Reprinted with permission from Guan et al., 2003
NCBI Bookshelf. A service of the National Library of Medicine, National Institutes of Health.
Institute of Medicine (US) Forum on Microbial Threats; Knobler S, Mahmoud A, Lemon S, et al., editors. Learning from SARS: Preparing for the Next Disease Outbreak: Workshop Summary. Washington (DC): National Academies Press (US); 2004.
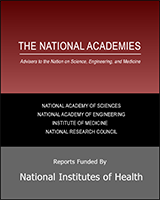
Learning from SARS: Preparing for the Next Disease Outbreak: Workshop Summary.
Show detailsA novel coronavirus (SCoV) is the etiological agent of severe acute respiratory syndrome (SARS). SCoV-like viruses were isolated from Himalayan palm civets found in a live-animal market in Guangdong, China. Evidence of virus infection was also detected in other animals (including a raccoon dog, Nyctereutes procyonoides) and in humans working at the same market. All the animal isolates retain a 29-nucleotide sequence that is not found in most human isolates. The detection of SCoV-like viruses in small, live wild mammals in a retail market indicates a route of interspecies transmission, although the natural reservoir is not known.
Severe acute respiratory syndrome (SARS) recently emerged as a human disease associated with pneumonia (WHO, 2003c). This disease was first recognized in Guangdong Province, China, in November 2002. Subsequent to its introduction to Hong Kong in mid-February 2003, the virus spread to more than 30 countries and caused disease in more than 7900 patients across five continents (WHO, 2003d). A novel coronavirus (SCoV) was identified as the etiological agent of SARS (Ksiazek et al., 2003; Peiris et al., 2003a), and the virus causes a similar disease in cynomolgous macaques (Fouchier et al., 2003). Human SCoV appears to be an animal virus that crossed to humans relatively recently. Thus, identifying animals carrying the virus is of major scientific interest and public health importance. This prompted us to examine a range of domestic and wild mammals in Guangdong Province.
Because the early cases of SARS in Guangdong reportedly occurred in restaurant workers handling wild mammals as exotic food (Zhong et al., 2003), our attention focused on wild animals recently captured and marketed for culinary purposes. We investigated a live-animal retail market in Shenzhen. Animals were held, one per cage, in small wire cages. The animals sampled included seven wild, and one domestic, animal species (see Table 3-2). They originated from different regions of southern China and had been kept in separate storehouses before arrival to the market. The animals remained in the markets for a variable period of time, and each stall holder had only a few animals of a given species. Animals from different stalls within the market were sampled. Nasal and fecal samples were collected with swabs and stored in medium 199 with bovine serum albumin and antibiotics. Where possible, blood samples were collected for serology. Before sampling, all animals were examined by a veterinary surgeon and confirmed to be free of overt disease. Serum samples were also obtained, after informed consent, from traders in animals (n = 35) and vegetables (n = 20) within the market. Sera (n = 60) submitted for routine laboratory tests from patients hospitalized for nonrespiratory disease in Guangdong were made anonymous and used for comparison.
TABLE 3-2
Animal Species Tested for Coronavirus Detection.
Nasal and fecal swabs from 25 animals were tested for SCoV viral nucleic acid by using reverse transcription–polymerase chain reaction (RT-PCR) for the N gene of the human SCoV. Swabs from four of six Himalayan palm civets were positive in the RT-PCR assay (see Table 3-2). All specimens were inoculated into FRhK-4 cells as previously described for virus isolation (Peiris et al., 2003a). A cytopathic effect was observed in cells inoculated with specimens from four Himalayan palm civets (Paguma larvata), two of which also positive for coronavirus in the original specimen by RT-PCR. A virus was also detected by virus isolation and direct RT-PCR from the fecal swab of a raccoon dog (Nyctereutes procyonoides). No virus was detectable in six other species sampled. Electron microscopy of one infected cell supernatant (SZ16) showed viral particles with a morphology compatible with coronavirus (see Figure S-1)6. Sera from five animals had neutralizing antibody to the animal coronavirus; these were from three palm civets, a raccoon dog, and a Chinese ferret badger, respectively (see Table 3-2).
To further validate the results from the neutralization test, a Western blot assay was used to detect SCoV-specific antibodies from these animal serum samples (see Figure 3-1). Indications of positive antibodies were observed from samples SZ2, SZ3, SZ11, and SZ17 (which were also positive in the neutralization assay) and from the positive control human serum. No positive signal was observed from those serum samples that were negative in the neutralization test. There was insufficient serum left over from the raccoon dog (SZ13) to be analyzed by this assay.

FIGURE 3-1
Detection of antibodies against recombinant nucleocapsid protein of SCoV in animal sera by Western blot assay. Recombinant nuleocapsid protein (NP, 49.6 kD) was used as an antigen to detect anti-ScoV antibodies in animal sera. Protein A-HRP was used as (more...)
Sera from humans working in the market were tested for antibody to SZ16 virus by neutralization and indirect immunofluorescence assays. Although 8 out of 20 (40 percent) of the wild-animal traders and 3 of 15 (20 percent) of those who slaughter these animals had evidence of antibody, only 1 (5 percent) of 20 vegetable traders was seropositive. None of these workers reported SARS-like symptoms in the past 6 months. In comparison, none of 60 control sera from patients admitted to a Guangdong hospital for nonrespiratory diseases was seropositive (see Table 3-3).
TABLE 3-3
Prevalence of Antibody to Animal SCoV SZ16 in Humans. Controls Are Serum Specimens from Patients Hospitalized for Nonrespiratory Diseases in Guangdong Made Anonymous
Two of the virus isolates (SZ3 and SZ16) isolated from the nasal swabs of palm civets were completely sequenced, and the amino acid sequence was deduced. Two other viruses were partially sequenced, from the S gene to the 3′ end of the virus (GenBank accession numbers AY304486 to AY304489). Viral RNA sequences from these original swab samples from animal were confirmed in an independent laboratory (Holmes K., unpublished observations). The full-length genome sequences had 99.8 percent homology to the human SCoV, which indicates that the human and animal SCoV-like viruses were closely related. Phylogenetic analysis of the S gene of both human and animal SCoV-like viruses indicated that the animal viruses are separate from the human virus cluster (see Figure 3-2 and Figure S-2)7. However, the viruses SZ1, SZ3, and SZ16 from palm civets were phylogenetically distinct. The viruses SZ3 and SZ16 had 18 nucleotide differences between them over the 29,709–base pair (bp) genome, whereas the human SCoV isolated from five geographically separate sites (GZ50, CUHK-W1, Tor-2, HKU-39848, and Urbani) differed by only 14 nucleotides (nt). Nevertheless, animal virus SZ13 (raccoon dog) and SZ16 (palm civet) were genetically almost identical, and transmission or contamination from one host to the other within the market cannot be excluded.

FIGURE 3-2
Phylogenetic analysis of the nucleotide acid sequence of the spike gene of SCoV-like viruses. Nucleotide sequences of representative SCoV Sgenes (Sgene coding region 21477 to 25244, 3768 bp) were analyzed. The phylogenetic tree was constructed by the (more...)
When the full genome of the animal (n = 2) and human (n = 5, see above) virus groups were compared, the most striking difference was that these human viruses have a 29-nt deletion (5′-CCTACTGGTTACCAACCTGAATG-GAATAT-3′, residue 27869 to 27897) that is 246 nt upstream of the start codon of the N gene (see Figure 3-3). Of human SCoV sequences currently available in GenBank, there was only one (GZ01) with this additional 29-nt sequence. In addition to that, there were 43 to 57 nucleotide differences observed over the rest of the genome. Most of these differences were found in the S gene coding region. The existence of the additional 29-nt sequence in the animal viruses results in demolishing the open reading frames (ORFs) 10 and 11 (Marra et al., 2003) and merging these two ORFs into a new ORF encoding a putative protein of 122 amino acids (see Figure 3-3). This putative peptide has a high homology to the putative proteins encoded by ORF10 and ORF11. Because ORF11 does not have a typical transcription regulatory sequence for SCoV (Marra et al., 2003), the putative ORF11 reported by others may just be the direct result of the deletion of the 29-nt sequence. BLAST search of this peptide yields no significant match to any other known peptide. Further investigation is required to elucidate the biological significance of this finding.

FIGURE 3-3
A 29-nt deletion in the human SCoV genome. (A) Genetic organization of SCoV-like viruses found in humans and animals. ORFs 1a and 1b, encoding the nonstructural polyproteins, and those encoding the S, E, M, and N structural proteins are indicated (green (more...)
When the S-gene sequences of the four animal viruses were compared with 11 human SCoV viruses, 38 nucleotide polymorphisms were noted, and 26 of them were nonsynonymous changes (see Table 3-4). The S genes among the four animal viruses had eight nucleotide differences, whereas there were 20 nucleotide differences among 11 human viruses. Thus, the animal viruses, although isolated from one market, are no less divergent than the human viruses isolated from Hong Kong, Guangdong, Canada, and Vietnam. However, whereas 14 (70 percent) of the 20 polymorphisms among the human viruses were nonsynonymous mutations, only two (25 percent) of the eight nucleotide substitutions within the animal viruses were. An amino acid deletion (nucleotide positions 21690 to 21692) was observed in two of the human viruses (GZ43 and GZ60). Of the 38 polymorphisms, there were 11 consistent nucleotide signatures that appeared to distinguish animal and human viruses. The observation that the human and animal viruses are phylogenetically distinct (see Figure 3-2) makes it highly unlikely that the SCoV-like viruses isolated in these wild animals is due to the transmission of SCoV from human to animals.
TABLE 3-4
Nucleotide Sequence Variation of the S Gene of Animal and Human SCoV.
Our findings suggest that the markets provide a venue for the animal SCoV-like viruses to amplify and to be transmitted to new hosts, including humans, and this is critically important from the point of view of public health. However, it is not clear whether any one or more of these animals are the natural reservoir in the wild. It is conceivable that civets, raccoon dog, and ferret badgers were all infected from another, as yet unknown, animal source, which is in fact the true reservoir in nature. However, because of the culinary practices of southern China, these market animals may be intermediate hosts that increase the opportunity for transmission of infection to humans. Further extensive surveillance on animals will help to better understand the animal reservoir in nature and the interspecies transmission events that led to the origin of the SARS outbreak.
Footnotes
- 6
Supporting online material, www
.sciencemag.org/cgi /content/full/1087139/DC1, Materials and Methods, Figures S1 and S2, and References and Notes. - 7
Supporting online material, www
.sciencemag.org/cgi /content/full/1087139/DC1, Materials and Methods, Figures S1 and S2, and References and Notes.
- 5
We thank the Department of Health and Department of Agriculture of Shenzhen Government for facilitating the study. We gratefully acknowledge the encouragement and support of L.C. Tsui, Vice-Chancellor, The University of Hong Kong. We thank X.Y. Zhao from the Department of Microbiology, The University of Hong Kong, for the excellent technical assistance. We also thank C.C. Hon and F.C. Leung from the Department of Zoology, The University of Hong Kong, and Richard Webby from St. Jude Children’s Research Hospital (Memphis, TN) for assistance in the phylogenetic analysis. We thank K.V. Holmes’ laboratory from the Department of Microbiology, University of Colorado Health Sciences Center (Denver, CO) for validating the animal viral sequences. Supported by research funding from Public Health Research (Grant A195357), the U.S. National Institute of Allergy and Infectious Diseases, the Wellcome Trust (067072/D/02/Z), and SARS research funds from The University of Hong Kong.
- ISOLATION AND CHARACTERIZATION OF VIRUSES RELATED TO THE SARS CORONAVIRUS FROM A...ISOLATION AND CHARACTERIZATION OF VIRUSES RELATED TO THE SARS CORONAVIRUS FROM ANIMALS IN SOUTHERN CHINA - Learning from SARS
Your browsing activity is empty.
Activity recording is turned off.
See more...