This book is distributed under the terms of the Creative Commons Attribution-NonCommercial-NoDerivatives 4.0 International (CC BY-NC-ND 4.0) ( http://creativecommons.org/licenses/by-nc-nd/4.0/ ), which permits others to distribute the work, provided that the article is not altered or used commercially. You are not required to obtain permission to distribute this article, provided that you credit the author and journal.
NCBI Bookshelf. A service of the National Library of Medicine, National Institutes of Health.
StatPearls [Internet]. Treasure Island (FL): StatPearls Publishing; 2024 Jan-.
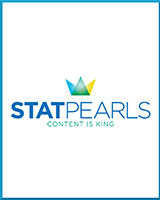
StatPearls [Internet].
Show detailsContinuing Education Activity
Encephalopathic electroencephalography (EEG) patterns are critical in diagnosing and managing various encephalopathies. These patterns can range from the generalized slowing of background activity to more specific findings, such as periodic lateralized epileptiform discharges in herpes simplex encephalitis, generalized periodic discharges in sporadic Creutzfeldt-Jakob disease, and extreme delta brushes in anti-N-methyl-D-aspartate receptor autoimmune encephalitis. While these EEG findings are not typically pathognomonic, they provide valuable insights into the underlying pathophysiology when interpreted within the clinical context. Continuous EEG monitoring is particularly useful for detecting nonconvulsive seizures or status epilepticus, contributing to timely and appropriate management for those who are critically ill.
Clinicians participating in this course enhance their competence in interpreting encephalopathic EEG patterns and understanding their clinical significance. The course provides a comprehensive overview of differentiating between EEG findings associated with different encephalopathies, recognizing patterns indicative of specific etiologies, and applying this knowledge to improve patient outcomes. Additionally, clinicians learn about the latest advancements in EEG technology and how to use continuous monitoring effectively to assess disease progression and treatment response. This course provides healthcare professionals with the skills necessary for accurate diagnosis, effective management, and improved interprofessional communication when caring for patients with encephalopathy.
Objectives:
- Identify distinct encephalography patterns associated with various types of encephalopathies, such as periodic lateralized epileptiform discharges and extreme delta brushes.
- Differentiate between epileptic and nonepileptic encephalography patterns to ensure accurate diagnosis and appropriate treatment plans.
- Apply knowledge of encephalography patterns to interpret brain wave activity accurately and guide treatment decisions.
- Coordinate with multidisciplinary teams to integrateencephalography results into comprehensive patient management plans.
Introduction
Encephalopathy is clinically described as an alteration in generalized attention, cognition, or consciousness. This diffuse cerebral dysfunction can vary in severity due to numerous etiologies, including toxic, metabolic, infectious, and degenerative derangements. Acute encephalopathy may range from mild confusion and delirium to coma, typically presenting with a fluctuating course that involves altered mental status, confusion, and changes in motor activity. Symptoms often include lethargy, cognitive impairment, altered memory and information processing, and disturbed sleep-wake cycles. In more chronic, slowly progressive, or static conditions of encephalopathy, initial attention retention may be followed by a loss of cognitive capacity.[1][2]
Encephalopathy is typically observed in the older population and is commonly seen in intensive care units and those in a postoperative status. Despite the new developments in intensive medical care, acute encephalopathy is still a significant cause of morbidity and mortality in hospitalized patients. In this regard, electroencephalography (EEG) enables rapid bedside electrophysiological monitoring, providing dynamic real-time information on neocortical brain activity and dysfunction.[1]
EEG is useful for evaluating patients with acute and chronic encephalopathies. The primary role of EEG in this setting is to rule out seizures as a cause of altered mental status. Various patterns can be seen in patients with encephalopathy; abnormal patterns, especially in an acute to subacute onset, are sensitive to encephalopathy but not specific to diagnosing causes. Most encephalopathies are associated with the slowing of dominant rhythms and background activity. This is most likely due to the involvement of both the cortical neurons and the subcortical white matter dysfunction. Overall, EEG is useful for assessing the degree of cerebral dysfunction in encephalopathy and monitoring the changes along with clinical progression.
A review on EEG emphasized that it is uncommonly pathognomonic in encephalopathy or encephalitis. However, when interpreted correctly and within the clinical context, some phenotypes may present specific pathophysiology, such as lateralized periodic discharges in herpes simplex virus-1, generalized periodic discharges in sporadic Creutzfeldt-Jakob disease, and extreme delta brushes in anti-N-methyl-D-aspartate receptor autoimmune encephalitis. Specialist guidelines include EEG for disease assessment, monitoring, and prognostication in hepatic, cancer immunotherapy, viral, prion, autoimmune encephalitis, and hypoxic-ischemic encephalopathy. EEG also plays a crucial role in confirming or excluding nonconvulsive seizures or status epilepticus, especially among those who are critically ill, and in understanding recent concepts like epileptic encephalopathy and the ictal-interictal continuum.[3]
Function
Electroencephalography
EEG is usually requested to assess the brain's electrical activity and correlate the findings to physiological and disease states. While primarily used to rule in or rule out seizures, it can also aid in diagnosing encephalopathy and nonepileptic spells. Standard EEGs typically last 30 minutes to an hour; however, the duration may extend based on the patient's clinical status and initial EEG findings. In the inpatient setting, patients with prolonged alterations in sensorium may require extended EEGs, often accompanied by video monitoring. This extended monitoring helps assess various encephalopathic states, utilizing advanced software to review prolonged studies accurately and promptly.[4][5]
The usual EEG recording in the laboratory lasts at least 20 minutes, uses 19 active electrodes, and includes rest periods, stimulation procedures, a 3-minute hyperventilation period, and intermittent photic stimulation. Meanwhile, bedside recording uses at least 8 electrodes, and the stimulation procedures, duration of the EEG, and need to repeat the examination depending on the indication. Furthermore, simultaneous video recording is recommended.[6]
The EEG background activity varies with age. In individuals older than 8, the usual background activity in relaxed wakefulness consists of alpha waves ranging from 8.5 to 12 Hz, with a posterior dominant rhythm that reacts to eye-opening and closure.[7] In children younger than 8, the posterior dominant rhythm is slower. There is an anterior-to-posterior gradient with faster beta range frequency waveforms over the frontal regions and slower waveforms over the posterior regions. There is only a small amount of theta activity (4-8 Hz) during wakefulness with no delta activity. During drowsiness, theta activity appears predominantly in the central or parasagittal regions. As the individual falls asleep, nonrapid eye movement (NREM) and rapid eye movement sleep appear and alternate. Delta activity is predominantly seen in NREM sleep.[7]
The video and EEG recordings allow the reviewer to access and review any clinical events and their associated waveforms in a time-locked fashion to determine if they are associated.[8] The typical EEG duration for encephalopathic patients is longer than routine EEG evaluation on an outpatient basis. Encephalopathic patients are usually seen in acute hospital settings and typically require a longer duration EEG of at least 24 to 48 hours to assess and understand the condition completely. This also helps in monitoring their progress and assessing for nonconvulsive status epilepticus.[9][10][11]
Issues of Concern
Characteristic EEG Patterns Encountered in Acute Encephalopathy
Diffuse Slowing
In any acute encephalopathy, slowing background frequencies are a common EEG finding. The severity of the impairment in attention or consciousness is related to the degree of slowing. The initial changes in mild encephalopathy typically slow the posterior dominant rhythm and reduce the beta activity in the frontal regions.[12][13] Except in benzodiazepine- or barbiturate-related cases, fast beta activity is abundant.[14] As encephalopathy worsens, there is an increase in theta and delta activity. These could be intermittent and then progress to being more continuous as the severity increases. In comatose states, the delta activity predominates and becomes more continuous and slower as the coma deepens. Other changes include loss of reactivity. As the coma deepens, the EEG background becomes discontinuous, which is called the burst-suppression pattern. The interburst intervals increase in duration as the severity of the coma increases further before becoming flat ultimately. This stage is called electrocerebral inactivity or electrocerebral silence.[15] Diffuse slowing is a common finding in anti-N-methyl-d-aspartate receptor encephalitis in children and pediatric acute liver failure with encephalopathy, as seen in case reports.[16][17]
Triphasic Waves
Triphasic waves (TWs) are characterized by a waveform with a triphasic morphology, with a blunt and broad contour, typically frontally dominant with an anterior to posterior phase lag; these could appear in a periodic pattern up to 2.5 Hz in frequency and may have a generalized distribution.[18] These waveforms were initially well associated with hepatic encephalopathy and were, at one point, considered a finding pathognomonic to this condition. The TWs were also associated with stages of progression of encephalopathy and elevated ammonia levels.[19] Studies have later highlighted the coexistence of various etiologies leading to metabolic abnormalities and diffuse white matter changes associated with this pattern.[18] TWs appear more commonly with stimulation or state changes, often overriding a slow background EEG activity.[20][21] Results from recent studies revealed that TWs were generated by large, bilateral cortical networks commonly linked to anterior frontal and temporopolar areas consistent with the typical pathophysiological changes of altered consciousness and cognitive changes observed in patients with TW encephalopathy.[22]
Generalized Rhythmic Delta Activity
Frontally dominant generalized rhythmic delta activity (GRDA), previously referred to as frontal intermittent rhythmic delta activity (FIRDA), is a 2- to 3-Hz high amplitude, rhythmic to semirhythmic activity, with anterior predominance.[23][24] This pattern was commonly felt to be a diagnostic indicator of a midline cerebral pathology, like a 3 ventricle region tumor, but is now well recognized as a nonspecific finding in encephalopathic EEGs. FIRDA is associated with encephalopathic states due to various etiologies like toxic, metabolic, infectious, neoplastic, and epileptic entities. Study results on FIRDA showed a transient rhythmic slow wave pattern over the anterior EEG leads reported in a wide range of cerebral lesions and different metabolic disturbances. Furthermore, FIRDA is more commonly associated with the neurocritical care setting than outpatient EEG clinics. Findings of studies among patients who had a stroke indicated that involved vascular territories may be related to the generation of FIRDA.[25]
GRDA with a posterior emphasis (commonly referred to as occipital intermittent rhythmic delta activity [OIRDA]) is commonly seen in children with absence epilepsy.[26] A recent case series identified an atypical pattern of OIRDA on the EEGs of patients with self-limited focal epilepsies, including childhood epilepsy with centrotemporal spikes and Panayiotopoulos syndrome. Beforehand, OIRDA was identified as a symmetric sinusoidal occipital-maximal activity, often associated with childhood idiopathic generalized epilepsies. However, it was also reported along with other physiologic or pathological entities like focal epilepsy. In this case series, it was observed that OIRDA, without prominent field effect, is lateralized or maximal on the hemispheric side ipsilateral to the more defining epileptiform discharges in these focal epilepsies. These waves also displayed a notched morphology because of the intermixed sharp wave activities, even if the sharp waves are not happening repetitively.[27]
Literature reviews also described that even if frontally predominant GRDA is not associated with seizures, occipitally predominant RDA may be associated with the absence of seizures. Lateralized RDA (LRDA) is more associated with seizure activity. Proper recognition of GRDA and LRDA may be confused with benign etiologies of RDA like phi rhythm, slow alpha variant, subclinical rhythmic electrographic discharges of adults, or hyperventilation-induced high-amplitude rhythmic slowing. Furthermore, Angelman syndrome and N-methyl-d-aspartate-receptor antibody encephalitis can also create morphologically distinct patterns of RDA.[24]
Lateralized Periodic Discharges or Periodic Lateralized Epileptiform Discharges
This EEG pattern is characterized by sharp waves or spikes of complexes 1 to 3 Hz frequency in a semirhythmic pattern with no clear progression or spread; these are usually associated with a subacute structural lesion.[28][29] The most common condition associated with this pattern is stroke, among several other etiologies.[30] There is still debate whether this pattern is associated with seizures.[31] Results from a retrospective study showed that LPDs were associated with the development of epilepsy in one-third of the cohort and associated with markers of epileptogenicity in about 18% of the patients.[32]
Recent study results showed that patients with LRDA had a similarly high rate of acute focal abnormalities ipsilateral to the EEG pattern compared with those with LPDs. In addition, electrographic features of LPDs may help identify seizure risk in patients with acute structural lesions. Sharply contoured morphology, overlying fast frequencies, or rhythmicity revealed a progressively higher risk of seizures on continuous EEG compared to the blunt delta morphology—which had the lowest risk of seizures. Meanwhile, case reports mentioned other conditions that have been recognized as the underlying pathology for PLEDs, namely alcohol withdrawal, Creutzfeldt-Jakob disease, anoxic brain injury, and hemiplegic migraine. Also, the increasing utilization of continuous video EEG in the acute care setting of the ictal-interictal continuum (IIC) has revealed that LPDs and other periodic and rhythmic patterns are extremely common. Reviews have been done to address the ongoing debate that LPDs and the pattern lying along the IIC are called "periictal," which means that LPDs are temporally associated with epileptic seizures (although not necessarily in the same recording).
The following criteria were proposed to determine which kind of LPDs should be considered as representing interictal/irritative brain injury versus ictal/periictal LPDs:
- Spiky or sharp LPDs followed by associated slow after-waves or periods of flattening giving rise to a triphasic morphology should be included in the definition of LPDs-plus.
- In its subtype, the "LPDs-max" pattern pertains to an ictal pattern and, therefore, a focal nonconvulsive status epilepticus, sometimes related to the subtle motor signs and epileptic seizures. LPDs-max consists of periodic polyspike-wave activity and focal burst-suppression-like patterns. LPDs-max also have a posterior predominance over the temporoparietooccipital regions and are refractory to antiseizure drugs.[33][34][35][29][36]
Generalized Periodic Epileptiform Discharges and Bilateral Independent Periodic Epileptiform Discharges
These patterns are seen in several conditions, including anoxic brain injury with severe diffuse cerebral dysfunction.[37][38] Current studies discussed that EEG, alongside clinical examination and imaging studies, is used to determine the prognosis following hypoxic encephalopathy postcardiac arrest. Generalized periodic epileptiform discharges are treated as a "malignant" EEG pattern related to very poor outcomes, with previous studies reporting no or few survivors. Recent case reports emphasize that bilateral independent periodic lateralized epileptiform discharges in EEGs are commonly caused by anoxic encephalopathy and central nervous system infections. They are also associated with coma and high mortality and are thus markers of poor prognosis.[39]
Alpha Coma and Spindle Coma
Diffuse alpha frequency activity can be seen in a comatose state. When the predominant alpha activity is noted in the posterior head regions and varies with noxious external stimuli, the etiology of the coma may be secondary to a brainstem lesion; this is associated with a poor prognosis.[40] More diffuse alpha activity with less reactivity to external stimuli is seen in anoxic injury after cardiac arrest and is also commonly associated with a poor prognosis. When alpha coma is noted on EEG, the overall outcome depends on the etiology and reactivity to external stimuli, with a better prognosis in toxic encephalopathies and worse in anoxic encephalopathies.[40][41] Spindle coma consists of paroxysmal bursts of 11 to 14 Hz activity appearing on a delta background and is usually known to occur in cases of anoxic injury, intracranial hemorrhage, diffuse cerebral insults, and head trauma.[42][43] EEG pattern spindle coma is associated with the involvement of the pontomesencephalic junction.[44]
A recent study analyzed the EEG of patients with coronavirus disease of 2019 (COVID-19) while considering the disease severity, respiratory failure, immune and metabolic dysfunction, sedation status, and neurological examination. The results revealed that the majority of patients experienced severe encephalopathy, and some had alpha coma. Disease severity, sedation, immune, and metabolic dysfunction were not different between those with alpha coma and those without. The comparatively high incidence of the rare alpha coma pattern may reflect direct severe acute respiratory syndrome coronavirus 2 neurotropism with preference to the brainstem ascending reticular system. The study concluded that systematic early EEG detection of encephalopathy related to severe COVID-19 is important for acute care and the management of long-term neurological and cognitive sequelae, and it may help us better understand pathophysiology.[45]
Burst Suppression
The burst-suppression pattern consists of periods of mixed-frequency activity (bursts) and inactivity or suppression of the background.[46] The bursts can have sharp and epileptiform discharges. This pattern can be seen in comatose individuals from various etiologies like toxic encephalopathies and anoxic encephalopathy due to cardiac arrest.[47] As the coma worsens, the interval between the bursts progressively increases, correlating with the clinical condition's severity.[48]
Other literature described burst-suppression EEG patterns of electrical activity as intermittent high-power broad-spectrum oscillations alternating with isoelectricity during general anesthesia, hypothermia, coma, and early infantile encephalopathy. Burst suppression contains bursts of high-voltage slow and sharp wave activity with alternating periods of background suppression in the EEG. If induced by deep anesthesia or encephalopathy, burst suppression is bihemispheric and often seen as a nonepileptic phenomenon.[49][50]
Electrocerebral inactivity or Electrocerebral Silence
Electrocerebral inactivity (ECI) or electrocerebral silence (ECS) is encountered when there is no EEG activity over 2 mV when recorded from scalp electrode pairs equal to or greater than 10 cm apart and with interelectrode impedances below 10,000 Ohms but above 100 Ohms for at least 30 minutes of the recording. A qualified technologist should perform these types of recordings. When other causes like drug overdose are excluded, this pattern is associated with brain death.[51]
A study discussed the EEG guidelines in diagnosing brain death in France, where the law states that either 2 EEG recordings separated by a 4-hour observation period, both showing ECI, or a cerebral angiography examination can diagnose brain death. Since EEG is available in most hospitals and clinics, it is often used in this indication at the patient's bedside, especially in the context of organ donation. ECI may be confirmed when a 30-minute good-quality EEG recording presents complete electrocerebral silence, described as no cerebral activity greater than 2 mV, having first ruled out the possible influence of sedative drugs, metabolic disorders, or hypothermia. Among newborns, the utmost caution is indicated since ECI can be observed in the absence of cerebral death.[50]
Clinical Significance
Encephalopathy is commonly described as an alteration of consciousness or attention ranging from mild to severe, with severe cases potentially leading to poor prognosis or death. Secondary to various etiologies, encephalopathy can manifest in acute and chronic forms. EEG is typically requested to assess for pattern and rhythm abnormalities, which may or may not be associated with ictal states, and continuous monitoring is also used to assess for progression and response to treatment.
Narrative reviews have focused on acute symptomatic seizures occurring among patients with electrolyte disturbances. Seizures are more commonly seen among those with sodium disorders such as hyponatremia, hypocalcemia, and hypomagnesemia. These seizures do not entail a diagnosis of epilepsy but are classified as acute symptomatic seizures. EEG has limited specificity in distinguishing between various electrolyte disturbances. The distinct EEG feature slows normal background activity, although other EEG findings may occur, including various epileptiform abnormalities.[52]
The following section provides a more detailed discussion of EEG findings in common acute encephalopathies.
Hepatic Encephalopathy
- This is encountered in patients with liver failure or insufficiency from any cause. Recent reviews mentioned minimal hepatic encephalopathy (MHE) as the earliest form of hepatic encephalopathy and can affect up to 80% of patients with liver cirrhosis. Also, MHE affects daily functioning, quality of life, driving, and overall mortality. Spectral analysis of EEG and quantitative analysis of sleep EEG provide early markers of cerebral dysfunction in patients with cirrhosis with MHE.[53]
- The EEG changes in the beginning commonly include slowing of the posterior dominant rhythm, followed by a gradual slowing of the background with the appearance of theta and delta activity.[12] FIRDA can appear even in the presence of the posterior dominant rhythm.
- Classic TWs are best viewed when the encephalopathy worsens and the ammonia levels are higher. As the severity worsens, sleep architecture is sparse.[13]
Renal (Uremic) Encephalopathy
- This condition is encountered in patients with renal dysfunction of any cause with increased blood urea nitrogen level.[54]
- These patients' EEGs are also similar to hepatic encephalopathic EEGs characterized by TWs and a slow background.
- Bursts of well-formed, smoothly contoured, negative-positive-negative, bilateral, symmetrical and synchronous, regular, reactive, periodic or rhythmic, 1.5 to 2.0 Hz, frontocentral, triphasic complexes with frontooccipital lag meet the criteria for typical TWs and are highly suggestive of toxic-metabolic encephalopathies frequently hepatic, uremic, or sepsis-associated encephalopathies with multi-organ failure.
- High-voltage rhythmic delta activity with bilateral spike-slow-wave complexes is often seen in patients with dialysis disequilibrium syndrome associated with obtundation after a dialysis session.[55]
Hypocalcemia
- Hypocalcemia is, by definition, a corrected total serum calcium level below 2.2 mmol/L. Decreased calcium levels are most commonly associated with vitamin D deficiency and hypoparathyroidism, among several other etiologies.[58]
- Initially, the most common EEG change is progressive slowing through theta and delta frequency activity dominance. There is also an association between generalized spikes and sharp waves and a burst of delta activity. A 3 to 4 Hz spike and wave discharges have been reported in neonatal EEG records.[59] 'Absence status' has also been reported in these patients.[60]
- Formula milk feeding, parathyroid hormone insufficiency, and low serum vitamin D concentration are related to the development of neonatal hypocalcemia. Symptoms, including tetany and QT interval prolongation, can lead to relatively mild hypocalcemia. Furthermore, transient neonatal hypocalcemia can cause transient EEG abnormalities.[53]
Hypercalcemia
- The EEG background changes with increased theta and delta activity when serum calcium levels reach over 13 mg/dL.[12] The EEG can be associated with spikes and sharp waves as well. With an even further increase in calcium levels, the background slowing increases mostly in the frontal regions with the appearance of a paroxysmal burst of theta and delta activity along with TWs.[62][63]
- Case reports also identified hypercalcemia as the cause of a subacute syndrome of progressive dementia and marked changes in the EEG, such as bursts of 1.5 to 2 Hz intermittent rhythmic delta activity superimposed on a low-voltage background activity in the EEG. Clinical and EEG abnormalities rapidly resolved after serum calcium levels returned to normal. The reports also recommended that serum calcium level and EEG should be performed to detect a Creutzfeldt–Jakob disease-like syndrome in hypercalcemia.[66]
Hypoglycemia
- Hypoglycemia is typically defined as a blood glucose level below 70 mg/dL. The correlation between blood glucose level, consciousness or attention level, and EEG changes is associated with a marked variation among individuals.[67]
- The lowest blood glucose level where detectable EEG changes may be seen is 29 to 40 mg/dL.[68]
- The blood glucose level threshold is slightly higher in hypoglycemic individuals with diabetes, where EEG changes can be seen.[69]
- The common finding associated with a lower glucose level is the slowing of the background, mainly in the theta frequency range. This is noted in both adults and children.[70]
Hyperglycemia
- With milder hyperglycemia, the EEG background activity may slow down slightly or not at all. As glucose levels increase, the diffuse delta slowing increases, and when a level of about 400 mg/dL is crossed, a sporadic spike can be seen.
Hypernatremia
Hyponatremia
- EEG changes in hyponatremia are associated with background slowing in theta to delta range frequencies as serum sodium levels drop, typically below 116 mg/dL.
Hypothyroidism
- EEG in hypothyroid states is commonly associated with a low voltage activity, predominantly in the theta frequency range. Comatose individuals in this condition may show diffuse suppression with minimal activity. Sporadically, periodic sharp waves may be encountered.[79]
Hyperthyroidism
Hashimoto Encephalopathy
- This is a chronic, relapsing autoimmune thyroid disorder associated with antithyroid antibodies. This condition is usually associated with other autoimmune disorders like systemic lupus erythematosus and rheumatoid arthritis, which are usually steroid-responsive.
- EEG is associated with generalized slowing and FIRDA in these patients. Often, TWs are also seen.[83]
- Recent study results revealed that the common neuropsychiatric features of patients with Hashimoto encephalopathy include consciousness disturbance and psychosis, followed by cognitive dysfunction, involuntary movements, seizures, and ataxia. Abnormalities in EEG and decreased cerebral blood flow in the brain through single photon emission computed tomography were common findings. Patients with Hashimoto encephalopathy also exhibited other clinical phenotypes, namely acute encephalopathy, chronic psychiatric form, and other particular clinical presentations, such as limbic encephalitis, progressive cerebellar ataxia, and a Creutzfeldt–Jakob disease-mimic form.[84]
Hypoxic and Anoxic Encephalopathy
- Anoxic or hypoxic injury is encountered in cardiac arrest, and the extent of brain injury correlates with the severity of anoxia. This includes a wide spectrum from mild, slowing, to severe suppression. Poor prognostic EEG findings include alpha or spindle coma with poor reactivity, burst suppression pattern with longer interburst intervals, and ECI or ECS.[12][85] Results from a recent study related microscopic synaptic dynamics in anoxic brain injury to typical EEG observations and their evolution in time. The finding showed that the range of EEG patterns relies on the excitation-inhibition imbalance, probably due to the long-term potentiation of excitatory neurotransmission. Moreover, the evolution of EEG patterns occurred from the gradual improvement of complete synaptic failure, where excitatory synapses are greatly affected than inhibitory synapses.[86]
- Hypoxic-ischemic encephalopathy in neonates is associated with a favorable outcome if the EEG performed within the first 8 hours after birth shows an active, normal background and portends a poorer outcome if the background activity is grossly abnormal or inactive.[87] Another study mentioned that neonates with hypoxic-ischemic encephalopathy with burst suppression, low voltage, and flat trace in the EEG most accurately forecast long-term neurodevelopmental results.[88]
Infections Associated with the Central Nervous System
- Central nervous system infections such as encephalitis, cerebral abscess, meningoencephalitis, or meningitis can manifest with diffuse changes and focal findings on the EEG. These can be associated with both generalized and focal epileptiform discharges with corresponding slowing.
- A commonly encountered example is herpes simplex encephalitis, typically associated with LPDs over the affected temporal lobe.[89]
Trauma and Intracranial Hemorrhage
- Traumatic brain injuries (TBI) can be associated with focal and diffuse changes based on the injury's extent and the affected intracranial structures. Global damages like diffuse axonal injury are typically associated with diffuse slowing, whereas contusion and hemorrhages are associated with focal slowing and epileptiform discharges.[90][91]
- In extreme TBI cases, different EEG patterns can be encountered, including alpha or spindle coma due to brainstem injury, burst suppression, and even ECI.
Drug-Induced or Toxic Encephalopathy
- The etiology of this type of encephalopathy is numerous. The EEG changes in this setting can range from the diffuse slowing of the background in theta and delta frequency activity to an abundance of superimposed beta activity, especially with benzodiazepine and barbiturate overdoses.
- Some drugs (eg, lithium) are associated with focal, multifocal, or diffuse epileptic activity and seizures as well.
- TWs are also seen in drug-induced encephalopathies.[13]
Other Issues
EEG in Chronic Encephalopathies
Chronic encephalopathies can also show EEG changes. The most common finding is slowing of the background in theta and delta range as the encephalopathy, or the disease, worsens. In neurodegenerative diseases like Alzheimer disease, Pick disease, Parkinson disease, and vascular dementia, EEG findings are typically normal at the initial stages.[92][93][94][95] A low amplitude EEG background activity is common in Huntington disease.[96] Periodic complexes with very high amplitude 2 to 4 delta waveforms intermixed with epileptiform or sharp discharges appearing every 5 to 7 seconds are seen in subacute sclerosing panencephalitis. Clinically, these complexes can be associated with myoclonic jerks.[97]
Creutzfeldt–Jakob Disease
Creutzfeldt–Jakob disease (CJD) is a rapidly progressive neurodegenerative disorder associated with prion protein, an abnormal isoform of a cellular glycoprotein. The majority of patients with CJD typically die within 1 year of contracting the illness. Clinically, in addition to rapidly progressive dementia, there can be myoclonus, visual or cerebellar signs, pyramidal/extrapyramidal signs, and akinetic mutism with variable association. EEG changes are commonly seen in this condition and are characterized by diffuse slowing, the appearance of periodic complexes, and GRDA.[98] The periodic complexes in CJD appear more frequently, usually at 1 Hz. These complexes typically contain a sharp and slow wave and can be either unilateral initially or bilateral as the disease progresses.[99] Moreover, the EEG pattern in CJD is described as diffuse abnormal activity, although lateralization to 1 hemisphere has been described in the first stages of the disease. The abnormal EEG activity in CJD is diffuse without clear spatial predominance in anterior or posterior brain regions.[100]
EEG Changes in Encephalopathy Associated with COVID-19 or SARS-CoV2 Infection
The common EEG changes noted in patients with COVID-19-related encephalopathy include generalized slowing of the background.[101][102][103] In another cohort of 18 patients, there was a direct correlation between oxygen saturation levels and EEG changes at the time of presentation. Lower oxygen levels were associated with more severe EEG patterns, like the association with epileptiform discharges.[104] Another cohort of 15 patients with COVID-19-related encephalopathy from 873 patients admitted for SARS-CoV2 infection reported rather homogenous EEG changes mainly comprising of a diffuse background activity slowing and loss of reactivity towards external stimulation. Two patients in this cohort were comatose from anoxic injury, with 1 case associated with a suppressed background and the other with a discontinuous activity consistent with postanoxic status epilepticus.[105]
A recent study reported EEG findings in both COVID-19 survivors and nonsurvivors who had EEG either due to seizure or encephalopathy. Of the total of 1468 COVID-19-positive patients, only 19 underwent EEG. Amon the 13 survivors, the most common EEG findings were normal, followed by mild diffuse slowing; however, among the 6 nonsurvivors, the most common EEG finding was moderate to severe slowing in 50% of the patients. From this, it can be concluded that COVID-19 infection does not increase the propensity for epileptiform discharges on EEG.[106]
Enhancing Healthcare Team Outcomes
Effectively managing encephalopathy requires a comprehensive skill set and strategic approach from the entire healthcare team. Physicians and advanced clinicians must be adept at identifying and interpreting encephalopathic EEG patterns, ensuring accurate diagnosis and timely intervention. Nurses play a critical role in continuous monitoring and documentation of patient status, while EEG technologists must skillfully perform and interpret EEG studies. Pharmacists contribute by managing and adjusting medications that may influence neurological status. An interprofessional team of clinicians is necessary to investigate and appropriately treat patients with encephalopathy who present to the hospitals in acute situations to achieve the best outcomes.[107][9]
Interprofessional communication and care coordination are paramount in enhancing patient-centered care, outcomes, patient safety, and team performance. Regular multidisciplinary meetings and clear, concise documentation help ensure that all team members are aware of the patient's condition and treatment plan. Effective communication allows for prompt adjustments to care plans based on real-time EEG findings and clinical assessments. Collaborative strategies, such as shared decision-making and cross-disciplinary training, empower each team member to contribute their expertise, leading to a more holistic and effective approach to managing encephalopathy.
References
- 1.
- Sutter R, Kaplan PW, Valença M, De Marchis GM. EEG for Diagnosis and Prognosis of Acute Nonhypoxic Encephalopathy: History and Current Evidence. J Clin Neurophysiol. 2015 Dec;32(6):456-64. [PubMed: 26629755]
- 2.
- Rayi A, Mandalaneni K. StatPearls [Internet]. StatPearls Publishing; Treasure Island (FL): Mar 4, 2023. Encephalopathic EEG Patterns. [PubMed: 33232041]
- 3.
- Morris H, Kaplan PW, Kane N. Electroencephalography in encephalopathy and encephalitis. Pract Neurol. 2024 Jan 23;24(1):2-10. [PubMed: 38050141]
- 4.
- Sarkis RA, Lee JW. Quantitative EEG in hospital encephalopathy: review and microstate analysis. J Clin Neurophysiol. 2013 Oct;30(5):526-30. [PubMed: 24084185]
- 5.
- Rayi A, Murr NI. StatPearls [Internet]. StatPearls Publishing; Treasure Island (FL): Oct 3, 2022. Electroencephalogram. [PubMed: 33085442]
- 6.
- Rosenberg SD, Périn B, Michel V, Debs R, Navarro V, Convers P. EEG in adults in the laboratory or at the patient's bedside. Neurophysiol Clin. 2015 Mar;45(1):19-37. [PubMed: 25660125]
- 7.
- Britton JW, Frey LC, Hopp JL, Korb P, Koubeissi MZ, Lievens WE, Pestana-Knight EM, St. Louis EK. Electroencephalography (EEG): An Introductory Text and Atlas of Normal and Abnormal Findings in Adults, Children, and Infants [Internet]. St. Louis EK, Frey LC, editors. American Epilepsy Society; Chicago: 2016. [PubMed: 27748095]
- 8.
- Nordli DR. Usefulness of video-EEG monitoring. Epilepsia. 2006;47 Suppl 1:26-30. [PubMed: 17044822]
- 9.
- Abend NS, Dlugos DJ, Hahn CD, Hirsch LJ, Herman ST. Use of EEG monitoring and management of non-convulsive seizures in critically ill patients: a survey of neurologists. Neurocrit Care. 2010 Jun;12(3):382-9. [PMC free article: PMC2944658] [PubMed: 20198513]
- 10.
- Schreiber JM, Zelleke T, Gaillard WD, Kaulas H, Dean N, Carpenter JL. Continuous video EEG for patients with acute encephalopathy in a pediatric intensive care unit. Neurocrit Care. 2012 Aug;17(1):31-8. [PubMed: 22565632]
- 11.
- Malhotra K, Rayi A. Gyriform Infarction in Cerebral Air Embolism: Imaging Mimicker of Status Epilepticus. Ann Indian Acad Neurol. 2017 Jul-Sep;20(3):313-315. [PMC free article: PMC5586131] [PubMed: 28904468]
- 12.
- Kaplan PW. The EEG in metabolic encephalopathy and coma. J Clin Neurophysiol. 2004 Sep-Oct;21(5):307-18. [PubMed: 15592005]
- 13.
- Kaplan PW, Rossetti AO. EEG patterns and imaging correlations in encephalopathy: encephalopathy part II. J Clin Neurophysiol. 2011 Jun;28(3):233-51. [PubMed: 21633250]
- 14.
- Feyissa AM, Tatum WO. Adult EEG. Handb Clin Neurol. 2019;160:103-124. [PubMed: 31277842]
- 15.
- Young GB. The EEG in coma. J Clin Neurophysiol. 2000 Sep;17(5):473-85. [PubMed: 11085551]
- 16.
- Özgör B, Gungor S, Aladağ M, Varol FI, Aslan M, Yilmaz S, Gungor S. The Value of Electroencephalogram (EEG) Findings in the Evaluation and Treatment Management of Pediatric Acute Liver Failure. Cureus. 2024 Feb;16(2):e54300. [PMC free article: PMC10944321] [PubMed: 38496192]
- 17.
- Yildirim M, Konuskan B, Yalnizoglu D, Topaloglu H, Erol I, Anlar B. Electroencephalographic findings in anti-N-methyl-d-aspartate receptor encephalitis in children: A series of 12 patients. Epilepsy Behav. 2018 Jan;78:118-123. [PubMed: 29179102]
- 18.
- Butterworth RF, Lavoie J, Giguère JF, Layrargues GP, Bergeron M. Cerebral GABA-ergic and glutamatergic function in hepatic encephalopathy. Neurochem Pathol. 1987 Feb-Apr;6(1-2):131-44. [PubMed: 2888065]
- 19.
- Bermeo-Ovalle A. Triphasic Waves: Swinging the Pendulum Back in this Diagnostic Dilemma. Epilepsy Curr. 2017 Jan-Feb;17(1):40-42. [PMC free article: PMC5340556] [PubMed: 28331470]
- 20.
- Brigo F, Storti M. Triphasic waves. Am J Electroneurodiagnostic Technol. 2011 Mar;51(1):16-25. [PubMed: 21516927]
- 21.
- Emmady PD, Murr NI. StatPearls [Internet]. StatPearls Publishing; Treasure Island (FL): Feb 1, 2023. EEG Triphasic Waves. [PubMed: 32491611]
- 22.
- Kural MA, Fabricius M, Christensen J, Kaplan PW, Beniczky S. Triphasic Waves Are Generated by Widespread Bilateral Cortical Networks. J Clin Neurophysiol. 2021 Sep 01;38(5):415-419. [PubMed: 32852286]
- 23.
- Hirsch LJ, Brenner RP, Drislane FW, So E, Kaplan PW, Jordan KG, Herman ST, LaRoche SM, Young B, Bleck TP, Scheuer ML, Emerson RG. The ACNS subcommittee on research terminology for continuous EEG monitoring: proposed standardized terminology for rhythmic and periodic EEG patterns encountered in critically ill patients. J Clin Neurophysiol. 2005 Apr;22(2):128-35. [PubMed: 15805813]
- 24.
- Schmitt SE. Generalized and Lateralized Rhythmic Patterns. J Clin Neurophysiol. 2018 May;35(3):218-228. [PubMed: 29718831]
- 25.
- Dericioglu N, Khasiyev F, Arsava EM, Topcuoglu MA. Frontal Intermittent Rhythmic Delta Activity (FIRDA) in the Neurological Intensive Care: Prevalence, Determinants, and Clinical Significance. Clin EEG Neurosci. 2018 Jul;49(4):272-277. [PubMed: 28118746]
- 26.
- Watemberg N, Linder I, Dabby R, Blumkin L, Lerman-Sagie T. Clinical correlates of occipital intermittent rhythmic delta activity (OIRDA) in children. Epilepsia. 2007 Feb;48(2):330-4. [PubMed: 17295627]
- 27.
- LaBarbera V, Nie D. Occipital intermittent rhythmic delta activity (OIRDA) in pediatric focal epilepsies: A case series. Epilepsy Behav Rep. 2021;16:100472. [PMC free article: PMC8353336] [PubMed: 34401708]
- 28.
- CHATRIAN GE, SHAW CM, LEFFMAN H. THE SIGNIFICANCE OF PERIODIC LATERALIZED EPILEPTIFORM DISCHARGES IN EEG: AN ELECTROGRAPHIC, CLINICAL AND PATHOLOGICAL STUDY. Electroencephalogr Clin Neurophysiol. 1964 Aug;17:177-93. [PubMed: 14204927]
- 29.
- Newey CR, Sahota P, Hantus S. Electrographic Features of Lateralized Periodic Discharges Stratify Risk in the Interictal-Ictal Continuum. J Clin Neurophysiol. 2017 Jul;34(4):365-369. [PubMed: 28166083]
- 30.
- Pohlmann-Eden B, Hoch DB, Cochius JI, Chiappa KH. Periodic lateralized epileptiform discharges--a critical review. J Clin Neurophysiol. 1996 Nov;13(6):519-30. [PubMed: 8978624]
- 31.
- Lin L, Drislane FW. Lateralized Periodic Discharges: A Literature Review. J Clin Neurophysiol. 2018 May;35(3):189-198. [PubMed: 29718828]
- 32.
- Punia V, Vakani R, Burgess R, Hantus S. Electrographic and Clinical Natural History of Lateralized Periodic Discharges. J Clin Neurophysiol. 2018 Jan;35(1):71-76. [PubMed: 29099408]
- 33.
- Alzawahmah M, Fong MWK, Gilmore EJ, Hirsch LJ. Neuroimaging Correlates of Lateralized Rhythmic Delta Activity, Lateralized Periodic Discharges, and Generalized Rhythmic Delta Activity on EEG in Critically Ill Patients. J Clin Neurophysiol. 2022 Mar 01;39(3):228-234. [PubMed: 33235178]
- 34.
- Greenblatt AS, Lui F. StatPearls [Internet]. StatPearls Publishing; Treasure Island (FL): Jun 25, 2023. Ictal-Interictal Continuum. [PubMed: 36944013]
- 35.
- Gelisse P, Crespel A, Genton P, Jallon P, Kaplan PW. Lateralized Periodic Discharges: Which patterns are interictal, ictal, or peri-ictal? Clin Neurophysiol. 2021 Jul;132(7):1593-1603. [PubMed: 34034086]
- 36.
- Takagaki K, Morales MK, Vitantonio D, Berkowitz F, Bell WL, Kumar PN, Motamedi GK. Periodic Lateralized Epileptiform Discharges (PLEDs) in Patients With Neurosyphilis and HIV Infection. Clin EEG Neurosci. 2016 Jul;47(3):247-50. [PubMed: 25326288]
- 37.
- Sully KE, Husain AM. Generalized Periodic Discharges: A Topical Review. J Clin Neurophysiol. 2018 May;35(3):199-207. [PubMed: 29718829]
- 38.
- Ruijter BJ, van Putten MJ, Hofmeijer J. Generalized epileptiform discharges in postanoxic encephalopathy: Quantitative characterization in relation to outcome. Epilepsia. 2015 Nov;56(11):1845-54. [PubMed: 26384469]
- 39.
- Iyer RS, Ramalingam RTC, Akhtar S, Ponnadan S. Bilateral independent periodic lateralised epileptiform discharges at presentation followed by rapid recovery: novel observations from a case of Epstein-Barr virus encephalitis. BMJ Case Rep. 2019 Jul 19;12(7) [PMC free article: PMC6663246] [PubMed: 31326904]
- 40.
- Westmoreland BF, Klass DW, Sharbrough FW, Reagan TJ. Alpha-coma. Electroencephalographic, clinical, pathologic, and etiologic correlations. Arch Neurol. 1975 Nov;32(11):713-8. [PubMed: 1180739]
- 41.
- Kaplan PW, Genoud D, Ho TW, Jallon P. Etiology, neurologic correlations, and prognosis in alpha coma. Clin Neurophysiol. 1999 Feb;110(2):205-13. [PubMed: 10210610]
- 42.
- Hansotia P, Gottschalk P, Green P, Zais D. Spindle coma: incidence, clinicopathologic correlates, and prognostic value. Neurology. 1981 Jan;31(1):83-7. [PubMed: 7192831]
- 43.
- Kaplan PW, Genoud D, Ho TW, Jallon P. Clinical correlates and prognosis in early spindle coma. Clin Neurophysiol. 2000 Apr;111(4):584-90. [PubMed: 10727908]
- 44.
- Bergamasco B, Bergamini L, Doriguzzi T, Fabiani D. EEG sleep patterns as a prognostic criterion in post-traumatic coma. Electroencephalogr Clin Neurophysiol. 1968 Apr;24(4):374-7. [PubMed: 4174009]
- 45.
- Koutroumanidis M, Gratwicke J, Sharma S, Whelan A, Tan SV, Glover G. Alpha coma EEG pattern in patients with severe COVID-19 related encephalopathy. Clin Neurophysiol. 2021 Jan;132(1):218-225. [PMC free article: PMC7527310] [PubMed: 33060058]
- 46.
- Niedermeyer E. The burst-suppression electroencephalogram. Am J Electroneurodiagnostic Technol. 2009 Dec;49(4):333-41. [PubMed: 20073416]
- 47.
- Reeves AL, Westmoreland BF, Klass DW. Clinical accompaniments of the burst-suppression EEG pattern. J Clin Neurophysiol. 1997 Mar;14(2):150-3. [PubMed: 9165410]
- 48.
- Hofmeijer J, Tjepkema-Cloostermans MC, van Putten MJ. Burst-suppression with identical bursts: a distinct EEG pattern with poor outcome in postanoxic coma. Clin Neurophysiol. 2014 May;125(5):947-54. [PubMed: 24286857]
- 49.
- Shanker A, Abel JH, Schamberg G, Brown EN. Etiology of Burst Suppression EEG Patterns. Front Psychol. 2021;12:673529. [PMC free article: PMC8222661] [PubMed: 34177731]
- 50.
- Szurhaj W, Lamblin MD, Kaminska A, Sediri H., Société de Neurophysiologie Clinique de Langue Française. EEG guidelines in the diagnosis of brain death. Neurophysiol Clin. 2015 Mar;45(1):97-104. [PubMed: 25687591]
- 51.
- Stecker MM, Sabau D, Sullivan L, Das RR, Selioutski O, Drislane FW, Tsuchida TN, Tatum WO. American Clinical Neurophysiology Society Guideline 6: Minimum Technical Standards for EEG Recording in Suspected Cerebral Death. J Clin Neurophysiol. 2016 Aug;33(4):324-7. [PubMed: 27482789]
- 52.
- Nardone R, Brigo F, Trinka E. Acute Symptomatic Seizures Caused by Electrolyte Disturbances. J Clin Neurol. 2016 Jan;12(1):21-33. [PMC free article: PMC4712283] [PubMed: 26754778]
- 53.
- Nardone R, Taylor AC, Höller Y, Brigo F, Lochner P, Trinka E. Minimal hepatic encephalopathy: A review. Neurosci Res. 2016 Oct;111:1-12. [PubMed: 27153746]
- 54.
- Glaser GH. Brain dysfunction in uremia. Res Publ Assoc Res Nerv Ment Dis. 1974;53:173-99. [PubMed: 4612659]
- 55.
- Hughes JR. Correlations between EEG and chemical changes in uremia. Electroencephalogr Clin Neurophysiol. 1980 May;48(5):583-94. [PubMed: 6153966]
- 56.
- Noriega-Sanchez A, Martinez-Maldonado M, Haiffe RM. Clinical and electroencephalographic changes in progressive uremic encephalopathy. Neurology. 1978 Jul;28(7):667-9. [PubMed: 566866]
- 57.
- Gadewar P, Acharya S, Khairkar P, Shukla S, Mahajan SN. Dynamics of electroencephalogram (EEG) in different stages of chronic kidney disease. J Clin Diagn Res. 2015 Mar;9(3):OC25-7. [PMC free article: PMC4413100] [PubMed: 25954651]
- 58.
- Fong J, Khan A. Hypocalcemia: updates in diagnosis and management for primary care. Can Fam Physician. 2012 Feb;58(2):158-62. [PMC free article: PMC3279267] [PubMed: 22439169]
- 59.
- Kossoff EH, Silvia MT, Maret A, Carakushansky M, Vining EP. Neonatal hypocalcemic seizures: case report and literature review. J Child Neurol. 2002 Mar;17(3):236-9. [PubMed: 12026245]
- 60.
- Vignaendra V, Frank AO. Absence status in a patient with hypocalcemia. Electroencephalogr Clin Neurophysiol. 1977 Sep;43(3):429-33. [PubMed: 70344]
- 61.
- Maier JD, Levine SN. Hypercalcemia in the Intensive Care Unit: A Review of Pathophysiology, Diagnosis, and Modern Therapy. J Intensive Care Med. 2015 Jul;30(5):235-52. [PubMed: 24130250]
- 62.
- Swash M, Rowan AJ. Electroencephalographic criteria of hypocalcemia and hypercalcemia. Arch Neurol. 1972 Mar;26(3):218-28. [PubMed: 4536776]
- 63.
- Moure JM. The electroencephalogram in hypercalcemia. Arch Neurol. 1967 Jul;17(1):34-51. [PubMed: 6026171]
- 64.
- Kaplan PW. Reversible hypercalcemic cerebral vasoconstriction with seizures and blindness: a paradigm for eclampsia? Clin Electroencephalogr. 1998 Jul;29(3):120-3. [PubMed: 9660011]
- 65.
- Chen TH, Huang CC, Chang YY, Chen YF, Chen WH, Lai SL. Vasoconstriction as the etiology of hypercalcemia-induced seizures. Epilepsia. 2004 May;45(5):551-4. [PubMed: 15101837]
- 66.
- Rösche J, Sieveking C, Kampf C, Benecke R. Creutzfeldt-Jakob-Like Syndrome due to Hypercalcemic Encephalopathy. Clin EEG Neurosci. 2015 Oct;46(4):327-30. [PubMed: 24973231]
- 67.
- Osorio I, Arafah BM, Mayor C, Troster AI. Plasma glucose alone does not predict neurologic dysfunction in hypoglycemic nondiabetic subjects. Ann Emerg Med. 1999 Mar;33(3):291-8. [PubMed: 10036343]
- 68.
- Blaabjerg L, Juhl CB. Hypoglycemia-Induced Changes in the Electroencephalogram: An Overview. J Diabetes Sci Technol. 2016 Nov;10(6):1259-1267. [PMC free article: PMC5094337] [PubMed: 27464753]
- 69.
- Sejling AS, Kjær TW, Pedersen-Bjergaard U, Diemar SS, Frandsen CS, Hilsted L, Faber J, Holst JJ, Tarnow L, Nielsen MN, Remvig LS, Thorsteinsson B, Juhl CB. Hypoglycemia-associated changes in the electroencephalogram in patients with type 1 diabetes and normal hypoglycemia awareness or unawareness. Diabetes. 2015 May;64(5):1760-9. [PubMed: 25488900]
- 70.
- Bjørgaas M, Sand T, Vik T, Jorde R. Quantitative EEG during controlled hypoglycaemia in diabetic and non-diabetic children. Diabet Med. 1998 Jan;15(1):30-7. [PubMed: 9472861]
- 71.
- Singh BM, Strobos RJ. Epilepsia partialis continua associated with nonketotic hyperglycemia: clinical and biochemical profile of 21 patients. Ann Neurol. 1980 Aug;8(2):155-60. [PubMed: 6775582]
- 72.
- Kamha A. Non Ketotic Hyperosmolar Hyperglycemia presenting as Epilepsia Partialis Continua: An unusual presentation of a common disorder. Libyan J Med. 2008 Jun 01;3(2):111-2. [PMC free article: PMC3074292] [PubMed: 21516157]
- 73.
- Bhatia S, Kapoor AK, Sharma A, Gupta R, Kataria S. Cerebral encephalopathy with extrapontine myelinolysis in a case of postpartum hypernatremia. Indian J Radiol Imaging. 2014 Jan;24(1):57-60. [PMC free article: PMC4028916] [PubMed: 24851006]
- 74.
- Tekgunduz KŞ, Caner I, Eras Z, Taştekin A, Tan H, Dinlen N. Prognostic value of amplitude-integrated electroencephalography in neonates with hypernatremic dehydration. J Matern Fetal Neonatal Med. 2014 May;27(7):658-63. [PubMed: 23919742]
- 75.
- CRAWFORD JD, DODGE P. COMPLICATIONS OF FLUID THERAPY IN NEUROLOGIC DISEASE; WATER INTOXICATION AND HYPERTONIC DEHYDRATION. Pediatr Clin North Am. 1964 Nov;11:1029-52. [PubMed: 14219222]
- 76.
- Itoh N, Matsui N, Matsui S. Periodic lateralized epileptiform discharges in EEG during recovery from hyponatremia: a case report. Clin Electroencephalogr. 1994 Oct;25(4):164-9. [PubMed: 7813098]
- 77.
- Azuma H, Akechi T, Furukawa TA. Absence status associated with focal activity and polydipsia-induced hyponatremia. Neuropsychiatr Dis Treat. 2008 Apr;4(2):495-8. [PMC free article: PMC2518380] [PubMed: 18728738]
- 78.
- Primavera A, Fonti A, Giberti L, Cocito L. Recurrent absence status epilepticus and hyponatremia in a patient with polydipsia. Biol Psychiatry. 1995 Aug 01;38(3):189-91. [PubMed: 7578663]
- 79.
- Gupta SP, Gupta PC, Kumar V, Ahuja MM. Electroencephalographic changes in hypothyroidism. Indian J Med Res. 1972 Jul;60(7):1101-6. [PubMed: 4661458]
- 80.
- Hirano M, Endo M, Kubo T. Triphasic delta waves in a case of hyperthyroidism with psychotic symptoms. Clin Electroencephalogr. 1982 Apr;13(2):97-102. [PubMed: 7094359]
- 81.
- Faigle R, Sutter R, Kaplan PW. Electroencephalography of encephalopathy in patients with endocrine and metabolic disorders. J Clin Neurophysiol. 2013 Oct;30(5):505-16. [PMC free article: PMC3826953] [PubMed: 24084183]
- 82.
- Kollmannsberger A, Kugler J, Scriba PC, Schwarz K. Thyrotoxic encephalopathy. Electroencephalogr Clin Neurophysiol. 1967 Oct;23(4):384. [PubMed: 4167789]
- 83.
- Henchey R, Cibula J, Helveston W, Malone J, Gilmore RL. Electroencephalographic findings in Hashimoto's encephalopathy. Neurology. 1995 May;45(5):977-81. [PubMed: 7746418]
- 84.
- Matsunaga A, Yoneda M. [Hashimoto Encephalopathy]. Brain Nerve. 2021 May;73(5):544-551. [PubMed: 34006687]
- 85.
- Sivaraju A, Gilmore EJ, Wira CR, Stevens A, Rampal N, Moeller JJ, Greer DM, Hirsch LJ, Gaspard N. Prognostication of post-cardiac arrest coma: early clinical and electroencephalographic predictors of outcome. Intensive Care Med. 2015 Jul;41(7):1264-72. [PubMed: 25940963]
- 86.
- Ruijter BJ, Hofmeijer J, Meijer HGE, van Putten MJAM. Synaptic damage underlies EEG abnormalities in postanoxic encephalopathy: A computational study. Clin Neurophysiol. 2017 Sep;128(9):1682-1695. [PubMed: 28753456]
- 87.
- Pressler RM, Boylan GB, Morton M, Binnie CD, Rennie JM. Early serial EEG in hypoxic ischaemic encephalopathy. Clin Neurophysiol. 2001 Jan;112(1):31-7. [PubMed: 11137658]
- 88.
- Awal MA, Lai MM, Azemi G, Boashash B, Colditz PB. EEG background features that predict outcome in term neonates with hypoxic ischaemic encephalopathy: A structured review. Clin Neurophysiol. 2016 Jan;127(1):285-296. [PubMed: 26105684]
- 89.
- Singh TD, Fugate JE, Hocker S, Wijdicks EFM, Aksamit AJ, Rabinstein AA. Predictors of outcome in HSV encephalitis. J Neurol. 2016 Feb;263(2):277-289. [PubMed: 26568560]
- 90.
- Ronne-Engstrom E, Winkler T. Continuous EEG monitoring in patients with traumatic brain injury reveals a high incidence of epileptiform activity. Acta Neurol Scand. 2006 Jul;114(1):47-53. [PubMed: 16774627]
- 91.
- Ianof JN, Anghinah R. Traumatic brain injury: An EEG point of view. Dement Neuropsychol. 2017 Jan-Mar;11(1):3-5. [PMC free article: PMC5619208] [PubMed: 29213487]
- 92.
- Soininen H, Partanen J, Laulumaa V, Pääkkönen A, Helkala EL, Riekkinen PJ. Serial EEG in Alzheimer's disease: 3 year follow-up and clinical outcome. Electroencephalogr Clin Neurophysiol. 1991 Nov;79(5):342-8. [PubMed: 1718706]
- 93.
- Yener GG, Leuchter AF, Jenden D, Read SL, Cummings JL, Miller BL. Quantitative EEG in frontotemporal dementia. Clin Electroencephalogr. 1996 Apr;27(2):61-8. [PubMed: 8681464]
- 94.
- Soikkeli R, Partanen J, Soininen H, Pääkkönen A, Riekkinen P. Slowing of EEG in Parkinson's disease. Electroencephalogr Clin Neurophysiol. 1991 Sep;79(3):159-65. [PubMed: 1714807]
- 95.
- Signorino M, Pucci E, Belardinelli N, Nolfe G, Angeleri F. EEG spectral analysis in vascular and Alzheimer dementia. Electroencephalogr Clin Neurophysiol. 1995 May;94(5):313-25. [PubMed: 7774518]
- 96.
- Scott DF, Heathfield KW, Toone B, Margerison JH. The EEG in Huntington's chorea: a clinical and neuropathological study. J Neurol Neurosurg Psychiatry. 1972 Feb;35(1):97-102. [PMC free article: PMC493983] [PubMed: 4260288]
- 97.
- Markand ON, Panszi JG. The electroencephalogram in subacute sclerosing panencephalitis. Arch Neurol. 1975 Nov;32(11):719-26. [PubMed: 1180740]
- 98.
- Cambier DM, Kantarci K, Worrell GA, Westmoreland BF, Aksamit AJ. Lateralized and focal clinical, EEG, and FLAIR MRI abnormalities in Creutzfeldt-Jakob disease. Clin Neurophysiol. 2003 Sep;114(9):1724-8. [PubMed: 12948802]
- 99.
- Wieser HG, Schindler K, Zumsteg D. EEG in Creutzfeldt-Jakob disease. Clin Neurophysiol. 2006 May;117(5):935-51. [PubMed: 16442343]
- 100.
- Appel S, Cohen OS, Chapman J, Gilat S, Rosenmann H, Nitsan Z, Kahana E, Blatt I. Spatial distribution of abnormal EEG activity in Creutzfeldt-Jakob disease. Neurophysiol Clin. 2021 Jun;51(3):219-224. [PubMed: 33781632]
- 101.
- Canham LJW, Staniaszek LE, Mortimer AM, Nouri LF, Kane NM. Electroencephalographic (EEG) features of encephalopathy in the setting of Covid-19: A case series. Clin Neurophysiol Pract. 2020;5:199-205. [PMC free article: PMC7329683] [PubMed: 32838076]
- 102.
- Pastor J, Vega-Zelaya L, Martín Abad E. Specific EEG Encephalopathy Pattern in SARS-CoV-2 Patients. J Clin Med. 2020 May 20;9(5) [PMC free article: PMC7291269] [PubMed: 32443834]
- 103.
- Vellieux G, Rouvel-Tallec A, Jaquet P, Grinea A, Sonneville R, d'Ortho MP. COVID-19 associated encephalopathy: Is there a specific EEG pattern? Clin Neurophysiol. 2020 Aug;131(8):1928-1930. [PMC free article: PMC7311351] [PubMed: 32615526]
- 104.
- Cecchetti G, Vabanesi M, Chieffo R, Fanelli G, Minicucci F, Agosta F, Tresoldi M, Zangrillo A, Filippi M. Cerebral involvement in COVID-19 is associated with metabolic and coagulation derangements: an EEG study. J Neurol. 2020 Nov;267(11):3130-3134. [PMC free article: PMC7299251] [PubMed: 32556572]
- 105.
- Pasini E, Bisulli F, Volpi L, Minardi I, Tappatà M, Muccioli L, Pensato U, Riguzzi P, Tinuper P, Michelucci R. EEG findings in COVID-19 related encephalopathy. Clin Neurophysiol. 2020 Sep;131(9):2265-2267. [PMC free article: PMC7367805] [PubMed: 32736327]
- 106.
- Gogia B, Thottempudi N, Ajam Y, Singh A, Ghanayem T, Dabi A, Fang X, Masel T, Rai P. EEG Characteristics in COVID-19 Survivors and Non-survivors With Seizures and Encephalopathy. Cureus. 2021 Oct;13(10):e18476. [PMC free article: PMC8502751] [PubMed: 34659916]
- 107.
- Herman ST, Abend NS, Bleck TP, Chapman KE, Drislane FW, Emerson RG, Gerard EE, Hahn CD, Husain AM, Kaplan PW, LaRoche SM, Nuwer MR, Quigg M, Riviello JJ, Schmitt SE, Simmons LA, Tsuchida TN, Hirsch LJ., Critical Care Continuous EEG Task Force of the American Clinical Neurophysiology Society. Consensus statement on continuous EEG in critically ill adults and children, part II: personnel, technical specifications, and clinical practice. J Clin Neurophysiol. 2015 Apr;32(2):96-108. [PMC free article: PMC4434600] [PubMed: 25626777]
Disclosure: Appaji Rayi declares no relevant financial relationships with ineligible companies.
Disclosure: Ria Monica Asuncion declares no relevant financial relationships with ineligible companies.
Disclosure: Kesava Mandalaneni declares no relevant financial relationships with ineligible companies.
- Review Electroencephalography in encephalopathy and encephalitis.[Pract Neurol. 2024]Review Electroencephalography in encephalopathy and encephalitis.Morris H, Kaplan PW, Kane N. Pract Neurol. 2024 Jan 23; 24(1):2-10. Epub 2024 Jan 23.
- Review EEG for Diagnosis and Prognosis of Acute Nonhypoxic Encephalopathy: History and Current Evidence.[J Clin Neurophysiol. 2015]Review EEG for Diagnosis and Prognosis of Acute Nonhypoxic Encephalopathy: History and Current Evidence.Sutter R, Kaplan PW, Valença M, De Marchis GM. J Clin Neurophysiol. 2015 Dec; 32(6):456-64.
- Electroencephalographic findings in anti-N-methyl-d-aspartate receptor encephalitis in children: A series of 12 patients.[Epilepsy Behav. 2018]Electroencephalographic findings in anti-N-methyl-d-aspartate receptor encephalitis in children: A series of 12 patients.Yildirim M, Konuskan B, Yalnizoglu D, Topaloglu H, Erol I, Anlar B. Epilepsy Behav. 2018 Jan; 78:118-123. Epub 2017 Nov 25.
- Review Utility and rationale for continuous EEG monitoring: a primer for the general intensivist.[Crit Care. 2024]Review Utility and rationale for continuous EEG monitoring: a primer for the general intensivist.Bitar R, Khan UM, Rosenthal ES. Crit Care. 2024 Jul 16; 28(1):244. Epub 2024 Jul 16.
- EEG in WNV Neuroinvasive Disease.[J Clin Neurophysiol. 2019]EEG in WNV Neuroinvasive Disease.Parsons AM, Grill MF, Feyissa AM, Britton J, Hocker S, Crepeau A. J Clin Neurophysiol. 2019 Mar; 36(2):135-140.
- Encephalopathic EEG Patterns - StatPearlsEncephalopathic EEG Patterns - StatPearls
Your browsing activity is empty.
Activity recording is turned off.
See more...