By agreement with the publisher, this book is accessible by the search feature, but cannot be browsed.
NCBI Bookshelf. A service of the National Library of Medicine, National Institutes of Health.
Gilbert SF. Developmental Biology. 6th edition. Sunderland (MA): Sinauer Associates; 2000.
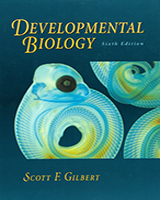
Developmental Biology. 6th edition.
Show detailsOrgans are complex structures composed of numerous types of tissues. In the vertebrate eye, for example, light is transmitted through the transparent corneal tissue and focused by the lens tissue (the diameter of which is controlled by muscle tissue), eventually impinging on the tissue of the neural retina. The precise arrangement of tissues in this organ cannot be disturbed without impairing its function. Such coordination in the construction of organs is accomplished by one group of cells changing the behavior of an adjacent set of cells, thereby causing them to change their shape, mitotic rate, or fate. This kind of interaction at close range between two or more cells or tissues of different history and properties is called proximate interaction, or induction*. There are at least two components to every inductive interaction. The first component is the inducer: the tissue that produces a signal (or signals) that changes the cellular behavior of the other tissue. The second component, the tissue being induced, is the responder.
Not all tissues can respond to the signal being produced by the inducer. For instance, if the optic vesicle (presumptive retina) of Xenopus laevis is placed in an ectopic location (i.e., in a different place from where it normally forms) underneath the head ectoderm, it will induce that ectoderm to form lens tissue. Only the optic vesicle appears to be able to do this; therefore, it is an inducer. However, if the optic vesicle is placed beneath ectoderm in the flank or abdomen of the same organism, that ectoderm will not be able to respond. Only the head ectoderm is competent to respond to the signals from the optic vesicle by producing a lens† (Figure 6.1; Saha et al. 1989; Grainger 1992).

Figure 6.1
Ectodermal competence and the ability to respond to the optic vesicle inducer in Xenopus. (1) The optic vesicle is able to induce lenses in the anterior portion of the ectoderm, but not in the presumptive trunk and abdomen (2). If the optic vesicle is (more...)
This ability to respond to a specific inductive signal is called competence (Waddington 1940). Competence is not a passive state, but an actively acquired condition. For example, in the developing chick and mammalian eye, the Pax6 protein appears to be important in making the ectoderm competent to respond to the inductive signal from the optic vesicle. Pax6 expression is seen in the head ectoderm, which can respond to the optic vesicle by forming lenses, and it is not seen in other regions of the surface ectoderm (See Figure 4.16 ; Li et al. 1994). Moreover, the importance of Pax6 as a competence factor was demonstrated by recombination experiments using embryonic rat eye tissue (Fujiwara et al. 1994). The homozygous Pax6-mutant rat has a phenotype similar to the homozygous Pax6-mutant mouse (see Chapter 4), lacking eyes and nose. It has been shown that part of this phenotype is due to the failure of lens induction (Figure 6.2). But which is the defective component—the optic vesicle or the surface ectoderm? When head ectoderm from Pax6-mutant rat embryos was combined with a wild-type optic vesicle, no lenses were formed. However, when the head ectoderm from wild-type rat embryos was combined with a Pax6-mutant optic vesicle, lenses formed normally (Figure 6.3). Therefore, Pax6 is needed for the surface ectoderm to respond to the inductive signal from the optic vesicle. The inducing tissue does not need it. It is not known how Pax6 becomes expressed in the anterior ectoderm of the embryo, although it is thought that its expression is induced by the anterior regions of the neural plate. Competence to respond to the optic vesicle inducer can be conferred on ectodermal tissue by incubating it next to anterior neural plate tissue (Henry and Grainger 1990; Li et al. 1994; Zygar et al. 1998).

Figure 6.2
Induction of optic and nasal structures by Pax6 in the rat embryo. (A, B) Histology of wild-type (A) and homozygous Pax6 mutant (B) embryos at day 12 of gestation shows induction of lenses and retinal development in the wild-type embryo, but neither lens (more...)

Figure 6.3
Recombination experiments showing that the induction deficiency of Pax6-deficient rats is caused by the inability of the surface ectoderm to respond to the optic vesicle. (Photographs courtesy of M. Fujiwara.)
Thus, there is no single inducer of the lens. Studies on amphibians suggest that the first inducers may be the pharyngeal endoderm and heart-forming mesoderm that underlie the lens-forming ectoderm during the early- and mid-gastrula stages (Jacobson 1963, 1966). The anterior neural plate may produce the next signals, including a signal that promotes the synthesis of Pax6 in the anterior ectoderm (Zygar et al. 1998; Figure 6.4). Thus, the optic vesicle appears to be the inducer, but the anterior ectoderm has already been induced by at least two other factors. (The situation is like that of the player who kicks the “winning goal” of a soccer match.) The optic vesicle appears to secrete two induction factors, one of which is BMP4 (see the discussion below), a protein that induces the transcription of the Sox2 and Sox3 transcription factors (and another, as yet unidentified, signal that induces the appearance of the l-Maf transcription factor; Ogino and Yasuda 1998). The combination of Pax6, Sox2, Sox3, and l-Maf ensures the production of the lens.

Figure 6.4
Lens induction in embryonic amphibians. (A) The additive effects of inducers, as shown by transplantation and extirpation (removal) experiments on the salamander Tarichosa torosa. The ability to produce lens tissue is first induced by pharyngeal endoderm, (more...)
Cascades of induction: Reciprocal and sequential inductive events
Another feature of induction is the reciprocal nature of many inductive interactions. Once the lens has formed, it can then induce other tissues. One of these responding tissues is the optic vesicle itself. Now the inducer becomes the induced. Under the influence of factors secreted by the lens, the optic vesicle becomes the optic cup, and the wall of the optic cup differentiates into two layers, the pigmented retina and the neural retina (Figure 6.5; Cvekl and Piatigorsky 1996). Such interactions are called reciprocal inductions.

Figure 6.5
Schematic diagram of the induction of the mouse lens. (A) At embryonic day 9, the optic vesicle extends toward the surface ectoderm from the forebrain. The lens placode (the prospective lens) appears as a local thickening of the surface ectoderm near (more...)
At the same time, the lens is also inducing the ectoderm above it to become the cornea. Like the lens-forming ectoderm, the cornea-forming ectoderm has achieved a particular competence to respond to inductive signals, in this case the signals from the lens (Meier 1977). Under the influence of the lens, the corneal ectodermal cells become columnar and secrete multiple layers of collagen. Mesenchymal cells from the neural crest use this collagen matrix to enter the area and secrete a set of proteins (including the enzyme hyaluronidase) that further differentiate the cornea. A third signal, the hormone thyroxine, dehydrates the tissue and makes it transparent (see Hay 1980; Bard 1990). Thus, there are sequential inductive events, and multiple causes for each induction.
Instructive and permissive interactions
Howard Holtzer (1968) distinguished two major modes of inductive interaction. In instructive interaction, a signal from the inducing cell is necessary for initiating new gene expression in the responding cell. Without the inducing cell, the responding cell would not be capable of differentiating in that particular way. For example, when the optic vesicle is experimentally placed under a new region of the head ectoderm and causes that region of the ectoderm to form a lens, that is an instructive interaction. Wessells (1977) has proposed three general principles characteristic of most instructive interactions:
- 1.
In the presence of tissue A, responding tissue B develops in a certain way.
- 2.
In the absence of tissue A, responding tissue B does not develop in that way.
- 3.
In the absence of tissue A, but in the presence of tissue C, tissue B does not develop in that way.
The second type of induction is permissive interaction. Here, the responding tissue contains all the potentials that are to be expressed, and needs only an environment that allows the expression of these traits.‡ For instance, many tissues need a solid substrate containing fibronectin or laminin in order to develop. The fibronectin or laminin does not alter the type of cell that is to be produced, but only enables what has been determined to be expressed.
Epithelial-mesenchymal interactions
Some of the best-studied cases of induction are those involving the interactions of sheets of epithelial cells with adjacent mesenchymal cells. These interactions are called epithelial-mesenchymal interactions. Epithelia are sheets or tubes of connected cells; they can originate from any germ layer. Mesenchyme refers to loosely packed, unconnected cells. Mesenchymal cells are derived from the mesoderm or neural crest. All organs consist of an epithelium and an associated mesenchyme, so epithelial-mesenchymal interactions are among the most important phenomena in nature. Some examples are listed in Table 6.1.
Table 6.1
Some epithelial-mesenchymal interactions.
Regional specificity of induction
Using the induction of cutaneous structures as our examples, we will look at the properties of epithelial-mesenchymal interactions. The first of these properties is the regional specificity of induction. Skin is composed of two main tissues: an outer epidermis, an epithelial tissue derived from ectoderm, and a dermis, a mesenchymal tissue derived from mesoderm. The chick epidermis signals the underlying dermal cells to form condensations (probably by secreting Sonic hedgehog and TGF-β2 proteins, which will be discussed below), and the condensed dermal mesenchyme responds by secreting factors that cause the epidermis to form regionally specific cutaneous structures (Figure 6.6; Nohno et al. 1995, Ting-Berreth and Chuong 1996). These structures can be the broad feathers of the wing, the narrow feathers of the thigh, or the scales and claws of the feet. Researchers can separate the embryonic epithelium and mesenchyme from each other and recombine them in different ways (Saunders et al. 1957). As Figure 6.7 demonstrates, the dermal mesenchyme is responsible for the regional specificity of induction in the competent epidermal epithelium. The same type of epithelium develops cutaneous structures according to the region from which the mesenchyme was taken. Here, the mesenchyme plays an instructive role, calling into play different sets of genes in the responding epithelial cells.

Figure 6.6
(A) Feather tracts on the dorsum of a day 9 chick embryo. Note that each feather primordium is located between the primordia of adjacent rows. (B) In situ hybridization of a day 10 chick embryo shows Sonic hedgehog expression (dark spots) in the ectoderm (more...)

Figure 6.7
Regional specificity of induction. When cells from different regions of the dermis (mesenchyme) are recombined with the epidermis (epithelium) in the chick, the type of cutaneous structure made by the epidermal epithelium is determined by the original (more...)
Genetic specificity of induction
The second property of epithelial-mesenchymal interactions is the genetic specificity of induction. Whereas the mesenchyme may instruct the epithelium as to what sets of genes to activate, the responding epithelium can comply with these instructions only so far as its genome permits. This property was discovered through experiments involving the transplantation of tissues from one species to another. In one of the most dramatic examples of interspecific induction, Hans Spemann and Oscar Schotté (1932) transplanted flank ectoderm from an early frog gastrula to the region of a newt gastrula destined to become parts of the mouth. Similarly, they placed presumptive flank ectodermal tissue from a newt gastrula into the presumptive oral regions of frog embryos. The structures of the mouth region differ greatly between salamander and frog larvae. The salamander larva has club-shaped balancers beneath its mouth, whereas the frog tadpole produces mucus-secreting glands and suckers (Figure 6.8). The frog tadpole also has a horny jaw without teeth, whereas the salamander has a set of calcareous teeth in its jaw. The larvae resulting from the transplants were chimeras. The salamander larvae had froglike mouths, and the frog tadpoles had salamander teeth and balancers. In other words, the mesodermal cells instructed the ectoderm to make a mouth, but the ectoderm responded by making the only kind of mouth it “knew” how to make, no matter how inappropriate.§

Figure 6.8
Genetic specificity of induction. Reciprocal transplantation between the presumptive oral ectoderm regions of salamander and frog gastrulae leads to newts with tadpole suckers and tadpoles with newt balancers. (After Hamburgh 1970.)
Thus, the instructions sent by the mesenchymal tissue can cross species barriers. Salamanders respond to frog signals, and chick tissue responds to mammalian inducers. The response of the epithelium, however, is species-specific. So, whereas organ type specificity (e.g., feather or claw) is usually controlled by the mesenchyme within a species, species specificity is usually controlled by the responding epithelium. As we will see in Chapters 21 and 22, large evolutionary changes can be brought about by changing the response to a particular inducer.
WEBSITE
6.1 Hen's teeth. Some inductive events between species can bring forth lost structures. Mouse molar mesenchyme may be able to induce teeth in the bird jaw. http://www.devbio.com/chap06/link0601.shtml
Footnotes
- *
Often, these inductions are called “secondary” inductions, whereas the tissue interactions that generate the neural tube are called “primary embryonic induction.” However, there is no difference in the molecular nature of “primary” and “secondary” inductions. Primary embryonic induction will be detailed separately in Chapters 10 and 11.
- †
When describing lens induction, one has to be careful to mention which species one is studying because there are numerous species-specific differences. In some species, induction will not occur at certain temperatures. In other species, the entire ectoderm can respond to the optic vesicle by forming lenses. These species-specific differences have made this area very difficult to study (Jacobson and Sater 1988; Saha et al. 1989; Saha et al. 1991).
- ‡
It is easy to distinguish permissive and instructive interactions by an analogy with a more familiar situation. This textbook is made possible by both permissive and instructive interactions. The reviewers can convince me to change the material in the chapters. This is an instructive interaction, as the information in the book is changed from what it would have been. However, the information in the book could not be expressed without permissive interactions with the publisher and printer.
- §
Spemann is reported to have put it this way: “The ectoderm says to the inducer, ‘you tell me to make a mouth; all right, I'll do so, but I can't make your kind of mouth; I can make my own and I'll do that'” (quoted in Harrison 1933).
- Induction and Competence - Developmental BiologyInduction and Competence - Developmental Biology
Your browsing activity is empty.
Activity recording is turned off.
See more...