This is a work of the US government and distributed under the terms of the Public Domain
NCBI Bookshelf. A service of the National Library of Medicine, National Institutes of Health.
Irwin RD, Malarkey DE, Bristol DW, et al. NTP Genetically Modified Model Report on the Toxicity Studies of Acesulfame Potassium (CASRN 55589-62-3) in FVB/N-TgN(v-Ha-ras)Led (Tg.AC) Hemizygous Mice and Carcinogenicity Studies of Acesulfame Potassium in B6.129-Trp53tm1Brd (N5) Haploinsufficient Mice (Feed Studies): NTP GMM 02 [Internet]. Research Triangle Park (NC): National Toxicology Program; 2005 Oct.
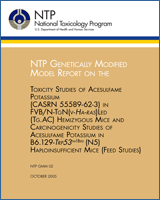
NTP Genetically Modified Model Report on the Toxicity Studies of Acesulfame Potassium (CASRN 55589-62-3) in FVB/N-TgN(v-Ha-ras)Led (Tg.AC) Hemizygous Mice and Carcinogenicity Studies of Acesulfame Potassium in B6.129-Trp53tm1Brd (N5) Haploinsufficient Mice (Feed Studies): NTP GMM 02 [Internet].
Show details
ACESULFAME POTASSIUM
CAS No. 55589-62-3
Chemical Formula: C4H4KNO4S Molecular Weight: 201.25
Synonyms: ASK; HOE-095K; 6-methyl-1,2,3-oxathiazine-4(3-H)-one-2,2-dioxide potassium salt
Trade names: Sunette, Sweet One
Chemical and Physical Properties
Acesulfame potassium is an odorless, colorless, crystalline powder approximately 200 times sweeter than sugar. Acesulfame potassium has a decomposition point of 225° C, is stable over a broad range of pH, and has a density of 1.81 g/cm3 (Merck Index, 1996; HSDB, 2003; Parchem, 2003). Acesulfame potassium is also highly soluble in water, has a long shelf life, and is synergistic when used in combination with other sweeteners (Parchem, 2003).
Production, Use, and Human Exposure
Acesulfame potassium is an artificial sweetener used throughout the world. It is currently available as a dry powder for use in food and beverages and is present as a flavoring agent in numerous food items and diet drinks; therefore, there is extensive human exposure.
Absorption, Distribution, and Excretion
After oral administration, acesulfame potassium is rapidly and completely absorbed then rapidly eliminated in the urine of rats, dogs, and humans. After oral administration to rats, blood concentrations peaked within 30 minutes then declined with a t1/2 of 4.8 hours. Following oral administration to humans (30 mg), blood levels peaked after 1 to 1.5 hours then declined with a t1/2 of 2.5 hours.
Acesulfame potassium is rapidly distributed after oral administration and rapidly eliminated with no evidence of bioaccumulation in any tissue. At the time of maximum blood concentration, the highest levels were present in the gastrointestinal tract, bile, kidneys, and urinary bladder. Following oral administration of [14C]-labeled acesulfame potassium to rats, 97.5% of the administered dose was excreted in the urine as parent compound within 24 hours; in humans, 98.4% was excreted in the urine. Similar results were obtained for groups of rats dosed for 8 or 60 days prior to a histopathologic examination (Volz et al., 1991).
Toxicity
Experimental Animals
One published prechronic study of acesulfame potassium was found in the literature (Sinkeldam et al., 1991a). Groups of 10 male and 10 female Wistar rats received acesulfame potassium in the diet at concentrations of 0%, 1%, 3%, or 10% for 13 weeks. Body weights and food and water consumption were measured. Urine samples were collected, and blood was drawn form the tip of the tail for hematology during week 13; blood was also collected at necropsy for determination of serum enzyme activities. At the end of the study, rats were necropsied, and adrenal gland, brain, cecal weights, heart, kidney, liver, ovary, spleen, testis, thymus, and thyroid gland weights were recorded. Tissues were collected, processed, and a histopathologic examination was conducted. There were no biologically significant differences in hematology or clinical chemistry parameters among the groups. There were slight decreases in mean relative spleen (males) and thymus (females) weights in rats that received 1% acesulfame potassium and in relative spleen weights of 3% females, which were small in magnitude and did not appear to be biologically significant. The only change that was biologically significant was the increase in relative cecal weights in males and females in the 10% groups. No exposure-related histologic lesions were observed.
Humans
No information on toxicity studies of acesulfame potassium in humans was found in the literature.
Carcinogenicity
Experimental Animals
Groups of 60 male and 60 female Wistar rats received diets that contained 0, 3,000, 10,000, or 30,000 ppm acesulfame potassium for 120 (males) or 123 (females) weeks (Sinkeldam et al., 1991b). Body weights were recorded weeks 1 to 5, 8, 10, and 12, and at 4-week intervals thereafter. Feed consumption was measured at regular intervals throughout the study, and water consumption was measured during week 62 and 63. At weeks 33, 53, 78, 102, and 119, blood was collected from 10 males and 10 females in each group for hematology. Blood was drawn from 10 rats per group at weeks 34, 52, and 104 for blood urea nitrogen and glucose and from 10 rats per group for serum chemistry at weeks 34, 52, and 109. At weeks 35, 52, 78, and 104, urine was collected from 10 rats per group for urinalysis. Survival of all exposed groups was similar to the control groups. Mean body weights of 3,000 and 10,000 ppm males and females were similar to the controls throughout the study. The mean body weight of 30,000 ppm males was slightly lower than the controls up to week 80, after which there appeared to be no differences between any of the male groups. The mean body weight of 30,000 ppm females was lower than that of the controls, but the difference was no more than 5%. The only potential exposure-related response was the increased incidences of mammary gland tumors in females. The incidences of fibroadenoma were greater in exposed groups of females (43%, 48%, 48%) than that in control group (30%), and the incidences of adenocarcinoma were greater in exposed females (3%, 5%, 5%) than that in controls (2%). The authors concluded that the increased incidences of adenocarcinoma were not exposure-related because mammary gland adenocarcinomas are common in Wistar rats (historical control rate is 5%), and the incidence in the concurrent control group was relatively low compared to historical control data.
Groups of 100 male and 100 female Swiss mice received diets that contained 0, 3,000, 10,000, or 30,000 ppm acesulfame potassium for 80 weeks (Beems et al., 1991). Body weights were recorded at weeks 1, 2, 4, and at 4-week intervals thereafter. Survival of all exposed groups was similar to that of the controls. Mean body weights of 30,000 ppm males and females were slightly lower than those of the other groups, but the differences were marginal. Mean liver weights of all exposed groups of males were less than that of the control group; kidney weights of exposed males and liver and kidney weights of exposed females were similar to the controls. No exposure-related lesions were observed.
Humans
No epidemiology studies of acesulfame potassium in humans were found in the literature.
Genetic Toxicity
Two studies measured cytogenetic effects in bone marrow cells of male Swiss albino mice administered acesulfame potassium by gavage. Mukherjee and Chakrabarti (1997) measured induction of chromosomal aberrations in mice treated with 15 to 2,250 mg/kg; significant increases in chromatid breaks were observed, and the increases in chromosomally abnormal cells were related to dose. A followup study by Mukhopadhyay et al. (2000) investigated induction of chromosomal aberrations in mice administered a mixture of acesulfame potassium (1.5, 15, and 150 mg/kg) and aspartame (3.5, 35, and 350 mg/kg); doses were chosen based on proposed limits of the two sweeteners together in soft drinks (150 ppm acesulfame potassium and 350 ppm aspartame); no induction of chromosomal aberrations was observed.
Jung et al. (1991) conducted a battery of genetic toxicity tests on acesulfame potassium. At doses from 4 to 10,000 µg/plate, acesulfame potassium failed to increase the frequency of revertants in Salmonella typhimurium strains TA98, TA100, TA1535, TA1537, and TA1538 with or without S9. Over the same dose range, no increase in the frequency point mutations leading to tryptophane independence was observed in Escherichia coli WP2uvrA. At concentrations of 10 to 1,000 µg/mL culture media, acesulfame potassium did not increase the frequency of azaguanine-resistant colonies of Chinese hamster V79 cells.
Incubation of freshly isolated hepatocytes from F344 rats for 20 hours in culture medium containing 25 to 5,000 µg/mL, acesulfame potassium did not increase incorporation of H3-thymidine into nuclear DNA, a measure of unscheduled DNA synthesis (Jung et al., 1991).
No increase in the frequency of micronuclei was observed in the bone marrow of male or female NMRI mice after an acute gavage exposure to 0, 450, 1,500, or 4,500 mg acesulfame potassium/kg body weight (Jung et al., 1991). There was no increase in the frequency of chromosomal aberrations in the bone marrow of male or female Chinese hamsters that received the same dose concentrations for 5 days.
Background on Genetically Altered Mice
Mutation and/or deletions of tumor suppressor genes or activation of protooncogenes can disrupt cell function and predispose an animal to cancer. In the current studies, two genetically altered mouse models with either a loss of heterozygosity in a critical cancer gene (Trp53) or a gain of oncogene function (Ha-ras) were used to determine how these animals would respond to acesulfame potassium exposure. These mouse models are susceptible to the rapid development of cancer. The Tg.AC hemizygous and p53 haploinsufficient mice are being evaluated by the National Institute of Environmental Health Sciences (NIEHS) as models for identifying chemical toxicity and/or chemical carcinogenic processes (Tennant et al., 1996).
FVB/N-TgN(v-Ha-ras)Led (Tg.AC) Hemizygous Mouse Model
The Tg.AC mouse (on an FVB/N background) was developed by Leder et al. (1990) by introduction via pronuclear injection of a tripartite transgene composed of the promotor of the mouse embryonic zeta-globin gene, through the v-Ha-ras coding sequence, with point mutations in codons 12 and 59, and an SV40 polyadenylation sequence.
The Tg.AC transgenic mouse model has been evaluated as a reporter phenotype (skin papillomas) in response to either genotoxic or nongenotoxic carcinogens, including tumor promoters (Spalding et al., 1993, 1999; Tennant et al., 1999). Tg.AC mice are hemizygous for a mutant v-Ha-ras transgene. The model was developed by Leder et al. (1990) with an inducible zeta-globin promoter driving the expression of a mutated v-Ha-ras oncogene and is regarded as a genetically initiated model. With the exception of bone marrow, constitutive expression of the transgene cannot be detected in adult tissues. The transgene is transcriptionally silent until activated by certain treatments including full-thickness wounding, ultraviolet irradiation, or exposure to some chemicals (Cannon et al., 1997; Trempus et al., 1998). The Tg.AC hemizygous mouse develops a high incidence of skin papillomas in response to topical application of 12-O-tetradecanoyl-phorbol-13-acetate (TPA), and TPA has been used as a positive control in NIEHS Tg.AC mouse studies (Spalding et al., 1993). Point mutations in the Ha-ras gene are believed to be early events in the induction of skin papillomas and malignancies. Topical application of carcinogens to the shaved dorsal surface of Tg.AC mice induces epidermal squamous cell papillomas or carcinomas, a reporter phenotype that defines the activity of the chemical. The oral route of administration can also generate tumor responses in the skin of Tg.AC mice and lead to squamous cell papillomas and/or carcinomas of the forestomach. To date, the appearance of either spontaneous or induced tumors has been shown to require activation of transgene expression. However, the mechanism of response by the Tg.AC model to chemical carcinogens is not yet understood.
In NIEHS studies, mice are exposed beginning at 2 months of age for a total of 6 to 9 months. Cutaneous papillomas at various sites have been reported at 10% and 7% incidence in 26-week-old control male and female Tg.AC mice, respectively (Mahler et al.,1998). Cutaneous papillomas occuring at sites such as the lip, pinnae, prepuce, and vulva suggest a possible relationship to grooming and chronic irritation. Up to 32% of Tg.AC homozygous and heterozygous male or female mice can develop odontogenic tumors as early as 26 weeks (Wright et al., 1995; Mahler et al., 1998). A number of different tumor types occur in untreated Tg.AC hemizygous mice at an incidence of greater than 3% including odontogenic tumors, forestomach papillomas, cutaneous papillomas, alveolar/bronchiolar adenomas, salivary gland duct carcinomas, and erythroleukemia (Mahler et al.,1998). In the FVB mouse (the background strain for the Tg.AC hemizygous mouse), alveolar/bronchiolar neoplasms occur at 14 months of age (Mahler et al., 1996).
The Tg.AC hemizygous mouse model was used in the current studies of acesulfame potassium and in the companion studies of aspartame (NTP, 2005) because this model has been reported to detect both nongenotoxic and genotoxic carcinogens (Spalding et al., 1993; Tennant et al., 1995, 1996; Pritchard et al., 2003).
B6.129-Trp53tm1Brd (N5) Haploinsufficient Mouse Model
The heterozygous B6.129-Trp53 (N12)tmlBrd(+/−) mouse (on a B6.129S7 background) was developed by Donehower et al. (1992). A null mutation was introduced into one p53 allele by homologous recombination in murine embryonic stem cells. Insertion of a neo cassette resulted in deletion of a 450-base pair gene fragment containing 106 nucleotides of exon 5 and approximately 350 nucleotides of intron 4.
Trp53, a nuclear protein, plays an essential role in the regulation of the cell cycle, specifically in the transition from G0 to G1, as well as G2 to M, and the spindle apparatus. The p53 protein has a short half-life and exists at a very low concentration under normal cell physiological conditions. However, in DNA damaged cells that are able to replicate, p53 is expressed in high amounts with a significant increase in half-life due to post-translational modification (phosphorylation or acetylation). Mutations in p53 may also increase the protein half-life and alter functions that may contribute to transformation and development of the malignant phenotype. p53 is a DNA-binding protein containing DNA-binding, oligomerization, and transcription activation domains. Many amino acid residues in different p53 domains may be phosphorylated or acetylated, which may determine specific p53 functions. It is postulated to bind as a tetramer to a p53-binding site and activate expression of downstream genes that inhibit growth and/or invasion or promote apoptosis, functioning as a tumor suppressor. This protein is critical to tumor suppression in humans and rodents. Mutants of p53 that fail to bind the consensus DNA binding site, and hence are unable to function as tumor suppressors, frequently occur in human cancers. Alterations of the Tp53 gene occur not only as somatic mutations in human malignancies, but also as germline mutations in some cancer-prone families with Li-Fraumeni syndrome.
The mouse heterozygous for a p53 null allele (+/−) has only a single functional wild-type p53 allele which provides a target for mutagens. The p53 tumor suppressor gene is one of the most common sites for mutations and gene alterations in human cancer (Harris, 1996a,b,c).
Heterozygous p53(+/−) transgenic mice develop normally, and like humans and other mammals, develop cancer (primarily lymphomas or sarcomas) with age, but often with decreased latency and increased susceptibility.
Study Rationale
Acesulfame potassium was nominated by The Center for Science in the Public Interest (CSPI) in 1996. At that time, the FDA was considering a food additive petition for the use of acesulfame potassium as an artificial sweetener in nonalcoholic beverages which would have substantially increased human exposure to acesulfame potassium. CSPI asked that the NTP review the data being submitted to support the petition, and if necessary, conduct additional testing or recommend to the FDA that additional testing be conducted by the sponsor. A review of the carcinogenicity data indicated that an apparently adequate conventional 80-week mouse carcinogenicity study had been conducted with acesulfame potassium (Beems et al., 1991). Because acesulfame potassium is not biotransformed after absorption and was negative in the 80-week mouse carcinogenicity study, it was considered a candidate for a negative control in the evaluation of Tg.AC and p53 mouse models.
The conventional rodent bioassay has been used for over three decades and is credible in identifying carcinogens thought to pose risks to humans (Tomatis et al., 1997). An ongoing goal of the NIEHS and the NTP is to seek other model systems for toxicology and carcinogenesis studies, especially those that can provide mechanistic information that will assist in understanding an agent’s mode of action. The use of genetically altered models holds promise for improving the accuracy and efficacy of experimental assessment of the carcinogenic potential of chemicals. Genetically altered mouse models carry activated oncogenes or inactivated tumor suppressor genes known to be involved in neoplastic processes in humans and rodents. This trait may allow them to respond to carcinogens more quickly than conventional rodent strains. In addition, the neoplastic effects of agents can be observed in genetically altered models within a time frame in which few, if any, spontaneous tumors would arise. The high incidences of spontaneous or background tumors, which occur most often late in the 2-year rodent studies, can hinder interpretation of the findings and their implications for human health. The use of target or reporter genes allows for direct molecular and cellular analysis of a chemical’s effects in genetically modified mouse models and can provide additional mechanistic information about the mode of action.
For the past few years, the NIEHS and the NTP have been actively evaluating genetically altered strains in toxicologic testing strategies. Based on completed evaluations, two models, the Tg.AC hemizygous (v-Ha-ras) and p53-deficient (p53 haploinsufficient) mice, have shown potential usefulness in identifying carcinogens (Pritchard et al., 2003). Acesulfame potassium was one of the test agents selected for the continued evaluation of the genetically modified Tg.AC hemizygous and p53 haploinsufficient strains.
- INTRODUCTION - NTP Genetically Modified Model Report on the Toxicity Studies of ...INTRODUCTION - NTP Genetically Modified Model Report on the Toxicity Studies of Acesulfame Potassium (CASRN 55589-62-3) in FVB/N-TgN(v-Ha-ras)Led (Tg.AC) Hemizygous Mice and Carcinogenicity Studies of Acesulfame Potassium in B6.129-Trp53tm1Brd (N5) Haploinsufficient Mice (Feed Studies)
Your browsing activity is empty.
Activity recording is turned off.
See more...