NCBI Bookshelf. A service of the National Library of Medicine, National Institutes of Health.
Institute of Medicine (US) Forum on Microbial Threats. The Causes and Impacts of Neglected Tropical and Zoonotic Diseases: Opportunities for Integrated Intervention Strategies. Washington (DC): National Academies Press (US); 2011.
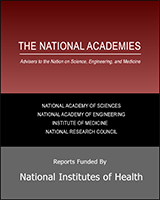
The Causes and Impacts of Neglected Tropical and Zoonotic Diseases: Opportunities for Integrated Intervention Strategies.
Show detailsAbstract: Hookworm infection and schistosomiasis rank among the most important health problems in developing countries. Both cause anaemia and malnutrition, and schistosomiasis also results in substantial intestinal, liver and genitourinary pathology. In sub-Saharan Africa and Brazil, co-infections with the hookworm, Necator americanus, and the intestinal schistosome, Schistosoma mansoni, are common. The development of vaccines for these infections could substantially reduce the global disability associated with these helminthiases. New genomic, proteomic, immunological and X-ray crystallographic data have led to the discovery of several promising candidate vaccine antigens. Here, we describe recent progress in this field and the rationale for vaccine development.
In terms of their global health impact on children and pregnant women, as well as on adults engaged in subsistence farming, human hookworm infection (known as ‘hookworm’) and schistosomiasis are two of the most common and important human infections (Hotez et al., 2008a, 2008b). Together, their disease burdens exceed those of all other neglected tropical diseases (Hotez et al., 2006; King and Dangerfield-Cha, 2008; King et al., 2005; WHO Expert Committee, 2002). They also trap the world's poorest people in poverty because of their deleterious effects on child development and economic productivity (Bleakley, 2007; Hotez et al., 2009; King, 2010). Until recently, the importance of these conditions as global health and economic problems had been underappreciated. Even the United Nations Millennium Development Goals for sustainable poverty reduction did not specifically mention these two conditions (Hotez et al., 2007). An important reason for this ‘neglect’ is that hookworm and schistosomiasis typically affect health without resulting in mortality, with infections such as HIV or malaria causing tenfold more deaths (Table A9-1). However, when the chronic morbidities of these two infections are fully considered according to disability-adjusted life years (DALYs; years of life lost owing to disability, ill health or death), hookworm and schistosomiasis combined rank among the most important diseases in developing countries, resulting in 4.5–92 million DALYs annually, the upper limit of which is greater than the DALYs due to malaria or HIV/AIDS (Hotez et al., 2006; King and Dangerfield-Cha, 2008; King et al., 2005). Current efforts to control hookworm and schistosomiasis are inadequate and new tools that combat hookworm and schistosomiasis, with an emphasis on disease caused by Necator americanus, the major hookworm of humans, and Schistosoma mansoni, the primary cause of intestinal schistosomiasis.
TABLE A9-1
Impact of hookworm, schistosomiasis, HIV/AIDS, and malaria.
Global Distribution and Pathobiology
Hookworms are roundworm parasites that belong to the phylum Nematoda. They share phylogenetic similarities with the free-living nematode Caenorhabditis elegans and with the parasitic nematodes Nippostrongylus brasiliensis and Heligmosomoides polygyrus, which are often used by immunologists to study T helper 2 (TH2) cell and related responses in mice (Finkelman et al., 2004) (Box A9-1). Schistosomes are platyhelminths (flatworms) that belong to the order Trematoda (commonly called the trematodes or flukes). Human infections with hookworms and schistosomes occur predominantly in areas of rural poverty in sub-Saharan Africa, Southeast Asia and tropical regions of the Americas. The epidemiology and pathobiology of both hookworm and schistosomiasis have been extensively reviewed recently (Bethony et al., 2006; Brooker et al., 2004; Gryseels et al., 2006; Hotez et al., 2004, 2005; Steinmann et al., 2006) and are only briefly discussed here.
Hookworm
The global distribution and life cycle of hookworms are shown in Figure A9-1. An estimated 600 million to 700 million people are infected worldwide, with the most infections occurring in the Asian countries of Indonesia, Bangladesh and India (60 million to 70 million people in each), followed by Nigeria and the Democratic republic of the Congo in Africa, and Brazil (30 million to 40 million people in each) (Bethony et al., 2006; de Silva et al., 2003; Hotez and Kamath, 2009; Hotez et al., 2008c). Approximately 85% of hookworm infections are caused by N. americanus, and the remainder are caused by Ancylostoma duodenale. Microscopic infective larvae (third-stage larvae, or L3) live as non-feeding, non-replicating, developmentally arrested environmental stages in the soil, where they survive for a few days to weeks, depending on the temperature and the level of moisture. Hookworm L3 phenotypically resemble the developmentally arrested dauer larvae of C. elegans (Tissenbaum et al., 2000). Human infection occurs when L3 come into contact with the skin. Larvae actively penetrate skin and migrate in the afferent vasculature to the lungs, where they ascend the pulmonary tree to the pharynx, are swallowed and moult to become adult male and female hookworms ~1 cm long. Adult hookworms burrow deep into the mucosa and submucosa of the small intestine, eventually rupturing capillaries and arterioles (Brooker et al., 2004; Hotez et al., 2004). Blood ingestion ensues, followed by the lysis of erythrocytes, an ordered enzymatic digestion of host haemoglobin (Ranjit et al., 2009; Williamson et al., 2004) and haeme detoxification (Zhan et al., 2005, 2010). Almost all of the pathology and morbidity owing to hookworm is the result of intestinal blood loss (Hotez et al., 2004). Female and male hookworms mate in the small intestine, and the females release microscopic eggs that exit the body in host faeces. The eggs hatch in the soil, resulting in a new generation of first-stage larvae, which feed on bacteria and other organic debris in the soil before they moult twice to the L3 stage and continue the life cycle.

FIGURE A9-1
Global distributions and life cycles of hookworms and schistosomes. a| The distribution of Schistosoma mansoni, which causes intestinal schitosomiasis and Necator americanus, a hookworm, are shown (deSilva et al., 2003; Hotez et al., 2004, 2005). b| The (more...)
Schistosomiasis
Approximately 90% of the world's 207 million cases of schistosomiasis occur in sub-Saharan Africa, with the most in Nigeria, Tanzania, the Democratic republic of the Congo and Ghana (Steinmann et al., 2006). In Africa, Schistosoma haematobium is the cause of urinary tract schistosomiasis (accounting for approximately two-thirds of the world's cases of schistosomiasis), whereas S. mansoni is the main cause of intestinal schistosomiasis (approximately one-third of total cases) (Figure A9-1). S. mansoni also causes intestinal schistosomiasis in Latin America, with most of the cases occurring in Brazil, whereas Schistosoma japonicum and Schistosoma mekongi cause fewer than one million cases of intestinal schistosomiasis in Asia (Steinmann et al., 2006). Schistosomiasis is a fresh-water-borne disease, and humans become infected when free-swimming microscopic cercariae penetrate the skin. These larvae shed their tails to become schistosomulae, which enter the vasculature and lungs before relocating to the venous system, where they become sexually mature adults that pair and mate (Gryseels et al., 2006). Adult S. haematobium schistosomes migrate to the venous plexus, which drains the bladder and reproductive organs, whereas S. mansoni and S. japonicum go to the mesenteric veins draining the intestine (Gryseels et al., 2006). Female schistosomes produce eggs that are equipped with a spine to facilitate penetration through blood vessels and into the urinary tract and genitals (S. haematobium) or into the intestine and liver (S. mansoni and S. japonicum). During chronic infection, which can last 5–7 years, much of the pathology from schistosome infection is a product of the immune response to parasite eggs in host tissues, and the resulting granulomatous lesions lead to fibrosis, which, in turn, can cause severe circulatory impairment of the affected organs (Gryseels et al., 2006; King and Dangerfield-Cha, 2008; Steinmann et al., 2006).
Hookworms, Schistosomes and Anaemia
Both hookworm and schistosomiasis cause chronic anaemia that, over the long term, can manifest as impaired neurological and cognitive functioning in children, diminished work capacity in adults, and adverse outcomes of pregnancy in both mother and child (Tolentino and Friedman, 2007). The WHO defines anaemia as a blood haemoglobin concentration of below 11–13 g per 100 ml, depending on age, sex and pregnancy status; severe anaemia in pregnancy is defined as a haemoglobin concentration of below 7 g per 100 ml (WHO, 2001). Approximately 50% of anaemia cases result from iron deficiency (iron deficiency anaemia (IDA)), with nearly three-quarters of the morbidity from IDA occurring in the poorest regions of Africa, Asia and the Americas (Stoltzfus, 2003). IDA is associated with ~841,000 deaths and ~35 million DALYs annually, mostly by contributing to both maternal and perinatal mortality and through adverse effects on childhood development and cognition (Larocque et al., 2005; Stoltzfus, 2003). Young children are particularly susceptible to IDA, because of their increased iron requirements during growth periods, as are women of reproductive age, because of menstrual losses and the high iron demands of a growing fetus during pregnancy (Tolentino and Friedman, 2007).
Hookworm-associated anaemia
IDA is the hallmark of hookworm and results from intestinal blood loss caused by the feeding of adult worms at the site of parasite attachment in the intestine (Crompton, 2000; Hotez et al., 2004). Infection with 25–30 adult N. americanus hookworms results in at least 1 ml of blood loss per day, a volume containing an amount of iron roughly equivalent to the daily requirement of an adolescent boy or girl and slightly more than the daily requirement of a younger child in order for them to grow (Crompton, 2000; FAO and WHO, 2002). As well as causing IDA, intestinal blood loss can result in protein malnutrition (Brooker et al., 2004; Hotez et al., 2004). Numerous epidemiological studies confirm the substantial contribution of hookworm to the global burden of IDA (Stoltzfus et al., 1997). Among school-aged children in Zanzibar, Tanzania, 41% of IDA and 57% of moderate to severe anaemia is attributable to hookworm (Stoltzfus et al., 1997). Hookworm has been shown to be an important risk factor for anaemia in Brazilian schoolchildren (Brooker et al., 2007), and it has been identified as a key determinant of IDA in preschool children in Kenya (Brooker et al., 1999), Tanzania (Sousa-Figueiredo et al., 2008) and Malawi (Calis et al., 2008). Similarly, studies have identified hookworm as an important cause of IDA in non-pregnant women in Zanzibar and Vietnam (Nguyen et al., 2006; Stoltzfus et al., 1997), whereas it accounts for 41–54% of the moderate to severe anaemia in pregnant women in Nepal (Dreyfuss et al., 2000; Stoltzfus et al., 1997). The positive association between intensity of the hookworm infection and anaemia in children and during pregnancy was confirmed in recent meta-analyses (Brooker et al., 2008; Smith and Brooker, 2010). For both children and women, anaemia is far more likely to be present in those with moderate to heavy hookworm infections (Brooker et al., 2008; Stoltzfus et al., 1997), which are defined on the basis of quantitative faecal egg counts exceeding 1,999 eggs per gram (epg) of faeces compared with counts of individuals with no or light infection (<2,000 epg of faeces) (WHO Expert Committee, 2002).
Schistosomiasis-associated anaemia
All of the major forms of human schistosomiasis are associated with anaemia (Friedman et al., 2005a, 2005b; King and Dangerfield-Cha, 2008; King et al., 2005; Koukounari et al., 2006, 2007, 2008; Stephenson, 1993; Sturrock et al., 1996; Tohon et al., 2008). As found for hookworm, children and pregnant women infected with schistosomes are especially susceptible to anaemia (Ajanga et al., 2006; Friedman et al., 2005a, 2005b, 2007; Koukounari et al., 2006, 2007, 2008; Prual et al., 1992; Stephenson, 1993; Sturrock et al., 1996; Tohon et al., 2008) and reduced haemoglobin concentrations in both have been associated with moderate to high faecal or urine schistosome egg counts (Friedman et al., 2005a; Koukounari et al., 2006, 2007, 2008; Prual et al., 1992; Sturrock et al., 1996; Tolentino and Friedman, 2007). The anaemia associated with schistosomiasis has been attributed to several mechanisms, including iron deficiency due to blood loss in the intestine or urine, splenic sequestration and destruction of erythrocytes, autoimmune haemolysis, and the chronic inflammatory response to schistosome eggs deposited in host tissues (Friedman et al., 2005b; Tolentino and Friedman, 2007).
Co-infections
In Africa and South America, co-infections with hookworm and schistosomes are common, and there are at least a dozen countries with more than five million cases of each helminth infection (Hotez et al., 2008b). It has been proposed that co-infection with N. americanus and S. mansoni is synergistic with respect to worm burdens and the resulting pathologic sequelae, including anaemia (Brito et al., 2006; Brooker et al., 2007; Ezeamama et al., 2008; Fleming et al., 2006; Friis et al., 2003; Guyatt et al., 2001; King, 2010; Lwambo et al., 1999; Raso et al., 2006; Stephenson, 1994; Stephenson et al., 1985). A similar relationship has been suggested between hookworm and S. japonicum infection in East Asia (Ezeamama et al., 2008). In sub-Saharan Africa, there is also extensive geographical overlap among areas of hookworm, schistosomiasis, and malaria transmission resulting from infection with Plasmodium falciparum, another notable cause of anaemia (Brooker et al., 2007; Demissie et al., 2009). In Kenya and Tanzania, the anaemias resulting from hookworm and P. falciparum co-infections have been shown to be additive (Brooker et al., 2007). There are conflicting data on whether hookworm or schistosomiasis increase host susceptibility to malaria or adversely affect the clinical course of the disease (Hotez et al., 2006). In Africa, female genital schistosomiasis caused by S. haematobium was shown to increase the odds ratio of acquiring HIV/AIDS threefold (Kjetland et al., 2006), and it has been suggested that S. mansoni may also affect susceptibility to HIV (Chenine et al., 2008; Da'dara and Harn, 2010). Hookworm and other intestinal nematode infections are immuno modulatory and may increase viral loads and the progression of HIV/AIDS (Bentwich et al., 2008), but larger studies are needed to confirm this relationship. Anaemia itself may adversely affect the course of HIV/AIDS (Moore, 2000), so hookworm and schistosomiasis may indirectly impact the progression of this disease. The global public health impact of anaemia and other pathological sequelae resulting from hookworm and schistosomiasis have stimulated efforts to develop more effective control strategies for these conditions. Such measures include the development of new control tools, foremost among these being anthelmintic vaccines. The current status of the development of vaccines is outlined below, first for hookworm and then for schistosomiasis.
Rationale For a Human Hookworm Vaccine
Hookworm and other common intestinal helminth infections such as ascariasis and trichuriasis are strongly associated with poverty and poor sanitation. However, improvements in sanitation or other environmental and personal protective control measures (such as footwear) frequently have a minimal impact on parasite prevalence or the intensity of infection, especially without accompanying programmes of health education and economic development (Asaolu and Ofoezie, 2003; Moraes et al., 2004). The WHO has proposed annual mass treatment (that is, deworming of an entire population or age stratum in an endemic area) with a benzimidazole such as albendazole or mebendazole as the most cost-effective means of reducing the childhood morbidity related to chronic intestinal helminth infection (although the impact on improving childhood cognition is variable) (Hotez, 2009; Olsen, 2007; Smith and Brooker, 2010; WHO, 2006, 2008). There is evidence that both albendazole and mebendazole interfere with invertebrate tubulin and microtubules and reduce the number of adult worms in the intestine (Geary et al., 2010). In 2001, the 54th World Health Assembly committed to providing annual deworming for school-aged children wherever the prevalence in this age group exceeds 50% (Hotez, 2009; Olsen, 2007; Smith and Brooker, 2010; WHO, 2006, 2008), although as of 2008 only 9% of school-aged children and 21% of preschool-aged children at risk of acquiring intestinal helminth infections have benefited from this intervention (Hotez, 2009; WHO, 2008).
New information indicates that annual deworming may be less effective for hookworm than other intestinal helminth infections. For example, single-dose albendazole or mebendazole typically achieves either cure or substantial reductions in worm burdens and faecal egg counts for ascariasis (Hotez, 2009; Keiser and Utzinger, 2008). However, for hookworm high rates of drug failure have been reported for mebendazole, with an average cure rate of only 15% (Keiser and Utzinger, 2008). Furthermore, after repeated administration in the same population, the efficacy of mebendazole has been reported to diminish over time (Albonico et al., 2003), raising concerns about possible drug resistance. Indeed, drug failures have been shown to occur with benzimidazoles when used ubiquitously in livestock and have been associated with specific point mutations in the parasite gene encoding β-tubulin (Geerts and Gryseels, 2000). Although the same mutations have not yet been associated with drug failure in humans, efforts are underway to determine whether benzimidazole failures for hookworm and other helminth infections result from similar resistance mechanisms (Geerts and Gryseels, 2000).
Evidence of widespread mebendazole drug failure indicates that the global control of human hookworm depends solely on the continued efficacy of albendazole. However, although treatment with albendazole cures existing infections, it does not confer protection from reinfection, which often occurs as early as 6 months after treatment in areas of high transmission (Albonico et al., 1995). Concerns about mebendazole drug failure, possible emerging resistance to the benzimidazoles and the rapid reinfection after treatment provided the impetus for establishing the Human Hookworm Vaccine Initiative, a non-profit product development partnership based at the Sabin Vaccine Institute (Washington DC, USA) that was established in 2000 to develop and test new vaccines for hookworm (Bethony et al., 2006; Bottazzi and Brown, 2008; Brooker et al., 2004; Diemert et al., 2008; Hotez and Brown, 2008; Hotez and Ferris, 2006; Hotez et al., 2008a, 2008b; Loukas et al., 2006). Prior efforts to develop human hookworm vaccines were limited to basic research conducted in university laboratories, although a live attenuated canine hookworm vaccine was developed by industry and briefly marketed for pet owners in the early 1970s (Bethony et al., 2006; Loukas et al., 2006; Miller, 1978). The HHVI is the only partnership in the world that is currently working on vaccine development for hookworm and consists of investigators from the Sabin Vaccine Institute, the George Washington University (Washington DC, USA), The Fundacao oswaldo Cruz (FIoCrUZ; Brazil), the Instituto Butantan (Sao Paulo, Brazil), James Cook University (Cairns, Australia) and the London School of Hygiene and Tropical Medicine (UK). The ultimate aim of vaccine development efforts is to prevent moderate and heavy hookworm infections (that is, infections associated with faecal egg counts exceeding 1,999 epg of faeces), which are associated with substantial intestinal blood loss. Such a vaccine could be administered to very young preschool-aged children in a programme of ‘vaccine-linked chemotherapy’ (Bergquist et al., 2008) before their exposure to infective larvae in the environment, or to both preschool-aged and school-aged children who may have already been exposed and even infected.
Targeting Hookworm Blood Feeding
The vaccines that are currently under development by the HHVI target the nutritional and metabolic requirements of the adult hookworm. The main approach has been to identify the essential components involved in parasite blood feeding, to genetically engineer these components as recombinant proteins and then to combine the recombinant components with one or more adjuvants to elicit protective antibodies on vaccination (Loukas et al., 2006). These protective antibodies would either directly neutralize the parasite macromolecules required for blood feeding and nutrition or indirectly damage important parasite structures. Protective immunity in vaccinated individuals would manifest as diminished hookwormrelated blood loss and reduced numbers of hookworms in the intestine compared with levels in unvaccinated people. Based on prior experiences with live attenuated helminth vaccines for veterinary use, including those for canine hookworm and bovine lungworm, sterilizing immunity is not considered an attainable — or necessary — goal for anthelmintic vaccines (Bethony et al., 2006; Miller, 1978; Urquhart, 1985) (Table A9-2). However, as the morbidity associated with hookworm is proportional to the number of worms harboured by individuals, a vaccine that prevents most moderate and heavy infections would be sufficient to have a major impact on the worldwide burden of hookworm and the associated anaemia.
TABLE A9-2
Successful vaccines against helminth infections.
Over the past decade, the molecular basis by which hookworms ingest and derive nutrition from host blood has been elucidated. As with all haematophagous parasites, N. americanus depends on host haemoglobin and serum proteins for survival. Adult hookworms ingest blood, lyse erythrocytes, degrade haemoglobin and serum proteins, and then absorb the digested peptides and amino acids (Ranjit et al., 2009; Williamson et al., 2004) (Figure A9-2). Following haemolysis, adult N. americanus hookworms use a hierarchical cascade of haemoglobinases (haemoglobin-degrading proteases), beginning with the cleavage of intact haemoglobin by an aspartic protease, APr1, followed by further proteolysis through the action of several cysteine proteases and metalloproteinases, all of which are expressed in the brush border membrane of the parasite's digestive tract (Ranjit et al., 2009). The resultant small peptides and free amino acids are possibly absorbed through the parasite gut through a homologue of the membrane-spanning amino acid transporter of the free-living nematode C. elegans (Meissner et al., 2004). After their cleavage from digested globin, both iron-containing haeme and iron-containing haematin are potentially toxic to hookworms, because these compounds can generate oxygen radicals that may damage parasite structures (Brophy and Pritchard, 1992). Therefore, in addition to haemoglobinases, all blood-feeding parasites such as hookworms and P. falciparum have evolved mechanisms to detoxify and transport haeme (Deponte and Becker, 2005; Jani et al., 2008; Zhan et al., 2002, 2005). In the case of N. americanus and other hookworms, one putative mechanism for neutralizing these toxic moieties involves the pairing of glutathione S-transferase 1 (GST1) molecules as homodimers to create specific pockets capable of binding haeme and haematin (Asojo et al., 2007; Zhan et al., 2002, 2005) (Figure A9-2).

FIGURE A9-2
Necator americanus degradation of host blood components and potential vaccine targets. Adult worlms in the gut ingest blood, and parasite haemolysins drill pores into the erythrocytes, releasing haemoglobin into the parasite gut lumen (step 1). Haemoglobin (more...)
Antigens of the Human Hookworm Vaccine
From approximately two-dozen proteins that are putatively involved in the hookworm blood-feeding process (Brooker et al., 2004; Loukas et al., 2006), two lead candidate antigens have been selected for clinical development. The antigen selection programme of the HHVI is based on a ranking system that includes several key criteria, such as efficacy in animal trials, immuno-epidemiological observations in individuals resident in endemic areas, and the feasibility of protein expression and scaled-up manufacture using low-cost expression systems such as yeast, bacteria, or plants (Loukas et al., 2006) (Table A9-3). In addition, antigens are prioritized if there is a plausible mechanism of protection associated with them, such as triggering the production of antibodies that inhibit crucial parasite enzymes or target important surface antigens. Both GST1 and APr1 are involved in parasite blood feeding, and it is thought that each antigen induces antibodies that interfere with the function of the respective protein and impair worm survival. Both antigens are therefore being considered for eventual combination in a human hookworm vaccine.
TABLE A9-3
Ranking of Lead Candidate Necator americanus Vaccine Antigens.
GST1 is a 24 kDa polypeptide that is expressed as a recombinant protein in the yeast Pichia pastoris to generate the antigen used for vaccines. Both GST1 from N. americanus and its orthologue from the canine hookworm Ancylostoma caninum have peroxidase activity that catalyses the conjugation of reduced glutathione to various electrophiles (Asojo et al., 2007; Zhan et al., 2002, 2005). Both proteins belong to the Nu class of nematode GSTs, which is characterized by a reduced peroxidase activity relative to other classes of GSTs but an increased binding capacity for haeme and related products (Asojo et al., 2007; Schuller et al., 2005; van Rossum et al., 2004; Zhan et al., 2002, 2005). According to X-ray crystallography studies, N. americanus GST1 forms homodimers in solution to create atypically large binding cavities that are accessible to a diversity of ligands, including haeme (Asojo et al., 2007), to which GST1 from both A. caninum and N. americanus binds with high affinity in vitro (Zhan et al., 2002, 2005). Both haeme and haematin contain oxidative iron that can result in the formation of oxygen radicals, which damage helminth structures. In vivo, GSTs may protect hookworms by binding and detoxifying haeme and the haematin byproducts that are generated during the blood digestion process (Zhan et al., 2002, 2005).
On the basis of their putative roles in hookworm blood feeding, GST1 from both N. americanus and A. caninum were tested in laboratory animal models of hookworm. In dogs, vaccination with recombinant A. caninum GST1 resulted in high levels of antibodies; following challenge with infective A. caninum larvae, the worm burdens and faecal egg counts of these vaccinated dogs were substantially lower than those observed in controls (Zhan et al., 2005). In hamsters, vaccination with recombinant A. caninum GST1 also resulted in cross-protection, with substantially lower worm burdens (less than half) following heterologous challenge with infective N. americanus larvae than those seen in controls (Xiao et al., 2008; Zhan et al., 2005), as did vaccination with N. americanus GST1 followed by homologous larval challenge (Zhan et al., 2002). A recombinant GST from the nematode parasite Wuchereria bancrofti is also showing promise in a jird model as a protective antigen against lymphatic filariasis (Veerapathran et al., 2009). Because of these encouraging results, recombinant N. americanus GST1 (formulated with Alhydrogel [aluminium hydroxide]) has been produced according to current good manufacturing practices in preparation for clinical trials.
N. americanus APr1 is a 45 kDa protein. For stability and safety reasons, including concerns about injecting humans with an active proteolytic enzyme, the version of this aspartic protease used for vaccination has been inactivated by substituting alanines for the catalytic aspartic acid residues (Pearson et al., 2009). The recombinant protein has been expressed in multiple systems, with Escherichia coli (Pearson et al., 2009) and tobacco plants (Yusibov and Rabindran, 2008) producing the highest yields. The rationale for selecting N. americanus APr1 for development was based on successful laboratory animal trials. In dogs vaccinated with either N. americanus APr1 or A. caninum APr1, high levels of antibody were induced that inhibited protease activity in vitro; this was associated with substantially diminished blood loss (measured by haemoglobin levels) and worm burdens following challenge with A. caninum larvae compared with those seen in controls (Loukas et al., 2005; Pearson et al., 2009). Vaccination with A. caninum APr1 also resulted in a substantial reduction in the worm burdens of hamsters challenged with N. americanus compared with burdens of controls (Xiao et al., 2008). These findings also suggest that cross-reactive immunity between Necator spp. and Ancylostoma spp. hookworms may occur. Following vaccination, APr1-specific antibodies are ingested by the parasite during blood feeding and localize to the parasite gut, where they can inhibit parasite feeding by neutralizing enzyme activity (Loukas et al., 2005; Xiao et al., 2008) (Figure A9-2). Efforts to optimize both the yield and the solubility of N. americanus APr1 are in progress. A chimeric protein consisting of N. americanus GST1 fused to epitopes of N. americanus APr1, to generate neutralizing antibodies and inhibit parasite blood digestion, is already under development (Pearson et al., 2010). Additional key molecules involved in hookworm blood feeding have been identified from proteomic and transcriptomic analyses of the hookworm gut (Ranjit et al., 2006). These molecules include a putative hookworm orthologue of a prolylcarboxypeptidase (‘contortin’) that protects sheep against Haemonchus contortus infection (Geldhof and Knox, 2008), and the extracellular domain of an intestinal peptide transporter that is essential for nutrient uptake and growth in C. elegans (Meissner et al., 2004).
Previous vaccine antigens
ASP2 from N. americanus infective larvae was previously under consideration as a candidate vaccine antigen (Bethony et al., 2005; Fujiwara et al., 2005, 2008; Goud et al., 2005). The canine hookworm orthologue of this molecule was determined to be a major immunogen associated with an effective vaccine consisting of live attenuated A. caninum L3 larvae (Bethony et al., 2005; Fujiwara et al., 2006). In a Phase I trial conducted in healthy volunteers in the United States, ASP2 adjuvanted with Alhydrogel was shown to be safe and immunogenic (Goud et al., 2005). However, in a second Phase I trial conducted at a hookworm endemic site in Brazil, some of the adult volunteers experienced generalized urticaria immediately after vaccination (D.J.D., unpublished observations). The study was halted, and it was found that individuals who developed urticaria had high levels of immunoglobulin E (IgE) against ASP2. This finding has led to testing for the levels of IgE specific for candidate vaccine antigens using sera from individuals resident in hookworm-endemic areas. No detectable levels of IgE specific to N. americanus GST1 (J.M.B. and D.J.D., unpublished observations) or N. americanus APr1 (REF. 100) have been found in individuals living in hookworm-endemic areas of Brazil, thus permitting their continued development as candidate vaccine antigens. The reason neither recombinant adult hookworm protein induces IgE during natural infection is unknown, but it may be related to antigen structure or presentation to the immune system.
Vaccinating Against Schistosomiasis
In both Brazil and most of sub-Saharan Africa, N. americanus hookworm infections are co-endemic with intestinal schistosomiasis caused by S. mansoni (Hotez et al., 2008b). Vaccines to combat each of these helminth infections are being developed because both diseases are associated with anaemia and malnutrition, especially in children. Ultimately, the two vaccines may be co-administered or combined in a multivalent anthelmintic vaccine (see below) (Hotez et al., 2008b). The justification for developing vaccines against schistosomiasis has been reviewed recently (Bergquist et al., 2008; McManus and Loukas, 2008) and includes the high disease burden (King and Dangerfield-Cha, 2008; King et al., 2005), the high rates of post-treatment re infection, the inability of chemotherapy-based morbidity control to interrupt transmission (King et al., 2006), the exclusive reliance on praziquantel for control and concerns about emerging drug resistance without new drugs in the development pipeline (Bergquist et al., 2008; McManus and Loukas, 2008). An important additional stimulus to develop new preventive approaches to schistosomiasis is the observation of so-called ‘rebound morbidity’: up to 80% of children living in high-transmission areas can suffer recurrent aggressive inflammation following interrupted annual chemotherapy because of reinfection. The feasibility of developing vaccines for schistosomiasis has been extensively reviewed (Bergquist et al., 2008; McManus and Loukas, 2008; Oliviera et al., 2008). Humans living in endemic areas can become resistant or partially immune to reinfection over time (Correa-Oliviera et al., 2000). Furthermore, irradiated cercariae can elicit high levels of protective immunity in laboratory animals, and several recombinant-protein vaccines have been shown to elicit comparable levels of protective immunity in immunized animals that were subsequently challenged with cercariae (McManus and Loukas, 2008).
Schistosome Antigens Under Development
To date, one vaccine for urinary schistosomiasis has entered clinical trials. The Institut Pasteur and the French Institut National de la Sante et de la recherché Medicale have taken a recombinant 28 kDa GST cloned from S. haematobium through both Phase I and Phase II clinical trials in Europe and West Africa (Capron et al., 2005; McManus and Loukas, 2008) (see also Le Project Bilhvax 3). Known as Bilhvax, this GST formulated with an aluminium hydroxide adjuvant has been reported to be immunogenic and safe after testing in healthy adults (Capron et al., 2005). However, further information regarding its efficacy, the duration of protection and the progress towards licensure are not available in the published literature. In addition, other vaccine candidates for intestinal schistosomiasis caused by S. mansoni will soon be ready for clinical testing (Oliviera et al., 2008). One candidate is a 14 kDa fatty acid-binding protein known as Sm14 (Moser et al., 1991), which in experimental animals (mice and rabbits) elicits protection against S. mansoni as well as against Fasciola hepatica, another trematode fluke (Tendler and Simpson, 2008). Recently, the group developing this vaccine reported success in stabilizing Sm14 by replacing a crucial cysteine residue in order to prevent dimerization (Ramos et al., 2009). Recombinant Sm14 is being developed as an anthelmintic vaccine by a partnership between private and government organizations in Brazil for use against both fascioliasis of livestock and human schistosomiasis caused by S. mansoni (Tendler and Simpson, 2008). Another S. mansoni vaccine potentially moving into clinical development is Sm-p80, which is a DNA vaccine encoding the large subunit of a calcium-dependent neutral protease, providing levels of protection in baboons that are comparable to irradiated cercariae (Zhang et al., 2010). Finally, the schistosome molecule paramyosin is undergoing pilot-scale production in Asia for use against S. japonicum infection, possibly as a transmission-blocking vaccine, administered to water buffaloes (Jiz et al., 2008).
The Sabin Vaccine Institute, in partnership with FIoCrUZ and the Instituto Butantan, is also working to transition a S. mansoni vaccine into clinical testing in Brazil (J.M.B., D.J.D. and P.J.H., unpublished observations). The primary targets of this vaccine development programme are schistosome membrane proteins identified by combined genomic, post-genomic and proteomic analyses of the adult S. mansoni outer surface, or tegument (Loukas et al., 2007) (Table A9-4). The tegument of adult schistosomes is a single syncytium covering the body wall and is thought to be a dynamic layer involved in several key physiological processes, including parasite nutrition, osmoregulation and evasion of host immunity (Loukas et al., 2007). Hence, the schistosome tegument is a potentially vulnerable target for immunological attack by host antibodies. However, analysis of the schistosome proteome predicts that surprisingly few membrane-spanning proteins of the tegument are accessible to the host immune response (Braschi and Wilson, 2006; Loukas et al., 2007). They include a family of tetraspanin integral membrane proteins (Tran et al., 2006) and several outer-membrane proteins of unknown function, such as Sm29 (Cardoso et al., 2006, 2008). The tetraspanins are so named because they contain four transmembrane domains, with two extracellular loops that are predicted to interact with exogenous proteins or ligands (Figure A9-3). The second extracellular domain fragment of a schistosome tetraspanin known as TSP2, from S. mansoni, has been selected for development as a human vaccine antigen. When this 9 kDa extracellular domain was expressed in either P. pastoris or E. coli and formulated with several adjuvants (including Freund's complete adjuvant (Tran et al., 2006), aluminium hydroxide, or aluminium hydroxide with CpGs) it provided high levels of protection in mice vaccinated with the antigen and then challenged with S. mansoni cercariae (A.L. and M.S.P., unpublished observations). In addition, evidence from human epidemiological studies indicates that putatively resistant individuals living in endemic areas of Brazil have elevated antibody responses to this protein compared with the responses of chronically infected individuals from the same endemic areas (Tran et al., 2006). recently, the S. japonicum orthologue of S. mansoni TSP2 was described and resulted in protection in mice that was similar to that described for S. mansoni TSP2, suggesting that this molecule may be effective against multiple human schistosome species (Yuan et al., 2010).
TABLE A9-4
Ranking of Lead Candidate Schistosoma mansoni Vaccine Antigens.

FIGURE A9-3
Schistosoma mansoni tegument. a| A fluorescence micrograph of the tegument of an adult male Schistosoma mansoni probed with a mouse antibody raised recombinant TSP2 (red), a tetraspinin. Nuclei. Stained with 4',6-diamidino-2-phenylindole (DAPI), are blue. (more...)
S. mansoni TSP2 is thought to have a crucial role in tegument development and maturation (Tran et al., 2010). The ultrastructural morphology of adult worms and schisto somula treated in vitro with S. mansoni tsp2 double-stranded rNA (dsrNA) displays a distinctly vacuolated and thinner tegument compared with that of controls, suggestive of impaired closure of tegumentary invaginations (Tran et al., 2010). Moreover, injection of mice with schistosomulae that had been pre-treated with S. mansoni tsp2 dsrNA resulted in 83% fewer parasites being recovered from the mesenteric veins 4 weeks later when compared with recovery from mice injected with untreated schistosomulae (Tran et al., 2010). These results suggest that tetraspanins have important structural roles in tegument development, maturation or stability. other tegument tetraspanins are attractive vaccine candidates; for example, S. mansoni tsp3 is the most highly upregulated mrNA in maturing schistosomula, a developmental stage that is widely accepted as being susceptible to damage by the human immune system (Fitzpatrick et al., 2009; Gobert et al., 2010). In addition, Sj23 (23 kDa integral membrane protein of S. japonicum) is a tegument tetra spanin that is showing promise as a DNA vaccine for water buffaloes, which are an important reservoir host for S. japonicum in China (Da'dara et al., 2008).
Future Directions
By targeting both hookworms and schistosomes, human helminth vaccines are being developed to reduce parasiteinduced morbidity, the symptoms of which include intestinal blood loss and inflammation (Hotez et al., 2008b). Administered in early childhood, such vaccines could prevent the major paediatric sequelae of these infections, including anaemia, malnutrition, growth failure and impaired cognitive development.
The human hookworm vaccine is being developed as a bivalent product consisting of two co-formulated recombinant proteins (GST1 and APr1 from N. americanus) to prevent moderate and heavy hookworm infections caused by N. americanus. The vaccine is intended primarily for preschool- and school-aged children (<10 years of age) living in N. americanus-endemic regions. Similarly, TSP2 from S. mansoni is being developed as a recombinant-protein vaccine for the prevention of heavy-intensity infections of S. mansoni, the leading cause of intestinal schistosomiasis. A paediatric population will be targeted for both vaccines because this age group is at the greatest risk of developing the severe developmental, growth and cognitive impairments associated with these chronic infections (Bethony et al., 2006; Brooker et al., 2004; Hotez et al., 2004, 2005, 2008a). Initially, vaccines containing the hookworm and schistosome antigens are being formulated with Alhydrogel; however, they will also be evaluated with an additional immunostimulant such as a lipid A derivative. The vaccines will be delivered by intramuscular injection, and the goal is to achieve the desired protection after one or two doses, depending on the number of doses required to achieve a protective response. The desired result is the prevention of moderate and heavy helminth infections, which would have a major impact on the anaemia, malnutrition and end-organ pathology associated with these parasitic infections in children (King and Dangerfield-Cha, 2008; Stoltzfus et al., 1997). The extension of protection into adulthood would also prevent the severe anaemia that is related to infection with these parasites during pregnancy, and reduce transmission. Such vaccines may also have an important impact on poverty reduction because of their anticipated effect on improving child and maternal health and development (Hotez and Ferris, 2006).
Both vaccines are being developed with the ultimate goal that even the most impoverished populations will have access to them as soon as they are available. As such, a strategic road map is being followed to ensure that lowcost manufacturing processes are used and that vaccine manufacturers in middle-income disease-endemic countries are involved from the start. In the Americas, Brazil is the furthest advanced, with two major vaccine manufacturers — FIoCrUZ/ Bio-Manguinhos and the Instituto Butantan — actively engaged in development (Morel et al., 2005). Accurate forecasting of the eventual demand for licensed vaccines is essential: for hookworm, it is estimated that there are approximately one billion children at risk globally, so that covering a global birth cohort would require the vaccination of 100 million children annually (WHO, 2008). Demand forecasting is underway for an intestinal schistosomiasis vaccine.
Substantial hurdles must be overcome during clinical development of hookworm and schistosomiasis vaccines, not least of which is securing adequate funding to conduct the clinical trials required for licensure. Additional obstacles include obtaining access to the novel adjuvants that may be required to induce an adequate immune response and the difficulty of conducting large-scale efficacy studies in endemic areas. As hookworm and schistosomiasis are prevalent in resourcelimited, rural areas of the tropics and subtropics, this is where Phase III clinical trials must be conducted, which can be logistically challenging. Furthermore, because the clinical effects of these parasitic infections are chronic, with sequelae such as IDA often appearing only after months or years of infection, efficacy trials will be necessarily long.
Combining hookworm and schistosomiasis vaccines
In the future, vaccines for hookworm and intestinal schistosomiasis could be combined in a multivalent anthelmintic vaccine (Hotez et al., 2008b), which may increase vaccine efficiency and reduce the timeframe for widespread distribution in affected areas of Africa and Latin America. The Sabin Vaccine Institute is currently working with the WHO to build consensus on the use of hookworm and schistosomiasis vaccines in resourcepoor settings (WHO, 2005). Health systems established through the integrated control of mass drug administration for neglected tropical diseases and expanded global deworming efforts for preschool- and school-aged children (Hotez et al., 2007, 2009) could ultimately provide an infrastructure for linking newly developed vaccines with anthelmintic chemotherapy.
Acknowledgements
The authors acknowledge the support of the Bill & Melinda Gates Foundation, the National Health and Medical Research Council of Australia, and support from M. Hyman, C. Hyman, R. Zuckerberg and the Blavatnik Charitable Trust.
Competing Interests Statement
The authors declare no competing financial interests.
References
- Ajanga A, et al. Schistosoma mansoni in pregnancy and associations with anaemia in northwest Tanzania. Trans. R. Soc. Trop. Med. Hyg. 2006;100:59–63. [PubMed: 16219330]
- Albonico M, et al. Rate of reinfection with intestinal nematodes after treatment of children with mebendazole or albendazole in a highly endemic area. Trans. R. Soc. Trop. Med. Hyg. 1995;89:538–541. [PubMed: 8560535]
- Albonico M, et al. Efficacy of mebendazole and levamisole alone or in combination against intestinal nematode infections after repeated targeted mebendazole treatment in Zanzibar. Bull. World Health Organ. 2003;81:343–352. [PMC free article: PMC2572452] [PubMed: 12856052]
- Andrade ZA. Schistosomiasis and liver fibrosis. Parasite Immunol. 2009;31:656–663. [PubMed: 19825105]
- Asaolu SO, Ofoezie IE. The role of health education and sanitation in the control of helminth infections. Acta Trop. 2003;86:283–294. [PubMed: 12745145]
- Asojo OA, et al. X-ray structures of Na-GST-1 and Na-GST-2 two glutathione s-transferase from the human hookworm Necator americanus. BMC Struct. Biol. 2007;7:42. [PMC free article: PMC1924862] [PubMed: 17594497]
This work describes the crystal structure of the leading hookworm candidate vaccine antigen GST1, revealing it to exist as a homodimer with a cavity in which ligands such as haeme can potentially be bound and thereby detoxified.
- Bentwich Z, Teicher CL, Borkow G. The helminth HIV connection: time to act. AIDS. 2008;22:1611–1614. [PubMed: 18670220]
- Bergquist R, Utzinger J, McManus DP. Trick or treat: the role of vaccines in integrated schistosomiasis control. PLoS Negl. Trop. Dis. 2008;2:e244. [PMC free article: PMC2430529] [PubMed: 18575619]
- Bethony J, et al. Emerging patterns of hookworm infection: influence of aging on the intensity of Necator infection in Hainan Province, People's Republic of China. Clin. Infect. Dis. 2002;35:1336–1344. [PubMed: 12439796]
- Bethony J, et al. Antibodies against a secreted protein from hookworm larvae reduce the intensity of hookworm infection in humans and vaccinated laboratory animals. FASEB J. 2005;19:1743–1745. [PubMed: 16037096]
- Bethony J, et al. Soil-transmitted helminth infections: ascariasis, trichuriasis, and hookworm. Lancet. 2006;367:1521–1532. [PubMed: 16679166]
This paper provides a comprehensive overview of the life cycles, epidemiology, global disease burden, clinical manifestations and immunology of soil-transmitted helminth infections.
- Bethony JM, Loukas A, Hotez PJ, Knox DP. Vaccines against blood-feeding nematodes of humans and livestock, Parasitology. Vol. 133. 2006. pp. S63–S79. [PubMed: 17274849]
A summary of the progress that has been made towards vaccine development using defined larval-stage and adult-stage antigens against hookworms in humans and against H. contortus in livestock, as well as the anticipated impact of the host immune response on vaccine design.
- Bleakley H. Disease and development: evidence from hookworm eradication in the American South. Q. J. Econ. 2007;122:73–117. [PMC free article: PMC3800113] [PubMed: 24146438]
- Bottazzi ME, Brown AS. Model for product development of vaccines against neglected tropical diseases: a vaccine against human hookworm. Expert Rev. Vaccines. 2008;7:1481–1492. [PubMed: 19053205]
- Braschi S, Wilson RA. Proteins exposed at the adult schistosome surface revealed by biotinylation. Mol. Cell. Proteomics. 2006;5:347–356. [PubMed: 16269422]
- Brito LL, et al. Moderate- and low-intensity co-infections by intestinal helminths and Schistosoma mansoni, dietary iron intake, and anemia in Brazilian children. Am. J. Trop. Med. Hyg. 2006;75:939–944. [PubMed: 17123992]
- Brooker S, et al. The epidemiology of hookworm infection and its contribution to anaemia among pre-school children on the Kenyan coast. Trans. R. Soc. Trop. Med. Hyg. 1999;93:240–246. [PubMed: 10492749]
- Brooker S, Bethony J, Hotez PJ. Human hookworm infection in the 21st century. Adv. Parasitol. 2004;58:197–288. [PMC free article: PMC2268732] [PubMed: 15603764]
- Brooker S, et al. Age-related changes in hookworm infection, anaemia and iron deficiency in an area of high Necator americanus hookworm transmission in south-eastern Brazil. Trans. R. Soc. Trop. Med. Hyg. 2007;101:146–154. [PubMed: 17027054]
- Brooker S, et al. Epidemiology of plasmodiumhelminth co-infection in Africa: populations at risk, potential impact on anemia, and prospects for combining control. Am. J. Trop. Med. Hyg. 2007;77:88–98. [PMC free article: PMC2637949] [PubMed: 18165479]
- Brooker S, Hotez PJ, Bundy DA. Hookworm-related anaemia among pregnant women: a systematic review. PLoS Negl. Trop. Dis. 2008;2:e291. [PMC free article: PMC2553481] [PubMed: 18820740]
- Brophy PM, Pritchard DI. Metabolism of lipid peroxidation products by the gastro-intestinal nematodes Necator americanus, Ancylostoma ceylanicum and Heligmosomoides polygyrus. Int. J. Parasitol. 1992;22:1009–1012. [PubMed: 1459777]
- Calis JC, et al. Severe anemia in Malawian children. N. Engl. J. Med. 2008;358:888–899. [PubMed: 18305266]
- Capron A, Riveau G, Capron M, Trottein F. Schistosomes: the road from host–parasite interactions to vaccines in clinical trials. Trends Parasitol. 2005;21:143–149. [PubMed: 15734662]
- Cardoso FC, Pacifico RN, Mortara RA, Oliveira SC. Human antibody responses of patients living in endemic areas for schistosomiasis to the tegumental protein Sm29 identified through genomic studies. Clin. Exp. Immunol. 2006;144:382–391. [PMC free article: PMC1941986] [PubMed: 16734606]
- Cardoso FC, et al. Schistosoma mansoni tegument protein Sm29 is able to induce a Th1-type of immune response and protection against parasite infection. PLoS Negl. Trop. Dis. 2008;2:e308. [PMC free article: PMC2553283] [PubMed: 18827884]
- Chenine AL, et al. Acute Schistosoma mansoni infection increases susceptibility to systemic SHIV clade C infection in rhesus macaques after mucosal virus exposure. PLoS Negl. Trop. Dis. 2008;2:e265. [PMC free article: PMC2447882] [PubMed: 18648516]
- Cohen MS, Hellmann N, Levy JA, DeCock K, Lange J. The spread, treatment, and prevention of HIV-1: evolution of a global pandemic. J. Clin. Invest. 2008;118:1244–1254. [PMC free article: PMC2276790] [PubMed: 18382737]
- Correa-Oliveira R, Caldas IR, Gazzinelli G. Natural versus drug-induced resistance in Schistosoma mansoni infection. Parasitol. Today. 2000;16:397–399. [PubMed: 10951600]
- Crompton DW. The public health importance of hookworm disease. Parasitology. 2000;121:S39–S50. [PubMed: 11386690]
- Da'dara AA, Harn DA. Elimination of helminth infection restores HIV-1C vaccine-specific T cell responses independent of helminth-induced IL-10. Vaccine. 2010;28:1310–1317. [PMC free article: PMC2831209] [PubMed: 19941988]
- Da'dara AA, et al. DNA-based vaccines protect against zoonotic schistosomiasis in water buffalo. Vaccine. 2008;26:3617–3625. [PMC free article: PMC2567122] [PubMed: 18524429]
- Demissie F, Kebede A, Shimels T, Beyene P. Assessment of public health implication of malariageohelminth co-infection with an emphasis on hookworm-malaria anemia among suspected malaria patients in Asendabo, southwest Ethiopia. Ethiop. Med. J. 2009;47:153–158. [PubMed: 19743795]
- Deponte M, Becker K. Glutathione S-transferase from malarial parasites: structural and functional aspects. Methods Enzymol. 2005;401:241–253. [PubMed: 16399390]
- de Silva NR, et al. Soil-transmitted helminth infections: updating the global picture. Trends Parasitol. 2003;19:547–551. [PubMed: 14642761]
- Diemert DJ, Bethony JM, Hotez PJ. Hookworm vaccines. Clin. Infect. Dis. 2008;46:282–288. [PubMed: 18171264]
This article discusses the feasibility of developing a hookworm vaccine on the basis of the previous success of an attenuated larval vaccine against the canine hookworm A. caninum. It also summarizes potential protective mechanisms, the stability of potential candidate antigens and the results of clinical testing of the first human hookworm candidate vaccine antigen, ASP2.
- Dreyfuss ML, et al. Hookworms, malaria and vitamin A deficiency contribute to anemia and iron deficiency among pregnant women in the plains of Nepal. J. Nutr. 2000;130:2527–2536. [PubMed: 11015485]
- Erb KJ. Helminths, allergic disorders and IgEmediated immune responses: where do we stand? Eur. J. Immunol. 2007;37:1170–1173. [PubMed: 17447233]
- Ezeamama AE, et al. The synergistic effect of concomitant schistosomiasis, hookworm, and trichuris infections on children's anemia burden. PLoS Negl. Trop. Dis. 2008;2:e245. [PMC free article: PMC2390851] [PubMed: 18523547]
- Finkelman FD, et al. Interleukin-4- and interleukin-13-mediated host protection against intestinal nematode parasites. Immunol. Rev. 2004;201:139–155. [PubMed: 15361238]
- Fitzpatrick JM, et al. Anti-schistosomal intervention targets identified by lifecycle transcriptomic analyses. PLoS Negl. Trop. Dis. 2009;3:e543. [PMC free article: PMC2764848] [PubMed: 19885392]
- Fleming FM, et al. Synergistic associations between hookworm and other helminth species in a rural community in Brazil. Trop. Med. Int. Health. 2006;11:56–64. [PubMed: 16398756]
- Food and Agriculture Organization (FAO) & WHO. Human Vitamin and Mineral Requirements: Report of a Joint FAO/WHO Expert Consultation. FAO/WHO; Rome: 2002. pp. 113–221.
- Friedman JF, et al. Relationship between Schistosoma japonicum and nutritional status among children and young adults in Leyte, the Philippines. Am. J. Trop. Med. Hyg. 2005;72:527–533. [PubMed: 15891125]
- Friedman JF, Kanzaria HK, McGarvey ST. Human schistosomiasis and anemia: the relationship and potential mechanisms. Trends Parasitol. 2005;21:386–392. [PubMed: 15967725]
This summarizes the animal and human data about the impact of schistosomiasis on anaemia and discusses four possible mechanisms: extracorporeal blood loss, autoimmune haemolysis and anaemia of inflammation, and splenomegaly with erythrocyte sequestration.
- Friedman JF, Mital P, Kanzaria HK, Olds GR, Kurtis JD. Schistosomiasis and pregnancy. Trends Parasitol. 2007;23:159–164. [PubMed: 17336160]
- Friis H, et al. Effects on haemoglobin of multimicronutrient supplementation and multi-helminth chemotherapy: a randomized, controlled trial in Kenyan school children. Eur. J. Clin. Nutr. 2003;57:573–579. [PubMed: 12700619]
- Fujiwara RT, et al. Immunogenicity of the hookworm Na-ASP-2 vaccine candidate: characterization of humoral and cellular responses after vaccination in the Sprague Dawley rat. Hum. Vaccin. 2005;1:123–128. [PubMed: 17012856]
- Fujiwara RT, et al. Vaccination with irradiated Ancylostoma caninum third stage larvae induces a TH2 protective response in dogs. Vaccine. 2006;24:501–509. [PubMed: 16140437]
- Geary TG, et al. Unresolved issues in anthelmintic pharmacology for helminthiases of humans. Int. J. Parasitol. 2010;40:1–13. [PubMed: 19932111]
- Geerts S, Gryseels B. Drug resistance in human helminths: current situation and lessons from livestock. Clin. Microbiol. Rev. 2000;13:207–222. [PMC free article: PMC100151] [PubMed: 10755998]
- Geldhof P, Knox D. The intestinal contortin structure in Haemonchus contortus: An immobilized anticoagulant? Int. J. Parasitol. 2008;38:1579–1588. [PubMed: 18599060]
- Gobert GN, et al. Transcriptional changes in Schistosoma mansoni during early schistosomula development and in the presence of erythrocytes. PLoS Negl. Trop. Dis. 2010;4:e600. [PMC free article: PMC2817720] [PubMed: 20161728]
- Goud GN, et al. Expression of the Necator americanus hookworm larval antigen Na-ASP-2 in Pichia pastoris and purification of the recombinant protein for use in human clinical trials. Vaccine. 2005;23:4754–4764. [PubMed: 16054275]
- Greenwood BM, et al. Malaria: progress, perils, and prospects for eradication. J. Clin. Invest. 2008;118:1266–1276. [PMC free article: PMC2276780] [PubMed: 18382739]
- Gryseels B, Polman K, Clerinx J, Kestens L. Human schistosomiasis. Lancet. 2006;368:1106–1118. [PubMed: 16997665]
- Guyatt HL, Brooker S, Kihamia CM, Hall A, Bundy DA. Evaluation of efficacy of school-based anthelmintic treatments against anaemia in children in the United Republic of Tanzania. Bull. World Health Organ. 2001;79:695–703. [PMC free article: PMC2566500] [PubMed: 11545325]
- Hotez PJ. Mass drug administration and integrated control for the world's high-prevalence neglected tropical diseases. Clin. Pharmacol. Ther. 2009;85:659–664. [PubMed: 19322166]
- Hotez PJ, Brown AS. Neglected tropical disease vaccines. Biologicals. 2009;37:160–164. [PubMed: 19278869]
- Hotez PJ, Ferris MT. The antipoverty vaccines. Vaccine. 2006;24:5787–5799. [PubMed: 16759763]
- Hotez PJ, Kamath A. Neglected tropical diseases in sub-Saharan Africa: review of their prevalence, distribution, and disease burden. PLoS Negl. Trop. Dis. 2009;3:e412. [PMC free article: PMC2727001] [PubMed: 19707588]
- Hotez PJ, et al. Hookworm infection. N. Engl. J. Med. 2004;351:799–807. [PubMed: 15317893]
- Hotez PJ, Bethony J, Bottazzi ME, Brooker S, Buss P. Hookworm: “the great infection of mankind” PLoS Med. 2005;2:e67. [PMC free article: PMC1069663] [PubMed: 15783256]
- Hotez PJ, et al. Incorporating a rapid-impact package for neglected tropical diseases with programs for HIV/AIDS, tuberculosis, and malaria. PLoS Med. 2006;3:e102. [PMC free article: PMC1351920] [PubMed: 16435908]
- Hotez PJ, et al. Control of neglected tropical diseases. N. Engl. J. Med. 2007;357:1018–1027. [PubMed: 17804846]
- Hotez PJ, et al. Helminth infections: the great neglected tropical diseases. J. Clin. Invest. 2008;118:1311–1321. [PMC free article: PMC2276811] [PubMed: 18382743]
- Hotez PJ, Bethony JM, Oliveira SC, Brindley PJ, Loukas A. Multivalent anthelminthic vaccine to prevent hookworm and schistosomiasis. Expert Rev. Vaccines. 2008;7:745–752. [PubMed: 18665774]
- Hotez PJ, Bottazzi ME, Franco-Paredes C, Ault SK, Periago MR. The neglected tropical diseases of Latin America and the Caribbean: a review of disease burden and distribution and a roadmap for control and elimination. PLoS Negl. Trop. Dis. 2008;2:e300. [PMC free article: PMC2553488] [PubMed: 18820747]
- Hotez PJ, Fenwick A, Savioli L, Molyneux DH. Rescuing the bottom billion through control of neglected tropical diseases. Lancet. 2009;373:1570–1575. [PubMed: 19410718]
- Jani D, et al. HDP—a novel heme detoxification protein from the malaria parasite. PLoS Pathog. 2008;4:e1000053. [PMC free article: PMC2291572] [PubMed: 18437218]
- Jiz M, et al. Pilot-scale production and characterization of paramyosin, a vaccine candidate for schistosomiasis japonica. Infect. Immun. 2008;76:3164–3169. [PMC free article: PMC2446706] [PubMed: 18426875]
- Keiser J, Utzinger J. Efficacy of current drugs against soil-transmitted helminth infections: systematic review and meta-analysis. JAMA. 2008;299:1937–1948. [PubMed: 18430913]
- King CH. Parasites and poverty: the case of schistosomiasis. Acta Trop. 2010;113:95–104. [PMC free article: PMC2812649] [PubMed: 19962954]
- King CH, Dangerfield-Cha M. The unacknowledged impact of chronic schistosomiasis. Chronic Illn. 2008;4:65–79. [PubMed: 18322031]
- King CH, Dickman K, Tisch DJ. Reassessment of the cost of chronic helmintic infection: a metaanalysis of disability-related outcomes in endemic schistosomiasis. Lancet. 2005;365:1561–1569. [PubMed: 15866310]
- King CH, Sturrock RF, Kariuki HC, Hamburger J. Transmission control for schistosomiasis — why it matters now. Trends Parasitol. 2006;22:575–582. [PubMed: 17030017]
- Kjetland EF, et al. Association between genital schistosomiasis and HIV in rural Zimbabwean women. AIDS. 2006;20:593–600. [PubMed: 16470124]
- Knox DP, et al. The nature and prospects for gut membrane proteins as vaccine candidates for Haemonchus contortus and other ruminant trichostrongyloids. Int. J. Parasitol. 2003;33:1129–1137. [PubMed: 13678629]
- Koukounari A, et al. Morbidity indicators of Schistosoma mansoni: relationship between infection and anemia in Ugandan schoolchildren before and after praziquantel and albendazole chemotherapy. Am. J. Trop. Med. Hyg. 2006;75:278–286. [PubMed: 16896133]
- Koukounari A, et al. Schistosoma haematobium infection and morbidity before and after large-scale administration of praziquantel in Burkina Faso. J. Infect. Dis. 2007;196:659–669. [PubMed: 17674306]
- Koukounari A, et al. Relationships between anaemia and parasitic infections in Kenyan schoolchildren: a Bayesian hierarchical modelling approach. Int. J. Parasitol. 2008;38:1663–1671. [PMC free article: PMC2649416] [PubMed: 18621051]
- Larocque R, Casapia M, Gotuzzo E, Gyorkos TW. Relationship between intensity of soil-transmitted helminth infections and anemia during pregnancy. Am. J. Trop. Med. Hyg. 2005;73:783–789. [PubMed: 16222026]
- Lightowlers MW. Cestode vaccines: origins, current status and future prospects. Parasitology. 2006;133:S27–S42. [PubMed: 17274847]
- Loukas A, et al. Vaccination with recombinant aspartic hemoglobinase reduces parasite load and blood loss after hookworm infection in dogs. PLoS Med. 2005;2:e295. [PMC free article: PMC1240050] [PubMed: 16231975]
This investigation finds that vaccination of dogs with recombinant A. caninum APR1 results in protection from anaemia, the major clinical manifestation of hookworm, after challenge with infective A. caninum larvae.
- Loukas A, Bethony J, Brooker S, Hotez P. Hookworm vaccines: past, present, and future. Lancet Infect. Dis. 2006;6:733–741. [PubMed: 17067922]
- Loukas A, Tran M, Pearson MS. Schistosome membrane proteins as vaccines. Int. J. Parasitol. 2007;37:257–263. [PubMed: 17222846]
This article describes the tetraspanin family of integral membrane proteins that are abundantly represented in the outermost surface of Schistosoma spp., and discusses the evidence from a mouse vaccine model and from human immunoepidemiology studies that indicate the potential of tetraspanins as vaccine candidates.
- Lwambo NJ, Siza JE, Brooker S, Bundy DA, Guyatt H. Patterns of concurrent hookworm infection and schistosomiasis in schoolchildren in Tanzania. Trans. R. Soc. Trop. Med. Hyg. 1999;93:497–502. [PubMed: 10696404]
- Maizels RM, Holland MJ, Falcone FH, Zang XX, Yazdanbakhsh M. Vaccination against helminth parasites - the ultimate challenge for vaccinologists. Immunol. Rev. 1999;171:125–147. [PubMed: 10582168]
- Maizels RM, et al. Helminth parasites – masters of regulation. Immunol. Rev. 2004;201:89–116. [PubMed: 15361235]
This paper reviews the immunoregulation induced by helminth infections, which is dominated by the TH2 phenotype and the selective loss of effector activity against a background of regulatory T cells, TH2-inducing dendritic cells and alternatively activated macrophages.
- McKeand JB. Vaccine development and diagnostics of Dictyocaulus viviparus. Parasitology. 2000;120:S17–S23. [PubMed: 10874707]
- McManus DP, Loukas A. Current status of vaccines for schistosomiasis. Clin. Microbiol. Rev. 2008;21:225–242. [PMC free article: PMC2223839] [PubMed: 18202444]
- Meissner B, Boll M, Daniel H, Baumeister R. Deletion of the intestinal peptide transporter affects insulin and TOR signaling in Caenorhabditis elegans. J. Biol. Chem. 2004;279:36739–36745. [PubMed: 15155758]
- Miller TA. Industrial development and field use of the canine hookworm vaccine. Adv. Parasitol. 1978;16:333–342. [PubMed: 364958]
- Moore RD. Anemia and human immunodeficiency virus disease in the era of highly active antiretroviral therapy. Semin. Hematol. 2000;37:18–23. [PubMed: 11068952]
- Moraes LR, Cancio JA, Cairncross S. Impact of drainage and sewerage on intestinal nematode infections in poor urban areas in Salvador, Brazil. Trans. R. Soc. Trop. Med. Hyg. 2004;98:197–204. [PubMed: 15049458]
- Morel CM, et al. Health innovation networks to help developing countries address neglected diseases. Science. 2005;309:401–404. [PubMed: 16020723]
- Moser D, Tendler M, Griffiths G, Klinkert MQ. A 14-kDa Schistosoma mansoni polypeptide is homologous to a gene family of fatty acid binding proteins. J. Biol. Chem. 1991;266:8447–8454. [PubMed: 2022660]
- Nguyen PH, et al. Risk factors for anemia in Vietnam. Southeast Asian J. Trop. Med. Public Health. 2006;37:1213–1223. [PubMed: 17333780]
- Oliveira SC, Fonseca CT, Cardoso FC, Farias LP, Leite LC. Recent advances in vaccine research against schistosomiasis in Brazil. Acta Trop. 2008;108:256–262. [PubMed: 18577363]
- Olsen A. Efficacy and safety of drug combinations in the treatment of schistosomiasis, soil-transmitted helminthiasis, lymphatic filariasis and onchocerciasis. Trans. R. Soc. Trop. Med. Hyg. 2007;101:747–758. [PubMed: 17481681]
- Pearson MS, et al. An enzymatically inactivated hemoglobinase from Necator americanus induces neutralizing antibodies against multiple hookworm species and protects dogs against heterologous hookworm infection. FASEB J. 2009;23:3007–3019. [PMC free article: PMC2735369] [PubMed: 19380510]
This study shows that vaccination of dogs with an N. americanus APR1 in which the catalytic aspartic acid residues are mutagenized results in substantially reduced parasite egg burdens after challenge with infective larvae of A. caninum, and induces antibodies that bind the native enzyme in the parasite gut, neutralizing its enzymatic activity.
- Pearson MS, et al. Neutralizing antibodies to the hookworm hemoglobinase Na-APR-1: implications for a multivalent vaccine against hookworm infection and schistosomiasis. J. Infect. Dis. 2010;201:1561–1569. [PubMed: 20367477]
- Ploeger HW. Dictyocaulus viviparus: re-emerging or never been away? Trends Parasitol. 2002;18:329–332. [PubMed: 12377272]
- Prual A, et al. Consequences of Schistosoma haematobium infection on the iron status of schoolchildren in Niger. Am. J. Trop. Med. Hyg. 1992;47:291–297. [PubMed: 1524142]
- Ramos CR, et al. Stability improvement of the fatty acid binding protein Sm14 from S. mansoni by Cys replacement: structural and functional characterization of a vaccine candidate. Biochim. Biophys. Acta. 2009;1794:655–662. [PubMed: 19150418]
- Ranjit N, Jones MK, Stenzel DJ, Gasser RB, Loukas A. A survey of the intestinal transcriptomes of the hookworms, Necator americanus and Ancylostoma caninum, using tissues isolated by laser microdissection microscopy. Int. J. Parasitol. 2006;36:701–710. [PubMed: 16545815]
- Ranjit N, et al. Proteolytic degradation of hemoglobin in the intestine of the human hookworm Necator americanus. J. Infect. Dis. 2009;199:904–912. [PubMed: 19434933]
- Raso G, et al. An integrated approach for risk profiling and spatial prediction of Schistosoma mansoni–hookworm coinfection. Proc, Natl Acad. Sci. USA. 2006;103:6934–6939. [PMC free article: PMC1458997] [PubMed: 16632601]
- Rowe AK, et al. The burden of malaria mortality among African children in the year 2000. Int. J. Epidemiol. 2006;35:691–704. [PMC free article: PMC3672849] [PubMed: 16507643]
- Schuller DJ, et al. Crystal structure of a new class of glutathione transferase from the model human hookworm nematode Heligmosomoides polygyrus. Proteins. 2005;61:1024–1031. [PubMed: 16189827]
- Smith JL, Brooker S. Impact of hookworm infection and deworming on anaemia in non-pregnant populations: a systematic review. Trop. Med. Int. Health. 2010;15:776–795. [PMC free article: PMC2916221] [PubMed: 20500563]
- Snow RW, Guerra CA, Noor AM, Myint HY, Hay SI. The global distribution of clinical episodes of Plasmodium falciparum malaria. Nature. 2005;434:214–217. [PMC free article: PMC3128492] [PubMed: 15759000]
- Sousa-Figueiredo JC, et al. A parasitological survey, in rural Zanzibar, of pre-school children and their mothers for urinary schistosomiasis, soil-transmitted helminthiases and malaria, with observations on the prevalence of anaemia. Ann. Trop. Med. Parasitol. 2008;102:679–692. [PubMed: 19000385]
- Steinmann P, Keiser J, Bos R, Tanner M, Utzinger J. Schistosomiasis and water resources development: systematic review, meta-analysis, and estimates of people at risk. Lancet Infect. Dis. 2006;6:411–425. [PubMed: 16790382]
- Stephenson L. The impact of schistosomiasis on human nutrition. Parasitology. 1993;107:S107–S123. [PubMed: 8115176]
- Stephenson LS. Helminth parasites, a major factor in malnutrition. World Health Forum. 1994;15:169–172. [PubMed: 8018283]
- Stephenson LS, et al. Relationships of Schistosoma haematobium, hookworm and malarial infections and metrifonate treatment to growth of Kenyan school children. Am. J. Trop. Med. Hyg. 1985;34:1109–1118. [PubMed: 3938925]
- Steves FE, Baker JD, Hein VD, Miller TA. Efficacy of a hookworm (Ancylostoma caninum) vaccine for dogs. J. Am. Vet. Med. Assoc. 1973;163:231–235. [PubMed: 4721752]
- Stoll NR. This wormy world. J. Parasitol. 1947;33:1–18. [PubMed: 20284977]
- Stoltzfus RJ. Iron deficiency: global prevalence and consequences. Food Nutr. Bull. 2003;24:S99–S103. [PubMed: 17016951]
- Stoltzfus RJ, Dreyfuss ML, Chwaya HM, Albonico M. Hookworm control as a strategy to prevent iron deficiency. Nutr. Rev. 1997;55:223–232. [PubMed: 9279058]
- Sturrock RF, et al. Schistosomiasis mansoni in Kenya: relationship between infection and anaemia in schoolchildren at the community level. Trans. R. Soc. Trop. Med. Hyg. 1996;90:48–54. [PubMed: 8730312]
- Tendler M, Simpson AJ. The biotechnology-value chain: development of Sm14 as a schistosomiasis vaccine. Acta Trop. 2008;108:263–266. [PubMed: 18834847]
- Tissenbaum HA, et al. A common muscarinic pathway for diapause recovery in the distantly related nematode species Caenorhabditis elegans and Ancylostoma caninum. Proc. Natl Acad. Sci. USA. 2000;97:460–465. [PMC free article: PMC26685] [PubMed: 10618440]
- Tohon ZB, et al. Controlling schistosomiasis: significant decrease of anaemia prevalence one year after a single dose of praziquantel in Nigerian schoolchildren. PLoS Negl. Trop. Dis. 2008;2:e241. [PMC free article: PMC2386241] [PubMed: 18509472]
- Tolentino K, Friedman JF. An update on anemia in less developed countries. Am. J. Trop. Med. Hyg. 2007;77:44–51. [PubMed: 17620629]
- Tran MH, et al. Tetraspanins on the surface of Schistosoma mansoni are protective antigens against schistosomiasis. Nature Med. 2006;12:835–840. [PubMed: 16783371]
- Tran MH, et al. Suppression of mRNAs encoding tegument tetraspanins from Schistosoma mansoni results in impaired tegument turnover. PLoS Pathog. 2010;6:e1000840. [PMC free article: PMC2855321] [PubMed: 20419145]
- Urquhart GM. Field experience with the bovine lungworm vaccine. Dev. Biol. Stand. 1985;62:109–112. [PubMed: 2938996]
- van Rossum AJ, et al. Binding of hematin by a new class of glutathione transferase from the blood-feeding parasitic nematode Haemonchus contortus. Infect. Immun. 2004;72:2780–2790. [PMC free article: PMC387910] [PubMed: 15102788]
- Veerapathran A, Dakshinamoorthy G, Gnanasekar M, Reddy MV, Kalyanasundaram R. Evaluation of Wuchereria bancrofti GST as a vaccine candidate for lymphatic filariasis. PLoS Negl. Trop. Dis. 2009;3:e457. [PMC free article: PMC2685978] [PubMed: 19513102]
- Vercruysse J, Knox DP, Schetters TP, Willadsen P. Veterinary parasitic vaccines: pitfalls and future directions. Trends Parasitol. 2004;20:488–492. [PubMed: 15363443]
- WHO. Iron deficiency anaemia: assessment, prevention and control, A guide for programme managers. WHO; Geneva: 2001.
- WHO. World health report 2004: changing history. WHO; Geneva: 2004.
- WHO. Deworming for Health and Development. WHO; Geneva: 2005. p. 27.
- WHO. Preventive chemotherapy in human helminthiasis: coordinated use of anthelminthic drugs in control interventions: a manual for health professionals and programme managers. WHO; Geneva: 2006.
- WHO. Soil-transmitted helminthiasis. Progress report on number of children treated with anthelminthic drugs: an update towards the 2010 global target. Wkly Epidemiol. Rec. 2008;82:237–252. [PubMed: 18613343]
- WHO Expert Committee. Prevention and control of schistosomiasis and soil-transmitted helminthiasis. World Health Organ Tech. Rep. Ser. 2002;912:1–57. [PubMed: 12592987]
- Williamson AL, et al. A multi-enzyme cascade of hemoglobin proteolysis in the intestine of blood-feeding hookworms. J. Biol. Chem. 2004;279:35950–35957. [PubMed: 15199048]
- Wilson MS, et al. Immunopathology of schistosomiasis. Immunol. Cell Biol. 2007;85:148–154. [PMC free article: PMC3437548] [PubMed: 17160074]
This article discusses how the granulomatous response to S. mansoni and S. japonicum eggs develops in the liver, as well as how regulatory cells and cytokine decoy receptors limit the extent of the immunopathology associated with these infections.
- Xiao S, et al. The evaluation of recombinant hookworm antigens as vaccines in hamsters (Mesocricetus auratus) challenged with human hookworm, Necator americanus. Exp. Parasitol. 2008;118:32–40. [PubMed: 17645877]
This article describes the protective effects of several candidate hookworm vaccine antigens, including N. americanus ASP2, A. caninum APR1 and A. caninum GST1, in hamsters challenged with infective N. americanus larvae following vaccination.
- Yuan C, et al. Schistosoma japonicum: Efficient and rapid purification of the tetraspanin extracellular loop 2, a potential protective antigen against schistosomiasis in mammalian. Exp. Parasitol. 2010;126:456–461. [PubMed: 20685202]
- Yusibov V, Rabindran S. Recent progress in the development of plant derived vaccines, Expert Rev. Vaccines. Vol. 7. 2008. pp. 1173–1183. [PubMed: 18844592]
- Zhan B, et al. Molecular cloning and purification of Ac-TMP, a developmentally regulated putative tissue inhibitor of metalloprotease released in relative abundance by adult Ancylostoma hookworms. Am. J. Trop Med. Hyg. 2002;66:238–244. [PubMed: 12139214]
- Zhan B, et al. Biochemical characterization and vaccine potential of a heme-binding glutathione transferase from the adult hookworm Ancylostoma caninum. Infect. Immun. 2005;73:6903–6911. [PMC free article: PMC1230892] [PubMed: 16177370]
- Zhan B, et al. Molecular cloning, biochemical characterization, and partial protective immunity of the heme-binding glutathione S-transferases from the human hookworm Necator americanus. Infect. Immun. 2010;78:1552–1563. [PMC free article: PMC2849424] [PubMed: 20145100]
This work documents the expression of recombinant GST proteins of N. americanus in P. pastoris, the characterization of these proteins and their effectiveness in preventing infection in a hamster vaccination–challenge model.
- Zhang W, et al. Sm-p80-based DNA vaccine provides baboons with levels of protection against Schistosoma mansoni infection comparable to those achieved by the irradiated cercarial vaccine. J. Infect. Dis. 2010;201:1105–1112. [PMC free article: PMC2922992] [PubMed: 20187746]
Footnotes
- 9
Reprinted from Hotez, P. J., Bethony, J. M., Diemert, D. J., Pearson M., and Loukas, A. 2010. Developing vaccines to combat hookworm infection and intestinal schistosomiasis. Nature reviews Microbiol 8(11):814-26, with permission from Nature Publishing Group.
- 10
Department of Microbiology, Immunology, and Tropical Medicine, George Washington University, Washington, DC 20037, and Sabin Vaccine Institute, Washington, DC 20037, USA.
- 11
Instituto René Rachou, Oswaldo Cruz Foundation, Belo Horizonte, 30190-002, Brazil.
- 12
Queensland Tropical Health Alliance, James Cook University, Cairns, QLD 4878, Australia. Correspondence to P.J.H. e-mail: ude.cmuwg@hjpmtm doi:10.1038/nrmicro2438.
- Global Distribution and Pathobiology
- Hookworms, Schistosomes and Anaemia
- Rationale For a Human Hookworm Vaccine
- Targeting Hookworm Blood Feeding
- Antigens of the Human Hookworm Vaccine
- Vaccinating Against Schistosomiasis
- Schistosome Antigens Under Development
- Future Directions
- Acknowledgements
- Competing Interests Statement
- References
- DEVELOPING VACCINES TO COMBAT HOOKWORM INFECTION AND INTESTINAL SCHISTOSOMIASIS ...DEVELOPING VACCINES TO COMBAT HOOKWORM INFECTION AND INTESTINAL SCHISTOSOMIASIS - The Causes and Impacts of Neglected Tropical and Zoonotic Diseases
- glsB [Proteus mirabilis HI4320]glsB [Proteus mirabilis HI4320]Gene ID:6802190Gene
Your browsing activity is empty.
Activity recording is turned off.
See more...