Except where otherwise noted, this work is licensed under a Creative Commons Attribution-NonCommercial-NoDerivatives 4.0 International License (CC-BY-NC-ND 4.0). To view a copy of this license, visit http://creativecommons.org/licenses/by-nc-nd/4.0/)
NCBI Bookshelf. A service of the National Library of Medicine, National Institutes of Health.
Ferretti JJ, Stevens DL, Fischetti VA, editors. Streptococcus pyogenes: Basic Biology to Clinical Manifestations [Internet]. 2nd edition. Oklahoma City (OK): University of Oklahoma Health Sciences Center; 2022 Oct 8.

Streptococcus pyogenes: Basic Biology to Clinical Manifestations [Internet]. 2nd edition.
Show detailsIntroduction
Streptococcal diseases have been known for centuries, although their delineation into separate disease entities did not begin to occur until the 16th century AD. The original writings of Hippocrates from the 4th century BC describe the disease erysipelas (ἐρυσίπελας, red skin), as well as the symptoms of childbed fever, and “Galen remarks that not only erysipelas, but also inflammation, when it attacks the impregnated uterus, generally proves fatal” (Adams, 1849). Centuries later, after epidemic rates of mortality, this particular disease was recognized in 1716 as puerperal fever or childbed fever (Strother, 1716). Many theories about the origin of diseases appeared in the intervening centuries, including associating their occurrence with natural phenomena like comets and eclipses; the spread of disease by vapors and smells; “contagion,” or the transferring of diseases from one to another; and the introduction of non-living matter into a patient (Karamanou, Panayiotakopoulos, Tsoucalas, Kousoulis, & Androutsos, 2012). It was not until the 18th century that further progress into the etiology of diseases was made. A major advancement was the invention of the microscope by Anton van Leeuwenhoek (1632–1723) as well as his descriptions of new life forms, including the shapes of cocci, bacilli, and spirochetes (Figure 1) (Dobell, 1932). However, as with a number of early scientific and medical discoveries or observations, the link between the microscopic discovery of these new life forms and diseases was not realized until almost two centuries later.

Figure 1.
Leeuwenhoek’s drawings of bacteria from the human mouth.
Scarlet Fever
Reviews of the writings of ancient scholars found many passages relating to sore throats or ulcerous tonsils, but lacked comment about an associated rash, which would be typical of what would later be known as scarlet fever. According to Rolleston (Rolleston, 1928) in his article on the history of scarlet fever, Giovanni Filippo Ingrassias, a Sicilian anatomist and practitioner, wrote in 1553 (Ingrassia, 1553) the first description of a disease termed “rossalia” that was characterized by “numerous spots, large and small, fiery and red, of universal distribution, so that the whole body appeared to be on fire.” He also reported that this “rossalia” or rash was different than that observed in measles. (Rolleston, 1928). According to most scholars, Johann Weyer of the Netherlands was the first to describe a sore throat occurring during epidemics of scarlatina anginosa, which he did in 1565. In 1578, Jean Cottyar of Poitiers gave the first definitive description of scarlet fever in France as a “general weariness, headache, redness of the eyes, sore throat, and fever. Purpura appeared on the second or third day, accompanied by delirium and soreness of throat”. Daniel Sennert (Sennert, 1628) described an epidemic in Wittenberg in the beginning of the seventeenth century and was the first to describe scarlatinal desquamation, arthritis, and post-scarlatinal dropsy and ascites. The term “scarlatina” was first introduced into the medical literature in 1675 by Sydenham (Sydenham, 1676), who identified it as a separate disease entity from other exanthemas, especially measles (Rolleston, 1928).
Epidemics of scarlet fever were reported throughout Europe and North America during the 17th and 18th centuries, some of which were associated with high mortalities. It was not until the 1920s that George and Gladys Dick showed that scarlet fever was associated with a sore throat caused by hemolytic streptococci that produced a secreted toxin known as scarlet fever toxin, or Dick toxin (Dick & Dick, 1924a; Dick & Dick, 1924b; Dick & Dick, 1924c). Scarlet fever remained an important infectious disease until the advent of antibiotic therapy in the 1940s, and prior to that time, a brightly colored quarantine sign was placed on the door of the house of diseased individuals to isolate the patient and prevent the further spread of this feared disease (Figure 2).

Figure 2.
Scarlet Fever Quarantine sign.
Puerperal Fever
Although streptococcal diseases were not recognized as a cause of puerperal fever at the time, epidemics associated with high mortality rates during childbirth were reported across Europe and North America in the 18th and 19th centuries. In 1843, the American surgeon Oliver Wendel Holmes, Sr. (Figure 3), published a paper on “The Contagiousness of Puerperal fever” (Holmes, 1843). Holmes wrote, “This disease seized such women only as they were visited or delivered by a practitioner, or taken care of by a nurse, who had previously attended patients affected with the disease” (Holmes, 1843). His observations were the subject of more debate than application, and many of his contemporaries responded to his report with more ridicule than acceptance.

Figure 3.
Oliver Wendell Holmes Sr., 1809–1894.
In 1842, Ignac Semmelweis, a young Hungarian physician (Figure 4) working in the obstetric wards of the Vienna Lying-in Hospital (Semmelweis, 1983; Semmelweis Society, 2009), independently monitored hospital mortality rates and observed that the mortality rates of women giving birth attended by physicians in Clinic 1 were almost ten times higher than when midwives attended the deliveries in Clinic 2. He observed that physicians and medical students who arrived at the hospital in the morning first visited the autopsy rooms before seeing patients in other wards. Because physicians and medical students did not wash their hands before going to the obstetric ward, Semmelweis surmised they were physically carrying the disease entity from cadaverous material directly to patients. In 1847, he ordered all medical attendants to scrub their hands in a chlorinated lime solution before entering the obstetric ward. After seven months, the mortality from puerperal fever in Clinic 1 decreased dramatically, from 11.4% to 2.7%. Additionally, he noted that soiled bed sheets containing blood or other bodily materials were not changed between patients. In 1848, chlorine solution washings were carried out during the year, and Semmelweis also ordered the changing of sheets between patients. His recordings showed that the mortality rate that year in Clinic 1 was 1.27% and in Clinic 2 was 1.3%. Though the results were profound, Semmelweis had no scientific evidence to explain the cause of the disease—except cleanliness. Nevertheless, his immediate superiors resented Semmelweis’s Lehre (teaching) methods and treated his findings with skepticism and ridicule. In 1849, Semmelweis returned to Budapest, because his contract was not renewed at the Vienna Hospital. In 1860, Semmelweis published his detailed observations in a treatise, The Etiology, Concept and Prophylaxis of Childbed Fever (Semmelweis, 1983). He was an early revolutionary in the medical sciences in the use of statistical methods to prove his hypothesis that childbed fever was caused by medical personnel failing to wash their hands and who then transmitted the disease to patients.

Figure 4.
Ignaz Semmelweis, 1818–1865.
A full recognition of Semmelweis’s brilliant work did not come until 14 years after his death in 1865. In a discussion on puerperal fever at the French Academy of Sciences in Paris in 1879, a physician named Hervieux elaborated on the causes of epidemics in lying-in hospitals, ascribing them to an undefined “puerperal miasma. Louis Pasteur interrupted him: ‘None of those things cause the epidemic; it is the nursing and medical staff who carry the microbe from an infected woman to a healthy one.’ And when the speaker replied that he feared the microbe would never be found, Pasteur went to the blackboard and drew a diagram of the dangerous chain-forming microbe, saying, ‘There: This is what it looks like’” (Pasteur, 1879; Schwartz, 1997). A complete and detailed history of puerperal fever can be found in two books by Irvine Louden: Childbed Fever, A Documentary History; 1995 (Loudon, 1995) and The Tragedy of Childbed Fever, 2000 (Loudon, 2000).
Discovery of Streptococci
The first description of streptococcal infection is attributed to the Austrian surgeon, Theodor Billroth (Figure 5), in 1874, when he described the organism in cases of erysipelas and wound infections (Billroth, 1874; Billroth, 1877). He described these “small organisms (Kettenkokken) as found in either isolated or arranged in pairs, sometimes in chains of four to twenty or more links (Streptococcus; Gr. strepto, a chain, and coccus, a berry).” The real importance and formal entry of streptococci to history came in 1879 when Louis Pasteur (Figure 6) isolated the microorganism from the uteruses and blood of women with puerperal fever (Alouf & Horaud, 1997). He further demonstrated that the streptococcus was the etiological agent responsible for the disease that caused the highest mortality rates of women and newborns at that time. Additional refinement of the name streptococcus came from Friedrich Julius Rosenbach in 1884, who examined bacteria isolated from suppurative lesions, and the species was named Streptococcus pyogenes (Gr., pyo, pus, and genes, forming) (Evans, 1936). Previously, Fehleisen isolated streptococci from a patient with erysipelas and Rosenbach named the organism S. erysepaltis (Evans, 1936; Rosenbach, 1884). However, later reviews showed that there was no particular characteristic associated with organisms isolated from specific diseases, and Andrewes and Christie suggested that the proposed species names of pyogenes, eryespaltis, scarlatinae, and puerperalis all be included in the single name Streptococcus pyogenes (Evans, 1936; Andrewes & Christie, 1932).

Figure 5.
Theodor Billroth, 1829–1894.

Figure 6.
Louis Pasteur, 1822–1895.
Differentiation and Classification of Streptococci
The introduction of blood agar plates by Hugo Schottmuller in 1903 was an important step forward for the differentiation of streptococci (Schottmuller, 1903; Becker, 1916). When mixtures of streptococci were streaked on blood agar plates, two types of hemolysis were observed: a clear zone surrounding a colony containing organisms in long chains, termed Streptococcus haemolyticus; and a green zone surrounding a colony with short chains, termed Streptococcus viridans. Further elaboration by Brown in 1919 (Brown, 1919) resulted in three classes of hemolytic patterns surrounding a colony on a blood agar plate: 1) “Alpha”—a green zone of discoloration, which is characteristic of the viridans streptococci; 2) “Beta”—a clear zone of complete hemolysis, which is found in the S. haemolyticus type organisms; and 3) “Gamma”—no change in the medium, which is characteristic of the enterococcus and S. faecalis organisms. At the same time, Dochez, Avery, and Lancefield began using immunological approaches to demonstrate that differences could be demonstrated between strains of S. haemolyticus (Dochez, Avery, & Lancefield, 1919). In 1933, Lancefield used surface antigen differences among various streptococci to further subdivide them into groups designated by the letters A through X (Köhler, 2008). The strains from human diseases were classified as Group A; the strains from bovine and dairy sources as Group B; the strains from a variety of animal sources as Group C; and so forth. For epidemiological studies, the determination of the T-antigens by slide agglutination was introduced by Fred Griffith of London in 1934 (Griffith, 1934) and was widely used, even in International Type Distributions Surveys of S. pyogenes at the end of the 1960s (Kohler, 1974). The Group A strains, Streptococcus pyogenes, were further subdivided according to the presence of a surface protein named M protein (due to its matte appearance in colony formations) into different antigenic types. Lancefield was able to demonstrate over 50 different M-types of group A streptococci during her career (Lancefield, 1962; National Academy of Sciences, 1987), and since then, more than 200 M-types have been identified using a combination of serological and molecular typing methods. Lancefield also identified the M protein as the major virulence factor of Streptococcus pyogenes due to its antiphagocytic property (Lancefield, 1962). Maclyn McCarty considered Lancefield’s monumental work as “the opening chapter in the modern investigation of hemolytic streptococci” (Figure 7) (National Academy of Sciences, 1987). The importance of this classification system is underscored by its continued development and use in clinical, bacteriologic, and epidemiologic studies. Facklam has provided a summary of the nomenclature and taxonomy changes of the Streptococcus genus that have occurred, up to the year 2002 (Facklam, 2002). More recent descriptions of the “present state of species within the genus Streptococcus and Enterococcus” by Köhler (Köhler, 2007), and a “phylogenomic analysis of genome evolution in the genus Streptococcus” by Richards et al. (Richards, et al., 2014) provide important new overviews of the genus Streptococcus.

Figure 7.
Walter Bauer, Rebecca Lancefield, and Maclyn McCarty at the Rockefeller Institute.
Biology of the Group A Streptococci
Early research in the first part of the 20th century on the biology of the hemolytic streptococci was perhaps more intensive than the research conducted on any other species of pathogenic bacteria. These studies focused on the basic biology and physiology of the organism, with an emphasis on the relationship of the organism to the important diseases of the day, scarlet fever and rheumatic fever. As mentioned earlier, one of the most important advances was the study by Lancefield that classified the organisms into various serological groups and identified the group A streptococcus, Streptococcus pyogenes, as the organism responsible for most of the hemolytic streptococcal infections in humans (Lancefield, 1941). An overview of many of the early studies on the biology of the group A streptococci is presented in the following sections; however, more detailed and comprehensive reviews can be found in McCarty (McCarty, 1952); Wannamaker and Matsen (Wannamaker & Matsen, 1972); Stollerman (Stollerman, 1975); and Köhler (Köhler, 2008).
An essay about collaborative research by Ferretti (Ferretti, 2008) describes “events during the early 1960s in which streptococcal research flourished and was propelled by interactions at many levels, particularly international conferences and symposia. The World Health Organization sponsored an international symposium on streptococci and streptococcal diseases in 1960 in Prague, Czechoslovakia. Two of the twenty-six participants in attendance were Werner Köhler from the German Democratic Republic and Dennis Watson from the United States, both technically non-members of the WHO. Following this conference, Köhler suggested continuation of these meetings, and three years later in 1963, the second conference was held in Jena, GDR, with a three-fold increase in attendance. The third conference was held in 1966 in Paris, and a fourth was again held in Jena in 1969. Thus, the first meeting in Jena was a starting point for what is now known as the Lancefield International Symposium on Streptococci and Streptococcal Diseases.” This symposium has been held every three years in various cities in the world (as recorded in Table 1) and continues to be an important forum for discussion, learning, and collaboration about current and new topics in streptococcal research.
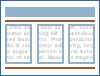
Table 1.
Lancefield International Symposium on Streptococci and Streptococcal Diseases
Cultural Conditions and Growth
The group A streptococci are fastidious organisms that have complex growth requirements. A highly nutritious growth medium that provides optimal growth was described in 1932 by Todd and Hewitt that consisted of a broth of meat extract, peptones, dextrose, and salts (Todd & Hewitt, 1932). This medium was subsequently prepared in a dehydrated form according to a formula by Updyke and Nickle in 1954, and is still used in present times for the growth of group A streptococci and other fastidious organisms (Updyke & Nickle, 1954). In order to further study the nature of extracellular toxins, such as streptolysin O, Bernheimer and Pappenheimer developed a defined protein-free medium that produced a large amount of growth (Bernheimer & Pappenheimer, Jr., 1942). Later studies showed that the alteration of environmental conditions could increase the amounts of various extracellular products. For example, the addition of a digested RNA fraction increased streptolysin S production (Bernheimer & Rodbart, 1948); while the addition of reducing agents (such as glutathione) increased streptolysin O production (Slade & Knox, 1950); maintaining a slightly acidic pH enhanced the production of the cysteine proteinase precursor (Elliott, 1950); and the addition of hyaluronate to the growth medium increased the production of hyaluronidase (Rogers, 1945). Various formulations of a chemically defined medium were described thereafter; however, most of these have suffered from the inability to provide adequate growth yields and/or the production of different virulence factors. A new chemically defined medium, described by van de Rijn and Kessler in 1980, overcame many of the previous deficiencies and provided high culture densities as well as adequate amounts of virulence factors, such as M protein and extracellular products (van de Rijn & Kessler, 1980). A more detailed description of the metabolism of the group A streptococci can be found in Chapter 7 of this volume.
Ultrastructure and Cellular Components
In initial studies of the biology of hemolytic streptococci, immunological differences were found to exist among strains isolated from human disease. These organisms were identified as being strongly Gram-positive and growing in chains of varying length (Dochez, Avery, & Lancefield, 1919). Colonies formed on agar plates were identified as small, smooth, and moist in appearance, but capsule formation was not identified at that time. Almost 20 years later, a polysaccharide composed of N-acetylglucosamine and glucuronic acid was isolated from encapsulated group A streptococci and was found to be identical to hyaluronic acid found in mammalian tissue (Kendall, Heidelberger, & Dawson, 1937). This capsular material did not stimulate antibody production, an observation consistent with the fact that this substance occurs naturally in mammalian tissues. A comprehensive review of the capsular polysaccharide of group A streptococci and its biochemistry, genetics, and role in virulence has been reported by Wessels (Wessels, 2006), and in Chapter 4 of this volume.
The studies of Lancefield and colleagues have provided a great deal of information about the antigenic structure of the streptococcal cell, particularly about the M protein and its importance as the major virulence factor of the group A streptococci (Lancefield, 1962). In addition to the 1962 review by Lancefield (Loudon, 2000), detailed reviews on the streptococcal M protein have been presented by Fox in 1974 (Fox, 1974), Fischetti in 1989 (Fischetti V. A., 1989), and Fischetti et al. in Chapter 2 of this volume.
Besides the M protein, many more proteins have been found on the cell surface by their ability to bind mammalian serum or secretory proteins, such as immunoglobulins, albumin, fibrinogen, and fibronectin, or by enzymatic digestion. These include T-protein, serum opacity factor, C5a peptidase, collagen-like protein Scl1, GRAB, and protein F, among others. Fischetti and colleagues showed that the C-terminal region of these anchored proteins contains a highly conserved heptapeptide consensus sequence of LPXTG, which is an anchor signal found in most surface proteins from Gram-positive bacteria for covalent attachment to the peptidoglycans (Fischetti, Pancholi, & Schneewind, 1990; Mazmanian, Ton-That, & Schneewind, 2001). Finally, Mora and colleagues reported that group A streptococci possess pilus-like structures that contain protective antigens and Lancefield T antigens (Mora, et al., 2005). A recent publication that used microarray analysis identified more than 80 different proteins on the surface of an M1 strain of group A streptococci (Galeotti, et al., 2012).
A major component of the streptococcal cell wall, making up approximately 50 percent of its weight, was found to be carbohydrate in nature and was identified as the group-specific carbohydrate (Lancefield, 1941). Eighteen groups of specific antigens were established by Lancefield, and the group A carbohydrate was subsequently shown to be composed of a polymer of rhamnose and N-acetylglucosamine (Krause & McCarty, 1961). The next several chapters of this volume present a more detailed and comprehensive view of the ultrastructure of the group A streptococci.
Streptococcal Bacteriophages
Bacteriophages were first independently identified by Twort in 1915 and were later confirmed by d’Herelle in 1917. Frederick Twort, an English bacteriologist, discovered agents that he termed “filter-passing viruses” which required bacteria for growth (Twort, 1915). Twort’s studies were interrupted by World War I, and as a result, he did not return to research until several years later. Felix d’Herelle, a French-Canadian microbiologist, first applied the name “bacteriophage” (from bacteria and the Greek word phagein, "to devour") to a phage that was able to kill a number of pathogenic bacteria, including streptococci (d'Herelle, 1915). Subsequent work relating to these two investigators resulted in the “Twort-d’Herelle Controversy,” a topic covered in detail in other historical reviews (Ackermann, Martin, Vieu, & Nicolle, 1982; Duckworth, 1976; Fruciano & Bourne, 2007; Summers, 1999).
In 1924, a report by Dick and Dick (Dick & Dick, 1924a) about the presence of a rash-producing substance in the culture filtrates of certain hemolytic streptococci stimulated further research on the substance, and was variously termed as scarlet fever toxin, Dick toxin (Dick & Dick, 1924b), scarlatinal toxin (Trask, 1926), or erythrogenic toxin (Stock, 1939). Shortly after, the role of a filterable factor (bacteriophage) that was able to change a non-toxigenic streptococcal strain to a toxigenic strain was reported by Cantacuzène and Bonciu (Cantacuzène, 1926) in 1926, and Frobisher and Brown in 1927 (Brown, 1927), and was later confirmed by Bingel in 1947 (Bingel, 1949).
In the intervening years, various investigators found both virulent and temperate phages in streptococci. Clark and Clark (Clark & Clark, 1927) and Evans (Evans, 1934a; Evans, 1934b; Evans, 1934c), identified virulent phages isolated from sewage, and Kjems (Kjems, 1955) later described methods to isolate temperate phages from S. pyogenes. Kjems further observed that the virulent phage obtained from sewage were unable to penetrate the hyaluronic capsule of S. pyogenes, whereas the temperate phage possessed its own hyaluronidase (Maxted, 1955) to penetrate the capsule (Kjems, 1958). In a following study, Kjems found that lysogeny was a common occurrence in group A streptococci and could be found in up to 83% of strains tested (Kjems, 1960).
Transduction by streptococcal bacteriophages was first demonstrated by Leonard et al. of streptomycin resistance by several group A streptococcal phages (Leonard, Colón, & Cole, 1968). Malke demonstrated the phage A25-mediated transfer induction of a prophage in S. pyogenes, as well as the transfer of antibiotic resistance markers in strains of 30 different representative group A streptococcal M types (Malke, 1972; Malke, 1973; Malke & Köhler, 1973). Tagg was able to transduce bacteriocin production and host cell immunity into three different group A strains (Tagg, Skjold, & Wannamaker, 1976). The advent of molecular cloning and successful artificial transformation procedures in group A streptococci transcended the further use of transduction as a tool in the genetic analysis of these organisms, but nevertheless, the importance of this form of horizontal gene transfer to streptococcal evolution remains an important consideration (McLaughlin & Ferretti, 1995).
In 1964, Zabriskie reported on the conversion of a non-toxigenic S. pyogenes strain T253 to toxin production by phage T12 isolated from toxigenic strains (Zabriskie, 1964). The new toxigenic strains were T253(T12) lysogens that produced erythrogenic toxin; or, as later termed, streptococcal pyrogenic exotoxin A(Watson, 1960). These observations were later confirmed by Nida and Ferretti (Nida, Houston, & Ferretti, 1979; Nida & Ferretti, 1982), Johnson et al. (Johnson & Schlievert, 1984; Johnson, Schlievert, & Watson, 1980), and McKane and Ferretti (McKane & Ferretti, 1981) employing the same T253 non-lysogen and T12 phage system that Zabriskie reported. The demonstration that T12 phage contained the structural gene for the streptococcal pyrogenic exotoxin A (speA) was confirmed independently by Weeks and Ferretti (Weeks & Ferretti, 1984) and Johnson and Schlievert (Johnson & Schlievert, 1984). In subsequent experiments, the speA containing T12 bacteriophage was shown to integrate into a gene that encodes a serine tRNA in the host chromosome (McShan, Tang, & Ferretti, 1997).
Most studies of S. pyogenes phages have focused on lysogenic phages, but recent genomic studies on lytic phage A25 have shown a high degree of similarity to lysogenic phages in the S. pyogenes chromosome. Apparently the A25 phage became a lytic phage when it escaped from lysogeny with the loss of an integrase module (McCullor, Postoak, Rahman, King, & McShan, 2018). Another study of the lytic phage A1 demonstrated the survival strategies of S. pyogenes to resist phage infection by producing membrane vesicles that bind phage particles and hence reduce their availability for further infection (Beerens, et al., 2021). Further information about bacteriophages can be found in Chapter 9 of this volume.
Extracellular Products
The group A streptococci produce a large number of proteins that are secreted into the extracellular fluid during growth. Many of these proteins were originally detected by their biological activities or by immunological detection with human antiserum. Historically, the first extracellular products studied were those thought to be directly related to diseases; namely, erythrogenic toxin, streptolysin O, streptolysin S, proteinase, streptokinase, DNase, RNase, and hyaluronidase (McCarty, 1952). As research in the area of genetics and genomics has increased in recent years, additional secreted extracellular products have been discovered, such as CAMP factor, streptococcal inhibitor of complement, immunogenic secreted protein, and a number of superantigens. Additionally, these secreted proteins may be encoded by either chromosomal or bacteriophage-associated genes. Many of these secreted proteins are known virulence factors and play important roles in colonization, invasion, spreading, and pathogenesis. Comprehensive reviews on the topic of group A streptococcus extracellular products have been published by Alouf (Proft & Fraser, 2006; Alouf, 1980), Ginsburg (Ginsburg, 1972), Hynes (Hynes, 2004), McCormick et al (McCormick, Peterson, & Schlievert, 2006), and in Chapter 13 of this volume.
Streptococcal Diseases
Streptococcus pyogenes is a strict human pathogen responsible for a wider variety of human diseases than perhaps any other microorganism. The classical diseases of the group A streptococci are now known by newer names. For example, streptococcal pharyngitis is a “strep sore throat,” and if accompanied by a rash, it is known as scarlet fever. Skin infections categorized as pyoderma or impetigo may include erysipelas and cellulitis, which infect deeper layers of the skin. If these infections spread to the fascia, the disease is known as necrotizing fasciitis, which is sometimes referred to as a “flesh-eating disease” by the popular press. This is a severely invasive disease that, unless given immediate surgical (mostly debridement) and medical intervention, results in a high mortality rate. Another severe invasive disease is streptococcal toxic shock syndrome (STSS), a systemic disease entity recognized in the early 1990s (The Working Group on Severe Streptococcal Infections, 1993; Stevens, 1995) and is described further in the chapter on toxic shock in Chapter 23 of this volume.
Additional diseases that occur as a result of a previous streptococcal infection are referred to as sequelae, including rheumatic fever (RF) and acute post-streptococcal glomerulonephritis (APSGN). Although the symptoms of these diseases were recognized in earlier times, the pathogenesis and association with group A streptococci were not clearly defined until the early 1900s. Excellent historical accounts of rheumatic fever are presented by Benedek (Benedek, 2006) and Stollerman (Stollerman, 1975), as well as in a subsequent chapter. Historical descriptions of APSGN are found in a review by Rodríguez-Iturbe and Batsford (Rodríguez-Iturbe & Batsford, 2007) in Chapter 27 of this volume.
An illness described in 1998 that may follow a streptococcal infection in children is Pediatric Autoimmune Neuropsychiatric Disorders Associated with Streptococcal infections (PANDAS) (Swedo, et al., 1998). This disorder may arise from multiple aetiologies, and its neuropsychiatric symptoms, such as tic disorders and obsessive-compulsive disorder (OCD), perhaps do not fit any known criteria of association with a streptococcal infection. However, PANDAS symptoms do seem to have similarities to neuropsychiatric symptoms that are associated with Sydenham’s chorea, which is a disease associated with a prior group A streptococcal infection. The interest in this topic is both important to many and controversial to others, but it is nevertheless worthy of presentation in this volume as presented in Chapter 26.
Treatment of Streptococcal Diseases
Treatments of diseases resembling streptococcal infections were described from the earliest writings in the 5th century BC, e.g., “when erysipelas supervenes, emetics, or purgatives are to be administered. When parts around the sore are swollen, a cataplasm (poultice) is to be applied” (Adams, 1849). Treatments over the centuries have also included bloodletting, as well as drugs derived from herbs with emetic, purgative, diaphoretic, or narcotic properties. Additional cures and remedies prescribed during the Middle Ages for all kinds of diseases can be found in the book “Fasciculus Medicinae,” a collection of medical treatises compiled in 1491 by the German physician Johannes de Ketham (Ketham, 1988).
It was not until the 1930s that streptococcal infections were treated with sulphur antibacterials—they were among the first infectious disease organisms to be treated in this way. Domagk found that certain azo dyes coupled with sulphur resulted in an antibacterial agent, named Prontosil, which was inhibitory to a highly virulent strain of Streptococcus hemolyticus (Domagk, 1936; Domagk, 1957). Later modifications of the azo dyes resulted in the sulphonamides, which were marketed as early as 1937 for the treatment of pneumonia and other bacterial infections (Bentley, 2009).
Prior to the discovery of sulphonamides, the Scottish physician Alexander Fleming began searching for antibacterial agents that killed invading bacteria. In 1921, he accidentally found that a substance in his own nasal mucosa was able to dissolve certain bacteria, but not streptococci or staphylococci. This substance was later found to be lysozyme (Bentley, 2009), an enzyme that cleaves the peptidoglycan found in the cell walls of many bacteria. In 1928, Fleming made another accidental discovery that a Petri dish containing Staphylococci also contained a contaminating fungal colony that lysed the bacteria in the surrounding areas. Fleming named the substance causing the bacterial lysis penicillin, after the Penicillium fungus found on the plate (Fleming, 1929). He continued work with attempts to isolate the penicillin from culture filtrates, but made little progress in ensuing years. It was not until 1939 that significant progress was made in the production and isolation of a stable form of penicillin by the Australian physiologist Howard Walter Florey and his German colleague, Ernst Boris Chain (Ligon, 2004). They were able to establish large-scale fermentation conditions to produce penicillin in sufficient quantities to begin effective treatment in the early 1940s. These important discoveries were not only the beginning of the antibiotic era, but also a new industry, based on fermentation, for the production of antibiotics and pharmaceuticals. For these outstanding achievements, Fleming, Chain, and Florey were awarded the 1945 Nobel Prize in physiology or medicine. Streptococcus pyogenes has been and is still extremely sensitive to penicillin-based antibiotics. In fact, despite being the treatment of choice for decades because of its efficacy and low toxicity, streptococci resistant to penicillin have not evolved. The reason for this is still unknown, but may be related to the inability of naked DNA to enter the streptococcal cell because of the presence of DNases in the cytoplasmic membrane, or that beta-lactamase may not be expressed or may be toxic to the organism (Horn, et al., 1998).
Genetics and Genomics
Maclyn McCarty is well known for his many contributions to streptococcal research, and his career of distinguished scientific achievements was documented in an essay by Gotschlich and Fischetti (Gotschlich & Fischetti, 2005). Along with Avery and MacCleod, McCarty is also known as one of the pioneers of molecular genetic research. The 1944 demonstration that DNA is the carrier of genetic information (Tagg, Skjold, & Wannamaker, 1976) was a truly revolutionary finding that transformed and propelled biological research into the next century (Avery, MacLeod, & McCarty, 1944). An interesting personal account of this historical discovery can be found in the book, “The Transforming Principle, Discovering that Genes are Made of DNA” (McCarty, 1986).
A genetic approach to the study of group A streptococci and other factors related to disease had a somewhat slower start, compared to other microorganisms, due to the lack of a convenient gene transfer mechanism, as well as the fastidious growth requirements of the organism. Although a bacteriophage transduction system was available, the lack of convenient selection markers limited the transduction analysis to positive selection markers, such as antibiotic resistance genes (Malke, 1972; Stuart & Ferretti, 1978), or to the analysis of transposon-Tn916–generated mutations (Caparon & Scott, 1991). Additionally, although many attempts were made to effect gene transfer by conjugation or natural transformation, no successful reports were recorded until artificial transformation was achieved by electroporation and other methods (McLaughlin & Ferretti, 1995).
The introduction of genetic engineering technology in the 1970s provided a new approach to analyze virulence factors at the gene level, but the power of this technique was not realized until appropriate streptococcal cloning vectors were made available. The first gene to be cloned in streptococci was an erythromycin resistance determinant (Behnke & Ferretti, 1980), and shortly thereafter, the genes for streptokinase (Malke & Ferretti, 1984), streptococcal pyrogenic exotoxin A(Johnson & Schlievert, 1984; Weeks & Ferretti, 1984), M protein (Scott & Fischetti, 1983), and many others were cloned. The availability of these genes allowed sequence analysis to be completed, as well as overexpression studies for further studies of the individual proteins. Outgrowths of these molecular studies were the area of the “genome,” the genetic material (DNA) of an organism; the “transcriptome,” the set of RNAs in an organism; and the “proteome,” the structure and function of the proteins of an organism. As they relate to the group A streptococci, these areas are presented in several other chapters of this volume.
The genome era began with the first complete genome sequence of Hemophilus influenza in 1995 (Fleischmann, et al., 1995), and was followed by the sequencing of several other microbial genomes. The first complete sequence of a group A streptococcal genome was reported for strain SF370 (M1 serotype) in 2001 (Ferretti, et al., 2001), and since then, numerous complete genomes of other M-type strains have been reported. Their sequences are available in the NIH genebank (National Center for Biotechnology Information, n.d.). Additionally, as the cost of DNA sequencing has decreased over time, hundreds of draft genomes of group A streptococcal strains were produced and analyzed, either by public institutions or by industry. One of the more impressive recent genome studies used the sequences of over 3,600 genomes to delineate the step-wise genetic events that led to the worldwide epidemic of an M1 serotype strain with increased virulence (Nasser, et al., 2014). A subsequent chapter presents an overview of comparative genomics of the group A streptococci.
Another important discovery from genome analyses was the identification of clustered regularly interspaced short palindromic repeats (or CRISPRs), and the associated DNA-cutting enzyme, Cas9 (Koonin & Makarova, 2009; Sorek, Kunin, & Hugenholz, 2008) was originally found in S. pyogenes. The CRISPR-Cas modules also found in many other bacteria are adaptive immunity systems that defend the organisms against invading genetic elements (Makarova, et al., 2011). This system also contains elements useful for directly editing or modulating the function of DNA sequences and provides a trailblazing new technology for genome editing (Deltcheva, et al., 2011; Jinek, Chylinski, Fonfara, Hauer, & Doudna, 2012).
The Nobel Prize in Chemistry 2020 was awarded jointly to Emmanuelle Charpentier and Jennifer A. Doudna "for the development of a method for genome editing." (Nevelius & Gustafsson, 2020)A complete description of the type II-A CRISPR-Cas system from S. pyogenes can be found in the review by LeRhun et al. (Le Rhun, Escalera-Maurer, Bratovič, & Charpentier, 2019) as well as in Chapter 10 of this volume.
Conclusion
Diseases caused by Streptococcus pyogenes have been well documented through the ages, and the overall importance of streptococci in medical history has been appropriately summarized by Schwartz: “not only were streptococci among the first organisms to be presumed to be the cause of contagious diseases, but also their existence forced the introduction of hygiene and asepsis into hospital wards” (Schwartz, 1997). Medical research using streptococci have also played a role in understanding the pathogenesis of infectious diseases, the development of antibiotics, the role of DNA as a carrier of genetic information, and in genetic engineering. Future research will be directed toward employing the tools of systems biology: namely, “investigating the behavior and relationships of all the elements in a particular biological system while it is functioning” (Ideker, Winslow, & Lauffenburger, 2006); understanding the role of epigenetic gene regulation, especially DNA methylation resulting in alterations in gene expression (Casadesús & Low, 2006; Euler, Ryan, Martin, & Fischetti, 2007); and deciphering the signals that influence transcriptional regulatory networks and control of virulence (as in the chapter on this topic). Additional research will focus on understanding the mechanisms of Streptococcus pyogenes disease, identifying ways to eliminate the carrier state, and better methods of disease control. Finally, further identification of the CRISPR-Cas9 system may provide revolutionary new tools for genome engineering and editing that will have broad applications for basic biology and medicine.
Acknowledgements
This chapter is dedicated to the memory of Prof. Dr. Dr. Dr. hc, Werner Köhler (1929–2021), an eminent scholar, colleague, supporter of science, and all things “Streptococcus”.
References
Ackermann, H. W., Martin, M., Vieu, J.-F., & Nicolle, P. (1982). Felix d’Herelle: His Life and Work and the Foundation of a Bacteriophage Reference Center. ASM News, 48(8), 347-348.
Adams, F. (1849). The genuine works of Hippocrates: translated from the Greek with a preliminary discourse and annotations. London: Sydenham Society.
Alouf, J. E. (1980). Streptococcal toxins (streptolysin O, streptolysin S, erythrogenic toxin). Pharmacology & Therapeutics, 11(3), 661-717.
Alouf, J. E., & Horaud, T. (1997). Streptococcal Research at Pasteur Institute from Louis Pasteur’s time to date. Advances in Experimental Medicine and Biology, 418, 7-14.
Andrewes, F. W., & Christie, E. M. (1932). The haemolytic streptococci: their grouping by agglutination. London: H.M. Stationery Office.
Avery, O. T., MacLeod, C. M., & McCarty, M. (1944). Studies on the Chemical Nature of the Substance Inducing Transformation of Pneumococcal Types: Induction of Transformation by a Desoxyribonucleic Acid Fraction Isolated from Pneumococcus Type III. The Journal of Experimental Medicine, 79(2), 137-158.
Becker, W. C. (1916). The necessity of a standard blood-agar plate for the determination of hemolysis by streptococci. The Journal of Infectious Diseases, 19(6), 754-759.
Beerens, D., Franch-Arroyo, S., Sullivan, T. J., Goosmann, C., Brinkmann, V., & Charpentier, E. (2021, April 2). Survival Strategies of Streptococcus pyogenes in Response to Phage Infection. Viruses(4), 612.
Behnke, D., & Ferretti, J. J. (1980). Molecular cloning of an erythromycin resistance determinant in streptococci. Journal of Bacteriology, 144(2), 806-813.
Benedek, T. G. (2006). The history of bacteriologic concepts of rheumatic fever and rheumatoid arthritis. Seminars in Arthritis and Rheumatism, 36(2), 109-123.
Bentley, R. (2009). Different roads to discovery; Prontosil (hence sulfa drugs) and penicillin (hence beta-lactams). Journal of Industrial Microbiology & Biotechnology, 36(6), 775-786.
Bernheimer, A. W., & Pappenheimer, Jr., A. W. (1942). Factors Necessary for Massive Growth of Group A Hemolytic Streptococcus. Journal of Bacteriology, 43(4), 481-494.
Bernheimer, A. W., & Rodbart, M. (1948). The effect of nucleic acids and of carbohydrates on the formation of streptolysin. The Journal of Experimental Medicine, 88(2), 149-168.
Billroth, T. (1874). Untersuchungen über die Vegetationsformen von Coccobacteria septica und der Antheil, welchen sie an der Entstehung und Verbreitung der accidentellen Wundkrankheiten haben. Berlin: G. Reimer.
Billroth, T. (1877). Lectures on surgical pathology and therapeutics. London: The New Sydenham Society.
Bingel, K. F. (1949). Neue Untersuchungen zur Scharlachatiologie. Deustche medizinische Wochenschrift, 74, 703-706.
Brown, J. H. (1919). The use of blood agar for the study of streptococci (Vol. Monograph No. 9). New York: The Rockefeller Institute for Medical Research.
Brown, J. H. (1927). Transmissible toxicogenicity of streptococci. Bulletin of the Johns Hopkins Hospital, 41, 167-173.
Cantacuzène, J. O. (1926). Modifications subies par des streptocoques d'origine non scarlatineuse au contact de produits scarlatineux filtrès. Comptes Rendus de l'Académie des Sciences, 182, 1185-1187.
Caparon, M. G., & Scott, J. R. (1991). Genetic manipulations of the pathogenic streptococci. Methods in Enzymology, 204, 556-586.
Caravano, R. (Ed.). (1968). Current Research on Group A Streptococcus: Proceedings of a Symposium Held at the International Children's Centre, Paris, France, 16th, 18th, and 19th July, 1966. Excerpta Medica Foundation.
Casadesús, J., & Low, D. (2006). Epigenetic gene regulation in the bacterial world. Microbiology and Molecular Biology Reviews, 70(3), 830-856.
Chhatwal, G. S. (2004). Streptococcus and Streptococcal Research. Indian Journal of Medical Research, 119(Suppl May 2004), 1-262.
Clark, P. F., & Clark, A. S. (1927). A bacteriophage active against a virulent hemolytic streptococcus. Experimental Biology and Medicine, 24, 635-639.
Deltcheva, E., Chylinski, K., Sharma, C. M., Gonzales, K., Chao, Y., Pirzada, Z. A., . . . Charpentier, E. (2011, March 30). CRISPR RNA maturation by trans-encoded small RNA and host factor RNase III. Nature, 471, 602-607.
d'Herelle, F. (1915). Sur un microbe invisible antagoniste des bacilles dysenterique. Académie des Sciences, 373-375.
Dick, G. F., & Dick, G. H. (1924a). The etiology of scarlet fever. Journal of the American Medical Association, 82(4), 301-302.
Dick, G. F., & Dick, G. H. (1924b). A skin test for susceptibility to scarlet fever. Journal of the American Medical Association, 82(4), 265-266.
Dick, G. F., & Dick, G. H. (1924c). Scarlet Fever. American Journal of Public Health, 14(12), 1022-1028.
Dobell, C. (1932). Antony van Leeuwenhoek and his "Little animals"; being some account of the father of protozoology and bacteriology and his multifarious discoveries in these disciplines. New York: Harcourt, Brace and Company.
Dochez, A. R., Avery, O. T., & Lancefield, R. C. (1919). Studies on the biology of streptococcus. I. Antigenic Relationships Between Strains of Streptococcus Haemolyticus. Journal of Experimental Medicine, 30(3), 179-213.
Domagk, G. (1936). Chemotherapie der streptokokken-infektionen. Klinische Wochenschrift, 15(44), 1585-1590.
Domagk, G. (1957). Twenty-five years of sulfonamide therapy. Annals of the New York Academy of Sciences, 69(3), 380-384.
Duckworth, D. H. (1976). "Who Discovered Bacteriophage?". Bacteriological Reviews, 40(4), 793-802.
Elliott, S. D. (1950). The crystallization and serological differentiation of a streptococcal proteinase and its precursor. The Journal of Experimental Medicine, 92(3), 201-218.
Euler, C. W., Ryan, P. A., Martin, J. M., & Fischetti, V. A. (2007). M.SpyI, a DNA methyltransferase encoded on a mefA chimeric element, modifies the genome of Streptococcus pyogenes. Journal of Bacteriology, 189(3), 1044-1054.
Evans, A. C. (1934a). The Prevalence of Streptococcus Bacteriophage. Science, 80(2063), 40-41.
Evans, A. C. (1934b). Streptococcus bacteriophage: a study of four serological types. Public Health Reports, 49(47), 1386-1401.
Evans, A. C. (1934c). Streptococcus Bacteriophage and Its Usefulness for the Identification of Strains of Hemolytic Streptococci. Journal of Bacteriology, 27, 49-50.
Evans, A. C. (1936). Studies on Hemolytic Streptococci: II. Streptococcus pyogenes. Journal of Bacteriology, 31(6), 611-624.
Facklam, R. (2002). What happened to the streptococci: Overview of taxonomic and nomenclature changes. Clinical Microbiology Reviews, 15(4), 613-630.
Ferretti, J. J. (2008). Streptococcal Research: A Success Story of Collaboration Between Jena and US Scientists. Vitalprinzip Akademie (pp. 323-336). Jena: Akademie gemeinnutziger Wissenschaften zu Erfurt.
Ferretti, J. J., McShan, W. M., Ajdic, D., Savic, D. J., Savic, G., Lyon, K., . . . McLaughlin, R. (2001). Complete genome sequence of an M1 strain of Streptococcus pyogenes. Proceedings of the National Academy of Sciences of the United States of America, 98(8), 4658-4663.
Fischetti, V. A. (1989). Streptococcal M protein: molecular design and biological behavior. Clinical Microbiology Reviews, 2(3), 285-314.
Fischetti, V. A., Pancholi, V., & Schneewind, O. (1990, September). Conservation of a hexapeptide sequence in the anchor region of surface proteins from gram-positive cocci. Molecular Microbiology, 4(9), 1603-1605.
Fleischmann, R. D., Adams, M. D., White, O., Clayton, R. A., Kirkness, E. F., Kerlavage, A. R., . . . Merrick, J. M. (1995). Whole-genome random sequencing and assembly of Haemophilus influenzae Rd. Science, 269(5223), 496-512.
Fleming, A. (1929). On the antibacterial action of cultures of a Penicillium with special reference to their use in the isolation of B. influenzae. British Journal of Experimental Pathology, 10(3), 226-236.
Fox, E. N. (1974). M proteins of group A streptococci. Bacteriological Reviews, 38(1), 57-86.
Fruciano, D. E., & Bourne, S. (2007). Phage as an antimicrobial agent: d'Herelle's heretical theories and their role in the decline of phage prophylaxis in the West. Canadian Journal of Infectious Diseases & Medical Microbiology, 18(1), 19-26.
Galeotti, C. L., Bove, E., Pezzicoli, A., Nogarotto, R., Norais, N., Pileri, S., . . . Grifantini, R. (2012). Surface interactome in Streptococcus pyogenes. Molecular & Cellular Proteomics, 11(4), M111 015206.
Ginsburg, I. (1972). Mechanisms of Cell and Tissue Injury Induced by Group-a Streptococci - Relation to Poststreptococcal Sequelae. The Journal of Infectious Diseases, 126(3), 294-340.
Gotschlich, E. C., & Fischetti, V. A. (2005). The career of Maclyn McCarty. The Journal of Experimental Medicine, 201(11), 1699-1707.
Griffith, F. (1934). The Serological Classification of Streptococcus pyogenes. Journal of Hygiene, 34(4), 542-584.
Haverkorn, M. J. (Ed.). (1974). Streptococcal Disease and the Community. New York: Excerpta Medica.
Holm, S. E., & Christensen, P. (Eds.). (1982). Basic Concepts of Streptococci and Streptococcal Diseases. Surrey: Reedbooks.
Holmes, O. W. (1843). The contagiousness of puerperal fever. New England Quarterly Journal of Medicine, 1, 503-530.
Horaud, T., Bouvet, A., Leclerq, R., de Montclos, H., & Sicard, M. (Eds.). (1997). Streptococci and the Host. New York: Plenum Press.
Horn, D. L., Zabriskie, J. B., Austrian, R., Cleary, P. P., Ferretti, J. J., Fischetti, V. A., . . . Wachtfogel, Y. (1998). Why have group A streptococci remained susceptible to penicillin? Report on a symposium. Clinical Infectious Diseases, 26(6), 1341-1345.
Hynes, W. (2004). Virulence factors of the group A streptococci and genes that regulate their expression. Frontiers in Bioscience, 9, 3399-3433.
Ideker, T., Winslow, L. R., & Lauffenburger, A. D. (2006). Bioengineering and systems biology. Annals of Biomedical Engineering, 34(2), 257-264.
Ingrassia, G. F. (1553). De tumoribus praeter naturum (Vol. 1). Naples.
Jinek, M., Chylinski, K., Fonfara, I., Hauer, M., & Doudna, J. A. (2012). A programmable dual-RNA-guided DNA endonuclease in adaptive bacterial immunity. Science, 337(6096), 816-821.
Johnson, L. P., & Schlievert, P. M. (1984). Group A streptococcal phage T12 carries the structural gene for pyrogenic exotoxin type A. Molecular and General Genetics, 194(1-2), 52-56.
Johnson, L. P., Schlievert, P. M., & Watson, D. W. (1980). Transfer of group A streptococcal pyrogenic exotoxin production to nontoxigenic strains of lysogenic conversion. Infection and Immunity, 28(1), 254-257.
Karamanou, M., Panayiotakopoulos, G., Tsoucalas, G., Kousoulis, A. A., & Androutsos, G. (2012). From miasmas to germs: a historical approach to theories of infectious disease transmission. Infez Med, 20(1), 58-62.
Kendall, F. E., Heidelberger, M., & Dawson, M. H. (1937). A serologically inactive polysaccharide elaborated by mucoid strains of group a hemolytic streptococcus. The Journal of Biological Chemistry, 118, 61-69.
Ketham, J. d. (1988). The Fasciculus Medicinae of Johannes de Ketham, Alemanus : facsimile of the first (Venetian) edition of 1491. (L. Demaitre, & C. Singer, Trans.) Birmingham, AL: The Classics of Medicine Library.
Kimura, Y., Kotani, S., & Shiokawa, Y. (Eds.). (1985). Recent advances in streptococci and streptococcal diseases: proceedings of the IXth Lancefield International Symposium on Streptococci and Streptococcal Diseases held in September 1984. Berkshire: Reedbooks.
Kjems, E. (1955). Studies on streptococcal bacteriophages. I. Technique of isolating phage-producing strains. Acta Pathologica Microbiologica Scandinavica, 36(5), 433-440.
Kjems, E. (1958). Studies on streptococcal bacteriophages. 2. Adsorption, lysogenization, and one-step growth experiments. Acta Pathologica Microbiologica Scandinavica, 42(1), 56-66.
Kjems, E. (1960). Studies on streptococcal bacteriophages. IV. The occurrence of lysogenic strains among group A hemolytic streptococci. Acta Pathologica Microbiologica Scandinavica, 49, 199-204.
Köhler, W. (1964). Symposium on Streptococcus pyogenes. Zbl. Bakteriol. I. Abt. Ref.(196), 645-723.
Kohler, W. (1974). Results of the Second International Type Distribution Survey. Amsterdam: Elsevier.
Köhler, W. (2007). The present state of species within the genera Streptococcus and Enterococcus. International Journal of Medical Microbiology, 297(3), 133-150.
Köhler, W. (2008). Geschichte der Streptokkenforschung. Nürnberg: Europforum-Verlag.
Koonin, E. V., & Makarova, K. S. (2009). CRISPR-Cas: an adaptive immunity system in prokaryotes. F1000 Biology Reports, 1, 95.
Krause, R. M., & McCarty, M. (1961). Studies on the chemical structure of the streptococcal cell wall. I. The identification of a mucopeptide in the cell walls of groups A and A-variant streptococci. The Journal of Experimental Medicine, 114, 127-140.
Lancefield, R. C. (1941). Specific Relationship of Cell Composition to Biological Activity of Hemolytic Streptococci. Harvey Lectures, 36, 251.
Lancefield, R. C. (1962). Current knowledge of type-specific M antigens of group A streptococci. The Journal of Immunology, 89(3), 307-313.
Le Rhun, A., Escalera-Maurer, A., Bratovič, M., & Charpentier, E. (2019, April). CRISPR-Cas in Streptococcus pyogenes. RNA Biology, 16(4), 380-389.
Leonard, C. G., Colón, A. E., & Cole, R. M. (1968). Transduction in group A streptococcus. Biochemical and Biophysical Research Communications, 30(2), 130-135.
Ligon, B. L. (2004). Sir Walter Howard Florey – The Force Behind the Development of Pencillin. Seminars in Pediatric Infectious Diseases, 15(2), 109-114.
Loudon, I. (Ed.). (1995). Childbed Fever: A Documentary History. New York: Garland Publishing.
Loudon, I. (2000). The Tragedy of Childbed Fever. Oxford: Oxford University Press.
Makarova, K. S., Haft, D. H., Barrangou, R., Brouns, S. J., Charpentier, E., Horvath, P., . . . Koonin, E. V. (2011). Evolution and classification of the CRISPR-Cas systems. Nature Reviews Microbiology, 9(6), 467-477.
Malke, H. (1972). Transduction in Group A Streptococci. In L. W. Wannamaker (Ed.), Streptococci and Streptococcal Diseases. New York: Academic Press.
Malke, H. (1973). Phage A25-mediated transfer induction of a prophage in Streptococcus pyogenes. Molecular and General Genetics, 125, 251-264.
Malke, H., & Ferretti, J. J. (1984). Streptokinase: cloning, expression, and excretion by Escherichia coli. Proceedings of the National Academy of Sciences of the United States of America, 81(11), 3557-3561.
Malke, H., & Köhler, W. (1973). Transduction among Group-a Streptococci - Transducibility of Strains Representative of Thirty-Different M-Types. Zentralblatt Fur Bakteriologie Mikrobiologie Und Hygiene Series a-Medical Microbiology Infectious Diseases Virology Parasitology, 224(2), 194-201.
Martin, D. R., & Tagg, J. R. (Eds.). (2000). Streptococci and Streptococcal Diseases: Entering the New Millennium : Proceedings of the XIV Lancefield International Symposium on Streptococci and Streptococcal Diseases, October 11-15th 1999, Auckland, New Zealand. Porirua: Securacopy.
Maxted, W. R. (1955). The influence of bacteriophage on Streptococcus pyogenes. Microbiology, 12, 484-495.
Mazmanian, S. K., Ton-That, H., & Schneewind, O. (2001). Sortase-catalysed anchoring of surface proteins to the cell wall of Staphylococcus aureus. Molecular Microbiology, 40(5), 1049-1057.
McCarty, M. (1952). The Biology of Group A Streptococci. Minneapolis: University of Minnesota Press.
McCarty, M. (1986). The Transforming Principle, Discovering that Genes are Made of DNA. New York: W.W. Norton and Company.
McCormick, J. K., Peterson, M. L., & Schlievert, P. M. (2006). Toxins and Superantigens of Group A Streptococci. In V. A. Fischetti, R. P. Novick, J. J. Ferretti, D. A. Portnoy, & J. I. Rood (Eds.), Gram-Positive Pathogens (2nd ed.). Washington, DC: ASM Press.
McCullor, K., Postoak, B., Rahman, M., King, C., & McShan, W. M. (2018, November). Genomic Sequencing of High-Efficiency Transducing Streptococcal Bacteriophage A25: Consequences of Escape from Lysogeny. Journal of Bacteriology, 200(23), e00358-18.
McKane, L., & Ferretti, J. J. (1981). Phage-host interactions and the production of type A streptococcal exotoxin in group A streptococci. Infection and Immunity, 34(3), 915-919.
McLaughlin, R. E., & Ferretti, J. J. (1995). Electrotransformation of Streptococci. Methods in Molecular Biology, 47, 185-193.
McShan, W. M., Tang, Y. F., & Ferretti, J. J. (1997). Bacteriophage T12 of Streptococcus pyogenes integrates into the gene encoding a serine tRNA. Molecular Microbiology, 23(4), 719-728.
Mora, M., Bensi, G., Capo, S., Falugi, F., Zingaretti, C., Manetti, A. G., . . . Telford, J. L. (2005). Group A Streptococcus produce pilus-like structures containing protective antigens and Lancefield T antigens. Proceedings of the National Academy of Sciences of the United States of America, 102(43), 15641-15646.
Nasser, W., Beres, S. B., Olsen, R. J., Dean, M. A., Rice, K. A., Long, S. W., . . . Musser, J. M. (2014). Evolutionary pathway to increased virulence and epidemic group A Streptococcus disease derived from 3,615 genome sequences. Proceedings of the National Academy of Sciences of the United States of America, 111(17), E1768-E1776.
National Academy of Sciences. (1987). Biographical Memoirs. Washington, DC: The National Academies Press.
National Center for Biotechnology Information. (n.d.). Genome. Retrieved May 31, 2022, from National Library of Medicine: https://www.ncbi.nlm.nih.gov/genome/
Nevelius, E., & Gustafsson, C. (2020, October 7). The Nobel Prize in Chemistry 2020 - Press Release. Retrieved May 31, 2022, from The Nobel Prize: https://www.nobelprize.org/uploads/2020/10/press-chemistryprize2020.pdf
Nida, S. K., & Ferretti, J. J. (1982). Phage influence on the synthesis of extracellular toxins in group A streptococci. Infection and Immunity, 36(2), 745-750.
Nida, S. K., Houston, C. W., & Ferretti, J. J. (1979). Erythrogenic-toxin production by group A streptococci. In M. T. Parker (Ed.), Pathogenic streptococci (p. 66). Chertsey, Surrey, England: Reed Books.
Orefici, G. (Ed.). (1992). New Perspectives on Streptococci and Streptococcal Infections: Proceedings of the XI Lancefield International Symposium on Streptococci and Streptococcal Infections. Stuttgart: Gustav Fischer.
Parker, M. T. (Ed.). (1979). Pathogenic Streptococci. Surrey: Reddbooks.
Pasteur, L. (1879). Sépticémie puerpérale. Bulletin de l'académie de médecine, 8, 271-274; 505-508.
Proft, T., & Fraser, J. D. (2006). Streptococcal superantigenic toxins. In J. E. Alouf, & M. R. Popoff (Eds.), The Comprehensive Sourcebook of Bacterial Protein Toxins (Third ed., pp. 844-861). Amsterdam: Elsevier.
Richards, V. P., Palmer, S. R., Pavinski Bitar, P. D., Qin, X., Weinstock, G. M., Highlander, S. K., . . . Burne, R. A. (2014). Phylogenomics and the dynamic genome evolution of the genus Streptococcus. Genome Biology and Evolution, 6(4), 741-753.
Rodríguez-Iturbe, B., & Batsford, S. (2007). Pathogenesis of poststreptococcal glomerulonephritis a century after Clemens von Pirquet. Kidney International, 71(11), 1094-1104.
Rogers, H. J. (1945). The conditions controlling the production of hyaluronidase by micro-organisms grown in simplified media. Biochemical Journal, 39(5), 435-443.
Rolleston, J. D. (1928, November). The History of Scarlet Fever. British Medical Journal, 2(3542), 926-929.
Rosenbach, F. J. (1884). Mikro-organismen bei den Wund-Infections-Krankheiten des Menschen. Wiesbaden: Bergmann.
Schottmuller, H. (1903). Die Artunterscheidung der für den Menschen pathogenen Streptokokken durch Blutagar. Münchener med Wochenschrift, 50, 848-853; 909-912.
Schwartz, M. (1997). Historical Streptococci. Advances in Experimental Medicine and Biology, 418, 1-2.
Scott, J. R., & Fischetti, V. A. (1983). Expression of streptococcal M protein in Escherichia coli. Science, 221(4612), 758-760.
Semmelweis Society. (2009). Biography. Retrieved April 21, 2015, from Semmelweis Society International: http://semmelweis.org/about/dr-semmelweis-biography/
Semmelweis, I. (1983). The Etiology, Concept and Prophylaxis of Childbed Fever. (K. C. Carter, Trans.) Madison, Wisconsin: University of Wisconsin Press.
Sennert, D. (1628). Synopsis doctrinae de febribus de libris de febribus excerpta et ab eodem XIV Disputationed comprehensa (disp XIII). Wittebergiae.
Slade, H. D., & Knox, G. A. (1950). Nutrition and the role of reducing agents in the formation of streptolysin O by a group A hemolytic streptococcus. Journal of Bacteriology, 60(3), 301-310.
Sorek, R., Kunin, V., & Hugenholz, P. (2008). CRISPR - a widespread system that provides acquired resistance against phages in bacteria and archaea. Nature Reviews Microbiology, 6(3), 181-186.
Sriprakash, K. S. (Ed.). (2006). Streptococci – New Insights into an Old Enemy. Amsterdam: Elsevier.
Stevens, D. L. (1995). Streptococcal toxic-shock syndrome: spectrum of disease, pathogenesis, and new concepts in treatment. Emerging Infectious Diseases, 1(3), 69-78.
Stock, A. H. (1939). Studies on the hemolytic streptococcus I. Isolation and concentration of erythrogenic toxin of the NY5 strain of hemolytic streptococci. 36(6), 489-498.
Stollerman, G. H. (1975). Rheumatic Fever and Streptococcal Infection. New York: Grune and Stratton.
Strother, E. (1716). Criticon Febrium, Or, a Critical Essay on Fevers; With the Diagnosticks and Methods of Cure, in All the Different Species of Them to Which Is Prefix'd (Vol. 8). London: Charles Rivington.
Stuart, J. G., & Ferretti, J. J. (1978). Genetic analysis of antibiotic resistance in Streptococcus pyogenes. Journal of Bacteriology, 133(2), 852-859.
Summers, W. C. (1999). Félix d`Herelle and the Origins of Molecular Biology. New Haven: Yale University Press.
Swedo, S. E., Leonard, H. L., Garvey, M., Mittleman, B., Allen, A. J., Perlmutter, S., . . . Dubbert, B. K. (1998). Pediatric autoimmune neuropsychiatric disorders associated with streptococcal infections: clinical description of the first 50 cases. The American Journal of Psychiatry, 155(2), 264-271.
Sydenham, T. (1676). Observationes Medicae circa morhonim acutorum Historiam et Curationeni. London.
Tagg, J. R., Skjold, S., & Wannamaker, L. W. (1976). Transduction of bacteriocin determinants in group A streptococci. The Journal of Experimental Medicine, 143(6), 1540-1544.
The Working Group on Severe Streptococcal Infections. (1993). Defining the group A streptococcal toxic shock syndrome. Rationale and consensus definition. The Journal of the American Medical Association, 269(3), 390-391.
Todd, E. W., & Hewitt, L. F. (1932). A new culture medium for the production of antigenic streptococcal hemolysin. The Journal of Pathology and Bacteriology, 35, 973-974.
Totolian, A. (1994). Pathogenic Streptococci: present and future. St. Petersburg: Lancer.
Trask, J. D. (1926). STUDIES IN SCARLET FEVER: I. The Amount of Scarlatinal Toxin in the Blood of Patients with Scarlet Fever. Journal of Clinical Investigation, 3(2), 391-396.
Twort, F. W. (1915). An investigation on the nature of ultra-microscopic viruses. Lancet, 186(4814), 1241-1243.
Updyke, E. L., & Nickle, M. I. (1954). A dehydrated medium for the preparation of type specific extracts of group A streptococci. Applied Microbiology, 2(2), 117-118.
van de Rijn, I., & Kessler, R. E. (1980). Growth characteristics of group A streptococci in a new chemically defined medium. Infection and Immunity, 27(2), 444-448.
Wannamaker, L. W., & Matsen, J. M. (1972). Streptococci and Streptococcal Diseases, Recognition, Understanding, and Management. New York: Academic Press.
Watson, D. W. (1960). Host-parasite factors in group A streptococcal infections. Pyrogenic and other effects of immunologic distinct exotoxins related to scarlet fever toxins. The Journal of Experimental Medicine, 111, 255-284.
Weeks, C. R., & Ferretti, J. J. (1984). The Gene for Type-a Streptococcal Exotoxin (Erythrogenic Toxin) Is Located in Bacteriophage-T12. Infection and Immunity, 46(2), 531-536.
Wessels, M. R. (2006). Polysaccharide of group A streptococci. Washignton, DC: ASM Press.
Zabriskie, J. B. (1964). The Role of Temperate Bacteriophage in the Production of Erythrogenic Toxin by Group a Streptococci. The Journal of Experimental Medicine, 119, 761-780.
- Introduction
- Scarlet Fever
- Puerperal Fever
- Discovery of Streptococci
- Differentiation and Classification of Streptococci
- Biology of the Group A Streptococci
- Cultural Conditions and Growth
- Ultrastructure and Cellular Components
- Streptococcal Bacteriophages
- Extracellular Products
- Streptococcal Diseases
- Treatment of Streptococcal Diseases
- Genetics and Genomics
- Conclusion
- Acknowledgements
- References
- Review History of Streptococcal Research.[Streptococcus pyogenes: Basic ...]Review History of Streptococcal Research.Ferretti J, Köhler W. Streptococcus pyogenes: Basic Biology to Clinical Manifestations. 2016
- Review Chapter 33: the history of movement disorders.[Handb Clin Neurol. 2010]Review Chapter 33: the history of movement disorders.Lanska DJ. Handb Clin Neurol. 2010; 95:501-46.
- Skin Changes in Suspected Lyme Disease.[Acta Dermatovenerol Croat. 2023]Skin Changes in Suspected Lyme Disease.Vržogić P, Perica A. Acta Dermatovenerol Croat. 2023 Dec; 31(4):225-228.
- Review Streptococcal puerperal sepsis and obstetric infections: a historical perspective.[Rev Infect Dis. 1986]Review Streptococcal puerperal sepsis and obstetric infections: a historical perspective.Charles D, Larsen B. Rev Infect Dis. 1986 May-Jun; 8(3):411-22.
- Clinical trials and the COVID-19 pandemic.[Hell J Nucl Med. 2020]Clinical trials and the COVID-19 pandemic.Retsas S. Hell J Nucl Med. 2020 Jan-Apr; 23(1):4-5.
- History of Streptococcus pyogenes Research - Streptococcus pyogenes: Basic Biolo...History of Streptococcus pyogenes Research - Streptococcus pyogenes: Basic Biology to Clinical Manifestations
Your browsing activity is empty.
Activity recording is turned off.
See more...