This book is distributed under the terms of the Creative Commons Attribution-NonCommercial-NoDerivatives 4.0 International (CC BY-NC-ND 4.0) ( http://creativecommons.org/licenses/by-nc-nd/4.0/ ), which permits others to distribute the work, provided that the article is not altered or used commercially. You are not required to obtain permission to distribute this article, provided that you credit the author and journal.
NCBI Bookshelf. A service of the National Library of Medicine, National Institutes of Health.
StatPearls [Internet]. Treasure Island (FL): StatPearls Publishing; 2024 Jan-.
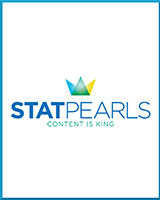
StatPearls [Internet].
Show detailsContinuing Education Activity
Manganese (Mn) toxicity is a well-established result of repeated occupational and nutritional exposures. Though this syndrome is relatively uncommon, its neurotoxicity renders it a feared poison encountered in toxicology. Familiarity with this uncommon toxidrome and its distinct etiologies will ensure prompt recognition and appropriate management early on in its manifestation. This activity describes the evaluation, management, and treatment of manganese toxicity (also known as Manganism), and reviews the role of the interprofessional team in evaluating, treating, managing, and improving care for patients with this toxicity.
Objectives:
- Identify the etiology of manganese toxicity.
- Describe the appropriate evaluation of patients suffering from suspected manganese toxicity.
- Review the management options available for manganese toxicity.
- Outline some interprofessional team strategies for improving care coordination and communication to understand and improve outcomes regarding manganese toxicity.
Introduction
Manganese (Mn) is an environmentally abundant essential metal required for numerous indispensable biochemical processes throughout the human body. Its function arises secondary to its inclusion within protein structures as a cofactor. Without its presence, the human body’s immune function, biochemical regulation of energy consumption, growth potential, coagulation, and hemostatic function, and mechanisms to remove byproducts of aberrant oxidative stress would be significantly diminished.[1]
Optimal nutritional sources of Mn are derived mainly from plants, yet supplementation via vitamins or health products is another notable contribution. Drinking water often contains trace amounts of Mn, with specific cut-offs measured to prevent toxic exposures.[1] Another beneficial source of Mn for infants is via breast milk and formula, which stave off deficiency during their critical developmental period.[2]
Manganese toxicity (manganism) is rarely encountered, yet it is important to recognize the consequence of occupational, accidental, or iatrogenic exposures to Mn. The main toxic effects attributable to this metal are extra-pyramidal side-effects that closely resemble symptoms of Parkinson syndrome.[3] These adverse effects are secondary to its deposition in specific components of the basal ganglia and alteration of dopaminergic neuronal enzyme activity. Other prominent effects include cardiotoxicity, hepatotoxicity, and increased mortality in infants.[4]
The most often documented etiologies for the development of manganism include chronic total parenteral nutrition (TPN) use in critically ill patients, consumption of contaminated well-water, and exposure through work in welding, smelting, and mining. The metal is readily absorbed through the intestinal tract, and absorption is variable based on the level of dietary intake, with biliary and pancreatic metabolism affecting excretion. However, these mechanisms are bypassed with IV administration, owing to the potentiality for manganism with IV preparations containing the essential metal.[2]
Etiology
There are several main mechanisms of manganese toxicity. Manganism was first described in the 19th and 20th centuries in several studies of miners by Couper and Rodier, respectively.[5][6] Along with clear associations with the mining industry, welding, smelting, and the manufacture of batteries also play a key role in toxicity. Individuals involved in metal processing are also critically at risk.[7] These environments are typically associated with an elevated atmospheric metal concentration, which is inhaled, leading to absorption and systemic effects.
The physiology of absorption of metals via inhalation has been postulated to occur via three routes:
- Retrograde axonal transport of Mn particles via olfactory and trigeminal nerve endings
- Direct transport across the pulmonary epithelium for absorption into the circulatory or lymphatic system
- Ingestion via mucociliary clearance and swallowing of respiratory secretions
The size of the particulate matter inhaled also appears to play a role, with medium-size molecules depositing in the lower airways contributing the most to respiratory absorption.[8][9]
Ingested Mn from plant sources is normally filtered through the liver, and the excess is removed to avoid toxicity. In individuals with hepatic dysfunction, patients are at higher risk of Mn toxic accumulation in the bloodstream.[10][11][12] In such patients, it has been found that blood Mn levels and basal ganglia. Mn deposits are significantly correlated with the degree of hepatotoxicity.
Another common source of concern for manganism is drinking water. Well-water specifically can accrue excessive amounts of the metal via breakdown from adjacent rock beds. Postulated means of Mn excess include the presence of anoxic water tables, carbon-rich soil, agricultural fertilizers, and run-off from sites of human deposition of materials rich in Mn (incorrectly disposed batteries, waste from mining facilities, sewer water).[13] In countries with a high net Mn level in water sources, children tend towards deficiencies in IQ scores, memory, reasoning, and general academic achievement.[14] Current US guidelines suggest a measured Mn level in drinking water of fewer than 400 micrograms of Mn per liter.[15][16]
Infants who are breastfed obtain their necessary nutritional requirements of Mn without risk for toxicity. Those who obtain their main dietary intake from infant formulas may receive higher concentrations of the metal beyond that of typically accepted values. This is based on variability in formula ingredients and the reconstitution of formula with potentially Mn-toxic water sources.[17] This risk is important to consider as infants lack significant biliary excretion mechanisms comparable to adults.[4]
Although not much has been published with regards to chronic TPN use, case reports in children and data with respect to safe intravenous dosages of daily Mn suggest intravenous TPN as a potential etiology for manganism.[18][19] Given the lack of removal via pancreatic and biliary secretions, intravenous routes of Mn consumption can easily lead to toxic doses resulting in the feared neuropsychiatric complications described below.[4] Toxicity can be attained via excessive exposure to gasoline, given the preponderance of the chemical methylcyclopentadienyl Mn tricarbonyl (MMT) in gasoline. This compound allows the phasing out of leaded gasoline, but concomitantly puts individuals at risk for manganism in the settings of chronic and excessive exposures.[20]
Epidemiology
There are specific populations most at risk for the development of manganism. This is due to physiological differences between ages and genders, underlying health conditions such as pre-exposure neurocognitive deficits and hepatic dysfunction, and those who are exposed environmentally via water sources or vocation.
The highest at-risk populations are infants and children secondary to decreased functionality of excretion mechanisms, increased ability of the gastrointestinal tract to absorb the metal, and increased permeability of the blood-brain barrier to manganese.[4] The elderly are also postulated to be at increased risk for the acceleration of neurotoxicity beyond that of their age-related proclivity. Specifically, research suggests Mn exposure exacerbates the fine-motor degeneration of study participants beyond that of unexposed peers, one of the known side-effects of both parkinsonism and manganism.[21]
Gender also appears to contribute to differences in Mn prevalence. Women have a higher preponderance of elevated Mn levels across multiple studies from different countries, owing possibly to unknown mechanisms allowing higher absorption from dietary sources.[4][22] This difference is believed to be secondary to lower levels of iron (Fe) present in the blood of females, which has been known to correlate with higher Mn levels. This physiology also explains the increased levels present in pregnant females compared to non-pregnant females.[23]
Countries with lax regulations on metal concentrations within water sources propose a significant risk for their respective populations. Several population studies from Bangladesh illustrate a causal link between Mn consumption from contaminated water and the deleterious effects on adolescent/child cognitive development and academic performance.[16][24]
Pathophysiology
The pathophysiology of manganese toxicity involves complex interactions between exposure and cell protein upregulation and downregulation. Occupational exposure from metalworking, battery manufacturing, mining, consumption of contaminated well water, tap water, infant formula, or TPN leads to excessive absorption and cellular uptake. Specific concomitant metal deficiencies and excess may lead to varying degrees of Mn absorption via the gastrointestinal tract, which preferentially accumulates in the basal ganglia of humans secondary to the location of Mn-specific transporters.
Bodily efflux via biliary and pancreatic elimination may be dysfunctional depending on comorbid disease states or dietary variation. Cellular efflux of Mn then may also be affected, typically via genetic loss-of-function mutations. Alterations in glutamate and glutamine metabolism and use, mitochondrial function, and triggering of cellular apoptosis and necrosis comprise the cellular response to manganism. These biochemical reactions eventually result in the neuropsychiatric manifestations of Mn toxicity. This will be discussed in greater detail in the toxicokinetics section of this article.
Toxicokinetics
Manganese toxicity is unique neurotoxicity that progresses from early psychiatric abnormalities to symptoms reminiscent of Parkinson disease, such as postural deficiencies, bradykinesia, shuffling gait, mask-like facies, micrographia, and speech difficulties. Neurons within the basal ganglia are specifically affected, yielding a parkinsonian syndrome.[25]
The transport within the CNS of Mn is carried out primarily by a family of proteins known as the natural resistance-associated macrophage proteins (Nramps) and the divalent metal transporter-1 (DMT-1).[26][27][28] Transport via these proteins is primarily of the Mn2+ form (the reduced form, which is predominant throughout the body). DMT-1 proteins are represented highly within the basal ganglia, which contributes to the substantially elevated levels of Mn in conditions of excess. It is also the main transporter causing passage across the blood-brain barrier (BBB). These proteins are also expressed more as individual ages.[29]
Transport of Mn3+ (oxidized form) is via transferrin in the bloodstream and transported across cell membranes via transferrin-receptor complexes. Transferrin receptors are located on cells of the BBB, neurons, and other cells of the central nervous system (CNS).[30] This ability of oxidized Mn to be carried via transferrin is one of the means that Fe deficiency contributes to manganism, given that transferrin levels are increased in the setting of iron deficiency.[31] Furthermore, deficiencies in Fe can lead to the up-regulation of Mn absorptive transporters in the intestines such as DMT-1, leading to Mn overload.[32]
Other known important proteins of Mn in the CNS include zinc transport proteins, the dopamine transporter (DAT), calcium channels, choline transporters, and citrate transporters.[20] DAT transporters are highly prevalent in the basal ganglia alongside the DMT-1 protein. DAT expression has been shown to be directly related to environmental exposure of Mn.[33] Mn has been shown to enter cells via store-operated calcium channels (SOCCs), which are present in the endothelial cells in the CNS, thus adding another route of Mn entry into the brain.[34]
Cells utilize channels known as ATPase 13A2, SLC30A10, Ferroportin, and SPCA1 for the export of Mn. Relationships between deficiencies in these efflux channels have been linked to the development of hereditary Mn-induced parkinsonism in recent studies.[35] Similar findings were displayed by the same authors in patients with deficiencies of the SLC30A10 transporter, with a uniquely early presentation of parkinsonism in a 12-year-old.[36] The SLC30A10 protein is expressed in the liver and cells of the basal ganglia. Within the liver, its function to excrete Mn in bile is lost, concomitant with an inability of basal ganglia neurons to remove Mn from its cytosol.[37] Little is known of ferroportin and SPCA1 transport abnormalities.
Manganese cytotoxicity is derived from the triggering of apoptosis in cells accumulating toxic doses of Mn. This discovery was made given similar chemical pathways are elevated in cells undergoing apoptosis, and those with exposure to excess Mn.[38][39][40] As discussed below, the mitochondrion is an organelle that accumulates much of Mn in times of chronic exposure. Mn triggers mitochondrial disruption by promoting the influx of calcium, which leads to the loss of function and terminal permeability of the mitochondrial membrane.[41] Mn also induces the production of reactive oxygen species within mitochondria, resulting in an increase in apoptotic protein expression and an increase in intracellular antioxidant proteins to attenuate the cascade.[42][43]
Mechanisms related to glutamate excess appear to predominate the picture of toxicity in the basal ganglia. Dopaminergic functionality in the globus pallidus appears unrelated to Mn exposure; however, there is some suggestion that down-regulation of dopaminergic transporters occurs in the striatum.[25][44][45][46][47] Despite Parkinson disease being a disease of primarily dopaminergic dysfunction, these main mechanisms of disease are not identical in manganism.
Disruption of transport and glutamine replenishment mechanisms (mainly extracellular reuptake) significantly increases extracellular concentrations of the excitatory neurotransmitter, minimizing neurotransmission in astrocytes, glia, and neighboring neurons of the basal ganglia.[48] This process occurs in a similar fashion with respect to glutamine in the CNS. Reuptake of glutamine is inhibited, and increased turnover is encouraged.[25][49][50] Additionally, the synthesis of glutamine from glutamate by glutamine synthetase (GS) is inhibited, which primarily affects astrocytes in the cerebellum and globus pallidus.[51] This disruption of CNS glutamate/glutamine functionality underlies the main toxic mechanisms of manganism in the CNS.
Mn appears to induce a pro-inflammatory state within glial cells via the production of interleukins and Tumor Necrosis Factor-Alpha (TNF-A).[52] There also appears to be an increased production of nitric oxide and expression of nitric oxide synthase (NOS) in astrocytes, which can lead to variations in astrocyte morphology and development of Alzheimer Type 2 astrocytosis in cells of the globus pallidus.[53][54]
Normal circulating manganese levels in the blood are from 4 to 15 micrograms per liter. Organs with the highest density of Mn include the liver, bone, pancreas, the adrenals, and pituitary glands. The high concentrations in the liver and pancreas are likely related to their roles in excretion.[55] These levels are variable depending on the age of the patient, gender, and exposure risks.
The predominant form of Mn in the human body is in the reduced Mn2+ form. This form of Mn is transported through the circulation via albumin, beta-globulin, and complexed to form stable compounds with weak acids and bases.[56][57] Once inside the CNS, Mn is circulated complexed with citrate and transported across membranes by the citrate transporter.[58] Transport into the CNS directly is postulated to occur via the choroid plexus, given cerebral spinal fluid (CSF) levels obtain Mn faster than CNS parenchymal cells in studies.[59]
Studies have identified the globus pallidus as the main target of toxic Mn accumulation in conditions of excess.[60] This is closely followed by the substantia nigra, thalamus, caudate, putamen, the hippocampus, axonal components, and cortical cells.[61] Mn appears to also have a predilection for astrocyte infiltration.[62] Cellular components most often affected, include mitochondria and nuclei. Chronic exposure leads to the highest magnitude of mitochondria infiltration, whereas acute exposures tend to sequester within the nucleus and nucleolus.[63]
Excretion of Mn occurs primarily via biliary means coincident with a lesser degree of urinary excretion.[64] The half-life (t1/2) of Mn in the basal ganglia has been shown to be approximately several days to a week, whereas, in the bloodstream, the t1/2 is several hours. In rat studies, Mn blood levels reached a steady-state concentration in approximately one month, while CNS levels continued to rise to about two months.[65]
History and Physical
An exposure history alongside idiopathic or early-onset parkinsonism and psychiatric deficits defines manganese toxicity. However, patients rarely present with all Parkinsonian symptoms concomitantly, so a high index of suspicion may be required for patients presenting with piece-meal symptomatology. History-gathering should proceed as standard, elucidating the patient's symptoms and changes from baseline. It is important to involve family members or corroborating parties in the history-taking as psychiatric impairments, and memory fluctuations characterize the early symptoms of manganism.[66] Physical examination consists of an extensive neurological examination, cardiovascular examination, and cognitive function testing. Given the patient's age or accompanying comorbidities, the following task will be to differentiate manganism from an idiopathic Parkinson disease presentation.
Early symptomatology of manganism involves changes in the patient's psychiatric and emotional state. The patient may experience personality changes concomitant with periods of rapid emotional fluctuations, which need to be qualitatively differentiated from the patient's baseline.[66] Psychiatric symptoms include hallucinations and psychosis.[67] Other symptoms reported in a case study from Beijing included memory impairment and insomnia.[68] In a group of California welders, Mn exposure during their everyday duties was analyzed over their work period from 2003 to 2004; neuropsychiatric symptoms developed in these welders included a measured decrease in IQ score, decreased libido, depression, and anxiety.[69]
Early neurological dysfunction was also a hallmark in these patients, characteristic of the basal ganglia/nigrostriatal system involvement. Symptoms discovered included tremor, gait abnormalities, headaches, dysfunctional speech, hyperreflexia, hypertonicity, and tremors.[68] Other acute neurological manifestations in the study introduced above from California included bradykinesia, postural instability, decreased motor dexterity and speed, and olfactory dysfunction.[69] Severe symptoms include the "cock-walk" described in the earliest Mn studies, which is characterized by patients walking on their toes with a forward tilt as they move.[70] Other severe symptoms are worsening tremor and dystonic movements of extremities.
Other systems affected by Mn exposure include the cardiovascular and pulmonary systems. A form of obstructive lung disease formed in approximately one-third of the welders involved in the California study by Bower et. al.[69] Animal studies have shown that overexposure to Mn causes hemodynamic changes, including hypotension and bradycardia, concomitant with a prolonged PR and QRS interval. These studies, however, have not been validated in humans, and often the reverse findings are present (tachycardia, hypertension, shortened PR interval).[71][72]
Focusing on the patient's social history can confirm the diagnosis in patients suspected of having manganism. Patients will have an occupational history consistent with exposure, including welding, metal manufacturing, mining, battery manufacturing, smelting, those intimately exposed to gasoline combustion, and steelworkers.[4][73] Inquire about personal protective equipment (PPE) the patients utilize daily, as those without appropriate respiratory protection are more at risk for the development of symptoms.[69] Delve into the patient's living conditions. If the patient recently immigrated or is traveling from a country with sub-standard public drinking water conditions, they may have been chronically ingesting high amounts of Mn-toxic water.[15][16] Identify patients whose water source is well-water, as their well may be spoiled by leaching from mineral-laden soil.[13][14]
Query the patient about drug use, as recent studies have shown, bath salts use intravenously puts patients at risk for Mn overload due to poor purification processes.[74] Chronic intravenous TPN also puts patients at risk of manganism, thus inquiring about recent hospitalizations may be helpful.
Further discussion of patients' past medical histories should include known or presumed hepatic insufficiency, as patients with decreased liver function are at increased risk for developing manganism.[75] Male patients who note difficulty conceiving with an unremarkable maternal work-up coincident with a known occupational history may also be a clue, as Mn toxicity results in decreased fertility.[76]
Evaluation
The determination of optimal serum biomarkers for manganese toxicity has proven difficult. Given that Mn is primarily removed via biliary mechanisms, urinary levels are significantly low at baseline and do not reliably correlate with serum toxic levels.[77] Due to the short half-life of Mn, blood levels are also difficult to quantify, and chronic exposures do not consistently yield expected and clinically meaningful results.[65] Saliva sampling also yields significant variations in those exposed to the same levels of Mn, making it a non-specific marker for toxicity.[78] Further, studies utilizing hair and nail concentrations of Mn as markers for toxicity again show inconsistent results, with some studies suggesting a reliable correlation and others showing a lack of reliability.[79][80][21] Grashow et al. suggested that nail sampling may be useful for quantifying exposure over a period of several months, but is not highly beneficial beyond.[81] More recent research suggests a reasonable correlation between Mn exposure and hair and nails Mn levels while supplementing the notion that salival levels do not correlate well with exposures.[82]
Promising biomarker capability was illustrated by measuring serum levels of both Mn and Fe together. Neil et al. utilized the ratio of Mn to Fe in both plasma (pMIR) and erythrocytes (eMIR) with respect to airborne exposure to Mn.[21]
Bone is a natural Mn sink, thus making it an ideal organ to study the effects of exposure to the natural metal. Liu et al. developed a novel testing system to quantify the Mn content in bone, called neutron activation analysis. They were able to reliably quantify Mn concentrations in the bones of hands, suggesting the viability of future studies with this methodology.[83]
In the context of recent or on-going exposure, MRI has also proven to be clinically useful alongside novel bone scanning. Mn, as described previously, preferentially accumulates within basal ganglia structures. The globus pallidus can be specifically analyzed by calculating T1-weighted signals and comparing it to frontal cortical white matter, creating what the authors called the pallidal index. Mn exposure can then be related to the degree of signal intensity as quantified by an increased pallidal index.[60][84]
Notably, these studies did not correlate with biological outcomes, as none of the analyzed population displayed clinical effects of manganism. These same researchers also studied the use of spectroscopy enhanced magnetic resonance studies. This technology allows the quantification of molecule concentrations in any portion of the brain. Magnetic resonance spectroscopy illustrated increased levels of GABA in the thalamus and basal ganglia of patients.[84]
The latest research presented at the 2016 conference on Mn neurotoxicity described new methodologies of identification of manganism, including the use of fMRI, PET scans, and new methods of using blood Mn and ferritin levels to detect globus pallidus accumulation of toxic metal concentrations.[85][86][85][87]
In summary, diagnosis requires a high clinical suspicion alongside recognition of the risk factors placing patients at risk for manganism. Ideal evaluation for the determination of Mn toxicity includes a team-based approach, based on early recognition and outpatient referral to neurology for definitive care. Early consultation with a clinical toxicologist may aid in the identification of the etiology for the patient's symptoms. Usage of MRI or serum-based studies should be done at the request of specialists familiar with toxicity and the latest research.
Treatment / Management
Treatment of manganism toxicity involves the treatment of the acute threats from toxicity and the management of chronic exposure. The most accessible form of treatment for manganism is the removal of the patient from the source exposure, whether the source is occupational, environmental, or iatrogenic.
Chelation therapy for manganism involves the use of CaNa2EDTA (EDTA) and Para-aminosalicylic Acid (PAS). The use of EDTA has been shown to effectively increase urine concentration of Mn and decrease blood levels of Mn. These findings did not coincide with the finding of decreased observed clinical toxicity, owing to the chronic nature of toxicity and incomplete reversibility.[68] However, EDTA prevents further Mn from crossing the blood-brain barrier, deactivating its ability to enter into the CNS to exert its toxic effects.[88][89][90] Unfortunately, EDTA has a poor bioavailability for brain parenchyma and does not appear to be able to completely reverse the toxic effects of Mn. Thus, in patients with chronic toxicity or advanced manganism, chelation therapy is likely to not reverse the significant clinical deterioration.[91]
Another chelation molecule, Para-aminosalicylic acid (PAS) - typically used as an anti-tuberculosis medication - has shown clinical benefit for use in patients with manganism. PAS and its metabolites concentrate within the choroid plexus, brain parenchyma, and CSF, making it an ideal chelator for this toxicity.[92] PAS has the additional benefit of chelating both the Mn2+ and Mn3+ moieties.[93] The anti-inflammatory properties of the salicylic acid component may also produce a beneficial effect, as studies have illustrated neuroprotective benefits from the use of other nonsteroidal anti-inflammatory medications in Alzheimer disease, Parkinson disease, and amyotrophic lateral sclerosis.[94][95]
Previously attempted treatments included Levodopa (L-dopa) supplementation. Initial studies supported a benefit with L-dopa administration, but more recent data suggests prolonged treatment results in poor clinical response.[96] Also, after the cessation of L-dopa use, patients had a progression of disease despite initial therapy.[97]
Several vitamins and supplements appear to augment clinical response and provide a means of prevention for patients. In addition to chelation therapy, iron supplementation was shown to improve neurological symptom improvement compared to a treatment group that received chelation alone.[5] Vitamin E was shown to prevent the supposed oxidative stress brought on by Mn-induced toxicity.[43] Glutathione and N-acetylcysteine have also shown to be beneficial in vitro for decreasing the downstream effects of Mn-induced cellular toxicity.[98] Several plants and plant extracts have also been shown to be beneficial, including Silymarin, Acai, Lycopene, Nicotine, and Melissa officinalis.[99][100][101][102]
Newer therapies being investigated include the use of taurine and Rasagiline. Taurine use decreases the toxic effects of Mn in vitro, mostly through the preservation of mitochondrial functionality in CNS tissues.[103][104] Taurine also appears to ameliorate the learning and memory impairments associated with chronic manganism.[105] Rasagiline is a monoamine oxidase inhibitor (MAO-I) used to block the metabolism of dopamine in patients with Parkinson disease. Rasagiline appeared to offer a benefit through protection from reactive oxygen species created by Mn toxicity.[106]
Differential Diagnosis
Idiopathic Parkinson disease: It is characterized by bradykinesia, shuffling gait, postural instability, and resting pill-rolling tremor; this disease is most clinically similar to manganese toxicity. Distinguishing differences between the two syndromes include less prominent tremors in manganism, the reversal of psychiatric symptom onset (later in Parkinson disease, earlier in manganism), the age of onset is typically younger in manganism as compared to Parkinson disease and clear occupational correlation with manganism.[107]
Dementia with Lewy bodies: Patients with dementia with Lewy bodies develop a parkinsonian syndrome concomitant with many cognitive dysfunctions in the 5th to 6th decade of life. Early cognitive deficits include short term memory difficulties, multi-tasking deficits, and visuospatial deficiencies. These symptoms will develop alongside visual hallucinations and motor difficulties consistent with Parkinson disease. This disease process can be differentiated from both Parkinson disease and manganism by the presence of characteristic hallucinations and concurrent development of neuropsychiatric and motor dysfunction.[108]
Frontotemporal dementia: This dementia is characterized by several different phenotypes, which include a behavioral/executive dysfunction type and two primary language deficiency types. Clinical features of the behavioral subtype include disinhibition, apathy, compulsive behaviors, hyper-orality, and overall diminished executive function skills. Features of the language dysfunction variants include difficulties with daily life due to speech deficits, aphasia, speech apraxia, and other speech and comprehension impairments. This syndrome can easily be mistaken for manganism in its early stages if behavioral symptoms predominate, given manganism’s early neuropsychiatric manifestations. An adequate history-taking can differentiate these two syndromes.[109]
Essential tremor: Essential tremor is a chronic, progressive neurologic disorder characterized by a high-frequency action tremor. This disorder can affect the neck, jaw, and other body regions alongside that of the arms and hands. Manganism will produce tremors early on in the disease process, typically after the development of psychiatric dysfunction. Differentiation of essential tremor from manganism involves elucidating occupational history, lack of family history, and identification of concomitant neuropsychiatric features in Mn toxicity.[110]
Multiple system atrophy: As its name denotes, multiple system atrophy (MSA) is a complex disorder characterized by autonomic dysfunction, orthostatic hypotension, cerebellar dysfunction, parkinsonian symptoms unresponsive to L-dopa, and earlier age in onset (greater than 30 years old). This neurologic disease can be distinguished from manganism due to its multiple concomitant system dysfunction, autonomic dysfunction, and cerebellar findings.[111]
Corticobasal degeneration: As the name denotes, this is a disease that affects predominantly the brain cortices and basal ganglia, resulting in apraxia, hemineglect symptoms, alterations in behavior and emotion, rigidity, bradykinesia, and myoclonus. This is another syndrome very similar in presentation to manganism; however, it can be differentiated based on history and chronicity in relation to exposure risk for Mn.[112]
Progressive supranuclear palsy: Progressive supranuclear palsy (PSP) is an L-dopa responsive parkinsonian syndrome similar to corticobasilar degeneration. Features of this syndrome include vertical supranuclear palsy and parkinsonian symptoms. These syndromes can be differentiated from manganism by their responsiveness to L-dopa therapy.[112]
Drug-induced Parkinsonism: The most common cause of parkinsonian symptoms, drug-induced parkinsonism, is an often under-appreciated disease process. Common precipitants include antipsychotic medications, anti-emetics, anti-motility agents, some rarely prescribed calcium channel blockers, and dopamine depleting medications. A clear and concise medication history can lead to the diagnosis in these cases.[113]
Methcathinone, this substance is a psychoactive stimulant used recreationally. Case reports have suggested its chronic use is linked to the development of Mn toxicity, substantiated by the evidence of parkinsonian features and MRI correlate abnormalities in the basal ganglia. Drug use and medication history may lead to the discovery of this diagnosis.[114]
Other neurodegenerative disorders, including Huntington disease, repeated and chronic head trauma, several infections such as syphilis, HIV, progressive multifocal leukoencephalopathy, structural brain disorders such as intraparenchymal masses or hydrocephalus, and metabolic disorders such as Wilson disease, hypoparathyroidism, chronic hepatic failure, and hemochromatosis.[115]
Prognosis
There exists a dearth of studies on the prognosis of patients suffering ongoing manganese exposure and of patients who have successfully removed themselves from exposure after the development of toxicity. What little literature exists suggests that, with removal from source exposure, recovery in some neuropsychiatric arenas is possible while improbable in others. Bouchard et al. followed a group of workers exposed to Mn over the course of a decade and a half and observed signs of recovery approximately 14 years after their documented exposure. Specific deficits that remained included poor performance on simple and alternating movements, drawing ability, and diminished hand stability.[116] These findings are corroborated by prior studies.[117][118] Bouchard et al. also found a trend towards more feelings of anger, hostility, confusion, and a sensation of fatigue. This finding was correlated with greater cumulative doses of Mn. Finally, their group identified a relationship between Mn exposure and respiratory tract pathology, possibly secondary to the induced inflammatory state.[116]
As previously mentioned, older patients who are exposed to Mn often develop more significant toxicity than younger patients, owing to possible pre-exposure neurological degenerative changes. This is postulated to lead to the observed age-related cognitive deficits that persist after Mn exposures cease. Research by Bouchard et al. in 2005 showed that individuals who were exposed that were at least greater than or equal to 45 years old had persistent cognitive deficits after cessation of toxic exposure to Mn.[119][120]
In another follow-up study by Roels et al., workers from a battery manufacturing plant were followed for eight years after cessation of Mn exposure. Variables measured included hand steadiness and reaction time. Analysis of their cohort yielded no improved steadiness of the hands and no improvement in reaction time. The group did, however, observe an improvement in hand-forearm movements beyond that of their baseline, which suggested some partial recovery from their toxicity.[121] Lucchini et al. measured improvement in Mn toxic patients five years after exposure was decreased and found similar results during and after their exposures.[122] This lack of improvement may be due to the fact that exposure was reduced but not definitively eliminated.
The trend following cessation of Mn exposure appears to be that of mostly recovery, with residual deficits remaining in the behavioral and neurologic domains. This lends credence to the mainstay of therapy, which is to abstain from any further activity, which puts individuals at risk for further toxicity. Overall, the prognosis is more related to morbidity than mortality and is overall favorable once removed from the exposure setting. More research will be needed to further delineate the expected course, especially with the addition of new treatment modalities such as supplementation and chelators.
Complications
Please refer to the history and physical portion of this article to review the complications of manganism.
Consultations
As with any instance where a toxic poisoning is suspected, it is prudent to touch base with the local poison control center for recommendations and treatment direction. Decisions with regards to disposition, treatment guidance, and further consultation recommendations may be provided. Once the call has been placed, request to discuss your patient's case with the on-call Toxicologist for optimal treatment guidance.
Ideally, once the diagnosis is confirmed or suspected, neurological services should be consulted in tandem, given the similarities to various different neurological diseases and advanced treatment therapies. This will ensure that the proper imaging modalities to confirm and rule out concomitant differential diagnoses are properly obtained.
Deterrence and Patient Education
An understanding of the exposure risk for individual populations is key to decreasing the risk of Mn toxicity. Current acceptable levels of Mn in drinking water are 400 micrograms per liter, and a tolerable intake of Mn from dietary sources should not exceed 60 micrograms/kg.[15] Knowledge of acceptable levels can assist patients in guiding the treatment of their home sources. Also, for providers in the third world or developing countries, this can assist in the guidance of treatment for children and adults who present with characteristic signs and symptoms of the disease.[13][14][15][16]
The possibility of occupational exposures should encourage workers to raise alarms to their managerial staff should OSHA infractions become evident. Patients should advocate for current standards in respirators while welding or operating in industrial manufacturing. Patients should compare their current workplace standards with those set by OSHA to ensure they are not at risk of developing manganism or other toxic metal exposures.[66]
Patients and families must be aware of the potential for infant toxicity. Reconstituting formula with Mn-laden water increases the risk dramatically in these patients due to naive Mn excretory function. Female patients with a history of metrorrhagia, menorrhagia, or another condition predisposing them to Fe deficiency should also have a heightened awareness, given their propensity for developing manganism secondary to elevated transferrin expression. Those on parenteral nutritional supplementation such as TPN should also be aware, given the by-passing of normal homeostatic regulatory functions that keep intestinal absorption low as well as those affected by the hepatic and pancreatic disease.[3][4][19][23][123]
In the setting of the above risk factors and exposure history, identification of the characteristic symptomatology should raise the alarm for Mn. Early psychiatric symptoms followed by neurologic deterioration similar to that of Parkinson disease are the hallmark of toxicity. Treatment with avoidance of further exposure, systemic chelators, and vitamin and nutritional supplementation will then ensure, likely in consultation with neurology and toxicology services.
The prognosis for patients exposed to Mn is generally favorable among the few studies recorded. Trends towards the partial recovery of most symptoms are common, with residual deficits in those of more advanced age at the time of onset and motor and behavioral deficits generally.
Enhancing Healthcare Team Outcomes
Patients suffering from manganism present a complex interplay between neurological and toxicological specialties. Their unique neuropsychiatric presentation can initially masquerade as many different clinical entities. Their symptoms also present the need for a multifaceted care team to adequately provide quality care. Medical toxicologists and neurologists will be required in order to accurately diagnose and treat this condition, with special attention to items on the differential diagnosis to ensure the appropriate treatment can be started quickly.
Skilled nursing and rehabilitative services will be required to manage early psychiatric disease and late parkinsonian dysfunction, respectively. Clinical pharmacists will be required to counsel the treatment team on management options and the latest treatment guidelines. Assuming ideal treatment teams are assembled in a timely fashion, the prognosis is generally favorable, with a trend towards the partial recovery of lost neuropsychiatric functionality. Thus, a multifaceted team-based approach is ideal for the diagnosis, evaluation, and treatment of this rare and unique toxicity.
Review Questions
References
- 1.
- Aschner M, Erikson K. Manganese. Adv Nutr. 2017 May;8(3):520-521. [PMC free article: PMC5421128] [PubMed: 28507016]
- 2.
- Horning KJ, Caito SW, Tipps KG, Bowman AB, Aschner M. Manganese Is Essential for Neuronal Health. Annu Rev Nutr. 2015;35:71-108. [PMC free article: PMC6525788] [PubMed: 25974698]
- 3.
- Pfalzer AC, Bowman AB. Relationships Between Essential Manganese Biology and Manganese Toxicity in Neurological Disease. Curr Environ Health Rep. 2017 Jun;4(2):223-228. [PMC free article: PMC5515274] [PubMed: 28417441]
- 4.
- O'Neal SL, Zheng W. Manganese Toxicity Upon Overexposure: a Decade in Review. Curr Environ Health Rep. 2015 Sep;2(3):315-28. [PMC free article: PMC4545267] [PubMed: 26231508]
- 5.
- Tuschl K, Mills PB, Clayton PT. Manganese and the brain. Int Rev Neurobiol. 2013;110:277-312. [PubMed: 24209443]
- 6.
- RODIER J. Manganese poisoning in Moroccan miners. Br J Ind Med. 1955 Jan;12(1):21-35. [PMC free article: PMC1037597] [PubMed: 14351643]
- 7.
- Roth JA. Homeostatic and toxic mechanisms regulating manganese uptake, retention, and elimination. Biol Res. 2006;39(1):45-57. [PubMed: 16629164]
- 8.
- Pityn P, Chamberlain MJ, Fraser TM, King M, Morgan WK. The topography of particle deposition in the human lung. Respir Physiol. 1989 Oct;78(1):19-29. [PubMed: 2813985]
- 9.
- Yu IJ, Kim KJ, Chang HK, Song KS, Han KT, Han JH, Maeng SH, Chung YH, Park SH, Chung KH, Han JS, Chung HK. Pattern of deposition of stainless steel welding fume particles inhaled into the respiratory systems of Sprague-Dawley rats exposed to a novel welding fume generating system. Toxicol Lett. 2000 Jul 27;116(1-2):103-11. [PubMed: 10906427]
- 10.
- Krieger D, Krieger S, Jansen O, Gass P, Theilmann L, Lichtnecker H. Manganese and chronic hepatic encephalopathy. Lancet. 1995 Jul 29;346(8970):270-4. [PubMed: 7630246]
- 11.
- Hauser RA, Zesiewicz TA, Martinez C, Rosemurgy AS, Olanow CW. Blood manganese correlates with brain magnetic resonance imaging changes in patients with liver disease. Can J Neurol Sci. 1996 May;23(2):95-8. [PubMed: 8738919]
- 12.
- Burkhard PR, Delavelle J, Du Pasquier R, Spahr L. Chronic parkinsonism associated with cirrhosis: a distinct subset of acquired hepatocerebral degeneration. Arch Neurol. 2003 Apr;60(4):521-8. [PubMed: 12707065]
- 13.
- Kullar SS, Shao K, Surette C, Foucher D, Mergler D, Cormier P, Bellinger DC, Barbeau B, Sauvé S, Bouchard MF. A benchmark concentration analysis for manganese in drinking water and IQ deficits in children. Environ Int. 2019 Sep;130:104889. [PubMed: 31200154]
- 14.
- Oulhote Y, Mergler D, Barbeau B, Bellinger DC, Bouffard T, Brodeur MÈ, Saint-Amour D, Legrand M, Sauvé S, Bouchard MF. Neurobehavioral function in school-age children exposed to manganese in drinking water. Environ Health Perspect. 2014 Dec;122(12):1343-50. [PMC free article: PMC4256698] [PubMed: 25260096]
- 15.
- Ljung K, Vahter M. Time to re-evaluate the guideline value for manganese in drinking water? Environ Health Perspect. 2007 Nov;115(11):1533-8. [PMC free article: PMC2072823] [PubMed: 18007980]
- 16.
- Wasserman GA, Liu X, Parvez F, Ahsan H, Levy D, Factor-Litvak P, Kline J, van Geen A, Slavkovich V, LoIacono NJ, Cheng Z, Zheng Y, Graziano JH. Water manganese exposure and children's intellectual function in Araihazar, Bangladesh. Environ Health Perspect. 2006 Jan;114(1):124-9. [PMC free article: PMC1332667] [PubMed: 16393669]
- 17.
- Frisbie SH, Mitchell EJ, Roudeau S, Domart F, Carmona A, Ortega R. Manganese levels in infant formula and young child nutritional beverages in the United States and France: Comparison to breast milk and regulations. PLoS One. 2019;14(11):e0223636. [PMC free article: PMC6830775] [PubMed: 31689314]
- 18.
- Khan A, Hingre J, Dhamoon AS. Manganese Neurotoxicity as a Complication of Chronic Total Parenteral Nutrition. Case Rep Neurol Med. 2020;2020:9484028. [PMC free article: PMC7196137] [PubMed: 32373376]
- 19.
- Takagi Y, Okada A, Sando K, Wasa M, Yoshida H, Hirabuki N. Evaluation of indexes of in vivo manganese status and the optimal intravenous dose for adult patients undergoing home parenteral nutrition. Am J Clin Nutr. 2002 Jan;75(1):112-8. [PubMed: 11756068]
- 20.
- Chen P, Chakraborty S, Mukhopadhyay S, Lee E, Paoliello MM, Bowman AB, Aschner M. Manganese homeostasis in the nervous system. J Neurochem. 2015 Aug;134(4):601-10. [PMC free article: PMC4516557] [PubMed: 25982296]
- 21.
- Cowan DM, Zheng W, Zou Y, Shi X, Chen J, Rosenthal FS, Fan Q. Manganese exposure among smelting workers: relationship between blood manganese-iron ratio and early onset neurobehavioral alterations. Neurotoxicology. 2009 Nov;30(6):1214-22. [PMC free article: PMC3983997] [PubMed: 19963104]
- 22.
- Lee JW, Lee CK, Moon CS, Choi IJ, Lee KJ, Yi SM, Jang BK, Yoon BJ, Kim DS, Peak D, Sul D, Oh E, Im H, Kang HS, Kim J, Lee JT, Kim K, Park KL, Ahn R, Park SH, Kim SC, Park CH, Lee JH. Korea National Survey for Environmental Pollutants in the Human Body 2008: heavy metals in the blood or urine of the Korean population. Int J Hyg Environ Health. 2012 Jul;215(4):449-57. [PubMed: 22341685]
- 23.
- Oulhote Y, Mergler D, Bouchard MF. Sex- and age-differences in blood manganese levels in the U.S. general population: national health and nutrition examination survey 2011-2012. Environ Health. 2014 Oct 24;13:87. [PMC free article: PMC4271487] [PubMed: 25342305]
- 24.
- Khan K, Wasserman GA, Liu X, Ahmed E, Parvez F, Slavkovich V, Levy D, Mey J, van Geen A, Graziano JH, Factor-Litvak P. Manganese exposure from drinking water and children's academic achievement. Neurotoxicology. 2012 Jan;33(1):91-7. [PMC free article: PMC3282923] [PubMed: 22182530]
- 25.
- Sidoryk-Wegrzynowicz M, Aschner M. Manganese toxicity in the central nervous system: the glutamine/glutamate-γ-aminobutyric acid cycle. J Intern Med. 2013 May;273(5):466-77. [PMC free article: PMC3633698] [PubMed: 23360507]
- 26.
- Aschner M, Gannon M. Manganese (Mn) transport across the rat blood-brain barrier: saturable and transferrin-dependent transport mechanisms. Brain Res Bull. 1994;33(3):345-9. [PubMed: 8293318]
- 27.
- Aschner M, Vrana KE, Zheng W. Manganese uptake and distribution in the central nervous system (CNS). Neurotoxicology. 1999 Apr-Jun;20(2-3):173-80. [PubMed: 10385881]
- 28.
- Fitsanakis VA, Au C, Erikson KM, Aschner M. The effects of manganese on glutamate, dopamine and gamma-aminobutyric acid regulation. Neurochem Int. 2006 May-Jun;48(6-7):426-33. [PubMed: 16513220]
- 29.
- Ke Y, Chang YZ, Duan XL, Du JR, Zhu L, Wang K, Yang XD, Ho KP, Qian ZM. Age-dependent and iron-independent expression of two mRNA isoforms of divalent metal transporter 1 in rat brain. Neurobiol Aging. 2005 May;26(5):739-48. [PubMed: 15708449]
- 30.
- Moos T, Morgan EH. Transferrin and transferrin receptor function in brain barrier systems. Cell Mol Neurobiol. 2000 Feb;20(1):77-95. [PubMed: 10690503]
- 31.
- Ye Q, Park JE, Gugnani K, Betharia S, Pino-Figueroa A, Kim J. Influence of iron metabolism on manganese transport and toxicity. Metallomics. 2017 Aug 16;9(8):1028-1046. [PMC free article: PMC5559333] [PubMed: 28620665]
- 32.
- Davidson LA, Lönnerdal B. Specific binding of lactoferrin to brush-border membrane: ontogeny and effect of glycan chain. Am J Physiol. 1988 Apr;254(4 Pt 1):G580-5. [PubMed: 2833117]
- 33.
- Huang CC, Weng YH, Lu CS, Chu NS, Yen TC. Dopamine transporter binding in chronic manganese intoxication. J Neurol. 2003 Nov;250(11):1335-9. [PubMed: 14648150]
- 34.
- Kim YV, Di Cello F, Hillaire CS, Kim KS. Differential Ca2+ signaling by thrombin and protease-activated receptor-1-activating peptide in human brain microvascular endothelial cells. Am J Physiol Cell Physiol. 2004 Jan;286(1):C31-42. [PubMed: 12944324]
- 35.
- Tuschl K, Clayton PT, Gospe SM, Gulab S, Ibrahim S, Singhi P, Aulakh R, Ribeiro RT, Barsottini OG, Zaki MS, Del Rosario ML, Dyack S, Price V, Rideout A, Gordon K, Wevers RA, Chong WK, Mills PB. Syndrome of hepatic cirrhosis, dystonia, polycythemia, and hypermanganesemia caused by mutations in SLC30A10, a manganese transporter in man. Am J Hum Genet. 2012 Mar 09;90(3):457-66. [PMC free article: PMC3309187] [PubMed: 22341972]
- 36.
- Tuschl K, Mills PB, Parsons H, Malone M, Fowler D, Bitner-Glindzicz M, Clayton PT. Hepatic cirrhosis, dystonia, polycythaemia and hypermanganesaemia--a new metabolic disorder. J Inherit Metab Dis. 2008 Apr;31(2):151-63. [PubMed: 18392750]
- 37.
- Butterworth RF. Parkinsonism in cirrhosis: pathogenesis and current therapeutic options. Metab Brain Dis. 2013 Jun;28(2):261-7. [PubMed: 23086199]
- 38.
- Roth JA, Horbinski C, Higgins D, Lein P, Garrick MD. Mechanisms of manganese-induced rat pheochromocytoma (PC12) cell death and cell differentiation. Neurotoxicology. 2002 Jul;23(2):147-57. [PubMed: 12224755]
- 39.
- Latchoumycandane C, Anantharam V, Kitazawa M, Yang Y, Kanthasamy A, Kanthasamy AG. Protein kinase Cdelta is a key downstream mediator of manganese-induced apoptosis in dopaminergic neuronal cells. J Pharmacol Exp Ther. 2005 Apr;313(1):46-55. [PubMed: 15608081]
- 40.
- Kitazawa M, Anantharam V, Yang Y, Hirata Y, Kanthasamy A, Kanthasamy AG. Activation of protein kinase C delta by proteolytic cleavage contributes to manganese-induced apoptosis in dopaminergic cells: protective role of Bcl-2. Biochem Pharmacol. 2005 Jan 01;69(1):133-46. [PubMed: 15588722]
- 41.
- Gavin CE, Gunter KK, Gunter TE. Manganese and calcium efflux kinetics in brain mitochondria. Relevance to manganese toxicity. Biochem J. 1990 Mar 01;266(2):329-34. [PMC free article: PMC1131135] [PubMed: 2317189]
- 42.
- Martinez-Finley EJ, Gavin CE, Aschner M, Gunter TE. Manganese neurotoxicity and the role of reactive oxygen species. Free Radic Biol Med. 2013 Sep;62:65-75. [PMC free article: PMC3713115] [PubMed: 23395780]
- 43.
- Cordova FM, Aguiar AS, Peres TV, Lopes MW, Gonçalves FM, Pedro DZ, Lopes SC, Pilati C, Prediger RD, Farina M, Erikson KM, Aschner M, Leal RB. Manganese-exposed developing rats display motor deficits and striatal oxidative stress that are reversed by Trolox. Arch Toxicol. 2013 Jul;87(7):1231-44. [PMC free article: PMC6543098] [PubMed: 23385959]
- 44.
- Rouse ST, Marino MJ, Bradley SR, Awad H, Wittmann M, Conn PJ. Distribution and roles of metabotropic glutamate receptors in the basal ganglia motor circuit: implications for treatment of Parkinson's disease and related disorders. Pharmacol Ther. 2000 Dec;88(3):427-35. [PubMed: 11337032]
- 45.
- Guilarte TR. Manganese and Parkinson's disease: a critical review and new findings. Cien Saude Colet. 2011 Nov;16(11):4549-66. [PubMed: 22124833]
- 46.
- McDougall SA, Reichel CM, Farley CM, Flesher MM, Der-Ghazarian T, Cortez AM, Wacan JJ, Martinez CE, Varela FA, Butt AE, Crawford CA. Postnatal manganese exposure alters dopamine transporter function in adult rats: Potential impact on nonassociative and associative processes. Neuroscience. 2008 Jun 23;154(2):848-60. [PMC free article: PMC2517246] [PubMed: 18485605]
- 47.
- Brouillet EP, Shinobu L, McGarvey U, Hochberg F, Beal MF. Manganese injection into the rat striatum produces excitotoxic lesions by impairing energy metabolism. Exp Neurol. 1993 Mar;120(1):89-94. [PubMed: 8477830]
- 48.
- Lee ES, Sidoryk M, Jiang H, Yin Z, Aschner M. Estrogen and tamoxifen reverse manganese-induced glutamate transporter impairment in astrocytes. J Neurochem. 2009 Jul;110(2):530-44. [PMC free article: PMC3920654] [PubMed: 19453300]
- 49.
- Nissen-Meyer LS, Popescu MC, Hamdani el H, Chaudhry FA. Protein kinase C-mediated phosphorylation of a single serine residue on the rat glial glutamine transporter SN1 governs its membrane trafficking. J Neurosci. 2011 Apr 27;31(17):6565-75. [PMC free article: PMC6622677] [PubMed: 21525297]
- 50.
- Sidoryk-Wegrzynowicz M, Lee ES, Ni M, Aschner M. Manganese-induced downregulation of astroglial glutamine transporter SNAT3 involves ubiquitin-mediated proteolytic system. Glia. 2010 Dec;58(16):1905-12. [PMC free article: PMC6996538] [PubMed: 20737472]
- 51.
- Martinez-Hernandez A, Bell KP, Norenberg MD. Glutamine synthetase: glial localization in brain. Science. 1977 Mar 25;195(4284):1356-8. [PubMed: 14400]
- 52.
- Filipov NM, Dodd CA. Role of glial cells in manganese neurotoxicity. J Appl Toxicol. 2012 May;32(5):310-7. [PubMed: 22120544]
- 53.
- Hazell AS, Normandin L, Norenberg MD, Kennedy G, Yi JH. Alzheimer type II astrocytic changes following sub-acute exposure to manganese and its prevention by antioxidant treatment. Neurosci Lett. 2006 Apr 03;396(3):167-71. [PubMed: 16384640]
- 54.
- Moreno JA, Sullivan KA, Carbone DL, Hanneman WH, Tjalkens RB. Manganese potentiates nuclear factor-kappaB-dependent expression of nitric oxide synthase 2 in astrocytes by activating soluble guanylate cyclase and extracellular responsive kinase signaling pathways. J Neurosci Res. 2008 Jul;86(9):2028-38. [PMC free article: PMC4618683] [PubMed: 18335517]
- 55.
- Zhang LL, Lu L, Pan YJ, Ding CG, Xu DY, Huang CF, Pan XF, Zheng W. Baseline blood levels of manganese, lead, cadmium, copper, and zinc in residents of Beijing suburb. Environ Res. 2015 Jul;140:10-7. [PMC free article: PMC4492836] [PubMed: 25836720]
- 56.
- Harris WR, Chen Y. Electron paramagnetic resonance and difference ultraviolet studies of Mn2+ binding to serum transferrin. J Inorg Biochem. 1994 Apr;54(1):1-19. [PubMed: 8151309]
- 57.
- Reaney SH, Kwik-Uribe CL, Smith DR. Manganese oxidation state and its implications for toxicity. Chem Res Toxicol. 2002 Sep;15(9):1119-26. [PubMed: 12230404]
- 58.
- Yokel RA. Manganese flux across the blood-brain barrier. Neuromolecular Med. 2009;11(4):297-310. [PubMed: 19902387]
- 59.
- Schmitt C, Strazielle N, Richaud P, Bouron A, Ghersi-Egea JF. Active transport at the blood-CSF barrier contributes to manganese influx into the brain. J Neurochem. 2011 May;117(4):747-56. [PubMed: 21395586]
- 60.
- Jiang Y, Zheng W, Long L, Zhao W, Li X, Mo X, Lu J, Fu X, Li W, Liu S, Long Q, Huang J, Pira E. Brain magnetic resonance imaging and manganese concentrations in red blood cells of smelting workers: search for biomarkers of manganese exposure. Neurotoxicology. 2007 Jan;28(1):126-35. [PMC free article: PMC3983995] [PubMed: 16978697]
- 61.
- Robison G, Zakharova T, Fu S, Jiang W, Fulper R, Barrea R, Marcus MA, Zheng W, Pushkar Y. X-ray fluorescence imaging: a new tool for studying manganese neurotoxicity. PLoS One. 2012;7(11):e48899. [PMC free article: PMC3501493] [PubMed: 23185282]
- 62.
- Robison G, Zakharova T, Fu S, Jiang W, Fulper R, Barrea R, Zheng W, Pushkar Y. X-ray fluorescence imaging of the hippocampal formation after manganese exposure. Metallomics. 2013 Nov;5(11):1554-65. [PMC free article: PMC3892963] [PubMed: 23999853]
- 63.
- Morello M, Canini A, Mattioli P, Sorge RP, Alimonti A, Bocca B, Forte G, Martorana A, Bernardi G, Sancesario G. Sub-cellular localization of manganese in the basal ganglia of normal and manganese-treated rats An electron spectroscopy imaging and electron energy-loss spectroscopy study. Neurotoxicology. 2008 Jan;29(1):60-72. [PubMed: 17936361]
- 64.
- Klaassen CD. Biliary excretion of metals. Drug Metab Rev. 1976;5(2):165-96. [PubMed: 802467]
- 65.
- O'Neal SL, Hong L, Fu S, Jiang W, Jones A, Nie LH, Zheng W. Manganese accumulation in bone following chronic exposure in rats: steady-state concentration and half-life in bone. Toxicol Lett. 2014 Aug 17;229(1):93-100. [PMC free article: PMC4126163] [PubMed: 24930841]
- 66.
- Jankovic J. Searching for a relationship between manganese and welding and Parkinson's disease. Neurology. 2005 Jun 28;64(12):2021-8. [PubMed: 15985567]
- 67.
- Olanow CW. Manganese-induced parkinsonism and Parkinson's disease. Ann N Y Acad Sci. 2004 Mar;1012:209-23. [PubMed: 15105268]
- 68.
- Crossgrove J, Zheng W. Manganese toxicity upon overexposure. NMR Biomed. 2004 Dec;17(8):544-53. [PMC free article: PMC3980863] [PubMed: 15617053]
- 69.
- Bowler RM, Roels HA, Nakagawa S, Drezgic M, Diamond E, Park R, Koller W, Bowler RP, Mergler D, Bouchard M, Smith D, Gwiazda R, Doty RL. Dose-effect relationships between manganese exposure and neurological, neuropsychological and pulmonary function in confined space bridge welders. Occup Environ Med. 2007 Mar;64(3):167-77. [PMC free article: PMC2092523] [PubMed: 17018581]
- 70.
- Huang CC, Chu NS, Lu CS, Calne DB. Cock gait in manganese intoxication. Mov Disord. 1997 Sep;12(5):807-8. [PubMed: 9380071]
- 71.
- Charash B, Placek E, Sos TA, Kligfield P. Dose-related effects of manganese on the canine electrocardiogram. J Electrocardiol. 1982 Apr;15(2):149-52. [PubMed: 7069332]
- 72.
- Vander Elst L, Colet JM, Muller RN. Spectroscopic and metabolic effects of MnCl2 and MnDPDP on the isolated and perfused rat heart. Invest Radiol. 1997 Oct;32(10):581-8. [PubMed: 9342116]
- 73.
- Michalke B, Fernsebner K. New insights into manganese toxicity and speciation. J Trace Elem Med Biol. 2014 Apr;28(2):106-116. [PubMed: 24200516]
- 74.
- Angoa-Pérez M, Anneken JH, Kuhn DM. Neurotoxicology of Synthetic Cathinone Analogs. Curr Top Behav Neurosci. 2017;32:209-230. [PMC free article: PMC6100737] [PubMed: 27753008]
- 75.
- Squitti R, Gorgone G, Panetta V, Lucchini R, Bucossi S, Albini E, Alessio L, Alberici A, Melgari JM, Benussi L, Binetti G, Rossini PM, Draicchio F. Implications of metal exposure and liver function in Parkinsonian patients resident in the vicinities of ferroalloy plants. J Neural Transm (Vienna). 2009 Oct;116(10):1281-7. [PubMed: 19680597]
- 76.
- Lauwerys R, Roels H, Genet P, Toussaint G, Bouckaert A, De Cooman S. Fertility of male workers exposed to mercury vapor or to manganese dust: a questionnaire study. Am J Ind Med. 1985;7(2):171-6. [PubMed: 3976664]
- 77.
- Schroeder HA, Balassa JJ, Tipton IH. Essential trace metals in man: manganese. A study in homeostasis. J Chronic Dis. 1966 May;19(5):545-71. [PubMed: 5338081]
- 78.
- Wang D, Du X, Zheng W. Alteration of saliva and serum concentrations of manganese, copper, zinc, cadmium and lead among career welders. Toxicol Lett. 2008 Jan 04;176(1):40-7. [PMC free article: PMC3980858] [PubMed: 18054180]
- 79.
- Viana GF, de Carvalho CF, Nunes LS, Rodrigues JL, Ribeiro NS, de Almeida DA, Ferreira JR, Abreu N, Menezes-Filho JA. Noninvasive biomarkers of manganese exposure and neuropsychological effects in environmentally exposed adults in Brazil. Toxicol Lett. 2014 Dec 01;231(2):169-78. [PubMed: 24992226]
- 80.
- Cowan DM, Fan Q, Zou Y, Shi X, Chen J, Aschner M, Rosenthal FS, Zheng W. Manganese exposure among smelting workers: blood manganese-iron ratio as a novel tool for manganese exposure assessment. Biomarkers. 2009 Feb;14(1):3-16. [PMC free article: PMC3980868] [PubMed: 19283519]
- 81.
- Grashow R, Zhang J, Fang SC, Weisskopf MG, Christiani DC, Cavallari JM. Toenail metal concentration as a biomarker of occupational welding fume exposure. J Occup Environ Hyg. 2014;11(6):397-405. [PMC free article: PMC4019688] [PubMed: 24372360]
- 82.
- Ntihabose R, Surette C, Foucher D, Clarisse O, Bouchard MF. Assessment of saliva, hair and toenails as biomarkers of low level exposure to manganese from drinking water in children. Neurotoxicology. 2018 Jan;64:126-133. [PubMed: 28867366]
- 83.
- Chung SE, Cheong HK, Ha EH, Kim BN, Ha M, Kim Y, Hong YC, Park H, Oh SY. Maternal Blood Manganese and Early Neurodevelopment: The Mothers and Children's Environmental Health (MOCEH) Study. Environ Health Perspect. 2015 Jul;123(7):717-22. [PMC free article: PMC4492260] [PubMed: 25734517]
- 84.
- Dydak U, Jiang YM, Long LL, Zhu H, Chen J, Li WM, Edden RA, Hu S, Fu X, Long Z, Mo XA, Meier D, Harezlak J, Aschner M, Murdoch JB, Zheng W. In vivo measurement of brain GABA concentrations by magnetic resonance spectroscopy in smelters occupationally exposed to manganese. Environ Health Perspect. 2011 Feb;119(2):219-24. [PMC free article: PMC3040609] [PubMed: 20876035]
- 85.
- de Water E, Proal E, Wang V, Medina SM, Schnaas L, Téllez-Rojo MM, Wright RO, Tang CY, Horton MK. Prenatal manganese exposure and intrinsic functional connectivity of emotional brain areas in children. Neurotoxicology. 2018 Jan;64:85-93. [PMC free article: PMC5723568] [PubMed: 28610744]
- 86.
- Yang HJ, Park SH, Seo M, Weon YC, Kim Y. 18F-FP-CIT dopamine transporter PET findings in cirrhotic patients with parkinsonism. Neurotoxicology. 2018 Jan;64:78-84. [PubMed: 28259768]
- 87.
- Casjens S, Dydak U, Dharmadhikari S, Lotz A, Lehnert M, Quetscher C, Stewig C, Glaubitz B, Schmidt-Wilcke T, Edmondson D, Yeh CL, Weiss T, Thriel CV, Herrmann L, Muhlack S, Woitalla D, Aschner M, Brüning T, Pesch B. Association of exposure to manganese and iron with striatal and thalamic GABA and other neurometabolites - Neuroimaging results from the WELDOX II study. Neurotoxicology. 2018 Jan;64:60-67. [PMC free article: PMC5808902] [PubMed: 28803850]
- 88.
- Fenstermacher J, Kaye T. Drug "diffusion" within the brain. Ann N Y Acad Sci. 1988;531:29-39. [PubMed: 3382143]
- 89.
- Schlageter NL, Carson RE, Rapoport SI. Examination of blood-brain barrier permeability in dementia of the Alzheimer type with [68Ga]EDTA and positron emission tomography. J Cereb Blood Flow Metab. 1987 Feb;7(1):1-8. [PubMed: 3100543]
- 90.
- von Holst H, Ericson K, Edner G. Positron emission tomography with 68-Ga-EDTA and computed tomography in patients with subarachnoid haemorrhage. Acta Neurochir (Wien). 1989;97(3-4):146-9. [PubMed: 2497623]
- 91.
- Bleich S, Degner D, Sprung R, Riegel A, Poser W, Rüther E. Chronic manganism: fourteen years of follow-up. J Neuropsychiatry Clin Neurosci. 1999 Winter;11(1):117. [PubMed: 9990570]
- 92.
- Hong L, Jiang W, Zheng W, Zeng S. HPLC analysis of para-aminosalicylic acid and its metabolite in plasma, cerebrospinal fluid and brain tissues. J Pharm Biomed Anal. 2011 Apr 05;54(5):1101-9. [PMC free article: PMC3046028] [PubMed: 21159459]
- 93.
- Jiang YM, Mo XA, Du FQ, Fu X, Zhu XY, Gao HY, Xie JL, Liao FL, Pira E, Zheng W. Effective treatment of manganese-induced occupational Parkinsonism with p-aminosalicylic acid: a case of 17-year follow-up study. J Occup Environ Med. 2006 Jun;48(6):644-9. [PMC free article: PMC4180660] [PubMed: 16766929]
- 94.
- Asanuma M, Miyazaki I, Ogawa N. Neuroprotective effects of nonsteroidal anti-inflammatory drugs on neurodegenerative diseases. Curr Pharm Des. 2004;10(6):695-700. [PubMed: 14965332]
- 95.
- Rothstein JD, Patel S, Regan MR, Haenggeli C, Huang YH, Bergles DE, Jin L, Dykes Hoberg M, Vidensky S, Chung DS, Toan SV, Bruijn LI, Su ZZ, Gupta P, Fisher PB. Beta-lactam antibiotics offer neuroprotection by increasing glutamate transporter expression. Nature. 2005 Jan 06;433(7021):73-7. [PubMed: 15635412]
- 96.
- Huang CC, Lu CS, Chu NS, Hochberg F, Lilienfeld D, Olanow W, Calne DB. Progression after chronic manganese exposure. Neurology. 1993 Aug;43(8):1479-83. [PubMed: 8351000]
- 97.
- Huang CC, Chu NS, Lu CS, Chen RS, Calne DB. Long-term progression in chronic manganism: ten years of follow-up. Neurology. 1998 Mar;50(3):698-700. [PubMed: 9521259]
- 98.
- Stephenson AP, Schneider JA, Nelson BC, Atha DH, Jain A, Soliman KF, Aschner M, Mazzio E, Renee Reams R. Manganese-induced oxidative DNA damage in neuronal SH-SY5Y cells: attenuation of thymine base lesions by glutathione and N-acetylcysteine. Toxicol Lett. 2013 Apr 26;218(3):299-307. [PMC free article: PMC3617125] [PubMed: 23296100]
- 99.
- Peres TV, Schettinger MR, Chen P, Carvalho F, Avila DS, Bowman AB, Aschner M. "Manganese-induced neurotoxicity: a review of its behavioral consequences and neuroprotective strategies". BMC Pharmacol Toxicol. 2016 Nov 04;17(1):57. [PMC free article: PMC5097420] [PubMed: 27814772]
- 100.
- da Silva Santos V, Bisen-Hersh E, Yu Y, Cabral IS, Nardini V, Culbreth M, Teixeira da Rocha JB, Barbosa F, Aschner M. Anthocyanin-rich açaí (Euterpe oleracea Mart.) extract attenuates manganese-induced oxidative stress in rat primary astrocyte cultures. J Toxicol Environ Health A. 2014;77(7):390-404. [PubMed: 24617543]
- 101.
- Martins EN, Pessano NT, Leal L, Roos DH, Folmer V, Puntel GO, Rocha JB, Aschner M, Ávila DS, Puntel RL. Protective effect of Melissa officinalis aqueous extract against Mn-induced oxidative stress in chronically exposed mice. Brain Res Bull. 2012 Jan 04;87(1):74-9. [PubMed: 22020131]
- 102.
- Getachew B, Csoka AB, Aschner M, Tizabi Y. Nicotine protects against manganese and iron-induced toxicity in SH-SY5Y cells: Implication for Parkinson's disease. Neurochem Int. 2019 Mar;124:19-24. [PMC free article: PMC6369010] [PubMed: 30557592]
- 103.
- Ommati MM, Heidari R, Ghanbarinejad V, Abdoli N, Niknahad H. Taurine Treatment Provides Neuroprotection in a Mouse Model of Manganism. Biol Trace Elem Res. 2019 Aug;190(2):384-395. [PubMed: 30357569]
- 104.
- Ahmadi N, Ghanbarinejad V, Ommati MM, Jamshidzadeh A, Heidari R. Taurine prevents mitochondrial membrane permeabilization and swelling upon interaction with manganese: Implication in the treatment of cirrhosis-associated central nervous system complications. J Biochem Mol Toxicol. 2018 Nov;32(11):e22216. [PubMed: 30152904]
- 105.
- Lu CL, Tang S, Meng ZJ, He YY, Song LY, Liu YP, Ma N, Li XY, Guo SC. Taurine improves the spatial learning and memory ability impaired by sub-chronic manganese exposure. J Biomed Sci. 2014 May 24;21(1):51. [PMC free article: PMC4045917] [PubMed: 24885898]
- 106.
- Neely MD, Davison CA, Aschner M, Bowman AB. From the Cover: Manganese and Rotenone-Induced Oxidative Stress Signatures Differ in iPSC-Derived Human Dopamine Neurons. Toxicol Sci. 2017 Oct 01;159(2):366-379. [PMC free article: PMC5837701] [PubMed: 28962525]
- 107.
- Antony PM, Diederich NJ, Krüger R, Balling R. The hallmarks of Parkinson's disease. FEBS J. 2013 Dec;280(23):5981-93. [PubMed: 23663200]
- 108.
- Gomperts SN. Lewy Body Dementias: Dementia With Lewy Bodies and Parkinson Disease Dementia. Continuum (Minneap Minn). 2016 Apr;22(2 Dementia):435-63. [PMC free article: PMC5390937] [PubMed: 27042903]
- 109.
- Bang J, Spina S, Miller BL. Frontotemporal dementia. Lancet. 2015 Oct 24;386(10004):1672-82. [PMC free article: PMC5970949] [PubMed: 26595641]
- 110.
- Clark LN, Louis ED. Essential tremor. Handb Clin Neurol. 2018;147:229-239. [PMC free article: PMC5898616] [PubMed: 29325613]
- 111.
- Palma JA, Norcliffe-Kaufmann L, Kaufmann H. Diagnosis of multiple system atrophy. Auton Neurosci. 2018 May;211:15-25. [PMC free article: PMC5869112] [PubMed: 29111419]
- 112.
- Levin J, Kurz A, Arzberger T, Giese A, Höglinger GU. The Differential Diagnosis and Treatment of Atypical Parkinsonism. Dtsch Arztebl Int. 2016 Feb 05;113(5):61-9. [PMC free article: PMC4782269] [PubMed: 26900156]
- 113.
- Brigo F, Erro R, Marangi A, Bhatia K, Tinazzi M. Differentiating drug-induced parkinsonism from Parkinson's disease: an update on non-motor symptoms and investigations. Parkinsonism Relat Disord. 2014 Aug;20(8):808-14. [PubMed: 24935237]
- 114.
- Iqbal M, Monaghan T, Redmond J. Manganese toxicity with ephedrone abuse manifesting as parkinsonism: a case report. J Med Case Rep. 2012 Feb 07;6:52. [PMC free article: PMC3292443] [PubMed: 22313512]
- 115.
- Koh J, Ito H. Differential diagnosis of Parkinson's disease and other neurodegenerative disorders. Nihon Rinsho. 2017 Jan;75(1):56-62. [PubMed: 30566295]
- 116.
- Bouchard M, Mergler D, Baldwin M, Panisset M, Bowler R, Roels HA. Neurobehavioral functioning after cessation of manganese exposure: a follow-up after 14 years. Am J Ind Med. 2007 Nov;50(11):831-40. [PubMed: 17096374]
- 117.
- Hochberg F, Miller G, Valenzuela R, McNelis S, Crump KS, Covington T, Valdivia G, Hochberg B, Trustman JW. Late motor deficits of Chilean manganese miners: a blinded control study. Neurology. 1996 Sep;47(3):788-95. [PubMed: 8797481]
- 118.
- Pal PK, Samii A, Calne DB. Manganese neurotoxicity: a review of clinical features, imaging and pathology. Neurotoxicology. 1999 Apr-Jun;20(2-3):227-38. [PubMed: 10385886]
- 119.
- Bouchard M, Mergler D, Baldwin M. Manganese exposure and age: neurobehavioral performance among alloy production workers. Environ Toxicol Pharmacol. 2005 May;19(3):687-94. [PubMed: 21783543]
- 120.
- Bouchard M, Mergler D, Baldwin M, Panisset M, Roels HA. Neuropsychiatric symptoms and past manganese exposure in a ferro-alloy plant. Neurotoxicology. 2007 Mar;28(2):290-7. [PubMed: 16962176]
- 121.
- Roels HA, Ortega Eslava MI, Ceulemans E, Robert A, Lison D. Prospective study on the reversibility of neurobehavioral effects in workers exposed to manganese dioxide. Neurotoxicology. 1999 Apr-Jun;20(2-3):255-71. [PubMed: 10385889]
- 122.
- Lucchini R, Selis L, Folli D, Apostoli P, Mutti A, Vanoni O, Iregren A, Alessio L. Neurobehavioral effects of manganese in workers from a ferroalloy plant after temporary cessation of exposure. Scand J Work Environ Health. 1995 Apr;21(2):143-9. [PubMed: 7618060]
- 123.
- Thompson KJ, Hein J, Baez A, Sosa JC, Wessling-Resnick M. Manganese transport and toxicity in polarized WIF-B hepatocytes. Am J Physiol Gastrointest Liver Physiol. 2018 Sep 01;315(3):G351-G363. [PMC free article: PMC6335010] [PubMed: 29792530]
Disclosure: Garrett Evans declares no relevant financial relationships with ineligible companies.
Disclosure: Lawrence Masullo declares no relevant financial relationships with ineligible companies.
- Review Manganese and aging.[Neurotoxicology. 2016]Review Manganese and aging.Parmalee NL, Aschner M. Neurotoxicology. 2016 Sep; 56:262-268. Epub 2016 Jun 10.
- Dietary glycation compounds - implications for human health.[Crit Rev Toxicol. 2024]Dietary glycation compounds - implications for human health.Hellwig M, Diel P, Eisenbrand G, Grune T, Guth S, Henle T, Humpf HU, Joost HG, Marko D, Raupbach J, et al. Crit Rev Toxicol. 2024 Sep; 54(8):485-617. Epub 2024 Aug 16.
- Review Is electric arc welding linked to manganism or Parkinson's disease?[Toxicol Rev. 2005]Review Is electric arc welding linked to manganism or Parkinson's disease?McMillan G. Toxicol Rev. 2005; 24(4):237-57.
- Pathophysiology of manganese-associated neurotoxicity.[Neurotoxicology. 2012]Pathophysiology of manganese-associated neurotoxicity.Racette BA, Aschner M, Guilarte TR, Dydak U, Criswell SR, Zheng W. Neurotoxicology. 2012 Aug; 33(4):881-6. Epub 2011 Dec 21.
- Review "Manganese-induced neurotoxicity: a review of its behavioral consequences and neuroprotective strategies".[BMC Pharmacol Toxicol. 2016]Review "Manganese-induced neurotoxicity: a review of its behavioral consequences and neuroprotective strategies".Peres TV, Schettinger MR, Chen P, Carvalho F, Avila DS, Bowman AB, Aschner M. BMC Pharmacol Toxicol. 2016 Nov 4; 17(1):57. Epub 2016 Nov 4.
- Manganese Toxicity - StatPearlsManganese Toxicity - StatPearls
Your browsing activity is empty.
Activity recording is turned off.
See more...