By agreement with the publisher, this book is accessible by the search feature, but cannot be browsed.
NCBI Bookshelf. A service of the National Library of Medicine, National Institutes of Health.
Siegel GJ, Agranoff BW, Albers RW, et al., editors. Basic Neurochemistry: Molecular, Cellular and Medical Aspects. 6th edition. Philadelphia: Lippincott-Raven; 1999.
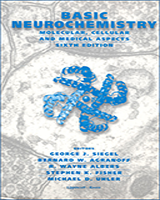
Basic Neurochemistry: Molecular, Cellular and Medical Aspects. 6th edition.
Show detailsAn uninterrupted supply of oxygen is vital for cerebral function
Although it is possible to metabolize glucose to lactate and supply some energy needs as ATP, this represents only a small fraction of that resulting from oxidative metabolism, as can be seen by comparing the following reactions:
Thus, anaerobic glycolysis cannot sustain the energy requirements of adult brain for more than a few minutes (see Chap. 31). Normal human brain consumes 3.3 ml of oxygen per 100 g of brain per minute (CMRO2), a value which remains relatively stable during periods of wakefulness and sleep and represents ~20% of total body resting oxygen consumption despite the fact that the brain represents only ~2% of body weight. With impaired cerebral function, CMRO2 declines in parallel with the severity of cerebral depression.
Experimental studies demonstrate that cerebral energy metabolism remains normal when mild to moderate hypoxia (PaO2 = 25–40 mm Hg) results in severe cognitive dysfunction in human volunteers [1,2,8]. In mild hypoxia, cerebral blood flow (CBF) increases in order to maintain oxygen delivery to the brain. However, CBF can increase only about twofold; beyond this, CMRO2 starts to fall and symptoms of hypoxia occur. In normal individuals exposed acutely to high altitudes (Table 38-2), difficulties with complex learning tasks and short-term memory appear at altitudes of around 10,000 feet as PaO2 falls below 45 mm Hg, and above 20,000 feet, where PaO2 = 30 mm Hg, cognitive disturbances and problems with motor coordination start to appear. Acute hypoxia of less than PaO2 = 20 mm Hg generally results in coma. Graded hypoxia in experimental animals reveals that brain ATP levels remain normal at PaO2 = 20 to 25 mm Hg (Fig. 38-3) despite EEG slowing and attenuated sensory evoked responses.
Table 38-2
Hypoxic Thresholds for CNS Dysfunctiona.

Figure 38-3
Brain concentrations of phosphocreatine (PCr) and ATP in rats anesthetized with 70% N2O and subjected to graded hypoxia for periods of 15 to 30 min. , Animals with ≤120 mm Hg mean arterial pressure;
, animals with >80 mm Hg mean arterial (more...)
Glycolysis is stimulated to maintain brain ATP levels in moderate hypoxia
Studies on experimental animals reveal that at PaO2 = 50 mm Hg an oxygen tension well in excess of that necessary to cause impaired ATP synthesis (Fig. 38-3), an increase in the [lactate]/[pyruvate] ratio and a decrease in brain tissue pH are observed. Increased lactate production is accompanied by a rise in CMRglc [8], indicating accelerated glycolysis. The cytosolic redox potential measured by the NADH:NAD+ ratio is shifted toward a more reduced state at PaO2 below 45 mm Hg. PCr concentrations start to decline (Fig. 38-3), falling precipitously below a PaO2 of 20 mm Hg. This steep fall in PCr coincides with a decrease in ATP and reflects failure of mitochondrial respiration and oxidative phosphorylation.
All forms of hypoxia result in alterations of neurotransmitter synthesis and release
Hypoxic hypoxia is a condition in which insufficient oxygen reaches the blood; this may result from either low oxygen tension in the environment, as encountered at high altitudes (Table 38-2), or the inability of oxygen to reach and cross the alveolar capillary membrane, as encountered in pulmonary disease.
Decreasing the oxygen content in the inspired air of anesthetized rats from 30 to 10% reduces the PaO2 from 120 to 42 mm Hg; arteriovenous differences for oxygen decrease by 25%, but this is offset by an increase in CBF so that the CMRO2 is unchanged. However, below 15% oxygen, glycolysis in brain is stimulated, leading to increased cerebral glucose utilization [8] and increased brain lactate. Neurotransmitter synthesis in brain is exquisitely sensitive to hypoxia. When rats are made to breathe 10% oxygen, ACh synthesis is decreased by 68%. This means that ACh synthesis in the brain is decreased during hypoxia when CMRO2 is maintained at normal values [9]. Hypoxia of a similar magnitude also results in altered synthesis and release of other neurotransmitters, including those derived from glucose. Arterial oxygen tension of below 50 mm Hg results in reductions in synthesis of glutamate and GABA in brain [10]. Synthesis of the biogenic amines may also be susceptible to hypoxia since tyrosine hydroxylase and tryptophan hydroxylase, the rate-limiting enzymes in monoamine neurotransmitter synthesis, require molecular oxygen, for which the Km (O2) is 12 μM, equivalent to 7 mm Hg [10].
Anemic hypoxia results when sufficient oxygen reaches the blood but the quantity of hemoglobin needed to bind and transport it is decreased. Either low hemoglobin due to anemia or chemical modifications of hemoglobin, for example, in carbon monoxide poisoning, may result in anemic hypoxia. Anemic hypoxia resulting from treatment of rats with sodium nitrite decreases synthesis of ACh, glutamate, GABA and the biogenic amines. Decreased neurotransmitter synthesis occurs at degrees of hypoxia which do not result in decreases in brain concentrations of ATP or adenylate energy charge [4,10].
Histotoxic hypoxia occurs when the ability of the tissue to utilize oxygen is impaired due to, for example, cyanide poisoning, which interferes with oxidative phosphorylation. Treatment of rats with potassium cyanide leads to reductions in synthesis of ACh and of glucose-derived amino acids in the brain [10].
In addition to decreased synthesis of neurotransmitters, hypoxia results in alterations in their release. In striatal slices, hypoxia increases the evoked release of glutamate, DA and, to a lesser extent, ACh. The effects of hypoxia on neurotransmitter release are mediated by cytosolic free Ca2+ or on neurotransmitter release (see Chap. 5).
- Hypoxic Encephalopathy - Basic NeurochemistryHypoxic Encephalopathy - Basic Neurochemistry
Your browsing activity is empty.
Activity recording is turned off.
See more...