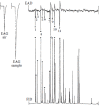
FIGURE 3.15
GC-FID/EAD analysis of the headspace gas volatiles from the fruit of Strychnos madagascariensis using a female P. femoralis antenna as a sensing element. Corresponding peaks in the FID and EAD traces are numbered consecutively in order of elution. For (more...)
Regarding the relative sizes of the EAD signals, these analyses were remarkably reproducible. The EAD signals were sharper, and with a few exceptions, their signal-to-noise ratios were higher than those obtained in GC-FID/EAD analyses of the VOCs of the male abdominal secretion of, for example, K. bonellii. Male and female antennae produced identical responses. The antennae of P. femoralis were viable for more than 8 hours. The EAD-active compounds are listed in Table 3.4. Many other constituents related to the EAD-active compounds, such as 1-hexanol, butanoic acid, hexanoic acid, and a large number of saturated and unsaturated esters from 2-methylpropyl ethanoate (2-methylpropyl acetate) to butyl dodecanoate that are present in the spineless monkey orange (Pretorius et al. 1987), gave no, or only very weak, EAD responses.
TABLE 3.4
Constituents of the Headspace Gas of the Fruit of the Spineless Monkey Orange Tree, Strychnos madagascariensis, That Elicited Antennal Responses in Excised Antennae of Pachylomerus femoralis
To obtain information on the composition of the odor plume of the fruit under field conditions, the VOCs released by the fleshy parts of a spineless monkey orange were determined in air flowing at approximately 0.16 km/h over 20 seeds and the fleshy parts of a single spineless monkey orange. The volatiles were trapped on an ultrathick film trap (film thickness 145 µm) (Burger et al. 1991). The quantitative composition of the EAD-active compounds in the odor plume of the fruit is given in Table 3.4.
Although dispensing a mixture of these EAD-active compounds from a small vial via a filter-paper wick was not expected to release the compounds in the same ratio in which they are present in the odor plume of the fruit, eight and 14 P. femoralis were caught within 2 hours, respectively, in two pitfall traps baited with the synthetic material, whereas five and 32 beetles were found, respectively, in two traps baited with spineless monkey orange. It was furthermore found that the individual compounds and mixtures of two or three of the compounds were also attractive to P. femoralis; three insects were found in a trap baited with a 1:1 mixture of methyl and ethyl butanoate, and eight insects in a trap baited with a 4:4:1 mixture of butyl 2-methylpropanoate, butyl butanoate, and butyl propanoate.
As in field tests with synthetic components of the abdominal secretion of male K. lamarcki combined with horse dung, large differences were found in the number of beetles caught in traps baited with either dung or fruit. One trap, for example, contained 38 P. femoralis, whereas not a single beetle of this species was found in a trap situated 100 m from the first one and baited with the same material. Due to dense vegetation, it was impossible to observe the movement of animals in that area and to determine how far a trap was situated from a fresh dung source or from the nearest spineless monkey orange tree. Under these circumstances, the resulting quantitative data were not amenable to statistical analysis. However, the results did indicate that the attractiveness of the mixture of synthetic compounds for P. femoralis is at least comparable to that of the fresh spineless monkey orange.
At the time that the field tests were carried out, large numbers of fruit (some of them opened by primates) were lying beneath the trees and the air was permeated with the smell of the fruit in areas with a large population of spineless monkey orange trees. No beetles were caught in traps baited with the fruit in these areas, whereas more than 30 P. femoralis were found in traps in the same area baited with horse dung. In contrast, P. femoralis beetles were attracted more strongly to the fruit than to horse dung in areas where there is much animal activity and where spineless monkey orange trees are scarce or the fruit has not yet ripened.
In spite of fierce competition for food on relatively small quantities of horse dung placed in the field, no dung ball formation or rolling of balls or fragments of dung from these dung sources by P. femoralis was observed. In contrast, practically all the P. femoralis attracted to the contents of spineless monkey oranges started frantically rolling seeds, either immediately on arriving at the fruit or after inspecting the fruit for a few seconds. A few beetles attempted to push seeds into existing holes in the ground.
The attraction to fruit or plant material is not unique to P. femoralis. Halffter and Matthews (1966) have reported the attraction of dung beetles to carrion and rotting fruit. Burger et al. (1991) found a few of the small golden-brown dung beetles, Proagoderus aureiceps d’Orbigny, in many of the traps spiked with spineless monkey orange. It is difficult to suggest a plausible explanation for this phenomenon. There are many imponderables to consider: Why do these dung beetles roll these seeds, of which the adhering fruit flesh is practically dried out by the time the seeds are buried? Could these dung beetles have learnt that rolling the seeds is a futile exercise in areas where there is an ample supply of the fruit? Are they so strongly territorial that they do not forage in neighboring areas, although such areas are not more than a few seconds apart at the speed at which they can fly? Could the confusing results of bioassays be ascribed to the attraction to either fruit or dung being overridden by the nature of the soil in the areas where the field tests were carried out?
In the light of the paucity of information currently available, an explanation of the phenomenon could be based on the attraction of P. femoralis by the male abdominal secretion of K. lamarcki, as discussed above, and the coincidental presence of identical, or at least structurally related, compounds in spineless monkey oranges. A second explanation could be based on the possibility that the attractants present in the fruit are probably excreted by the primates that consume the fruit (especially when they overindulge) and it is thus possible that P. femoralis could associate the aroma of the fruit with the availability of the feces of these animals.
3.11. DEFENSIVE MECHANISMS IN ONITICELLUS EGREGIUS
Elaborate chemical defense and associated escape behavior is found in the endocoprid dung beetle Oniticellus egregius Klug (Davis 1989). This species constructs its brood ovoids of dung in the soil immediately under the edge of the dropping, enveloping each ovoid in a soil shell before clearing the loose earth around the ovoids to produce a brood chamber (Davis 1989). This species has been recorded mainly from the course dung of elephant, rhinoceros, and zebra (Davis 1977).
Several protective mechanisms are utilized by O. egregius. The dorsal surface of this insect (9.8–15.5 mm) is metallic blue-black with a yellow border (Figure 3.1h), while the ventral surface is mottled yellow and gold. If disturbed, O. egregius flip themselves onto their backs and exhibit thanatosis, holding the middle and hind legs away from the body. This exposes the ventral surface that is colored similarly to the fibers of dry rhinoceros or elephant dung and renders them inconspicuous (Figure 3.1i). Another escape mechanism could also be employed: the forelegs that are held close to the body are suddenly released with sufficient force to lift the beetle as high as 60 cm into the air; it flips and becomes cryptic where it lands among the shredded dung. It simultaneously releases a brown fluid from the lateral edge of the anterior abdominal segments just posterior to the hind legs (Davis 1977). This secretion, reminiscent of oil of wintergreen, has been identified as methyl salicylate (oil of wintergreen) and 1,4-benzoquinone (Burger et al. 1995b). 1,4-Benzoquinone and its derivatives have been identified in several orders of the Diplopoda and Insecta and these compounds appear to be relatively common in the defensive secretions of the Coleoptera (e.g., Blum 1981). The esters of silacylic acid seem to be much less well represented. Methyl salicylate has been found in the secretion of the carabid beetle (Schildknecht et al. 1968). Ants and termites frequently inhabit dung pats in winter where they would encounter O. egregius and would likely be repelled by the wintergreen odor.
3.12. CONCLUSIONS, PERSPECTIVES, AND PROSPECTS
The identification of the constituents of the male abdominal sex-attractant secretions of the four Kheper species was the main focus of this first investigation into the semiochemistry of dung beetles in South Africa. The research was only partly successful, mainly due to the complexity of the volatile organic fraction of the male sex-attracting secretions, the time-consuming development of appropriate instrumentation and methodology for GC-FID/EAD analyses, and the untrustworthiness of the results of bioassays, which resulted from the fact that dung and not the sex attractant is the primary factor in the attraction of both males and females to the secretion-producing beetle. Nevertheless, some interesting information came to light.
In three of the four species, several of the constituents that reproducibly elicited EAD responses in male as well as female antennae are terpenoids or compounds with terpenoid-like structures that lack one or two carbon atoms. The role of the long-chain compounds in the male abdominal secretions was not satisfactorily elucidated. However, even though not reproducible with instrumentation available at the time, the EAD responses that some of these compounds elicited in the antennae of the insects could be seen as an indication that further investigation into the role of these compounds in chemical signaling in these species might be worthwhile.
The white abdominal pheromone-disseminating secretions of three of the four South African Kheper species were investigated. They were found to consist of only two proteins, with molecular masses of approximately 17 kDa and approximately 31 kDa, respectively. The proteins are similar, but not identical, in the three species. Semiochemical communication appears to be more complex in the Coleoptera than in other orders. Investigation of the phenomenon in dung beetles was complicated by weak EAD responses in GC-FID/EAD analyses. The unreliability of bioassays in the field was a further complicating factor. The research carried out to date has only scratched the surface of a wide and extremely interesting topic. The main benefit of this part of the research was that it demonstrated the potential that lies in this field of research for entomologists and chemists interested in semiochemical communication in the Coleoptera.
An investigation into the possible role of semiochemicals in the courtship behavior between male and female after arrival of the insects on a dung pat could be a difficult, but nevertheless very rewarding endeavor, particularly because of the extremely important ecological role of dung beetles. In this regard, the role of these insects in the reduction of the production of methane in the dung of herbivores has to date been largely disregarded.
In contrast to the somewhat disappointing results of the investigation of the sex attractants of the Kheper species, the serendipitously discovered seed-rolling behavior of P. femoralis produced interesting and exciting results. In GC-FID/EAD analyses of the VOCs present in the fruit of the spineless monkey orange tree, S. madagascariensis, reproducibly elicited strong EAD responses in both male and female antennae of this dung beetle.
Identical and similar short-chain esters were identified in extracts of the male abdominal secretion of K. lamarcki. The success of this part of the research largely made up for many disappointments in the research on the Kheper species. Unfortunately, the limited time that was annually available for the dung beetle research precluded pursuing this research to a satisfactory conclusion. The bioassays should be repeated with the synthetic esters as well as with the fruit in areas where there are no spineless monkey orange trees or when there is no ripe fruit available in the field.
The Laboratorium vir Ekologiese Chemie Universiteit van Stellenbosch (LECUS) has now concluded its research on dung beetles. It is hoped that the research discussed in this chapter will encourage other scientists to become interested in a still largely unexplored and very lucrative field of research that holds promises of many exciting discoveries—there are so many questions still to be answered.
ACKNOWLEDGMENTS
I am indebted to Dr. Zenda Ofir (néé Munro) and Dr. Warren Petersen, who devoted a total of 18 difficult years of their lives to carry out the research discussed in this chapter, to my wife, Wina, who collected the dung beetle secretions at unbearable temperatures, and to the KwaZulu-Natal Parks Board for permission to collect dung beetles and carry out behavioral experiments in the Mkuzi and Hluhluwe Game Reserves. I am grateful for the invaluable entomological expertise and assistance of Dr. Geoff Tribe, who also read the manuscript to verify its entomological content. The research has been funded by the National Research Foundation of South Africa and by Stellenbosch University.
REFERENCES
- Arthur C. L, Pawliszyn J. Solid phase microextraction with thermal desorption using fused silica optical fibers. Anal. Chem. 1990;62:2145–2148.
- Beens J, Adahchour M, Vreuls R. J. J, Klaas K. V, Brinkman U. A. Th. Simple, non-moving modulation interface for comprehensive two-dimensional gas chromatography. J. Chromatogr. A. 2001;919:127–132. [PubMed: 11459298]
- Blum M. S. Chemical Defences of Arthropods. Academic Press; New York: 1981.
- Bornemissza G. F, Williams C. H. An effect of dung beetle activity on plant yield. Pedobiologia. 1970;10:1–7.
- Burger B. V, Röth M, le Roux M, Spies H. S. C, Geertsema H. The chemical nature of the defensive larval secretion of the citrus swallowtail, Papilio demodocus. J. Insect Physiol. 1978;24:803–805.
- Burger B. V, Munro Z, Röth M, Spies H. S. C, Truter V, Tribe G. D, Crewe R. M. Studies on the pheromones of Scarabaeinae, I. Composition of the heterogeneous sex attracting secretion of the dung beetle, Kheper lamarcki. Zeitschrift Naturforsch. 1983;38c:848–855.
- Burger B. V, Munro Z, Brandt W. F. Pheromones of the Scarabaeinae, II. Composition of the pheromone disseminating carrier material secreted by male dung beetles of the genus Kheper. Zeitschrift Naturforsch. 1990;45c:863–872.
- Burger B. V, Petersen W. G. B. Semiochemicals of the Scarabaeinae, III. Identification of an attractant for the dung beetle Pachylomerus femoralis in the fruit of the spineless monkey orange tree, Strychnos madagascariensis. Zeitschrift Naturforsch. 1991;46c:1073–1079.
- Burger B. V, Petersen W. G. B, Tribe G. D. Semiochemicals of the Scarabaeinae, IV: Identification of an attractant for the dung beetle Pachylomerus femoralis in the abdominal secretion of the dung beetle Kheper lamarcki. Zeitschrift Naturforsch. 1995a;50c:675–860.
- Burger B. V, Petersen W. G. B, Tribe G. D. Semiochemicals of the Scarabaeinae, V: Characterization of the defensive secretion of the dung beetle Oniticellus egregius. Zeitschrift Naturforsch. 1995b;50c:681–684.
- Burger B. V, Petersen W. G. B. Semiochemicals of the Scarabaeinae, VI. Identification of EAD-active constituents of abdominal secretion of male dung beetle Kheper nigroaeneus. J. Chem. Ecol. 2002;28:501–513. [PubMed: 11944827]
- Burger B. V, Petersen W. G. B, Weber W. G, Munro Z. M. Semiochemicals of the Scarabaeinae, VII. Identification and synthesis of EAD-active constituents of abdominal sex attracting secretion of the male dung beetle Kheper subaeneus. J. Chem. Ecol. 2002;28:2527–2539. [PubMed: 12564798]
- Burger B. V, Petersen W. G. B, Ewig B. T, Neuhaus J, Tribe G. D, Spies H. S. C, Burger W. J. G. Semiochemicals of the Scarabaeinae, VIII. Identification of active constituents of the abdominal sex-attracting secretion of the male dung beetle, Kheper bonellii, using gas chromatography with flame ionization and electroantennographic detection in parallel. J. Chromatogr. A. 2008;1186:245–253. [PubMed: 17920608]
- Davis A. L. V. Grahamstown, South Africa: Rhodes University; 1977. The endocoprid dung beetles of southern Africa (Coleoptera: Scarabaeidae). MSc thesis.
- Davis A. L. V. Nesting of Afrotropical Oniticellus (Coleoptera: Scarabaeidae) and its evolutionary trend from the soil to dung. Ecol. Entomol. 1989;14:11–21.
- Dormont L, Epinat G, Lumaret J. P. Trophic preferences mediated by olfactory cues in dung beetles colonizing cattle and horse dung. Environ. Entomol. 2004;33:370–377.
- Dormont L, Rapior S, McKey D. B, Lumaret J. P. Influence of dung volatiles on the process of resource selection by coprophagous beetles. Chemoecol. 2007;17:23–30.
- Edwards P. B, Aschenborn H. H. Male reproductive behavior of the African ball-rolling dung beetle, Kheper nigroaeneus (Coleoptera: Scarabaeidae). The Coleopterists Bull. 1988;42:17–27.
- Ferreira M. C. Os escarabideos de África (Sul do Sáara). Revista. Ent. Moçamb. 1972;11(1968-1969/1972):1–1088.
- Grob K, Habich A. Headspace gas analysis: The role and the design of concentration traps specifically suitable for capillary gas chromatography. J. Chromatogr. A. 1985;321:45–58.
- Grob K, Müller R. Some technical aspects of the preparation of a retention gap in capillary gas chromatography. J. Chromatogr. A. 1982;244:185–196.
- Hadley N. F. Wax secretion and color phases of the desert tenebrionid beetle Cryptoglossa verrucosa LeConte. Science. 1979;203:367–369. [PubMed: 17772446]
- Halffter G, Matthews E. G. The natural history of dung beetles of the subfamily Scarabaeidae. Folia Entomol. Mexicana. 1966;12–14:1–312.
- Heinrich B, Bartholomew G. A. Roles of endothermy and size in inter- and intraspecific competition for elephant dung in an African dung beetle, Scarabaeus laevistriatus. Physiol. Zool. 1979;52:484–496.
- Hughes R. D. The bushfly. Aust. Nat. Hist. 1970;16:331–334.
- Leal W. S. Chemical ecology of phytophagous scarab beetles. Ann. Rev. Entomol. 1998;43:39–61. [PubMed: 15012384]
- Marshall A. T. Paraffin tubules secreted by the cuticule of an insect, Epipyrops anamala (Epipyropidae: Lepidoptera). J. Ultrastruct. Res. 1974;47:41–60. [PubMed: 4595994]
- Munro Z. M. University of Stellenbosch; South Africa: 1988. Semiochemiese kommunikasie: Ontwikkeling en toepassing van analitiese tegnieke vir die bepaling van die chemiese samestelling van die abdominale afskeiding van Kheper lamarcki, K. Subaeneus en K. nigroaeneus (Coleoptera: Scarabaeidae). PhD dissertation.
- Nair S, Braman S. K. A scientific review on the ecology and management of the azalea lace bug Stephanitis pyrioides Scott (Tingidae: Hemiptera). J. Entomol. Sci. 2012;47:247–263.
- Noverr M. C, Huffnagle G. B. Regulation of Candida albicans morphogenesis by fatty acid metabolites. Infect. Immun. 2004;72:6206–6210. [PMC free article: PMC523025] [PubMed: 15501745]
- Petersen W. G. B. South Africa: University of Stellenbosch; 1990. Aanwending van elektroantennografiese metodes in die chemiese karakterisering van semioverbindings van kewers. MSc thesis.
- Petersen W. G. B. University of Stellenbosch; South Africa: 1997. Semiochemiese kommunikasie by miskruiers van die genus Kheper (Scarabaeinae). PhD dissertation.
- Pretorius V, Rohwer E. R, Rapp A, Mandery H. Volatile constituents of the spineless monkey orange. Dtsch. Lebensm. Rundsch. 1987;83:180–182.
- Rohwer E. R, Pretorius V, Apps P. J. Simple press-fit connectors for flexible fused silica tubing in gas-liquid chromatography. J. High Resolut. Chromatogr. 1986;9:295–297.
- Schildknecht H, Winkler H, Krauss B, Maschwitz U. Über Arthropoden-Abwehrstoffe, XXVIII: Über das Abwehrsekret von Idiochroma dorsalis. Zeitschrift Naturforsch. 1968;23b:46–49. [PubMed: 4387853]
- Simmons L. W, Ridsdill-Smith T. J. Ecology and Evolution of Dung Beetles. Chichester, UK: Wiley-Blackwell; 2011.
- Tribe G. D. South Africa: University of Natal; 1976. The ecology and ethology of ball-rolling dung beetles (Coleoptera: Scarabaeidae). MSc (Agric.) thesis.
- Waterhouse D. F. The biological control of dung. Sci. Am. 1974;230:100–109.
- Wilson S. R, Prodan K. A. The synthesis and stereochemistry of cascarillic acid. Tetrahedron Lett. 1976;17:4231–4234.
- INTRODUCTION
- CHEMICAL ECOLOGY OF THE GENUS KHEPER
- COLLECTION OF THE ABDOMINAL SECRETION
- SAMPLE PREPARATION AND ANALYSIS
- OPTIMIZATION OF GC-FID/EAD INSTRUMENTATION
- CHEMICAL CHARACTERIZATION OF ABDOMINAL SECRETIONS OF THE MALE KHEPER SPECIES
- LONG-CHAIN CONSTITUENTS OF THE ABDOMINAL SECRETIONS
- COMPOSITION OF THE PHEROMONE-DISSEMINATING CARRIER MATERIAL
- INTERGENERIC AND INTERSPECIFIC CHEMICAL SIGNALING IN DUNG BEETLES
- PECULIAR BEHAVIOR IN P. FEMORALIS
- DEFENSIVE MECHANISMS IN ONITICELLUS EGREGIUS
- CONCLUSIONS, PERSPECTIVES, AND PROSPECTS
- ACKNOWLEDGMENTS
- REFERENCES
- A game theoretic model of kleptoparasitism with strategic arrivals and departures of beetles at dung pats.[J Theor Biol. 2012]A game theoretic model of kleptoparasitism with strategic arrivals and departures of beetles at dung pats.Barker HA, Broom M, Rychtář J. J Theor Biol. 2012 May 7; 300:292-8. Epub 2012 Feb 1.
- Dung beetle community assemblages in a southern African landscape: niche overlap between domestic and wild herbivore dung.[Bull Entomol Res. 2022]Dung beetle community assemblages in a southern African landscape: niche overlap between domestic and wild herbivore dung.Sands B, Mgidiswa N, Curson S, Nyamukondiwa C, Wall R. Bull Entomol Res. 2022 Feb; 112(1):131-142. Epub 2021 Aug 20.
- Greenhouse gas emissions from dung pats vary with dung beetle species and with assemblage composition.[PLoS One. 2017]Greenhouse gas emissions from dung pats vary with dung beetle species and with assemblage composition.Piccini I, Arnieri F, Caprio E, Nervo B, Pelissetti S, Palestrini C, Roslin T, Rolando A. PLoS One. 2017; 12(7):e0178077. Epub 2017 Jul 12.
- Review Engineering Aspects of Olfaction.[Neuromorphic Olfaction. 2013]Review Engineering Aspects of Olfaction.Persaud KC. Neuromorphic Olfaction. 2013
- Review A Review of Dung Beetle Introductions in the Antipodes and North America: Status, Opportunities, and Challenges.[Environ Entomol. 2021]Review A Review of Dung Beetle Introductions in the Antipodes and North America: Status, Opportunities, and Challenges.Pokhrel MR, Cairns SC, Hemmings Z, Floate KD, Andrew NR. Environ Entomol. 2021 Aug 12; 50(4):762-780.
- First Investigation of the Semiochemistry of South African Dung Beetle Species -...First Investigation of the Semiochemistry of South African Dung Beetle Species - Neurobiology of Chemical Communication
Your browsing activity is empty.
Activity recording is turned off.
See more...