NCBI Bookshelf. A service of the National Library of Medicine, National Institutes of Health.
Elkouby YM, Frank D. Wnt/β-Catenin Signaling in Vertebrate Posterior Neural Development. San Rafael (CA): Morgan & Claypool Life Sciences; 2010.
In this chapter, we will focus on the Wnt activity that induces hindbrain formation, with an emphasis on the induction of downstream transcription factors specifically mediating this process. We will also discuss the interactions and hierarchy of the Wnt/β-catenin pathway with other signaling molecules and attempt to integrate the accumulated data into a gene regulatory network that drives hindbrain development downstream of Wnt signaling.
Paralogous group 1 (PG1) Hox proteins are key factors controlling early hindbrain specification. The PG1 Hox proteins are homologues of the Drosophila Labial gene and include HoxA1, HoxB1, and HoxD1 proteins. Neural expression of the PG1 hox genes precedes all other hox genes. These genes exhibit a very similar expression pattern in the presumptive hindbrain region of zebrafish, Xenopus, chick and mouse embryos, as early as gastrula stages, which persists through neurula stages (Frohman et al., 1990; Frohman and Martin, 1992; Godsave et al., 1994; Kolm and Sive, 1995; McClintock et al., 2002; McNulty et al., 2005; Murphy and Hill, 1991; Rossel and Capecchi, 1999; Sundin et al., 1990; Wilkinson et al., 1989). PG1 genes are essential for hindbrain determination. Combined knockdown of HoxB1a and HoxB1b in zebrafish, and double HoxA1 and HoxB1 null mouse embryos significantly reduce or completely lose rhombomeres (r) 4 and 5 (McClintock et al., 2002; Rossel and Capecchi, 1999). In Xenopus embryos, triple knockdown of all PG1 genes HoxA1, HoxB1, and HoxD1 results in a complete loss of r2–6, and the entire hindbrain resembled the Hox nonexpressing r1 region (McNulty et al., 2005). This phenotype strongly resembles the loss-of-function of the hox co-factor Pbx2 in zebrafish embryos (Waskiewicz et al., 2002). These various PG1 loss-of-function phenotypes were hindbrain specific, and spinal cord formation was not perturbed in these embryos. The combined PG1 loss-of-function phenotype was synergistically stronger than that of each of the individual inhibitions, and the Xenopus PG1 knockdown could be rescued by overexpression of HoxD1 alone, thus arguing for at least partial redundancy between the PG1 members (McNulty et al., 2005).
As discussed previously, Wnt/β-catenin signaling is obligatory for expression of most hindbrain and spinal cord hox genes during neural plate specification in vertebrates, including the PG1 Hox proteins. However, further examination of the initial hindbrain induction step, during gastrula stages, PG1 hox genes were shown in Xenopus to be direct targets of the pathway (Elkouby et al., 2010; In der Rieden et al., 2010). Using an inducible constitutively activated Tcf protein, HoxA1, HoxB1, and HoxD1 expression were induced in both embryos and AC explants at mid-gastrula stages, when TCF activity was induced at early gastrula stages. This induction persisted in the presence of cyclohexamide, suggesting that TCF directly binds these promoters and does not require de novo synthesized intermediate transcription factors. Thus, Wnt/β-catenin signaling directly activates the earliest expression of the specific hindbrain-promoting PG1 Hox genes during gastrula stages. Other, later, hindbrain Hox genes are indirectly regulated by the Wnt pathway (McNulty et al., 2005). Because the PG1 proteins are required for the proper sequential expression of these later hindbrain hox genes (Dibner et al., 2004; McNulty et al., 2005), their direct induction by the Wnt/β-catenin pathway seems to constitute one of the earliest steps of the hindbrain developmental program, positioning Wnt signaling high up in this hierarchy.
Gbx2 is a homeoprotein expressed as early as gastrula stages in the presumptive anterior hindbrain region of vertebrates embryos (Gbx1 in zebrafish) (Rhinn et al., 2009; Shamim and Mason, 1998; von Bubnoff et al., 1996; Wassarman et al., 1997). As discussed previously (see Chapter 4, “Induction of the Midbrain–Hindbrain Border”), Gbx2 protein acts as a key player in formation of the MHB region and also specifies the anterior hindbrain. Gbx2–/– mouse embryos do not lose the entire hindbrain region, but specifically fail to form r1–3, and this region is transformed to midbrain fates (Wassarman et al., 1997). Such a role in anterior hindbrain specification was also demonstrated for Gbx1 in zebrafish embryos (Rhinn et al., 2009). Overexpression of Gbx1 phenocopied the overexpression of Wnt8, resulting in caudalized zebrafish embryos that lacked forebrain structures. However, while it shifted A–P levels of gene expression to the anterior, Gbx1 robustly expanded the r3 domain of Krox20 expression, while repressing that of Otx2 at neurula stages. In a complementary manner, expression of HoxA1 and HoxB1 was not eliminated in Gbx1 morphant embryos at gastrula stages, but their anterior domains of expression were missing, and Otx2 expression was spread caudally, filling this gap. Thus, Gbx proteins seem to play a specific role in the development of the anterior hindbrain in vertebrates. Moreover, overexpression of Gbx1 was able to rescue the Wnt8 morphant phenotype, repressing expanded Otx2 expression, while restoring and expanding the inhibited Krox20 expression. In Xenopus embryos, Gbx2 expression is directly regulated by the Wnt/β-catenin pathway, having a Tcf/Lef site in its promoter (Li et al., 2009). Inducible β-catenin activated Gbx2 expression in the presence of cyclohexamide. Moreover, both β-catenin binding and the requirement of this site for promoter activity were confirmed by ChIP and transgenic promoter reporter gene analyses, respectively (Li et al., 2009). In contrast, activation of Gbx1 expression by Wnt8 in zebrafish gastrulae required FGF signaling (Rhinn et al., 2005). One possibility that may reconcile these observations is that FGF signaling may provide cells with the competence to respond to the Wnt signal. However, because β-catenin activated Xenopus Gbx2 expression in the absence of protein synthesis, such an effect by FGF might be achieved by posttranslational modification. In this scenario, Gbx could also be a direct target of FGF signaling, and this remains to be determined. Alternatively, this gap could simply arise from differences between Gbx1 and Gbx2 genes or between the two species. Either way, during the early induction of the hindbrain, Wnt/β-catenin signaling induces expression of the Gbx1/2 genes, which then mediates the specification of the rostral r1–3 of the hindbrain.
Meis3, a TALE family homeoprotein, is essential for hindbrain formation in both Xenopus and zebrafish and is expressed in the presumptive hindbrain region as early as gastrula stages (Dibner et al., 2001; Salzberg et al., 1999; Vlachakis et al., 2001; Waskiewicz et al., 2001). Loss of Meis3 function by either a dominant negative or anti-morph proteins, or MO knockdown, results in the similar loss of the entire hindbrain region, accompanied by the parallel loss in expression of a plethora of hindbrain markers, including Hox genes of PGs1–4, with a concomitant posterior expansion of anterior forebrain markers and enlarged forebrain structures (Dibner et al., 2001; Dibner et al., 2004; Elkouby et al., 2010; Gutkovich et al., 2010; Salzberg et al., 1999; Vlachakis et al., 2001; Waskiewicz et al., 2001). Meis3 is specifically required for hindbrain formation because spinal cord development is not perturbed in Meis3 morphant embryos. Meis3 also suffices for hindbrain induction, as its overexpression induces ectopic hindbrain formation while repressing forebrain formation as shown by both embryo morphology and marker gene expression in both embryos and explants (Dibner et al., 2001; Dibner et al., 2004; Elkouby et al., 2010; Gutkovich et al., 2010; Salzberg et al., 1999; Vlachakis et al., 2001). In addition, Meis3 synergistically interacts with HoxB1b to induce expression of hindbrain markers such as HoxB1a and Krox20 in zebrafish embryos (Vlachakis et al., 2001). Moreover, HoxD1 is a direct target of Meis3 in Xenopus, which acts downstream of it and is essential for its posterior neural cell fate–inducing activity (Dibner et al., 2004; Gutkovich et al., 2010).
Phenotypes of either loss- or gain-of-function of both Meis3 and Wnt/β-catenin signaling are strikingly similar, and recently, Meis3 was shown to be a direct target gene of the pathway, which is crucial for mediating its caudalizing activity (Elkouby et al., 2010). Wnt3a was identified in this study as the Wnt ligand initially inducing the hindbrain during gastrula stages (see Chapter 12, “The Role of Mesoderm and Specific Wnt Ligands in Neural Patterning”). Wnt3a-Induced Wnt/β-catenin signaling is required for Meis3 expression, as both Wnt3a-MO and overexpression of Dkk1 completely prevented the onset of its expression (Figure 3.2A). In addition, overexpression of either CMV-Wnt3a or constitutive TCF (activated at the onset of gastrulation) strongly induced ectopic Meis3 expression in both embryos and explants. Activated-TCF also induced Meis3 expression in the presence of cyclohexamide, and two Tcf/Lef sites are found in Meis3 promoter. ChIP analysis showed β-catenin binding to this region, and transgenic promoter reporter analysis confirmed the requirement of these two sites for promoter activity in vivo. Furthermore, Meis3 acts downstream of Wnt/β-catenin signaling. Wnt3a MO and Dkk1 overexpression eliminate hindbrain, primary neuron, and NC types (Figure 3.2C). On these backgrounds, Meis3 could fully rescue specification of these posterior cell fates. However, reciprocally, Wnt3a could not rescue these very same cell fates on the Meis3 morphant background. Moreover, in neuralized AC explants, Wnt3a absolutely required functional Meis3 to exert its effects. Thus, Meis3 is required downstream of the initial Wnt3a signal to induce the hindbrain region.
The identification of Meis3 as a mediator of the caudalizing morphogenic activity of the Wnt/β-catenin pathway reveals the hierarchy of the initial hindbrain inducing events and allows the construction of a gene regulatory network controlling this process. Placing Meis3 high in this hierarchy is the observation that Meis3 is required for the expression of the PG1 Hox and Gbx2 genes (Dibner et al., 2004; Elkouby et al., 2010; Gutkovich et al., 2010). Although both the PG1 and Gbx2 genes were shown to be direct targets of the Wnt/β-catenin pathway, Wnt3a-mediated induction of these earliest hindbrain-promoting factors was blocked when Meis3 was inhibited (Elkouby et al., 2010). Different factors may simultaneously converge on the same promoter early during this complicated process, and Meis3 and the Wnt/β-catenin pathway may cooperate in such a way. Indeed, HoxD1 is a shared direct target of Meis3, the Wnt/β-catenin pathway, and RA signaling (Dibner et al., 2004; Elkouby et al., 2010; In der Rieden et al., 2010; Kolm and Sive, 1997). Thus, Wnt/β-catenin signaling may induce expression of genes like Meis3, with which it then subsequently interacts with to induce more downstream targets, such as HoxD1.
The hierarchy of Wnt/β-catenin, FGF, and RA signaling during hindbrain formation is also clarified. In Xenopus, zebrafish, and chick embryos, FGF signaling was shown to act downstream of the Wnt signal (Domingos et al., 2001; Nordstrom et al., 2006; Rhinn et al., 2005). Meis3 protein directly activates FGF3 and FGF8 gene expression (Aamar and Frank, 2004; Gutkovich et al., 2010), and Meis3 protein cannot induce posterior cell fates in the absence of downstream FGF/MAPK signaling (Aamar and Frank, 2004; Ribisi et al., 2000). Like in the case of the PG1 Hox genes, FGF3 and FGF8 expression in Wnt-caudalized explants is also dependent on Meis3 protein activity (Elkouby et al., 2010). In addition, RA signaling also acts downstream of Wnt in chick hindbrain formation (Nordstrom et al., 2006). Furthermore, in Xenopus, RA signaling interacts with Meis3 activity in optimizing specific Hox gene expression and so inducing specific rhombomers formation, thus fine-tuning later hindbrain patterning (Dibner et al., 2004). Therefore, Meis3 seems to act as a connector between upstream Wnt/β-catenin signaling, downstream FGF signaling, and parallel RA signaling in the early induction of the hindbrain region and its subsequent patterning. In this core gene regulatory network (Figure 5.1) Wnt/β-catenin signaling directly activates Meis3 expression, which in turn directly activates expression of FGF3, FGF8 and the PG1 gene HoxD1. PG1 Hox genes regulate expression of PG1, 2, 3 and 4 Hox genes to eventually form the hindbrain. Meis3 also cooperates with RA signaling to induce specific Hox gene expression within the hindbrain, in addition to regulating Gbx2 expression that controls anterior hindbrain development. PG1 and Gbx2 expression is also dependent on direct activation by the Wnt/β-catenin pathway, however, as it requires Meis3 activity downstream of it, this could possibly reflect another later Wnt activity further downstream of Meis3, or acting in parallel to it.
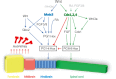
FIGURE 5.1
Gene regulatory networks (GRNs) controlling hindbrain and spinal cord development activated by the Wnt/β-catenin pathway. The Wnt/β-catenin pathway activates two GRNs controlling hindbrain (blue) and spinal cord (green) development. In (more...)
- Induction of the Hindbrain - Wnt/β-Catenin Signaling in Vertebrate Posterior Neu...Induction of the Hindbrain - Wnt/β-Catenin Signaling in Vertebrate Posterior Neural Development
- Induction of the Midbrain–Hindbrain Border - Wnt/β-Catenin Signaling in Vertebra...Induction of the Midbrain–Hindbrain Border - Wnt/β-Catenin Signaling in Vertebrate Posterior Neural Development
- Downstream of Wnt: Hindbrain or Spinal Cord? - Wnt/β-Catenin Signaling in Verteb...Downstream of Wnt: Hindbrain or Spinal Cord? - Wnt/β-Catenin Signaling in Vertebrate Posterior Neural Development
- Homo sapiens peptidoglycan recognition protein 3, mRNA (cDNA clone MGC:97229 IMA...Homo sapiens peptidoglycan recognition protein 3, mRNA (cDNA clone MGC:97229 IMAGE:7262478), complete cdsgi|47479714|gb|BC069741.1|Nucleotide
Your browsing activity is empty.
Activity recording is turned off.
See more...