NCBI Bookshelf. A service of the National Library of Medicine, National Institutes of Health.
National Academies of Sciences, Engineering, and Medicine; Division on Earth and Life Studies; Board on Agriculture and Natural Resources; Committee on Nutrient Requirements of Dairy Cattle. Nutrient Requirements of Dairy Cattle: Eighth Revised Edition. Washington (DC): National Academies Press (US); 2021 Aug 30.
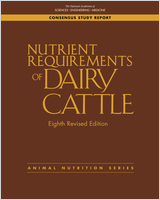
Nutrient Requirements of Dairy Cattle: Eighth Revised Edition.
Show detailsGROUP HOUSED WITH TOTAL MIXED RATIONS
Group housing with total mixed ration (TMR) feeding is the predominant production system used on commercial dairy farms in the United States (Schingoethe, 2017). In addition, most nutrition research with dairy cattle uses TMRs; therefore, no adjustments are needed to the nutrient requirements discussed in individual chapters when cows are fed a TMR. However, cow grouping strategies need to be considered when setting ration formulation parameters. Advantages of a TMR system over component feeding (e.g., concentrate separate from forage or hay separate for silage) include (Schingoethe, 2017) the following: (1) increased, but not absolute, control of what cows consume, making feeding balanced diets easier; (2) ability to include wet ingredients such as brewers grains into the diet; (3) reduced negative effect when including less palatable ingredients in diets; (4) ability to increase energy intake while reducing the risk of rumen upsets such as acidosis; (5) greater feed efficiency (Holter et al., 1977); and (6) increased mechanization and reduced labor costs.
Ideally, in a well-mixed TMR, every mouthful of the ration should provide the exact blend of nutrients that was formulated; however, in reality, cows sort diets and in most situations appear to select against longer particles (Miller-Cushon and DeVries, 2017). Dry matter (DM) concentration of the TMR over a range of about 45 to 65 percent does not consistently affect sorting (Felton and DeVries, 2010; Fish and DeVries, 2012), but including liquid molasses in the TMR (approximately 4 percent of diet DM) reduced sorting (DeVries and Gill, 2012). Perhaps the diet factor that has the greatest effect on cow sorting is particle size. Diets with a larger proportion of longer particles are more easily sorted than diets with more uniform particle size (Kononoff et al., 2003; Leonardi and Armentano, 2003; Onetti et al., 2004; Leonardi et al., 2005). Because large particles are usually forage with high-fiber concentrations, selecting against large particles can increase energy intake, but it also increases the risk for acidosis and rumen upset (see Chapter 5). A cow's desire or ability to sort may be associated with that cow's risk for acidosis (Coon et al., 2019).
Diet Formulation for Groups of Cows
Most confinement dairy farms and some grazing farms have multiple pens (or paddocks). With multiple pens, decisions must be made regarding how cows will be grouped within pens and what the different groups will be fed. Numerous factors go into these decisions such as herd demographics (i.e., distribution among parities, stage of lactation, milk yield distributions, reproductive stage, etc.), size of pens, size of mixer wagon, feed and forage inventories, and feed and milk prices. It is beyond the scope of this section to discuss all of these; readers are referred to a review by Cabrera and Kalantari (2015). The necessity of having a dry cow group and feeding them a specific diet has been known for decades and will not be discussed. The value of transition groups is discussed in Chapter 12.
Independent of any diet differences, separating first-lactation cows from more mature cows often improves milk production and increases behaviors that likely will improve health (e.g., increased lying time) (Krohn and Konggaard, 1979; Phillips and Rind, 2001). Some data (Krohn and Konggaard, 1979) suggest that the benefits of separating first-lactation cows diminish as group size gets larger (>70 cows per pen); however, in that experiment, multiple factors were confounded with group size. The benefits of separating first-lactation cows likely are related to social rank because first-lactation cows often have low rank and cannot compete effectively for resources when housed with older cows.
Based mostly on computer simulations, grouping cows according to nutritional needs (within parity) usually increases income over feed costs (Williams and Oltenacu, 1992; St-Pierre and Thraen, 1999; Kalantari et al., 2015). Two major questions arise with respect to grouping: (1) what criteria should be used to group cows, and (2) what diet formulation specifications should be used for a group of cows as contrasted to a single cow? Grouping to reduce variation in requirements for metabolizable protein (MP) and net energy within a group is usually economically optimal (Cabrera and Kalantari, 2015; Bach et al., 2020). To realize the saving in feed cost caused by grouping, diets must be formulated correctly for each group. Direct experimental evidence is lacking regarding optimal formulation strategies for different groups, but computer simulation models have been used. Formulating a diet to meet the requirements of an average cow in a pen will likely result in a loss of production from the pen. This is because cows that have lower requirements than the average will likely not increase milk yield in response to additional nutrients (i.e., production is limited by something other than nutrition), but cows that have requirements substantially greater than the average cow will consume inadequate nutrients and production will decrease. The degree of overformulation (i.e., the excess supply of a nutrient relative to the requirement for the average cow in the group) depends on variation in requirements within the pen, feed costs, environmental regulations, and the degree to which intake and production are correlated (Cabrera and Kalantari, 2015). Milk yield and dry matter intake (DMI) have a moderate positive correlation (Hristov et al., 2004); however, the correlation is much weaker in early lactation than in later lactation (Kramer et al., 2008). A strong correlation between DMI and milk yield implies that supply of nutrients will be greater by high-producing cows than low-producing cows when fed the same diet because of differences in DMI. The typical range in marginal response in milk yield to increased DMI is about 2 kg of milk per 1-kg increase in DMI (Bach et al., 2020) when very early lactation cows are excluded. Depending on the variation in milk yields within a pen, expected differences in DMI likely will not be enough to provide adequate nutrients, especially MP, to high-producing cows when diets are formulated for group-average milk yield. A high-producing cow will produce more milk than the average cow, but milk yield will be less than if a more nutrient-dense diet were fed. Stallings and McGilliard (1984) were among the first to propose factors (i.e., lead factors) that could be used to formulate diets for groups of cows. They concluded that diets for a pen of cows should be formulated to meet the energy and protein needs of the average cows plus 1 standard deviation in milk yield. The majority of cows within a pen would be consuming excess protein and energy, but high-producing cows would be fed adequately to maintain high production. Overfeeding protein has an environmental cost because excess nitrogen (N) is excreted by cows; however, excess consumed energy is retained by cows as body fat. This problem was identified using simulation models and resulted in the development of different lead factors for energy and protein (Kalantari et al., 2015). Kalantari et al. (2015) confirmed that for MP formulating for pen, mean milk yield plus 1 within-pen standard deviation is optimal; however, for net energy for lactation (NEL), diets should be formulated for pen mean production. This will result in fewer obese cows. Optimal formulation strategies need to be evaluated with actual data.
Last, grouping cows by stage of lactation rather than production can be useful in managing body condition. Because of the interaction between diet composition and stage of lactation on feed intake (see Chapter 2), diet formulation can be used to modulate energy intake and partitioning of energy between milk and body reserves.
PASTURE-BASED SYSTEMS
Nutrient Supply
Nutrient supply often differs between cows managed under grazing and confinement systems. When no supplemental feed is offered, DMI by grazing cows is almost always lower than for cows fed a TMR in confinement (Bargo et al., 2003). Several factors affect DMI in both confinement and grazing cattle (discussed in Chapter 2), but chewing fatigue and time available to graze can be additional intake constraints for grazing cows. In addition to dietary factors that affect intake under all management systems, pasture allowance (PA), which is the amount of consumable herbage offered per cow per unit of land area; density and height of the sward; and DM concentration of the herbage can affect intake by grazing cattle (Bargo et al., 2003). Taller plants and denser swards will increase DMI assuming no change in forage quality (Rook et al., 1994; Gibb et al., 1999). However, for grazing cows fed no supplemental feed, PA has the greatest effect on DMI. As PA increased, intake of herbage DM increased quadratically, reaching a plateau when PA was approximately 110 kg of DM/cow/d (Bargo et al., 2003) for average Holstein cows grazing high-quality herbage. The recommended equation to estimate DMI when cows are not given supplemental feed is as follows:
The average body weight (BW) of cows included in that meta-analysis (Bargo et al., 2003) was not presented, but it likely is based mostly on Holstein data; therefore, Equation 13-1 will overestimate DMI for Jersey cows. The above equation is grazing cattle consuming only herbage; however, supplemental concentrates are often fed to grazing cows. Bargo et al. (2003) evaluated the accuracy of different equations to estimate total DMI by grazing dairy cows fed supplemental concentrate and concluded the DMI equation developed by NRC (2001) for confinement dairy cows was acceptable. Equation 2-1 (Chapter 2) is recommended to estimate DMI by grazing cows fed supplemental concentrates. A more complex equation (Caird and Holmes, 1986) that required more inputs (including sward height, pasture allowance, and amount of concentrate fed) was also accurate.
For this discussion, concentrates include starchy, fibrous, and proteinaceous feedstuffs derived from the seed portion of plants. The type of concentrate, especially starchy versus fibrous, can affect responses as discussed below. Providing supplemental concentrates increases DMI and milk yield, but DMI is often still less than that for cows fed a TMR (Bargo et al., 2003; Roche et al., 2006; Golder et al., 2014; Auldist et al., 2016). Supplementing concentrate twice daily at milking times increases total DMI but usually reduces consumption of herbage DM. Across numerous studies, substitution rates for concentrates (i.e., kilograms of reduced herbage DMI/kg of consumed concentrate DM) range from about 0.2 to 0.7 (Bargo et al., 2003). The substitution rate tends to increase as PA increases and is greater when starchy concentrates are fed compared to fibrous concentrates. If concentrates are blended with forages, the substitution rate for the blend appears similar to that of concentrate alone (Bargo et al., 2002; Auldist et al., 2012, 2016).
Providing concentrates, especially starchy concentrates, usually (Bargo et al., 2003; Doyle et al., 2005) but not always (Reis and Combs, 2001) reduces neutral detergent fiber (NDF) digestibility. The negative effect on NDF digestibility when supplemental concentrates are fed often results in little or no improvement in energy digestibility of the total diet; however, intake of digestible energy usually increases. Based on rumen pH, volatile fatty acid (FA) patterns, and other measures, feeding concentrate blended with forage results in a more stable rumen (Golder et al., 2014; Greenwood et al., 2014; Auldist et al., 2016). This has not, however, resulted in consistently improved digestibility of DM or fiber compared with supplementing concentrates separate from forage (Bargo et al., 2002; Greenwood et al., 2014). The effect of increasing supplemental concentrate on intake of metabolizable energy is likely not linear and probably follows a diminishing return function. Doyle et al. (2005) calculated that the increase in metabolizable energy intake becomes marginal when more than about 8.5 kg of DM from starchy supplements was fed.
Grazing cattle often have lower N use efficiency (grams of milk N/g of N intake) than cows fed a TMR. When grazing cows are fed starchy concentrates, urinary excretion of N decreases, and milk protein yield and concentration and N use efficiency increase (Stockdale, 2004; Sairanen et al., 2005; Roche et al., 2013). However, these data should not be interpreted to imply efficiency of nutrient use differs because of management system. It likely reflects nutrient composition of diets under the different systems. Indirect measures (e.g., urine allantoin) have been used to estimate microbial protein synthesis by grazing cattle (Carruthers et al., 1996; Carruthers and Neil, 1997), and efficiency of microbial protein synthesis (g/g of digested organic matter) was similar to values obtained with cows fed a TMR. Silva et al. (2014) measured lower efficiency for microbial protein synthesis for grazing cows fed supplemental concentrate compared with the efficiency used by the previous NRC (2001), but that study did not include a treatment with cattle fed a TMR to allow direct comparison.
Fresh forages usually have high concentrations of β-carotene and α-tocopherol (see Chapter 8); therefore, supply of those vitamins from the basal diet can be high for grazing cattle. This should reduce the need for supplemental vitamin E, β-carotene, and vitamin A. Grazing cattle can also have greater exposure to sunlight than confined cattle, which likely reduces the need for supplemental vitamin D. Some data with sheep have shown that soil ingestion reduces copper (Cu) absorption (Suttle et al., 1984). However, in another study with sheep, soil ingestion did not affect liver Cu concentrations (Grace et al., 1996). Sheep tend to graze herbage closer to the ground than cattle, and soil ingestion may be less an issue with cattle than with sheep. Grazing cattle can have low magnesium absorption, but this is likely a function of high potassium rather than any unique aspect of grazing (see Chapter 7).
Nutrient Requirements
The requirements for grazing cattle do not differ from confinement cattle for any nutrient except energy and perhaps protein. Grazing cows expend more energy harvesting feed (walking to collect herbage, prehension, and chewing) than do cows fed a TMR. Because of topography and location of the paddocks, grazing cows also may expend more energy walking to and from the milking center than do cows in confinement. However, the distance between pens and the milking system can be substantial in some confinement systems. Although these energy costs are real, they are currently difficult to quantify, and many necessary inputs will not be known under most situations. However, with pedometers, global positioning devices, and topography maps, these inputs can be known with high accuracy. Energy expended walking within a paddock depends on size of the paddock, topography, and allowance of pasture. Many of these effects have not been quantified or modeled; therefore, energy expended walking within a paddock was assumed to equal the energy expended within a pen. That energy expenditure is incorporated into the maintenance term. Energy expended by walking to and from the milking center is a function of distance, topographical elevation changes, and BW of the cow. Reasonable estimates of BW and distance traveled to and from the milking center can be obtained under field conditions; therefore, that expenditure of energy is calculated as a separate component (i.e., activity). In the seventh revised edition (NRC, 2001), the energetic cost of horizontal locomotion was set at 0.00045 Mcal NEL/kg BW per kilometer. Based on newer data derived from beef cows (Brosh et al., 2006; Aharoni et al., 2009; Brosh et al., 2010), the energy cost for horizontal locomotion for cattle was set at 0.00035 Mcal NEL/kg of BW per kilometer of total distance walked between the paddock and milking center (approximate range in measured values was 0.0003 to 0.0004 Mcal/kg BW per kilometer). This represents a 22 percent decrease in energy required for horizontal walking from NRC (2001); however, this may still be an overestimation of the cost. D'Hour et al. (1994) reported no difference in milk production or blood nonesterified FA concentrations when grazing cows were forced to walk an additional 6.4 km/d over flat ground. Milk yields started to decrease when cows were forced to walk an additional 10 km/d. Vertical distance traveled is more difficult to estimate, and measuring its energetic cost is less precise than for horizontal distance. For cattle, estimates for energetic cost of vertical locomotion have ranged from about 9 to 19 times the cost of horizontal locomotion (Di Marco and Aello, 1998; Aharoni et al., 2009; Brosh et al., 2010). Because of all of the uncertainties related to measurement, the committee chose the highest value and estimated the cost of vertical locomotion as 0.0067 Mcal NEL/kg of BW per kilometer. Because of newer data, this is a substantial reduction in energy expenditure for vertical locomotion compared with the previous NRC (2001). For a 650-kg cow walking 0.2 km of vertical distance, activity requirement is currently 0.9 Mcal of NEL compared with 3.9 Mcal NEL/d based on NRC (2001). However, NRC (2001) used an incorrect efficiency value for work associated with vertical distance. The value in the NRC (2001) example should only be 1.4 Mcal NEL/d. The value used in this edition is based on a broader set of experimental data and is likely more accurate than both the previous incorrectly calculated value and the corrected value. In the model, energy associated with vertical distance can be calculated from user-entered vertical distance (if known) or, to better reflect the qualitative nature of the estimated requirements for vertical travel, qualitative descriptors can be selected: mild (0.05 km of total vertical distance per day), moderate (approximately 0.2 km of vertical distance), and severe (approximately 0.5 km of vertical distance). These three classes result in 0.2, 0.9, and 2.2 Mcal of NEL expended per day for a 650-kg cow.
The amount of energy expended by the animal harvesting pasture depends on amount of herbage consumed and on PA. When PA is reduced, cows expend more energy to gather food. Angus steers (BW = 259 kg) expended 3.3 times more energy grazing pasture that contained 148 g of DM/m2 compared with pasture at 228 g DM/m2 (Di Marco et al., 1996). No supplemental feed was provided in that study. Estimating the energy required for grazing (prehension, mastication, and walking while grazing) is difficult, and data are both limited and highly variable. Estimated energy expended for grazing ranged from about 0.003 to 0.025 Mcal/kg BW0.75 per day when cattle were fed no supplemental concentrate (Di Marco et al., 1996; Aharoni et al., 2009; Brosh et al., 2010). The average was 0.0075 Mcal/kg BW0.75, which would be the cost of food gathering (in excess of that in confinement) when no supplemental concentrate was fed. Cattle in those studies grazed about 10 hours per day. Dairy cattle fed no supplemental concentrate also graze about 10 hours per day, and on average, that is reduced by 12 minutes for every kilogram of concentrate DM fed (Bargo et al., 2003). The equation in the model adjusts grazing time based on supplemental feeds (which could include corn silage, hay, concentrate, etc.). Changing the amount of nonpasture intake from 2 to 12 kg/d reduces energy expenditure by about 0.2 Mcal for a 650-kg cow. The model calculates daily NEL required for grazing as follows:
Therefore, the total activity requirement for grazing cattle will include horizontal locomotion between the paddock and milking center adjusted for positive vertical distance traveled plus activity associated with gathering food. As an example, a 650-kg cow fed 6 kg of concentrate daily grazing a pasture located 0.6 km from the milking center with a total of 0.2 km change in elevation (i.e., moderate) that makes four one-way trips daily will have an estimated grazing activity requirement of the following:
The energetic cost of grazing for heifers is not known. During a 9-hour period (0700 to 1600 h), Holstein and Holstein × Jersey heifers only walked about 2 km after they had adapted to grazing, which takes 5 to 8 days after being first introduced to a grazing system (Lopes et al., 2013). In that study, for the first 8 days after being put on pasture, heifers walked 2 to 5.5 km per 9 hours. Assuming reasonably flat ground, this walking would not be a major energy expenditure. On hilly ground and on sparse pasture, energy expended to graze would be higher.
The equations used to estimate grazing energy requirements are based on the best available data; however, accurate inputs will limit the overall accuracy of the equations. Users should know the amount of concentrate consumed and the approximate distance between the paddock and milking parlor, but vertical distance traveled will usually not be known with accuracy. The additional work expended by grazing cattle may increase protein requirement. Relative to maintenance, strenuous exercise by humans can increase energy expenditure by a factor of 10, but the protein requirement only doubled, and some of the increased protein requirement was to replace amino acids that were oxidized to provide energy (Lemon, 1998). In most situations, the work associated with grazing is not strenuous, and effects on protein requirements are probably small.
FEEDING AND AUTOMATIC MILKING SYSTEMS
Automatic milking systems (AMSs) can be used to conduct daily milking routines (Jacobs and Siegford, 2012). In 2016, as many as 15,000 commercial dairy farms worldwide were using AMSs (Rodenburg et al., 2017). By design, cow movement or traffic differs in facilities equipped with AMSs. Free-flow traffic design refers to facilities that allow cows unrestricted access to all animal areas of the barn. Guided-flow traffic refers to facilities that are equipped with one-way and selection gates. These gates are used to manage traffic by guiding cows to milking, feeding, and resting areas of the barn. Guided-flow designs may be further distinguished by two different flow patterns, namely, “milk first” and “feed first.” In milk-first designs, cows exiting the resting area pass through a selection gate. If she is eligible for milking, the gate will operate and guide her to the AMS, but if she is not eligible, it will guide her to the area where feed is located, and she can only reenter the resting area through a one-way gate. The flow of cows through feed-first designs is reversed; cows exiting the feeding area pass through a selection gate. If she is eligible for milking, the gate will operate to guide her to the AMS, but if she is not eligible, it will guide her to the resting area (Endres and Salfer, 2017).
Regardless of the design, a portion of the nutrients supplied to the cow is usually offered during milking times when the cow enters the AMS. This is usually offered in the form of a pellet and intended to supply her with nutrients but also as a reward for visiting the AMS. The mixed feed fed to cows in this system is often referred to as a partially mixed ration or PMR (Bach and Cabrera, 2017). The feeding strategies of free-flowing and feed-first guided designs are similar, while in milk-first guided designs, the amount of feed offered as a reward by the AMS is low, and a greater portion of the nutrients is supplied in the mixed ration available in the feed bunk. Offering more nutrients in the PMR is often more economical than feeding more pellets in the AMS. In general, managers of AMSs strive to have all cows reach a set minimum of visits to an AMS and that these visits be spaced out across the day. The number of visits to the AMS is influenced by the nature of the reward and by other management, environmental, and animal factors that may work to dampen the cows' urge to reach the offering or impede visit to the AMS itself (Bach and Cabrera, 2017). Research manipulating the amount or concentration of nutrients offered by the AMS is lacking. Survey data have indicated that on average, North American producers offer 15.9 kg of concentrate for every 100 kg of milk (Tremblay et al., 2016). As mentioned above, cows are often offered a pelleted feed in the AMS. The pelleting process results in a feed that is easier to handle and also of higher density, but very little research has been conducted on the effects of the pelleting process on digestibility and rumen fermentation. In one study, cows consuming pelleted oats had greater fiber digestion than those consuming rolled or flaked oats (Tosta et al., 2019). Conversely, in one study, pelleting a TMR reduced fiber digestibility (Bofante et al., 2016). Additional research should be conducted on the impacts of the pelleting process on nutrient availability of feeds when they are pelleted and offered in an AMS. Data are also lacking on factors that affect feed preference, but because this may affect frequency of visits to the AMS, then it should be considered in formulation procedures.
Amount of Reward
Because the nutritional needs of a lactating cow are influenced by milk yield, it logically follows that increasing the amount of concentrate offered would increase milk yield, but this is not always observed in an AMS (Bach et al., 2007; Tremblay et al., 2016; Paddick et al., 2019). This may be because not all feed offered is consumed; a positive but nonlinear relationship between the amount of concentrate offered to the cow in an AMS and that refused exists. When more than 4 kg of concentrate is offered at a milking event, the amount of unconsumed concentrate often increases (Bach and Cabrera, 2017). Increasing the concentrate offered may also reduce the amount of PMR consumed and reduce overall DMI but not affect milk yield (Bach et al., 2007). In a feed-first guided-traffic flow barn, providing greater proportions of nutrients in the PMR and not in the AMS may be beneficial and stimulate feed intake. Hare et al. (2018) observed that for every 1 kg of concentrate provided in the AMS, the PMR intake was reduced by 1.58 kg, and in this system, large quantities of feed offered in the AMS were likely not needed. The relationship in substitution ratio is not consistent across studies and ranges from 0.84 to 1.58 (Paddick et al., 2019). Within the same system, these investigators also observed that the forage to concentrate ratio in the PMR and the amount of concentrate offered in the AMS may work as independent factors influencing feed intake and production. Specifically, increasing concentrate offered from 2 to 6 kg/d in the AMS reduced intake of PMR with marginal effects on production but increased variability of AMS concentrate consumption. In contrast, increasing concentrate contained in the PMR increased milk production but did not affect variability of feed intake from either the PMR or AMS (Menajovsky et al., 2018).
Even less research has been conducted in pasture-based systems, and observed effects on visitation to the AMS from increasing the amount of the reward are conflicting (Jago et al., 2007; Lessire et al., 2017). In addition to the reward itself, pasture allocation may have significant effects on intervals of time between milking and milking frequencies (Lyons et al., 2013). Additional research is needed to determine the nature of reward amount and the effect on rumen fermentation, as well as milk yield and composition.
Reward Composition
Effects on number of visits to the AMS by manipulation of the composition of the concentrate feed reward have been evaluated in several studies. Manipulations include starch content, grain type, and flavoring. Increasing the concentration of starch in the concentrate did not affect the frequency of visits to the AMS (Miron et al., 2004; Halachmi et al., 2006, 2009), and milk production increased in only one study (Halachmi et al., 2009). A study designed to test the effect of preference for a range of different ingredients (barley, wheat, barley–oat mix, corn, grass, fat) on the frequency of visits to the AMS showed that the barley–oat mixture was most preferred so the frequency of visits increased when the feed offered was barley–oat mixture (Madsen et al., 2010). In addition, pellets containing grass and fat resulted in the greater proportion of fetch cows (cows that had to be brought manually to the AMS), suggesting that these ingredients were least preferred. Flavoring the concentrate in the AMS increased the frequency of visits in one study (Migliorati et al., 2009) but not in another (Migliorati et al., 2005). In a study evaluating a molasses-based liquid feed supplement, no differences were observed in either milk production or visits to the AMS, but some measures of metabolic and overall health such as β-hydroxybutyrate and body condition score (BCS) were improved (Moore et al., 2020). The composition of concentrate may have some effect on cows visiting the AMS, but research is too limited to make broad recommendations.
With AMS, cows usually consume nutrients from more than one location (i.e., the concentrate offered by the AMS and a PMR). The supply of minerals and vitamins from both sources should be considered. In addition, by changing the amount of concentrate provided to individual cows, AMS offers the potential for users to adjust diets for individual animal factors such as milk yield, body condition, pregnancy status, health status, age, and growth (André et al., 2009; Bach and Cabrera, 2017; King et al., 2018). Given the rapid adoption of AMS by the dairy industry, there is an urgent need to determine how manipulation of feeding practices and nutritional manipulations may improve production, health, and welfare of dairy cattle.
ORGANIC DAIRY SYSTEMS
All available evidence indicates that nutrient requirements do not differ between dairy cattle managed under an organic-certified system or a conventional system; however, because of economics and regulations, nutrient supply can differ between systems. At least during a portion of the year, organically managed dairy cows must graze, which affects nutrient requirements (discussed above); however, those effects would be the same under conventional grazing systems. In reality, energy expenditure for grazing likely will be greater for organic herds simply because on average, less supplemental feed is given.
Direct comparisons of nutrient composition between feeds grown under organic conditions and those grown conventionally are limited, but most data indicate that at the macronutrient level, organic feedstuffs and conventional feedstuffs are essentially equal (e.g., Kyntäjä et al., 2014). Most studies find little difference in macronutrients between organically grown and conventionally grown human foods, but concentrations of some minerals are often greater in organically grown foods (Bourn and Prescott, 2002). However, mineral concentrations in organically grown hay crop forages and barley grain did not differ substantially from their conventionally grown counterparts, perhaps because manure may have been used as a fertilizer under both systems, and factors other than type of farming system (e.g., year variation) were more important (Gustafson et al., 2007). Although nutrient composition of organic feeds generally does not differ greatly from their conventional counterparts, because of cost and availability, feedstuff choice and diet (not feed) composition can differ between systems. Organic by-product feeds such as distillers grains, brewers grains, and cottonseed are not readily available and not commonly fed to organic herds (Sorge et al., 2016). Forages are usually the primary fiber source. Because of cost (and, in some countries, organic regulations), concentrate inclusion rates are typically lower for organic herds than conventional herds. However, increasing supplementation of concentrates on organic farms is associated with greater milk production (Sehested et al., 2003; Hardie et al., 2014) and greater income over feed costs (Hardie et al., 2014). This likely is related to increased DM and energy intake that often occurs when forage-based diets are supplemented with increasing amounts of concentrates. Similar to what is observed with conventional herds, type of forage (corn silage versus hay crop silage) did not affect milk yields in organic farms, but feed costs were significantly greater for those fed corn silage because of the need to purchase organic protein supplements (Marston et al., 2011). Cows fed organically grown rapeseed meal had similar yields of milk and milk components as cows fed conventionally grown rapeseed meal (Khalili et al., 1999).
Similar to what would be expected with conventionally fed dairy cows, milk and milk component yields are increased when cows are fed organic diets that are properly formulated to meet nutrient requirements (e.g., fiber, protein, and energy) rather than when a single-ingredient concentrate such as barley or beets (Mogensen and Kristensen, 2003) is supplemented. With conventional diets, substituting about 6 percent molasses for ground corn can increase milk and milk component yields (Broderick and Radloff, 2004). However, in a study with organically fed cows, replacing cornmeal with molasses resulted in linear decreases in milk and milk component yields (Ghedini et al., 2018). This may be a result of very different dietary starch concentrations between the two studies. In the study with organically managed cows, diets were low in starch (decreased from 10 to 2 percent as molasses was added) but ranged from 23 to 31 percent with the conventional diets. Because of cost, organic diets are often lower in starch than conventional diets, and that may limit the value of molasses.
Mineral nutrition of organically managed dairy herds is essentially the same as conventionally managed cows because in the United States, many of the mineral supplements commonly fed are approved for organic farms. Mineral concentrations in milk from organically managed herds are generally similar to milk from conventional herds and depend more on total diet concentrations of minerals rather than production system (Schwendel et al., 2015). In other countries that are more restrictive relative to supplemental minerals, milk from organic herds can have lower concentrations of several trace minerals (Cu, iodine, selenium, and zinc) because dietary concentrations are less (Rey-Crespo et al., 2013). Mineral requirements include minerals secreted into milk; however, the differences obtained, although statistically different, are quantitatively small and would have little impact on overall mineral requirements. Vitamin requirements are likely not different between organically managed herds and conventional herds. However, organic programs require cows to graze a portion of the year, which can affect the need for supplemental vitamins (see above section on grazing systems). Based on blood concentrations of retinol, β-carotene, and α-tocopherol, cows managed organically with a diet based on pasture or high-quality hay crop silage (no corn silage) were in adequate vitamin status without any supplemental synthetic vitamins during most of the lactation cycle (Johansson et al., 2014). However, cows not fed supplemental vitamin E had less than recommended concentrations of α -tocopherol in blood at calving.
Organic dairy producers frequently feed cattle a number of ingredients that are less frequently used in conventional systems. One of these ingredients is brown seaweed, Ascophyllum nodosum (kelp meal) (Sorge et al., 2016). It is commonly used as a mineral supplement and is rich in several macrominerals and iodine. The high iodine concentration (and possibly other components) is thought to improve the health of cows; however, this has not been observed in experimental conditions (Antaya et al., 2015, 2019).
GENETICALLY ENGINEERED CROPS AND DAIRY CATTLE
Genetically engineered, commonly referred to as genetically modified (GM), crops have historically been developed to minimize the extent of insect damage and to simplify herbicide use for weed management (Benbrook, 2004). From 1996 to 2019, the global area growing GM crops increased from 1.7 to 190.4 million hectares. The United States plants approximately 71.5 million hectares of GM crops, followed by Brazil (52.8 million hectares), Argentina (24.0 million hectares), Canada (12.5 million hectares), and India (11.9 million hectares). Of the total area planted worldwide, GM soybean, corn, cotton, and canola represent 48, 32, 14, and 5 percent, respectively (ISAAA, 2019). Globally, livestock probably consume 70 to 90 percent of the GM crops produced, while in the United States, 95 percent of food-producing animals may consume diets containing GM ingredients (Van Eenennaam and Young, 2014). Existing evidence for the potential negative consequences and positive benefits of the commercialization of GM crops has been evaluated and reviewed (NASEM, 2016). That committee suggested that producers of soybeans, corn, and cotton have experienced positive economic outcomes through improvements in productive efficiencies. The committee also reviewed several peer-reviewed publications (Phipps et al., 2003; Nemeth et al., 2004; Calsamiglia et al., 2007; Guertler et al., 2009; Rizzi et al., 2012; Einspanier, 2013; Furgał-Dierżuk et al., 2015) that examined milk from dairy cows consuming GM crops. None reported the detection of whole transgenes or GM proteins in the milk these animals produced; however, fragments of chloroplast DNA have been detected. These conclusions are supported by a more recent review further supporting the notion that recombinant DNA cannot be reliably or consistently detected in milk from dairy cows consuming GM feedstuffs (Van Eenennaam and Young, 2017).
Presently, GM corn traits designed to specifically improve the nutritional quality or feed value of corn silage are not available, and those commercially available are designed to facilitate agronomic practices (e.g., herbicide and insect resistance) or, as in one case, industrial ethanol production. More recently, a corn containing an α-amylase enzyme that is activated during the dry milling ethanol process has also been introduced. Improvements in feed efficiency in beef cattle consuming this corn have been reported (Jolly-Breithaupt et al., 2019). In dairy studies containing corn silage incorporating the α-amylase enzyme, improved milk and protein yields have been reported (Rebelo et al., 2020; Welchez et al., 2020). There are also GM corn hybrids that contain nutritional enhancements, but these traits have been introduced through conventional breeding practices and not through genetic engineering. One example is the brown midrib trait for reduced lignin and improved fiber digestibility. In general, there is little difference in the chemical composition of corn silage GM hybrids and genetically similar non-GM counterparts. Thus, it is not surprising that a meta-analysis comparing GM hybrids and isoline controls did not find any differences in milk production and composition (Ferraretto and Shaver, 2015). Genetic engineering may serve as a tool to manipulate the chemical composition of feedstuffs, and this, in turn, may be beneficial in improving efficiency and altering milk composition. Two examples of this are GM reduced-lignin alfalfa and high–oleic acid soybeans. Lignin negatively affects fiber digestion (Palmonari et al., 2014); thus, reducing the lignin content of alfalfa may be advantageous. A GM reduced-lignin alfalfa is now commercially available (McCaslin et al., 2014). To date, no feeding studies evaluating fiber digestibility and milk production in dairy cattle fed these commercialized reduced-lignin alfalfa varieties have been published. In a study using growing Angus heifers, feeding reduced-lignin alfalfa did not affect DMI, BW, or average daily gain (Staudenmeyer et al., 2017). In addition, in vitro fiber digestibility was similar, but in vivo fiber digestibility was not tested. Other studies have demonstrated that the GM reduced-lignin alfalfa forage had less lignin and higher in vitro NDF digestibility compared with reference alfalfa varieties when harvested at the same time (Grev et al., 2017; Sulc et al., 2017). Linolenic and linoleic acids (18:2 and 18:3) play a role in milk fat depression; thus, reducing their concentration in soybeans may prove beneficial when they are fed to cows. In addition, increased intake of 18:1 by cows results in more monounsaturated FAs in milk, and this may improve milk quality, especially as it relates to consumer perceptions and expectations. A GM high–oleic acid soybean has been developed and results in greater 18:1 and less 18:2 and 18:3 FAs (Szabala et al., 2014; Lopes et al., 2017). Cows fed extruded soybean meal from high–oleic acid soybeans had increased milk fat concentration and reduced trans fatty acids in milk compared with cows fed extruded meal from conventional soybeans (Lopes et al., 2017).
REFERENCES
- Aharoni Y, Henkin Z, Ezra A, Dolev A, Shabtay A, Orlov A, Yehuda Y, Brosh A. Grazing behavior and energy costs of activity: A comparison between two types of cattle. J. Anim. Sci. 2009;87(8):2719–2731. [PubMed: 19395522]
- André G, Bleumer EJB, van Duinkerken G. Evaluation of an application for dynamic feeding of dairy cows. Proc. Precis. Livestock Farm. 2009;9:25–32.
- Antaya NT, Soder KJ, Kraft J, Whitehouse NL, Guindon NE, Erickson PS, Conroy AB, Brito AF. Incremental amounts of Ascophyllum nodosum meal do not improve animal performance but do increase milk iodine output in early lactation dairy cows fed high-forage diets. J. Dairy Sci. 2015;98:1991–2004. [PubMed: 25547299]
- Antaya NT, Ghelichkhan M, Pereira ABD, Soder KJ, Brito AF. Production, milk iodine, and nutrient utilization in Jersey cows supplemented with the brown seaweed Ascophyllum nodosum (kelp meal) during the grazing season. J. Dairy Sci. 2019;102:8040–8058. [PubMed: 31279546]
- Auldist MJ, Marett LC, Greenwood JS, Hannah M, Jacobs JL, Wales WJ. Effects of different strategies for feeding supplements on milk production responses in cows grazing a restricted pasture allowance. J. Dairy Sci. 2012;96(2):1218–1231. [PubMed: 23219117]
- Auldist MJ, Marett LC, Greenwood JS, Wright MM, Hannah M, Jacobs JL, Wales WJ. Milk production responses to different strategies for feeding supplements to grazing dairy cows. J. Dairy Sci. 2016;99(1):657–671. [PubMed: 26585473]
- Bach A, Cabrera V. Robotic milking: Feeding strategies and economic returns. J. Dairy Sci. 2017;100:7720–7728. [PubMed: 28215885]
- Bach A, Iglesias C, Calsamiglia S, Devant M. Effect of amount of concentrate offered in automatic milking systems on milking frequency, feeding behavior, and milk production of dairy cattle consuming high amounts of corn silage. J. Dairy Sci. 2007;90:5049–5055. [PubMed: 17954744]
- Bach A, Terré M, Vidal M. Symposium review: Decomposing efficiency of milk production and maximizing profit. J. Dairy Sci. 2020;103:5709–5725. [PubMed: 31837781]
- Bargo F, Muller LD, Delahoy JE, Cassidy TW. Performance of high producing dairy cows with three different feeding systems combining pasture and total mixed rations. J. Dairy Sci. 2002;85:2948–2963. [PubMed: 12487461]
- Bargo F, Muller LD, Kolver ES, Delahoy JE. Invited review: Production and digestion of supplemented dairy cows on pasture. J. Dairy Sci. 2003;86:1–42. [PubMed: 12613846]
- Benbrook CM. Genetically Engineered Crops and Pesticide Use in the United States: The First Nine Years. Leopold Center Pubs and Papers 137. Ames: Iowa State University; 2004.
- Bonfante E, Palmonari A, Mammi L, Canestrari G, Fustini M, Formigoni A. Effects of a completely pelleted diet on growth performance in Holstein heifers. J. Dairy Sci. 2016;99:9724–9731. [PubMed: 27692717]
- Bourn D, Prescott J. A comparison of the nutritional value, sensory qualities, and food safety of organically and conventionally produced foods. Crit. Rev. Food Sci. Nutr. 2002;42(1):1–34. [PubMed: 11833635]
- Broderick GA, Radloff WJ. Effect of molasses supplementation on the production of lactating dairy cows fed diets based on alfalfa and corn silage. J. Dairy Sci. 2004;87:2997–3009. [PubMed: 15375061]
- Brosh A, Henkin Z, Ungar ED, Dolev A, Orlov A, Yehuda Y, Aharoni Y. Energy cost of cows' grazing activity: Use of the heart rate method and the Global Positioning System for direct field estimation. J. Anim. Sci. 2006;84:1951–1967. [PubMed: 16775080]
- Brosh A, Henkin Z, Ungar ED, Dolev A, Shabtay A, Orlov A, Yehuda Y, Aharoni Y. Energy cost of activities and locomotion of grazing cows: A repeated study in larger plots. J. Anim. Sci. 2010;88(1):315–323. [PubMed: 19717764]
- Cabrera VE, Kalantari AS. Economics of production efficiency: Nutritional grouping of the lactating cow. J. Dairy Sci. 2015;99(1):825–841. [PubMed: 26519971]
- Caird L, Holmes W. The prediction of voluntary intake of grazing dairy cows. J. Agric. Sci. 1986;107:43–54.
- Calsamiglia S, Hernandez B, Hartnell GF, Phipps R. Effects of corn silage derived from a genetically modified variety containing two transgenes on feed intake, milk production, and composition, and the absence of detectable transgenic deoxyribonucleic acid in milk in Holstein dairy cows. J. Dairy Sci. 2007;90:4718–4723. [PubMed: 17881694]
- Carruthers VR, Neil PG. Milk production and ruminal metabolites from cows offered two pasture diets supplemented with nonstructural carbohydrates. N. Z. J. Agric. Res. 1997;40:513–521.
- Carruthers VR, Neil PG, Dalley DE. Microbial protein synthesis and milk production in cows offered pasture diets differing in non-structural carbohydrate content. Proc. N. Z. Soc. Anim. Prod. 1996;56:255–259.
- Coon RE, Duffield TF, DeVries TJ. Short communication: Risk of subacute ruminal acidosis affects the feed sorting behavior and milk production of early lactation cows. J. Dairy Sci. 2019;102:652–659. [PubMed: 30447980]
- DeVries TJ, Gill RM. Adding liquid feed to a total mixed ration reduces feed sorting behavior and improves productivity of lactating dairy cows. J. Dairy Sci. 2012;95:2648–2655. [PubMed: 22541492]
- D'Hour P, Hauwuy A, Coulon JB, Garel JP. Walking and dairy cattle performance. Ann. Zootech. 1994;43:369–378.
- Di Marco ON, Aello MS. Energy cost of cattle walking on the level and on a gradient. J. Range Manage. 1998;51:9–13.
- Di Marco ON, Aello MS, Méndez DG. Energy expenditure of cattle grazing on pastures of low and high availability. Anim. Sci. 1996;63(1):45–50.
- Doyle PT, Francis SA, Stockdale CR. Associative effects between feeds when concentrate supplements are fed to grazing dairy cows: A review of likely impacts on metabolisable energy supply. Aust. J. Agric. Res. 2005;56(12):1315–1329.
- Einspanier R. The fate of transgenic DNA and newly expressed proteins. In: Flachowsky G, editor. Animal Nutrition with Transgenic Plants. Wallingford, UK: CABI Publishing; 2013. pp. 130–139.
- Endres MI, Salfer JA. Feeding practices for dairy cows milked with robotic milking systems—EXtension. 2017. [September 22, 2017]. http://articles
.extension .org/pages/73959 /feeding-practices-for-dairy-cows-milked-with-robotic-milking-systems . - Felton CA, DeVries TJ. Effect of water addition to a total mixed ration on feed temperature, feed intake, sorting behavior, and milk production of dairy cows. J. Dairy Sci. 2010;93:2651–2660. [PubMed: 20494174]
- Ferraretto LF, Shaver RD. Effects of whole-plant corn silage hybrid type on intake, digestion, ruminal fermentation, and lactation performance by dairy cows through a meta-analysis. J. Dairy Sci. 2015;98:2662–2675. [PubMed: 25648819]
- Fish JA, DeVries TJ. Short communication: Varying dietary dry matter concentration through water addition: Effect on nutrient intake and sorting of dairy cows in late lactation. J. Dairy Sci. 2012;95:850–855. [PubMed: 22281348]
- Furgał-Dierżuk I, Strzetelski J, Twardowska M, Kwiatek K, Mazur M. The effect of genetically modified feeds on productivity, milk composition, serum metabolite profiles and transfer of tDNA into milk of cows. J. Anim. Feed Sci. 2015;24:19–30.
- Ghedini CP, Moura DC, Santana RAV, Oliveira AS, Brito AF. Replacing ground corn with incremental amounts of liquid molasses does not change milk enterolactone but decreases production in dairy cows fed flaxseed meal. J. Dairy Sci. 2018;101:2096–2109. [PubMed: 29274976]
- Gibb MJ, Huckle CA, Nuthall R, Rook AJ. The effect of physiological state (lactating or dry) and sward surface height on grazing behaviour and intake by dairy cows. Appl. Anim. Behav. Sci. 1999;63(4):269–287.
- Golder HM, Denman SE, McSweeney C, Wales WJ, Auldist MJ, Wright MM, Marett LC, Greenwood JS, Hannah MC, Celi P, Bramley E, Lean IJ. Effects of partial mixed rations and supplement amounts on milk production and composition, ruminal fermentation, bacterial communities, and ruminal acidosis. J. Dairy Sci. 2014;97(9):5763–5785. [PubMed: 24997657]
- Grace ND, Rounce JR, Lee J. Effect of soil ingestion on the storage of Se, vitamin B12, Cu, Cd, Fe, Mn, and Zn in the liver of sheep fed lucerne pellets. N. Z. J. Agric. Res. 1996;39(3):325–331.
- Greenwood JS, Auldist MJ, Marett LC, Hannah MC, Jacobs JL, Wales WJ. Ruminal pH and whole-tract digestibility in dairy cows consuming fresh cut herbage plus concentrates and conserved forage fed either separately or as a partial mixed ration. Anim. Prod. Sci. 2014;54(8):1056–1063.
- Grev AM, Wells MS, Samac DA, Martinson KL, Sheaffer CC. Forage accumulation and nutritive value of reduced lignin and reference alfalfa cultivars. Agronomy J. 2017;109:2749–2761.
- Guertler P, Paul V, Albrecht C, Meyer HHD. Sensitive and highly specific quantitative real-time PCR and ELISA for recording a potential transfer of novel DNA and Cry1Ab protein from feed into bovine milk. Anal. Bioanal. Chem. 2009;393:1629–1638. [PubMed: 19225766]
- Gustafson GM, Salomon E, Jonsson S. Barn balance calculations of Ca, Cu, K, Mg, Mn, N, P, S and Zn in a conventional and organic dairy farm in Sweden. Agric. Ecosyst. Environ. 2007;119:160–170.
- Halachmi I, Shoshani E, Solomon R, Maltz E, Miron J. Feeding of pellets rich in digestible neutral detergent fiber to lactating cows in an automatic milking system. J. Dairy Sci. 2006;89:3241–3249. [PubMed: 16840642]
- Halachmi I, Shoshani E, Solomon R, Maltz E, Miron J. Feeding soyhulls to high-yielding dairy cows increased milk production, but not milking frequency, in an automatic milking system. J. Dairy Sci. 2009;92:2317–2325. [PubMed: 19389990]
- Hardie CA, Wattiaux M, Dutreuil M, Gildersleeve R, Keuler NS, Cabrera VE. Feeding strategies on certified organic dairy farms in Wisconsin and their effect on milk production and income over feed costs. J. Dairy Sci. 2014;97(7):4612–4623. [PubMed: 24819131]
- Hare K, DeVries TJ, Schwartkopf-Genswein KS, Penner GB. Does the location of concentrate provision affect voluntary visits, and milk and milk component yield for cows in an automated milking system? Can. J. Anim. Sci. 2018;98:399–404.
- Holter JB, Urban WE Jr, Hayes HH, Davis HA. Utilization of diet components fed blended or separately to lactating cows. J. Dairy Sci. 1977;60:1288–1293.
- Hristov AN, Price WJ, Shafii B. A meta-analysis examining the relationship among dietary factors, dry matter intake, and milk and milk protein yield in dairy cows. J. Dairy Sci. 2004;87:2184–2196. [PubMed: 15328233]
- ISAAA (International Service for the Acquisition of Agri-biotech Applications). global Status of Commercialized Biotech/gM Crops in 2019: Biotech Crops Drive Socio Economic Development and Sustainable Environment in the New Frontier. ISAAA Brief No. 55. Ithaca, NY: ISAAA; 2019.
- Jacobs JA, Siegford JM. Invited review: The impact of automatic milking systems on dairy cow management, behavior, health, and welfare. J. Dairy Sci. 2012;95:2227–2247. [PubMed: 22541453]
- Jago JG, Davis KL, Copeman PJ, Ohnstad I, Woolford MM. Supplementary feeding at milking and minimum milking interval effects on cow traffic and milking performance in a pasture-based automatic milking system. J. Dairy Res. 2007;74:492–499. [PubMed: 17922936]
- Johansson B, Persson Waller K, Jensen SK, Lindqvist H, Nadeau E. Status of vitamins E and A and β-carotene and health in organic dairy cows fed a diet without synthetic vitamins. J. Dairy Sci. 2014;97:1682–1692. [PubMed: 24440269]
- Jolly-Breithaupt ML, Harris ME, Nuttelman BL, Burken DB, MacDonald JC, Luebbe MK, Iragavarapu TK, Erickson GE. Effects of Syngenta Enogen Feed Corn containing an α-amylase trait on finishing cattle performance and carcass characteristics. Transl. Anim. Sci. 2019;3:504–512. [PMC free article: PMC7200490] [PubMed: 32704821]
- Kalantari AS, Armentano LE, Shaver RD, Cabrera VE. Economic impact of nutritional grouping in dairy herds. J. Dairy Sci. 2015;99(2):1672–1692. [PubMed: 26686706]
- Khalili H, Kuusela E, Saarisalo E. Use of rapeseed and pea grain protein supplements for organic milk production. Agric. Food Sci. 1999;8(3):239–252.
- King MTM, Sparkman KJ, LeBlanc SJ, DeVries TJ. Milk yield relative to supplement intake and rumination time differs by health status for fresh cows milked with automated systems. J. Dairy Sci. 2018;101:10168–10176. [PubMed: 30146280]
- Kononoff PJ, Heinrichs AJ, Lehman HA. The effect of corn silage particle size on eating behavior, chewing activities, and rumen fermentation in lactating dairy cows. J. Dairy Sci. 2003;86:3343–3353. [PubMed: 14594254]
- Kramer E, Stamer E, Mahlkow K, Lüpping W, Krieter J. Relationship between water intake, dry matter intake and daily milk yield on a German research farm. Livestock Sci. 2008;115(1):99–104.
- Krohn CC, Konggaard SP. Effects of isolating first-lactation cows from older cows. Livestock Prod. Sci. 1979;6(2):137–146.
- Kyntäjä S, Partanen K, Siljander-Rasi H, Jalava T. Tables of Composition and Nutrient Value of Organically Produced Feeds Materials for Pigs and Poultry. MTT Report 164. Jok, Finland: MTT; 2014.
- Lemon PW. Effects of exercise on dietary protein requirements. Int. J. Sports Nutr. 1998;8:426–447. [PubMed: 9841962]
- Leonardi C, Armentano LE. Effect of quantity, quality, and length of alfalfa hay on selective consumption by dairy cows. J. Dairy Sci. 2003;86:557–564. [PubMed: 12647962]
- Leonardi C, Giannico F, Armentano LE. Effect of water addition on selective consumption (sorting) of dry diets by dairy cattle. J. Dairy Sci. 2005;88:1043–1049. [PubMed: 15738239]
- Lessire F, Froidmont E, Shortall J, Hornick JL, Dufrasne I. The effect of concentrate allocation on traffic and milk production of pasture-based cows milked by an automatic milking system. Animal. 2017;11(11):2061–2069. [PubMed: 28376936]
- Lopes F, Coblentz W, Hoffman PC, Combs DK. Assessment of heifer grazing experience on short-term adaptation to pasture and performance as lactating cows. J. Dairy Sci. 2013;96(5):3138–3152. [PubMed: 23522679]
- Lopes JC, Harper MT, Giallongo F, Oh J, Smith L, Ortega-Perez AM, Harper SA, Melgar A, Kniffen DM, Fabin RA, Hristov AN. Effect of high-oleic-acid soybeans on production performance, milk fatty acid composition, and enteric methane emission in dairy cows. J. Dairy Sci. 2017;100:1122–1135. [PubMed: 27988126]
- Lyons NA, Kerrisk KL, Garcia SC. Comparison of 2 systems of pasture allocation on milking intervals and total daily milk yield of dairy cows in a pasture-based automatic milking system. J. Dairy Sci. 2013;96:4494–4504. [PubMed: 23684034]
- Madsen J, Weisbjerg MR, Hvelplund T. Concentrate composition for automatic milking systems—Effect on milking frequency. Livestock Sci. 2010;127:45–50.
- Marston SP, Clark GW, Anderson GW, Kersbergen RJ, Lunak M, Marcinkowski DP, Murphy MR, Schwab CG, Erickson PS. Maximizing profit on New England organic dairy farms: An economic comparison of 4 total mixed rations for organic Holsteins and Jerseys. J. Dairy Sci. 2011;94(6):3184–3201. [PubMed: 21605788]
- McCaslin M, Reisen P, Ho J. Proceedings of the 44th California Alfalfa, Forage, and grain Symposium. December 10–12, 2014. Long Beach, CA. Davis: University of California; 2014. New strategies for forage quality improvement in alfalfa; pp. 10–12.
- Menajovsky SB, Walpole CE, DeVries TJ, Schwartzkopf-Genswein KS, Walpole ME, Penner GB. The effect of the forage-to-concentrate ratio of the partial mixed ration and the quantity of concentrate in an automatic milking system for lactating Holstein cows. J. Dairy Sci. 2018;101:9941–9953. [PubMed: 30219424]
- Migliorati L, Speroni M, Susanna L, Calza F. Effect of concentrate feeding on milking frequency and milk yield in an automatic milking system. Ital. J. Anim. Sci. 2005;4(Suppl. 2):221–223.
- Migliorati L, Speroni M, Stelletta C, Pirlo G. Influence of feeding flavouring-appetizing substances on activity of cows in an automatic milking system. Ital. J. Anim. Sci. 2009;8:417–419.
- Miller-Cushon EK, DeVries TJ. Feed sorting in dairy cattle: Causes, consequences, and management. J. Dairy Sci. 2017;100:4172–4183. [PubMed: 28041726]
- Miron J, Nikbachat M, Zenou A, Ben-Ghedalia D, Solomon R, Shoshani E, Halachmi I, Livshin N, Antler A, Maltz E. Lactation performance and feeding behavior of dairy cows supplemented via automatic feeders with soy hulls or barley based pellets. J. Dairy Sci. 2004;87:3808–3815. [PubMed: 15483165]
- Mogensen L, Kristensen T. Concentrate mixture, grass pellets, fodder beets, or barley as supplements to silage ad libitum for high-yielding dairy cows on organic farms. Acta Agric. Scand. A Anim. Sci. 2003;53:186–196.
- Moore SM, King MTM, Carpenter AJ, DeVries TJ. Behavior, health, and productivity of early-lactation dairy cows supplemented with molasses in automated milking systems. J. Dairy Sci. 2020;103:10506–10518. [PubMed: 32921474]
- NASEM (National Academies of Sciences, Engineering, and Medicine). genetically Engineered Crops: Experiences and Prospects. Washington, DC: The National Academies Press; 2016. [PubMed: 28230933]
- Nemeth A, Wurz A, Artim L, Charlton S, Dana G, Glenn K, Hunst P, Jennings J, Shilito R, Song P. Sensitive PCR analysis of animal tissue samples for fragments of endogenous and transgenic plant DNA. J. Agric. Food Chem. 2004;52:6129–6135. [PubMed: 15453677]
- NRC (National Research Council). Nutrient Requirements of Dairy Cattle. 7th rev. Washington, DC: National Academy Press; 2001.
- Onetti SG, Reynal SM, Grummer RR. Effect of alfalfa forage preservation method and particle length on performance of dairy cows fed corn silage-based diets and tallow. J. Dairy Sci. 2004;87:652–664. [PubMed: 15202650]
- Paddick KS, DeVries TJ, Schwartzkopf-Genswein K, Steele MA, Walpole ME, Penner GB. Effect of the amount of concentrate offered in an automated milking system on dry matter intake, milk yield, milk composition, ruminal digestion, and behavior of primiparous Holstein cows fed isocaloric diets. J. Dairy Sci. 2019;102:2173–2187. [PubMed: 30712933]
- Palmonari A, Fustini M, Canestrari G, Grilli E, Formigoni A. Influence of maturity on alfalfa hay nutritional fractions and indigestible fiber content. J. Dairy Sci. 2014;97:7729–7734. [PubMed: 25262189]
- Phillips CJC, Rind MI. The effects on production and behavior of mixing uniparous and multiparous cows. J. Dairy Sci. 2001;84:2424–2429. [PubMed: 11768083]
- Phipps RH, Deaville ER, Maddison BC. Detection of transgenic and endogenous plant DNA in rumen fluid, duodenal digesta, milk, blood, and feces of lactating dairy cows. J. Dairy Sci. 2003;86:4070–4078. [PubMed: 14740846]
- Rebelo L, Lee C, Weiss W, Eastridge M. Effects of Enogen feed corn silage and corn grain on nutrient digestibility, production, and enteric methane emission in lactating cows. J. Dairy Sci. 2020;103(Suppl. 1):171. [PubMed: 37225579]
- Reis RB, Combs DK. Effects of increasing levels of grain supplementation on rumen environment and lactation performance of dairy cows grazing grass-legume pasture. J. Dairy Sci. 2001;83(12):2888–2898. [PubMed: 11132861]
- Rey-Crespo F, Miranda M, López-Alonso M. Essential trace and toxic element concentrations in organic and conventional milk in NW Spain. Food Chem. Toxicol. 2013;55(Suppl. C):513–518. [PubMed: 23391598]
- Rizzi A, Raddadi N, Sorlini C, Nordgrd L, Nielsen KM, Daffonchio D. The stability and degradation of dietary DNA in the gastrointestinal tract of mammals: Implications for horizontal gene transfer and the biosafety of GMOs. Crit. Rev. Food Sci. Nutr. 2012;52:142–161. [PubMed: 22059960]
- Roche JR, Kolver ES, Berry DP. Holstein-Friesian strain and feed effects on milk production, body weight, and body condition score profiles in grazing dairy cows. J. Dairy Sci. 2006;89:3532–3543. [PubMed: 16899689]
- Roche JR, Kay JK, Rius AG, Grala TM, Sheahan AJ, White HM, Phyn CVC. Short communication: Immediate and deferred milk production responses to concentrate supplements in cows grazing fresh pasture. J. Dairy Sci. 2013;96(4):2544–2550. [PubMed: 23375970]
- Rodenburg J, Lyons NA, Kerrisk KL. Dairy systems with automatic milking (robots). In: Beede DK, editor. Large Dairy herd Management. 3rd ed. Champaign, IL: American Dairy Science Association; 2017. pp. 127–142.
- Rook AJ, Huckle CA, Penning PD. Effects of sward height and concentrate supplementation on the ingestive behaviour of spring-calving dairy cows grazing grass-clover swards. Appl. Anim. Behav. Sci. 1994;40(2):101–112.
- Sairanen A, Khalili H, Nousiainen JI, Ahvenjarvi S, Huhtanen P. The effect of concentrate supplementation on nutrient flow to the omasum in dairy cows receiving freshly cut grass. J. Dairy Sci. 2005;88:1443–1453. [PubMed: 15778313]
- Schingoethe DJ. A 100-year review: Total mixed ration feeding of dairy cows. J. Dairy Sci. 2017;100(12):10143–10150. [PubMed: 29153159]
- Schwendel BH, Wester TJ, Morel PCH, Tavendale MH, Dead-man C, Shadbolt NM, Otter DE. Invited review: Organic and conventionally produced milk—An evaluation of factors influencing milk composition. J. Dairy Sci. 2015;98:721–746. [PubMed: 25497795]
- Sehested J, Kristensen T, Soegaard K. Effect of concentrate supplementation level on production, health and efficiency in an organic dairy herd. Livestock Prod. Sci. 2003;80:153–165.
- Silva ÁC d, Pd Figueiredo M, Bonomo P, Pereira MLA, Luz YdS, Santos EdJ. Microbial protein synthesis and nitrogen metabolism in cows bred on tropical pasture and fed on cassava root and corn. Acta Sci. Anim. Sci. 2014;36:185–192.
- Sorge US, Moon R, Wolff LJ, Michels L, Schroth S, Kelton DF, Heins B. Management practices on organic and conventional dairy herds in Minnesota. J. Dairy Sci. 2016;99(4):3183–3192. [PubMed: 26830734]
- Stallings CC, McGilliard ML. Lead factors for total mixed ration formulation. J. Dairy Sci. 1984;67(4):902–907. [PubMed: 6547154]
- Staudenmeyer DM, Bowman JGP, Endecott RL, Mack AL, Glunk EC. The effects of feeding reduced-lignin alfalfa on growing beef cattle performance. Transl. Anim. Sci. 2017;1:62–65.
- Stockdale CR. Effects of level of feeding of concentrates during early lactation on the yield and composition of milk from grazing dairy cows with varying body condition score at calving. Aust. J. Exp. Agric. 2004;44:1–9.
- St-Pierre NR, Thraen CS. Animal grouping strategies, sources of variation, and economic factors affecting nutrient balance on dairy farms. J. Anim. Sci. 1999;77(Suppl. 2):72–83. [PubMed: 15526782]
- Sulc RM, Parker A, Albrecht K, Cassida K, Hall M, Min D, Orloff S, Xu X, Undersander D. Proceedings of the 26th Tri-State Dairy Nutrition Conference. April 17–19, 2017. Fort Wayne, IN. Columbus: Ohio State University; 2017. Agronomic and nutritional attributes of reduced lignin alfalfa; pp. 79–86.
- Suttle NF, Abrahams P, Thornton I. The role of a soil × dietary sulphur interaction in the impairment of copper absorption by ingested soil in sheep. J. Agric. Sci. 1984;103(1):81–86.
- Szabala BM, Osipowski P, Malepszy S. Transgenic crops: The present state and new ways of genetic modification. J. Appl. genet. 2014;55:287–294. [PubMed: 24736992]
- Tosta MR, Prates LL, Christensen DA, Yu P. Effects of processing methods (rolling vs. pelleting vs. steam-flaking) of cool-season adapted oats on dairy cattle production performance and metabolic characteristics compared with barley. J. Dairy Sci. 2019;102(12):10916–10924. [PubMed: 31563319]
- Tremblay M, Hess JP, Christenson BM, McIntyre KK, Smink B, van der Kamp AJ, de Jong LG, Döpfer D. Factors associated with increased milk production for automatic milking systems. J. Dairy Sci. 2016;99:3824–3837. [PubMed: 26898275]
- Van Eenennaam AL, Young AE. Prevalence and impacts of genetically engineered feedstuffs on livestock populations. J. Anim. Sci. 2014;92:4255–4278. [PubMed: 25184846]
- Van Eenennaam AL, Young AE. Detection of dietary DNA, protein, and glyphosate in meat, milk, and eggs. J. Anim. Sci. 2017;95:3247. [PubMed: 28727079]
- Welchez SFC, Stefenoni H, Melgar A, Lage CFA, Räisänen SE, Wasson D, Fetter ME, Hristov AN. Effect of high-amylase corn silage on lactational performance and enteric methane emission in dairy cows. J. Dairy Sci. 2020;103(Suppl. 1):68.
- Williams CB, Oltenacu PA. Evaluation of criteria used to group cows using a dairy production model. J. Dairy Sci. 1992;75:155–160.
- Dairy Production Systems - Nutrient Requirements of Dairy CattleDairy Production Systems - Nutrient Requirements of Dairy Cattle
Your browsing activity is empty.
Activity recording is turned off.
See more...