NCBI Bookshelf. A service of the National Library of Medicine, National Institutes of Health.
National Academies of Sciences, Engineering, and Medicine; Division on Earth and Life Studies; Board on Agriculture and Natural Resources; Committee on Nutrient Requirements of Dairy Cattle. Nutrient Requirements of Dairy Cattle: Eighth Revised Edition. Washington (DC): National Academies Press (US); 2021 Aug 30.
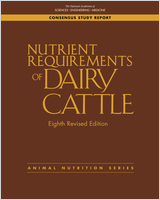
Nutrient Requirements of Dairy Cattle: Eighth Revised Edition.
Show detailsINTRODUCTION
The costs of raising replacement heifers and the impact of heifer growth on lifetime milk production and profits underscore the importance of accurate predictions for heifer nutrient requirements (Tozer and Heinrichs, 2001). The energy and protein requirements for growing heifers are determined primarily by the animals' maintenance requirements, the amount of daily body tissue gain and its composition, and the efficiency of converting feeds to body tissues. Targets for daily gain depend on targets for age and body weight (BW) at breeding and first calving. How heifers are fed can affect not only growth rate and feed costs but also the timing of puberty, the composition of gain, and future milk production.
Improvements Made from the Seventh Revised Edition
The growth requirements in the seventh edition were based on equations developed for beef cattle, which typically have a higher proportion of fat than do dairy breeds. The model contained new terms for its size-scaling approach that made it confusing, and it had mathematical incongruities; for example, gut fill was 14.5 percent of BW but only 4 percent of BW gain. In the past 20 years, new publications have reported body composition for Holstein cattle, and these data were used to develop equations based on Holsteins that are size-scaled for use in other dairy breeds.
Terminology and Relationships for Body Weight and Body Weight Gain
In this edition, the following terms are used to describe growth. BW is the normal live weight of an animal without fasting, and BW gain is the increase in BW over a defined time period such as average daily gain (ADG). Empty BW (EBW) is BW without ingesta, and empty body gain (EBG) is gain without digesta. These cannot be measured easily in a live animal and are estimated as 85 percent of BW for heifers (NRC, 1989) and supported by Waldo et al. (1997).
(Equation 11-1a)
(Equation 11-1b)
The committee recognizes that the mass of ingesta, or gut fill, is not a constant function of BW; rather, gut fill is generally a function of feed intake and digestion kinetics and can be considerably different for heifers fed a poorly digestible diet ad libitum than for heifers fed a highly digestible diet at restricted intake. To date, no solutions seem adequate to accurately predict gut fill. Thus, the committee decided to use a constant value of 15 percent, with the recognition that this value is not adequate for all situations; for example, in Waldo et al. (1997), change in gut fill ranged from 11 percent of ADG for heifers gaining 1,000 g/d on a corn silage–based diet to 19 percent of ADG for heifers gaining 770 g/d on an alfalfa-based diet. Further work is needed.
ENERGY AND PROTEIN REQUIREMENTS FOR GROWING DAIRY HEIFERS
Setting energy and protein requirements for growing heifers requires quantitative estimates for maintenance requirements and composition of gain as heifers mature, for the effects of diet on structural growth and milk production potential, and for the efficiency of metabolic conversions.
Maintenance Requirements
The maintenance energy requirement for heifers was set on a metabolizable energy (ME) basis as follows:
This matches the maintenance requirement for cows and is similar to the value used by the beef NASEM (2016) for dairy breeds of 0.095 × BW0.75 on a net energy (NE) basis. Activity is assumed to be 10 percent of the requirement; heifers in large dry lots or on pasture may be more active and thus have greater maintenance requirements. Adjustments for compensatory growth, body condition score (BCS), or previous temperature were not included. More data are needed to refine maintenance estimates. Equations to generate the maintenance requirement for metabolizable protein (MP) are the same as for adult cows (see Chapter 6) and include scurf, endogenous urinary loss, and metabolic fecal losses. The efficiency of converting MP to net protein (NP) was assumed to equal the target efficiency of 0.69 for scurf and metabolic fecal protein (MFP) and 1.0 for endogenous urinary nitrogen (N) (see Chapter 6 for details). Although some of these losses are as nitrogen rather than protein or amino acids, all values are put on a crude protein (CP) basis (N × 6.25).
(Equation 11-3a)
(Equation 11-3b)
(Equation 11-3c)
The Composition of Gain and Growth Requirements
The NE required for growth is defined as the energy retained in body tissues during growth and is a function of the proportion of retained fat and protein (Garrett et al., 1959). As animals mature, the percentage of protein diminishes and the percentage of fat increases in the empty body, and chemical maturity is achieved when weight gain contains little protein and is mostly fat. Simpfendorfer (1974) summarized data on the body composition of growing cattle from birth to maturity; within cattle of similar mature size, 96 to 99 percent of the variation in chemical composition was associated with differences in BW.
Previous committees on dairy and beef cattle nutrition (e.g., NRC, 2001; NASEM, 2016) adopted the equation developed by Garrett (1980) to predict the energy content of weight gain. Garrett's data set included 72 comparative slaughter experiments conducted at the University of California between 1960 and 1980 with approximately 3,500 cattle (predominantly British breed beef steers) fed a variety of diets. The Garrett equation describes the relationship between retained energy (RE) and EBG for a given EBW and the composition of EBW gain at a particular stage of growth in cattle. Because the BW at which cattle reach a given chemical composition varies depending on mature size and sex, body composition may differ among animals of similar BW (NRC, 1996). Thus, most systems to predict body composition and nutrient requirements in the past 20 years have used a size-scaling approach to account for differences in composition at a specific BW that are due to differences in mature BW (MatBW). The size-scaling approach adopted by NRC (2001) involved calculation of the relationship between an animal's current BW, its MatBW, and a standard reference weight. The MatBW of the standard reference animal (NRC, 2001) was 500 kg and defined as the weight at which skeletal development is complete and the empty body contains 25 percent fat corresponding to a BCS of 3 on a 1 to 5 scale.
In the past 20 years, several studies have measured body composition for Holstein cattle. Based on a meta-analysis of 26 studies with 129 treatment means on Holsteins, de Souza and VandeHaar (2018) showed that the equations of the seventh edition generally underestimated the fat content of the empty body and the RE per kilogram of gain in young heifers and overestimated the fat content of the empty body and the RE per kilogram of gain in older heifers. Moreover, the fat content of mature Holsteins at a body condition of 3 on a 5-point scale was 22 percent, not 25 percent. Part of the reason for underestimating the fat content of young heifers was that in NRC (2001), the energy content of gain was assumed to be proportional to the 0.75 power. Reasons were not provided for the use of the 0.75 power function, and it lacks biological support. A natural log function provided a better fit of the relationship of BW to body composition for Holsteins from birth to maturity (de Souza and VandeHaar, 2018).
The text within NRC (2001) implies that the composition of body gain is highly sensitive to the rate of gain, with animals gaining faster depositing a greater proportion of fat than animals growing slower. However, Table 11-1 in NRC (2001) does not bear this out; RE per day was proportional to ADG to the 1.097 power, and thus the RE content of EBG was proportional to ADG to the 0.097 power. Hence, the RE content of EBG was only 7 percent greater for a heifer gaining 1.2 kg/d than for one gaining 0.6 kg/d, which is much less of a response in body composition to rate of gain than in the literature cited (Radcliff et al., 1997; Waldo et al., 1997).
The current committee developed new equations to describe growth of heifers based on 26 publications with 129 treatment means for body composition of Holstein cattle from birth to maturity. Publications and variables used in the model are shown in Table 11-1. The composition of gain had a greater fat content, and thus energy content, in heifers with faster growth rates. However, the committee deemed that setting requirements based on the higher proportion of fat gain during faster growth was not reasonable because the resulting diets would be low in protein relative to energy and might limit proper frame and mammary growth. Instead, fat content of EBW was regressed against EBW for cattle from birth to maturity. For EBW between weaning (~80 kg) and first calving (~570 kg), a linear regression based on EBW fit the data as well as a quadratic function or functions based on log BW or BW to any power. The fat content of gain was derived from the fat content of EBW. To ensure mathematical consistency, the fat content of EBW or EBG was used to calculate fat-free matter (FFM), and the composition of FFM was assumed to be a constant of 21.5 percent protein, 5.6 percent ash, and 72.9 percent water, as shown by Waldo et al. (1997) and proposed for use in the seventh edition. The composition of FFM may change slightly as cattle age, but the effect of this change on the protein content of EBG is trivial compared to the effect of changes in FFM content. The RE content of EBW and EBG was calculated as a sum of the energy from retained fat and protein, using RE values of 9.4 Mcal/kg for fat and 5.55 Mcal/kg for protein (NRC, 2001).
TABLE 11-1Publications Used to Develop Equations for Composition of Gain in Dairy Heifersa
Reference | Method for Composition | EBW Measured? | Range in EBW, kg | Range in ADG, g/d |
---|---|---|---|---|
Heifers | ||||
Brown et al., 2005b | Carcass composition | No | 51 to 97 | 379 to 900 |
Chelikani et al., 2003 | Urea dilution | No | 239 to 269 | 520 to 1,040 |
Davis Rincker et al., 2008b | Rib composition | No | 140 to 173 | 645 to 1,100 |
Diaz et al., 2001 | EBW composition | Yes | 40 to 99 | 590 to 1,210 |
Meinert et al., 1992 | Urea dilution | No | 476 to 584 | 720 to 810 |
Meyer, 2005 | EBW composition | Yes | 85 to 310 | 610 to 963 |
Moallem et al., 2004 | EBW composition | Yes | 79 to 241 | 820 to 961 |
Radcliff et al., 1997 | Carcass composition | No | 286 to 349 | 770 to 1,270 |
Steen et al., 1992 | Urea dilution | No | 386 to 401 | 714 to 769 |
Waldo et al., 1997 | EBW composition | Yes | 155 to 289 | 766 to 1,004 |
Whitlock et al., 2002 | Carcass composition | No | 272 to 277 | 1,130 to 1,180 |
Calves | ||||
Bartlett et al., 2006 | EBW composition | Yes | 44 to 68 | 250 to 700 |
Blome et al., 2003 | EBW composition | Yes | 47 to 58 | 380 to 620 |
Chapman et al., 2017 | D2O dilution | No | 57 to 63 | 422 to 747 |
Donnelly and Hutton, 1976 | EBW composition | Yes | 61 to 70 | 610 to 830 |
Hill et al., 2008 | EBW composition | Yes | 59 to 88 | 360 to 450 |
Mills et al., 2010 | EBW composition | Yes | ~83 | 880 to 990 |
Rius et al., 2005 | Carcass composition | Yes | 63 to 150 | 1,090 to 1,230 |
Robelin and Chilliard, 1989 | EBW composition | Yes | 83 to 111 | 740 to 800 |
Swartz et al., 1991 | Urea dilution | No | 83 to 87 | 840 to 890 |
Tikofsky et al., 2001 | EBW composition | Yes | ~80 | 760 to 810 |
Cows | ||||
Agnew et al., 2005 | EBW composition | Yes | 412 | |
Andrew et al., 1994 | EBW composition | Yes | 452 to 480 | |
Belyea et al., 1978 | K40 | No | 441 to 507 | |
Chibisa et al., 2008 | Urea dilution | Yes | 553 to 656 | |
Komaragiri et al., 1998 | D2O dilution | Yes | 408 to 520 | |
Komaragiri and Erdman, 1997 | D2O dilution | No | 450 to 638 | |
Martin and Ehle, 1986 | D2O dilution | Yes | 595 to 689 | |
McGuffey et al., 1991 | EBW composition | Yes | 461 to 476 | |
Soderholm et al., 1988 | D2O dilution | No | 491 to 594 | |
von Soosten et al., 2012 | EBW composition | Yes | 398 to 447 |
- a
If EBW was not measured, EBW was the sum of components parts, or 0.85 × BW for calves and heifers, or 0.82 × BW for cows.
Assuming Holsteins in this data set had an average MatBW of 700 kg, a size-scaling approach was developed with the standard reference MatBW at 700 kg and a mature EBW at 574 kg (82 percent of 700). This size-scaling approach enables all equations to be used for other breeds, for which data are lacking. A similar approach was used in NRC (2001), but the current equations are simpler and use Holsteins as the reference. Equations were then converted, and composition of BW and BW gain were based on a percentage of an animal's expected MatBW. Gut fill for heifers was set at 15 percent of BW (instead of 18 percent for cows), so that EBG was 85 percent of ADG.
The resulting regressions are in Figure 11-1. As EBW increases, the composition of EBW changes linearly, with protein content decreasing and fat content increasing. The average content of EBW is 20 percent protein and 9 percent fat at 70 kg EBW (12 percent of mature EBW) and 17 percent protein and 22 percent fat at 470 kg EBW (82 percent of mature EBW). The assumption was made that these represent the average body composition for normal growth.

FIGURE 11-1
Fat and protein content of EBW in Holstein cattle. Points are treatment means, after adjustment for study effects, from studies using direct chemical measures of empty body mass (G_Direct), carcass or rib sections (G_Carcass) in growing calves or heifers, (more...)
The equations that best described the data of studies from Table 11-1 were as follows:
(Equation 11-4a)
(Equation 11-4b)
(Equation 11-4c)
(Equation 11-4d)
(Equation 11-4e)
Using the above equations to describe the composition of EBW at various ages, the composition of EBG (i.e., true growth) is the following:
(Equation 11-5a)
(Equation 11-5b)
(Equation 11-5c)
(Equation 11-5d)
(Equation 11-5e)
(Equation 11-5f)
where EBW = 0.85 × BW and EBG = 0.85 × ADG in kg and kg/d, respectively. Estimated ash and water concentration in EBW and EBG are not needed in the model but are shown here for completeness.
Thus, the composition of live BW gain is as follows:
(Equation 11-6a)
(Equation 11-6b)
(Equation 11-6c)
The expected composition of gain is shown from weaning to first calving in Figure 11-2, with comparisons to the NRC (2001) system for gains of 0.6 and 1.0 kg/d. The fat and energy contents of gain in the new equations are similar to NRC (2001) for midweight heifers but are greater for young prepubertal heifers and less for older heifers. The protein content of gain is less than NRC (2001) in all cases because that model assumed that body gain was 96 percent tissue (EBG/ADG was 0.96). As an animal approaches maturity (95 percent of MatBW), the composition of ADG as true frame growth approaches 36 percent fat, 10.5 percent protein, and 4.0 Mcal of RE/kg.

FIGURE 11-2
Expected fat, protein, and energy content of ADG in cattle from weaning to first calving using the new equations of this edition (black solid line) or equations from NRC (2001) for animals gaining 0.6 (red long dashed line) or 1.0 kg BW/d (red short dashed (more...)
After first calving, frame growth will continue but will be only a small portion of total requirements. Assuming that BCS is maintained at 3.0 to 3.5 and that gut fill is constant at 18 percent, Equations 11-5a to 11-5d are used to estimate body composition of the growth; however, Equations 11-6a to 11-6c are modified by replacing 0.85 with 0.82 because of differences in gut fill. In contrast, the composition of BW change when associated with BCS change is 62 percent fat, 8 percent protein, and 6.3 Mcal/kg.
The efficiencies of converting ME to net energy-gain (i.e., retained energy [RE]) and of converting MP to retained protein (equivalent to net protein gain, or NP) were not changed from NRC (2001).
As animals mature, the efficiency of converting MP to NP is decreased; the equation used by NRC (2001) dropped NP/MP from 0.77 to 0.39 as BW increased from 12 percent to 82 percent of MatBW. That equation yields values for young heifers greater than is reasonable based on Chapter 10. In NASEM (2016), NP/MP is essentially 0.5 for most growing cattle. The current committee set NP/MP at 0.6 for heifers at 12 percent of MatBW and decreased it linearly to 0.39 for heifers at 82 percent:
where EBW cannot exceed Mature EBW and EBW/Mature EBW can be approximated as BW/MatBW.
EFFECTS OF PLANE OF NUTRITION ON FUTURE MILK PRODUCTION
Energy Nutrition
How heifers are fed, starting at birth, can have long-term impacts on milk production through effects on the developing mammary gland and BW and BCS at first calving. Effects of preweaning nutrition are covered in Chapter 10. Recent reviews on heifer-rearing programs include Le Cozler et al. (2008), Lohakare et al. (2012), and Heinrichs et al. (2017).
First calving at 22 to 24 months is considered to best balance the cost of growing heifers with their production and lifetime income potential (Ettema and Santos, 2004; Heinrichs et al., 2017). Calving at <22 months usually is associated with smaller BW at first calving or requires rapid growth from birth to calving. Both inadequate size at first calving and rapid growth rates may limit subsequent milk production (Heinrichs et al., 2017).
The idea that smaller heifers at calving produce less milk is based almost entirely on correlations, often with all heifers fed and managed the same; these correlations are useful for formulating hypotheses, but they are not causal. A notable exception is a study of 500 dairy heifers of mixed breeds by Lin et al. (1986). Heifers were randomly assigned to two groups for breeding eligibility at 11.5 or 15 months, and those heifers bred early, compared to those bred late, calved 3 months earlier (23 versus 26 months), weighed 50 kg less at calving, and produced 300 kg less milk in their first lactation. Abeni et al. (2000) fed 42 Holstein–Friesian heifers and set breeding eligibility at 370 or 420 kg; those that calved early weighed 50 kg less at calving and produced 550 kg (7 percent) less energy-corrected milk in their first lactation. Assuming the lower milk yield of early bred heifers in both studies was due to their lower BW at calving, each kilogram decrease in BW could be expected to decrease milk production about 10 kg during the first lactation.
Target BWs for milestones in the life of a heifer are the same as those in NRC (2001) and shown in Table 11-2. As in NRC (2001), these targets are set as a percentage of MatBW to enable use for breeds other than Holstein or Jersey. Target BW for individual Holstein or Jersey heifers also might be adjusted as genomic predictions of MatBW become more available. The target BWs after the first and second calvings are 82 percent and 92 percent of MatBW, or 570 kg and 640 kg for Holsteins. The target BW at conception is 55 percent of MatBW, or about 380 kg for Holsteins. Target BCS at all milestones (breeding and calving) is 3.0 to 3.5. If the target age at first calving is 22 months, conception must occur at 13 months. Given that larger BW (with proper BCS) at calving results in more milk, the target at first calving may in some situations be >82 percent of MatBW. Given that conception often requires more than one insemination, optimal BW at first breeding may be 50 percent of MatBW or even less. Lighter BW at breeding may be acceptable if heifers are fed in confined systems for optimal growth once pregnant. If they are in systems where they will grow more slowly during gestation, they should be heavier at breeding.
To attain a BW of 350 to 380 kg (50 to 55 percent of 700 kg) by 13 months, Holsteins must gain ~0.85 kg/d on average from birth to 13 months, and because gains this fast are unlikely in the first 2 months, gains of ~0.90 kg/d are needed during much of the rest of the first year to attain first calving at 22 months. Based on the seminal article by Sinha and Tucker (1969), the mammary gland grows at an allometric rate between 3 and 9 months of age. However, on a fractional growth basis, the mass and DNA content of the mammary gland per 100 kg BW increased 50-fold from 1 to 3 months of age but only 3-fold from 3 to 5 months. Brown et al. (2005a) reported that the mass of mammary parenchyma per 100 kg BW increased 4- to 8-fold between 8 and 14 wk of age; in their study, they were unable to detect parenchymal tissue at 2 d of age. Thus, mammary growth is allometric from birth to about the time of puberty, when it slows down to the rate of other body tissues; during this time, the milk production potential of a heifer may be responsive to nutrition. An increased plane of nutrition before weaning enhances later milk production, but an increased plane of nutrition between the time of weaning and puberty may impair it.
Retrospective correlation analyses often find that high rates of gain in heifers do not impair and may even enhance subsequent milk production. For example, Krpálková et al. (2014) examined relationships of BW, BCS, and ADG of 780 heifers to their milk yield as cows; heifers were divided retrospectively into the three groups based on BW at 14 months. They found that heifers with the greatest BW at 14 months (ADG > 0.95 kg/d from 5 to 14 months) produced the most milk over their first three lactations. The larger heifers had slightly higher BCS at 14 months (3.5 versus 3.2) and calved 1 month earlier (24 versus 25 months) than the smallest heifers. Volkmann et al. (2019) found that rapid growth rates from birth to 1 year did not impair milk production of 2,300 Holsteins. These data suggest that rapid growth rates in heifers can be consistent with high milk production as cows. However, correlations of gain with milk production in animals that are all fed and managed the same are not causal and can be misleading. Some of the endogenous controls of lean growth also control milk production (such as somatotropin); hence, faster growth should be associated with greater milk yield. In setting targets for ADG when formulating diets, the question is whether average gains >0.95 kg/d would promote more milk than average gains <0.85 kg/d.
Based on studies where heifers were randomly assigned to diets that promoted fast or slow growth, ADG >0.9 kg/d during the period between weaning and breeding generally decreased milk yield in the first lactation (Zanton and Heinrichs, 2005). However, diets that promoted gains between 0.9 and 1.0 kg/d caused only small drops in future milk yield (5 percent or less), and only diets promoting gains >1.0 kg/d decreased milk production by greater than 10 percent. Two additional studies not cited by Zanton and Heinrichs (2005) also support that diet-induced gains >1.0 kg/d decrease subsequent milk yield by >10 percent (Gardner et al., 1977; Peri et al., 1993). Van Amburgh et al. (2019) proposed that this decrease in milk yield from feeding high energy in the first year of life was due to excess body condition carried over to first calving; however, this was not the case for all studies. For example, Radcliff et al. (2000) fed diets promoting gains of 0.8 or 1.1 kg/d from 4 months of age until confirmed pregnant and found that heifers fed for faster growth had greater BCS at first insemination (4.2 versus 3.5) but the same BCS at calving (3.5 versus 3.7) and same postpartum BW (515 versus 539 kg); they calved 3 months earlier (631 versus 719 days) and produced 10 percent less energy-corrected milk in the first lactation.
The etiology for the effect of high-energy diets fed to prepuberty heifers on milk production potential is not clear. Ever since Sinha and Tucker (1969) showed that the mammary gland slowed from allometric to isometric growth at puberty, one mechanism considered for the decreased mass of mammary parenchyma per unit of BW at puberty was that high-energy diets hasten the age of puberty and thus truncate the period of allometric mammary growth. This was demonstrated by Meyer et al. (2006), who killed heifers at 50-kg increments of BW that were fed high- or low-energy diets; heifers fed high-energy diets gained 0.95 kg/d, compared to 0.65 kg/d for low-energy diets, but the rate of mammary parenchymal accretion was the same in each. Heifers fed high-energy diets attained puberty earlier and had less parenchymal mass at puberty. Van Amburgh et al. (2019) further demonstrated that early puberty with truncated mammary growth likely could explain results of several other studies. However, the question remains whether mammary growth would have been constant outside the bounds of 0.65 to 0.95 kg/d and why diet might alter mammary development in heifers before 2 months of age but not between 2 months and puberty.
Possibly more important than rate of gain per se is the extent of fat deposition in body and mammary tissues, as proposed by Swanson (1960) 60 years ago. Silva et al. (2002) examined the relationships of ADG and body fat content of individual heifers fed for rapid gains in several studies. They found that for heifers around the time of puberty, those with the greatest amount of body fat had the least amount of mammary parenchyma, and for heifers that were bred and followed through lactation, those with higher BCS at breeding produced less milk as cows (Silva et al., 2002). Thus, although a high rate of gain is often considered the cause of impaired milk production potential, the problem may actually be that diets that promote rapid gains also promote excessive fat accretion. The idea that fat could interfere with mammary development is supported by studies showing negative effects of leptin on insulin-like growth factor-I (IGF-I)–stimulated mammary cell proliferation in heifers (Silva et al., 2008) and that diet-induced obesity impairs normal mammary development in other species, such as mice (Kamikawa et al., 2009). If excess fattening is the cause of impaired mammary development, then feeding programs should focus on sound growth without fattening. In Meyer et al. (2006), heifers fed high energy to gain 0.95 kg/d had greater mammary fat pad mass but not greater body fat content per kilogram BW during the prepubertal period; this rate of gain seems reasonable for modern Holsteins.
Whether the decrease in mammary parenchymal mass at puberty is important is not clear. Perhaps the mammary gland compensates after puberty for the potential development that was not realized prior to puberty. However, as discussed earlier, multiple studies have demonstrated that prepubertal diets that promote rapid gains in the prepubertal period decrease first-lactation milk yield. Rapid growth often increases BCS, and in some of these studies, increased BCS might have been carried over to the time of calving; however, in some studies, heifers calved at similar BCS, which indicates that postpubertal development may not be able to compensate for decreased prepubertal development. If heifers grow rapidly without fattening, then perhaps gains >1.0 kg/d are acceptable for Holsteins, but no studies to date have shown that gains greater than 1.0 kg/d during the prepubertal period result in as much milk once heifers have calved. Optimal rates of gain should be adjusted for expected MatBW and are shown in Table 11-2; the upper threshold for prepubertal Jersey heifers is ~750 g/d.
TABLE 11-2Target Weights (kg), Ages, and ADGs (kg/d) for Growing Dairy Cattle
Percent of Mature BW | Holstein | Jersey | |
---|---|---|---|
Mature BW | 100 | 700 | 520 |
Birth BW | 6 | 42 | 31 |
Weaning BW | 12 | 84 | 62 |
Conception BW | 55 | 385 | 286 |
First calving prepartum BW | 91 | 638 | 426 |
First calving postpartum BW | 82 | 574 | 474 |
Second calving postpartum BW | 92 | 644 | 478 |
Conception age, months | 13 | 13 | |
First calving age, months | 22 | 22 | |
Prepubertal ADG | 0.13 | 0.90 | 0.67 |
Postpubertal ADG | 0.10 | 0.69 | 0.51 |
Postpubertal gain + pregnancy | 0.13 | 0.92 | 0.69 |
First-lactation ADG | 0.027 | 0.19 | 0.14 |
Second-lactation ADG | 0.022 | 0.15 | 0.11 |
Protein Nutrition
The requirement for dietary protein in NRC (2001) was calculated based on the rate of protein accretion, the efficiency with which MP is converted to retained protein, and the digestibility, rumen degradability, rumen kinetics, and microbial metabolism of dietary CP. Because the major target for protein deposition is muscle, muscle accretion was the major determinant of the final dietary protein requirements. However, optimal heifer-rearing programs must consider effects of protein nutrition not only on muscle accretion but also on structural growth, feed efficiency, and future milk production potential. Dietary protein supplies amino acids, which are the building blocks for protein synthesis in all of these functions, but amino acids also can alter hormonal signals and cellular machinery in ways that are not easily quantified (Rezaei et al., 2016). Setting protein requirements based solely on relationships between dietary protein and protein accretion does not account for effects of protein on physiological regulation. Several studies in the past 20 years have examined protein requirements for dairy heifers as a function of ME intake, and most have shown that the required ratios of protein to energy in NRC (2001) were reasonable. From NRC (2001), the required protein to energy ratio in diets for heifers at 150 kg BW gaining 900 g/d was 72 g CP/Mcal ME. The required ratio was sensitive to level of maturity and to rate of gain. The required ratio decreased from 72 to 64 g/Mcal for 150-kg heifers as gain decreased from 900 to 600 g/d and decreased from 72 to 52 for heifers gaining 900 g/d as BW increased from 150 to 350 kg BW. However, these requirements were based on equations where the protein content of EBG for young heifers and EBG as a percentage of ADG of all heifers were too high (EBG was 96 percent of ADG). Correcting these equations results in lower requirements for protein relative to energy, and these new requirements may be inconsistent with recommendations for optimal mammary development.
Studies in which excess energy intake depressed mammary development or subsequent milk production the most were those with the lowest protein content per unit energy in the diet of the heifers fed for rapid growth (Whitlock et al., 2002). Whitlock et al. (2002) directly tested the effect of protein in Holstein heifers by feeding ad libitum high-energy diets containing 48, 57, and 66 g CP per Mcal ME (corresponding to estimates of 37, 41, and 44 g MP) from 130 to 320 kg BW. The diets had no effect on carcass gain or carcass composition and no significant effect overall on mammary development. However, for heifers that naturally grew the fastest and achieved puberty earliest, those fed the high-protein diet had greater mammary development. Lammers and Heinrichs (2000) fed prepubertal heifers from 200 to 340 kg BW diets containing 46, 54, and 61 g CP/Mcal ME with dry matter intake (DMI) restricted to 2.45 percent of BW per day; heifers grew 1,000 to 1,100 g/d. The rate of gain, feed efficiency, structural growth, and teat elongation were greater in heifers fed 61 than 46 or 54 g CP/Mcal ME. Even in heifers growing at slower rates, dietary protein may affect mammary development. Pirlo et al. (1997) fed prepubertal Friesian heifers diets with high or low energy and high or low protein. The high-energy diets promoted gains of 820 g/d and contained 62 or 50 g CP/Mcal ME from 100 to 200 kg of BW and 49 or 40 g CP/Mcal ME from 200 to 300 kg. Compared to groups fed low-energy diets, heifers fed high energy with low protein tended to produce 15 percent less milk protein as cows, but those fed high energy with high protein produced as much as those fed low energy. Gabler and Heinrichs (2003) fed 60 prepubertal Holstein heifers between 125 and 234 kg BW diets with varying protein content at restricted intakes to gain 0.8 kg/d; they found that diets with a CP/ME ratio of 59 or 68 outperformed diets containing 48 or 77 g/Mcal when considering structural growth, feed efficiency, and protein efficiency.
On the basis of the above studies, the current committee determined that diets for heifers from weaning to first calving should contain a minimum amount of MP per Mcal of ME to meet desired rates of gain, minimize the risk of excessive fat deposition, optimize lean tissue and structural growth, and maximize mammary development and subsequent milk production:
(Equation 11-10)
TOTAL REQUIREMENTS FOR METABOLIZABLE ENERGY AND METABOLIZABLE PROTEIN
Requirements for maintenance and growth were discussed above and requirements for pregnancy are discussed in Chapters 3 and 6.
Because of effects on future milk yield (discussed above), the total MP requirement should be increased if below the minimum threshold of MP/ME for optimal development.
If MP (g/d) < (53 – 25 × BW/MatBW) × ME (Mcal/d), then
(Equation 11-11)
Using these equations, requirements for ME and MP are shown in Tables 11-3 and 11-4 for cattle with a mature BW of 700 kg and 520 kg, the typical MatBW for Holsteins and Jerseys. For these examples, the minimum MP to ME ratio was the determining factor for setting the requirement for MP. The committee suggests that the minimal dietary protein for optimal milk production potential may be higher than that for structural growth. Further research is needed to refine MP requirements for heifers that support both growth and future milk production potential.
TARGET BODY WEIGHTS AT BREEDING AND CALVING
Targets in Table 11-2 are set for heifers reared in intensive or dry lot environments. Heifers grown on pasture or in situations where poor-quality feedstuffs are cost-effective should use similar targets for BW but should consider later targets for age at each BW target. These targets assume that heifers will have BCS consistent with normal, sound growth.
PREDICTING GAIN FROM AVAILABLE METABOLIZABLE ENERGY AND METABOLIZABLE PROTEIN
The above equations can be reversed to predict gain. Although the composition of gain was not adjusted with changes in rate of gain when setting requirements, the change in composition is used to adjust the predicted gain based on available energy. In NRC (2001), RE per day was proportional to ADG to the 1.097 power, so the RE content of gain was proportional to ADG to the 0.097 power. Both the current calf and heifer models will continue to use this value, albeit rounded to 0.10, for predicting gains. In addition, in the heifer model, the prediction is adjusted so that the base RE content of gain is set for a heifer of MatBW at 700 kg gaining 840 g/d, or 0.12 percent of MatBW per day. For heifers of 700 kg MatBW, gains greater than 840 g/d will result in greater RE per kilogram of gain, or less gain than predicted without the adjustment. For small breeds, 840 g/d would be a fast rate of gain; the base for a heifer with 520 kg MatBW would be at 620 g/d gain. Because the minimum MP to ME ratio was the determining factor for setting the requirement for MP in most cases, allowable gains based on protein were not predicted. If the MP to ME ratio of a diet is less than that shown in Table 11-3 or 11-4 for a heifer of a given BW, the diet has insufficient protein for optimal growth and development. The equations to predict gain from ME intake are as follows:
(Equation 11-12a)
(Equation 11-12b)
(Equation 11-12c)
(Equation 11-12d)
(Equation 11-12e)
TABLE 11-3Requirements for Energy and Protein in Heifers with MatBW of 700 kga
Live BW | 112 | 224 | 336 | 420 | 560 |
---|---|---|---|---|---|
BW as % of mature BW | 16 | 32 | 48 | 60 | 80 |
Estimated DMI, kg/d | 3.3 | 6.0 | 8.0 | 9.3 | 10.9 |
Maintenance | |||||
ME, Mcal/d | 5.2 | 8.7 | 11.8 | 13.9 | 17.3 |
MP, g/d | 121 | 228 | 325 | 389 | 486 |
Body composition | |||||
Body fat, % | 8.2 | 10.8 | 13.3 | 15.3 | 18.4 |
Body protein, % | 16.5 | 16.0 | 15.4 | 15.0 | 14.3 |
Body energy, Mcal/kg | 1.69 | 1.90 | 2.11 | 2.27 | 2.53 |
Composition of gain | |||||
Fat in ADG, % | 10.8 | 15.9 | 21.0 | 24.8 | 31.2 |
Protein in ADG, % | 16.0 | 14.9 | 13.8 | 12.9 | 11.6 |
Energy in ADG, Mcal/kg | 1.90 | 2.32 | 2.74 | 3.05 | 3.58 |
Retained energy, Mcal/d | |||||
ADG = 700 g/d | 1.33 | 1.62 | 1.92 | 2.14 | 2.50 |
ADG = 840 g/d | 1.60 | 1.95 | 2.30 | 2.56 | 3.00 |
ADG = 980 g/d | 1.86 | 2.27 | 2.68 | 2.99 | 3.50 |
Protein gain, g/d | |||||
ADG = 700 g/d | 112 | 104 | 96 | 91 | 81 |
ADG = 840 g/d | 134 | 125 | 116 | 109 | 97 |
ADG = 980 g/d | 156 | 146 | 135 | 127 | 113 |
ME for pregnancy, Mcal/d | 4.16 | ||||
MP for pregnancy, g/d | 250 | ||||
ME requirement, Mcal/d | |||||
ADG = 700 g/d | 8.5 | 12.7 | 16.6 | 19.3 | 27.7 |
ADG = 840 g/d | 9.2 | 13.6 | 17.5 | 20.3 | 28.9 |
ADG = 980 g/d | 9.8 | 14.4 | 18.5 | 21.4 | 30.2 |
MP to NP conversion | 0.59 | 0.54 | 0.50 | 0.46 | 0.40 |
Minimum MP/ME | 49 | 45 | 41 | 38 | 33 |
MP requirement, g/d | |||||
ADG = 700 g/d | 416 | 573 | 679 | 732 | 939 |
ADG = 840 g/d | 448 | 610 | 718 | 772 | 979 |
ADG = 980 g/d | 481 | 646 | 758 | 813 | 1,020 |
ME/kg diet DM | |||||
ADG = 700 g/d | 2.5 | 2.1 | 2.1 | 2.1 | 2.5 |
ADG = 840 g/d | 2.7 | 2.3 | 2.2 | 2.2 | 2.7 |
ADG = 980 g/d | 2.9 | 2.4 | 2.3 | 2.3 | 2.8 |
CP, % of diet DM | |||||
ADG = 700 g/d | 19.9 | 15.4 | 13.6 | 12.7 | 13.5 |
ADG = 840 g/d | 21.5 | 16.4 | 14.4 | 13.4 | 14.1 |
ADG = 980 g/d | 23.1 | 17.4 | 15.2 | 14.1 | 14.7 |
- a
Predicted DMI and the resulting ME and CP densities were based on the DMI prediction from Chapter 2 and using a conversion of CP to MP of 0.62. Heifer for last column is 40 days prepartum.
Reported diet-induced changes in composition of gain vary widely. Waldo et al. (1997) measured EBG to be 21 percent fat for 330-kg heifers that had gained 780 g/d but 25 percent fat for those gaining 990 g/d. Radcliff et al. (1997) observed 17 percent fat in carcasses of 340-kg heifers gaining 770 g/d for 4 months and 25 percent fat in those gaining 1,200 g/d; BCS was 2.9 and 3.9, respectively. Davis Rincker et al. (2008b) estimated the RE content of ADG to be 1.6 Mcal/kg (8 percent fat) in young heifers gaining 640 g/d for 12 weeks and 3.0 Mcal/kg (25 percent fat) for those gaining 1.08 kg/d. Meyer et al. (2006) found little difference in the composition of ADG in heifers fed low- or high-energy diets from birth to 250 kg BW and gaining ~660 versus ~930 g/d. As BW increased from 100 to 250 kg, the RE content of ADG increased from ~1.4 to 2.7 Mcal/kg (from 10 to 21 percent fat) with little difference between diets. However, for heifers killed at 300 or 350 kg, the RE of ADG was ~2.1 Mcal/kg (20 percent fat) on the low-energy diet and nearly double that on the high-energy diet. Thus, neither the previous nor current NRC models adequately account for changes in the composition of gain that occur when heifers are fed diets supporting different rates of gain. The current committee decided that data were lacking to change the assumptions from NRC (2001) or NASEM (2016); thus, the RE content of ADG is proportional to the 0.1 power of ADG. In the future, a reasonable approach for predicting composition of gain would be to predict the composition of frame gain and then to use changes in body condition for significant deviations from the standard growth rates.
TABLE 11-4Requirements for Energy and Protein in Heifers with MatBW of 520 kga
Live BW | 83 | 166 | 250 | 312 | 416 |
---|---|---|---|---|---|
BW as % of mature BW | 16 | 32 | 48 | 60 | 80 |
Estimated DMI, kg/d | 2.5 | 4.5 | 6.0 | 6.9 | 8.1 |
Maintenance | |||||
ME, Mcal/d | 4.1 | 6.9 | 9.4 | 11.1 | 13.8 |
MP, g/d | 90 | 170 | 242 | 290 | 362 |
Body composition | |||||
Body fat, % | 8.2 | 10.8 | 13.3 | 15.3 | 18.4 |
Body protein, % | 16.5 | 16.0 | 15.4 | 15.0 | 14.3 |
Body energy, Mcal/kg | 1.69 | 1.90 | 2.11 | 2.27 | 2.53 |
Composition of gain | |||||
Fat in ADG, % | 10.8 | 15.9 | 21.0 | 24.8 | 31.2 |
Protein in ADG, % | 16.0 | 14.9 | 13.8 | 12.9 | 11.6 |
RE in ADG, Mcal/kg | 1.90 | 2.32 | 2.74 | 3.05 | 3.58 |
Retained energy, Mcal/d | |||||
ADG = 520 g/d | 0.99 | 1.21 | 1.42 | 1.59 | 1.86 |
ADG = 624 g/d | 1.19 | 1.45 | 1.71 | 1.90 | 2.23 |
ADG = 728 g/d | 1.38 | 1.69 | 1.99 | 2.22 | 2.60 |
Protein gain, g/d | |||||
ADG = 520 g/d | 83 | 77 | 72 | 67 | 60 |
ADG = 624 g/d | 100 | 93 | 86 | 81 | 72 |
ADG = 728 g/d | 116 | 108 | 100 | 94 | 84 |
ME for pregnancy, Mcal/d | 3.04 | ||||
MP for pregnancy, g/d | 183 | ||||
ME requirement, Mcal/d | |||||
ADG = 520 g/d | 6.6 | 10.0 | 13.0 | 15.1 | 21.5 |
ADG = 624 g/d | 7.1 | 10.6 | 13.7 | 15.9 | 22.4 |
ADG = 728 g/d | 7.6 | 11.2 | 14.4 | 16.7 | 23.4 |
MP to NP conversion | 0.59 | 0.54 | 0.50 | 0.46 | 0.40 |
Minimum MP/ME | 49 | 45 | 41 | 38 | 33 |
MP requirement, g/d | |||||
ADG = 520 g/d | 323 | 448 | 532 | 574 | 710 |
ADG = 624 g/d | 348 | 475 | 561 | 604 | 740 |
ADG = 728 g/d | 372 | 503 | 590 | 634 | 771 |
ME, Mcal/kg diet DM | |||||
ADG = 520 g/d | 2.6 | 2.2 | 2.2 | 2.2 | 2.7 |
ADG = 624 g/d | 2.8 | 2.4 | 2.3 | 2.3 | 2.8 |
ADG = 728 g/d | 3.0 | 2.5 | 2.4 | 2.4 | 2.9 |
CP, % of diet DM | |||||
ADG = 520 g/d | 20.9 | 16.2 | 14.4 | 13.4 | 14.1 |
ADG = 624 g/d | 22.4 | 17.2 | 15.1 | 14.1 | 14.7 |
ADG = 728 g/d | 24.0 | 18.2 | 15.9 | 14.8 | 15.3 |
- a
Predicted DMI and the resulting ME and CP densities were based on the DMI prediction from Chapter 2 and using a conversion of CP to MP of 0.62. Heifer for last column is 40 days prepartum.
HEIFER GROWTH PROGRAMS
Between birth and first calving, feed quality and availability may vary due to environmental and price constraints. Periods of slow growth on low-energy diets may be followed by periods of rapid growth on high-energy diets, and in the end, the development of the heifer may be similar. Such “stairstep” programs may improve lifetime productively (Ford and Park, 2001). Periods of alternating slow and rapid growth might also provide advantages in efficiency due to compensatory gain (NASEM, 2016) and allow use of poor-quality feeds or pastures. At the least, periods of alternating fast and slow growth seem to cause no disadvantage to heifers in the long term. Based on the potential impacts on mammary development discussed earlier, one period to promote slower growth would be between 3 months of age and puberty. Another possibility would be to feed for slower growth when heifers are being managed for breeding, so that those taking longer to conceive are less likely to gain excess body condition. In addition, if grain is relatively cheap, the most cost-effective way to feed heifers may be to feed high-energy diets with more grain at a restricted intake (Zanton and Heinrichs, 2007, 2008). Restricted feeding of higher-energy diets can promote optimal growth provided feed is uniformly provided so all animals maintain proper body condition. In addition, increased grain and decreased fiber in heifer diets fed at restricted intake would decrease methane and manure output.
MODEL LIMITATIONS AND RESEARCH NEEDS
The current model assumes that the digestibility of feeds and the conversion of DE to ME are the same in heifers as in cows and that the conversion of ME to RE is 0.40, as in NRC 2001. These assumptions need validation via research. The committee recommends that future studies monitor and report BCS, along with diets, intake, and rates and composition of gain. When feeding heifers, BW gain and BCS should be closely monitored to ensure optimal skeletal growth. In addition to diet, activity, environment, genetics, and health can alter the expected gain for a given energy intake. Data to include these factors in determining requirements or predicting gains are lacking. Therefore, when feeding heifers, BW gain and BCS should be closely monitored to ensure that target growth rates are achieved.
REFERENCES
- Abeni F, Calamari L, Stefanini L, Pirlo G. Effects of daily gain in pre- and postpubertal replacement dairy heifers on body condition score, body size, metabolic profile, and future milk production. J. Dairy Sci. 2000;83:1468–1478. [PubMed: 10908055]
- Agnew RE, Yan T, McCaughey WJ, McEvoy JD, Patterson DC, Porter MG, Steen RWJ. Relationships between urea dilution measurements and body weight and composition of lactating dairy cows. J. Dairy Sci. 2005;88:2476–2486. [PubMed: 15956310]
- Andrew SM, Waldo DR, Erdman RA. Direct analysis of body composition of dairy cows at three physiological stages. J. Dairy Sci. 1994;77:3022–3033. [PubMed: 7836590]
- Bartlett KS, McKeith FK, VandeHaar MJ, Dahl GE, Drackley JK. Growth and body composition of dairy calves fed milk replacers containing different amounts of protein at two feeding rates. J. Anim. Sci. 2006;84:1454–1467. [PubMed: 16699102]
- Belyea RL, Frost GR, Martz FA, Clark JL, Forkner LG. Body composition of dairy cattle by Potassium-40 liquid scintillation detection. J. Dairy Sci. 1978;61:206–211.
- Blome RM, Drackley J, McKeith F, Hutjens M, McCoy G. Growth, nutrient utilization, and body composition of dairy calves fed milk replacers containing different amounts of protein. J. Anim. Sci. 2003;81:1641–1655. [PMC free article: PMC7110375] [PubMed: 12817512]
- Brown EG, VandeHaar MJ, Daniels KM, Liesman JS, Chapin LT, Forrest JW, Akers RM, Pearson RE, Weber Nielsen MS. Effect of increasing energy and protein intake in heifer calves on mammary development. J. Dairy Sci. 2005a;88:595–603. [PubMed: 15653526]
- Brown EG, VandeHaar MJ, Daniels KM, Liesman JS, Chapin LT, Keisler DH, Weber Nielsen MS. Effect of increasing energy and protein intake on body growth and carcass composition of heifer calves. J. Dairy Sci. 2005b;88:585–594. [PubMed: 15653525]
- Chapman CE, Stone Wilkinson P, Murphy MR, Erickson PS. Technical note: Evaluating nuclear magnetic resonance spectroscopy for determining body composition in Holstein dairy calves using deuterium oxide dilution methods. J. Dairy Sci. 2017;100:2807–2811. [PubMed: 28161168]
- Chelikani PK, Ambrose JD, Kennelly JJ. Effect of dietary energy and protein density on body composition, attainment of puberty, and ovarian follicular dynamics in dairy heifers. Theriogenology. 2003;60:707–725. [PubMed: 12832019]
- Chibisa GE, Gozho GN, Van Kessel AG, Olkowski AA, Mutsvangwa T. Effects of peripartum propylene glycol supplementation on nitrogen metabolism, body composition, and gene expression for the major protein degradation pathways in skeletal muscle in dairy cows. J. Dairy Sci. 2008;91:3512–3527. [PubMed: 18765610]
- Davis Rincker LE, Weber Nielsen MS, Chapin LT, Liesman JS, Daniels KM, Akers RM, VandeHaar MJ. Effects of feeding prepubertal heifers a high-energy diet for three, six, or twelve weeks on mammary growth and composition. J. Dairy Sci. 2008a;91:1926–1935. [PubMed: 18420624]
- Davis Rincker LE, Weber Nielsen MS, Chapin LT, Liesman JS, VandeHaar MJ. Effects of feeding prepubertal heifers a high-energy diet for three, six, or twelve weeks on feed intake, body growth, and fat deposition. J. Dairy Sci. 2008b;91:1913–1925. [PubMed: 18420623]
- de Souza RA, VandeHaar M. Predicting composition of empty body weight of Holstein heifers and cows. J. Dairy Sci. 2018;101(Suppl. 2):126–127.
- Diaz MC, Van Amburgh M, Smith J, Kelsey J, Hutten E. Composition of growth of Holstein calves fed milk replacer from birth to 105-kilogram body weight. J. Dairy Sci. 2001;84:830–842. [PubMed: 11352160]
- Donnelly PE, Hutton JB. Effects of dietary protein and energy on the growth of Friesian hull calves. N. Z. J. Agric. Res. 1976;19:289–297.
- Ettema JF, Santos JPE. Impact of age at calving on lactation, reproduction, health, and income in first-parity Holsteins on commercial farms. J. Dairy Sci. 2004;87:2730–2742. [PubMed: 15328299]
- Ford JA Jr, Park CS. Nutritionally directed compensatory growth enhances heifer development and lactation potential. J. Dairy Sci. 2001;84:1669–1678. [PubMed: 11467817]
- Gabler MT, Heinrichs AJ. Dietary protein to metabolizable energy ratios on feed efficiency and structural growth of prepubertal Holstein heifers. J. Dairy Sci. 2003;86:268–274. [PubMed: 12613870]
- Gardner RW, Schuh JD, Vargus LG. Accelerated growth and early breeding of Holstein heifers. J. Dairy Sci. 1977;60:1941.
- Garrett W. Energy Metabolism. Oxford, UK: Butterworth-Heinemann; 1980. Energy utilization by growing cattle as determined in 72 comparative slaughter experiments; pp. 3–7.
- Garrett WN, Meyer JH, Lofgreen GP. The comparative energy requirements of sheep and cattle for maintenance and gain. J. Anim. Sci. 1959;18:528–547.
- Heinrichs AJ, Zanton GI, Lascano GJ, Jones CM. A 100-year review: A century of dairy heifer research. J. Dairy Sci. 2017;100:10173–10188. [PubMed: 29153161]
- Hill SR, Knowlton KF, Daniels KM, James RE, Pearson RE, Capuco AV, Akers RM. Effect of milk replacer composition on growth, body composition, and nutrient excretion in preweaned Holstein heifers. J. Dairy Sci. 2008;91:3145–3155. [PubMed: 18650291]
- Kamikawa A, Ichii O, Yamaji D, Imao T, Suzuki C, Okamatsu-Ogura Y, Terao A, Kon Y, Kimura K. Diet-induced obesity disrupts ductal development in the mammary glands of nonpregnant mice. Dev. Dyn. 2009;238:1092–1099. [PubMed: 19384959]
- Komaragiri M, Erdman RA. Factors affecting body tissue mobilization in early lactation dairy cows: 1. Effect of dietary protein on mobilization of body fat and protein. J. Dairy Sci. 1997;80:929–937. [PubMed: 9178133]
- Komaragiri M, Casper DP, Erdman RA. Factors affecting body tissue mobilization in early lactation dairy cows: 2. Effect of dietary fat on mobilization of body fat and protein. J. Dairy Sci. 1998;81:169–175. [PubMed: 9493092]
- Krpálková L, Cabrera VE, Vacek M, Štípková M, Stádník L, Crump P. Effect of prepubertal and postpubertal growth and age at first calving on production and reproduction traits during the first 3 lactations in Holstein dairy cattle. J. Dairy Sci. 2014;97:3017–3027. [PubMed: 24612798]
- Lammers BP, Heinrichs JA. The response of altering the ratio of dietary protein to energy on growth, feed efficiency, and mammary development in rapidly growing prepubertal heifers. J. Dairy Sci. 2000;83:977–983. [PubMed: 10821573]
- Le Cozler Y, Lollivier V, Lacasse P, Disenhaus C. Rearing strategy and optimizing first-calving targets in dairy heifers: A review. Animal. 2008;2(9):1393–1404. [PubMed: 22443830]
- Lin CY, McAllister AJ, Batra TR, Lee AJ, Roy GL, Vesely JA, Wauthy JM, Winter KA. Production and reproduction of early and late bred dairy heifers. J. Dairy Sci. 1986;69(3):760–768. [PubMed: 3711408]
- Lohakare JD, Südekum KH, Pattanaik AK. Nutrition-induced changes of growth from birth to first calving and its impact on mammary development and first-lactation milk yield in dairy heifers: A review. Asian Aust. J. Anim. Sci. 2012;25(9):1338–1350. [PMC free article: PMC4092940] [PubMed: 25049699]
- Martin RA, Ehle FR. Body composition of lactating and dry Holstein cows estimated by deuterium dilution. J. Dairy Sci. 1986;69:88–98. [PubMed: 3009575]
- McGuffey RK, Basson RP, Spike TE. Lactational response and body composition of cows receiving somatotropin and three ratios of forage to concentrate. J. Dairy Sci. 1991;74:3095–3102. [PubMed: 1779063]
- Meinert RA, Yang C-MJ, Heinrichs AJ, Varga GA. Effect of monensin on growth, reproductive performance, and estimated body composition in Holstein heifers. J. Dairy Sci. 1992;75:257–261. [PubMed: 1541735]
- Meyer MJ. Department of Animal Science, Cornell University; Ithaca, NY: 2005. Developmental, nutritional, and hormonal regulation of mammary growth, steroid receptor gene expression and chemical composition of retained tissues in prepubertal bovine PhD.
- Meyer MJ, Capuco AV, Ross DA, Lintault LM, Van Amburgh ME. Developmental and nutritional regulation of the prepubertal bovine mammary gland: II. Epithelial cell proliferation, parenchymal accretion rate, and allometric growth. J. Dairy Sci. 2006;89:4298–4304. [PubMed: 17033017]
- Mills JK, Ross DA, Van Amburgh ME. The effects of feeding medium-chain triglycerides on the growth, insulin responsiveness, and body composition of Holstein calves from birth to 85 kg of body weight. J. Dairy Sci. 2010;93:4262–4273. [PubMed: 20723699]
- Moallem U, Dahl GE, Duffey EK, Capuco AV, Erdman RA. Bovine somatotropin and rumen-undegradable protein effects on skeletal growth in prepubertal dairy heifers. J. Dairy Sci. 2004;87:3881–3888. [PubMed: 15483172]
- NASEM (National Academies of Sciences, Engineering and Medicine). Nutrient Requirements of Beef Cattle. 8th rev. Washington, DC: The National Academies Press; 2016.
- NRC (National Research Council). Nutrient Requirements for Dairy Cattle. 6th rev. Washington, DC: National Academy Press; 1989.
- NRC. Nutrient Requirements of Beef Cattle. 7th rev. Washington, DC: National Academy Press; 1996.
- NRC. Nutrient Requirements of Dairy Cattle. 7th rev. Washington, DC: National Academy Press; 2001.
- Peri I, Gertler A, Bruckental I, Barash H. The effect of manipulation in energy allowance during the rearing period of heifers on hormone concentrations and milk production in first lactation cows. J. Dairy Sci. 1993;76:742. [PubMed: 8463486]
- Pirlo G, Capelletti M, Marchetto G. Effects of energy and protein allowances in the diets of prepubertal heifers on growth and milk production. J. Dairy Sci. 1997;80:730–739. [PubMed: 9149967]
- Radcliff RP, VandeHaar MJ, Skidmore AL, Chapin LT, Radke BR, Lloyd JW, Staisiewski EP, Tucker HA. Effect of diet and bST on heifer growth and mammary development. J. Dairy Sci. 1997;80:1996–2003. [PubMed: 9313140]
- Radcliff RP, VandeHaar MJ, Chapin LT, Pilbeam TE, Beede DK, Stanisiewski EP, Tucker HA. Effects of diet and injection of bovine somatotropin on prepubertal growth and first-lactation milk yields of Holstein cows. J. Dairy Sci. 2000;83:23–29. [PubMed: 10659959]
- Rezaei R, Wu Z, Hou Y, Bazer FW, Wu G. Amino acids and mammary gland development: Nutritional implications for milk production and neonatal growth. J. Anim. Sci. Biotechnol. 2016;7:20. [PMC free article: PMC4818943] [PubMed: 27042295]
- Rius AG, Connor EE, Capuco AV, Kendall PE, AuchtungMontgomery TL, Dahl GE. Long-day photoperiod that enhances puberty does not limit body growth in Holstein heifers. J. Dairy Sci. 2005;88:4356–4365. [PubMed: 16291627]
- Robelin J, Chilliard Y. Short-term and long-term effects of early nutritional deprivation on adipose tissue growth and metabolism in calves. J. Dairy Sci. 1989;72:505–513. [PubMed: 2703573]
- Silva LFP, VandeHaar MJ, Whitlock BK, Radcliff RP, Tucker HA. Short communication: Relationship of body growth to mammary development in dairy heifers. J. Dairy Sci. 2002;85:2600–2602. [PubMed: 12416813]
- Silva LFP, Etchebarne BE, Weber Nielsen MS, Liesman JS, Kiupel M, VandeHaar MJ. Intramammary infusion of leptin decreases proliferation of mammary epithelial cells in prepubertal heifers. J. Dairy Sci. 2008;91:3034–3044. [PubMed: 18650280]
- Simpfendorfer S. Cornell University; Ithaca, NY: 1974. Relationship of body type, size, sex, and energy intake to the body composition of cattle PhD.
- Sinha YN, Tucker HA. Mammary development and pituitary prolactin levels of heifers from birth through puberty and during the estrous cycle. J. Dairy Sci. 1969;52:507. [PubMed: 5813504]
- Soderholm CG, Otterby DE, Linn JG, Ehle FR, Wheaton JE, Hansen WP, Annexstad RJ. Effects of recombinant bovine somatotropin on milk production, body composition, and physiological parameters. J. Dairy Sci. 1988;71:355–365. [PubMed: 3379169]
- Steen TM, Quigley JD, Heitmann RN, Gresham JD. Effects of lasalocid and undegradable protein on growth and body composition of Holstein heifers. J. Dairy Sci. 1992;75:2517–2523. [PubMed: 1452855]
- Swanson EW. Effect of rapid growth with fattening of dairy heifers on their lactational ability. J. Dairy Sci. 1960;43:377–387.
- Swartz LA, Heinrichs AJ, Varga GA, Muller LD. Effects of varying dietary undegradable protein on dry matter intake, growth, and carcass composition of Holstein calves. J. Dairy Sci. 1991;74:3884–3890. [PubMed: 1757628]
- Tikofsky JN, Van Amburgh ME, Ross DA. Effect of varying carbohydrate and fat content of milk replacer on body composition of Holstein bull calves. J. Anim. Sci. 2001;79:2260–2267. [PubMed: 11583412]
- Tozer PR, Heinrichs JA. What affects the costs of raising replacement dairy heifers: A multiple-component analysis. J. Dairy Sci. 2001;84:1836–1844. [PubMed: 11518308]
- Van Amburgh ME, Soberon F, Meyer MJ, Molano RA. Integration of postweaning nutrient requirements and supply with composition of growth and mammary development in modern dairy heifers. J. Dairy Sci. 2019;102:3692–3705. [PubMed: 30660424]
- Volkmann N, Kemper N, Romer A. Impacts of prepubertal rearing intensity and calf health on first lactation yield and lifetime performance. Ann. Anim. Sci. 2019;19:201–214.
- von Soosten D, Meyer U, Piechotta M, Flachowsky G, Dänicke S. Effect of conjugated linoleic acid supplementation on body composition, body fat mobilization, protein accretion, and energy utilization in early lactation dairy cows. J. Dairy Sci. 2012;95:1222–1239. [PubMed: 22365206]
- Waldo DR, Tyrrell HF, Capuco AV, Rexroad CE Jr. Components of growth in Holstein heifers fed either alfalfa or corn silage diets to produce two daily gains. J. Dairy Sci. 1997;80:1674–1684. [PubMed: 9276807]
- Whitlock BK, VandeHaar MJ, Silva LFP, Tucker HA. Effect of dietary protein on prepubertal mammary development in rapidly-growing dairy heifers. J. Dairy Sci. 2002;85:1516–1525. [PubMed: 12146484]
- Zanton GI, Heinrichs JA. Meta-analysis to assess effect of prepubertal average daily gain of Holstein heifers on first-lactation production. J. Dairy Sci. 2005;88:3860–3867. [PubMed: 16230691]
- Zanton GI, Heinrichs JA. The effects of controlled feeding of a high-forage or high-concentrate ration on heifer growth and first-lactation milk production. J. Dairy Sci. 2007;90:3388–3396. [PubMed: 17582124]
- Zanton GI, Heinrichs AJ. Rumen digestion and nutritional efficiency of dairy heifers limit-fed high forage ration to four levels of dry matter intake. J. Dairy Sci. 2008;91:3579–3588. [PubMed: 18765616]
- INTRODUCTION
- ENERGY AND PROTEIN REQUIREMENTS FOR GROWING DAIRY HEIFERS
- EFFECTS OF PLANE OF NUTRITION ON FUTURE MILK PRODUCTION
- TOTAL REQUIREMENTS FOR METABOLIZABLE ENERGY AND METABOLIZABLE PROTEIN
- TARGET BODY WEIGHTS AT BREEDING AND CALVING
- PREDICTING GAIN FROM AVAILABLE METABOLIZABLE ENERGY AND METABOLIZABLE PROTEIN
- HEIFER GROWTH PROGRAMS
- MODEL LIMITATIONS AND RESEARCH NEEDS
- REFERENCES
- Growth - Nutrient Requirements of Dairy CattleGrowth - Nutrient Requirements of Dairy Cattle
- CHGG_02595 [Chaetomium globosum CBS 148.51]CHGG_02595 [Chaetomium globosum CBS 148.51]Gene ID:4389924Gene
Your browsing activity is empty.
Activity recording is turned off.
See more...