NCBI Bookshelf. A service of the National Library of Medicine, National Institutes of Health.
Mitkus R, Zhao J, Stanek J, et al. Provisional Peer-Reviewed Toxicity Values for Ammonium Salts of Inorganic Phosphates: Monoammonium Phosphate (MAP) (CASRN 7722-76-1) Diammonium Phosphate (DAP) (CASRN 7783-28-0). Cincinnati (OH): U.S. Environmental Protection Agency; 2021 Sep.
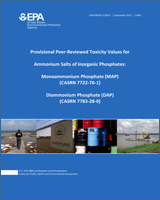
Provisional Peer-Reviewed Toxicity Values for Ammonium Salts of Inorganic Phosphates: Monoammonium Phosphate (MAP) (CASRN 7722-76-1) Diammonium Phosphate (DAP) (CASRN 7783-28-0).
Show detailsTables 3A and 3B provide overviews of the relevant noncancer and cancer evidence bases, respectively, for ammonium phosphate salts, and include all potentially relevant acute, repeated short-term, subchronic, and chronic studies as well as reproductive and developmental toxicity studies identified from the literature screening results. Principal studies used in the PPRTV assessment for derivation of provisional toxicity values are identified in bold. The phrase “statistical significance,” and term “significant,” used throughout the document, indicates a p-value of < 0.05 unless otherwise specified.
Table 3A
Summary of Potentially Relevant Noncancer Data for Ammonium Phosphate Salts (MAP, CASRN 7722-76-1; DAP, CASRN 7783-28-0; APP, CASRN 68333-79-9).
Table 3B
Summary of Potentially Relevant Cancer Data for Ammonium Phosphate Salts (MAP, CASRN 7722-76-1; DAP, CASRN 7783-28-0; APP, CASRN 68333-79-9).
2.1. HUMAN STUDIES
2.1.1. Occupational Studies
Bhat and Ramaswamy (1993)
In a published occupational health study, 91 workers in fertilizer plants in India were evaluated for respiratory function and compared with 68 controls matched for age, sex, body surface area, and socioeconomic status (Bhat and Ramaswamy, 1993). Of the 91 workers, 30 worked at a DAP plant, 30 worked at a urea plant, and 31 worked at an ammonia plant. Smokers were excluded from the study due to potential for confounding. No air samples were taken, and DAP exposure concentrations were not reported. Spirometry parameters (forced vital capacity [FVC], forced expiratory volume of 1 second [FEV1], and peak expiratory flow rate [PEFR]/minute) were evaluated using a portable spirometer. The study authors did not report the timing of spirometry measurements (e.g., before or after shift; before, during, or after a work week) or any further information on the selected controls.
Presumed exposure to DAP had a greater association with respiratory parameters than presumed exposure to urea or ammonia (Bhat and Ramaswamy, 1993). As reported in Table B-1, significant reductions in FVC, FEV1, and PEFR/minute were observed in workers at the DAP plant. Workers in all plants combined (not stratified by fertilizer type) were categorized by duration of employment: workers exposed (presumed) 0−10 years (51), workers exposed (presumed) for >10 years (40), and nonworker controls (68). Significant decreases in FEV1 and PEFR/minute were observed in workers exposed (presumed) 0−10 years compared to controls. All parameters were significantly decreased in workers exposed (presumed) >10 years. Due to a lack of any exposure information, these data are inadequate to establish effect levels.
Ruhe and Ehrenberg (1985)
NIOSH conducted an occupational health survey of 43 workers at an electric power facility construction site. At the site, insulation containing fiberglass, long-chain hydrocarbons, and ammonium phosphate was being cut (with a saw), resulting in airborne particles. Personal monitoring samples were collected and analyzed for formaldehyde and particulate but not for ammonium phosphate. Workers were asked to report irritation and “constitutional” symptoms occurring during the 24 hours and the 7 days prior to the site visit. Common symptoms reported by the workers were chest congestion, nasal irritation, and throat irritation. No relationship was found between exposure to the insulation particulate and prevalence of any symptom.
2.1.2. Other Human Studies
Fire extinguishers contain large, varying amounts of MAP in powder form (34−40% in some reports). Intentional inhalation and/or ingestion of fire extinguishing powder (during suicide attempts) has reportedly caused electrolyte imbalance and metabolic acidosis in numerous case studies [Becker et al. (2018) (published in German with English abstract), Doyon and McGrath (2003) (abstract only), Lee et al. (2016), Lin et al. (2009), Senthilkumaran et al. (2012)]. MAP doses were not estimated for these cases; however, serum phosphate levels in the patients were reported, ranging between 9.8 and 30.6 mg/dL (normal range is 2.3−4.5 mg/dL) [Doyon and McGrath (2003) (abstract only), Lee et al. (2016), Lin et al. (2009), Senthilkumaran et al. (2012)]. Effects reported in the patients included respiratory tract irritation, hyperphosphatemia, hypocalcemia, metabolic acidosis, delayed aspiration pneumonia, acute kidney failure, and cardiac arrest. A case study (Blumenthal and Hänert-Van der Zee, 2018) detailing autopsy results after a suicide reported a number of findings consistent with injury due to fire extinguisher pressure (e.g., ethmoid fracture, esophageal rupture, alveolar distension and rupture) as well as histology changes that may or may not be related to these injuries (pulmonary edema, vascular congestion, crystalline lattice in some alveoli). Effects attributable to ammonium phosphate exposure could not be discerned in this case.
Two studies conducted in the 1930s evaluated the use of ammonium phosphate salts (not further described) as urinary acidifiers, apparently to enhance the action of “urinary antiseptics” such as hexamine. Scott (1931) administered 20 g ammonium phosphate in solution to five volunteers 4 times/day, alternating 2 days of administration and 2 days off for 20 days (10 days of treatment). Urine samples collected over the course of the study showed that urine tended to be at lower pH when administered DAP when compared with samples collected on days that DAP was not administered; no other endpoints were evaluated. Alstead (1936) administered “acid ammonium phosphate” in doses of 2.1−6 g to 34 hospital patient volunteers 3 or 4 times/day for an unspecified duration and compared the patients’ urinary pH levels with those seen in patients receiving sodium phosphate. The results showed decreased urinary pH with administration of ammonium phosphate, compared with sodium phosphate.
2.2. ANIMAL STUDIES
2.2.1. Oral Exposures
Subchronic Studies
Huntingdon (2002) as cited in OECD (2007b) and ECHA (2002)
In an unpublished, Good Laboratory Practice (GLP)-compliant, OECD 422 guideline study cited in OECD (2007b), OECD (2007f), and ECHA (2002), Sprague Dawley rats (5/sex per toxicity subgroup; 10 females and 5 males per reproductive subgroup) were administered 0, 250, 750, or 1,500 mg DAP/kg-day [purity >87% as reported in OECD (2007b) and OECD (2007c)] via gavage in water for 35 days (toxicity subgroup) or through Lactation Day (LD) 4 in females (28 and 53 days of exposure for parental males and females, respectively) of the reproductive subgroup [Huntingdon (2002) as cited in OECD (2007b)]. Doses were analytically verified by spectrophotometry. The source, nature, and composition of the animals’ diet, including the calcium and baseline phosphate contents, were not reported. Mortality, clinical signs, body weight, and food consumption were monitored. In the toxicity subgroup, blood was collected during Week 5 for determination of hematology (comprehensive endpoints including clotting parameters) and clinical chemistry (alkaline phosphatase [ALP], alanine aminotransferase [ALT], aspartate aminotransferase [AST], γ-glutamyl transferase [GGT], total bilirubin, albumin, total protein, urea, creatinine, glucose, total cholesterol, and electrolytes) for the toxicity, but not the reproductive, subgroup. Functional operational battery (FOB) endpoints (approach response, touch response, auditory startle reflex, tail pinch response, forelimb and hindlimb grip strength, and motor activity) were evaluated after 4 weeks. The following tissues from the toxicity subgroup were weighed: adrenals, brain, epididymides, heart, kidneys, liver, ovaries, pituitary, prostate, seminal vesicles, spleen, testes, thymus, thyroids with parathyroid, uterus with cervix, and vagina. Although organ weights measured in the reproductive subgroup were not specified, the test guideline followed in the study (OECD 422) (OECD, 1996) indicates that organs weighed for reproductive effects include gonads (testes and ovaries), accessory sex organs (uterus and cervix, epididymides, prostate, seminal vesicles plus coagulating glands), and vagina. In the toxicology subgroup, organs fixed for histological analysis included any with observed abnormalities, as well as the adrenals, aorta, brain, caecum, colon, duodenum, epididymides, eyes, heart, ileum, jejunum, kidneys, liver, lungs, lymph nodes, mammary area, esophagus, ovaries, pancreas, pituitary, prostate, rectum, salivary glands, sciatic nerves, seminal vesicles, skin, spinal cord, spleen, sternum (bone marrow), stomach, testes, thymus, thyroid, trachea, urinary bladder, uterus, and vagina. In the reproductive subgroup, organs fixed for histological analysis included those with abnormalities, as well as reproductive organs (not specified, but likely included gonads and accessory sex organs based on OECD 422). Reproductive parameters (mating, gestation, and parturition parameters, including: precoital interval, mating performance, and fertility; gestation length and gestation index; litter size; and offspring survival indices) were assessed, and offspring were evaluated (litter size, offspring survival indices, sex ratio, offspring body weight, and gross pathology) through Postnatal Day (PND) 4.
Statistical tests were conducted using Fisher’s exact test for categorical data and Bartlett’s test for continuous data comparisons to control, incorporating multiple comparisons where needed. In the instance of a positive Bartlett’s test, a Behrens’s Fisher test was used for pairwise comparisons; otherwise, a Dunnett’s test was used.
One female in the 1,500 mg DAP/kg-day toxicity subgroup died during the study (time point not reported); ECHA (2002) noted that findings in this animal were consistent with dosing error.2 Dose-dependent increased incidences of the clinical signs of postdosing salivation and reddening of the extremities were noted starting at 250 mg DAP/kg-day. Decreased body-weight gain (78% of control value) was reported in males, but not females, of the 1,500-mg DAP/kg-day toxicity subgroup. Food consumption was marginally suppressed in males of the 1,500-mg DAP/kg-day group only. In the reproductive subgroup, an initial decrease in body-weight gain was noted in females during the 1st week of gestation, but body weights recovered to control levels after the week and remained normal through PND 4. No neurological effects were observed during the FOB. The only hematological finding was reduced activated partial thromboplastin time in males, but not females, administered 750 or 1,500 mg DAP/kg-day [26 and 24% less than controls, respectively; statistically significant changes reported here and below based on ECHA (2002) and OECD (2007b) unless otherwise noted]. Clinical chemistry alterations in males were increased ALP (32 and 31% higher than controls at 750 and 1,500 mg DAP/kg-day, respectively), decreased glucose (21% less than control) and phosphorus (18% less than control) at 1,500 mg/kg-day, decreased total protein (7 and 9% less than control at 750 and 1,500 mg DAP/kg-day, respectively), and a 17% increase in albumin:globulin (A/G) ratio at 1,500 mg DAP/kg-day. Clinical chemistry alterations in females were decreased phosphorus levels (19% less than controls) and a nonsignificant increase in ALP (22%) at 1,500 mg DAP/kg-day. Relative liver and kidney weights were increased (quantitative data not reported) in females at 1,500 mg DAP/kg-day; no organ-weight changes were noted in males. Both sexes exhibited horizontal banding on the incisors of teeth in the 750 and 1,500-mg DAP/kg-day dose groups; histological examination showed that this was limited to the enamel and likely reflected direct effects on tooth mineralization. Thickening of the stomach was also noted upon gross examination in both sexes at doses ≥750 mg DAP/kg-day. In the toxicity subgroup, histological evidence of submucosal inflammation in the stomach was noted in 0/5, 3/5, 4/5, and 2/5 males and 0/5, 2/5, 4/5, and 4/5 females at doses of 0, 250, 750, and 1,500 mg DAP/kg-day, respectively (see also Table B-2). The severity of these lesions was reported to be only minimal or slight in all cases. Incidences were statistically significant in females at doses ≥750 mg DAP/kg-day and males at 750 mg DAP/kg-day. Because the available data did not suggest sex differences in the inflammation, the incidences in males and females were combined for this review to increase statistical power. When incidences for males and females in the toxicity subgroup were combined (0/10, 5/10, 8/10, 6/10), the incidences at all doses were significantly increased relative to controls (p ≤ 0.05 by Fisher’s exact test performed for this review) (see Table B-2). Stomachs were not examined microscopically in the reproductive subgroup. No other histological findings were reported. No effects were reported on mating or fertility, and no effects on offspring were observed through PND 4 in the reproductive subgroup.
A reproductive/developmental no-observed-adverse-effect level (NOAEL) of 1,500 mg DAP/kg-day (the highest dose tested) was identified by ECHA (2002) and OECD (2007b); a lowest-observed-adverse-effect level (LOAEL) could not be determined. OECD (2007f) identified a systemic NOAEL of 250 mg DAP/kg-day and a LOAEL of 750 mg DAP/kg-day for this study based on degenerative changes in the stomach,3 noting that the incidences of histologic changes in the stomach were not statistically significant in males or females at the low dose. ECHA (2002) identified the same effect levels, but based the LOAEL on dental banding, which was of questionable biological relevance; ECHA (2002) attributed the stomach effects to local irritation rather than systemic toxicity and did not consider this local effect as a potential basis for the LOAEL. Based on the significant increase in the incidence of stomach lesions in male and female rats (combined) at all doses in the study, the LOAEL determined for this review is 250 mg DAP/kg-day; a NOAEL could not be determined. As noted above, the calcium and baseline phosphate contents of the feed administered in this study were not reported. Although the ratio of calcium to phosphate can be an important determinant of phosphate toxicity in mammals, there were no indications of excess phosphate intake (e.g., laxative or renal effects) in the animals, and inadequate calcium intake is not considered a plausible cause of stomach inflammation in the current study with ammonium phosphate. The critical effects observed in the principal study (contact irritant effects in the stomach) might be attributable to the ammonium anion, since damage to the gastrointestinal mucosa has been observed in rats after oral exposure to other ammonium compounds (ammonium hydroxide, ammonium chloride, and ammonia) [reviewed by ATSDR (2004)]. Gavage bolus dosing also would have delivered a high, instantaneous local exposure to the stomach, which may have contributed to the observed local effects. Local stomach irritation (although more extensive, including submucosal inflammation, epithelial hyperplasia, acantholysis, increased numbers of mucous secreting cells) was also observed at all (identical) doses in another GLP-compliant, OECD 422 guideline study conducted also by gavage with granular triple superphosphate (GTSP; composed of calcium and phosphate) in rats. The effects were attributed to irritation along with the low pH (2−3) of that test substance (OECD, 2007c). Finally, it is possible that dental bands and/or increased serum ALP observed in Huntingdon (2002) [as cited in OECD (2007b), OECD (2007f), and ECHA (2002)] could be related to higher phosphate intake, but these effects occurred at higher doses (≥750 mg/kg-day) than the stomach inflammation.
Chronic Studies
Fazekas (1954) as cited in Weiner et al. (2001)
In a study published in German and summarized in a review by Weiner et al. (2001), 10 female rabbits (strain not specified) were exposed to DAP in drinking water for 5−16 months. The review by Weiner et al. (2001) reported the doses as 300−700 mg/kg-day and indicated that parathyroid gland weight was the only toxicological endpoint assessed in the study. According to Weiner et al. (2001), the mean parathyroid weight was increased by 235% compared to controls. No further information was provided in the secondary source. Effect levels could not be determined because of the limited toxicological evaluations and limited study details provided in the secondary source.
Reproductive/Developmental Studies
The study by Huntingdon (2002) as cited in OECD (2007b) and ECHA (2002) included a screening analysis for reproductive and developmental effects (reproductive subgroup). Details and results are described above in the “Subchronic Studies” section. As reported there, a reproductive/developmental NOAEL of 1,500 mg DAP/kg-day (the highest dose tested) is identified for this study.
2.2.2. Inhalation Exposures
No repeated-dose studies of animals exposed to MAP, DAP, or APP by inhalation have been identified in the literature searches or secondary sources reviewed.
2.3. OTHER DATA (SHORT-TERM TESTS, OTHER EXAMINATIONS)
Table 4 provides an overview of genotoxicity studies of DAP and MAP.
Table 4
Summary of Ammonium Phosphate Salts (MAP, CASRN 7722-76-1; DAP, CASRN 7783-28-0; and APP, CASRN 68333-79-9) Genotoxicity.
2.3.1. Genotoxicity
Data pertaining to the genotoxic activity of ammonium phosphate salts are very limited, and the only studies available are unpublished studies reported in secondary sources, although OECD and ECHA peer reviewed these primary studies. DAP was not mutagenic to Salmonella typhimurium or Escherichia coli with or without metabolic activation [Wagner and Klug (2001) as cited in OECD (2007b) and ECHA (2001a)] and did not increase chromosomal aberrations (CAs) in Chinese hamster ovary (CHO) cells in vitro, with or without metabolic activation [Gudi and Brown (2001) as cited in OECD (2007b) and ECHA (2001b)]. MAP was not mutagenic to mouse L5178Y/TK+/− lymphoma cells with or without metabolic activation (ECHA, 2010a).
2.3.2. Other Animal Studies
Ammonium phosphate salts exhibit low acute lethal potential based on unpublished data in peer-reviewed secondary sources. Unpublished acute lethality limit tests performed according to OECD Guideline 405 (OECD, 2021) were reported in OECD (2007a), OECD (2007b), and OECD (2007d). The oral median lethal dose (LD50) values determined in rats were >2,000 mg/kg for MAP, DAP, and APP, and no clinical signs or body-weight changes were reported [Merkel (2000) as cited in OECD (2007a), OECD (2007b), and OECD (2007d)]. In a review, Weiner et al. (2001) reported the following oral LD50 values for rats: >1,000 and 5,750 mg/kg for MAP; >1,000, 6,500, and >25,100 mg/kg for DAP; and >2,000 mg/kg for APP, as well as the following dermal LD50 values for rabbits: >7,940 mg/kg (MAP), >10,000 mg/kg (DAP), and >2,000 mg/kg (APP), citing unpublished studies by Stauffer, Solutia, and Albright and Wilson. A 4-hour inhalation median lethal concentration (LC50) value of >5.09 mg/L for APP was noted in the same review (Weiner et al., 2001). No details of study design or results, and no information on clinical signs, body-weight changes, or necropsy findings were reported by Weiner et al. (2001).
In an acute lethality study of the ammonium phosphate fire retardant PHOS-CHEK 259-F (>90% DAP and <5% guar gum according to material safety data sheet; other ingredients not reported) (ICL, 2015) in male and female rats, gavage doses of 2,000, 2,520, 3,175, and 4,000 mg/kg resulted in the following mortality incidences: 9/10, 1/10, 8/10, and 7/10. However, no LD50 values could be estimated from these data (Monsanto, 1992). Clinical signs of sedation, ataxia, and ptosis, as well as gastrointestinal distress, were observed, and necropsy showed gastrointestinal distension and darkened stomachs. Monsanto (1992) estimated an LD50 of >5,000 mg/kg in a rabbit dermal lethality study of PHOS-CHEK 259-F. In the dermal study, body-weight loss was noted in 7/10 rabbits, erythema and edema were observed, and at necropsy, there was a loss of body fat (10/10 rabbits), as well as hepatic, renal, and splenic abnormalities; in addition, enlarged gall bladder was observed in 2/5 males. Other LD50 values for ammonium phosphate fire retardants were reported; however, these products (PHOS-CHEK XAF and PHOS-CHEK 75-D) are of low or unknown ammonium phosphate composition. No composition information was located for PHOS-CHEK XAF, while PHOS-CHEK 75-D is reportedly composed of >65% diammonium sulfate, >5% DAP, and >15% MAP (ICL, 2006); thus, these LD50 values, all higher than those reported above, are not reported here.
Following studies showing that other phosphates exhibited a cariostatic effect, DAP was tested for prevention of dental caries (cavities) in white rats (sex and strain not reported) (McClure, 1964). DAP administered in the diet at concentrations between 0.55 and 3.33% for 60−90 days reduced the incidence of rats with caries, the numbers of carious teeth per rat, and the caries severity score per rat. No other toxicological endpoints were evaluated. In a study reported only in abstract form, Ivy et al. (1974) observed lower body weight and percent femur ash in rats administered DAP in the diet (at levels equivalent to 0.5, 0.7, or 0.9% phosphorus) for 70 days when compared with rats exposed to sodium phosphate, which provided equivalent concentrations of phosphorus. Finally, turkeys given DAP in the diet for 8 weeks exhibited similar tibia breaking strength when compared with those administered other dietary phosphate sources with varying fluorine content (Struwe and Sullivan, 1975).
Clawson and Armstrong (1981) administered APP (replacing 0, 50, or 100% phosphorus in diet, in place of defluorinated rock phosphate) to groups of seven rats (three males and four females) for 4 weeks and observed increased food intake and weight gain, although the changes were not monotonic with exposure. The study authors did not evaluate other toxicological endpoints. Other studies with APP are limited to evaluations of growth in agricultural species, in which APP was tested as a source of nutritional nitrogen and phosphorus. Administration of APP did not affect growth or feed consumption in pigs when compared with other supplements, such as dicalcium phosphate (Tunmire et al., 1983; Clawson and Armstrong, 1981; Kornegay, 1972). In cows, Colenbrander et al. (1971) found that addition of APP in the diet for 8 weeks increased growth and plasma phosphorus while decreasing urinary pH. In sheep and lambs, addition of APP to the diet or in drinking water for 1−13 weeks likewise resulted in higher body-weight gains, blood phosphorus concentrations, and retention of phosphorus (Koolivand et al., 2019;Hemingway and Fishwick, 1975; Fishwick and Hemingway, 1974). In 3-week experiments in chickens, supplementation with APP in the drinking water resulted in increased food consumption, growth, phosphorus intake, and phosphorus concentration in tibia ash compared with controls (Damron and Flunker, 1990; Jensen and Edwards, 1980).
The ammonium phosphates (APP, MAP, and DAP), as well as PHOS-CHEK 259-F, were considered either nonirritating or slightly to mildly irritating when applied to the skin or eyes in tests conducted in rabbits (Weiner et al., 2001; Monsanto, 1992; Aoyama, 1975). DAP was also determined to be nonsensitizing by the dermal route (ECHA, 2010b).
2.3.3. Metabolism/Toxicokinetic Studies
Gastrointestinal absorption of phosphate from DAP has been studied in dogs. Summerill and Lee (1985) administered DAP (15 mmol in two doses 2 hours apart) by stomach tube to eight mongrel dogs and measured plasma and urinary phosphate levels for up to 4 hours. Plasma phosphate levels were increased in exposed dogs (1.63 and 1.91 mmol/L at 1.5−2 and 3.5−4 hours, respectively, compared with 0.88 and 0.94 mmol/L in four control animals), while creatinine clearance was unchanged. The study authors estimated phosphate absorption to be about 50%, based on plasma phosphate levels obtained in the 4 hours after the first dose and the assumption that phosphate was distributed evenly throughout the extracellular fluid.
2.3.4. Mode-of-Action/Mechanistic Studies
An in vitro study used neuro-derived cell lines (PC12 pheochromocytoma and B35 neuroblastoma) to evaluate the neurotoxic potential of several fire retardants, including APP (Hendriks et al., 2014). Exposure to concentrations up to 1,300 μM APP was not cytotoxic to PC12 cells, but cytotoxicity was observed in B35 cells at concentrations of at least 13 μM. Hendriks et al. (2014) reported that APP concentrations of 7 or 700 μM increased reactive oxygen species production in B35 and PC12 cells but noted that the results may have been confounded by interaction of the compound with the fluorescent dye used in the assay. APP did not affect basal levels of intracellular calcium in either cell type but did inhibit the depolarization-evoked rise in intracellular calcium concentration. Finally, APP reportedly exhibited antagonistic effects on human nicotinic acetylcholine receptor at ≥1,300 μM (Hendriks et al., 2014). The study authors concluded that APP exhibited low neurotoxic potential based on the in vitro results.
A number of other in vitro studies were identified in which ammonium phosphate was used as a phosphate source to evaluate mechanisms of changing membrane porosity during mitochondrial swelling (Sitaramam and Rao, 1992; Stoner and Sirak, 1978; Hommes et al., 1975; Lundberg, 1975; Chateaubodeau et al., 1974; Stoner and Sirak, 1971). The relevance of these studies to mechanisms of toxicity for ammonium phosphate compounds is uncertain.
Footnotes
- 2
Despite the death, histopathology incidence data provided in OECD (2007b), OECD (2007f), and ECHA (2002) are reported for five females in this group, suggesting that the animal that died prematurely was included in the results.
- 3
The OECD (2007f) study for phosphates also reported degenerative changes in the kidneys as a basis for the LOAEL; however, no evidence of kidney effects was reported in the OECD (2007b) robust summary or in the ECHA (2002) summary of the study.
- REVIEW OF POTENTIALLY RELEVANT DATA (NONCANCER AND CANCER) - Provisional Peer-Re...REVIEW OF POTENTIALLY RELEVANT DATA (NONCANCER AND CANCER) - Provisional Peer-Reviewed Toxicity Values for Ammonium Salts of Inorganic Phosphates: Monoammonium Phosphate (MAP) (CASRN 7722-76-1) Diammonium Phosphate (DAP) (CASRN 7783-28-0)
Your browsing activity is empty.
Activity recording is turned off.
See more...