Readers may use this article as long as the work is properly cited, the use is educational and not for profit, and the work is not altered. See http://creativecommons.org/licenses/by-nc-nd/3.0 for details.
NCBI Bookshelf. A service of the National Library of Medicine, National Institutes of Health.
This publication is the third in a series of American Diabetes Association compendia on the diabetic foot. Previous installments focused on the diagnosis and management of diabetes foot complications and infections. Here, the authors turn their attention to the latest evidence-based therapies for diabetic foot ulcers (DFUs). The monograph begins with an overview of the current state of diabetic foot care, as well as a brief history of oxygen therapy for the treatment of DFUs. The most recently published evidence-based data concern topical oxygen therapies, and these are described in detail. Subsequent sections summarize the evidence published mainly in the past decade for specific treatments, including autologous leucocyte, platelet, and fibrin multilayered patches; sucrose octasulfate dressings; and negative pressure wound therapy. The authors discuss the evidence related to the use of new therapies specifically for the treatment of neuropathic and neuroischemic lesions. They then look to the future at new treatment approaches in the development pipeline, as well as the emerging role of wearable technologies such as digitally connected insoles and socks in preventing DFU recurrence. Throughout the compendium, the authors present their view of current and forthcoming treatment options and identify areas worthy of additional research in the years ahead.
After the outstanding success of two previous American Diabetes Association (ADA) compendia on the diabetic foot—Diagnosis and Management of Diabetic Foot Complications (1) and Diagnosis and Management of Diabetic Foot Infections (2)—the Association asked us to proceed with a third volume.
At the time of writing, the International Diabetes Federation had just published the 10th edition of its IDF Diabetes Atlas (3), which, in many ways, makes for depressing reading. The past 2 years have seen a 16% increase in the global prevalence of diabetes, with one in 10, or >537 million, adults now having the disease. However, depressing though these data are, they do not take into account the impact the current global coronavirus disease 2019 (COVID-19) pandemic will likely have on the worldwide prevalence of diabetes and its complications. Our pessimism regarding this possible impact is supported by a recent study from the United Kingdom which, using A1C as a surrogate, estimated the effect of the pandemic on diabetes diagnosis and management (4). An 80% reduction in A1C testing was reported in April 2020; in the first 6 months of the pandemic, an estimated 1.4 million A1C tests were missed for routine monitoring of glycemic control, and >5 million more tests were missed for the diagnosis of diabetes. Thus, we fear a tsunami of diabetes and its late complications in the next decade.
Sadly, despite recent progress in prevention, diagnosis, and management, these recent developments will likely result in an increased incidence of diabetic foot ulcers (DFUs). Thus, the need for good, evidence-based, efficacious treatments for chronic DFUs is more important than ever.
Previously, we reported on a renaissance in diabetic foot care, with new evidence-based treatments (1,2). A number of new therapies are now available, with efficacy supported by well-designed, randomized controlled trials (RCTs).
Although there is a long history of the use of oxygen therapies for chronic DFUs, hyperbaric oxygen therapy (HBOT), which is most commonly used in the United States, has little evidence to support its use, and almost all RCTs of this treatment have been negative (5). However, recent trials of topical oxygen therapy (TOT) for DFUs have been encouraging (6,7). Thus, we asked our expert writing group first to review the history of oxygen therapies in the diabetic foot and then to discuss the increasing evidence that TOT can accelerate the healing of chronic DFUs.
Other new, evidence-based therapies for DFUs include autologous leucocyte, platelet, and fibrin multilayered patches for hard-to-heal ulcers and sucrose octasulfate dressings for hard-to-heal neuroischemic ulcers (8,9). This treatise includes reviews of both of these new therapies by members of our author group (F.L.G. and M.E.E.) who participated in the clinical trials of the respective agents. Additionally, the use of negative pressure wound therapy (NPWT), which is also supported by evidence from RCTs, is reviewed. The final two sections of this compendium explore putative new, evidence-based therapies for DFUs that are in the pipeline and, most importantly, how we might engage digital technology and other aids to facilitate the prevention of DFU recurrence.
History of Oxygen Therapy for the Treatment of DFUs
Oxygen is essential for energy production and tissue survival in humans. However, it is not only a prerequisite for aerobic cell metabolism. Reactive oxygen species such as hydrogen peroxide and superoxide are crucial in the oxidative killing of bacteria. They also serve as cellular messengers to stimulate key processes in wound healing, including cell motility, cytokine action, and angio-genesis. Inflammatory reactions and reparative processes, including cell proliferation and collagen synthesis following tissue injuries such as DFUs, increase oxygen requirements. If the need for oxygen is beyond the body's delivery capacity to the affected area, the healing process will be compromised, increasing the risk of severe infections and gangrene. Although the role of oxygen in ulcer healing is not yet completely understood, many experimental and clinical studies have shown DFU healing to be impaired in hypoxic conditions.
In diabetes, macrovascular and microvascular disease contribute to impaired blood circulation in the lower extremities. It is mandatory to evaluate peripheral circulation early in the course of DFU treatment, as an open or endovascular procedure might restore the vascular and oxygen-delivering capacity to a level conducive to ulcer healing. Macrovascular disease tends to occur at a younger age and engages more distal vessels in people with diabetes. Microvascular dysfunction is an even more treacherous companion to diabetes, as it progresses over a long time and engages all organ systems. The consequences of the capillary basement membrane thickening with endothelial hypertrophy, increased permeability, and decreased responsiveness to environmental and physical changes are frequently present in people with DFUs. These changes result in diminished blood flow, decreased oxygen tension, tissue edema, and subsequent capillary rarefaction. Because the rate of oxygen delivery is inversely proportional to the square of the distance and directly proportional to the partial pressure of oxygen (PO2) at the initial point at the capillary, these consequences lead to reduced oxygen delivery capacity and increased risk of clinically signifi-cant ischemia.
Accordingly, as a rational consequence of the observation that the lack of oxygen decreases ulcer healing, applying oxygen either topically or systemically has a long history, and several methods have been implemented to increase DFU healing by modifying oxygen concentration.
In 1775, Joseph Priestley tested his discovered dephlogisticated air (later called oxygen by Lavoisier) on himself and wrote, “The feeling of it in my lungs was not sensibly different from that of common air, but I fancied that my breast felt peculiarly light and easy for some time afterwards” (10,11). The first investigations on the efficacy of oxygen in treating disease occurred from 1798 to 1800 at the Pneumatic Institution in Bristol, U.K., and it is possible that this is where the first oxygen inhalation treatment for a DFU was given. Apart from that, many of the techniques used in modern oxygen therapy, including corrugated noncrushable breathing tubes, mouthpieces, and the mass production of gases, originate from this early work (12). These early rational years were followed by dark ages when intermittent oxygen treatment became a panacea and was brought to the market by charlatans and profiteers. This era climaxed in 1869, when an article in The Lancet advocated oxygenated bread and water (13). The early 1890s were a new dawning for oxygen therapy, during which continuous inhaling was successfully introduced in people with pneumonia (14). The origin of rational systemic medical oxygen use can be dated to early 1917, when John Scott Hal-dane published an article titled “The Therapeutic Administration of Oxygen” (15).
Oxygen was added to the armamentarium of DFUs some 50 years later. Anecdotal stories of reduced infection and hastened healing resulting from daily flooding of DFUs with oxygen in hospitalized patients might be considered the origin of TOT (A. Nilsson, personal communication). TOT, the administration of oxygen applied topically over injured tissue by either continuous or pressurized delivery, was introduced in the mid-20th century (16). A bag, boot, or extremity chamber is placed around the DFU, sealed tightly to prevent leakage, and attached to an oxygen delivery device or tank. In TOT, oxygen is given with either constant or cyclical pressure. If not continuous, a typical session lasts 90 minutes, and the therapy is given three to five times per week. In animal models, TOT has been shown to increase wound tissue PO2 levels tenfold, accompanied by increased vascular endothelial growth factor (VEGF) levels, signs of improved angiogenesis, and better collagen quality. Until recently, clinical evidence supporting TOT in the healing of DFUs has been scarce, but in recent years, several positive, well-designed trials of TOT have been published and are discussed in detail below.
Parallel to the introduction of TOT, Brummelkamp et al. (17) reported beneficial effects of HBOT for infected ischemic leg ulcers, and in 1979, Hart and Strauss (18) published the first DFU study. HBOT is a short-term, high-dose oxygen inhalation and diffusion therapy that is delivered systemically through airways and blood and achieved by having the patient breathe concentrated oxygen at a pressure >1 absolute atmosphere (ATA). The treatment is given in hyperbaric chambers. Patients with DFUs are usually treated once daily for 80–90 minutes at 2.0–2.5 ATA (the pressure 10–15 m below sea surface), on 5 days per week for 6–8 weeks.
The rationale for HBOT is to restore abnormal tissue oxygen tension by applying basic physical gas laws. Compared to normobaric air-breathing, the volume of dissolved oxygen in plasma and tissue during an HBOT session increases 20-fold, allowing survival without erythrocytes. In cell and animal models, HBOT has been shown to improve leukocyte function, enhance neovascularization, reduce edema, downregulate inflammation, and enhance granulation tissue formation. Altogether, 210 patients with hard-to-heal ulcers without the need for or possibility of vascular intervention at the time of randomization have been included in RCTs reporting long-term follow-up of at least 1 year (19–21). Of the patients receiving HBOT, 63% healed compared to 20% of those in control groups. Two RCTs that included patients with severe peripheral artery disease (PAD) and allowed for early vascular intervention have been published. In 68 hospitalized patients with severe infection or PAD, Faglia et al. (22) demonstrated a statistically significant reduction in major amputation of 9 versus 33% in favor of HBOT, although this trial later came under criticism. More recently, Santema et al. (23) showed a nonsignificant 45% reduction in major amputations of 12 versus 22% during the first year after HBOT. However, this study exemplified one of the main problems with HBOT studies in that it was underpowered, with pre-terminated enrollment when only 53% of the preplanned 226 participants had been randomized.
Robust evidence is lacking for the selection of a treatment regimen leading to optimal therapeutic benefit (i.e., hyperbaric pressure level, duration of treatment sessions, number of HBOT sessions, and—not least—timing of HBOT). Transcutaneous oxygen pressure (TcPO2), in contrast to ankle-brachial index (ABI) or toe-brachial index (TBI), seems to be helpful to predict treatment outcome, with the increment during hyperbaric conditions being the best predictor. Furthermore, the cost of HBOT, especially in the United States, has resulted in questioning of its usefulness. Finally, there have been a number of negative studies on HBOT in DFUs in the past two decades, although these, too, received much criticism (5).
In the 21st century, new methods to increase ulcer oxygenation have focused on dressings and local treatments such as a topical spray containing purified porcine hemoglobin to facilitate oxygen transport from the surface to the bottom of the wound bed. The clinical efficacy of these methods remains to be proven. Future possible noteworthy methods include an alginate gel containing oxygen-storing droplets and a gel containing microspheres with hydrogen peroxide.
History repeats itself. Mirroring the use of oxygen a century earlier, HBOT was, during parts of the 20th century, promoted as a cure for almost any disease, often without supporting evidence beyond single case reports. These issues have affected the reputation of the therapy. Hypoxia impairs the healing of DFUs. Both TOT and HBOT can remedy tissue hypoxia, and several RCTs have shown their potential for improving DFU healing. However, rigorously designed, adequately powered, and well-executed RCTs are needed to accurately validate the potential benefits of these and other oxygen concentration– increasing therapies in the plausible future DFU armamentarium.
New, Evidence-Based Therapies for DFUs
TOPICAL OXYGEN THERAPY
TOT has been misunderstood and sometimes maligned since it was first described in 1969 (16). Another, later article (24) reported on a poorly designed trial of topical “hyperbaric” oxygen therapy that demonstrated no significant differences in healing chronic DFUs after only 2 weeks of treatment compared to best-practice standard care treatment in 28 hospitalized patients. Nonetheless, TOT continued to be used clinically throughout the ensuing decades, albeit with primarily observational studies that suggested positive outcomes in a variety of wounds (25–29).
Oxygen is obviously essential for life itself, and it is no less essential for wound repair, being a necessary co-factor for several oxygen-dependent enzymes that are crucial in the wound healing cascade (Table 1) (26). The over-arching and long-debated concern is whether topically administered oxygen can actually promote wound repair. Despite the premise by proponents of HBOT that TOT could not meaningfully affect wound healing, clinical comparative studies in both DFUs and venous leg ulcers have suggested otherwise (27,29).
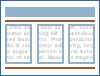
TABLE 1
Role of Oxygen in Wound Healing
A particularly compelling animal study in 2005 (30) augmented many clinical observations by demonstrating histological, biochemical, and regenerative advantages of using topically administered oxygen compared to ambient air as a control treatment. Recognizing that more rigorous studies were required to provide the evidence necessary to fully embrace this therapy as a proven wound healing adjunct, multiple formal clinical trials were initiated and have been reported in the past decade (6,31–33).
TOT Delivery Devices
There are three general types of delivery systems for TOT, each of which allows for ambulatory or home-based treatment: 1) those generating continuous delivery of oxygen (CDO) at negligible pressures, 2) low constant pressure delivery in a contained chamber, and 3) higher cyclically pressurized and humidified delivery in a contained extremity chamber (Table 2) (32,34). CDO devices apply topical continuous diffusion of nonpressurized oxygen through small cannulas to semi-occlusive or proprietary wound dressings. Small, portable, battery-powered electrochemical oxygen generators supply a continuous flow of pure oxygen over the wounds 24 hours per day at a flow rate of up to 15 mL/hour. The low-constant-pressure (22-mmHg) device uses an oxygen concentrator to deliver oxygen in a simple plastic boot that is placed over the extremity with the ulcer. The third system differs from the other devices in being a multimodality approach that applies cyclically pressurized (10–50 mbar) oxygen within a disposable extremity chamber connected to a controller unit and oxygen concentrator. Humidity can be added to this system if required. The higher PO2 produced especially by the latter devices results in a larger pressure gradient that promotes the diffusion of oxygen molecules into the hypoxic wound tissue, thereby enhancing multiple molecular and enzymatic functions (32,34).
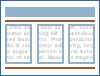
TABLE 2
Types of Topical Oxygen Devices
New Evidence for TOT
Most of the previous clinical studies on TOT for chronic wounds were observational, including several comparative cohort studies. Even when conducted prospectively, lack of blinding and effective randomization brought their generally positive outcomes into question. These concerns have been remedied with the recent publication of several RCTs and systematic reviews/meta-analyses in chronic DFUs that confirm enhanced healing rates in topical oxygen– treated patients compared to good standard care control treatments.
Although inconclusive, the first formal, sham-controlled, multi-center RCT using a CDO device on University of Texas (UT) 1A category DFUs was published in 2017 (31). For the primary endpoint of complete healing at 12 weeks in the intention-to-treat (ITT) population (n = 128), 53.8% of active CDO patients healed compared to 49.2% of those receiving the control sham-plus-standard-care treatment (P = 0.42). This trial was generally well-designed and conducted and incorporated important facets of high-quality DFU trial design: a run-in period, centralized randomization, double blinding of treatment allocation, and a primary outcome of complete healing at 12 weeks based on ITT populations.
Subsequently, the pivotal trial of another CDO device reported positive results in a 12-week multi-center, blinded, sham-controlled, parallel-group clinical trial of UT 1A category DFUs (6). After a 2-week run-in period of standard care treatment with <30% wound area reduction, 146 eligible patients were randomized. The primary outcome again was the percentage of patients in each group achieving complete healing at 12 weeks. Significantly, 32.4% of CDO-treated patients completely healed compared to 17.7% of those in the sham control group (95% CI 1.05–3.59, P = 0.033). Time to ulcer closure was also shorter in patients who received CDO therapy (P = 0.015).
The most recent multicenter RCT comparing another CDO device against standard care treatment for healing chronic DFUs was published in 2021 (33). This 12-week open-label, unblinded study randomized 145 patients with chronic DFUs to either standard care treatment using primarily a total contact cast or to the active group receiving TOT plus standard care/cast. Once more, the primary outcome was complete healing at 12 weeks using an ITT analysis. Significantly, 44.4% of those in the TOT group healed at 12 weeks compared with 28.1% of those in the standard care group (P = 0.044). As with other reported TOT studies, there were very few device-related adverse events.
Using the cyclically pressurized topical wound oxygen device for healing recalcitrant DFUs (UT category 1A–2D), a robust multicenter, sham-controlled, double-blinded RCT was reported in 2020 (7). At the first planned (a priori) interim analysis point, the active therapy was found to be superior to the sham, with a closure rate at 12 weeks of 41.7% compared to 13.5% (P = 0.007). Enhanced healing rates in the TOT group were also demonstrated by adjusted Cox proportional hazards modeling that yielded a hazard ratio of 4.66 (97.8% CI 1.36– 15.98, P = 0.004). Distinct from the other RCTs, researchers in this trial also found that 56% of active-treatment patients achieved 100% healing at 12 months vs. 27% in the sham arm (P = 0.013). Of note, patient adherence to the home-based therapy was very high, and there were no device-related adverse events.
Very recently, this same device was studied to examine its real-world impact on hospitalizations and amputations in patients with DFUs (35). This retrospective, comparative cohort study of 202 patients with DFUs found that 6.6 and 12.1% of those using cyclically pressurized topical oxygen had hospitalizations and amputations, respectively, at 1 year compared to 54.1 and 41.4%, respectively, of those who had not used this adjunctive topical oxygen modality (each P <0.0001). This represents an 88% reduction in hospitalizations and a 71% reduction in amputations at 1 year compared to patients who did not receive TOT but had access to all other available advanced modalities. Adjusted logistic regression of a matched cohort of these patients demonstrated a nearly ninefold greater risk of wound-related hospitalization (odds ratio [OR] 8.667, 95% CI 3.101–24.219, P <0.0001) and a nearly fivefold greater risk of amputation (OR 4.887, 95% CI 1.840–12.985, P = 0.0015) for patients not treated with TOT compared to those who were treated with cyclically pressurized topical oxygen.
Three recent systematic reviews with meta-analyses addressed the clinical effectiveness of TOT for healing chronic DFUs (36–38). Despite some methodological deficiencies and heterogeneity in populations and study types, they uniformly indicated that TOT (using CDO and cyclically pressurized devices) can significantly improve wound healing among people with chronic DFUs. At the time of writing, a fourth systematic review has been submitted for publication with the title “Efficacy of Topical Wound Oxygen Therapy in Healing Chronic Diabetic Foot Ulcers: Systematic Review and Meta-Analysis” (MJ Carter, RG Frykberg, A Oropallo, CK Sen, DG Armstrong HKR Nair, TE Serena, unpublished observations). Focused exclusively on recent, high-quality RCTs, this meta-analysis reported an overall 59% higher probability of healing chronic DFUs at 12 weeks by using adjunctive TOT versus optimal standard care treatment alone (relative risk 1.59, 95% CI 1.02–2.48).
With the growing body of evidence supporting the use of TOT for the treatment of chronic DFUs, an expert multidisciplinary panel developed a Delphi consensus to establish guidelines for prescribing TOT (39). Engaging participants on such topics as published clinical evidence, pre-treatment assessments, indications, duration of therapy, and a focused clinical algorithm, the Delphi approach resulted in the consensus that TOT should be incorporated into clinical practice as an evidence-based treatment for chronic DFUs.
In summary, TOT has come of age and the evidence supporting its efficacy in healing chronic DFUs can no longer be disputed. Indeed, all four recent systematic reviews corroborate the many observational and controlled studies published in the past two decades that demonstrated the clinical efficacy of TOT. In 2021, an expert consensus panel provided treatment guidelines for this therapy and supported its use in clinical practice. Accordingly, it is anticipated that future evidence-based clinical practice guidelines will similarly recognize the proven benefits of TOT in healing chronic DFUs and establish recommendations for its use.
TOPICAL THERAPIES FOR NEUROPATHIC DFUs
DFUs are estimated to be prevalent in ~1.7% of people with diabetes, with an annual incidence of 2.2% (40). In almost all health care economies, the treatment of unhealing wounds consumes a large proportion of total health care resources. Among the reasons for this high use of health care resources is the apparent slow healing of DFUs. Data from the National Diabetic Footcare Audit of England and Wales indicate that less than half of all the 33,155 DFUs registered between 2015 and 2018 healed by 12 weeks (41), and some never heal.
Despite this, it is surprising how little high-quality evidence we have to support best practices in the choice of wound care dressing.
Defining “High-Quality Evidence”
When evaluating the evidence for wound care products, it is important to ensure that all basic aspects of best-practice care were included in the study. These include sharp debridement when appropriate, revascularization where needed and possible, treatment of clinical infection, and, most importantly for neuropathic ulcers, off-loading of the area in line with guidance from the International Working Group of the Diabetic Foot (IWGDF) (42). When best-practice care is not standardized in any intervention study of DFUs, it is difficult to be certain that the effects seen in the study are the result of the intervention or simply of differences in the quality of basic care between the comparison groups.
RCTs provide the most robust evidence of effect, although assessment of the quality of an RCT requires care because of the overall number of criteria that must be satisfied (43). The concept of “bias” is frequently used in the assessment of intervention trials and refers to any factor other than the treatment being studied that could have contributed to the study outcome. Repeated systematic reviews undertaken on behalf of the IWGDF (44) have concluded that many of the trials of interventions to improve healing of DFUs were at high risk of bias.
However, there are a few interventions for which the quality of evidence is sufficiently high that we can be relatively certain of their efficacy in improving healing of some DFUs when best-practice care alone has not sufficed. These are described in the sections below.
Topical Sucrose Octasulfate– Impregnated Dressings
In chronic wounds, it is thought that expression of matrix metal-loproteinases (MMPs) can be exaggerated, leading to abnormal tissue breakdown and prolonged healing. A novel dressing has been developed that incorporates sucrose octasulfate into a nonadherent dressing and inhibits the action of MMPs. The evidence to support the clinical efficacy of this product comes from one high-quality, multinational, multicenter, double-blinded RCT reporting a statistically signifi-cant benefit from the use of these dressings compared to a placebo, as described in more detail in the next section (9). The U.K. National Institute for Care and Clinical Excellence has approved the product for use in hard-to-heal neuropathic ulcers even in the absence of apparent ischemia (45). In the United States, this product is not yet available but is undergoing clinical studies.
Topical Fibrin and Leucocyte Platelet Patch
One possible treatment option for nonhealing ulcers is the use of platelet-rich plasma or platelet-rich fibrin, which might promote healing of DFUs by promoting the release of cytokines and growth factors involved in tissue repair, angiogenesis, and inflammation. Although the use of platelet preparations is not new, evidence of their benefits has been inconsistent. However, the recent development of multilayered patches comprising autologous leucocytes, platelets, and fibrin, which can be made at the bedside without adding any reagents, is a new option.
The use of these patches was recently assessed in a high-quality, large, multinational, multicenter, outcome-blinded RCT (8). Participants were patients with hard-to-heal ulcers, defined as those with <50% reduction in ulcer size after a 4-week run-in period with good basic care and that were not infected at the time of randomization. Inclusion criteria included an ABI of the index limb ≥0.7 or palpable foot pulses. Just over half of the participants (52%) had what could be considered a normal ABI (1.0–1.4), although no subgroup analysis has been presented regarding any possible influence of PAD on the final outcome. Overall, though, significantly more ulcers achieved complete healing by 20 weeks in the intervention group than in the group receiving standard care only (45/132 [34%] vs. 29/134 [22%]). A limitation of this study was that it was not possible to blind the patients or those delivering the therapy; however, healing was assessed clinically by an independent assessor blinded to treatment allocation. The intervention involved weekly visits for venesection, preparation, and application of the patch, which may have significant cost implications. Nevertheless, the IWGDF guidelines include a cautious recommendation for the use of this intervention (46).
Placenta–Derived Products
Human placental membranes contain a combination of growth factors, collagen-rich extracellular matrix, and cells, including mesenchymal stem cells, neonatal fibroblasts, and epithelial cells, that provide mechanisms for coordinated wound healing. Several products derived from different components of the placenta and umbilical cord have been developed. Cryopreserved preparations contain living cells and growth factors, whereas dehydrated products, which are easier to store and handle, contain growth factors but no living cells.
A number of trials have been published (46), and interest in this type of therapy has developed rapidly. Three RCTs of note have been assessed as being at low risk of bias; although none was blinded to patients or care providers, all had outcomes assessed in a manner that was blinded with regard to allocation group.
The first compared a cryopre-served amniotic membrane allograft to good standard care in a single-blinded, multicenter trial (47) and found a significant increase in the incidence of ulcer closure at 12 weeks (31/50 [62%] vs. 10/47 [21.3%]). This study included participants with an index ulcer that was ≥1 cm2 and not infected at randomization. Both neuropathic and neuroischemic ulcers were included, although the majority were not overtly ischemic; ~22% of the index limbs had an ABI of 0.7–0.9, with the remainder being >0.9. Whether there may have been a difference in outcome dependent on ischemia is not known.
The second multicenter RCT, which assessed the use of an umbilical cord product, reported a significant improvement in ulcers healed at 12 weeks compared to good standard care (71/101 [70%] vs. 26/54 [48%]) (48).
The third study, a multicenter RCT of a dehydrated amniotic membrane allograft, also found significant differences in DFU healing at 12 weeks versus good standard care (38/54 [70%] vs. 28/56 [50%]) (49).
The latter two studies included only neuropathic ulcers, as their protocol specified that the per-fusion of the affected limb was “adequate” at randomization, although no further details were given. Ulcers were ≥1 cm2 and clinically noninfected at randomization, as in the first study.
Thus, the available evidence suggests that placenta-derived products may have a beneficial effect on neuropathic ulcer healing, although the evidence to date is insufficient to support the superiority of one product over another, and cost-effectiveness in many health care settings remains to be established.
In summary, despite the global burden of disease and the high costs to patients and health care economies alike, earlier evidence regarding many topical interventions promoted to improve wound healing was poor. The quality of current research is improving, however, and as a result, there are now several interventions that can be recommended with some degree of confidence for use to improve healing of neuropathic or neuroischemic DFUs when usual best-practice care alone has been insufficient to achieve complete wound healing.
THERAPIES FOR NEUROISCHEMIC DFUs
Recently, there has been an increasing realization that ulceration in ischemic feet is a more common form of DFU than ulceration in purely neuropathic feet (50). Ulceration in ischemic feet can be divided into pure ischemic ulcers, which occur in severely or critically ischemic feet, and neuroischemic ulcers, which develop in mild or moderately ischemic feet. Neuroischemic feet ulcerate in the presence of a lesser degree of ischemia because of coexisting neuropathy. However, both neuroischemic and ischemic ulcers are more challenging to heal than nonischemic neuropathic ulcers and are associated with a higher rate of amputation and mortality (51).
Until recently, the evidence for the treatment of DFUs was lacking and evidence for treating ischemic/neuroischemic DFUs was almost nonexistent because these ulcer types were not included in clinical trials. However, the past few years have seen a renaissance in diabetic foot care with the advent of well-designed clinical trials and associated cost-effectiveness analyses (52). Furthermore, moderately ischemic feet have been included in these trials, together with neuropathic nonischemic feet. Additionally, one trial, the Explorer study, was primarily devoted to the treatment of ulcers in neuroischemic feet (9).
The Explorer study was a double-blinded RCT investigating the effect of sucrose octasulfate dressing, also known as technology lipido-colloid with nano-oligosaccharide factor (TLC-NOSF) (Figure 1). This dressing is a polyester mesh impregnated with a TLC, which is a matrix containing NOSF (sucrose octasulfate potassium salt). In the Explorer study, the sucrose octasulfate dressing was shown to be beneficial in the treatment of noninfected, neuroischemic DFUs that were difficult to heal despite best-practice standard care. Neuroischemic feet were defined by the presence of both neuropathy and moderate ischemia. This diagnosis was determined by an ABI of ≤0.9 but a toe pressure ≥50 mmHg (or an ankle pressure ≥70 mmHg if toe pressure could not be measured). After the trial started, a protocol amendment specified that patients with an ABI >0.9 were also eligible provided they had a TBI ≤0.7 and toe pressure ≥50 mmHg. This amendment took account of the artifactually high ABI values resulting from medial arterial calcification.

FIGURE 1
Design of the Explorer study, a double-blinded, stratified RCT conducted in two parallel groups. D, day; M, month; NOSF, nano-oligosaccharide factor; W, week.
In total, 126 participants were randomized to the sucrose octasulfate dressing and 114 to the control dressing, with both groups having excellent standard care (Figure 1). After 20 weeks of treatment, the proportion of patients whose DFUs healed was significantly greater in the sucrose octasulfate dressing group, at 60 patients (48%) compared to 34 patients (30%) in the control dressing group (95% CI 5–30) yielding an adjusted OR of 2.60 (95% CI 1.43–4.73, P = 0.002). There was also a significantly shorter healing time of 120 days (95% CI 110–129) as estimated from the Kaplan Meier analysis in participants from the sucrose octasulfate dressing group compared to 180 days (95% CI 163–198, P = 0.029) in the control group. Three cost-effectiveness models were derived from the results of the Explorer study with particular regard to the French (53), U.K. (45), and German perspectives (54). The analyses demonstrated that sucrose octasulfate is a cost-effective treatment compared to a neutral dressing and generates cost savings.
In a post hoc analysis that categorized patients according to quartiles of ulcer duration (0–2, 3–5, 6–11, or >11 months), ulcer healing rates decreased as the duration of ulceration at baseline increased (from 57% in ulcers presenting in ≤2 months to 19% in ulcers presenting at >11 months) (55). Regardless of ulcer duration quartile, higher healing rates were reported in ulcers treated with sucrose octasulfate than in those in the control group. Regarding different locations of DFUs, outcomes were always in favor of the sucrose octasulfate treatment, with healing rates ranging between 43 and 61% within the sucrose octasulfate group compared to 25–40% in the control group.
Delayed healing of neuroischemic DFUs has been related to excess MMPs, which can impair the extracellular matrix and destroy growth factors. The potassium salt of sucrose octasulfate inhibits MMPs and interacts with and restores the biological functions of growth factors (56). Furthermore, it stimulates angiogenesis through the migration and proliferation of endothelial cells. Evidence that sucrose octasulfate improves perfusion came in a further study of sucrose octasulfate dressing to treat neuroischemic ulcers (57). Eleven patients with neuroischemic ulcers were included in a prospective pilot study between July 2019 and March 2020. TcPO2 values were assessed at day 0 and monthly until wound healing was achieved. TcPO2 values increased significantly between day 0 (29.45 ± 7.38 mmHg) and ulcer healing (46.54 ± 11.45 mmHg, P = 0.016)
Although the Explorer study was devoted to neuroischemic ulcers, a recent trend in the diabetic foot care renaissance has been the inclusion in trials of some ischemic feet together with neuropathic feet, but these trials have not been designed to examine outcomes in ischemic feet alone. However, a trial of the multilayered patches comprising autologous leucocytes, platelets, and fibrin that was described earlier in this monograph (8), in addition to reporting overall outcomes, also noted outcomes of ulcers in patients with ischemic feet, as defined by an ABI <1.0. In patients with an ABI of 0.5–0.79, 5/14 (35.7%) healed in the group receiving the multilayered patch compared to 2/16 (12.5%) in the control group. In patients with an ABI of 0.8–0.99, 8/30 (26.7%) healed in the multilayered patch group compared to 6/23 (26.0%) in the control group.
Unhealed DFUs are susceptible to infection and are a prelude to 84% of lower-extremity amputations (58). The aim should be to heal these ulcers as quickly as possible to avoid the catastrophic loss of a leg to infection. In diabetic foot clinics, there has been a paradigm shift away from a focus on ulcers in neuropathic feet and toward ulcers in neuroischemic feet, which occur more frequently. There is now good evidence to support the successful treatment of neuroischemic ulcers with sucrose octasulfate in addition to best-practice standard care (59).
NEGATIVE PRESSURE WOUND THERAPY
NPWT was introduced by Argenta and Morykwas in 1996 and has revolutionized wound care (60,61). It is now the preferred method of treating large and complex wounds in diverse care settings around the globe. “Negative pressure” is a misnomer, as pressure is a positive quantity, but many calculate the difference in pressure applied from atmospheric pressure and report it as a negative number. Others have referred to this form of treatment perhaps more accurately as vacuum-assisted closure or sub-atmospheric pressure therapy (62).
Much work has been done on the mechanism of action of NPWT, and it appears that, in both experimental diabetic animals and humans, it increases granulation tissue (62–65) through upregulation of the hypoxia-inducible factor 1α (HIF-1α)/VEGF pathway (66). Experimental studies in diabetic mice have shown a dramatic increase in the rate of granulation tissue formation and that blood vessels formed when subjected to NPWT are more normal and less ectatic than new vessels in wounds treated with an occlusive dressing (65–67).
NPWT therapy systems are quite variable (68). A basic schematic of an NPWT system is depicted in Figure 2, including components that may vary depending on manufacturer and clinical setting. It is important to note the details of these components when comparing studies using different NPWT systems.
DFUs are diverse, occurring throughout the foot with depths sometimes going to the bone. People who develop DFUs are typically older and have type 2 diabetes, often with obesity and several other comorbid conditions (69). To make clinical decisions, clinicians must rely on the literature, with the highest level of evidence derived from well-designed, prospective RCTs (64). However, there have been only a few good RCTs for the treatment of DFUs (70–72).
In designing an RCT, investigators must select a group of patients whose ulcers do not heal completely with conventional treatment and include enough patients in each arm of the study to show a difference between the treatment arm and a standard care comparison group. Researchers usually exclude patients with severe cardiac, respiratory, or renal diseases that would put them at high risk of complications, unrelated to the treatment being studied, during the trial. Once a trial is published, many clinicians extrapolate its results to excluded patient groups, often not realizing that the efficacy of treatment has not been proven in these populations.
In one well-designed RCT, Armstrong et al. (70) compared NPWT to standard moist wound care in 162 people with diabetes who had partial foot amputations up to the transmetatarsal level. They found a healing rate at 16 weeks of 56% compared to 39% in the standard care group. When Armstrong et al. (71) reanalyzed these data in 2007, they found NPWT to be superior in both acute and chronic wounds. In another high-quality RCT, Blume et al., (72) compared NPWT to advanced moist wound therapy in 342 patients with Wagner grade 2 or 3 ulcers and found a healing rate at 16 weeks of 43.2 vs. 28.9%.
Although RCTs are the gold-standard for medical evidence, many prospective RCTs have still been proven wrong after publication. There are many reasons why this can occur, but one common criticism is that a study included too few patients, rendering it prone to statistical anomalies.
While most pre-clinical studies of NPWT have shown that it works primarily by increasing granulation tissue (62), most RCTs have measured the rate of complete wound closure as a primary endpoint (73). Because many clinicians use NPWT as one of several treatments to heal a wound, complete wound closure may be an imprecise metric to assess the effectiveness of NPWT.
Because NPWT has been available for 25 years and is commonly used in clinical practice, many clinicians feel that conducting a prospective RCT at this late date would be unethical, as it would deny therapy (which they already consider to be effective) to some patients. However, in some areas of the world where NPWT has not yet been established, some recent prospective RCTs have been completed (74).
The use of NPWT in DFUs has been studied with mixed results. In a Cochrane review of NPWT in patients with diabetes, Liu et al. (75) focused on diabetic foot infections treated with NPWT compared to conventional dressings in eight studies involving 640 participants and were able to pool data from five of the studies (486 participants). They concluded that there is low-certainty evidence that NPWT may increase the proportion of wounds healed and reduce the time to healing compared to conventional dressings.
A systematic review and meta-analysis performed by Liu et al. (76) also compared NPWT to conventional dressings. This analysis of data from 11 RCTs involving 1,044 patients found that NPWT was 1.48 times more likely than conventional dressings to heal wounds, with a decreased time to closure (by 8 days) and a reduced risk of amputation (relative risk 0.31).
Three RCTs compared conventional NPWT to NPWT with saline instillation. Lavery et al. (77) showed no difference in 150 patients, whereas Giri et al. (78) reported decreased bacterial burden and decreased wound size in 48 patients. Kim et al. (79) found no differences in their primary endpoints but did show a 3.1-fold decrease in the need for readmission of patients treated with saline instillation with NPWT compared to NPWT alone.
In summary, NPWT has resulted in a paradigm shift in the way complex DFUs are treated. There is good evidence to suggest that this form of therapy increases granulation tissue, and prospective RCTs have shown that it speeds wound healing. The supporting literature has been criticized for comprising low-certainty evidence from trials with risk of bias and imprecision. Additional clinical RCTs comparing NPWT to standard wound dressings may be difficult to perform. However, evidence for additional improvement using saline instillation with NPWT is mixed and warrants further study.
Table 3 lists the therapeutic technologies for DFU treatment described above and summarizes their indications, supporting evidence, and relative costs.
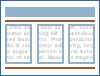
TABLE 3
Indications, Relative Cost, Supporting Evidence, and Possible Future Directions of Commonly Used Therapeutic Technologies to Treat DFUs
Looking Ahead: Therapeutic Approaches in the Research and Development Pipeline
CHALLENGES OF DEVELOPING THERAPEUTICS FOR DFUs
As an organ, the skin is readily accessible and thus uniquely suited to routine visual assessment and minimally invasive manipulation. This is advantageous when the skin incurs injury, permitting rapid diagnostic assessment and simple procedural interventions such as debridement. Moreover, external behavior modifications such as pressure off-loading can more directly modulate skin injury compared to injuries involving other organ systems. Such procedures and modifications can be and are routinely done as best-practice standard care, but an unintended consequence is that these opportunities create an additional layer of variance that complicates evaluation of new therapeutics.
For example, a multicenter clinical trial of any biologic dressing or treatment that seeks to assess the rate of wound closure is inherently likely to find variable results, in part because of both the thoroughness of debridement, which varies even for the same physician treating different patients, and variable patient compliance with off-loading instructions. Compared to trials of cancer therapies, in which tumor aggressiveness can be partially determined through regression based on pre- and post-treatment metrics, the variance in wound healing studies creates additional “noise” that undermines the ability to statistically power large-scale trials. As a result, clinical trials are either under-powered because traditional power analyses are used that do not take this variance into account or abandoned as unfeasible based on more accurate power analyses that recommend huge treatment groups to obtain meaningful results. Recent examples of failed phase 2 and phase 3 wound healing trials include studies of topical application of repifermin (recombinant human keratinocyte growth factor-2) for the treatment of venous leg ulcers and human platelet-derived growth factor-BB for chronic pressure ulcers. In both cases, investigators were unable to establish significant changes in the time to complete wound closure, which is the only primary endpoint the U.S. Food and Drug Administration (FDA) would accept for these trials (80). Thus, although wound healing has been a large focus for the pharmaceutical industry, little real progress has been made in this area.
CONTRARIAN STRATEGIES BASED ON THE CURRENT REGULATORY LANDSCAPE
Given the inherent challenges associated with wound healing studies, investigators seeking FDA approval for treatments for DFUs have begun seeking alternative approaches in place of the traditional clinical trial regulatory pathway. In 2016, the U.S. Congress enacted the 21st Century Cures Act to streamline the federal drug approval process (81). A key aspect of this legislation was the explicit engagement by the FDA with both real-world evidence (RWE) and real-world data (RWD) in the drug approval process, including the use of clinical efficacy and safety data from previous trials for the purpose of drug approval for alternative indications. A consequence of this policy, whether intentional or not, is an incentive for pharmaceutical companies to first trial their nascent drugs against “orphan” conditions (defined as those that affect <200,000 people in the United States), for which drug development is incentivized with tax breaks and prolonged exclusivity rights) to obtain the most fast-tracked approval possible, and then to use data from those trials as RWE to promote approval for the same drugs to treat a broader indication (82). This approach is particularly appealing in the context of DFU healing, given that the broad pathology associated with these lesions can be easily abstracted to orphan indications for multiple skin conditions.
POTENTIAL TARGETS
One example of the orphan drug strategy has been the development of the small molecule deferoxamine (DFO), an iron chelator traditionally used to treat hemochromatosis (alternatively referred to as “bronze diabetes”). Diabetic wound healing is associated with significant impairment in new blood vessel growth and elevated oxidative stress, and DFO has been shown to promote neovascularization during tissue repair through stabilization of the master hypoxia regulator HIF-1α (83). Investigators at Stanford University recently partnered with the University of Alabama, Birmingham, to trial DFO (delivered through a proprietary hydrogel patch) for the treatment of an orphan indication ascribed to skin ulcers in patients with sickle cell anemia (84), which is characterized by decreased peripheral oxygenation and impaired healing of skin lesions. In seeking evidence to support an orphan drug indication, it is possible to conduct a smaller, less expensive clinical trial and thereby obtain RWE and RWD that can then be used to support approval of DFO drug delivery devices for the treatment of similar (non-orphan) conditions, including DFUs. These studies will also pave the way for support of similar hypoxia rescue agents such as dimethyloxalylglycine and HIF-1α itself.
In addition to targeting hypoxia and impaired blood vessel growth, strategies for diabetic wound healing have also focused on the aberrant fibrotic response associated with diabetic skin injury that results in dysfunctional healing. After tissue repair, wound scars never fully return to their pre-injury state, in part because of hyperproliferation of fibroblast cells and their over-secretion of collagen and other extracellular membrane proteins. This hyperfibrotic response leads to both delayed healing and reduced tissue strength in the resulting scar, increasing the risk of recidivism (85). Strategies aimed at attenuating this problem have focused on the role of mechanotransduction (i.e., the sensation of mechanical force by resident tissue fibroblasts). Both direct mechanical off-loading and pharmaceutical abrogation of mechanotransduction signaling have shown early promise as methods to limit the hyperfibrotic response and promote true tissue regeneration (86,87).
EVOLUTION OF EVIDENCE-BASED TREATMENT ALGORITHMS
In addition to new therapeutic products in development, another avenue toward increasing treatment efficacy for DFUs has been to optimize the application of existing therapeutic modalities. This effort has been embodied in treatment heuristics, wherein providers are given a decision tree from which to determine optimal interventions based on observed clinical situations. This approach has become increasingly popular in large health care systems such as Kaiser Permanente and Banner Health, as well as large, national wound center networks such as Healogics (88). The motivation for this strategy is that aggregate RWE collected through nationwide data-mining efforts can be superior to the clinical experiences of individual providers in shaping decision-making. Such approaches are appealing, but often are not grounded in or validated through publicly available study data, and the ADA has yet to endorse a single wound healing heuristic. It is likely that nationwide consortiums would be required to gather the RWD needed to develop a comprehensive treatment algorithm. Recently, the National Institutes of Health has pioneered a Diabetic Foot Consortium to sponsor collaboration among academic hospitals (89). Early trials are still in development, but this initiative reflects the promise of collaborative research to gather the necessary wound healing data across time and space to power the RWD needed to inform decision guidance.
BIG DATA DECISION SUPPORT TO FLAG HIGH-RISK WOUNDS
Since the passage in the United States of the Affordable Care Act and the resulting widescale implementation of electronic health record systems, it has become increasingly clear that traditional approaches to the analysis of RWD such as logistic regression and multivariate mixed effects modeling are insufficient to handle the rapidly expanding number of measured clinical variables. More sophisticated machine learning techniques such as neural networks, support vector machines, and deep learning approaches have already been adopted in electrocardiogram assessment and radiographic image detection, in which data are less modular. Unlike traditional studies that compare outcomes of interest against pre-determined parameters expected to influence those outcomes, artificial intelligence–based approaches are capable of taking unbiased surveys of all available data parameters with the goal of classifying one or more outcome sets. This approach has proven successful most notably in the field of diagnostic radiology, in which automated lesion detection now serves as a standard-of-care tool for decision support at most large medical centers.
Similar efforts applied to wound classification have yet to gain traction, in part because of the challenges of incorporating direct wound assessment (90). However, as image processing becomes faster and less expensive, decision support based on raw wound photos should become more achievable. Furthermore, the increased adoption of wearable, so-called “smart” devices in the United States, discussed in more detail below, is likely to provide vast quantities of new data to assess outcomes using time course measurements. Early efforts are already underway to translate wearable wound bandages into systems for the early detection of problematic wounds, with the prospect of direct therapeutic intervention similar to that achieved with automated insulin pumps. Such personalized treatment strategies may exemplify the next generation of diabetic wound care in the coming decades.
Keeping the Ulcer Healed: Patients' Views on Digital Technology in the Prevention of Ulcer Recurrence
Despite substantial advances in DFU management, ulcer recurrence rates remain high, ranging from 40% within 1 year to 65% within 5 years (91). The reasons for DFU recurrence are believed to be both biological and behavioral (92). Because people at high DFU risk have no symptoms to prompt them to check their feet, psycho-educational interventions have traditionally focused on behavioral modifications designed to serve as substitute self-care cues in the absence of foot sensation and minimize the impact of neuropathic risk factors. These behavioral changes include the adoption of preventive foot self-care actions (e.g., daily foot checks) and avoidance of behaviors that, although appropriate for people with intact sensation in their feet, can potentially damage the feet of people affected by neuropathy (e.g., walking barefoot). Among the commonly examined psychological determinants of foot self-care are patients' cognitive and emotional representations of DFU risk (93–95), depression (96–98), and cognitive function (99), with the strongest evidence to date supporting a link between patients' interpretation of their DFU risk, associated emotional responses, and preventive foot self-care (100).
However, mounting evidence indicates that commonly advocated behavioral advice may not be effective enough to prevent DFU recurrence (98,101,102). Several reports have shown that depression and nonadherence to foot self-care predict first but not recurrent DFUs (96,98), findings that were recently supported by a systematic review (103). The observation that basic foot self-care behavioral strategies are ineffective for secondary DFU prevention was also supported by several trials demonstrating that, although participants in enhanced foot care education groups reported improved foot self-care, no significant differences in DFU recurrence were observed between the control and the intervention groups (101), as participants had biological DFU risk factors that are beyond control by such interventions (102).
To augment current preventive foot self-care behaviors, wearable technologies are being developed that can continuously monitor DFU risk factors and provide real-time alerts to people at high DFU risk, thereby prompting them to undertake protective action (104). Digitally connected, or “smart,” flexible sensors implanted in insoles or socks connect with mobile apps to allow monitoring and remote visualize of in-shoe plantar pressure and temperature. This strategy not only represents a paradigm shift in DFU risk screening and monitoring, but also, crucially, transforms foot-care education. The adage that “a picture is worth 1,000 words” is particularly relevant to this patient population, for whom symptoms and signs cannot be relied on when conveying messages about DFU risk. As a result, people often have poor comprehension of the potential for serious complications, especially with regard to their intrinsic DFU risk from factors such as foot deformities or elevated foot pressures, which, in turn, leads to a lack of effective foot self-care (93). By allowing people to visualize their personal DFU risk, digital technologies are likely to enhance patients' active involvement in DFU prevention.
Although promising, digital technologies create additional layers of complexity to preventive foot self-care for people at high DFU risk. These complexities include differing levels of familiarity with and dependence on technology and conditions of functionality such as other coexisting diabetes complications and reduced mobility, as well as variable need for support from health care providers and family members (105). Yet, there is a dearth of research examining determinants of patient acceptance of digital technology in DFU prevention. Several systematic reviews that evaluated telemedicine in diabetic foot disease either focused on the effectiveness of the devices or evaluated users' experience in the management of active DFUs rather than prevention (106–108).
A recent systematic review (109) of patient and provider perspectives on smart wearable technology in DFU prevention identified only five publications (110–114) of low to moderate methodological quality. Two studies used a quantitative/ questionnaire study design and focused on the patient perspective (110,111), whereas three studies included a mixed, questionnaire/interview design and explored patient and/or podia-trist perspectives (112–114). Four studies focused on a smart insole system to measure plantar foot pressures (110,112–114), whereas one included a smart sock device for monitoring plantar foot temperatures (111). Only one group of researchers, using the Unified Theory of Acceptance and Use of Technology (UTAUT)-based questionnaire (115), explicitly addressed the psychological factors influencing patient and podiatrist behavioral intention to adopt a smart insole device (112–114). These researchers identified important differences between patients and podiatrists with regard to factors determining their behavioral intention to adopt a smart insole. Although positive attitudes to digital technology and the belief that one could develop the skills to adopt a smart insole (self-efficacy) were key in activating patients (112), performance expectancy or the belief that a smart insole is effective in mitigating DFU risk was the single most important factor motivating podiatrists to use smart insoles in their clinical practice (113). Qualitative analyses revealed that participating podiatrists believed that the insole would increase patient engagement and self-efficacy. However, concerns were raised about cost, footwear issues, and the device's utility for elderly and remote populations.
The same research group recently evaluated the feasibility of podiatrist-led health coaching to facilitate adoption of a smart shoe insole in people at high DFU risk (114). The 4-week intervention assessed participants' intention to adopt smart insoles and actual insole usage. Using health coaching techniques, podiatrists successfully facilitated the adoption of a smart insole by study participants, as evidenced by insole wear time that exceeded that reported in previous studies using a similar device but without health coaching (110). However, there was a significant decline over time in responses to alert-based cues for foot pressure off-loading. This finding contrasts with a study by Najafi et al. (110) showing that individuals who received more alert-based cues for plantar pressure off-loading had reported better adherence than those in a group receiving fewer alerts.
It is possible that there is an upper threshold at which alerts would lead to declining adherence. Because the participants in the health coaching intervention (114) received, on average, twice as many alerts per hour than those participating in a study by Najafi et al. (110), they may have developed response fatigue, contributing to a lower percentage of successful responses. Furthermore, scores on the UTAUT-based questionnaire demonstrated significant post-trial reductions in attitude toward and behavioral intention to use the smart insole (114). Qualitative findings from this study demonstrated that behavioral intention to use digital technology may change as a function of a person's experience with the device. Study participants reported frustration when the device malfunctioned and felt that repeated alerts were becoming intrusive during daily activities. For participants who had not previously experienced a DFU, the feedback appeared random and significantly diminished their level of trust in the device. On the other hand, those with a previous DFU, although they believed the device provided accurate feedback, felt that there was little they could do to constantly mitigate high pressure areas on the bottoms of their feet. These observations resonate with earlier reports highlighting the importance of DFU experience in shaping patients' views about their DFU risk and foot self-care (93). Moreover, unsatisfactory patient experiences with the smart insole negatively affected podiatrists' intentions to adopt the device in practice (114). However, both, patients and podiatrists still saw value in real-time foot monitoring and indicated that refinement of the device would increase the likelihood of future adoption. Thus, the results of the focus group discussions clarified, at least to some extent, the somewhat unexpected trend toward a significant reduction in perceived usefulness of the device: it did not meet participants' initial expectations. There is, therefore, a need for early patient and provider involvement in the development and evaluation of digital technology devices if we are to initiate and sustain the desired foot self-care behavior change.
Additionally, findings from these reports highlight an important limitation of using behavioral intention as a proxy for technology acceptance: behavioral intention provides little insight into actual technology use. Furthermore, theoretical models such as the UTAUT are typically social cognition models and thus do not incorporate illness-specific domains such as patient perceptions of their DFU risk and specific emotional responses that were previously identified as important predictors of preventive foot self-care (93). It is therefore unlikely that people will adopt digital technology if they do not appreciate their DFU risk. Furthermore, digital technology adoption is a dynamic and interactive process. This fact necessitates that technology implementation be evaluated longitudinally so that emerging issues between people at high DFU risk and health care delivery can be identified and addressed. Nonetheless, even in its infancy, this rapidly evolving area of research provides valuable insights into patient and provider views of digital technology. Evaluation should continue into interventions to improve patients' acceptance and sustained use of digital technology and to reduce DFU recurrence.
Conclusions
Complications of the diabetic foot remain common, complex, and costly. This situation has been exacerbated by reduced access to care during the COVID-19 pandemic (116–119). However, as with any existential tragedy, positive pressure toward innovation can emerge. In this case, we are enjoying an unprecedented surge in pragmatic outreach to and the use of digital technology in high-risk populations, for both monitoring and extending the number of ulcer-free days in remission (120–123).
Additionally, external pressure for intensive assessment of innovation has coincided with the refinement of existing technologies such as NPWT, as well as the development of novel technologies such as autologous leukocyte dressings and sodium octasulfate, which are now supported by data from well-designed RCTs (8,9). Furthermore, therapies that previously were considered less mainstream, such as TOT, have recently gained in popularity as a result of a more robust clinical evidence base from multiple RCTs and meta-analyses (37,38).
Finally, and perhaps most importantly, we are making strides in our understanding of the diabetic foot in remission. Our assessment of any new therapies should not only consider reduction in the time to ulcer healing, but also the impact of the therapy in reducing ulcer recurrence rates, which are, of course, represented by hospitalization and amputation rates post-healing. In this regard, the recent real-world publication of TOT experience in two U.S. Department of Veterans Affairs hospitals (35) reported reductions of 88 and 71% in hospitalizations and amputations, respectively, at 12 months in patients receiving TOT compared to those in the standard care group. With the understanding that ~40% of DFUs will recur on the same or contralateral limb by 1 year (rising to ~66% by 3.75–5 years) (124), maximizing ulcer-free, hospital-free, and activity-rich days for our patients becomes a more noble (and realistic) goal than preventing every single DFU recurrence (91,125). The concept of remote patient monitoring, once an exotic idea, is now incorporated more routinely (126,127). Efforts to use thermometry and other tools in the way we have collectively used glucometry are emerging. In other words, dosing activity by checking for inflammation of the foot, just as we might dose insulin by checking glucose levels, may soon become commonplace (128).
This ADA Clinical Compendium is the third in a series focusing on foot care for people with diabetes (1,2). Although published in the midst of a global pandemic, it is paradoxically the most optimistic installment yet. Focusing not just on treating and preventing communicable disease, but also on improving our approaches to noncommunicable diseases may be our collective therapeutic North Star. Mitigating acute and chronic disease that starts at the end of the body—the humble foot—is a worthwhile endeavor that may yield substantive rewards that will benefit our patients and society long after the pandemic subsides.
Acknowledgments
Editorial and project management services were provided by Debbie Kendall of Kendall Editorial in Richmond, VA.
Dualities of Interest
M.L. has received research funding from Reapplix. R.G.F. has received research funding from and is a consultant to Advanced Oxygen Therapies, Inc. F.L.G.'s employer received funding for her research time to conduct the LeucoPatch trial, which was funded by Reapplix. She has also received honoraria to speak at educational meetings sponsored by Urgo Medical and MiMedx. M.E.E. has received honoraria from Urgo Medical for consultancy, advisory board attendance, and lectures. D.P.O. has received research funding from KCI through a sponsored grant to Brigham and Women's Hospital. G.C.G. is the founder of and has equity in Theris Medical. No other potential conflicts of interest relevant to this publication were reported.
Author Contributions
A.J.M.B. and D.G.A. served as co-editors and, as such, co-wrote the introduction and conclusion and reviewed and edited the entire manuscript. M.L. wrote “History of Oxygen Therapy for the Treatment of DFUs.” R.G.F. wrote “Topical Oxygen Therapy.” F.L.G. wrote “Topical Therapies for Neuropathic DFUs.” M.E.E. wrote “Therapies for Neuroischemic DFUs.” D.P.O. and K.K. wrote “Negative Pressure Wound Therapy.” G.C.G. and M.J. wrote “Looking Ahead: Therapeutic Approaches in the Research and Development Pipeline.” L.V. wrote “Keeping the Ulcer Healed: Patients' Views on Digital Technology in the Prevention of Ulcer Recurrence.” A.J.M.B. and D.G.A. are the guarantors of this work.
ABBREVIATIONS
- ABI
Ankle-brachial index
- ADA
American Diabetes Association
- ATA
Absolute atmosphere
- CDO
Continuous delivery of oxygen
- COVID-19
Coronavirus disease 2019
- D
Day
- DFO
Deferoxamine
- DFU
Diabetic foot ulcer
- FDA
U.S. Food and Drug Administration
- HBOT
Hyperbaric oxygen therapy
- HIF-1α
Hypoxia-inducible factor 1α
- ITT
Intention-to-treat
- IWGDF
International Working Group of the Diabetic Foot
- M
Month
- MMP
Matrix metalloproteinase
- NOSF
Nano-oligosaccharide
- NPWT
Negative pressure wound therapy
- OR
Odds ratio
- PAD
Peripheral artery disease
- PDGF
Platelet-derived growth factor
- PO2 Partial pressure of oxygen RCT
Randomized controlled trial
- RWD
Real-world data
- RWE
Real-world evidence
- TBI
Toe-brachial index
- TcPO2 Transcutaneous oxygen pressure TLC
Technology lipido-colloid
- TOT
Topical oxygen therapy
- UT
University of Texas
- UTAUT
Unified Theory of Acceptance and Use of Technology
- VEGF
Vascular endothelial growth factor
- W
Week
References
- 1.
- Boulton AJM, Armstrong DG, Kirsner RS, et al Diagnosis and Management of Diabetic Foot Complications. Arlington., VA, American Diabetes Association, 2018 [PMC free article: PMC538977] [PubMed: 30958663]
- 2.
- Boulton AJM, Armstrong DG, Hardman MJ, et al Diagnosis and Management of Diabetic Foot Infections. Arlington. VA, American Diabetes Association, 2020 [PMC free article: PMC554227] [PubMed: 32105420]
- 3.
- International Diabetes Federation. IDF Diabetes Atlas. 10th ed. Brussels, Belgium, International Diabetes Federation, 2021
- 4.
- Holland D, Heald AH, Stedman M, et al Assessment of the effect of the COVID-19 pandemic on UK HbA1c testing: implications for diabetes management and diagnosis. J Clin Pathol. Online ahead of print on 13 December 2021 (doi: 10
/1136/jclinpath-2021-207776) [PubMed: 34645702] - 5.
- Löndahl M, Boulton AJM. Hyperbaric oxygen therapy in diabetic foot ulceration: useless or useful3 A battle. Diabet Metab Res Rev 2020;36(Suppl. 1):e3233 [PubMed: 31927787]
- 6.
- Niederauer MQ, Michalek JE, Liu Q, Papas KK, Lavery LA, Armstrong DG. Continuous diffusion of oxygen improves diabetic foot ulcer healing when compared with a placebo control: a randomised, double-blind, multicentre study. J Wound Care 2018;27(Suppl. 9):S30–S45 [PubMed: 30207844]
- 7.
- Frykberg RG, Franks PJ, Edmonds M, et al; TWO2 Study Group. A multinational, multicenter, randomized, double-blinded, placebo-controlled trial to evaluate the efficacy of cyclical topical wound oxygen (TWO2) therapy in the treatment of chronic diabetic foot ulcers: the TWO2 Study. Diabetes Care 2020;43:616–624 [PubMed: 31619393]
- 8.
- Game F, Jeffcoate W, Tarnow L, et al; LeucoPatch II Trial Team. LeucoPatch system for the management of hard-to-heal diabetic foot ulcers in the UK, Denmark, and Sweden: an observer-masked, randomised controlled trial. Lancet Diabetes Endocrinol 2018;6:870–878 [PubMed: 30243803]
- 9.
- Edmonds M, Lázaro-Martínez JL, Alfayate-Garcia JM, et al Sucrose octasulfate dressing versus control dressing in patients with neuroischaemic diabetic foot ulcers (Explorer): an international, multicentre, double-blind, randomised, controlled trial. Lancet Diabetes Endocrinol 2018;6:186–196 [PubMed: 29275068]
- 10.
- Priestley J. Experiments and Observations on Different Kinds of Air. Vol. 2. London, England, Johnson, 1776
- 11.
- Lavoisier A. Brief on general combustion. Mémoire de L'Académie Royale 1777, p.592 [in French]
- 12.
- Grainge C. Breath of life: the evolution of oxygen therapy. J R Soc Med 2004;97:489–493 [PMC free article: PMC1079621] [PubMed: 15459264]
- 13.
- Birch SB. Some remarks on the exhibition of oxygen as a therapeutic, in connexion with a new, agreeable, and easy form of administration by the stomach. Lancet 1869;93:492–493
- 14.
- Osler W. The Principles and Practice of Medicine. 3rd ed. New York, Appleton & Co, 1898
- 15.
- Haldane J.S. The therapeutic administration of oxygen. BMJ 1917;1: 181–183 [PMC free article: PMC2348015] [PubMed: 20768466]
- 16.
- Fischer BH. Topical hyperbaric oxygen treatment of pressure sores and skin ulcers. Lancet 1969;23: 405–409 [PubMed: 4184490]
- 17.
- Brummelkamp WH, Boerema I, Hoogendyk L. Treatment of clostridial infections with hyperbaric oxygen drenching: a report on 26 cases. Lancet 1963;1:235–238 [PubMed: 14016240]
- 18.
- Hart G, Strauss M. Response of ischemic ulcerative conditions to OHP. In Sixth International Congress on Hyperbaric Medicine. Aberdeen, U.K., Aberdeen University Press, 1979, p. 312–314
- 19.
- Abidia A, Laden G, Kuhan G, et al The role of hyperbaric oxygen therapy in ischaemic diabetic lower extremity ulcers: a double-blind randomised-controlled trial. Eur J Vasc Endovasc Surg 2003;25:513–518 [PubMed: 12787692]
- 20.
- Duzgun AP, Satir HZ, Ozozan O, Saylam B, Kulah B, Coskun F. Effect of hyperbaric oxygen therapy on healing of diabetic foot ulcers. J Foot Ankle Surg 2008;47:515–519 [PubMed: 19239860]
- 21.
- Löndahl M, Katzman P, Nilsson A, Hammarlund C. Hyperbaric oxygen therapy facilitates healing of chronic foot ulcers in patients with diabetes. Diabetes Care 2010;33:998–1003 [PMC free article: PMC2858204] [PubMed: 20427683]
- 22.
- Faglia E, Favales F, Aldeghi A, et al Adjunctive systemic hyperbaric oxygen therapy in treatment of severe prevalently ischemic diabetic foot ulcer: a randomized study. Diabetes Care 1996;19:1338–1343 [PubMed: 8941460]
- 23.
- Santema KTB, Stoekenbroek RM, Koelemay MJW, et al Hyperbaric oxygen therapy in the treatment of ischemic lower-extremity ulcers in patients with diabetes: results of the DAMO2CLES multicenter randomized clinical trial. Diabetes Care 2018;41:112–119 [PubMed: 29074815]
- 24.
- Leslie CA, Sapico FL, Ginunas VJ, Adkins RH. Randomized controlled trial of topical hyperbaric oxygen for treatment of diabetic foot ulcers. Diabetes Care 1988;11:111–115 [PubMed: 3289861]
- 25.
- Kalliainen LK, Gordillo GM, Schlanger R, Sen CK. Topical oxygen as an adjunct to wound healing: a clinical case series. Pathophysiology 2003;9:81–87 [PubMed: 14567939]
- 26.
- Gordillo GM, Sen CK. Evidence-based recommendations for the use of topical oxygen therapy in the treatment of lower extremity wounds. Int J Low Extrem Wounds 2009;8:105–111 [PMC free article: PMC3814219] [PubMed: 19443899]
- 27.
- Blackman E, Moore C, Hyatt J, Railton R, Frye C. Topical wound oxygen therapy in the treatment of severe diabetic foot ulcers: a prospective controlled study. Ostomy Wound Manage 2010;56:24–31 [PubMed: 20567051]
- 28.
- Kemp DG, Hermans M. An evaluation of the efficacy of transdermal continuous oxygen therapy in patients with recalcitrant diabetic foot ulcer. Journal of Diabetic Foot Complications 2011;3:6–12
- 29.
- Tawfick WA, Sultan S. Technical and clinical outcome of topical wound oxygen in comparison to conventional compression dressings in the management of refractory nonhealing venous ulcers. Vasc Endovascular Surg 2013;47:30–37 [PubMed: 23223182]
- 30.
- Fries RB, Wallace WA, Roy S, et al Dermal excisional wound healing in pigs following treatment with topically applied pure oxygen. Mutat Res 2005;579:172–181 [PubMed: 16105672]
- 31.
- Driver VR, Reyzelman A, Kawalec J, French M. A prospective, randomized, blinded, controlled trial comparing transdermal continuous oxygen delivery to moist wound therapy for the treatment of diabetic foot ulcers. Ostomy Wound Manage 2017;63:12–28 [PubMed: 28448266]
- 32.
- Frykberg RG. Topical wound oxygen therapy in the treatment of chronic diabetic foot ulcers. Medicina (Kaunas) 2021;57:917 [PMC free article: PMC8467973] [PubMed: 34577840]
- 33.
- Serena TE, Bullock NM, Cole W, et al Topical oxygen therapy in the treatment of diabetic foot ulcers: a multicentre, open, randomised controlled clinical trial. J Wound Care 2021;30(Suppl. 5):S7–S14 [PubMed: 33979229]
- 34.
- Gottrup F, Dissemond J, Baines C, et al Use of oxygen therapies in wound healing. J Wound Care 2017;26(Suppl. 5):S1–S43 [PubMed: 28475468]
- 35.
- Yellin JI, Gaebler JA, Zhou FF, et al Reduced hospitalizations and amputations in patients with diabetic foot ulcers treated with cyclical pressurized topical wound oxygen therapy: real-world outcomes. Adv Wound Care (New Rochelle). Online ahead of print on 6 December 2021 (doi:10
.1089/wound.2021.0118) [PMC free article: PMC9527050] [PubMed: 34714167] - 36.
- Nataraj M, Maiya AG, Karkada G, et al Application of topical oxygen therapy in healing dynamics of diabetic foot ulcers: a systematic review. Rev Diabet Stud 2019;15:74–82 [PMC free article: PMC6946089] [PubMed: 31904759]
- 37.
- Thanigaimani S, Singh T, Golledge J. Topical oxygen therapy for diabetes-related foot ulcers: a systematic review and meta-analysis. Diabet Med 2021:38:e14585 [PubMed: 33871095]
- 38.
- Connaghan F, Avsar P, Patton D, O'Connor T, Moore Z. Impact of topical oxygen therapy on diabetic foot ulcer healing rates: a systematic review. J Wound Care 2021;30:823–829 [PubMed: 34644137]
- 39.
- Serena T, Andersen C, Cole W, Garoufalis M, Frykberg R. Guidelines for the use of topical oxygen therapy in the treatment of hard-to-heal wounds based on a Delphi consensus. J Wound Care 2021;30(Suppl. 9):S30–S34 [PubMed: 34554851]
- 40.
- Abbott CA, Carrington AL, Ashe H, et al; North-West Diabetes Foot Care Study. North-West Diabetes Foot Care Study: incidence of, and risk factors for, new diabetic foot ulceration in a community-based patient cohort. Diabet Med 2002;19:377–384 [PubMed: 12027925]
- 41.
- National Health Service. National Diabetes Foot Care Audit: Fourth Annual Report. Available from https://www
.hqip.org .uk/resource/national-diabetes-foot-care-audit-fourth-annual-report/# .Yasq3fHP3bI. Accessed 30 November 2021 - 42.
- Schaper NC, van Netten JJ, Apelqvist J, Bus SA, Hinchliffe RJ, Lipsky BA; IWGDF Editorial Board. Practical guidelines on the prevention and management of diabetic foot disease (IWGDF 2019 update). Diabetes Metab Res Rev 2020;36(Suppl. 1):e3266 [PubMed: 32176447]
- 43.
- Jeffcoate WJ, Bus SA, Game FL, Hinchliffe RJ, Price PE, Schaper NC; International Working Group on the Diabetic Foot and the European Wound Management Association. Reporting standards of studies and papers on the prevention and management of foot ulcers in diabetes: required details and markers of good quality. Lancet Diabetes Endocrinol 2016;4:781–788 [PubMed: 27177729]
- 44.
- Vas P, Rayman G, Dhatariya K, et al Effectiveness of interventions to enhance healing of chronic foot ulcers in diabetes: a systematic review. Diabetes Metab Res Rev 2020;36(Suppl. 1):e3284 [PubMed: 32176446]
- 45.
- National Institute for Health and Care Excellence. UrgoStart for treating diabetic foot ulcers and leg ulcers. Available from https://www
.nice.org .uk/guidance/mtg42/resources /urgostart-for-treating-diabetic-foot-ulcers-and-leg-ulcers-pdf-64372052418757. Accessed 1 December 2021 - 46.
- Rayman G, Vas P, Dhatariya K, et al; International Working Group on the Diabetic Foot (IWGDF). Guidelines on use of interventions to enhance healing of chronic foot ulcers in diabetes (IWGDF 2019 update). Diabetes Metab Res Rev 2020;36(Suppl. 1):e3283 [PubMed: 32176450]
- 47.
- Lavery LA, Fulmer J, Shebetka KA, et al; Grafix Diabetic Foot Ulcer Study Group. The efficacy and safety of Grafix for the treatment of chronic diabetic foot ulcers: results of a multi-centre, controlled, randomised, blinded, clinical trial. Int Wound J 2014;11:554–560 [PMC free article: PMC7951030] [PubMed: 25048468]
- 48.
- Tettelbach W, Cazzell S, Sigal F, et al A multicentre prospective randomised controlled comparative parallel study of dehydrated human umbilical cord (EpiCord) allograft for the treatment of diabetic foot ulcers. Int Wound J 2019;16:122–130 [PMC free article: PMC7380046] [PubMed: 30246926]
- 49.
- Tettelbach W, Cazzell S, Reyzelman AM, Sigal F, Caporusso JM, Agnew PS. A confirmatory study on the efficacy of dehydrated human amnion/chorion membrane dHACM allograft in the management of diabetic foot ulcers: a prospective, multicentre, randomised, controlled study of 110 patients from 14 wound clinics. Int Wound J 2019;16:19–29 [PMC free article: PMC7379535] [PubMed: 30136445]
- 50.
- Ndip A, Jude EB. Emerging evidence for neuroischemic diabetic foot ulcers: model of care and how to adapt practice. Int J Low Extrem Wounds 2009;8:82–94 [PubMed: 19443897]
- 51.
- Armstrong DG, Cohen K, Courric S, Bharara M, Marston W. Diabetic foot ulcers and vascular insufficiency: our population has changed, but our methods have not. J Diabetes Sci Technol 2011;5:1591–1595 [PMC free article: PMC3262731] [PubMed: 22226282]
- 52.
- Edmonds M. A renaissance in diabetic foot care: new evidence-based treatments. Lancet Diabetes Endocrinol 2018;6:837–838 [PubMed: 30243804]
- 53.
- Maunoury F, Oury A, Fortin S, Thomassin L, Bohbot S; Explorer Study. Cost-effectiveness of TLC-NOSF dressings versus neutral dressings for the treatment of diabetic foot ulcers in France. PLoS One 2021;16:e0245652 [PMC free article: PMC7822547] [PubMed: 33481840]
- 54.
- Lobmann R, Augustin M, Lawall H, et al Cost-effectiveness of TLC-sucrose octasulfate versus control dressings in the treatment of diabetic foot ulcers. J Wound Care 2019;28:808–816 [PubMed: 31825772]
- 55.
- Lázaro-Martínez JL, Edmonds M, Rayman G, et al Optimal wound closure of diabetic foot ulcers with early initiation of TLC-NOSF treatment: post-hoc analysis of Explorer. J Wound Care 2019;28:358–367 [PubMed: 31166858]
- 56.
- Volkin DB, Verticelli AM, Marfia KE, Burke CJ, Mach H, Middaugh CR. Sucralfate and soluble sucrose octasulfate bind and stabilize acidic fibroblast growth factor. Biochim Biophys Acta 1993;1203:18–26 [PubMed: 7692970]
- 57.
- Lázaro-Martínez JL, García-Madrid M, García-Alamino JM, Bohbot S, García-Klepzig JL, García-Álvarez Y. Increasing transcutaneous oxygen pressure in patients with neuroischemic diabetic foot ulcers treated with a sucrose octasulfate dressing: a pilot study. Int J Low Extrem Wounds. Online ahead of print on 28 August 2020 (doi: 10
.1177/1534734620952244) [PubMed: 32856522] - 58.
- Pecoraro RE, Reiber GE, Burgess EM. Pathways to diabetic limb amputation: basis for prevention. Diabetes Care 1990;13:513–521 [PubMed: 2351029]
- 59.
- Game F. Treatment strategies for neuroischaemic diabetic foot ulcers. Lancet Diabetes Endocrinol 2018;6:159–160 [PubMed: 29275069]
- 60.
- Argenta LC, Morykwas MJ. Vacuum-assisted closure: a new method for wound control and treatment: clinical experience. Ann Plast Surg 1997;38: 563–576 [PubMed: 9188971]
- 61.
- Morykwas MJ, Argenta LC, Shelton-Brown EI, McGuirt W. Vacuum-assisted closure: a new method for wound control and treatment: animal studies and basic foundation. Ann Plast Surg 1997:38:553–562 [PubMed: 9188970]
- 62.
- Huang C, Leavitt T, Bayer LR, Orgill DP. Effect of negative pressure wound therapy on wound healing. Curr Probl Surg 2014 Jul;51:301–331 [PubMed: 24935079]
- 63.
- Lancerotto L, Bayer LR, Orgill DP. Mechanisms of action of microdeformational wound therapy. Semin Cell Dev Biol 2012;23:987–992 [PubMed: 23036531]
- 64.
- Orgill DP, Manders EK, Sumpio BE, et al The mechanisms of action of vacuum assisted closure: more to learn. Surgery 2009;146:40–51 [PubMed: 19541009]
- 65.
- Scherer SS, Pietramaggiori G, Mathews JC, Prsa MJ, Huang S, Orgill DP. The mechanism of action of the vacuum-assisted closure device. Plast Reconstr Surg 2008;122:786–797 [PubMed: 18766042]
- 66.
- Erba P, Ogawa R, Ackermann M, et al Angiogenesis in wounds treated by microdeformational wound therapy. Ann Surg 2011;253:402–409 [PMC free article: PMC3403722] [PubMed: 21217515]
- 67.
- Saxena V, Hwang C-W, Huang S, Eichbaum Q, Ingber D, Orgill DP. Vacuum-assisted closure: microdeformations of wounds and cell proliferation. Plast Reconstr Surg 2004;114:1086–1096 [PubMed: 15457017]
- 68.
- Schaum KD. A new Medicare part B wound care policy. Adv Skin Wound Care 2001;14:238–240 [PubMed: 11905971]
- 69.
- Alavi A, Sibbald RG, Mayer D, et al Diabetic foot ulcers: Part I. Pathophysiology and prevention. J Am Acad Dermatol 2014;70:1.e1–e18 [PubMed: 24355275]
- 70.
- Armstrong DG, Lavery LA; Diabetic Foot Study Consortium. Negative pressure wound therapy after partial diabetic foot amputation: a multicentre, randomised controlled trial. Lancet 2005;366:1704–1710 [PubMed: 16291063]
- 71.
- Armstrong DG, Lavery LA, Boulton AJM. Negative pressure wound therapy via vacuum-assisted closure following partial foot amputation: what is the role of wound chronicity? Int Wound J 2007:4;79–86 [PMC free article: PMC7951344] [PubMed: 17425550]
- 72.
- Blume PA, Walters J, Payne W, Ayala J, Lantis J. Comparison of negative pressure wound therapy using vacuum-assisted closure with advanced moist wound therapy in the treatment of diabetic foot ulcers: a multicenter randomized controlled trial. Diabetes Care 2008;31:631–636 [PubMed: 18162494]
- 73.
- Seidel D, Storck M, Lawall H, et al Negative pressure wound therapy compared with standard moist wound care on diabetic foot ulcers in real-life clinical practice: results of the German DiaFu-RCT. BMJ Open 2020;10:e026345 [PMC free article: PMC7202734] [PubMed: 32209619]
- 74.
- James SMD, Sureshkumar S, Elamurugan TP, Debasis N, Vijayakumar C, Palanivel C. Comparison of vacuum-assisted closure therapy and conventional dressing on wound healing in patients with diabetic foot ulcer: a randomized controlled trial. Niger J Surg 2019;25:14–20 [PMC free article: PMC6452767] [PubMed: 31007506]
- 75.
- Liu Z, Dumville JC, Hinchliffe RJ, et al Negative pressure wound therapy for treating foot wounds in people with diabetes mellitus. Cochrane Database Syst Rev 2018;10:CD010318 [PMC free article: PMC6517143] [PubMed: 30328611]
- 76.
- Liu S, He C-Z, Cai YT, et al Evaluation of negative-pressure wound therapy for patients with diabetic foot ulcers: systematic review and meta-analysis. Ther Clin Risk Manag 2017;13:533–544 [PMC free article: PMC5403129] [PubMed: 28458556]
- 77.
- Lavery LA, Davis KE, La Fontaine J, et al Does negative pressure wound therapy with irrigation improve clinical outcomes? A randomized clinical trial in patients with diabetic foot infections. Am J Surg 2020;220:1076–1082 [PubMed: 32139102]
- 78.
- Giri P, Krishnaraj B, Sistla SC, et al Does negative pressure wound therapy with saline instillation improve wound healing compared to conventional negative pressure wound therapy? A randomized controlled trial in patients with extremity ulcers. Ann Med Surg (Lond) 2020;61:73–80 [PMC free article: PMC7773677] [PubMed: 33408857]
- 79.
- Kim PJ, Lavery LA, Galiano RD, et al The impact of negative-pressure wound therapy with instillation on wounds requiring operative debridement. Int Wound J 2020;17:1194–1208 [PMC free article: PMC7540575] [PubMed: 32567234]
- 80.
- Darwin E, Tomic-Canic M. Healing chronic wounds: current challenges and potential solutions. Curr Dermatol Rep 2018;7:296–302 [PMC free article: PMC6585977] [PubMed: 31223516]
- 81.
- U.S. House of Representatives. Implementing the 21st Century Cures Act: An Update from FDA and NIH: Hearing Before the Subcommittee on Health of the Committee on Energy and Commerce, House of Representatives, One Hundred Fifteenth Congress, First Session, November 30, 2017. Washington, D.C. U.S. Government Publishing Office, 2019
- 82.
- Miller KL. Do investors value the FDA orphan drug designation? Orphanet J Rare Dis 2017;12:114 [PMC free article: PMC5477091] [PubMed: 28629392]
- 83.
- Thangarajah H, Yao D, Change EI, et al The molecular basis for impaired hypoxia-induced VEGF expression in diabetic tissues. Proc Natl Acad Sci U S A 2009;106:13505–13510 [PMC free article: PMC2726398] [PubMed: 19666581]
- 84.
- Cohen J. Challenges of developing and facilitating access to new sickle cell disease therapies: case of the deferoxamine patch. Forbes. 15 October 2020
- 85.
- Gurtner GC, Werner S, Barrandon Y, Longaker MT. Wound repair and regeneration. Nature 2008;453: 314–321 [PubMed: 18480812]
- 86.
- Lim AF, Weintraub J, Kaplan EN, et al The embrace device significantly decreases scarring following scar revision surgery in a randomized controlled trial. Plast Reconstr Surg 2014;133:398–405 [PMC free article: PMC4874339] [PubMed: 24105084]
- 87.
- Mascharak S, des Jardins-Park HE, Davitt MF, et al Preventing Engrailed-1 activation in fibroblasts yields wound regeneration without scarring. Science 2021;372:eaba2374 [PMC free article: PMC9008875] [PubMed: 33888614]
- 88.
- Fulkerson G. Presentation to referring physicians: wound care in the 21st century. Available from http://www
.kafp.org/wp-content /uploads/2021/08/008-HBOT.pdf. Accessed 14 January 2021 - 89.
- National Institutes of Health. First-ever research network tackles diabetic foot complications. Available from https://www
.nih.gov/news-events /news-releases /first-ever-research-network-tackles-diabetic-foot-complications. Accessed 14 January 2022 - 90.
- Jung K, Covington A, Sen CK, et al Rapid identification of slow healing wounds. Wound Repair Regen 2016;24:181–188 [PMC free article: PMC4820011] [PubMed: 26606167]
- 91.
- Armstrong DG, Boulton AJM, Bus SA. Diabetic foot ulcers and their recurrence. N Engl J Med 2017;376: 2367–2375 [PubMed: 28614678]
- 92.
- Jeffcoate WJ, Vileikyte L, Boyko EJ, Armstrong DG, Boulton AJM. Current challenges and opportunities in the prevention and management of diabetic foot ulcers. Diabetes Care 2018;41:645–652 [PubMed: 29559450]
- 93.
- Vileikyte L, Gonzalez JS, Leventhal H, et al Patient Interpretation of Neuropathy (PIN) questionnaire: an instrument for assessment of cognitive and emotional factors associated with foot self-care. Diabetes Care 2006;29:2617–2624 [PubMed: 17130194]
- 94.
- Perrin BM, Swerissen H, Payne CB, Skinner TC. Cognitive representations of peripheral neuropathy and self-reported foot-care behaviour of people at high risk of diabetes-related foot complications. Diabet Med 2014;31:102–106 [PubMed: 23869945]
- 95.
- Corbett C, Jolley J, Barson E, Wraight P, Perrin B, Fisher C. Cognition and understanding of neuropathy of inpatients admitted to a specialized tertiary diabetic foot unit with diabetes-related foot ulcers. Int J Low Extrem Wounds 2019;18:294–300 [PubMed: 31307246]
- 96.
- Williams LH, Rutter CM, Katon WJ, et al Depression and incident diabetic foot ulcers: a prospective cohort study. Am J Med 2010;123:748–754.e3 [PMC free article: PMC2913143] [PubMed: 20670730]
- 97.
- Iversen MM, Tell GS, Espehaug B, et al Is depression a risk factor for diabetic foot ulcers? 11-years follow-up of the Nord-Trøndelag Health Study (HUNT). J Diabetes Complications 2015;29:20–25 [PubMed: 25283486]
- 98.
- Gonzalez JS, Vileikyte L, Ulbrecht JS, et al Depression predicts first but not recurrent diabetic foot ulcers. Diabetologia 2010;53:2241–2248 [PubMed: 20556354]
- 99.
- Kloos C, Hagen F, Lindloh C, et al Cognitive function is not associated with recurrent foot ulcers in patients with diabetes and neuropathy. Diabetes Care 2009;32:894–896 [PMC free article: PMC2671092] [PubMed: 19244093]
- 100.
- Vileikyte L, Pouwer F, Gonzalez JS. Psychosocial research in the diabetic foot: are we making progress? Diabetes Metab Res Rev 2020; 36(Suppl. 1):e3257 [PubMed: 31850665]
- 101.
- Lincoln NB, Radford KA, Game FL, Jeffcoate WJ. Education for secondary prevention of foot ulcers in people with diabetes: a randomised controlled trial. Diabetologia 2008;51:1954–1961 [PubMed: 18758747]
- 102.
- Annersten Gershater M, Pilhammar E, Apelqvist J, Alm-Roijer C. Patient education for the prevention of diabetic foot ulcers. European Diabetes Nursing 2011:8:102–107b
- 103.
- Westby M, Norman G, Vedhara K, Game F, Cullum N. Psychosocial and behavioural prognostic factors for diabetic foot ulcer development and healing: a systematic review. Diabet Med 2020;37:1244–1255 [PubMed: 32315474]
- 104.
- Najafi B, Mishra R. Harnessing digital health technologies to remotely manage diabetic foot syndrome: a narrative review. Medicina (Kaunas) 2021;57:377 [PMC free article: PMC8069817] [PubMed: 33919683]
- 105.
- Boodoo C, Perry JA, Hunter PJ, et al Views of patients on using mHealth to monitor and prevent diabetic foot ulcers: qualitative study. JMIR Diabetes 2017;2:e22 [PMC free article: PMC6238864] [PubMed: 30291089]
- 106.
- Hazenberg CEVB, Aan de Stegge WB, Van Baal SG, Moll FL, Bus SA. Telehealth and telemedicine applications for the diabetic foot: a systematic review. Diabetes Metab Res Rev 2020;36:e3247 [PMC free article: PMC7079242] [PubMed: 31808288]
- 107.
- Foong HF, Kyaw BM, Upton Z, Car LT. Facilitators and barriers of using digital technology for the management of diabetic foot ulcers: a qualitative systematic review. Int Wound J 2020;17:1266–1281 [PMC free article: PMC7948580] [PubMed: 32390305]
- 108.
- Tchero H, Noubou L, Becsangele B, Mukisi-Mukaza M, Retali G-R, Rusch E. Telemedicine in diabetic foot care: a systematic literature review of interventions and meta-analysis of controlled trials. Int J Low Extrem Wounds 2017;16:274–283 [PubMed: 29168418]
- 109.
- Orlando G, Prior Y, Reeves ND, Vileikyte L. Patient and provider perspective of smart wearable technology in diabetic foot ulcer prevention: a systematic review. Medicina (Kaunas) 2021;57:1359 [PMC free article: PMC8707196] [PubMed: 34946304]
- 110.
- Najafi B, Ron E, Enriquez A, Marin I, Razjouyan J. Smarter sole survival: will neuropathic patients at high risk for ulceration use a smart insole-based foot protection system? J Diabetes Sci Technol 2017;11:702–713 [PMC free article: PMC5588829] [PubMed: 28627227]
- 111.
- Reyzelman AM, Koelewyn K, Murphy M, et al Continuous temperature-monitoring socks for home use in patients with diabetes: observational study. J Med Internet Res 2018;20:e12460 [PMC free article: PMC6315272] [PubMed: 30559091]
- 112.
- Macdonald EM, Perrin BM, Hyett N, Kingsley MIC. Factors influencing behavioural intention to use a smart shoe insole in regionally based adults with diabetes: a mixed methods study. J Foot Ankle Res 201912:29 [PMC free article: PMC6528213] [PubMed: 31139261]
- 113.
- Macdonald EM, Perrin BM, Kingsley MIC. Factors influencing Australian podiatrists' behavioural intentions to adopt a smart insole into clinical practice: a mixed methods study. J Foot Ankle Res 2020;13:28 [PMC free article: PMC7268265] [PubMed: 32487234]
- 114.
- Macdonald EM, Perrin BM, Cleeland L, Kingsley MIC. Podiatrist-delivered health coaching to facilitate the use of a smart insole to support foot health monitoring in people with diabetes-related peripheral neuropathy. Sensors (Basel) 2021;21:3984 [PMC free article: PMC8227881] [PubMed: 34207743]
- 115.
- Kohnke A, Cole ML, Bush R. Incorporating UTAUT predictors for understanding home care patients' and clinician's acceptance of healthcare telemedicine equipment. Journal of Technology Management & Innovation 2014;9:29–41
- 116.
- Shin L, Bowling FL, Armstrong DG, Boulton AJM. Saving the diabetic foot during the COVID-19 pandemic: a tale of two cities. Diabetes Care 2020;43:1704–1709 [PMC free article: PMC7372052] [PubMed: 32532755]
- 117.
- Du C, Li Y, Xie P, et al The amputation and mortality of inpatients with diabetic foot ulceration in the COVID-19 pandemic and postpandemic era: a machine learning study. Int Wound J. Online ahead of print 24 November 2021 (doi: 10
.1111/iwj.13723) [PMC free article: PMC9493239] [PubMed: 34818691] - 118.
- Armstrong DG. Managing the surge: delayed chronic wound care during COVID-19. Available from https://www
.ajmc.com /view/managing-the-surge-delayed-chronic-wound-care-during-covid-19. Accessed 22 September 2021 - 119.
- Armstrong DG, Rowe VL, D'Huyvetter K, Sherman RA. Telehealth-guided home-based maggot debridement therapy for chronic complex wounds: peri- and post-pandemic potential. Int Wound J 2020;17:1490–1495 [PMC free article: PMC7948734] [PubMed: 32558362]
- 120.
- Rogers LC, Armstrong DG, Capotorto J, et al Wound center without walls: the new model of providing care during the COVID-19 pandemic. Wounds 2020;32:178–185 [PMC free article: PMC8356413] [PubMed: 32335520]
- 121.
- Rogers LC, Lavery LA, Joseph WS, Armstrong DG. All feet on deck: the role of podiatry during the COVID-19 pandemic: preventing hospitalizations in an overburdened healthcare system, reducing amputation and death in people with diabetes. J Am Podiatr Med Assoc. Online ahead of print on 25 March 2020 (doi:10
.7547/20-051) [PubMed: 32208983] - 122.
- Swerdlow M, Shin L, D'Huyvetter K, Mack WJ, Armstrong DG. Initial clinical experience with a simple, home system for early detection and monitoring of diabetic foot ulcers: the foot selfie. J Diabetes Sci Technol. Online ahead of print on 31 October 2021 (doi: 10
.1177/19322968211053348) [PMC free article: PMC9846401] [PubMed: 34719973] - 123.
- de Mestral C, Gomez D, Wilton AS, et al A population-based analysis of diabetes-related care measures, foot complications, and amputation during the COVID-19 pandemic in Ontario, Canada. JAMA Netw Open 2022;5:e2142354 [PMC free article: PMC8733837] [PubMed: 34985514]
- 124.
- Petersen BJ, Rothenberg GM, Lakhani PJ, et al Ulcer metastasis? Anatomical locations of recurrence for patients in diabetic foot remission. J Foot Ankle Res 2020;13:1 [PMC free article: PMC6958592] [PubMed: 31956341]
- 125.
- Khan T, Armstrong DG. Ulcer-free, hospital-free and activity-rich days: three key metrics for the diabetic foot in remission. J Wound Care 2018;27(Suppl. 4):S3–S4 [PubMed: 29641340]
- 126.
- Armstrong DG. Subscription prescription: remote patient monitoring using smart shoes, socks and insoles. J Wound Care 2019;28(Suppl. 9):S3 [PubMed: 31509490]
- 127.
- Brooks E, Burns M, Ma R, Scholten HJ, Becker S. Remote diabetic foot temperature monitoring for early detection of diabetic foot ulcers: a cost-effectiveness analysis. Clinicoecon Outcomes Res 2021;13:873–881 [PMC free article: PMC8504713] [PubMed: 34675567]
- 128.
- Fernando ME, Woelfel SL, Perry D, et al Dosing activity and return to preulcer function in diabetes-related foot ulcer remission. J Am Podiatr Med Assoc 2021;111:Article 2 [PubMed: 33783527]
Suggested citation: Boulton AJM, Armstrong DG, Löndahl M, Frykberg RG, Game FL, Edmonds ME, Orgill DP, Kramer K, Gurtner GC, Januszyk M, Vileikyte L. New Evidence-Based Therapies for Complex Diabetic Foot Wounds. Arlington, Va., American Diabetes Association, 2022 (https://doi.org/10.2337/db2022-02) [PubMed: 35763580]
The opinions expressed are those of the authors and do not necessarily reflect those of the American Diabetes Association. The content was developed by the authors and does not represent the policy or position of the American Diabetes Association, any of its boards or committees, or any of its journals or their editors or editorial boards.
To request permission to reuse or reproduce any portion of this publication, please contact gro.setebaid@snoissimrep.
- History of Oxygen Therapy for the Treatment of DFUs
- New, Evidence-Based Therapies for DFUs
- Looking Ahead: Therapeutic Approaches in the Research and Development Pipeline
- Keeping the Ulcer Healed: Patients' Views on Digital Technology in the Prevention of Ulcer Recurrence
- Conclusions
- Acknowledgments
- ABBREVIATIONS
- References
- NLM CatalogRelated NLM Catalog Entries
- PMCPubMed Central citations
- PubMedLinks to PubMed
- Review Advancing pharmacotherapy for diabetic foot ulcers.[Expert Opin Pharmacother. 2019]Review Advancing pharmacotherapy for diabetic foot ulcers.Eleftheriadou I, Tentolouris A, Tentolouris N, Papanas N. Expert Opin Pharmacother. 2019 Jun; 20(9):1153-1160. Epub 2019 Apr 8.
- Poorly designed research does not help clarify the role of hyperbaric oxygen in the treatment of chronic diabetic foot ulcers.[Diving Hyperb Med. 2016]Poorly designed research does not help clarify the role of hyperbaric oxygen in the treatment of chronic diabetic foot ulcers.Mutluoglu M, Uzun G, Bennett M, Germonpré P, Smart D, Mathieu D. Diving Hyperb Med. 2016 Sep; 46(3):133-134.
- Effectiveness of interventions to enhance healing of chronic foot ulcers in diabetes: a systematic review.[Diabetes Metab Res Rev. 2020]Effectiveness of interventions to enhance healing of chronic foot ulcers in diabetes: a systematic review.Vas P, Rayman G, Dhatariya K, Driver V, Hartemann A, Londahl M, Piaggesi A, Apelqvist J, Attinger C, Game F. Diabetes Metab Res Rev. 2020 Mar; 36 Suppl 1:e3284.
- Impact of wound duration on diabetic foot ulcer healing: evaluation of a new sucrose octasulfate wound dressing.[J Wound Care. 2020]Impact of wound duration on diabetic foot ulcer healing: evaluation of a new sucrose octasulfate wound dressing.Lobmann R, Grünerbel A, Lawall H, Lüdemann C, Morbach S, Tigges W, Völkel L, Rychlik RP. J Wound Care. 2020 Oct 2; 29(10):543-551.
- Review Diagnosis and Management of Diabetic Foot Infections[ 2020]Review Diagnosis and Management of Diabetic Foot InfectionsBoulton AJM, Armstrong DG, Hardman MJ, Malone M, Embil JM, Attinger CE, Lipsky BA, Aragón-Sánchez J, Li HK, Schultz G, et al. 2020 Jan
- New Evidence-Based Therapies for Complex Diabetic Foot WoundsNew Evidence-Based Therapies for Complex Diabetic Foot Wounds
Your browsing activity is empty.
Activity recording is turned off.
See more...